- 1NASA Goddard Space Flight Center, Greenbelt, MD, United States
- 2Department of Astronomy, University of Maryland-College Park, College Park, MD, United States
- 3Center for Research and Exploration in Space Science and Technology, University of Maryland-College Park, College Park, MD, United States
- 4Department of Physics, Indiana University, Bloomington, IN, United States
Multi-messenger observations and theories of astrophysical objects are rapidly becoming a critical research area in the astrophysics scientific community. In particular, point-like objects such as BL Lacertae (BL Lac) objects, flat-spectrum radio quasars (FSRQs), and blazar candidates of uncertain type (BCUs) are of distinct interest to researchers studying the synchrotron, Compton, neutrino, and cosmic ray emissions sourced from compact objects. Notably, there is also much interest in the correlation between multi-frequency observations of blazars and neutrino surveys on source demographics. In this review, we look at such multi-frequency and multi-physics correlations of the radio, X-ray, and
1 Introduction
Active galactic nuclei (AGN) are the largest, most luminous, and persistent extragalactic objects observed in the Universe. These sources feature emissions across the full gamut of electromagnetic spectra, from radio to
The Fermi-LAT collaboration (Atwood et al., 2009) has generated one of the most extensive catalogs of AGN in the high-energy regime (Ajello et al., 2020; Abdollahi et al., 2020; Ballet et al., 2023). A growing number of developing probe and mission concepts are dedicated to the multi-messenger aspects of observing these energetic objects with variable emissions. Additionally, when considering correlations of higher-energy observations with radio emissions of blazars, the joint Monitoring Of Jets in Active galactic nuclei with VLBA Experiments (MOJAVE)–FERMI (Lister et al., 2011) catalog correlates these emission regimes observed by Fermi-LAT and MOJAVE collaborations. Similarly, on the lower end of the frequency spectrum, the MOJAVE (Lister et al., 2009) is stated as being a long-term program that observes the brightness and polarization of radio jets in AGN. Furthermore, sources are continuously added to the joint MOJAVE-FERMI AGN catalog (Kramarenko et al., 2021). Recommendations from the Pathways to Discovery in Astronomy and Astrophysics for the 2020s (Astro2020) (National Academies of Sciences and Medicine, 2023) have generated a number of products and initiatives that prioritize science gaps for time-domain and multi-messenger (TDAMM) (ESA/ATG medialab, 2023) astrophysics. The
The remainder of this review is organized as follows: Section 2 provides a focused description of state-of-the-art physical characteristics of blazars and their emitted jets across a multi-physics regime looking at the intersecting physics of jet launching; Section 3 reviews current efforts that explore multi-spectral correlations and variability in blazars; and lastly, we end this paper with a discussion on multi-messenger science gaps, making parallels with other high-energy point-like objects that show similar emission characteristics as blazars. This section also highlights ongoing efforts and projects that attempt to reveal new areas of scientific interest in relation to a central black hole.
2 Multi-physics characteristics of blazars
2.1 Power spectrum
Relativistic jets comprise non-thermal emission within the AGN spectra, ranging from synchrotron sources of radio emission to higher-energy
where the critical frequency,
with
2.2 Jet emission mechanisms
Currently, the mechanisms for relativistic jet emissions associated with AGN and other high-energy astrophysical objects like
where Eq. 3 provides the BZ luminosity. Here, we define the parameters
As mentioned, a relativistic jet is described as a beam of light that carries linear momentum and, thus, is influenced by an appreciable amount of external angular momentum in both the non-relativistic and relativistic regimes. This angular momentum would then be dependent on the origin of an associated coordinate system, owing to the intrinsic gauge dependence of angular momentum in fundamental physics descriptions. If we then proceed to describe BL Lac and FSRQ blazars as energetic point sources, we can infer the physical characteristics of the jet emission as relativistic beams transported across galactic distances. These point sources should then inherently carry rotational symmetry corresponding to rotated field lines with respect to the host black hole (Gamble, 2022). The following equations of motion described in Eq. 4, specifically under the influence of curved spacetime near the jet-launching region, illustrate the complexities of jet launching from the supermassive black holes of blazar types. Here, the potentials parameterizing particle paths in this near-horizon region are defined, yielding a set of Hamilton–Jacobi equations for each direction. It is easy to see the expected symmetries in the particle paths for the
where the functions
Here,
3 Multi-spectral variability of blazars
3.1 Variability and flaring of VLBI-selected blazars
Observing the variability of blazars can reveal the necessary information to infer the composition of the jet emissions, the mechanisms behind the jet formation, and changes in the accretion rate of the accretion disk and can allow for the localization of the innermost emitting regions (Lawrence, 2016; Valverde et al., 2020). As the central supermassive black hole (SMBH) at the cores of blazars accretes matter and forms the surrounding accretion disk, it launches relativistic jets that emit across the electromagnetic spectrum (radio to
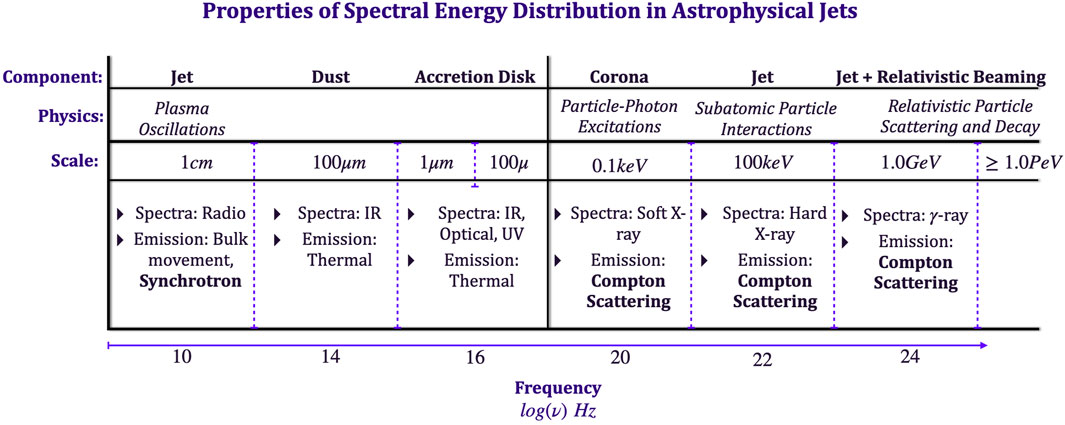
Figure 1. Properties of relativistic jet spectra and their corresponding radiation transfer phenomena (Gamble, 2022).
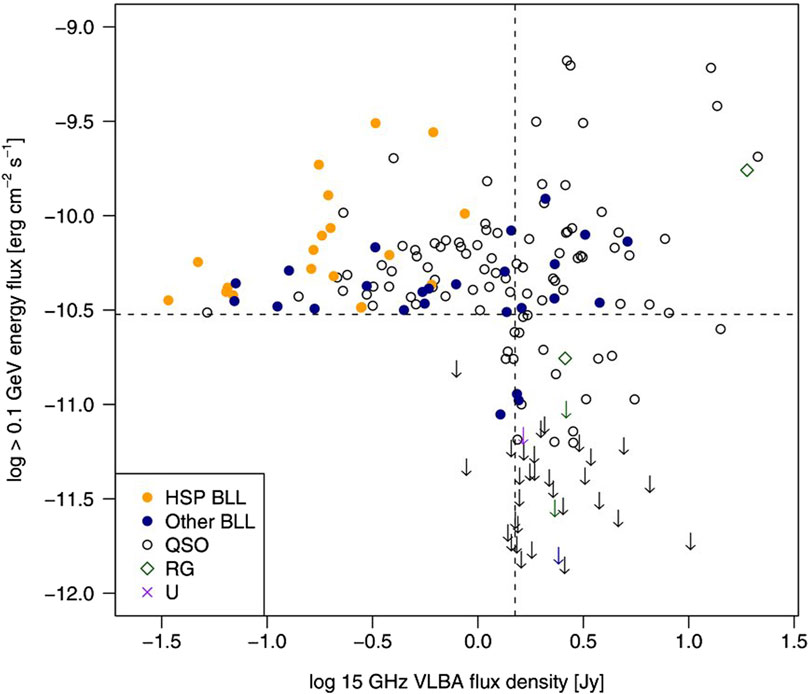
Figure 2. Plot of 11-month Fermi average
Figure 3 shows that there exists a delayed variability in the radio emission for the blazar TXS 0506 + 056 (4FGL J0509.4 + 0542) compared to its higher-energy counterpart in the light curve at
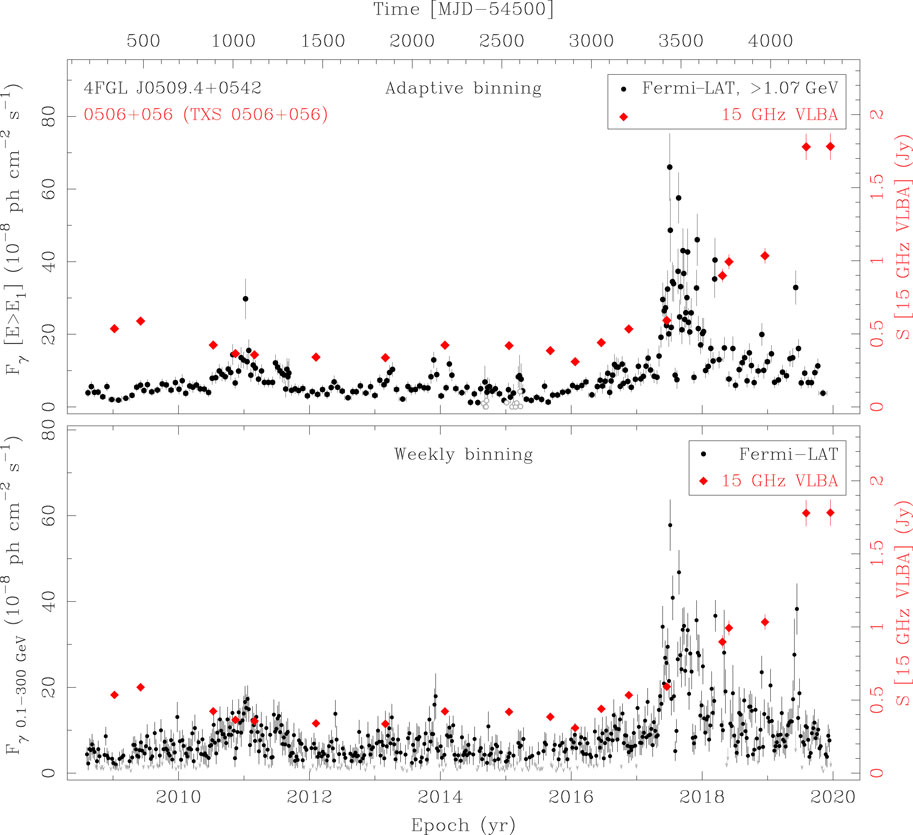
Figure 3. Intra-week variability overlap of the
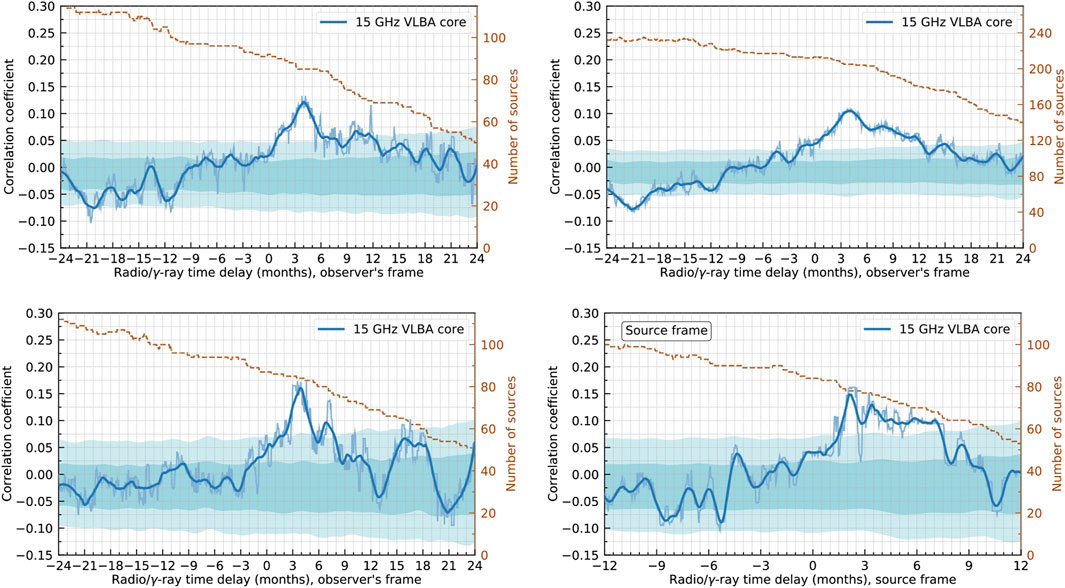
Figure 4. Intra-week variability cross-correlation at 15-GHz VLBA. Reproduced with permission from Kramarenko et al. (2021).
4 Discussion
4.1 Blazar parallels with -ray bursts
Given the nature of the high-energy emission characteristics of BL Lac and FSRQ blazars, it is additionally safe to compare them to GRBs. Both types of high-energy sources are considered to be sourced by compact objects (i.e., SMBH, X-ray binaries, neutron star mergers, core-collapse supernovae, and stellar mass black holes). Both energetic phenomena exhibit similar physical characteristics when considering their respective ejecta mechanisms. It is no coincidence that GRBs and blazar jets also feature similarities in the spectral peaks, illuminating commonalities in their respective radiation physics (Nemmen et al., 2012). A more detailed description of these physical comparisons can be found in works highlighting such comparisons (Lyu et al., 2014; Srinivasaragavan et al., 2023). An even more interesting recent inclusion in the “AGN zoo” is changing-look blazars. These are blazars that feature changes in their accretion processes, intrinsically changing from FSRQ-type to BL Lac and vice versa (Kang et al., 2024). This suggests that further investments in TDAMM science and its technological developments are needed to further elucidate the dynamical properties of AGN with blazar types, BL Lac, FSRQs, and BCUs.
4.2 Ground-based follow-up
4.2.1 ALMA: radio
Specifically, within the radio frequency regime, the ground-based ALMA (Wootten and Thompson, 2009) is extraordinary for observing, in general, AGN of different classifications as it provides a perspective of these high-energy objects in the radio and infrared spectrum. With its ground-breaking interferometric array of 66 high-precision antennas, its performance results in high-resolution images with the brightness sensitivity of a single-antenna array (Brown et al., 2004). LSP BL Lac objects offer a distinctive spectral climb when comparing their
Quasar PKS 1549–79 was previously observed by Oosterloo et al. (2019) in order to analyze its radio jet, using millimeter- and very long baseline interferometry 2.3-GHz continuum observations. PKS 1549–79 is known as a radio-loud quasar, having a stronger radio emission and higher energy than the more common radio-quiet quasar (Barvainis et al., 2005). PKS 1549–79 is also the closest quasar that has been observed merging with an AGN in the first phases of its evolution. Oosterloo et al. (2019) also presented CO (1–0) and CO (3–2) observations of its molecular gas. Their results showed that the massive outflow of 650
4.2.2 IceCube: neutrinos and cosmic rays
The flaring and variability of the blazar spectra listed in the MOJAVE-FERMI catalog, the Fermi-LAT catalogs, and various others that feature high-energy
This interaction of accelerated protons with
Ultimately, the presence of these cascades detected by neutrino and Cherenkov telescopes is a prominent clue for finding relativistic protons in the jet (Muecke et al., 1999; Cerruti, 2020). The IceCube Neutrino Observatory (Aartsen et al., 2017) has made significant progress in detecting neutrinos of astrophysical origin emanating from blazars. Blazars, such as TXS 0506 + 056 (4FGL J0509.4 + 0542) and PKS 0735 + 178 (4FGL J0738.1 + 1742), have been extensively studied in recent years (Padovani et al., 2015; IceCubeFermi-LATMAGICAGILEASAS-SNHAWC et al., 2018; Prince et al., 2023). Multi-messenger observations and their follow-up have thus proven to be a powerful methodology for determining the VHE characteristics of blazars.
5 Conclusion
This focused review of blazars of type FSRQ, BL Lac, and BCU shows just how dynamic these point-like objects are regarding their relativistic properties. The multi-physical nature of such astronomical objects suggests significant gaps in our understanding of their multi-messenger characteristics. The recommendations from the Astro2020 decadal survey offer an initiation of thoughts surrounding TDAMM science gaps. Further investments from the broader astronomy/astrophysics community are required to elucidate and decipher the true nature of blazars, their relativistic jet emission, and future multi-spectral analyses and missions. The utilization of unconventional thoughts and methodologies would prove useful in our quest to understand the energetic Universe. The synergy between radio (ALMA and MOJAVE), X-ray (IXPE, XRISM, Chandra, and SWIFT),
Author contributions
RG: conceptualization, data curation, formal analysis, funding acquisition, investigation, methodology, project administration, resources, software, supervision, validation, visualization, writing–original draft, and writing–review and editing. JF: conceptualization and writing–original draft. AB: conceptualization and writing–original draft. GS: conceptualization and writing–review and editing. IH: conceptualization and writing–review and editing. MJ: conceptualization and writing–review and editing.
Funding
The author(s) declare that financial support was received for the research, authorship, and/or publication of this article. The material is based upon work supported by NASA under award number 80GSFC21M0002.
Acknowledgments
This research used data from the MOJAVE database that is maintained by the MOJAVE team (Lister et al., 2011). The authors thank the reviewers for their valuable remarks and comments.
Conflict of interest
The authors declare that the research was conducted in the absence of any commercial or financial relationships that could be construed as a potential conflict of interest.
Publisher’s note
All claims expressed in this article are solely those of the authors and do not necessarily represent those of their affiliated organizations, or those of the publisher, the editors, and the reviewers. Any product that may be evaluated in this article, or claim that may be made by its manufacturer, is not guaranteed or endorsed by the publisher.
References
Aartsen, M., Ackermann, M., Adams, J., Aguilar, J., Ahlers, M., Ahrens, M., et al. (2017). The icecube neutrino observatory: instrumentation and online systems. J. Instrum. 12, P03012. doi:10.1088/1748-0221/12/03/p03012
Abdo, A. A., Ackermann, M., Agudo, I., Ajello, M., Aller, H. D., Aller, M. F., et al. (2010a). The spectral energy distribution of fermi bright blazars. Astrophysical J. 716, 30–70. doi:10.1088/0004-637X/716/1/30
Abdo, A. A., Ackermann, M., Ajello, M., Baldini, L., Ballet, J., Barbiellini, G., et al. (2010b). Fermilarge area telescope observations of misaligned active galactic nuclei. Astrophys. J. 720, 912–922. doi:10.1088/0004-637X/720/1/912
Abdollahi, S., Acero, F., Ackermann, M., Ajello, M., Atwood, W. B., Axelsson, M., et al. (2020). Fermi large area telescope fourth source catalog. Astrophysical J. 247, 33. doi:10.3847/1538-4365/ab6bcb
Ajello, M., Angioni, R., Axelsson, M., Ballet, J., Barbiellini, G., Bastieri, D., et al. (2020). The fourth catalog of active galactic nuclei detected by the fermi large area telescope. Astrophysical J. 892, 105. doi:10.3847/1538-4357/ab791e
Akiyama, K., Alberdi, A., Alef, W., Carlos Algaba, J., Anantua, R., et al. (2022). First Sagittarius A* event horizon telescope results. V. Testing astrophysical models of the galactic center black hole. Astrophys. J. Lett. 930, L16. doi:10.3847/2041-8213/ac6672
Atwood, W. B., Abdo, A. A., Ackermann, M., Althouse, W., Anderson, B., Axelsson, M., et al. (2009). The large area telescope on the fermi gamma-ray space telescope mission. Astrophysical J. 697, 1071–1102. doi:10.1088/0004-637x/697/2/1071
Badole, S., Jackson, N., Hartley, P., Sluse, D., Stacey, H., and Vives-Arias, H. (2021). VLA and ALMA observations of the lensed radio-quiet quasar SDSS J0924 + 0219: a molecular structure in a 3 μJy radio source. Oxf. Acad. 496, 138–151. doi:10.1093/mnras/staa1488
Ballet, J., Bruel, P., Burnett, T. H., and Lott, B.The Fermi-LAT collaboration (2023). Fermi large area telescope fourth source catalog data release 4 (4FGL-DR4). arXiv:2307.12546.
Barvainis, R., Lehar, J., Birkinshow, M., Falcke, H., and Blundell, K. M. (2005). Radio variability of radio-quiet and radio-loud quasars. Astrophysical J. 618, 1. doi:10.1086/425859
Blandford, R. D., and Znajek, R. L. (1977). Electromagnetic extraction of energy from Kerr black holes. Mon. Notices R. Astronomical Soc. 179, 433–456. doi:10.1093/mnras/179.3.433
Böttcher, M. (2007). Modeling the emission processes in blazars. Astrophysics Space Sci. 309, 95–104. doi:10.1007/978-1-4020-6118-9_16
Brown, R. L., Wild, W., and Cunningham, C. (2004). Alma - the atacama large millimeter array. Adv. Space Res. 34, 555–559. doi:10.1016/j.asr.2003.03.028
Burns, E., Coughlin, M., Ackley, K., Andreoni, I., Bizouard, M.-A., Broekgaarden, F., et al. (2023). Gamma-ray transient network science analysis group report. arXiv:2308.04485.
Cerruti, M. (2020). Leptonic and hadronic radiative processes in supermassive-black-hole jets. Galaxies 8, 72. doi:10.3390/galaxies8040072
D’Ammando, F. (2019). Relativistic jets in gamma-ray-emitting narrow-line Seyfert 1 galaxies. Galaxies 7, 87. doi:10.3390/galaxies7040087
ESA/ATG medialab (2023). The dynamic universe: realizing the science potential of time domain and multi-messenger astrophysics (tdamm).
Fan, J. H., Yang, J. H., Liu, Y., Luo, G. Y., Lin, C., Yuan, Y. H., et al. (2016). The spectral energy distributions of fermi blazars. Astrophysical J. Suppl. Ser. 226, 20. doi:10.3847/0067-0049/226/2/20
Fossati, G. a., Maraschi, L., Celotti, A., Comastri, A., and Ghisellini, G. (1998). A unifying view of the spectral energy distributions of blazars. Mon. Notices R. Astronomical Soc. 299, 433–448. doi:10.1046/j.1365-8711.1998.01828.x
Garofalo, D., and Singh, C. B. (2021). The astrophysics of rotational energy extraction from a black hole. Nat. Astron. 5, 1086–1088. doi:10.1038/s41550-021-01527-5
Giommi, P., and Padovani, P. (1994). BL Lac reunification. Mon. Notices R. Astronomical Soc. 268, L51–L54. doi:10.1093/mnras/268.1.L51
Gupta, A. C., Kalita, N., Gaur, H., and Duorah, K. (2016). Peak of spectral energy distribution plays an important role in intra-day variability of blazars? Mon. Notices R. Astronomical Soc. 462, 1508–1516. doi:10.1093/mnras/stw1667
Harris, D. E., and Krawczynski, H. (2006). X-ray emission from extragalactic jets. Annu. Rev. Astronomy Astrophysics 44, 463–506. doi:10.1146/annurev.astro.44.051905.092446
Homan, D. C., Cohen, M. H., Hovatta, T., Kellermann, K. I., Kovalev, Y. Y., Lister, M. L., et al. (2021). Mojave. xix. brightness temperatures and intrinsic properties of blazar jets. Astrophysical J. 923, 67. doi:10.3847/1538-4357/ac27af
IceCube, Fermi-LAT, MAGIC, AGILE, ASAS-SN, HAWC Ackermann, M., Adams, J., Aguilar, J. A., Ahlers, M., Ahrens, M., et al. (2018). Multimessenger observations of a flaring blazar coincident with high-energy neutrino icecube-170922a. Science 361, eaat1378. doi:10.1126/science.aat1378
Kang, S.-J., Lyu, B., Wu, Q., Zheng, Y.-G., and Fan, J. (2024). The physical properties of changing-look blazars. Astrophysical J. 962, 122. doi:10.3847/1538-4357/ad0fdf
King, A. R., and Pringle, J. E. (2021). Can the blandford–znajek mechanism power steady jets? Astrophysical J. Lett. 918, L22. doi:10.3847/2041-8213/ac19a1
Koide, S. (2020). Generalized general relativistic magnetohydrodynamic equations for plasmas of active galactic nuclei in the era of the event horizon telescope. Astrophysical J. 899, 95. doi:10.3847/1538-4357/aba743
Komissarov, S. S. (2005). Observations of the blandford-znajek process and the magnetohydrodynamic penrose process in computer simulations of black hole magnetospheres. Mon. Notices R. Astronomical Soc. 359, 801–808. doi:10.1111/j.1365-2966.2005.08974.x
Kramarenko, I. G., Pushkarev, A. B., Kovalev, Y. Y., Lister, M. L., Hovatta, T., and Savolainen, T. (2021). A decade of joint mojave–fermi agn monitoring: localization of the gamma-ray emission region. Mon. Notices R. Astronomical Soc. 510, 469–480. doi:10.1093/mnras/stab3358
Lawrence, A. (2016). Clues to the structure of AGN through massive variability surveys. arXiv:1605.09331.
Liodakis, I., Blinov, D., Potter, S. B., and Rieger, F. M. (2021). Constraints on magnetic field and particle content in blazar jets through optical circular polarization. Mon. Notices R. Astronomical Soc. Lett. 509, L21–L25. doi:10.1093/mnrasl/slab118
Lister, M. L., Aller, M., Aller, H., Hovatta, T., Kellermann, K. I., Kovalev, Y. Y., et al. (2011). Ray and parsec-scale jet properties of a complete sample of blazars from the MOJAVE program. Astrophysical J. 742, 27. doi:10.1088/0004-637X/742/1/27
Lister, M. L., Cohen, M. H., Homan, D. C., Kadler, M., Kellermann, K. I., Kovalev, Y. Y., et al. (2009). MOJAVE: monitoring of jets in active galactic nuclei with VLBA Experiments. VI. Kinemat. Analysis a Complete Sample Blazar Jets 138, 1874–1892. doi:10.1088/0004-6256/138/6/1874
Lyu, F., Liang, E.-W., Liang, Y.-F., Wu, X.-F., Zhang, J., Sun, X.-N., et al. (2014). Distributions of gamma-ray bursts and blazars in the lp–ep-plane and possible implications for their radiation physics. Astrophysical J. 793, 36. doi:10.1088/0004-637X/793/1/36
Max-Moerbeck, W., Hovatta, T., Richards, J. L., King, O. G., Pearson, T. J., Readhead, A. C. S., et al. (2014). Time correlation between the radio and gamma-ray activity in blazars and the production site of the gamma-ray emission. Mon. Notices R. Astronomical Soc. 445, 428–436. doi:10.1093/mnras/stu1749
Mohana A, K., Gupta, A. C., Marscher, A. P., Sotnikova, Y. V., Jorstad, S. G., Wiita, P. J., et al. (2023). Multiband cross-correlated radio variability of the blazar 3c 279. Mon. Notices R. Astronomical Soc. 527, 6970–6980. doi:10.1093/mnras/stad3583
Mufakharov, T., Mingaliev, M., Sotnikova, Y., Naiden, Y., and Erkenov, A. (2015). The observed radio/gamma-ray emission correlation for blazars with the Fermi-LAT and the RATAN-600 data. Mon. Notices R. Astronomical Soc. 450, 2658–2669. doi:10.1093/mnras/stv772
Muecke, A., Rachen, J. P., Engel, R., Protheroe, R. J., and Stanev, T.(1999). Photomeson production in astrophysical sources. arXiv:astro-ph/9905153.
Nathanail, A., and Contopoulos, I. (2014). Black hole magnetospheres. Astrophysical J. 788, 186. doi:10.1088/0004-637x/788/2/186
National Academies of Sciences, E. and Medicine (2023). Pathways to Discovery in astronomy and astrophysics for the 2020s. Washington, DC: The National Academies Press. doi:10.17226/26141
Nemmen, R. S., Georganopoulos, M., Guiriec, S., Meyer, E. T., Gehrels, N., and Sambruna, R. M. (2012). A universal scaling for the energetics of relativistic jets from black hole systems. Science 338, 1445–1448. doi:10.1126/science.1227416
Oosterloo, T., Morganti, R., Tadhunter, C., Oonk, J. R., Bignall, H. E., Tzioumis, T., et al. (2019). Alma observations of pks 1549–79: a case of feeding and feedback in a young radio quasar. Astron. Astrophys. 632, A66. doi:10.1051/0004-6361/201936248
Padovani, P., Alexander, D., Assef, R., De Marco, B., Giommi, P., Hickox, R., et al. (2017). Active galactic nuclei: what’s in a name? Astronomy Astrophysics Rev. 25, 2–91. doi:10.1007/s00159-017-0102-9
Padovani, P., Petropoulou, M., Giommi, P., and Resconi, E. (2015). A simplified view of blazars: the neutrino background. Mon. Notices R. Astronomical Soc. 452, 1877–1887. doi:10.1093/mnras/stv1467
Pei, G., Nampalliwar, S., Bambi, C., and Middleton, M. J. (2016). Blandford–znajek mechanism in black holes in alternative theories of gravity. Eur. Phys. J. C 76, 534. doi:10.1140/epjc/s10052-016-4387-z
Prandini, E., and Ghisellini, G. (2022). The blazar sequence and its physical understanding. Galaxies 10 (1), 35. doi:10.3390/galaxies10010035
Prince, R., Das, S., Gupta, N., Majumdar, P., and Czerny, B. (2023). Dissecting the broad-band emission from ray blazar PKS 0735 + 178 in search of neutrinos. Mon. Notices R. Astronomical Soc. 527, 8746–8754. doi:10.1093/mnras/stad3804
Rieger, F. M. (2019). Gamma-ray astrophysics in the time domain. Galaxies 7, 28. doi:10.3390/galaxies7010028
Sahakyan, N. (2020). Broad-band study of high-synchrotron-peaked BL Lac object 1ES 1218 + 304. Mon. Notices R. Astronomical Soc. 496, 5518–5527. doi:10.1093/mnras/staa1893
Srinivasaragavan, G. P., Swain, V., O’Connor, B. M., Anand, S., Ahumada, T., Perley, D. A., et al. (2023). Characterizing the ordinary broad-lined type ic sn 2023pel from the energetic grb 230812b. arXiv:2310.14397.
Toma, K., and Takahara, F. (2016). Where is the electric current driven in the blandford-znajek process? Proc. Int. Astronomical Union 12, 19–22. doi:10.1017/s1743921316012849
Urry, C. M., and Padovani, P. (1995). Unified schemes for radio-loud active galactic nuclei. Publ. Astronomical Soc. Pac. 107, 803. doi:10.1086/133630
Valverde, J., Horan, D., Bernard, D., Fegan, S., Abeysekara, A. U., Archer, A., et al. (2020). A decade of multiwavelength observations of the TeV blazar 1ES 1215 + 303: extreme shift of the synchrotron peak frequency and long-term optical-gamma-ray flux increase. Astrophysical J. 891, 170. doi:10.3847/1538-4357/ab765d
Wagner, S. J., and Witzel, A. (1995). Intraday variability in quasars and BL lac objects. Annu. Rev. Astronomy Astrophysics 33, 163–197. doi:10.1146/annurev.aa.33.090195.001115
Williams, R. K. (1995). Extracting x rays, pairs from supermassive kerr black holes using the penrose mechanism. Phys. Rev. D. 51, 5387–5427. doi:10.1103/PhysRevD.51.5387
Williams, R. K. (2004). Collimated Escaping Vortical Polare−e+Jets Intrinsically Produced by Rotating Black Holes and Penrose Processes. Astrophysical J. 611, 952–963. doi:10.1086/422304
Wootten, A., and Thompson, A. R. (2009). The atacama large millimeter/submillimeter array. Proc. IEEE 97, 1463–1471. doi:10.1109/JPROC.2009.2020572
Zhang, L. X., and Fan, J. H. (2018). The luminosity correlation analysis for Fermi blazars. Astrophysics Space Sci. 363, 142. doi:10.1007/s10509-018-3363-5
Keywords: high-energy astrophysics, multi-messenger astrophysics, supermassive black holes, blazars, active galactic nuclei, γ-ray, X-ray
Citation: Gamble R Jr, Forman J, Barnes A, Srinivasaragavan G, Holt I and Jones M Jr (2024) Multi-messenger emission characteristics of blazars. Front. Astron. Space Sci. 11:1401891. doi: 10.3389/fspas.2024.1401891
Received: 16 March 2024; Accepted: 10 July 2024;
Published: 05 September 2024.
Edited by:
Alexandre Marcowith, UMR5299 Laboratoire Univers et Particules de Montpellier (LUPM), FranceReviewed by:
Aldo Morselli, Universities and Research, ItalyFilippo D’Ammando, University of Bologna, Italy
Copyright © 2024 Gamble, Forman, Barnes, Srinivasaragavan, Holt and Jones. This is an open-access article distributed under the terms of the Creative Commons Attribution License (CC BY). The use, distribution or reproduction in other forums is permitted, provided the original author(s) and the copyright owner(s) are credited and that the original publication in this journal is cited, in accordance with accepted academic practice. No use, distribution or reproduction is permitted which does not comply with these terms.
*Correspondence: Ronald Gamble, cm9uYWxkLnMuZ2FtYmxlQG5hc2EuZ292