- 1Department of Physics and Astronomy, Texas Tech University, Lubbock, TX, United States
- 2Jet Propulsion Laboratory, California Institute of Technology, Pasadena, CA, United States
- 3National Radio Astronomy Observatory, Charlottesville, VA, United States
- 4Space Telescope Science Institute, NASA, Baltimore, MD, United States
- 5Department of Physics and Astronomy, Johns Hopkins University, Baltimore, MD, United States
Compact objects across the mass spectrum–from neutron stars to supermassive black holes–are progenitors and/or central engines for some of the most cataclysmic phenomena in the Universe. As such, they are associated with radio emission on a variety of timescales and represent key targets for multi-messenger astronomy. Observations of transients in the radio band can unveil the physics behind their central engines, ejecta, and the properties of their surroundings, crucially complementing information on their progenitors gathered from observations of other messengers (such as gravitational waves and neutrinos). In this contribution, we summarize observational opportunities and challenges ahead in the multi-messenger study of neutron stars and black holes using radio observations. We highlight the specific contribution of current U.S. national radio facilities and discuss expectations for the field focusing on the science that could be enabled by facilities recommended by the 2020 Decadal survey such as the next generation Very Large Array (ngVLA).
1 Introduction
The study of compact objects across the mass spectrum—from neutron stars with masses comparable to that of the Sun to supermassive black holes at the center of galaxies hundreds of thousands to billions times more massive—has entered a golden era. Indeed, electromagnetic observations of transients associated with compact objects are being enriched, if not revolutionized, by observations of completely independent messengers, namely, gravitational waves and high-energy neutrinos (e.g., Abbott et al., 2017b; IceCube Collaboration et al., 2018a). While currently multi-messenger studies of compact objects remain limited to a relatively small number of sources, continued effort and investment in the field an greatly impact our understanding of the physics of compact objects across the whole mass spectrum of neutron stars and black holes. Indeed, the mass spectrum of neutron stars and black holes includes regions that are currently poorly characterized, such as the mass range where the dividing line between neutron stars and stellar-mass black holes (the lower mass gap, e.g., Abbott et al., 2020b; Gupta et al., 2020) lies, and the mass range thought to be populated by intermediate-mass black holes (e.g., Abbott et al., 2020a; Greene et al., 2020; Abbott et al., 2024). Improved gravitational-wave and particle detectors envisioned to be operational in the next decade and beyond are key to opening new opportunities for multi-messenger discoveries ahead. At the same time, it is critical that our observational capabilities across the bands of the electromagnetic spectrum continue to improve in parallel with that of gravitational-wave and particle detectors. Otherwise, we will soon reach a stage at which multi-messenger studies of transients associated with compact objects will be limited by the sensitivity of electromagnetic facilities rather than by the horizon distances of gravitational-wave and particle detectors (the current major limitation).
In this short review, we discuss the role that the radio band of the electromagnetic spectrum plays in multi-messenger studies of compact objects, focusing on the science enabled by current and future U.S. national radio facilities. Our paper is organized as follows. In Section 2, we briefly summarize the past and present of time-domain multi-messenger astronomy done with radio observations; in Section 3, we discuss some future opportunities that have great potential for enabling new discoveries and conclude.
2 The radio contribution to multi-messenger studies of compact objects
Radio observations play a key role in all three scientific priorities for the coming decade identified in the Pathways to Discovery in Astronomy and Astrophysics for the 2020s report (hereafter, Astro 2020; National Academies of Sciences Engineering and Medicine, 2021), and are critical to the “New Windows on the Dynamic Universe” science priority area. This priority includes using “time-resolved multi-wavelength electromagnetic observations from space and the ground with non-electromagnetic signals to probe the nature of black holes, neutron stars, and the explosive events and mergers that give rise to them.” In fact, radio wavelength observations play a crucial role in the study of black holes and neutron stars, as emission in this band probes the presence of fast, non-thermally emitting ejecta largely independently of geometric effects. Radio wavelength observations also are critical for enabling very high-resolution observations that can either resolve the ejecta and/or enable proper motion measurement of the source structure (unveiling fast jet components via observations of superluminal motion). Several recent observational results demonstrate the central role played by observations in the radio band in multi-messenger discoveries that are revolutionizing the way we study the cosmos. We briefly summarize these key discoveries in what follows.
2.1 Radio observations and gravitational-wave astronomy
The multi-messenger discovery of GW170817 (Abbott et al., 2017b), a binary neutron star merger for which the gravitational-wave siren was unveiled by LIGO (LIGO Scientific Collaboration et al., 2015) and Virgo (Acernese et al., 2015), initiated what can be considered a revolution in time-domain multi-messenger astronomy of stellar-mass compact objects. GW170817 was accompanied by a short γ-ray burst (GRB; Abbott et al., 2017a), and extensive follow-up identified its kilonova counterpart—a quasi-thermal transient associated with r-process nucleosynthesis occurring in the merger neutron-rich debris (Chornock et al., 2017; Coulter et al., 2017; Cowperthwaite et al., 2017; Drout et al., 2017; Evans et al., 2017; Kasliwal et al., 2017; Nicholl et al., 2017; Pian et al., 2017; Smartt et al., 2017; Soares-Santos et al., 2017; Tanvir et al., 2017; Valenti et al., 2017; Villar et al., 2017). The kilonova identification enabled the arcsec localization of GW170817 and measurement of its distance at approximately 40 Mpc (Hjorth et al., 2017; Im et al., 2017; Levan et al., 2017; Palmese et al., 2017; Pan et al., 2017). Subsequent X-ray-to-radio follow up probed the GRB afterglow (Alexander et al., 2017; Haggard et al., 2017; Hallinan et al., 2017; Margutti et al., 2017; Troja et al., 2017; Mooley et al., 2018a; Mooley et al., 2018b; Margutti et al., 2018). The radio band, in particular, proved unique. Extensive monitoring of GW170817 with the Karl G. Jansky Very Large Array (VLA) revealed a steady increase of the optically thin 3 GHz flux during the first
Overall, radio observations of compact binary mergers containing at least one neutron star can constrain the ejecta structures (energy-speed distributions), the viewing geometries, the densities of the media around the merger sites, the structure of the magnetic field, and provide hints on the nature of the merger remnant (e.g., Nakar and Piran, 2011; Metzger and Bower, 2014; Fong et al., 2016; Horesh et al., 2016; Mooley et al., 2018a; Mooley et al., 2018b; Corsi et al., 2018; Dobie et al., 2018; Hotokezaka et al., 2018; Lazzati et al., 2018; Kathirgamaraju et al., 2019; Gill and Granot, 2020; Liu et al., 2020; Balasubramanian et al., 2021; Makhathini et al., 2021; Nedora et al., 2021; Teboul and Shaviv, 2021; Balasubramanian et al., 2022; Nedora et al., 2023; Sadeh et al., 2024). Looking to the future, as the sensitivities of the LIGO, Virgo, and KAGRA detectors continue to improve (Akutsu et al. 2019; Abbott et al., 2021; Abbott et al., 2022), a collection of a larger sample of multi-messenger detections with deep radio follow-up observations would shed light on many currently open questions (e.g., Corsi et al., 2024). For example, what is the diversity of radio counterparts to compact binary mergers? Do all neutron star binary mergers power jets? As the horizon of multi-messenger studies of neutron star binary mergers reaches the peak of star formation with next-generation, ground-based gravitational-wave detectors such as Cosmic Explorer and the Einstein Telescope (
Radio observations also promise to be critical for extending multi-messenger studies of gravitational wave sources to the highest end of the mass spectrum of compact objects, i.e., the region populated by supermassive black holes found at the center of galaxies (Volonteri et al., 2021). Pulsar timing arrays (PTAs), such as the North American Nanohertz Observatory for Gravitational Waves (NANOGrav), and the Laser Interferometer Space Antenna (LISA) are opening, or will soon open, complementary observational windows on massive black-hole binaries. While PTAs currently probe the stochastic gravitational-wave background from massive black-hole binary populations, over the next decade, both PTAs and LISA will detect individual black hole binaries. Multi-messenger studies of these massive black holes in binaries are critical to constrain, on large scales, the merger rate of massive galaxies and, on smaller scales, the dynamics of stars and gas in galactic cores (e.g., Burke-Spolaor et al., 2019; Arzoumanian et al., 2021; Mangiagli et al., 2022; Amaro-Seoane et al., 2023; Arzoumanian et al., 2023; Agazie et al., 2023; D’Orazio and Charisi, 2023; Liu et al., 2023; Stegmann et al., 2023). Currently, the uncertainties that affect the dynamics of massive black-hole binaries leave open various scenarios predicting different delay times between the galaxy mergers and the black-hole coalescences. This delay time determines, e.g., the LISA detection rate, and depends critically on the residence time (or how long the binary stays) at parsec-scale separations (Katz et al., 2020; DeGraf et al., 2024). The residence time at a given separation can in turn be constrained via radio observations. In fact, when one or both black holes are actively accreting, their AGN jets produce radio emission and jet cores trace the location of the black holes at small separations (1 pc–100 pc), which are spatial scales that can be sampled via very long baseline radio interferometry (VLBI, Burke-Spolaor, 2011; Breiding et al., 2021). Radio wavelength observations also can probe jets that may form right before, during, and after the merger phase, via the interaction between the plasma surrounding the black holes and the magnetic fields, as well as jets originating from accretion on the black holes or their final merger remnant (Schnittman, 2011; Bogdanović et al., 2022).
2.2 Radio observations and high-energy neutrino astronomy
Neutrino astronomy has boomed in recent years, as multi-messenger observations of high-energy neutrinos have been enabled by the IceCube detector (Aartsen et al., 2017b). Cosmic neutrinos are produced when accelerated cosmic rays (high-energy nuclei) interact with radiation fields (photons) or with matter. Because neutrinos can traverse the Universe without being deflected by magnetic fields, they can pinpoint the astrophysical sources that produce them. Identifying the sources of high-energy neutrinos can also shed light on their parent cosmic rays and the physics behind their acceleration (e.g., Ahlers and Halzen, 2018; Halzen and Kheirandish, 2022).
IceCube has discovered an extra-galactic diffuse flux of cosmic high-energy neutrinos (IceCube Collaboration, 2013). The radio band offers key insights for understanding the role of stellar-mass compact objects and their jets as sources of high-energy neutrinos and contributors to the high-energy neutrino background. While stacking analyses have shown that transients such as cosmological GRBs do not contribute a major fraction of the all-sky neutrino flux (Aartsen et al., 2017a; Abbasi et al., 2022; IceCube Collaboration et al., 2023; Lucarelli et al., 2023), theoretical models suggest that radio-emitting but γ-ray–dark, choked jets may lead to efficient high-energy neutrino production (e.g., Murase, 2015; Senno et al., 2016; Esmaili and Murase, 2018; Senno et al., 2018; Chang et al., 2022). Recently, Guarini et al. (2023) have emphasized that, while a significant fraction of the explosion energy of astrophysical transients associated with collapsing massive stars can be emitted in the infrared-optical-ultraviolet band, the optical signal alone is not optimal for neutrino searches. Instead, neutrino emission is strongly correlated with radio emission arising from either strong circumstellar medium interactions or with the presence of a central engine (e.g., Corsi et al., 2014; Corsi et al., 2023). Perhaps one of the most exciting prospects for future multi-messenger detections of stellar-mass compact objects would be to identify compact binary mergers containing neutron stars that could be probed not only via gravitational waves and radio light (as for the case of GW170817) but also via high-energy neutrino counterparts (e.g., Albert et al., 2017; Aartsen et al., 2020; Abbasi et al., 2023b; Mukhopadhyay et al., 2024). The last would probe dissipation mechanisms in relativistic outflows driven by the mergers (Albert et al., 2017; Abbasi et al., 2023a; Matsui et al., 2023).
The identification of the cosmic neutrino IceCube-170922A from the known blazar TXS 0506 + 056 (IceCube Collaboration et al., 2018a) has also established a link between high-energy neutrinos and supermassive black holes in AGNs with jets aligned with our line of sight (γ-ray emitting blazars). Additional associations of high-energy neutrinos with sources other than blazars, such as the Seyfert II galaxy NGC 1068 (IceCube Collaboration et al., 2022) and a few tidal disruption event (TDE) candidates (e.g., Stein et al., 2021), leave open the debate on the relative role of potential γ-ray bright and γ-ray dark (or jet-quiet) high-energy neutrino emitters (Senno et al., 2017; Franckowiak et al., 2020; Kreter et al., 2020; Murase et al., 2020; Plavin et al., 2020; Kimura et al., 2021; McDonough et al., 2023; Murase and Stecker, 2023). In fact, IceCube identified a six-month-long cluster of events from TXS 0506 + 056 in 2014–2015 that was not accompanied by increased γ-ray activity. Both the 2014–2015 neutrino flare and the IceCube-170922A neutrino event from TXS 0506 + 056 are associated with intervals of enhanced radio emission (IceCube Collaboration et al., 2018b). In July 2019, the high-energy neutrino event IC190730A was found spatially coincident with the bright flat-spectrum radio quasar PKS 1502 + 106. While PKS 1502 + 106 was not found to be in a particularly elevated γ-ray state, it exhibited a bright radio outburst at the time of the neutrino detection. In 2022, the IceCube neutrino event IC220225A was identified in spatial coincidence with the flat-spectrum radio quasar PKS 0215 + 015 in a high optical and γ-ray state accompanied by a bright radio outburst (Eppel et al., 2023a; Eppel et al., 2023b).
In AGN jets, radio emission is a good proxy for the general jet activity (Hovatta et al., 2021). An increase in the radio flux density before a γ-ray flare could signal a long-term increase in the total jet power. Statistical studies aimed at understanding the connection between radio-loud AGNs and high-energy neutrinos are particularly important. For example, Plavin et al. (2020) investigated the association of neutrinos with radio-bright AGN and found an average increase of radio emission at frequencies above 10 GHz around neutrino arrival times for several AGNs. Plavin et al. (2021) found a 3σ significance for the correlation between the IceCube point-source likelihood map and the VLBI radio fundamental catalog of AGN. Hovatta et al. (2021) found that observations of flares in OVRO-monitored blazars (at 15 GHz) at the same time as a neutrino events are unlikely to be random coincidences. Suray and Troitsky (2024) highlighted how IceCube neutrinos with energies over 200 TeV previously found to be associated with bright radio blazars are significantly more likely to be accompanied by flares of lower-energy events, compared to those lacking blazar counterparts. On the other hand, Zhou et al. (2021) investigated the possibility that radio-bright AGN are responsible for the TeV-PeV neutrinos detected by IceCube using 3,388 radio-bright AGN selected from the Radio Fundamental Catalog and found that stacking analyses show no significant correlation between the whole catalog and IceCube neutrinos. In summary, it is clear that radio plays an important role in shedding light on supermassive black holes as sources of high-energy neutrinos, though a larger number of high-confidence multi-messenger detections are needed to clarify the exact link between radio emission and sources high-energy neutrinos.
3 Discussion
Among the so-called “Large Programs That Forge the Frontiers,” the Astro2020 report recognized as essential that “the Karl Jansky Very Large Array (VLA) and Very Long Baseline Array (VLBA), which have been the world-leading radio observatories, be replaced by an observatory that can achieve roughly an order of magnitude improvement in sensitivity compared to those facilities. The Next Generation Very Large Array (ngVLA) will achieve this, with a phased approach where design, prototyping, and cost studies are completed and reviewed in advance of commencing construction.” Indeed, the ngVLA promises to be a key facility enabling studies of radio emission from sources of gravitational waves and high-energy neutrinos described in Section 2 to be extended to the larger distance horizons (Ahlers and Halzen, 2014; Aartsen et al., 2021; Evans et al., 2023; Gupta et al., 2023; Corsi et al., 2024).
The ngVLA (Murphy E. J. et al., 2018) is being designed as an interferometric array of 263 antennas with
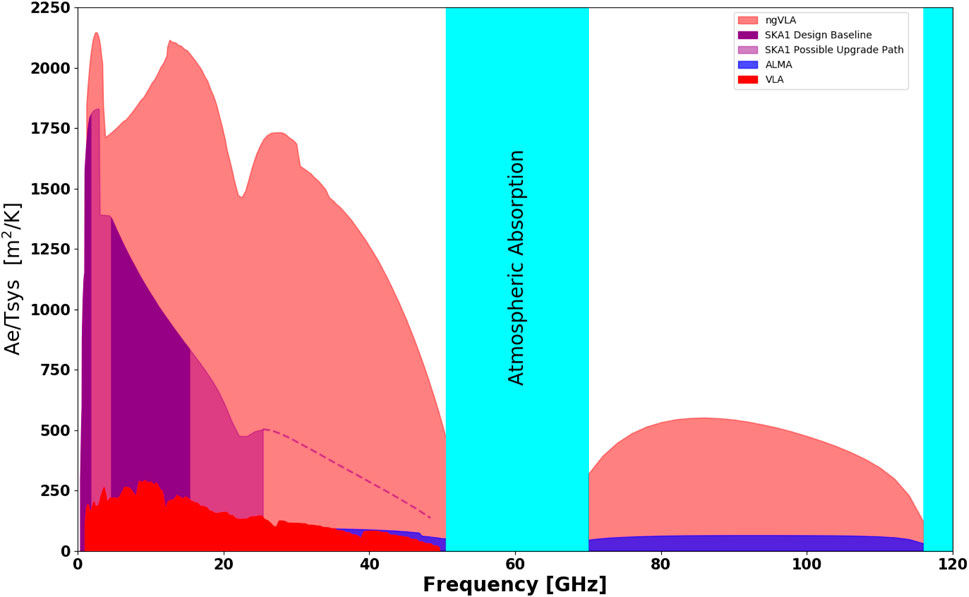
Figure 1. This Figure is an updated version of Figure 9 in Selina et al. (2018). The effective collecting area for the ngVLA is plotted versus frequency and compared to that for other existing (VLA and ALMA) or planned (SKA; Braun et al., 2019) facilities. Compared to the VLA, the ngVLA will have 10×the sensitivity and 10×the resolution at comparable frequencies. As highlighted by Murphy E. et al. (2018), this implies that with the ngVLA it will become possible to map a
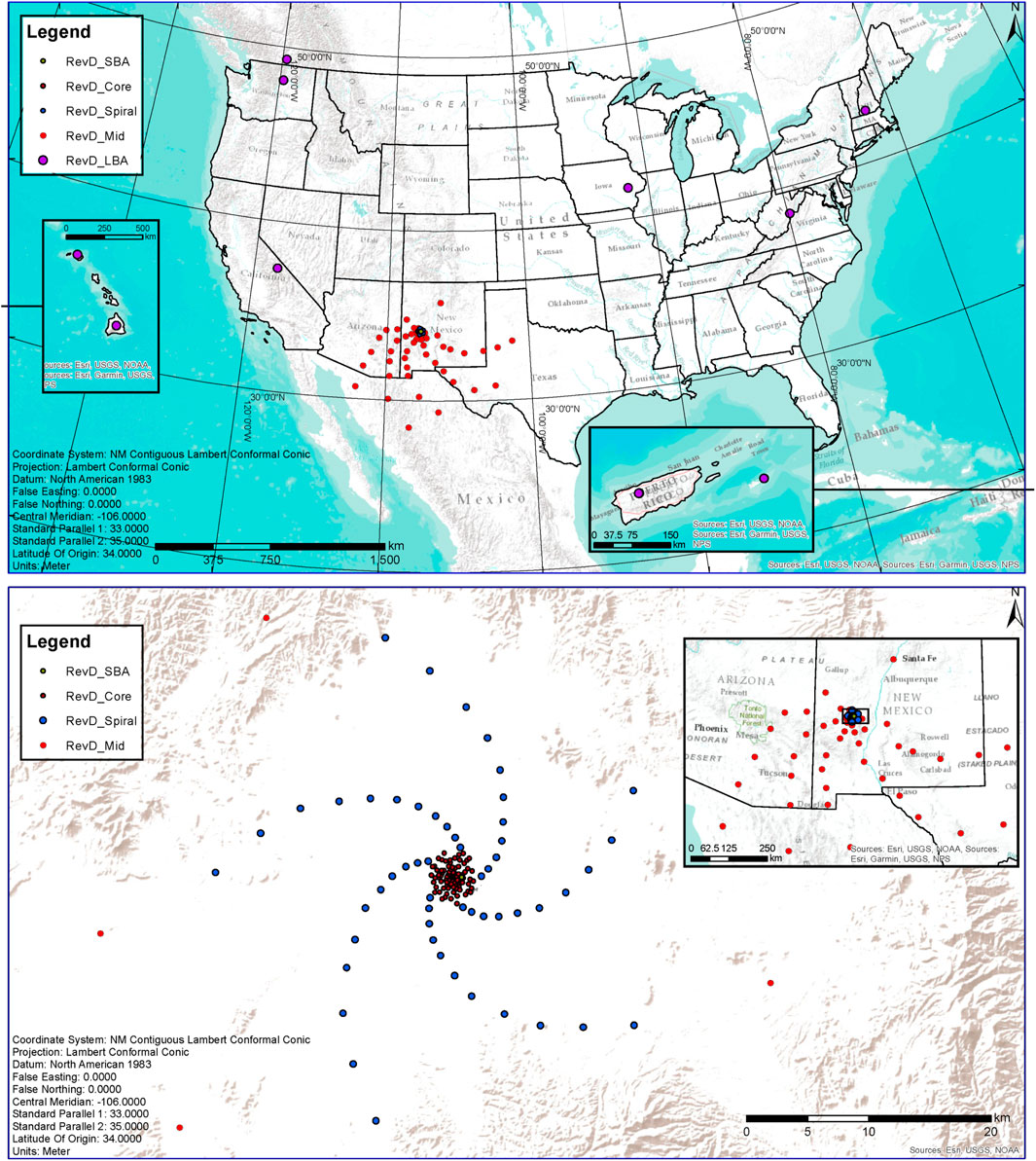
Figure 2. This Figure is reproduced from Murphy (2022). Top: A potential configuration layout for the ngVLA showing all 263 antennas spread across the North American Continent. The Array is centered at the current VLA site on the plains of San Agustin in New Mexico. The legend associates each antenna with a logistical sub-component of the full array. Each of the long-baseline stations (purple dots) consists of three antennas. Bottom: A zoom-in of the main array showing the five-arm spiral pattern (54 18 m antennas) and dense core (114 18 m antennas). The maximum baseline of the spiral and core antennas is 29.3 and 4.3 km, respectively. The 19 6 m Short Baseline Array antennas are located within the central core.
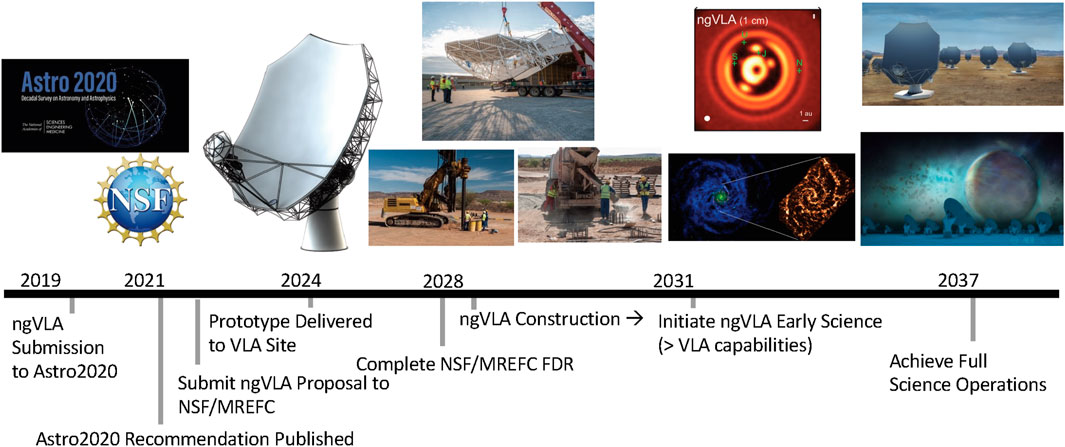
Figure 3. Projected timeline of the ngVLA, showing key milestones on the path to its full scientific capability. This Figure is reproduced from https://ngvla.nrao.edu/download/MediaFile/283/original. Note that dates are tentative and subject to change.
Based on the summary of §2, we expect the ngVLA to begin operations at the culmination of a phase of rapid growth in gravitational-wave and high-energy neutrino astronomy. The detection of radio emission from cataclysmic multi-messenger sources associated with neutron stars and black holes across the mass spectrum can enable their precise localization, help measure their energetics, and provide clues on their surrounding environments. The combination of multi-messenger information will provide a complete picture of the life-cycle of massive stars, the micro-physics of their explosive deaths, and the formation and evolution of neutron stars, stellar-mass black holes, and supermassive black holes. The future of multi-messenger astronomy looks bright, and it is key that the U.S. keeps a leading role in enabling this multi-messenger science in the radio band1.
Author contributions
AC: Writing–original draft, Writing–review and editing. AE: Writing–original draft, Writing–review and editing. TL: Writing–original draft, Writing–review and editing. EM: Writing–original draft, Writing–review and editing. RO: Writing–original draft, Writing–review and editing.
Funding
The author(s) declare that financial support was received for the research, authorship, and/or publication of this article. AC acknowledges support from NSF Grants No. AST-2431072, AST-2307358 and PHY-2011608. The NANOGrav collaboration receives support from National Science Foundation (NSF) Physics Frontiers Center award Nos. 1430284 and 2020265. Part of this research was carried out at the Jet Propulsion Laboratory, California Institute of Technology, under a contract with the National Aeronautics and Space Administration. The next generation Very Large Array (ngVLA) is a design project of the National Radio Astronomy Observatory (NRAO). NRAO is a facility of the NSF operated under cooperative agreement by Associated Universities, Inc.
Conflict of interest
The authors declare that the research was conducted in the absence of any commercial or financial relationships that could be construed as a potential conflict of interest.
Publisher’s note
All claims expressed in this article are solely those of the authors and do not necessarily represent those of their affiliated organizations, or those of the publisher, the editors and the reviewers. Any product that may be evaluated in this article, or claim that may be made by its manufacturer, is not guaranteed or endorsed by the publisher.
Footnotes
1library.nrao.edu/public/memos/ngvla/NGVLA_19.pdf
References
Aartsen, M. G., Abbasi, R., Ackermann, M., Adams, J., Aguilar, J. A., Ahlers, M., et al. (2021). IceCube-Gen2: the window to the extreme Universe. J. Phys. G Nucl. Phys. 48, 060501. doi:10.1088/1361-6471/abbd48
Aartsen, M. G., Ackermann, M., Adams, J., Aguilar, J. A., Ahlers, M., Ahrens, M., et al. (2017a). Extending the search for muon neutrinos coincident with gamma-ray bursts in IceCube data. ApJ 843, 112. doi:10.3847/1538-4357/aa7569
Aartsen, M. G., Ackermann, M., Adams, J., Aguilar, J. A., Ahlers, M., Ahrens, M., et al. (2017b). The IceCube Neutrino Observatory: instrumentation and online systems. J. Instrum. 12, P03012. doi:10.1088/1748-0221/12/03/P03012
Aartsen, M. G., Ackermann, M., Adams, J., Aguilar, J. A., Ahlers, M., Ahrens, M., et al. (2020). IceCube search for neutrinos coincident with compact binary mergers from LIGO-virgo’s first gravitational-wave transient catalog. ApJ 898, L10. doi:10.3847/2041-8213/ab9d24
Abbasi, R., Ackermann, M., Adams, J., Agarwalla, S. K., Aguilar, J. A., Ahlers, M., et al. (2023a). A search for IceCube sub-TeV neutrinos correlated with gravitational-wave events detected by LIGO/Virgo. ApJ 959, 96. doi:10.3847/1538-4357/aceefc
Abbasi, R., Ackermann, M., Adams, J., Aggarwal, N., Aguilar, J. A., Ahlers, M., et al. (2023b). IceCube search for neutrinos coincident with gravitational wave events from LIGO/Virgo run O3. ApJ 944, 80. doi:10.3847/1538-4357/aca5fc
Abbasi, R., Ackermann, M., Adams, J., Aguilar, J. A., Ahlers, M., Ahrens, M., et al. (2022). Searches for neutrinos from gamma-ray bursts using the IceCube neutrino observatory. ApJ 939, 116. doi:10.3847/1538-4357/ac9785
Abbott, B. P., Abbott, R., Abbott, T. D., Acernese, F., Ackley, K., Adams, C., et al. (2017a). Gravitational waves and gamma-rays from a binary neutron star merger: GW170817 and GRB 170817A. ApJ 848, L13. doi:10.3847/2041-8213/aa920c
Abbott, B. P., Abbott, R., Abbott, T. D., Acernese, F., Ackley, K., Adams, C., et al. (2017b). Multi-messenger observations of a binary neutron star merger. ApJ 848, L12. doi:10.3847/2041-8213/aa91c9
Abbott, R., Abbott, T. D., Abraham, S., Acernese, F., Ackley, K., Adams, C., et al. (2020a). GW190521: a binary black hole merger with a total mass of 150 M⊙. Phys. Rev. Lett. 125, 101102. doi:10.1103/PhysRevLett.125.101102
Abbott, R., Abbott, T. D., Abraham, S., Acernese, F., Ackley, K., Adams, C., et al. (2020b). GW190814: gravitational waves from the coalescence of a 23 solar mass black hole with a 2.6 solar mass compact object. ApJ 896, L44. doi:10.3847/2041-8213/ab960f
Abbott, R., Abbott, T. D., Abraham, S., Acernese, F., Ackley, K., Adams, C., et al. (2021). Search for gravitational waves associated with gamma-ray bursts detected by fermi and swift during the LIGO-virgo run O3a. ApJ 915, 86. doi:10.3847/1538-4357/abee15
Abbott, R., Abbott, T. D., Acernese, F., Ackley, K., Adams, C., Adhikari, N., et al. (2022). Search for gravitational waves associated with gamma-ray bursts detected by fermi and swift during the LIGO-virgo run O3b. ApJ 928, 186. doi:10.3847/1538-4357/ac532b
Abbott, R., Abbott, T. D., Acernese, F., Ackley, K., Adams, C., Adhikari, N., et al. (2024). GWTC-2.1: deep extended catalog of compact binary coalescences observed by LIGO and Virgo during the first half of the third observing run. Phys. Rev. D. 109, 022001. doi:10.1103/PhysRevD.109.022001
Acernese, F., Agathos, M., Agatsuma, K., Aisa, D., Allemandou, N., Allocca, A., et al. (2015). Advanced Virgo: a second-generation interferometric gravitational wave detector. Class. Quantum Gravity 32, 024001. doi:10.1088/0264-9381/32/2/024001
Agazie, G., Anumarlapudi, A., Archibald, A. M., Arzoumanian, Z., Baker, P. T., Bécsy, B., et al. (2023). The NANOGrav 15 yr data set: evidence for a gravitational-wave background. ApJ 951, L8. doi:10.3847/2041-8213/acdac6
Ahlers, M., and Halzen, F. (2014). Pinpointing extragalactic neutrino sources in light of recent IceCube observations. Phys. Rev. D. 90, 043005. doi:10.1103/PhysRevD.90.043005
Ahlers, M., and Halzen, F. (2018). Opening a new window onto the universe with IceCube. Prog. Part. Nucl. Phys. 102, 73–88. doi:10.1016/j.ppnp.2018.05.001
Albert, A., André, M., Anghinolfi, M., Ardid, M., Aubert, J. J., Aublin, J., et al. (2017). Search for high-energy neutrinos from binary neutron star merger GW170817 with ANTARES, IceCube, and the pierre auger observatory. ApJ 850, L35. doi:10.3847/2041-8213/aa9aed
Alexander, K. D., Berger, E., Fong, W., Williams, P. K. G., Guidorzi, C., Margutti, R., et al. (2017). The electromagnetic counterpart of the binary neutron star merger LIGO/Virgo GW170817. VI. Radio constraints on a relativistic jet and predictions for late-time emission from the kilonova ejecta. ApJ 848, L21. doi:10.3847/2041-8213/aa905d
Akutsu, T., Ando, M., Arai, K., Arai, Y., Araki, S., Araya, A., et al. (2019). KAGRA: 2.5 generation interferometric gravitational wave detector. Nat. Astron. 3, L17. doi:10.1038/s41550-018-0658-y
Amaro-Seoane, P., Andrews, J., Arca Sedda, M., Askar, A., Baghi, Q., Balasov, R., et al. (2023). Astrophysics with the laser interferometer space antenna. Living Rev. Relativ. 26, 2. doi:10.1007/s41114-022-00041-y
Arzoumanian, Z., Baker, P. T., Blecha, L., Blumer, H., Brazier, A., Brook, P. R., et al. (2023). The NANOGrav 12.5 yr data set: bayesian limits on gravitational waves from individual supermassive black hole binaries. ApJ 951, L28. doi:10.3847/2041-8213/acdbc7
Arzoumanian, Z., Baker, P. T., Brazier, A., Brook, P. R., Burke-Spolaor, S., Becsy, B., et al. (2021). The NANOGrav 11 yr data set: limits on supermassive black hole binaries in galaxies within 500 mpc. ApJ 914, 121. doi:10.3847/1538-4357/abfcd3
Balasubramanian, A., Corsi, A., Mooley, K. P., Brightman, M., Hallinan, G., Hotokezaka, K., et al. (2021). Continued radio observations of GW170817 3.5 yr post-merger. ApJ 914, L20. doi:10.3847/2041-8213/abfd38
Balasubramanian, A., Corsi, A., Mooley, K. P., Hotokezaka, K., Kaplan, D. L., Frail, D. A., et al. (2022). GW170817 4.5 Yr after merger: dynamical ejecta afterglow constraints. ApJ 938, 12. doi:10.3847/1538-4357/ac9133
Bogdanović, T., Miller, M. C., and Blecha, L. (2022). Electromagnetic counterparts to massive black-hole mergers. Living Rev. Relativ. 25, 3. doi:10.1007/s41114-022-00037-8
Branchesi, M., Maggiore, M., Alonso, D., Badger, C., Banerjee, B., Beirnaert, F., et al. (2023). Science with the Einstein Telescope: a comparison of different designs. J. Cosmol. Astropart. Phys. 2023, 068. doi:10.1088/1475-7516/2023/07/068
Braun, R., Bonaldi, A., Bourke, T., Keane, E., and Wagg, J. (2019) Anticipated performance of the square kilometre array – phase 1 (SKA1). arXiv e-prints, arXiv:1912.12699. doi:10.48550/arXiv.1912.12699
Breiding, P., Burke-Spolaor, S., Eracleous, M., Bogdanović, T., Lazio, T. J. W., Runnoe, J., et al. (2021). The search for binary supermassive black holes among quasars with offset broad lines using the very long baseline array. ApJ 914, 37. doi:10.3847/1538-4357/abfa9a
Burke-Spolaor, S. (2011). A radio Census of binary supermassive black holes. MNRAS 410, 2113–2122. doi:10.1111/j.1365-2966.2010.17586.x
Burke-Spolaor, S., Taylor, S. R., Charisi, M., Dolch, T., Hazboun, J. S., Holgado, A. M., et al. (2019). The astrophysics of nanohertz gravitational waves. A&A Rev. 27, 5. doi:10.1007/s00159-019-0115-7
Chang, P.-W., Zhou, B., Murase, K., and Kamionkowski, M. (2022) High-energy neutrinos from choked-jet supernovae: searches and implications. arXiv e-prints, arXiv:2210.03088. doi:10.48550/arXiv.2210.03088
Chornock, R., Berger, E., Kasen, D., Cowperthwaite, P. S., Nicholl, M., Villar, V. A., et al. (2017). The electromagnetic counterpart of the binary neutron star merger LIGO/Virgo GW170817. IV. Detection of near-infrared signatures of r-process nucleosynthesis with gemini-south. ApJ 848, L19. doi:10.3847/2041-8213/aa905c
Corsi, A., Barsotti, L., Berti, E., Evans, M., Gupta, I., Kritos, K., et al. (2024) Multi-messenger astrophysics of black holes and neutron stars as probed by ground-based gravitational wave detectors: from present to future. arXiv e-prints , arXiv:2402.13445. doi:10.48550/arXiv.2402.13445
Corsi, A., Hallinan, G. W., Lazzati, D., Mooley, K. P., Murphy, E. J., Frail, D. A., et al. (2018). An upper limit on the linear polarization fraction of the GW170817 radio continuum. ApJ 861, L10. doi:10.3847/2041-8213/aacdfd
Corsi, A., Ho, A. Y. Q., Cenko, S. B., Kulkarni, S. R., Anand, S., Yang, S., et al. (2023). A search for relativistic ejecta in a sample of ZTF broad-lined type ic supernovae. ApJ 953, 179. doi:10.3847/1538-4357/acd3f2
Corsi, A., Ofek, E. O., Gal-Yam, A., Frail, D. A., Kulkarni, S. R., Fox, D. B., et al. (2014). A multi-wavelength investigation of the radio-loud supernova PTF11qcj and its circumstellar environment. ApJ 782, 42. doi:10.1088/0004-637X/782/1/42
Costa, E., Frontera, F., Heise, J., Feroci, M., Zand, J., Fiore, F., et al. (1997). Discovery of an X-ray afterglow associated with the γ-ray burst of 28 February 1997. Nature 387, 783–785. doi:10.1038/42885
Coulter, A. D., Foley, R. J., Kilpatrick, C. D., Drout, M. R., Piro, A. L., Shappee, B. J., et al. (2017). Swope Supernova Survey 2017a (SSS17a), the optical counterpart to a gravitational wave source. Sci 358 (6370), 1556–1558. doi:10.48550/arXiv.1710.05452
Cowperthwaite, P. S., Berger, E., Villar, V. A., Metzger, B. D., Nicholl, M., Chornock, R., et al. (2017). The electromagnetic counterpart of the binary neutron star merger LIGO/Virgo GW170817. II. UV, optical, and near-infrared light curves and comparison to kilonova models. ApJ 848, L17. doi:10.3847/2041-8213/aa8fc7
DeGraf, C., Chen, N., Ni, Y., Di Matteo, T., Bird, S., Tremmel, M., et al. (2024). High-redshift supermassive black hole mergers in simulations with dynamical friction modelling. MNRAS 527, 11766–11776. doi:10.1093/mnras/stad3084
Dobie, D., Kaplan, D. L., Murphy, T., Lenc, E., Mooley, K. P., Lynch, C., et al. (2018). A turnover in the radio light curve of GW170817. ApJ 858, L15. doi:10.3847/2041-8213/aac105
D’Orazio, D. J., and Charisi, M. (2023) Observational signatures of supermassive black hole binaries. arXiv e-prints, arXiv:2310.16896. doi:10.48550/arXiv.2310.16896
Drout, M. R., Piro, A. L., Shappee, B. J., Kilpatrick, C. D., Simon, J. D., Contreras, C., et al. (2017). Light curves of the neutron star merger GW170817/SSS17a: implications for r-process nucleosynthesis. Science 358, 1570–1574. doi:10.1126/science.aaq0049
Eppel, F., Kadler, M., Ros, E., Benke, P., Giroletti, M., Hessdoerfer, J., et al. (2023a) VLBI probes of jet physics in neutrino-candidate blazars. arXiv e-prints, arXiv:2308.04311. doi:10.48550/arXiv.2308.04311
Eppel, F., Kadler, M., Ros, E., Rösch, F., Heßdörfer, J., Benke, P., et al. (2023b). VLBI scrutiny of a new neutrino-blazar multiwavelength-flare coincidence. In Proc. Int. Astron. Union, the multimessenger chakra of blazar jets, eds. I. Liodakis, M. F. Aller, H. Krawczynski, A. Lähteenmäki, and T. J. Pearson 375, 91–95. doi:10.1017/S1743921323000893
Esmaili, A., and Murase, K. (2018). Constraining high-energy neutrinos from choked-jet supernovae with IceCube high-energy starting events. J. Cosmol. Astropart. Phys. 2018, 008. doi:10.1088/1475-7516/2018/12/008
Evans, M., Corsi, A., Afle, C., Ananyeva, A., Arun, K. G., Ballmer, S., et al. (2023) Cosmic explorer: a submission to the NSF mpsac ngGW subcommittee. arXiv e-prints, arXiv:2306.13745. doi:10.48550/arXiv.2306.13745
Evans, P. A., Cenko, S. B., Kennea, J. A., Emery, S. W. K., Kuin, N. P. M., Korobkin, O., et al. (2017). Swift and NuSTAR observations of GW170817: detection of a blue kilonova. Science 358, 1565–1570. doi:10.1126/science.aap9580
Fong, W., Metzger, B. D., Berger, E., and Özel, F. (2016). Radio constraints on long-lived magnetar remnants in short gamma-ray bursts. ApJ 831, 141. doi:10.3847/0004-637X/831/2/141
Franckowiak, A., Garrappa, S., Paliya, V., Shappee, B., Stein, R., Strotjohann, N. L., et al. (2020). Patterns in the multiwavelength behavior of candidate neutrino blazars. ApJ 893, 162. doi:10.3847/1538-4357/ab8307
Ghirlanda, G., Salafia, O. S., Paragi, Z., Giroletti, M., Yang, J., Marcote, B., et al. (2019). Compact radio emission indicates a structured jet was produced by a binary neutron star merger. Science 363, 968–971. doi:10.1126/science.aau8815
Gill, R., and Granot, J. (2020). Constraining the magnetic field structure in collisionless relativistic shocks with a radio afterglow polarization upper limit in GW 170817. MNRAS 491, 5815–5825. doi:10.1093/mnras/stz3340
Greene, J. E., Strader, J., and Ho, L. C. (2020). Intermediate-mass black holes. ARA&A 58, 257–312. doi:10.1146/annurev-astro-032620-021835
Guarini, E., Tamborra, I., Margutti, R., and Ramirez-Ruiz, E. (2023). Transients stemming from collapsing massive stars: the missing pieces to advance joint observations of photons and high-energy neutrinos. Phys. Rev. D. 108, 083035. doi:10.1103/PhysRevD.108.083035
Gupta, A., Gerosa, D., Arun, K. G., Berti, E., Farr, W. M., and Sathyaprakash, B. S. (2020). Black holes in the low-mass gap: implications for gravitational-wave observations. Phys. Rev. D. 101, 103036. doi:10.1103/PhysRevD.101.103036
Gupta, I., Afle, C., Arun, K. G., Bandopadhyay, A., Baryakhtar, M., Biscoveanu, S., et al. (2023) Characterizing gravitational wave detector networks: from A♯ to cosmic explorer. arXiv e-prints, arXiv:2307.10421. doi:10.48550/arXiv.2307.10421
Haggard, D., Nynka, M., Ruan, J. J., Kalogera, V., Cenko, S. B., Evans, P., et al. (2017). A deep chandra X-ray study of neutron star coalescence GW170817. ApJ 848, L25. doi:10.3847/2041-8213/aa8ede
Hallinan, G., Corsi, A., Mooley, K. P., Hotokezaka, K., Nakar, E., Kasliwal, M. M., et al. (2017). A radio counterpart to a neutron star merger. Science 358, 1579–1583. doi:10.1126/science.aap9855
Halzen, F., and Kheirandish, A. (2022) IceCube and high-energy cosmic neutrinos. arXiv e-prints , arXiv:2202.00694. doi:10.48550/arXiv.2202.00694
Hjorth, J., Levan, A. J., Tanvir, N. R., Lyman, J. D., Wojtak, R., Schrøder, S. L., et al. (2017). The distance to NGC 4993: the host galaxy of the gravitational-wave event GW170817. ApJ 848, L31. doi:10.3847/2041-8213/aa9110
Horesh, A., Hotokezaka, K., Piran, T., Nakar, E., and Hancock, P. (2016). Testing the magnetar model via a late-time radio observations of two macronova candidates. ApJ 819, L22. doi:10.3847/2041-8205/819/2/L22
Hotokezaka, K., Kiuchi, K., Shibata, M., Nakar, E., and Piran, T. (2018). Synchrotron radiation from the fast tail of dynamical ejecta of neutron star mergers. ApJ 867, 95. doi:10.3847/1538-4357/aadf92
Hovatta, T., Lindfors, E., Kiehlmann, S., Max-Moerbeck, W., Hodges, M., Liodakis, I., et al. (2021). Association of IceCube neutrinos with radio sources observed at owens valley and metsähovi radio observatories. A&A 650, A83. doi:10.1051/0004-6361/202039481
IceCube Collaboration Aartsen, M. G., Abbasi, R., Abdou, Y., Ackermann, M., Adams, J., Aguilar, J. A., et al. (2013). Evidence for high-energy extraterrestrial neutrinos at the IceCube detector. Science 342, 1242856. doi:10.1126/science.1242856
IceCube Collaboration Aartsen, M. G., Ackermann, M., Adams, J., Aguilar, J. A., Ahlers, M., Ahrens, M., et al. (2018a). Multimessenger observations of a flaring blazar coincident with high-energy neutrino IceCube-170922A. Science 361, eaat1378. doi:10.1126/science.aat1378
IceCube Collaboration Aartsen, M. G., Ackermann, M., Adams, J., Aguilar, J. A., Ahlers, M., Ahrens, M., et al. (2018b). Neutrino emission from the direction of the blazar TXS 0506+056 prior to the IceCube-170922A alert. Science 361, 147–151. doi:10.1126/science.aat2890
IceCube Collaboration Abbasi, R., Ackermann, M., Adams, J., Aguilar, J. A., Ahlers, M., Ahrens, M., et al. (2022). Evidence for neutrino emission from the nearby active galaxy NGC 1068. Science 378, 538–543. doi:10.1126/science.abg3395
IceCube Collaboration Abbasi, R., Ackermann, M., Adams, J., Agarwalla, S. K., Aguilar, J. A., Ahlers, M., et al. (2023) Search for 10–1,000 GeV neutrinos from gamma ray bursts with IceCube. arXiv e-prints, arXiv:2312.11515. doi:10.48550/arXiv.2312.11515
Im, M., Yoon, Y., Lee, S.-K. J., Lee, H. M., Kim, J., Lee, C.-U., et al. (2017). Distance and properties of NGC 4993 as the host galaxy of the gravitational-wave source GW170817. ApJ 849, L16. doi:10.3847/2041-8213/aa9367
Kasliwal, M. M., Nakar, E., Singer, L. P., Kaplan, D. L., Cook, D. O., Van Sistine, A., et al. (2017). Illuminating gravitational waves: a concordant picture of photons from a neutron star merger. Science 358, 1559–1565. doi:10.1126/science.aap9455
Kathirgamaraju, A., Giannios, D., and Beniamini, P. (2019). Observable features of GW170817 kilonova afterglow. MNRAS 487, 3914–3921. doi:10.1093/mnras/stz1564
Katz, M. L., Kelley, L. Z., Dosopoulou, F., Berry, S., Blecha, L., and Larson, S. L. (2020). Probing massive black hole binary populations with LISA. MNRAS 491, 2301–2317. doi:10.1093/mnras/stz3102
Kimura, S. S., Murase, K., and Mészáros, P. (2021). Soft gamma rays from low accreting supermassive black holes and connection to energetic neutrinos. Nat. Commun. 12, 5615. doi:10.1038/s41467-021-25111-7
Kreter, M., Kadler, M., Krauß, F., Mannheim, K., Buson, S., Ojha, R., et al. (2020). On the detection potential of blazar flares for current neutrino telescopes. ApJ 902, 133. doi:10.3847/1538-4357/abb5b1
Lazzati, D., Perna, R., Morsony, B. J., Lopez-Camara, D., Cantiello, M., Ciolfi, R., et al. (2018). Late time afterglow observations reveal a collimated relativistic jet in the ejecta of the binary neutron star merger GW170817. Phys. Rev. Lett. 120, 241103. doi:10.1103/PhysRevLett.120.241103
Levan, A. J., Lyman, J. D., Tanvir, N. R., Hjorth, J., Mandel, I., Stanway, E. R., et al. (2017). The environment of the binary neutron star merger GW170817. ApJ 848, L28. doi:10.3847/2041-8213/aa905f
LIGO Scientific Collaboration Aasi, J., Abbott, B. P., Abbott, R., Abbott, T., Abernathy, M. R., Ackley, K., et al. (2015). Advanced LIGO. Class. Quantum Gravity 32, 074001. doi:10.1088/0264-9381/32/7/074001
Liu, L.-D., Gao, H., and Zhang, B. (2020). Constraining the long-lived magnetar remnants in short gamma-ray bursts from late-time radio observations. ApJ 890, 102. doi:10.3847/1538-4357/ab6b24
Liu, T., Cohen, T., McGrath, C., Demorest, P. B., and Vigeland, S. J. (2023). Multi-messenger approaches to supermassive black hole binary detection and parameter estimation. II. Optimal strategies for a pulsar timing array. ApJ 945, 78. doi:10.3847/1538-4357/acb492
Lucarelli, F., Oganesyan, G., Montaruli, T., Branchesi, M., Mei, A., Ronchini, S., et al. (2023). Neutrino search from γ-ray bursts during the prompt and X-ray afterglow phases using 10 years of IceCube public data. A&A 672, A102. doi:10.1051/0004-6361/202244815
Makhathini, S., Mooley, K. P., Brightman, M., Hotokezaka, K., Nayana, A. J., Intema, H. T., et al. (2021). The panchromatic afterglow of GW170817: the full uniform data set, modeling, comparison with previous results, and implications. ApJ 922, 154. doi:10.3847/1538-4357/ac1ffc
Mangiagli, A., Caprini, C., Volonteri, M., Marsat, S., Vergani, S., Tamanini, N., et al. (2022). Massive black hole binaries in LISA: multimessenger prospects and electromagnetic counterparts. Phys. Rev. D. 106, 103017. doi:10.1103/PhysRevD.106.103017
Margutti, R., Alexander, K. D., Xie, X., Sironi, L., Metzger, B. D., Kathirgamaraju, A., et al. (2018). The binary neutron star event LIGO/Virgo GW170817 160 Days after merger: synchrotron emission across the electromagnetic spectrum. ApJ 856, L18. doi:10.3847/2041-8213/aab2ad
Margutti, R., Berger, E., Fong, W., Guidorzi, C., Alexander, K. D., Metzger, B. D., et al. (2017). The electromagnetic counterpart of the binary neutron star merger LIGO/Virgo GW170817. V. Rising X-ray emission from an off-axis jet. ApJ 848, L20. doi:10.3847/2041-8213/aa9057
Matsui, R., Kimura, S. S., Toma, K., and Murase, K. (2023). High-energy neutrino emission associated with gravitational-wave signals: effects of cocoon photons and constraints on late-time emission. ApJ 950, 190. doi:10.3847/1538-4357/acd004
McDonough, K., Hughes, K., Smith, D., and Vieregg, A. G. (2023) A search for AGN sources of the IceCube diffuse neutrino flux. arXiv e-prints, arXiv:2307.04194. doi:10.48550/arXiv.2307.04194
Metzger, B. D., and Bower, G. C. (2014). Constraints on long-lived remnants of neutron star binary mergers from late-time radio observations of short duration gamma-ray bursts. MNRAS 437, 1821–1827. doi:10.1093/mnras/stt2010
Mooley, K. P., Deller, A. T., Gottlieb, O., Nakar, E., Hallinan, G., Bourke, S., et al. (2018a). Superluminal motion of a relativistic jet in the neutron-star merger GW170817. Nature 561, 355–359. doi:10.1038/s41586-018-0486-3
Mooley, K. P., Nakar, E., Hotokezaka, K., Hallinan, G., Corsi, A., Frail, D. A., et al. (2018b). A mildly relativistic wide-angle outflow in the neutron-star merger event GW170817. Nature 554, 207–210. doi:10.1038/nature25452
Mukhopadhyay, M., Kimura, S. S., and Murase, K. (2024). Gravitational wave triggered searches for high-energy neutrinos from binary neutron star mergers: prospects for next generation detectors. Phys. Rev. D. 109, 043053. doi:10.1103/PhysRevD.109.043053
Murase, K. (2015). On the origin of high-energy cosmic neutrinos. Am. Inst. Phys. Conf. Ser. 1666, 040006. doi:10.1063/1.4915555
Murase, K., Kimura, S. S., Zhang, B. T., Oikonomou, F., and Petropoulou, M. (2020). High-energy neutrino and gamma-ray emission from tidal disruption events. ApJ 902, 108. doi:10.3847/1538-4357/abb3c0
Murase, K., and Stecker, F. W. (2023). High-energy neutrinos from active galactic nuclei. Neutrino Phys. Astrophysics 2, 483–540. doi:10.1142/9789811282645_0010
Murphy, E., Bolatto, A., Chatterjee, S., Casey, M. C., Chomiuk, L., Dale, D., et al. (2018a). The ngvla science case and associated science requirements. Available at: https://ngvla.nrao.edu/system/media_files/binaries/130/original/ngVLA-Project-Summary_Jan2019.pdf?1548895473.
Murphy, E. J. (2022). The role of radio observations in studies of infrared-bright galaxies: prospects for a next-generation very large array. Universe 8, 329. doi:10.3390/universe8060329
Murphy, E. J., Bolatto, A., Chatterjee, S., Casey, C. M., Chomiuk, L., Dale, D., et al. (2018b). The ngVLA science case and associated science requirements. Astronomical Soc. Pac. Conf. Ser. 3, 517. doi:10.48550/arXiv.1810.07524
Nakar, E., and Piran, T. (2011). Detectable radio flares following gravitational waves from mergers of binary neutron stars. Nature 478, 82–84. doi:10.1038/nature10365
National Academies of Sciences Engineering and Medicine (2021) Pathways to discovery in astronomy and Astrophysics for the 2020s. United States: National Academies Press. doi:10.17226/26141
Nedora, V., Dietrich, T., and Shibata, M. (2023). Synthetic radio images of structured GRB and kilonova afterglows. MNRAS 524, 5514–5523. doi:10.1093/mnras/stad2128
Nedora, V., Radice, D., Bernuzzi, S., Perego, A., Daszuta, B., Endrizzi, A., et al. (2021). Dynamical ejecta synchrotron emission as a possible contributor to the changing behaviour of GRB170817A afterglow. MNRAS 506, 5908–5915. doi:10.1093/mnras/stab2004
Nicholl, M., Berger, E., Kasen, D., Metzger, B. D., Elias, J., Briceño, C., et al. (2017). The electromagnetic counterpart of the binary neutron star merger LIGO/Virgo GW170817. III. Optical and UV spectra of a blue kilonova from fast polar ejecta. ApJ 848, L18. doi:10.3847/2041-8213/aa9029
Palmese, A., Hartley, W., Tarsitano, F., Conselice, C., Lahav, O., Allam, S., et al. (2017). Evidence for dynamically driven formation of the GW170817 neutron star binary in NGC 4993. ApJ 849, L34. doi:10.3847/2041-8213/aa9660
Pan, Y. C., Kilpatrick, C. D., Simon, J. D., Xhakaj, E., Boutsia, K., Coulter, D. A., et al. (2017). The old host-galaxy environment of SSS17a, the first electromagnetic counterpart to a gravitational-wave source. ApJ 848, L30. doi:10.3847/2041-8213/aa9116
Pian, E., D’Avanzo, P., Benetti, S., Branchesi, M., Brocato, E., Campana, S., et al. (2017). Spectroscopic identification of r-process nucleosynthesis in a double neutron-star merger. Nature 551, 67–70. doi:10.1038/nature24298
Plavin, A., Kovalev, Y. Y., Kovalev, Y. A., and Troitsky, S. (2020). Observational evidence for the origin of high-energy neutrinos in parsec-scale nuclei of radio-bright active galaxies. ApJ 894, 101. doi:10.3847/1538-4357/ab86bd
Plavin, A. V., Kovalev, Y. Y., Kovalev, Y. A., and Troitsky, S. V. (2021). Directional association of TeV to PeV astrophysical neutrinos with radio blazars. ApJ 908, 157. doi:10.3847/1538-4357/abceb8
Ronchini, S., Branchesi, M., Oganesyan, G., Banerjee, B., Dupletsa, U., Ghirlanda, G., et al. (2022). Perspectives for multimessenger astronomy with the next generation of gravitational-wave detectors and high-energy satellites. A&A 665, A97. doi:10.1051/0004-6361/202243705
Sadeh, G., Linder, N., and Waxman, E. (2024) Non-thermal emission from mildly relativistic dynamical ejecta of neutron star mergers: spectrum and sky image. arXiv e-prints, arXiv:2403.07047. doi:10.48550/arXiv.2403.07047
Schnittman, J. D. (2011). Electromagnetic counterparts to black hole mergers. Class. Quantum Gravity 28, 094021. doi:10.1088/0264-9381/28/9/094021
Selina, R. J., Murphy, E. J., McKinnon, M., Beasley, A., Butler, B., Carilli, C., et al. (2018). The ngVLA reference design. Astronomical Soc. Pac. Conf. Ser. 15, 517. doi:10.48550/arXiv.1810.08197
Senno, N., Murase, K., and Mészáros, P. (2016). Choked jets and low-luminosity gamma-ray bursts as hidden neutrino sources. Phys. Rev. D. 93, 083003. doi:10.1103/PhysRevD.93.083003
Senno, N., Murase, K., and Mészáros, P. (2017). High-energy neutrino flares from X-ray bright and dark tidal disruption events. ApJ 838, 3. doi:10.3847/1538-4357/aa6344
Senno, N., Murase, K., and Mészáros, P. (2018). Constraining high-energy neutrino emission from choked jets in stripped-envelope supernovae. J. Cosmol. Astropart. Phys. 2018, 025. doi:10.1088/1475-7516/2018/01/025
Smartt, S. J., Chen, T. W., Jerkstrand, A., Coughlin, M., Kankare, E., Sim, S. A., et al. (2017). A kilonova as the electromagnetic counterpart to a gravitational-wave source. Nature 551, 75–79. doi:10.1038/nature24303
Soares-Santos, M., Holz, D. E., Annis, J., Chornock, R., Herner, K., Berger, E., et al. (2017). The electromagnetic counterpart of the binary neutron star merger LIGO/Virgo GW170817. I. Discovery of the optical counterpart using the dark energy camera. ApJ 848, L16. doi:10.3847/2041-8213/aa9059
Stegmann, J., Zwick, L., Vermeulen, S. M., Antonini, F., and Mayer, L. (2023) Observing supermassive black holes with deci-Hz gravitational-wave detectors. arXiv e-prints, arXiv:2311.06335. doi:10.48550/arXiv.2311.06335
Stein, R., van Velzen, S., Kowalski, M., Franckowiak, A., Gezari, S., Miller-Jones, J. C. A., et al. (2021). A tidal disruption event coincident with a high-energy neutrino. Nat. Astron. 5, 510–518. doi:10.1038/s41550-020-01295-8
Suray, A., and Troitsky, S. (2024). Neutrino flares of radio blazars observed from TeV to PeV. MNRAS 527, L26–L31. doi:10.1093/mnrasl/slad136
Tanvir, N. R., Levan, A. J., González-Fernández, C., Korobkin, O., Mandel, I., Rosswog, S., et al. (2017). The emergence of a lanthanide-rich kilonova following the merger of two neutron stars. ApJ 848, L27. doi:10.3847/2041-8213/aa90b6
Teboul, O., and Shaviv, N. J. (2021). Impact of the ISM magnetic field on GRB afterglow polarization. MNRAS 507, 5340–5347. doi:10.1093/mnras/stab2491
Troja, E., Piro, L., van Eerten, H., Wollaeger, R. T., Im, M., Fox, O. D., et al. (2017). The X-ray counterpart to the gravitational-wave event GW170817. Nature 551, 71–74. doi:10.1038/nature24290
Valenti, S., Sand, D. J., Yang, S., Cappellaro, E., Tartaglia, L., Corsi, A., et al. (2017). The discovery of the electromagnetic counterpart of GW170817: kilonova at 2017gfo/DLT17ck. ApJ 848, L24. doi:10.3847/2041-8213/aa8edf
Villar, V. A., Guillochon, J., Berger, E., Metzger, B. D., Cowperthwaite, P. S., Nicholl, M., et al. (2017). The combined ultraviolet, optical, and near-infrared light curves of the kilonova associated with the binary neutron star merger GW170817: unified data set, analytic models, and physical implications. ApJ 851, L21. doi:10.3847/2041-8213/aa9c84
Volonteri, M., Habouzit, M., and Colpi, M. (2021). The origins of massive black holes. Nat. Rev. Phys. 3, 732–743. doi:10.1038/s42254-021-00364-9
Keywords: gravitational waves, high energy neutrino astrophysics, radio astronomy, radio array, black holes, supermassive binary black holes, neutron star binaries, AGN -active galactic nucleus
Citation: Corsi A, Eddins A, Lazio TJW, Murphy EJ and Osten RA (2024) Radio transients from compact objects across the mass spectrum in the era of multi-messenger astronomy. Front. Astron. Space Sci. 11:1401792. doi: 10.3389/fspas.2024.1401792
Received: 15 March 2024; Accepted: 29 May 2024;
Published: 01 July 2024.
Edited by:
Francesca M. Civano, Goddard SPace Glight Center, NASA, United StatesReviewed by:
Francesco Carotenuto, University of Oxford, United KingdomCopyright © 2024 Corsi, Eddins, Lazio, Murphy and Osten. This is an open-access article distributed under the terms of the Creative Commons Attribution License (CC BY). The use, distribution or reproduction in other forums is permitted, provided the original author(s) and the copyright owner(s) are credited and that the original publication in this journal is cited, in accordance with accepted academic practice. No use, distribution or reproduction is permitted which does not comply with these terms.
*Correspondence: Alessandra Corsi, YWNvcnNpMkBqaC5lZHU=
†Present address: Alessandra Corsi, Department of Physics and Astronomy, Johns Hopkins University, Baltimore, MD, United States