- National Institute for Astrophysics, Astrophysical Observatory of Torino, Pino Torinese, Italy
SQUARE2 is the acronym for Spacecraft in QUAdrature for solaR Exploration, and is a mission concept for multi-instrumental two-point observations of the Sun and its environment. It stems from the need to have two probes that are systematically in orbital configurations of interest, such as quadratures or radial alignments, in order to successfully address some science topics that joint measurements by different spacecraft, not specifically designed though to operate in synergy, can only partially solve. This perspective paper describes the mission profile that SQUARE2 should have in order to achieve a better understanding of how the Sun creates and controls the heliosphere. Specifically, the combined use of remote-sensing and in-situ instrumentation aboard the twin SQUARE2 probes would allow the connection of the locally sampled solar-wind plasma flow with its coronal drivers and a proper investigation of solar wind evolution, dynamics, and transient events in the inner heliosphere. The potential impact of SQUARE2 and the science topics covered by such a solar mission are here discussed.
1 Introduction
Some of the most important scientific advances in the investigation of the Sun and its region of influence, the heliosphere, came from coordinated multi-spacecraft, multi-instrument observations. The study of the evolution of the dynamical/energetic properties of Coronal Mass Eruptions (CMEs, e.g., Nakwacki et al., 2011; Telloni et al., 2020) or solar wind turbulence (e.g., D’Amicis et al., 2010; Alberti et al., 2022), the investigation of the origin and propagation of the solar wind plasma (e.g., Poletto et al., 2002; Telloni et al., 2021a), the stereoscopic reconstructions of eruptive phenomena (such as prominences or CMEs, Gissot et al., 2008; Wood et al., 2009) or large-scale coronal structures (e.g., Aschwanden et al., 2008; Decraemer et al., 2019), the analysis of widespread solar energetic particle events in interplanetary space (e.g., Kollhoff et al., 2021; Mason et al., 2021), are just some of the many possible examples. Such investigations are performed by exploiting particular orbital configurations between two or more spacecraft. Radial alignments between the Sun and two different probes, when crossed by a same solar event (whether it is a transient structure, such as a CME, or the same solar-wind plasma parcel), represent the only way to study its evolution during expansion throughout heliosphere (e.g., Witasse et al., 2017; Telloni et al., 2021b). It is true that other approaches, based for example on the statistical study of many events observed by different spacecraft, at different distances from the Sun and at different times, provide information, e.g., on how the morphology of CMEs varies with distance from the Sun (e.g., Janvier et al., 2019) or the fast solar wind becomes more and more turbulent as it expands into interplanetary space (e.g., Telloni et al., 2015), but these are far from being satisfactory, as they provide just average information, which does not take into account, for example, the different solar cycle (and, therefore, the different background conditions) in which the observations are performed. On the other hand, during quadrature configurations, obtained when the angular separation between the two spacecraft is 90° with the Sun at the apex of the angle, one of the two probes remotely observes the coronal/heliospheric plasma that lately impinges on the second one: therefore, this orbital geometry allows the study of the magnetic connection of the plasma observed in situ to its solar source (Telloni et al., 2021a). The conjunction between two or more spacecraft finally allows local two-point studies, thus providing three-dimensional information of solar events, otherwise not feasible with single-spacecraft measurements (e.g., Davies et al., 2021).
Synergetic studies, based on joint remote-sensing and in-situ instrument observations from different solar observatories, represent the cutting edge of the potential of space missions currently probing the Sun and the heliosphere. This is evidenced by the growing number of international Working Groups and International Space Science Institute (ISSI) proposed teams established to coordinate activities between traditionally disjoint space plasma communities and to maximize the scientific return of spacecraft configurations in the inner heliosphere. Nevertheless, such a multi-spacecraft approach remains one of the largest unexplored territories in the field of heliophysics. Its limited blossoming undoubtedly depends on the fact that the solar missions launched so far comprise only a single probe (excluding some magnetospheric missions, such as Cluster (Escoubet et al., 2001) or Magnetospheric MultiScale (MMS, Burch et al., 2016), consisting of four-spacecraft constellation in a tetrahedral flight formation and only sporadically entering the solar wind). Exception is the Solar TErrestrial RElations Observatory (STEREO, Kaiser et al., 2008), a solar mission consisting of two nearly identical probes, carrying both in-situ and remote-sensing instrumentation, placed in orbit around the Sun at different positions along Earth’s orbit, with the ahead spacecraft (STEREO-A) preceding Earth and the behind spacecraft (STEREO-B) following it. This enabled the first ever stereoscopic view of the Sun and its large-scale manifestations (such as CMEs, see the review by Thernisien et al., 2011, and references therein).
Most of the current understanding of how the Sun creates and controls the heliosphere comes from near-Earth space observatories, resulting in a limited view of the Sun’s environment. The most recent heliospheric missions, namely BepiColombo (BC, Benkhoff et al., 2010), Parker Solar Probe (PSP, Fox et al., 2016), and Solar Orbiter (SO, Müller et al., 2020), which are probing in situ and remotely the inner heliosphere along complementary trajectories, has partially overcome this gap, while providing exciting and unprecedented opportunities for coordinated studies, in conjunction with the current near-Earth orbiting fleet, of the complex heliospheric dynamics and structures. Preliminary investigations were carried out by Velli et al. (2020) and Hadid et al. (2021) to identify the useful spacecraft configurations for such synergistic studies and highlight their potential for discovery. Since the first studies relying on these special orbital configurations (Jannet et al., 2021; Telloni et al., 2021a,b; Davies et al., 2021; Weiss et al., 2021; Musset et al., 2021; Möstl et al., 2022; Alberti et al., 2022; Réville et al., 2022), it became immediately clear that multi-point, multi-instrument observations by BC, PSP, and SO represent an exceptional added value in order to successfully address all the scientific goals the three heliospheric missions aim at. Nevertheless, the BC, PSP, and SO space missions, specifically their instrumentation payload and orbits, are not designed to operate in synergy. It follows that the potential science return of the interconnected science is not fully exploited. For this purpose it would be useful an “ad hoc” solar mission consisting of two (or more) twin probes placed in different orbits around the Sun, but optimized to maximize the number of orbital geometries, i.e., quadratures, radial alignments, conjunctions and so on.
SQUARE2 is a twin-probe solar mission concept specifically tailored for multi-spacecraft studies of solar interconnected processes in the heliosphere. It stands for Spacecraft in QUAdrature for solaR Exploration, and the superscript “2” both indicates that the mission consists of twin probes and recalls the concept of “squared”, namely that one of the goals of the mission is to maximize the quadrature configurations between the two probes. This perspective paper is devoted to describing SQUARE2, i.e., what its orbital features should be (§2), what scientific questions would be addressed (§3), and a concluding outlook (§4).
2 SQUARE2 Orbital Geometries
This section deals with the orbital characteristics that SQUARE2 should have in order to maximize the number of time windows in which the two probes are in particular geometries useful for multi-spacecraft studies. It should be immediately stressed that the orbits shown in the following are unrealistic examples helpful only for the purpose of presenting what should be the spacecraft configurations to be maximized during the SQUARE2 solar mission. The actual mission design, the different and complementary trajectories of the two probes eventually combined with multiple gravitational assists (at Earth, Venus, or Mercury), are the subject of the mission profile development that is obviously beyond the scope of this paper, which instead aims only to present the potential richness of such a solar mission. It is only worth noting here that the two probes are expected to have very different and complementary orbits, with different eccentricities, periods of revolution, and distances from the Sun (provided that the number of useful geometries is maximized), eventually including periods of quasi-co-rotation between the two spacecraft. Both probes would be then equipped with both remote-sensing (disk imager, magnetograph, coronagraph, spectrometer, heliospheric imager) and in-situ (plasma sensors, magnetometers, particle detector, radio antenna) instruments in order to address all the science objectives of SQUARE2. To satisfy telemetry requirements, remote-sensing instruments would observe only during relevant orbital configurations, while in-situ sensors would operate continuously.
Figure 1 displays four interesting orbital geometries between the two SQUARE2 twin probes s/c #1 and #2 (in red and blue, respectively) orbiting along different trajectories (color-coded dotted lines) around the Sun (yellow star).
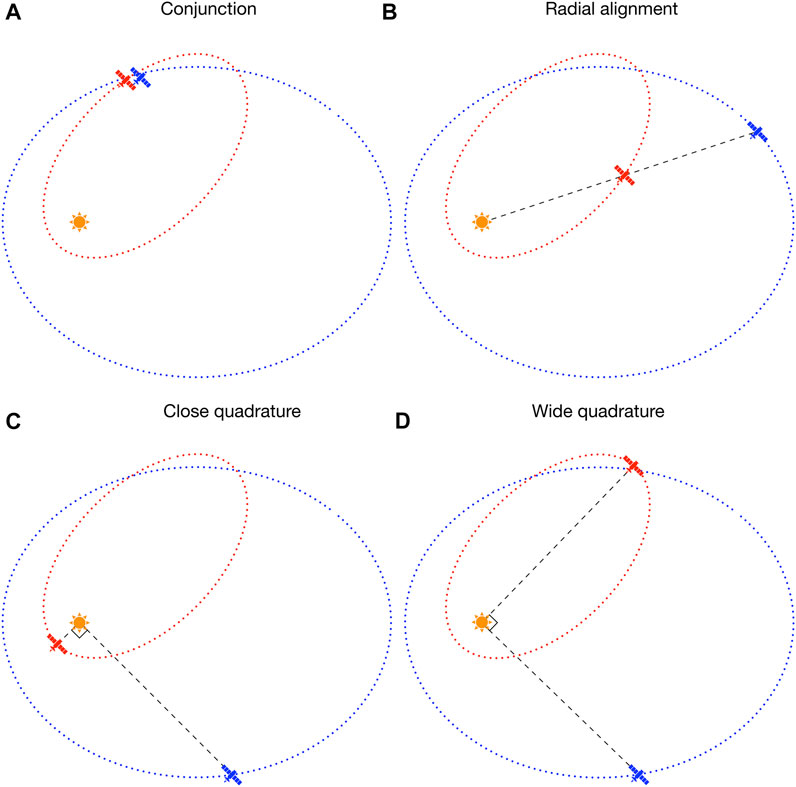
FIGURE 1. Sketches of particular geometrical configurations between SQUARE2 s/c #1 (red) and s/c #2 (blue) while orbiting around the Sun (yellow star) along their relative trajectories (color-coded dotted lines): spacecraft conjunction (A), radial alignment (B), close (C) and wide (D) quadratures. The s/c—Sun lines, during alignments and quadratures, are marked as dashed lines.
Spacecraft conjunctions (Figure 1A) occur when the s/c #1—s/c #2 longitudinal and radial separation is very small, and enable two-point in-situ investigations of the evolving 3D properties of the solar wind turbulence or transient (if any). Indeed, probing the same region of space at two distinct points and at different times would allow scientists to overcome the spatiotemporal ambiguity intrinsic to any single-spacecraft measurement, thus disentangling temporal from spatial fluctuations.
When s/c #1 and s/c #2 are radially aligned (i.e., their longitudinal separation is very small, even though orbiting at different distances from the Sun, Figure 1B), the evolution of solar wind properties and turbulence, as well as of the heliospheric counterpart of CMEs, while propagating into interplanetary space, can be studied. Different radial alignments, with the probes having different radial separations, over the whole course of the SQUARE2 mission would allow extensive analysis of the evolution of the expanding solar plasma fairly uniformly throughout the inner heliosphere.
Unlike the previous orbital configurations, during close or wide spacecraft quadratures (Figures 1C,D, respectively), occurring when the s/c #1—Sun—s/c #2 angle is 90°, remote-sensing observations of the Sun and its atmosphere play a crucial role. Indeed, quadratures enable studies on the magnetic connection of solar wind plasma measured locally by in-situ instrumentation to its solar sources observed remotely in coronagraphic images. In this context, remote-sensing observations from the first spacecraft gather information on the large-scale configuration of the coronal drivers of the plasma flows later crossing the second spacecraft, which measures in situ its physical properties. However, all quadratures are not equal, and the scientific targets that can be achieved depend greatly on the relative distance of the two probes from the Sun. Solar-wind connectivity can indeed be successfully addressed only in the case of a close quadrature (Figure 1C), with one of the two probes observing the Sun from afar and the second locally sampling the wind plasma very close to the Sun (which ensures that the plasma has not been significantly reprocessed during its propagation from the Sun to the spacecraft, i.e., it is still pristine). When the observation points of both probes in quadrature are very far from the Sun (Figure 1D), this kind of investigation is in fact (at least partially) prevented, and other (and complementary with respect to close quadratures) science objectives are enabled. For instance, observations of the solar corona from two (widely separated) vantage points would provide information about the 3D structure and dynamics of the large-scale solar atmosphere. Furthermore, local measurements of interplanetary CMEs by in-quadrature spacecraft at small radial separations but larger longitudinal separations (as in Figure 1D) shed light on their 3D structure.
In addition, if the two probes experienced, when in particular orbital configurations, periods of quasi-co-rotation with the Sun and/or each other, this would provide interesting opportunities to lengthen the periods of connection with solar sources, thus allowing the possibility to answer long-standing question such as the origin of the slow solar wind, to distinguish between temporal and spatial scales of locally sampled small-scale variations (and, in turn, between turbulence-related fluctuations and flux-tube-like structures), and to monitor the evolution of CMEs and the associated post-CME current sheets. Finally, noteworthy is that, being different from each other, the various orbits should be dedicated to specific science topics, as discussed in the following section.
3 Major Science Topics Advanced by SQUARE2
SQUARE2 could potentially advance the current understanding of the Sun—heliosphere coupling, by providing an unprecedented large set of useful orbital configurations for enhancing the study of the 3D structure of the heliosphere, the origin of the solar wind, transient events, and, most importantly, their connection to the coronal drivers. Specifically, the SQUARE2 systematic two-point, multi-instrument observations would be used to address, mainly yet not exclusively, the following science topics and their interplay.
Solar wind origin and propagation By simultaneously measuring the solar atmosphere, from the photosphere to the corona, remotely and the solar wind plasma and magnetic field in situ during systematic s/c #1—s/c #2 quadratures, SQUARE2 would efficiently link the small-scale properties of the plasma flow with the large-scale structures of solar drivers at the Sun (Telloni et al., 2021a). This would provide a plethora of observations to potentially represent a breakthrough in the investigation of the origin of the slow solar wind, which is still matter of strong debate (Abbo et al., 2016). In addition, joint remote-sensing and in-situ observations of coronal flows would provide insight into the link and interplay between macrophysics and microphysics in the processes underlying the evolution of the solar wind, and a better understanding of how stream-stream interactions reprocess the plasma during its expansion. Radial alignments would be of critical importance in this context, representing in fact the only way to satisfactorily study the propagation of solar wind from the very inner heliosphere to Earth’s orbit and beyond, and its interaction with the surrounding environment. Finally, combined observations at both coronal and heliospheric heights during quadratures and/or alignments would contribute significantly to solving the question of how the plasma is heated and accelerated as it escapes from the outer layers of the solar atmosphere. Comparison of SQUARE2 results of outflow velocity and plasma density of accelerating coronal flows with currently existing solar wind models (see the exhaustive review by Zank et al., 2021, and reference therein) would represent a significant step forward in ascertaining what processes regulate the heating and acceleration of coronal plasma.
Turbulence, waves, kinetic physics in the solar wind The investigation of the radial evolution of turbulence, waves and kinetic phenomena in the solar wind is primarily based on large sets of measurements belonging to different plasma streams, acquired at different times and distances from the Sun (e.g., Bruno et al., 2014; Alberti et al., 2020; Chen et al., 2020), and therefore inevitably suffers from the natural inhomogeneity and non-stationarity of the solar wind and its solar drivers, thus yielding results at least partially biased by the different solar conditions pertinent to the different observations. It turns out that the only really accurate way to learn about the evolution of turbulence, waves and heating is to rely on the same solar-wind plasma parcel observed at different heliocentric distances during radial lineups between two or more space vehicles (e.g., D’Amicis et al., 2010; Bruno and Telloni, 2015; Telloni et al., 2021b). In this context, SQUARE2 would provide a major improvement in the availability of instances for exploring how turbulence/waves of the same solar flow evolve while expanding in the inner heliosphere, as it would maximize the number of radial alignments between the twin probes comprising the space mission. By comparing remote observations of the solar drivers and in-situ measurements of the plasma and magnetic field fluctuations, SQUARE2 would help address the origin of plasma turbulence, diagnose wave-particle interactions, and advance understanding of the role of turbulence/instabilities/kinetic processes in heating the solar wind plasma. Finally, SQUARE2’s observations would be of critical importance in distinguishing between competing theories of the generation, transport and dissipation of the magnetohydrodynamic (MHD) turbulence (Zank et al., 2021).
Solar wind transient events The investigation of the solar-wind transient perturbations, such as interplanetary counterpart of CMEs, would also benefit greatly from the multi-point observations provided by SQUARE2. The availability of systematic measurements in the corona and the very inner heliosphere, i.e., the region where the transient structures evolve more rapidly, would indeed ensure to gain insights on a wide variety of their properties at both large and small scales. Specifically, wide s/c #1—s/c #2 quadratures would allow studies of the global morphology, i.e., the 3D structure, of erupting phenomena, and their propagation in the extended corona, during the initial acceleration phases. Should and when the interplanetary CME hit the two SQUARE2 probes, widely separate in longitude but at approximately the same heliocentric distance, this would provide information on the extent and/or distortion of the CME structure. Relying on close quadratures, on the other hand, it would be possible to assess the local properties of the still fast-evolving CMEs. However, the maximum scientific return would be obtained during the s/c #1—s/c #2 radial alignments. Indeed, when the two probes are lined up, the radial evolution of the CME MHD properties, such as the degree of twisting of the embedded flux rope and its energetic budget (Telloni et al., 2020) or the turbulence and plasma heating development (Sorriso-Valvo et al., 2021), as well as the local dynamical interaction with the ambient solar wind, could be estimated. The joint operation of the SQUARE2 twin spacecraft over a wide range of distances from the Sun would thus provide a powerful analysis platform for studying the interplay between the various physical processes underlying the onset, acceleration and propagation/evolution of the most important manifestation of solar activity.
4 Concluding Remarks
The launch in just 3 years, from 2018 to 2020, of the three fantastic inner heliospheric missions BepiColombo, Parker Solar Probe, and Solar Orbiter has opened a new era in the exploration of the inner heliosphere and its complex dynamics, while showing the potential of the multi-spacecraft approach in solar physics studies. Yet, it also showed the need for a space mission comprising multiple twin probes and specifically dedicated to multi-point, multi-instrument observations of the Sun and its environment. SQUARE2, Spacecraft in QUAdrature for solaR Exploration, is a solar mission concept specifically conceived to address this need and perform synergetic observations of the Sun and heliosphere, in order to fully capture the temporal and spatial dynamical evolution of the solar wind and transient events, and connect them to their coronal sources. Its mission profile is precisely designed to maximize the number of useful orbital configurations (such as conjunctions, radial alignments, and quadrature). This paper has briefly summarized the SQUARE2 characteristics and orbital geometries, as well as the advantages and new insights of such a space mission. In particular, it has appeared evident that systematic coordinated observations of several in-situ and remote-sensing instruments would enable breakthrough science in a way no other solar mission could, allowing the scientific community to successfully address still unsolved and profoundly interconnected problems, such as the origin, acceleration and propagation of the coronal plasma flows, the spatiotemporal evolution of turbulence, waves, and kinetic processes, and dynamical interaction of transient events with the surrounding solar wind throughout interplanetary space.
Data Availability Statement
All relevant data to the present work are included in the paper, further inquiries can be addressed to the corresponding author.
Author Contributions
The author confirms being the sole contributor to this paper, having conceived and written it, and approved it for publication.
Funding
The author was partially supported by the Italian Space Agency (ASI) under contract 2018-30-HH.0.
Conflict of Interest
The author declares that the research was conducted in the absence of any commercial or financial relationships that could be construed as a potential conflict of interest.
Publisher’s Note
All claims expressed in this article are solely those of the authors and do not necessarily represent those of their affiliated organizations, or those of the publisher, the editors and the reviewers. Any product that may be evaluated in this article, or claim that may be made by its manufacturer, is not guaranteed or endorsed by the publisher.
References
Abbo, L., Ofman, L., Antiochos, S. K., Hansteen, V. H., Harra, L., Ko, Y.-K., et al. (2016). Slow Solar Wind: Observations and Modeling. Space Sci. Rev. 201, 55–108. doi:10.1007/s11214-016-0264-1
Alberti, T., Laurenza, M., Consolini, G., Milillo, A., Marcucci, M. F., Carbone, V., et al. (2020). On the Scaling Properties of Magnetic-Field Fluctuations through the Inner Heliosphere. Astrophys. J. 902, 84. doi:10.3847/1538-4357/abb3d2
Alberti, T., Milillo, A., Heyner, D., Hadid, L. Z., Auster, H.-U., Richter, I., et al. (2022). The “Singular” Behavior of the Solar Wind Scaling Features during Parker Solar Probe-BepiColombo Radial Alignment. Astrophys. J. 926, 174. doi:10.3847/1538-4357/ac478d
Aschwanden, M. J., Wülser, J.-P., Nitta, N. V., and Lemen, J. R. (2008). First Three-Dimensional Reconstructions of Coronal Loops with the STEREO A and B Spacecraft. I. Geometry. Astrophys. J. 679, 827–842. doi:10.1086/529542
Benkhoff, J., van Casteren, J., Hayakawa, H., Fujimoto, M., Laakso, H., Novara, M., et al. (2010). BepiColombo—Comprehensive Exploration of Mercury: Mission Overview and Science Goals. Planet. Space Sci. 58, 2–20. doi:10.1016/j.pss.2009.09.020
Bruno, R., Telloni, D., Primavera, L., Pietropaolo, E., D’Amicis, R., Sorriso-Valvo, L., et al. (2014). Radial Evolution of the Intermittency of Density Fluctuations in the Fast Solar Wind. Astrophys. J. 786, 53. doi:10.1088/0004-637X/786/1/53
Bruno, R., and Telloni, D. (2015). Spectral Analysis of Magnetic Fluctuations at Proton Scales from Fast to Slow Solar Wind. Astrophys. J. Lett. 811, L17. doi:10.1088/2041-8205/811/2/L17
Burch, J. L., Moore, T. E., Torbert, R. B., and Giles, B. L. (2016). Magnetospheric Multiscale Overview and Science Objectives. Space Sci. Rev. 199, 5–21. doi:10.1007/s11214-015-0164-9
Chen, C. H. K., Bale, S. D., Bonnell, J. W., Borovikov, D., Bowen, T. A., Burgess, D., et al. (2020). The Evolution and Role of Solar Wind Turbulence in the Inner Heliosphere. Astrophys. J. Suppl. Ser. 246, 53. doi:10.3847/1538-4365/ab60a3
D’Amicis, R., Bruno, R., Pallocchia, G., Bavassano, B., Telloni, D., Carbone, V., et al. (2010). Radial Evolution of Solar Wind Turbulence during Earth and Ulysses Alignment of 2007 August. Astrophys. J. 717, 474–480. doi:10.1088/0004-637X/717/1/474
Davies, E. E., Möstl, C., Owens, M. J., Weiss, A. J., Amerstorfer, T., Hinterreiter, J., et al. (2021). In Situ multi-spacecraft and Remote Imaging Observations of the First CME Detected by Solar Orbiter and BepiColombo. Astron. Astrophys. 656, A2. doi:10.1051/0004-6361/202040113
Decraemer, B., Zhukov, A. N., and Van Doorsselaere, T. (2019). Three-dimensional Density Structure of a Solar Coronal Streamer Observed by SOHO/LASCO and STEREO/COR2 in Quadrature. Astrophys. J. 883, 152. doi:10.3847/1538-4357/ab3b58
Escoubet, C. P., Fehringer, M., and Goldstein, M. (2001). Introduction the Cluster Mission. Ann. Geophys 19, 1197–1200. doi:10.5194/angeo-19-1197-2001
Fox, N. J., Velli, M. C., Bale, S. D., Decker, R., Driesman, A., Howard, R. A., et al. (2016). The Solar Probe Plus Mission: Humanity’s First Visit to Our Star. Space Sci. Rev. 204, 7–48. doi:10.1007/s11214-015-0211-6
Gissot, S. F., Hochedez, J. F., Chainais, P., and Antoine, J. P. (2008). 3D Reconstruction from SECCHI-EUVI Images Using an Optical-Flow Algorithm: Method Description and Observation of an Erupting Filament. Sol. Phys. 252, 397–408. doi:10.1007/s11207-008-9270-0
Hadid, L. Z., Génot, V., Aizawa, S., Milillo, A., Zender, J., Murakami, G., et al. (2021). BepiColombo’s Cruise Phase: Unique Opportunity for Synergistic Observations. Front. Astron. Space Sci. 8, 154. doi:10.3389/fspas.2021.718024
Jannet, G., Dudok de Wit, T., Krasnoselskikh, V., Kretzschmar, M., Fergeau, P., Bergerard-Timofeeva, M., et al. (2021). Measurement of Magnetic Field Fluctuations in the Parker Solar Probe and Solar Orbiter Missions. J. Geophys. Res. Space Phys. 126, e28543. doi:10.1029/2020JA028543
Janvier, M., Winslow, R. M., Good, S., Bonhomme, E., Démoulin, P., Dasso, S., et al. (2019). Generic Magnetic Field Intensity Profiles of Interplanetary Coronal Mass Ejections at Mercury, Venus, and Earth from Superposed Epoch Analyses. J. Geophys. Res. Space Phys. 124, 812–836. doi:10.1029/2018JA025949
Kaiser, M. L., Kucera, T. A., Davila, J. M., Cyr, St. O. C., Guhathakurta, M., and Christian, E. (2008). The STEREO Mission: An Introduction. Space Sci. Rev. 136, 5–16. doi:10.1007/s11214-007-9277-0
Kollhoff, A., Kouloumvakos, A., Lario, D., Dresing, N., Gómez-Herrero, R., Rodríguez-García, L., et al. (2021). The First Widespread Solar Energetic Particle Event Observed by Solar Orbiter on 2020 November 29. Astron. Astrophys. 656, A20. doi:10.1051/0004-6361/202140937
Mason, G. M., Cohen, C. M. S., Ho, G. C., Mitchell, D. G., Allen, R. C., Hill, M. E., et al. (2021). Solar Energetic Particle Heavy Ion Properties in the Widespread Event of 2020 November 29. Astron. Astrophys. 656, L12. doi:10.1051/0004-6361/202141310
Möstl, C., Weiss, A. J., Reiss, M. A., Amerstorfer, T., Bailey, R. L., Hinterreiter, J., et al. (2022). Multipoint Interplanetary Coronal Mass Ejections Observed with Solar Orbiter, BepiColombo, Parker Solar Probe, Wind, and STEREO-A. Astrophys. J. Lett. 924, L6. doi:10.3847/2041-8213/ac42d0
Müller, D., Cyr, St. O. C., Zouganelis, I., Gilbert, H. R., Marsden, R., Nieves-Chinchilla, T., et al. (2020). The Solar Orbiter Mission. Sci. overviewAstron. Astrophys. 642, A1. doi:10.1051/0004-6361/202038467
Musset, S., Maksimovic, M., Kontar, E., Krupar, V., Chrysaphi, N., Bonnin, X., et al. (2021). Simulations of Radio-Wave Anisotropic Scattering to Interpret Type III Radio Burst Data from Solar Orbiter, Parker Solar Probe, STEREO, and Wind. Astron. Astrophys. 656, A34. doi:10.1051/0004-6361/202140998
Nakwacki, M. S., Dasso, S., Démoulin, P., Mandrini, C. H., and Gulisano, A. M. (2011). Dynamical Evolution of a Magnetic Cloud from the Sun to 5.4 AU. Astron. Astrophys. 535, A52. doi:10.1051/0004-6361/201015853
Poletto, G., Suess, S. T., Biesecker, D. A., Esser, R., Gloeckler, G., Ko, Y. K., et al. (2002). Low-latitude Solar Wind during the Fall 1998 SOHO-Ulysses Quadrature. J. Geophys. Res. Space Phys. 107, 1300. doi:10.1029/2001JA000275
Réville, V., Fargette, N., Rouillard, A. P., Lavraud, B., Velli, M., Strugarek, A., et al. (2022). Flux Rope and Dynamics of the Heliospheric Current Sheet. Study of the Parker Solar Probe and Solar Orbiter Conjunction of June 2020. Astron. Astrophys. 659, A110. doi:10.1051/0004-6361/202142381
Sorriso-Valvo, L., Yordanova, E., Dimmock, A. P., and Telloni, D. (2021). Turbulent Cascade and Energy Transfer Rate in a Solar Coronal Mass Ejection. Astrophys. J. Lett. 919, L30. doi:10.3847/2041-8213/ac26c5
Telloni, D., Andretta, V., Antonucci, E., Bemporad, A., Capuano, G. E., Fineschi, S., et al. (2021a). Exploring the Solar Wind from its Source on the Corona into the Inner Heliosphere during the First Solar Orbiter-Parker Solar Probe Quadrature. Astrophys. J. Lett. 920, L14. doi:10.3847/2041-8213/ac282f
Telloni, D., Bruno, R., and Trenchi, L. (2015). Radial Evolution of Spectral Characteristics of Magnetic Field Fluctuations at Proton Scales. Astrophys. J. 805, 46. doi:10.1088/0004-637X/805/1/46
Telloni, D., Sorriso-Valvo, L., Woodham, L. D., Panasenco, O., Velli, M., Carbone, F., et al. (2021b). Evolution of Solar Wind Turbulence from 0.1 to 1 au during the First Parker Solar Probe-Solar Orbiter Radial Alignment. Astrophys. J. Lett. 912, L21. doi:10.3847/2041-8213/abf7d1
Telloni, D., Zhao, L., Zank, G. P., Liang, H., Nakanotani, M., Adhikari, L., et al. (2020). Magnetohydrodynamic Turbulent Evolution of a Magnetic Cloud in the Outer Heliosphere. Astrophys. J. Lett. 905, L12. doi:10.3847/2041-8213/abcb03
Thernisien, A., Vourlidas, A., and Howard, R. A. (2011). CME Reconstruction: Pre-STEREO and STEREO Era. J. Atmos. Sol.-Terr. Phys. 73, 1156–1165. doi:10.1016/j.jastp.2010.10.019
Velli, M., Harra, L. K., Vourlidas, A., Schwadron, N., Panasenco, O., Liewer, P. C., et al. (2020). Understanding the Origins of the Heliosphere: Integrating Observations and Measurements from Parker Solar Probe, Solar Orbiter, and Other Space- and Ground-Based Observatories. Astron. Astrophys. 642, A4. doi:10.1051/0004-6361/202038245
Weiss, A. J., Möstl, C., Davies, E. E., Amerstorfer, T., Bauer, M., Hinterreiter, J., et al. (2021). Multi-point Analysis of Coronal Mass Ejection Flux Ropes Using Combined Data from Solar Orbiter, BepiColombo, and Wind. Astron. Astrophys. 656, A13. doi:10.1051/0004-6361/202140919
Witasse, O., Sánchez-Cano, B., Mays, M. L., Kajdič, P., Opgenoorth, H., Elliott, H. A., et al. (2017). Interplanetary coronal mass ejection observed at STEREO-A, Mars, comet 67P/Churyumov-Gerasimenko, Saturn, and New Horizons en route to Pluto: Comparison of its Forbush decreases at 1.4, 3.1, and 9.9 AU. J. Geophys. Res. Space Phys. 122, 7865–7890. doi:10.1002/2017JA023884
Wood, B. E., Howard, R. A., Thernisien, A., Plunkett, S. P., and Socker, D. G. (2009). Reconstructing the 3D Morphology of the 17 May 2008 CME. Sol. Phys. 259, 163–178. doi:10.1007/s11207-009-9391-0
Keywords: space probes, space vehicle instruments, orbits, the Sun, heliosphere, space plasmas, solar wind, solar coronal mass ejections
Citation: Telloni D (2022) SQUARE2: Spacecraft in QUAdrature for Solar Exploration—A Mission Concept for Synergetic Observations of the Sun and Heliosphere. Front. Astron. Space Sci. 9:923463. doi: 10.3389/fspas.2022.923463
Received: 19 April 2022; Accepted: 02 May 2022;
Published: 30 May 2022.
Edited by:
Alessandro Retino, UMR7648 Laboratoire de physique des plasmas, FranceReviewed by:
Feng Wang, Guangzhou University, ChinaCopyright © 2022 Telloni. This is an open-access article distributed under the terms of the Creative Commons Attribution License (CC BY). The use, distribution or reproduction in other forums is permitted, provided the original author(s) and the copyright owner(s) are credited and that the original publication in this journal is cited, in accordance with accepted academic practice. No use, distribution or reproduction is permitted which does not comply with these terms.
*Correspondence: D. Telloni, daniele.telloni@inaf.it