- 1Xinjiang Astronomical Observatory, Chinese Academy of Sciences, Urumqi, China
- 2University of Chinese Academy of Sciences, Beijing, China
- 3Xinjiang Key Laboratory of Radio Astrophysics, Urumqi, China
- 4Beijing National Laboratory for Molecular Sciences (BNLMS), Institute of Chemistry, Chinese Academy of Sciences, Beijing, China
In carbon-rich environment, C2H emission is seen to be associated with HC3N, but whether it can trace the HC3N molecule in oxygen-rich environment is unknown. Here, we have searched for rotational emission from ethynyl radical (C2H) in the oxygen-rich circumstellar envelope (CSE) of the post-asymptotic giant branch (post-AGB) star OH 231.8+4.2, a renowned galactic proto-planetary nebula (PPN) known to display cyanoacetylene (HC3N) emission. Our observations were conducted using the James Clerk Maxwell Telescope (JCMT), and the total on-source time is 11.9 h. Base on local thermodynamic equilibrium (LTE) excitation analysis, we have calculated the column density and the abundance relative to H2 for C2H. The calculated column density for C2H is less than 1.4
1 Introduction
The post-asymptotic giant branch (post-AGB) star, or proto-planetary nebula (PPN), OH 231.8+4.2, has been extensively studied on account of its unusual chemistry (Sánchez Contreras et al., 1997). This complex object exhibits a massive bipolar outflow and a rotating disk (Sánchez Contreras et al., 2022). The central star, named QX Pup, is accompanied by a binary companion star of type A0 V, indirectly identified through the analysis of the stellar spectrum reflected by the nebular dust (Sánchez Contreras et al., 2004). OH 231.8+4.2 features an oxygen-rich (O-rich) circumstellar envelope (CSE) surrounding an evolved intermediate-mass star. In addition to typical oxygen-containing species, a variety of detected ions including HCO+, H13CO+, SO+, N2H+, and H3O+ have been observed (Sánchez Contreras et al., 2015). Furthermore, chloride species like NaCl and KCl, as well as carbon-containing species such as HCN and HNC, were identified (Sánchez Contreras et al., 1997; Sánchez Contreras et al., 2022). CSEs are categorized based on their carbon to oxygen abundance ratio ([C]/[O]). If the [C]/[O] > 1, they are considered carbon-rich AGB stars (C-rich), while if it is <1, they are classified as O-rich AGB stars (objects with [C]/[O] ∼ 1 are known as S-type AGB stars). The chemical composition of CSEs is greatly influenced by the relative abundances of carbon and oxygen (Velilla Prieto et al., 2015). In O-rich CSEs, carbon often acts as the limiting reactant and is primarily converted into carbon monoxide (CO), a highly abundant and stable molecule. The remaining oxygen can then combine with other elements to form additional oxygen-bearing molecules. Consequently, O-rich envelopes typically exhibit lower abundances of carbon-bearing molecules aside from CO, whereas C-rich CSEs tend to show limited amounts of oxygen-bearing species (Bujarrabal et al., 1994).
However, Velilla Prieto et al. (2015) detected HC3N molecule for the first time in OH 231.8+4.2, which also represents the first observation of HC3N in an oxygen-rich CSE. For linear carbon chain molecules, Agúndez et al. (2017) investigated their formation in the C-rich AGB star IRC+10216 utilizing a combined approach of observations and modeling. Their study suggests that the dissociation of acetylene (C2H2) and hydrogen cyanide (HCN) induced by ultraviolet photons can drive the production of steadily increasing length of linear carbon chains. In the outer regions of stars, C2H2 and HCN are photodissociated, producing radicals C2H and CN, which can drive the formation of carbon chain molecules (Huggins and Glassgold, 1982; Cherchneff et al., 1993; Li et al., 2014; Agúndez et al., 2017). In carbon-rich environments, C2H is often used to “trace” linear carbon chain molecules. Therefore, to explore the carbon chemistry mechanism in OH 231.8+4.2, We reached for C2H emission using the James Clerk Maxwell Telescope (JCMT).
In this paper, we report the upper limits of the column density of C2H and the abundance of C2H relative to H2 in the post-AGB star OH 231.8+4.2.
2 Observations and data reduction
The observations were conducted using the JCMT 15 m at Maunakea, Hawaii, from 2021 to 2023. This work used about 11.9 h (on-source) of integration on project E21AZ002 and M23BP059. We used the Namakanui instrument within 230 GHz `Ū`ū receiver. The central frequency we observed was 262.0042266 GHz. The observations were carried out in beam-switch mode and performed towards the center of OH 231.8+4.2 (RA (2000) = 07:42:16.947, Dec (2000) = −14:42:50.20). The local standard of rest (LSR) velocity of this source is 34 km s-1 (Alcolea et al., 2001). We obtained pipeline-processed data by first utilizing the Starlink1 software package ORAC-DR, and then converting the spectra to the CLASS format. Following this, we performed additional data reduction using the GILDAS2 software including CLASS. The conversion of antenna temperature
In the present study, we have considered the molecular outflow emanating from OH 231.8+4.2 as a homogenous elliptical source, characterized by its major and minor axes
where the FWHM is Full Width at Half Maximum of the obtained beam. We adopt an angular source size of
3 Results and analysis
The lower panel of Figure 1 shows the JCMT spectrum with a center frequency of 262004.2266 MHz and a bandwidth of 1 GHz, while the upper panel displays an expanded view of the spectrum between −100 and 100 km s-1. In the lower panel spectrum, we detect lines arising from SO (J = 76–65) and SO2 (J = 4(4,0) – 5(3,3), J = 11(3,9) – 11(2,10)). Note that their shape fits quite well to those of 13CO transitions on Figure 2 of Velilla Priet et al. (2015), including even some of the minor spectral features identified. However, not much can be seen as far as the shape of HC3N transitions are concerned. In the upper panel, we mark the line positions of the J = 7/2–5/2 and J = 5/2–3/2 transitions in the N = 3–2 rotational transition of C2H. In our observations the hyperfine transitions of both rotational lines (N = 3–2, J = 7/2–5/2, F = 4–3 (262004.2266 MHz) and F = 3–2 (262006.40340 MHz) and N = 3–2, J = 5/2–3/2, F = 4–3 (262064.8433 MHz) and F = 2–1 (262006.40340 MHz)) could be resolved with the original resolution, however, even if detected cannot be resolved after resampling. Despite the undertaken observational efforts, the emission of C2H is not detected at a noise level of 1 mK. Table 1 summarizes the upper limits for the C2H lines in OH 231.8+4.2.
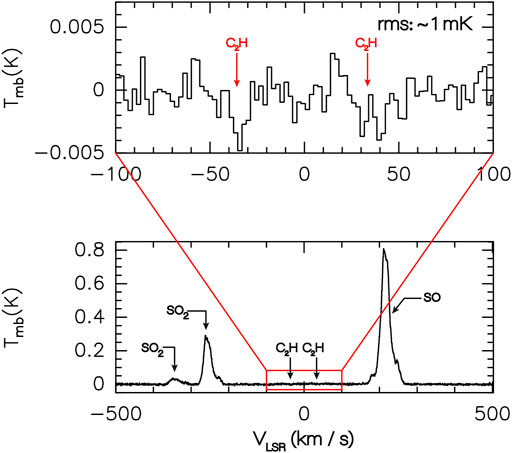
Figure 1. Molecular spectra observed toward OH 231.8+4.2 identified with the JCMT 15 m. The lower panel spectrum with a center frequency of 262004.2266 MHz and a bandwidth of 1 GHz, including two emission lines for SO2 and one emission line for SO; The top panel spectrum shows frequency positions of the J = 7/2–5/2 and J = 5/2–3/2 transitions in the N = 3–2 rotational transition of C2H, the root mean square (rms) noise level is 1 mK.
To determine the column density and fractional abundance with relative to H2, we assume that the spectral line is optically thin and all levels involved in the transitions are under local thermodynamic equilibrium (LTE) conditions. We use the rotational diagram method (see Eq. 2) to estimate the abundance of C2H. This method has been extensively described and discussed by Goldsmith and Langer. (1999). The equation is as follows:
Here, N is the total column density of the molecule, Q is the partition function,
We assume that the excitation temperature and linewidth of C2H are the same as HC3N reported by Velilla Prieto et al. (2015), which are 17 K and 27 km s-1. Based on our calculations, the column density of C2H is less than 1.4
In order to determine the relative abundance of C2H to H2, it is necessary to evaluate the average H2 column density within the radius occupied by C2H. This can be achieved by employing the following equation (Gong et al., 2015).
Here,
Due to the lack of mapping observations on C2H in this source, it is hypothesized that the C2H molecule originates from the slow-moving central component of the molecular outflow that emanates from OH 231.8+4.2. The outer radius of this PPN is 7
4 Discussion
In carbon-rich environment, HC3N is formed demanding the presence of CN and C2H2 as parent molecules. Since C2H2 cannot be observed in radio wavelengths and C2H is a result of C2H2 photodissociation, we have decided to search for the C2H transitions in OH 231.8+4.2. Molecular C2H are often used to explain the formation of HC3N, such as in the case of PPNe CRL 618, CRL 2688, where both C2H and HC3N molecules have been detected (Pardo et al., 2005; Zhang et al., 2013) and which are in the same evolutionary stage as OH 231.8+4.2. Unlike CRL 618 and CRL 2688, OH 231.8+4.2 exhibits a massive bipolar outflow and a rotating disk (Sánchez Contreras et al., 2022). This leads to the presence of a diverse array of species within this source, including ions and carbon-containing species (Sánchez Contreras et al., 1997; Sánchez Contreras et al., 2015). The observed relative abundance of C2H is higher than that of HC3N in C-rich environments of evolved stars. For example, in IRC+10216, the relative abundances of C2H and HC3N are 2.8
5 Conclusion
Despite a sensitive search to a noise level of 1 mK, we did not detect emission from C2H in OH 231.8+4.2. This result is surprising given the close correspondence between C2H and HC3N seen in C-rich AGB stars and the fact that in these objects, the acetylene molecule, C2H2, is thought to be a direct chemical precursor through its reaction with CN. Its non-detection may be due to one or more of several factors: one, that the C2H is removed very rapidly in the oxygen-rich environment of OH 231.8+4.2; two, that the intrinsic physical properties (shocks, jets) prevent its existence; three, that C2H2 may not be present in sufficient abundance to form abundant C2H through photodissociation. In this case, the HC3N detected in OH 231.8+4.2 must be formed via a different chemical route than that which occurs in C-rich AGB circumstellar envelopes. Our study indicates the need for future research on the formation and evolution of carbon chain molecules in oxygen-rich environment.
Data availability statement
The raw data supporting the conclusion of this article will be made available by the authors, without undue reservation.
Author contributions
JY: Writing–original draft, Writing–review and editing. XY: Funding acquisition, Investigation, Resources, Writing–review and editing. CZ: Investigation, Methodology, Writing–review and editing.
Funding
The author(s) declare that financial support was received for the research, authorship, and/or publication of this article. CZ thanks the Xinjiang Tianchi Talent Program (2024). This work is supported by the National SKA Program of China No. 2022SKA0110203 and Xiaofeng Yang’s CAS Pioneer Hundred Talents Program. The James Clerk Maxwell Telescope is operated by the East Asian Observatory on behalf of The National Astronomical Observatory of Japan; Academia Sinica Institute of Astronomy and Astrophysics; the Korea Astronomy and Space Science Institute; the National Astronomical Research Institute of Thailand; Center for Astronomical Mega-Science (as well as the National Key R&D Program of China with No. 2017YFA0402700); Additional funding support is provided by the Science and Technology Facilities Council of the United Kingdom and participating universities and organizations in the United Kingdom and Canada. This work was supported by the JCMT E21AZ002 and M23BP059 project.
Conflict of interest
The authors declare that the research was conducted in the absence of any commercial or financial relationships that could be construed as a potential conflict of interest.
Publisher’s note
All claims expressed in this article are solely those of the authors and do not necessarily represent those of their affiliated organizations, or those of the publisher, the editors and the reviewers. Any product that may be evaluated in this article, or claim that may be made by its manufacturer, is not guaranteed or endorsed by the publisher.
Footnotes
2http://www.iram.fr/IRAMFR/GILDAS
3https://splatalogue.online//advanced.php
References
Agúndez, M., Cernicharo, J., Quintana-Lacaci, G., Castro-Carrizo, A., Velilla Prieto, L., Marcelino, N., et al. (2017). Growth of carbon chains in IRC+10216 mapped with ALMA. Astronomy Astrophysics 601, A4. doi:10.1051/0004-6361/201630274
Alcolea, J., Bujarrabal, V., Sánchez Contreras, C., Neri, R., and Zweigle, J. (2001). The highly collimated bipolar outflow of OH 231.8+4.2. Astronomy Astrophysics 373 (3), 932–949. doi:10.1051/0004-6361:20010535
Bosman, A. D., Alarcón, F., Zhang, K., and Bergin, E. A. (2021). Destruction of refractory carbon grains drives the final stage of protoplanetary disk chemistry. Astrophysical J. 910, 3. doi:10.3847/1538-4357/abe127
Bujarrabal, V., Fuente, A., and Omont, A. (1994). Molecular observations of O-rich and C-rich circumstellar envelopes. Astronomy Astrophysics 285 (1), 247–271.
Cherchneff, I., Glassgold, A. E., and Mamon, G. A. (1993). The Formation of cyanopolyyne molecules in IRC+10216. Astrophysical J. 410 (1), 188–201. doi:10.1086/172737
Goldsmith, P. F., and Langer, W. D. (1999). Population diagram analysis of molecular line emission. Astrophysical J. 517, 209–225. doi:10.1086/307195
Gong, Y., Henkel, C., Spezzano, S., Thorwirth, S., Menten, K. M., Wyrowski, F., et al. (2015). A 1.3 cm line survey toward IRC+10216. Astronomy Astrophysics 574, A56. doi:10.1051/0004-6361/201424819
Huggins, P. J., and Glassgold, A. E. (1982). The photochemistry of carbon-rich circumstellar shells. Astrophysical J. 252 (1), 201–207. doi:10.1086/159547
Li, X., Millar, T. J., Walsh, C., Heays, A. N., and van Dishoeck, E. F. (2014). Photodissociation and chemistry of N2 in the circumstellar envelope of carbon-rich AGB stars. Astronomy Astrophysics 568, A111. doi:10.1051/0004-6361/201424076
Morris, M., Guilloteau, S., Lucas, R., and Omont, A. (1987). The rich molecular-spectrum and the rapid outflow of OH 231.8+4.2. Astrophysical J. 321 (2), 888–897. doi:10.1086/165681
Pardo, J. R., Cernicharo, J. R., Goicoechea, J. R., Guélin, M., and Phillips, T. G. (2005). The millimeter and submillimeter spectrum of CRL618. Proc. Dusty Mol. Universe A Prelude Herschel Alma 577, 455–456.
Sánchez Contreras, C., Alcolea, J., Rodríguez-Cardoso, R., Bujarrabal, V., Castro-Carrizo, A., Velilla-Prieto, L., et al. (2022). ALMA (finally!) discloses a rotating disk+bipolar wind system at the centre of the wind-prominent pPN OH 231.8+4.2. Proc. Int. Astronomical Union 16 (S366), 301–307. doi:10.1017/s1743921322000102
Sánchez Contreras, C., Bujarrabal, V., and Alcolea, J. (1997). The rich molecular content of OH 231.8+4.2. Astronomy Astrophysics 327 (2), 689–698.
Sánchez Contreras, C., de Paz, A. G., and Sahai, R. (2004). The companion to the central mira star of the protoplanetary nebula OH 231.8+4.2. Astrophysical J. 616 (1), 519–524. doi:10.1086/424827
Sánchez Contreras, C., Velilla Prieto, L., Agúndez, M., Cernicharo, J., Quintana-Lacaci, G., Bujarrabal, V., et al. (2015). Molecular ions in the O-rich evolved star OH231.8+4.2: HCO+, H13CO+ and first detection of SO+, N2H+, and H3O+. Astronomy Astrophysics 577, A52. doi:10.1051/0004-6361/201525652
Velilla Prieto, L., Sánchez Contreras, C., Cernicharo, J., Agúndez, M., Quintana-Lacaci, G., Alcolea, J., et al. (2015). New N-bearing species towards OH 231.8+4.2. Astronomy Astrophysics 575, A84. doi:10.1051/0004-6361/201424768
Keywords: post-AGB star, circumstellar envelopes (CSEs), column density, C2H, OH 231.8+4.2
Citation: Yang J, Yang X and Zhu C (2024) A deep search for C2H in the oxygen-rich post-AGB star OH 231.8+4.2. Front. Astron. Space Sci. 11:1431250. doi: 10.3389/fspas.2024.1431250
Received: 11 May 2024; Accepted: 27 May 2024;
Published: 25 June 2024.
Edited by:
Tom Millar, Queen’s University Belfast, United KingdomReviewed by:
Xuan Fang, Chinese Academy of Sciences (CAS), ChinaSchmidt Schmidt, Polish Academy of Sciences, Poland
Copyright © 2024 Yang, Yang and Zhu. This is an open-access article distributed under the terms of the Creative Commons Attribution License (CC BY). The use, distribution or reproduction in other forums is permitted, provided the original author(s) and the copyright owner(s) are credited and that the original publication in this journal is cited, in accordance with accepted academic practice. No use, distribution or reproduction is permitted which does not comply with these terms.
*Correspondence: Xiaofeng Yang, eGZ5YW5nQHhhby5hYy5jbg==