- 1Solar Science Observatory, National Astronomical Observatory of Japan, Mitaka, Japan
- 2Solar Eclipse Digital Imaging and Processing Network, Tsukuba, Japan
- 3Chiba Prefectural Ichihara High School, Ichihara, Japan
This study measured the polarization of the white-light corona during the total solar eclipse on 20 April 2023, which occurred under high solar activity. The same instrument that was used for the 2017 and 2019 eclipse observations was employed, and despite the short duration of totality, the obtained data could be used for high-accuracy polarization analysis. We derived the brightness and polarization of the K + F corona and estimated the brightness distributions of the K- and F-coronae using polarization information. The polarization data of the corona are the key to estimating the amount of coronal hot plasma and its electron density distribution. Therefore, we examined the consistency between the eclipse data and those taken by the C2 coronagraph of the Large Angle Spectrometric Coronagraph (LASCO) on board the Solar and Heliospheric Observatory. Consequently, a systematic difference was observed; the polarization measured by LASCO-C2 was approximately 30% smaller than the results from the eclipse. Data from eclipses, which are captured under low background sky brightness and no scattered light due to the Sun’s disk, can be a good calibration source of the brightness and polarization of the white-light corona.
1 Introduction
During a total solar eclipse, the solar white-light corona can be observed from immediately above the limb to several solar radii under a low sky-background level. X-ray and EUV observations of the corona, which are conducted by spacecraft every day, show coronal plasma at specific temperatures. In contrast, white-light observations map the density structure of the corona irrespective of the temperature. White-light corona is also regularly observed by coronagraphs. However, the spaceborne coronagraphs, such as the Large Angle Spectrometric Coronagraph (LASCO) (Brueckner et al., 1995) of the Solar and Heliospheric Observatory (SOHO), cannot observe the inner corona (typically
The white-light corona primarily comprises the K- and F-coronae, which represent the hot plasma produced by the Sun and interplanetary dust, respectively. The electron density distribution of coronal hot plasma, which is an important piece of fundamental information about the corona, can be derived from the brightness distribution of the K-corona, as proposed by van de Hulst (1950). Therefore, to study the distribution of coronal hot plasma, the K- and F-coronae should be quantitatively separated. This can be done using polarization imaging data of the white-light corona because the K-corona, wherein radiation is produced by Thomson scattering, exhibits polarization, whereas the F-corona does not exhibit polarization up to several solar radii (see Lamy et al., 2021, references therein). Therefore, coronagraphs for white-light observation, both ground-based and spaceborne, can measure polarization.
Polarimetry has also been performed during total solar eclipses. Recently digital instruments have been used for polarimetry. Capobianco et al. (2012) observed the 2006 eclipse using an ‘E-Kpol’ polarimeter and Skomorovsky et al. (2012) observed the 2008 eclipse using a triple-polarized-image telescope. In particular, during the total eclipse of 2017, wherein the path of totality passed the mainland of the United States of America, many observers challenged polarization measurements, and certain successful results were reported (Judge et al., 2019; Vorobiev et al., 2020; Bemporad, 2020; Liang et al., 2023). Similar observations were made during the subsequent eclipses (Liberatore et al., 2023 for the 2019 eclipse; Edwards et al., 2023 for the 2020 eclipse). We also succeeded in conducting polarimetry at multiple sites during the 2017 and 2019 eclipses (Hanaoka et al., 2021).
The derivation of the electron density distribution of the corona has been attempted in certain of the above eclipse studies as well as using spaceborne coronagraphs (e.g., Hayes et al., 2001; Quémerais and Lamy, 2002). To derive the correct electron densities, well-calibrated brightness data for the K-corona are required. Fortunately, the accuracy of polarimetry has significantly improved in recent digital observations. For example, the above-mentioned results for the 2017 eclipse show good agreement; in particular, the consistency between the results by Vorobiev et al. (2020) and those by Hanaoka et al. (2021) is remarkable (Figure 7 in Hanaoka et al., 2021).
However, a systematic discrepancy between the polarimetry results was observed for the data obtained during the 2017 and 2019 eclipses and those captured with LASCO-C2 (one of the coronagraphs comprising LASCO covering up to 6.5
We observed a total solar eclipse in Australia in 2023 to obtain polarimetry data. We used the same instrument that was used for the 2017 and 2019 eclipses. Although the duration of totality of the 2023 eclipse was less than 1 min, we successfully obtained data from which we could derive high-accuracy polarization signals. This paper presents the results of the polarimetry performed during the 2023 eclipse.
This study is a repetition of the analyses performed for the 2017 and 2019 eclipses. However, not only is it a wide field-of-view record of the corona during the 2023 eclipse, but it also has other significant advantages.
1. Eclipse polarimetry data are often calibrated using the polarization brightness measured by K-Cor of MLSO. However, during the 2023 eclipse, K-Cor was not working, and in such cases, reference data other than the K-Cor data are required. The COR1 coronagraph of Solar Terrestrial Relations Observatory/Sun Earth Connection Coronal and Heliospheric Investigation (Howard et al., 2008), which was located near the Earth during the 2023 eclipse, measured the brightness and polarization of the corona during the eclipse. However, the COR1 data show considerable errors particularly in the inter-streamer low light-level regions, as indicated by Frazin et al. (2012). Therefore, the accuracy of the calibration using the COR1 data is presumed to be limited. We can calibrate the data using our solar disk images captured along with eclipse images.
2. In contrast to the 2017 and 2019 eclipses, which occurred in the declining phase and near the minimum solar activity, the 2023 eclipse occurred during high solar activity. As previously stated, a discrepancy between the polarimetry results was observed in the 2017 and 2019 eclipses. We can check whether the same tendency is observed in the results obtained under high solar activity.
The remainder of this paper is organized as follows. The observations of the 2023 eclipse and reduction for the obtained data are described in Section 2. The measurement results of the brightness and polarization of the K + F corona and the respective brightness distributions of the K- and F-coronae estimated from the polarimetry data are presented in Section 3. Section 4 is devoted to a summary and discussion including a comparison between the eclipse data and LASCO-C2 measurements for the 2023 eclipse as well as the 2017 and 2019 eclipses.
2 Observations and data reduction
The observation and reduction methods were similar to those employed in the study of the 2017 and 2019 eclipses described by Hanaoka et al. (2021). Therefore, we briefly present them here.
2.1 Observations
The observations were conducted under professional-amateur collaborations for eclipse observations, which produced scientific results for former eclipses (Hanaoka et al., 2012; 2014; 2018; 2021). We conducted the observations of the 2023 eclipse on 20 April 2023, at Exmouth of Western Australia, Australia (21.93S, 114.13E) in clear weather. The maximum eclipse occurred at 03:30:17 UT at an altitude of
The polarimetry instrument comprised a 60-mm refractor (Takahashi FS-60Q; focal length = 600 mm), a filter turret (fabricated by Koheisha, Kawagoe, Japan), and a digital single-lens reflex camera (DSLR; Canon EOS6D). The filter turret was equipped with three linear polarizers with transmission axis orientations of
The instruments had a wide field-of-view covering 4
In addition to the coronal images acquired during totality, images of the solar disk before the first contact and after the fourth contact of the eclipse and images of the partially eclipsed Sun were also taken using additional neutral density filters. Solar disk images were used to calibrate the brightness of the corona with respect to the mean brightness of the disk. As stated above, such calibration data are required for the 2023 eclipse, when the K-Cor data are not available. We performed the calibrations using the solar disk images for the data taken during the former eclipses, even when the K-Cor data were available, because the calibration could be performed free from the possible error of the K-Cor data caused by the bright background sky.
2.2 Data reduction
Images captured with each of the polarizers and the ND filter were stacked to produce a single, wide dynamic-range, low-noise map. Each map was produced from a set of ten images (five different exposure times
Furthermore, before the image stacking, certain preparations were required as described below.
1. Derivation of the positional shifts of the Sun in images. The position of the Sun’s disk center on the raw images fluctuated due to tracking errors and wind effects. Because the solar disk is hidden by the Moon, the shifts in the images were derived by referencing fine coronal structures.
2. Conversion from the data number of raw images to those showing the amount of incident light (brightness of the corona) per second. Basically, the amount of incident light and analog-to-digital converted data number (dark level removed) show a linear relationship. However, certain cameras, including the one used for our polarimetry observation, show a non-linear response. This non-linearity can be examined comparing two images of the solar corona captured at different exposure times. We derived a non-linear function and corrected the raw data numbers of the images. In addition, we estimated the true ratio of the exposure times. The exposure times were 1/2, 1/8, ..., 1/512 s, and the ratio of the adjacent exposure times was nominally 4. However, systematic errors may occur because the shutter is a mechanical device. A comparison of two images with different exposure times (non-linearity corrected) also provides the true ratio of the exposure times. With the corrected exposure times, the data numbers were converted into numbers per second.
3. Images captured at various exposure times have respective brightness ranges that were properly exposed because the dynamic range of the coronal brightness extends over several orders of magnitude. Before stacking the images, underexposed and overexposed parts were discarded.
At this stage, the corrections of the non-linearity and exposure-time errors were applied to the flat-field data, and all the raw images were corrected with the new flat-field. With these newly corrected images, the non-linearity and exposure-time errors were again examined. Following the above preparations, we stacked the images and obtained wide dynamic-range maps for the three polarizers and ND filter.
Certain stars can be found in the stacked maps, and their positions can be found in the Hipparcos catalog (http://archive.eso.org/skycat/servers/ASTROM). Using these stars as a reference, we derived the position of the center of the solar disk, the direction of the solar north, and the pixel scale (
To measure the brightness of the solar disk, which is the reference for coronal brightness, we calculated the data number of the disk image without the additional ND filters used to capture the disk images. The effective transmissions of the ND filters were calculated by combining the spectral transmissions of the ND filters, solar spectrum, and spectral response curve of the camera. We checked the variation in the transparency of the atmosphere from the start to the end of the eclipse using the full-disk images and the partial eclipse images, and estimated the disk brightness during totality, which was used as the reference for brightness.
By combining the stacked maps for the three polarizer positions, we obtained the Stokes
The camera used for polarimetry was a commercial DSLR camera, which can capture images of three (red, green, and blue, or R, G, and B) color channels, with central wavelengths of approximately 600, 530, and 470 nm, respectively. Image stacking and brightness calibration were independently performed for each channel, and we obtained brightness and polarization distribution data for the R, G, and B channels.
Ordinary white-light imaging data of the corona obtained with the other telescopes and cameras (not equipped with polarizers) were processed in a similar manner and used to check the consistency of the brightness calibration.
3 Results
The distributions of the derived polarization signals are shown in Figure 1. Figure 1A shows the raw polarization signals (orange ticks) plotted on a coronal image (gray scale). The raw polarization signals include the sky background, which shows the polarization approximately along the zenith-horizon direction (the zenith direction is indicated with arrows in the Figure). The polarization signals distant from the Sun in Figure 1A are dominated by the sky component.
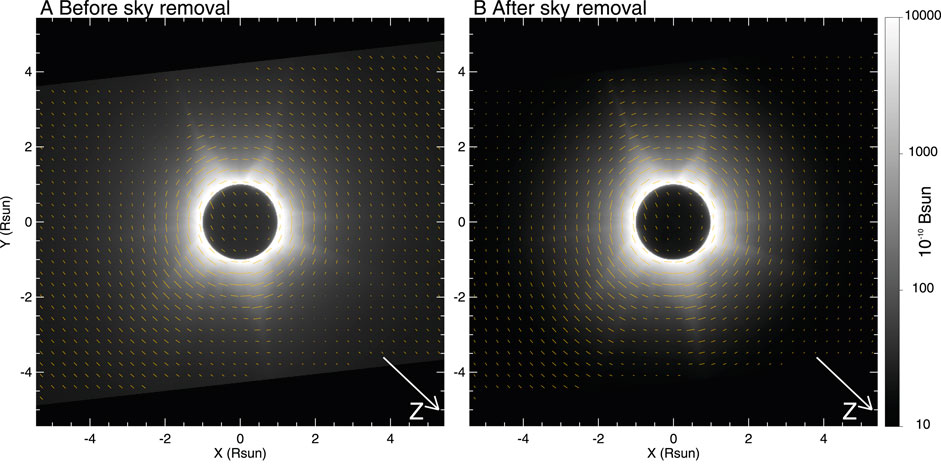
Figure 1. White-light polarization maps of the G-channel covering
The brightness and polarization of the sky were estimated as follows. The linear polarization signals of the corona are tangential to the Sun. Therefore, after the removal of the appropriate polarization of the sky (assumed to be constant in the field of view), only the tangential polarization signals remain. In the outermost area in the field of view, the K-corona is very weak, and therefore, the observed brightness comprises the components of the sky and the F-corona. On the assumption that the degree of polarization of the sky does not depend on the wavelength and the spectral distribution of the F-corona is
Figure 1B shows a map of the K + F corona after removing thus derived sky background. Tangentially aligned linear polarization signals produced by the K-corona were observed. The brightness of the corona presented in Figures 1A,B is expressed in units of the mean brightness of the solar disk (
Figure 2 presents an enlarged view of polarization parameters of part of the inner K + F corona, demonstrating the quality of the polarization measurements. Figure 2A shows the brightness distribution of the K + F corona and Figure 2B shows the distribution of the degree of polarization. Figure 2A shows some high-lying prominences denoted by arrows. Unlike the low-lying saturated prominences, the polarization signals of the high-lying prominences were measured correctly. Figure 2B shows that the degree of polarization of these prominences (also denoted by arrows) was lower than that of the surrounding corona. This is because the prominences emit unpolarized free-bound and free-free radiation in addition to Thomson-scattering light (Jejčič et al., 2018). Figure 2B does not show spurious signals around the prominences caused by insufficient alignment corrections.
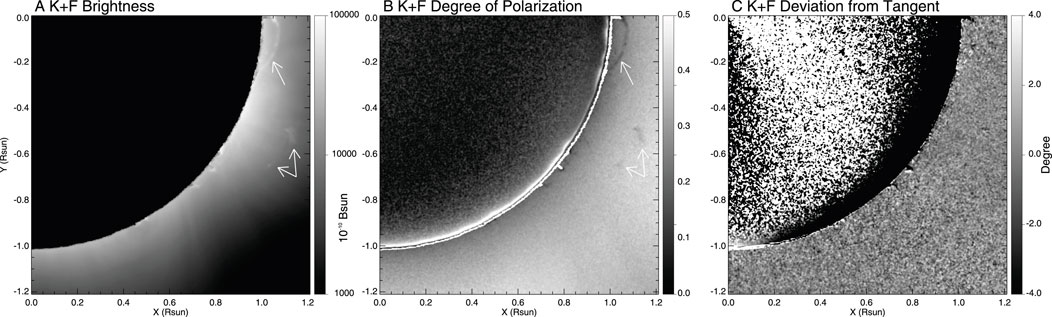
Figure 2. Maps showing polarization parameters in the southwest quadrant of the inner K + F corona. Panels (A), (B), and (C) show the brightness distribution, distribution of the degree of polarization, and deviation in the orientation of the linear polarization signals from the tangential direction, respectively. There are certain high-lying prominences denoted by arrows in (A) and (B).
Figure 2C shows the deviation in the orientation of the linear polarization signals from the tangential direction. Pixel-wise random errors were found in the corona; however, no systematic errors, which are caused by errors in polarization calibration and/or brightness and polarization estimation of the sky, were observed. In fact, the average unsigned error of the orientation angle of the linear polarization signals within 1.2
Figure 3 shows the brightness (
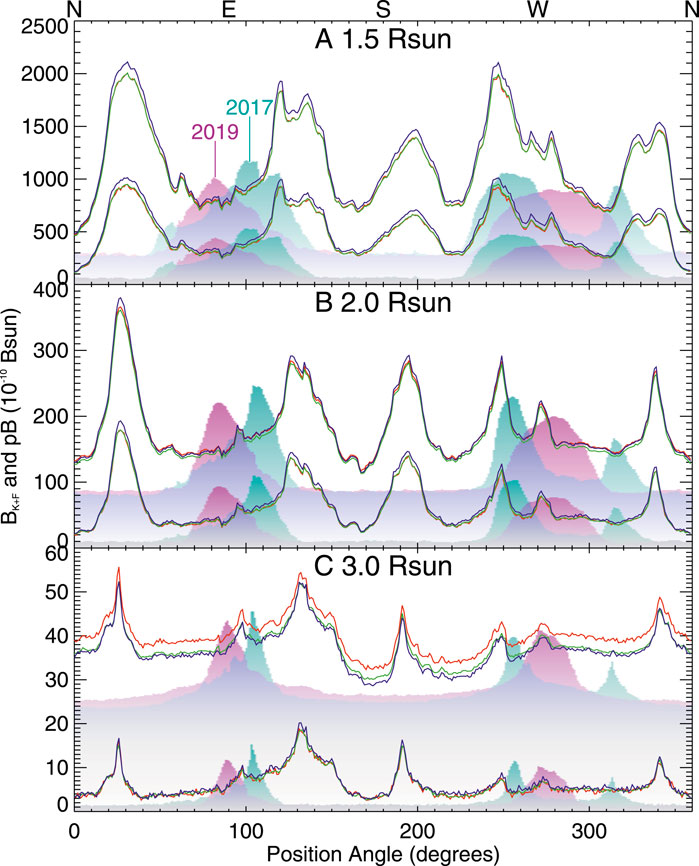
Figure 3. Position angle distributions of the total brightness of the K + F corona (
As previously stated, the polarization signals originate only from the K-corona. The polarization parameters shown in Figures 1B, 2, 3 are the sum of the K- and F-coronae; however, we can discriminate them using the polarization brightness
Figure 4 shows the approximate distributions of the K- and F- coronae derived based on thus estimated
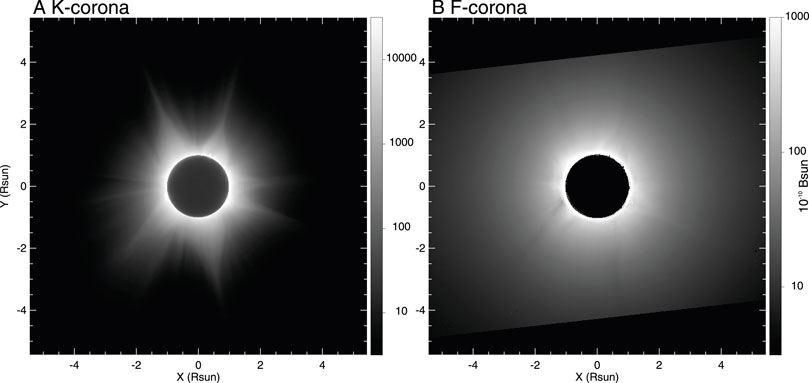
Figure 4. Gray-scale maps of (A) the K-corona and (B) the F-corona for the area shown in Figure 1.
4 Summary and discussion
We successfully obtained well-calibrated total brightness
Our eclipse data covering a wide field-of-view can be compared with the data of the outer white-light corona, such as those obtained by LASCO-C2 of SOHO, which covers the corona in the range of 2.5–6.5
The 2017 and 2019 eclipses occurred under low solar activity. We then compared the results of the 2023 eclipse, which occurred under high solar activity, with those of LASCO-C2. LASCO-C2 data were obtained from the “LASCO-C2 Legacy Archive” (http://idoc-lasco.ias.u-psud.fr/sitools/client-portal/doc/; refer to Lamy et al., 2020). Figure 5 shows the radial distribution of the brightness (
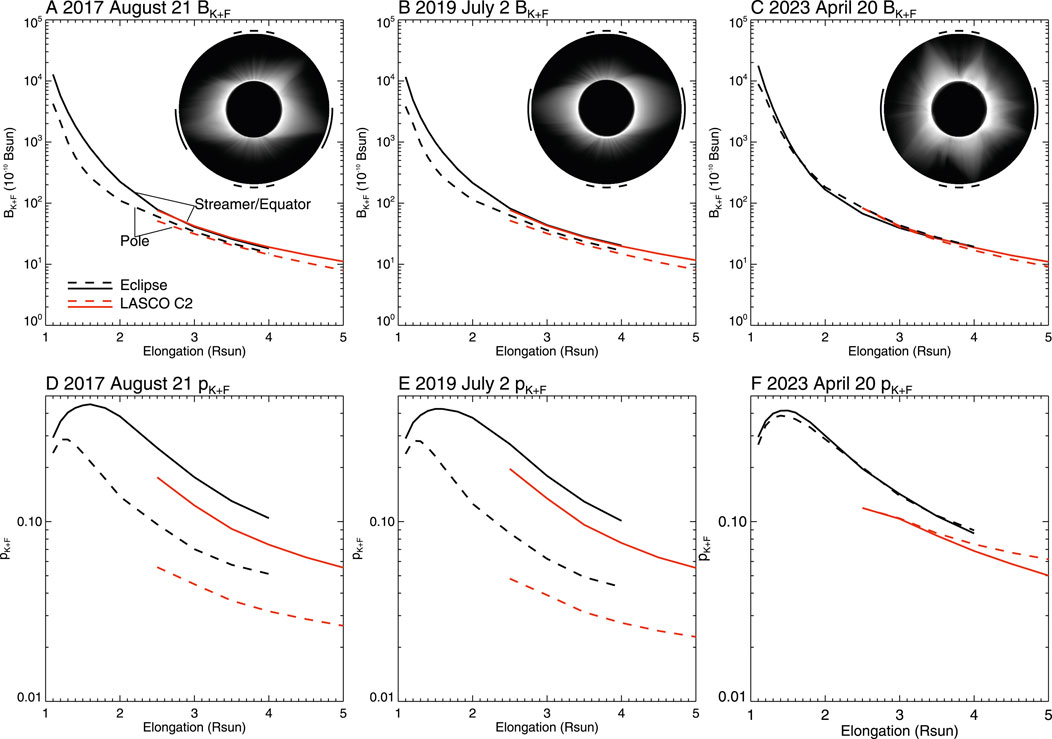
Figure 5. Panels (A–C) present the comparison of the radial distribution of the brightness of the K + F corona
The results in Figure 5 indicate that there is a discrepancy in the polarization brightness,
These results highlight the importance of eclipse observations in the present day. Total solar eclipses provide a well-calibrated brightness distribution of the corona over a wide height range under very small disturbances, and such data are difficult to acquire using other methods. The eclipse data can also be used as a good calibration source for the brightness and polarization of the white-light corona, not only for existing coronagraphs, but also for future missions.
To render the data useable for intercomparison among the observations to further check consistency, we have presented our data of the 2023 eclipse along with those of the 2017 and 2019 eclipses at https://solarwww.mtk.nao.ac.jp/mitaka_solar/solar_eclipse/polarization/.
Data availability statement
The datasets presented in this study can be found in online repositories. The names of the repository/repositories and accession number(s) can be found below: http://archive.eso.org/skycat/servers/ASTROM (the Hipparcos catalog), http://idoc-lasco.ias.u-psud.fr/sitools/client-portal/doc/ (the LASCO-C2 Legacy Archive). The datasets generated for this study can be found in: https://solarwww.mtk.nao.ac.jp/mitaka_solar/solar_eclipse/polarization/ (Solar Science Observatory, National Astronomical Observatory of Japan).
Author contributions
YH: Conceptualization, Formal Analysis, Methodology, Writing–original draft, Writing–review and editing. YS: Investigation, Writing–review and editing. YM: Investigation, Writing–review and editing.
Funding
The author(s) declare that no financial support was received for the research, authorship, and/or publication of this article.
Acknowledgments
Mr. Shin-ichiro Mishima also joined the professional-amateur collaborative observations and succeeded to take coronal images and provided us the data. This work makes use of the LASCO-C2 legacy archive data produced by the LASCO-C2 team at the Laboratoire d’Astrophysique de Marseille and the Laboratoire Atmosphères, Milieux, Observations Spatiales, both funded by the Centre National d’Etudes Spatiales (CNES). LASCO was built by a consortium of the Naval Research Laboratory, United States, the Laboratoire d’Astrophysique de Marseille (formerly Laboratoire d’Astronomie Spatiale), France, the Max-Planck-Institut für Sonnensystemforschung (formerly Max Planck Institute für Aeronomie), Germany, and the School of Physics and Astronomy, University of Birmingham, United Kingdom SOHO is a project of international cooperation between ESA and NASA.
Conflict of interest
The authors declare that the research was conducted in the absence of any commercial or financial relationships that could be construed as a potential conflict of interest.
Publisher’s note
All claims expressed in this article are solely those of the authors and do not necessarily represent those of their affiliated organizations, or those of the publisher, the editors and the reviewers. Any product that may be evaluated in this article, or claim that may be made by its manufacturer, is not guaranteed or endorsed by the publisher.
References
Bemporad, A. (2020). Coronal electron densities derived with images acquired during the 2017 august 21 total solar eclipse. Astrophys. J. 904, 178. doi:10.3847/1538-4357/abc482
Boe, B., Habbal, S., Downs, C., and Druckmüller, M. (2021). The color and brightness of the F-corona inferred from the 2019 july 2 total solar eclipse. Astrophys. J. 912, 44. doi:10.3847/1538-4357/abea79
Brueckner, G. E., Howard, R. A., Koomen, M. J., Korendyke, C. M., Michels, D. J., Moses, J. D., et al. (1995). The large angle spectroscopic coronagraph (LASCO). Sol. Phys. 162, 357–402. doi:10.1007/BF00733434
Burtovoi, A., Naletto, G., Dolei, S., Spadaro, D., Romoli, M., Landini, F., et al. (2022). Measuring the F-corona intensity through time correlation of total and polarized visible light images. Astron. Astrophys. 659, A50. doi:10.1051/0004-6361/202141414
Capobianco, G., Fineschi, S., Massone, G., Balboni, E., Malvezzi, A. M., Crescenzio, G., et al. (2012). Electro-optical polarimeters for ground-based and space-based observations of the solar K-corona. Mod. Technol. Space- Ground-based Telesc. Instrum. II 8450, 845040. doi:10.1117/12.926896
Edwards, L., Bunting, K. A., Ramsey, B., Gunn, M., Fearn, T., Knight, T., et al. (2023). Derived electron densities from linear polarization observations of the visible-light corona during the 14 december 2020 total solar eclipse. Sol. Phys. 298, 140. doi:10.1007/s11207-023-02231-5
Frazin, R. A., Vásquez, A. M., Thompson, W. T., Hewett, R. J., Lamy, P., Llebaria, A., et al. (2012). Intercomparison of the LASCO-C2, SECCHI-COR1, SECCHI-COR2, and Mk4 coronagraphs. Sol. Phys. 280, 273–293. doi:10.1007/s11207-012-0028-3
Hanaoka, Y., Hasuo, R., Hirose, T., Ikeda, A. C., Ishibashi, T., Manago, N., et al. (2018). Solar coronal jets extending to high altitudes observed during the 2017 august 21 total eclipse. Astrophys. J. 860, 142. doi:10.3847/1538-4357/aac49b
Hanaoka, Y., Kikuta, Y., Nakazawa, J., Ohnishi, K., and Shiota, K. (2012). Accurate measurements of the brightness of the white-light corona at the total solar eclipses on 1 august 2008 and 22 july 2009. Sol. Phys. 279, 75–89. doi:10.1007/s11207-012-9984-x
Hanaoka, Y., Nakazawa, J., Ohgoe, O., Sakai, Y., and Shiota, K. (2014). Coronal mass ejections observed at the total solar eclipse on 13 november 2012. Sol. Phys. 289, 2587–2599. doi:10.1007/s11207-014-0476-z
Hanaoka, Y., Sakai, Y., and Takahashi, K. (2021). Polarization of the corona observed during the 2017 and 2019 total solar eclipses. Sol. Phys. 296, 158. doi:10.1007/s11207-021-01907-0
Hayes, A. P., Vourlidas, A., and Howard, R. A. (2001). Deriving the electron density of the solar corona from the inversion of total brightness measurements. Astrophys. J. 548, 1081–1086. doi:10.1086/319029
Hou, J., de Wijn, A. G., and Tomczyk, S. (2013). Design and measurement of the Stokes polarimeter for the COSMO K-coronagraph. Astrophys. J. 774, 85. doi:10.1088/0004-637X/774/1/85
Howard, R. A., Moses, J. D., Vourlidas, A., Newmark, J. S., Socker, D. G., Plunkett, S. P., et al. (2008). Sun Earth connection coronal and heliospheric investigation (SECCHI). Space Sci. Rev. 136, 67–115. doi:10.1007/s11214-008-9341-4
Jejčič, S., Kleint, L., and Heinzel, P. (2018). High-density off-limb flare loops observed by SDO. Astrophys. J. 867, 134. doi:10.3847/1538-4357/aae650
Judge, P., Berkey, B., Boll, A., Bryans, P., Burkepile, J., Cheimets, P., et al. (2019). Solar eclipse observations from the ground and air from 0.31 to 5.5 microns. Sol. Phys. 294, 166. doi:10.1007/s11207-019-1550-3
Koutchmy, S., and Lamy, P. L. (1985). “The F-corona and the circum-solar dust evidences and properties [G. Nikolsky memorial lecture],” in The F-corona and the circum-solar dust evidences and properties (ir), 63, 63–74. doi:10.1007/978-94-009-5464-9_14
Lamy, P., Gilardy, H., Llebaria, A., Quémerais, E., and Ernandez, F. (2021). LASCO-C3 observations of the K- and F-coronae over 24 Years (1996 - 2019): photopolarimetry and electron density distribution. Sol. Phys. 296, 76. doi:10.1007/s11207-021-01819-z
Lamy, P., Llebaria, A., Boclet, B., Gilardy, H., Burtin, M., and Floyd, O. (2020). Coronal photopolarimetry with the LASCO-C2 coronagraph over 24 Years [1996 - 2019]. Sol. Phys. 295, 89. doi:10.1007/s11207-020-01650-y
Liang, Y., Qu, Z., Hao, L., Xu, Z., and Zhong, Y. (2023). Imaging-polarimetric properties of the white-light inner corona during the 2017 total solar eclipse. Mon. Not. R. Astron. Soc. 518, 1776–1788. doi:10.1093/mnras/stac3183
Liberatore, A., Zender, J., Capobianco, G., Fineschi, S., Panasenco, O., Tomuta, D., et al. (2023). Polarimetric study of the solar corona during the total solar eclipse on july 2, 2019 with a liquid-crystal polarimeter. Sol. Phys. 298, 85. doi:10.1007/s11207-023-02175-w
Quémerais, E., and Lamy, P. (2002). Two-dimensional electron density in the solar corona from inversion of white light images - application to SOHO/LASCO-C2 observations. Astron. Astrophys. 393, 295–304. doi:10.1051/0004-6361:20021019
Saito, K., Poland, A. I., and Munro, R. H. (1977). A study of the background corona near solar minimum. Sol. Phys. 55, 121–134. doi:10.1007/BF00150879
Skomorovsky, V. I., Trifonov, V. D., Mashnich, G. P., Zagaynova, Y. S., Fainshtein, V. G., Kushtal, G. I., et al. (2012). White-light observations and polarimetric analysis of the solar corona during the eclipse of 1 august 2008. Sol. Phys. 277, 267–281. doi:10.1007/s11207-011-9910-7
van de Hulst, H. C. (1950). The electron density of the solar corona. Bull. Astron. Inst. Neth. 11, 135.
Keywords: sun, solar corona, while-light observation, polarimetry, total solar eclipses
Citation: Hanaoka Y, Sakai Y and Masuda Y (2024) High-accuracy polarization measurements of the white-light corona during the 2023 total solar eclipse. Front. Astron. Space Sci. 11:1458746. doi: 10.3389/fspas.2024.1458746
Received: 03 July 2024; Accepted: 10 October 2024;
Published: 24 October 2024.
Edited by:
Ritesh Patel, Southwest Research Institute Boulder, United StatesReviewed by:
Vaibhav Pant, Aryabhatta Research Institute of Observational Sciences, IndiaJoan Burkepile, National Center for Atmospheric Research (UCAR), United States
Satabdwa Majumdar, Austrian Space Weather Office, Austria
Copyright © 2024 Hanaoka, Sakai and Masuda. This is an open-access article distributed under the terms of the Creative Commons Attribution License (CC BY). The use, distribution or reproduction in other forums is permitted, provided the original author(s) and the copyright owner(s) are credited and that the original publication in this journal is cited, in accordance with accepted academic practice. No use, distribution or reproduction is permitted which does not comply with these terms.
*Correspondence: Yoichiro Hanaoka, eW9pY2hpcm8uaGFuYW9rYUBuYW8uYWMuanA=