- 1Department of Earth, Ocean, and Atmospheric Sciences, University of British Columbia, Vancouver, BC, Canada
- 2Laboratory for Space Research, University of Hong Kong, Hong Kong SAR, China
During the late asymptotic giant branch (AGB) phase of stellar evolution, the element carbon is created through the triple-α nuclear reaction. This is followed by the synthesis of other heavy elements via neutron capture in the core and the dredge-up of these elements to the surface by convection. Simple molecules and solid-state minerals begin to form in the upper photosphere. These molecules and solids are ejected into the interstellar medium by a strong stellar wind. During the subsequent post-AGB evolution, complex organics with aromatic and aliphatic structures are synthesized in the circumstellar envelope. Planetary nebulae, formed by swept-up circumstellar material, are found to show strong spectral signatures of ions, atoms, molecules, and inorganic and organic solids. These ejected materials of planetary nebulae serve as primordial ingredients of formation of new stars and planetary systems. Stellar synthesized organic solids may survive their journeys through the interstellar medium and evidence for remnants of such organics can be found in our own Solar System. In this paper, we summarize the recent observations of circumstellar synthesis of molecules and solids and discuss the implications of these ejected circumstellar materials on the chemical enrichment of the Galaxy and planetary systems.
Introduction
Planetary nebulae were first identified as extended objects in the sky and were grouped together with stellar clusters, galaxies, diffuse nebulae, and supernova remnants in Charles Messier’s 1784 catalogue of nebulous objects. After the development of astronomical spectroscopy in the 19th century, planetary nebulae were recognized to be different from other nebulous objects in that they show strong atomic emission lines in their spectra. The presence of a hot star in the center of planetary nebula suggests that the nebula is illuminated by radiation from the central star (Hubble 1922). Ultraviolet radiation from the central star photoionizes the surrounding gaseous nebulae, and the recombination between electrons and hydrogen (H) and helium (He) led to the strong emission lines observed (Zanstra, 1827; Menzel 1926). Other strong emission lines in the nebulae are from collisionally excited lines of heavy elements. Together, these lines are responsible for the visible brightness of planetary nebulae.
Planetary nebulae are different from other emission nebulae (e.g., the Orion nebula) in that they show some degree of symmetry in their nebular morphology. The nebulae are the ejecta of the envelopes of their central stars, which are in the process of evolving from the red giant branch to the white dwarf stage of stellar evolution (Shklovsky 1956). The central stars of planetary nebulae are powered by H-shell burning on an electron-degenerate carbon-oxygen (C–O) core. Through a combination of nuclear burning and mass loss, the H envelope continues to thin, resulting in a gradual increase in the effective temperature of the central star while maintaining a constant luminosity determined by the mass of the core (Paczyński 1971). The discovery of extensive mass loss on the AGB phase prior to the planetary nebulae phase led to the realization that the well-defined shell-like morphology of the nebulae is the result of the interaction between the remnant of the AGB wind with a later-developed fast wind from the central star (Kwok et al., 1978).
Development in infrared and millimeter-wave astronomy in the 1970s show that planetary nebulae are also strong sources of emissions from molecules and solid-state materials, and the true extent of planetary nebulae encompasses more than just the optical nebulae. A large fraction of the energy output from planetary nebulae is in the form of infrared continuum radiation emitted by solid dust grains (Zhang and Kwok 1991). Mapping of the molecular distribution in planetary nebulae shows that planetary nebulae contain a large amount of neutral matter, and in some cases, neutral matter mass exceeds the mass observed in the ionized nebulae. We now recognize that planetary nebulae contain matter in the forms of ionized, neutral atomic, molecular, to solid-state, and emit radiation throughout the electromagnetic spectrum from radio to X-ray. A modern view of the origin and evolution of planetary nebulae is given by Kwok (2000).
Planetary Nebulae as Sources of Heavy Elements
Although nuclear burning can theoretically occur in the core of AGB stars through to the synthesis of iron (Fe), for most stars their core masses never grow large enough to ignite carbon (C) (under either electron-degenerate or electron-non-degenerate conditions) due to surface mass loss. For stars in the Galaxy with initial masses under 8 M⊙, the star will undergo H and He shell burning on the AGB, evolve through the planetary nebulae stage, and end as white dwarfs. Since this mass range covers >95% of all stars in the Galaxy, C is the last element synthesized by direct fusion (through the triple-α process) for most stars. Heavy elements such as Y, Zr, Ba, La, Ce, Pr, Nd, Sm, Eu are synthesized through neutron capture followed by beta decay.
Because of their strong emission-line spectrum, abundance of heavy elements can be determined from their recombination or collisionally excited lines. The elemental abundances deduced are useful checks on stellar nucleosynthesis models. A recent review on elemental abundance determination in planetary nebulae is given by Kwitter and Henry (2022).
Planetary Nebulae as Sources of Molecules
As a star evolves on the AGB, its H envelope expands, resulting in a size hundreds of times larger than the Sun. This is accompanied by an increasing luminosity to thousands of times that of the Sun, and a corresponding decreasing surface effective temperatures to as low as 3000 K. Such low atmospheric temperatures allow the formation of molecules in the photosphere. Beyond the common diatomic molecule CO, molecules such as C2, C3, and CN can form in stars that are C-rich (elemental abundance of C exceeding that of O). For O-rich stars (elemental abundance of O exceeding that of C), the surplus O atoms are tied into OH, H2O, SiO, and other oxides.
The development of millimeter-wave astronomy led to the detection of interstellar and circumstellar molecules through their rotational transitions. With infrared spectroscopy, the vibrational transitions of molecules can also be detected in absorption against the stellar photospheric or dust continuum. These molecules can be used to probe the physical conditions as well as kinematic structures of planetary nebulae.
Molecular Synthesis During the AGB Stage
A large variety of molecules including inorganics (e.g., CO, SiO, SiS, NH3, AlCl, etc.), organics (C2H2, CH4, H2CO, CH3CN, etc.), radicals (CN, C2H, etc.), molecular ions (HCO+), chains (e.g., HCN, HC3N, HC5N, etc.), and rings (C3H2) are detected in the circumstellar envelopes of AGB stars (Ziurys, 2006; Ziurys et al., 2016). The spatial distribution of these molecules in the circumstellar envelopes can be mapped by interferometric observations, showing that these molecules are synthesized in the stellar wind under very low density conditions. AGB stars are prolific molecular factories.
Molecules detected in the circumstellar envelopes of AGB stars include molecules involving heavy elements such as Si, Mg, Fe, Ti, and Al and detected molecules include SiH4, MgCN, FeCN, TiO2, and AlOH. The biological important element phosphorus (P) (Maciá-Barber, 2019) is detected in the forms of CP, PN, PO, HCP, CCP, PH3 (Agúndez et al., 2007; Agúndez et al., 2008; Halfen et al., 2008; Tenenbaum and Ziurys, 2008; Agúndez et al., 2014).
Molecular species detected in O- and C-rich AGB stars are also different. The proto-typical C-rich molecular source is IRC+10216 (CW Leo), where over 50 molecules have been detected (Ziurys 2006; McGuire 2022). The most common molecules in C-rich stars are CO, HCN, HC3N, CN, and CS. The number of molecules detected in O-rich stars is more limited, with OH, H2O, SiO being the most common. Recent surveys of the O-rich star VY CMa has expanded the list of molecules detected (Tenenbaum et al., 2010). The versatility of carbon chemistry is illustrated by the fact that only diatomic and triatomic compounds were observed in VY CMa, while species with four or more atoms are common in IRC+10216.
Molecular Synthesis During the Planetary Nebulae Stage
The largest molecule detected in planetary nebulae is fullerene (C60). Fullerene was discovered in the planetary nebula Tc-1 through its vibrational bands at 7.0, 8.5, 17.4 and 18.9 µm (Cami et al., 2010). Further detections of C60 are found in H-rich planetary nebulae (García-Hernández et al., 2010), proto-planetary nebulae (Zhang and Kwok 2011), and reflection nebulae (Sellgren et al., 2010).
Also seen in the spectrum of Tc-1 are strong broad plateau features around 8, 12, and 17 µm (Figure 1). These plateau features are commonly seen in planetary nebulae and proto-planetary nebulae showing unidentified infrared emission (UIE) bands. These plateau features cannot be associated with any specific molecule and the 8 and 12 µm plateau features have been suggested to arise from superpositions of in-plane and out-of-plane vibrational modes of aliphatic side groups attached to aromatic compounds (Kwok et al., 2001). The presence of 8 and 12 µm plateau features in C60 sources (Otsuka et al., 2013; Zhang and Kwok 2013) suggests that amorphous carbonaceous solids could be precursors of fullerenes (Bernard-Salas et al., 2012; García-Hernández et al., 2012).
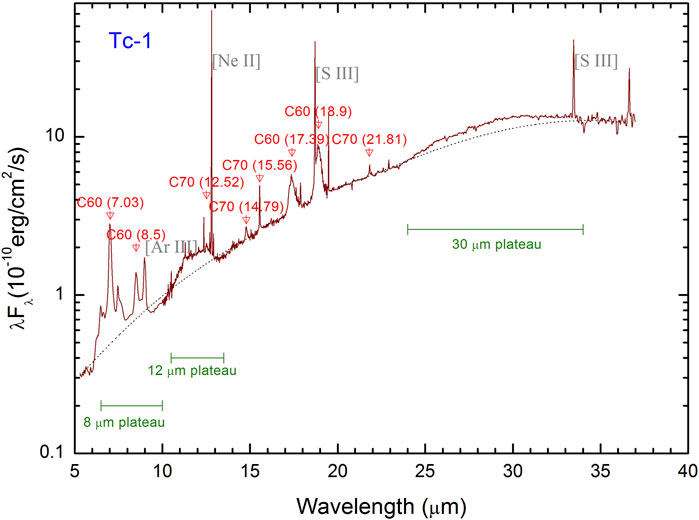
FIGURE 1. Spitzer IRS spectrum of planetary nebula Tc-1 shows C60 and C70 emission bands (in red) as well as broad emission plateau features at 8, 12, and 30 µm (in green). The narrow lines are atomic lines (in grey). The dotted line represents an approximate fit to the continuum. No UIE bands are seen in this object.
The Molecular Component of Planetary Nebulae
Although planetary nebulae are usually known for their optical appearances and their optical nebulae are the most extensively studied, millimeter and submm-wave interferometric observations can map the distribution of the molecular component (Dinh et al., 2008; Nakashima et al., 2010; Santander-García et al., 2017; Andriantsaralaza et al., 2020). For the CO molecule, entire ladder of rotational transitions (up to J = 37–36) have been measured, making possible the estimate of the total molecular masses (Justtanont et al., 2000). In the near infrared, the v = 1–0, J = 3–1 S (1) vibrational-rotational transition of H2 at 2.121 µm is widely observed in planetary nebulae. Assuming that this line is shock or UV exited, the molecular mass in the H2-emitting region can be estimated (Rosado and Arias 2003). From single-dish CO observations, masses of the molecular envelopes of NGC 7027 and IRAS 21282 + 5050 are found to be 1.3 and 4.7 M⊙ respectively (Santander-García et al., 2022), much larger than the masses of their ionized components (0.03 and 0.16 M⊙ for NGC 7027 and IRAS 21282 + 5050, respectively).
Planetary Nebulae as Sources of Solid Minerals
Solid Condensations in Stellar Winds
The most common solid-state condensate observed in the stellar winds of O-rich AGB stars is amorphous silicates. This mineral can be detected through their Si–O stretching mode at 9.7 µm and the Si–O–Si bending mode at 17 µm and is widely present in the spectra of O-rich AGB stars. Also seen in the spectra of O-rich stars are crystalline silicates (pyroxenes and olivines, Jäger et al., 1998) and various kinds of refractory oxides (corundum α-Al2O3, spinel MgAl2O4, rutile TiO2, Posch et al., 1999, 2002).
In C-rich stars, the most common feature is the 11.3 µm feature of silicon carbide (SiC). The strong featureless continuum emission is commonly attributed to amorphous carbon.
As the strength of the stellar wind increases as the star ascend the AGB, the amount of solid-state condensate in the circumstellar environment can begin to obscure the photosphere of the star. In some cases, the entire photospheric visible output of the star is absorbed by circumstellar material and the star will only appear as an infrared object.
The Solid-State Component of Planetary Nebulae
Since planetary nebulae evolve from their AGB progenitors, remnants of the dust envelopes of AGB stars should still be detecable in planetary nebulae (Kwok 1982). Figure 2 shows the spectral energy distribution of the planetary nebula NGC 7027 over the wavelength range of 0.1 µm–1 m. In the long wavelength region (λ > 1 mm), the spectrum is dominated by free-free emission from the ionized nebula. Between 3 µm and 1 mm, thermal radiation from solid-state grains dominates. The UIE bands and the 30 µm feature can be seen in emission above the dust continuum. In the optical region, nebular emission is due to atomic line emission and bound-free continuum emission from the ionized nebula. The central star is heavily obscured by circumstellar dust and is very faint in the visible. The molecular lines are not plotted in this diagram.
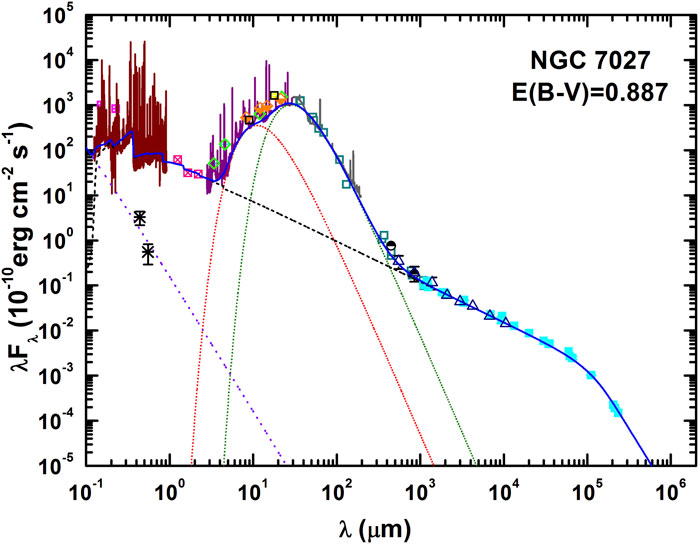
FIGURE 2. Spectral energy distribution of the planetary nebula NGC 7027 from wavelength 0.1 to 1 m. Various symbols represent photometric measurements and the lines are spectroscopic observations. The red and green curves are approximate fits to the dust continuum. The dashed line covering the entire spectrum is a model bound-free and free-free emission from the ionized nebula. The dashed line in the visible and UV region is an approximate fit to the central star continuum. The UV and optical photometry and spectroscopy has been corrected for circumstellar/interstellar reddening with E (B-V) = 0.887. Figure courtesy of C.H. Hsia.
Planetary nebulae can be classified as O- or C-rich based on the respective strengths of the atomic lines in the ionized component (Zuckerman and Aller 1986; Delgado-Inglada and Rodríguez 2014). A similar independent classification is also possible using their infrared spectra of their solid components (Kwok et al., 1997). The presence of silicate feature is a signature of O-richness, and the UIE and 30 µm features are generally only seen in C-rich nebulae (Cohen et al., 1986; Cohen et al., 1989). The Spitzer Space Telescope was used to survey a large sample of galactic and extragalactic planetary nebulae and their spectra have been used to classify planetary nebulae as O- or C-rich types (Stanghellini et al., 2007; Bernard-Salas et al. 2009; Stanghellini et al., 2012; García-Hernández and Górny 2014).
Planetary Nebulae as Sources of Organics
Before the mid-20th century, organics were commonly believed to be within the sole domain of the Earth, and the existence of organic matter in space was not expected. The discovery of complex organics in planetary nebulae came as a surprise and represents a new beginning of our understanding of abiotic synthesis of organics in space.
Observational Evidence of Aromatic and Aliphatic Organics in Planetary Nebulae
A family of broad unidentified infrared emission (UIE) bands at 3.3, 6.2, 7.7, 8.6 and 11.3 μm was first found in the spectrum of the planetary nebula NGC 7027 (Russell et al., 1977), and later found to be commonly present in C-rich planetary nebulae. By comparison with laboratory spectra of organic compounds, these bands are suggested to arise from stretching and bending modes of aromatic compounds (Duley and Williams 1981). Also present in spectra are emission features around 3.4 μm, which correspond to the symmetric and anti-symmetric C–H stretching modes of aliphatic methyl and methylene groups (Puetter et al., 1979; Geballe et al., 1992). The bending modes of these groups manifest themselves at 6.9 and 7.3 μm (De Muizon et al., 1990; Chiar et al., 2020). In addition, there are weaker unidentified emission features at 15.8, 16.4, 17.4, 17.8, and 18.9 μm which may be due to C skeleton vibrational modes of aromatic structures. These discoveries represent the first discovery of stars as sources of organic matter in the Universe.
Figure 3 shows the infrared spectrum of the planetary nebula IRAS 21282 + 5050. In addition to the 3.3, 6.2, 7.7, 8.6, 11.3, 12.4, 13.4, and 16.8 μm UIE bands, emission bands around 3.4 μm due to aliphatic C–H stretching modes can also be seen (Hrivnak et al., 2007).
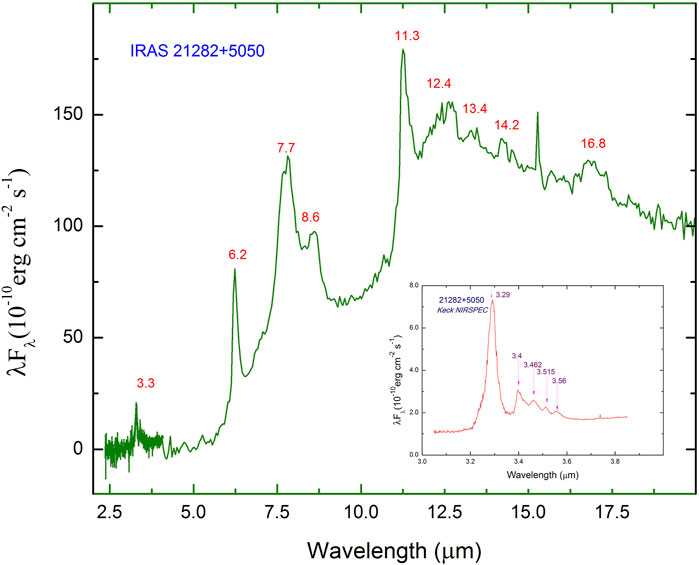
FIGURE 3. Infrared Space Observatory spectrum of planetary nebula IRAS 21282 + 5050. The UIE bands (in units of µm) are marked in red. The insert box shows the expanded spectrum in the 3 µm region obtained with the Keck Telescope, where both the aromatic and aliphatic C–H stretch emissions can be seen.
Circumstellar Synthesis of Complex Organics
Since the UIE bands are seen in planetary nebulae but not AGB stars, they are probably the product of circumstellar synthesis during the post-AGB phase of evolution. The dynamical age of planetary nebulae of ∼104 yr places a limit on the time scale of chemical synthesis. The first signs of UIE bands are seen in proto-planetary nebulae, transition objects between the AGB and planetary nebulae phases before the onset of photoionization (Kwok et al., 1999). The first step to an aromatic compound is the formation of the ring molecule benzene (C6H6), which was detected in the proto-planetary nebula AFGL 618 through one of its bending modes in the infrared (Cernicharo et al., 2001). Acetylene (C2H2), a linear molecule and building block of benzene, is commonly detected in evolved carbon stars through its ν5 fundamental band at 13.7 μm (Volk et al., 2000). Polymerization of C2H2 leads to the formation of diacetylene (C4H2) and triacetylene (C6H2) in proto-planetary nebulae, cumulating in the possible formation of benzene. Benzene molecules can group into multiple rings in the form of aromatic islands, which can be linked to each other via aliphatic bridges of variable lengths and orientations. A possible structure of such mixed aromatic/aliphatic organic nanoparticles (MAONs) was proposed by Kwok and Zhang 2011, Kwok and Zhang 2013. Since MAONs are amorphous and have an extremely sturdy structure, they can survive journeys through the interstellar medium after their ejection during the planetary nebulae stage.
Enrichment of Planetary Systems
Planetary systems are formed from proto-stellar nebulae condensed from interstellar clouds. The chemical composition of the pre-solar nebula is therefore determined by the chemical composition of star-forming regions, which are enriched by ejecta from AGB stars and planetary nebulae. Supernovae, which occur much more rarely in the Galaxy, are responsible for heavy elements produced by rapid-process nucleosynthesis. A large part of condensed objects in the early Solar System are made of ice (H2O, CO) and minerals made of heavy elements (O, Si, Fe, etc). The outer planets are results of condensation of gases and ices, and the inner planets are products of aggregation of solid particles in the primordial Solar Nebula.
Recent studies of meteorites, comets, asteroids, planetary satellites, interplanetary dust particles, have led to unexpected discoveries that complex organics are commonly present in the Solar System. The presence of organics is not only extensive, but also diverse. Almost every class of organic molecules (including carboxylic acids, sulfonic and phosphonic acids, amino acids, aromatic hydrocarbons, heterocyclic compounds, aliphatic hydrocarbons, amines and amides, alcohols, aldehydes, ketones, and sugar related compounds) have been identified in the soluble component of carbonaceous chondrites (Schmitt-Kopplin et al., 2010; Remusat 2015).
Most interestingly, a large fraction (70–90%) of organic carbon in carbonaceous chondrites is in the form of a complex, insoluble, macromolecular material often referred to as insoluble organic matter (IOM, Cronin et al., 1987). Laboratory analyses of IOM reveal a chemical structure consisting of small islands of aromatic rings connected by short aliphatic chains (Cody et al., 2001; Cody et al., 2002). Evidence for the interstellar origin of IOM can be found in its isotope anomalies (Busemann et al., 2006).
Aromatic, aliphatic, and thiophenic (sulfur-containing rings) compounds have been found in Mars’ Gale crater, with kerogen-like materials being their most likely precursor (Eigenbrode et al., 2018). The origin of organic plumes from Enceladus can be traced to complex macromolecular organics in subsurface oceans (Postberg et al., 2018). The atmosphere of Saturn’s moon Titan is filled with organic haze and it surface is covered by lakes of liquid methane and ethane, as well dunes made of organic particles (Lorenz et al., 2008).
The Rosetta mission to the comet 67P/Churyumov-Gerasimenko has revealed an array of organic compounds on the surface of the comet (Goesmann et al., 2015). The Stardust mission to Comet 81P/Wide 2 has detected amino acid glycine with a carbon isotopic ratio suggestive of extra-solar origin (Elsila et al., 2009). Infrared spectra of cometary dust show the 3.3 µm aromatic C–H stretch and 3.4 µm aliphatic C–H stretch, implying the presence of both aromatic and aliphatic materials like IOM in meteorites (Keller et al., 2006; Fray et al., 2016).
Complex organics are also found in interplanetary dust particles (IDP) collected in the Earth’s stratosphere (Flynn et al., 2003). Since IDPs originated from comets and asteroids, this gives us indirect evidence of the presence of organics in comets and asteroids. The anomalous H and N isotopic ratios of IDP organics suggest that they are of pre-solar origin (Keller et al., 2004).
In the asteroid belt, the Dawn mission has detected the 3.4 µm aliphatic C–H stretch over a 1000 km2 area near the Ernutet Crater of the dwarf planet Ceres, therefore confirming the presence of complex organics in the main belt asteroids (De Sanctis et al., 2017). The red color and low albedo of D-type asteroids have been attributed to kerogen-like macromolecular organics covering their surfaces (Cruikshank and Kerridge 1992).
In the outer Solar System, the New Horizons mission found the surface of Pluto to be covered with a range of colored regions, which could be the result of complex organics embedded in water ice on the surface or liquid organics in subsurface reservoirs (Cruikshank et al., 2019). The low albedo found in trans- Neptunian objects has also been suggested to be due to surface complex organics (Giri et al., 2016).
The origin of Solar System organics is a subject of active debate. They are either synthesized in situ from simple molecules, or they are remnants of stellar ejecta that were embedded in the primordial solar nebula. The Solar System is already known to have inherited pre-solar grains of AGB star origin (Zinner 1998). Pre-solar grains of diamonds (Lewis et al., 1987), silicon carbide (Bernatowicz et al., 1987), corundum, and spinel (Nittler et al., 1997) have been identified by isotopic studies of meteorites. It is therefore quite conceivable that stellar organic grains were also embedded in the pre-solar nebula and later deposited into Solar System objects.
Summary
Planetary nebulae have a long history of research on their morphology, structure, and evolution. They are also known to be sources of chemical elements that carry the chemical evolution of the Galaxy. However, it was not until the emergence of infrared and millimeter-wave observations that we realize that they are also major sources of molecules and solids in the Galaxy. The products synthesized in the outflows from evolved stars provide the primordial ingredients for the formation of next generation of stars and planetary systems.
A more significant implication of these recent discoveries is the possible organic enrichment of primordial planetary systems by planetary nebulae. Planetary nebulae (together with novae, Endo et al., 2021) are the only astronomical objects that the synthesis of complex organic compounds is directly observed. Although complex organics could have been synthesized in situ in the Solar System, their presence in carbonaceous chondrites, the most primitive of all Solar System objects, suggests that these organics are most likely previously embedded in the nebula from stellar ejecta materials. The delivery of these Solar System organics onto the early Earth could have implications on the origin of life on Earth.
Planetary nebulae therefore represent a crucial link in the lives of stars. Formed during the last stages of stellar evolution, planetary nebulae ejecta provide primordial ingredients for the formation of the next generation of stars and planetary system. The atomic elements, molecules, minerals, and organic solids are major constituents of the planetary systems. Observations of planetary nebulae show that abiotic organic synthesis can occur extremely efficiently under very low nebular density conditions and over very short (103 yr) time scales. This opens the possibility that organic matter is common in the Universe, and the chemical composition of the Universe is more complex that we previously believed. Since planetary nebulae are a common stage of evolution for most stars, the synthesis and distribution of stellar organics may have significant implications on the existence and distribution of life in our Galaxy.
Author Contributions
SK is responsible for the research performed and writing of this article.
Funding
This work is supported by a grant from the Natural Sciences and Engineering Research Council of Canada.
Conflict of Interest
The author declares that the research was conducted in the absence of any commercial or financial relationships that could be construed as a potential conflict of interest.
Publisher’s Note
All claims expressed in this article are solely those of the authors and do not necessarily represent those of their affiliated organizations, or those of the publisher, the editors and the reviewers. Any product that may be evaluated in this article, or claim that may be made by its manufacturer, is not guaranteed or endorsed by the publisher.
References
Agúndez, M., Cernicharo, J., Decin, L., Encrenaz, P., and Teyssier, D. (2014). Confirmation of Circumstellar Phosphine. Astrophys. J. 790, L27. doi:10.1088/2041-8205/790/2/l27
Agúndez, M., Cernicharo, J., and Guélin, M. (2007). Discovery of Phosphaethyne (HCP) in Space: Phosphorus Chemistry in Circumstellar Envelopes. Astrophys. J. 662, L91–L94. doi:10.1086/519561
Agúndez, M., Cernicharo, J., Pardo, J. R., Guélin, M., and Phillips, T. G. (2008). Tentative Detection of Phosphine in IRC +10216. Astron. Astrophys. 485, L33–L36. doi:10.1051/0004-6361:200810193
Andriantsaralaza, M., Zijlstra, A., and Avison, A. (2020). CO in the C1 Globule of the Helix Nebula with ALMA. Mon. Not. R. Astron. Soc. 491, 758–772. doi:10.1093/mnras/stz3026
Bernard-Salas, J., Cami, J., Peeters, E., Jones, A. P., Micelotta, E. R., and Groenewegen, M. A. T. (2012). On the Excitation and Formation of Circumstellar Fullerenes. ApJ 757, 41. doi:10.1088/0004-637x/757/1/41
Bernard-Salas, J., Peeters, E., Sloan, G. C., Gutenkunst, S., Matsuura, M., Tielens, A. G. G. M., et al. (2009). Unusual Dust Emission from Planetary Nebulae in the Magellanic Clouds. ApJ 699, 1541–1552. doi:10.1088/0004-637x/699/2/1541
Bernatowicz, T., Fraundorf, G., Ming, T., Anders, E., Wopenka, B., Zinner, E., et al. (1987). Evidence for Interstellar SiC in the Murray Carbonaceous Meteorite. Nature 330, 728–730. doi:10.1038/330728a0
Busemann, H., Young, A. F., Alexander, C. M. O. D., Hoppe, P., Mukhopadhyay, S., and Nittler, L. R. (2006). Interstellar Chemistry Recorded in Organic Matter from Primitive Meteorites. Science 312, 727–730. doi:10.1126/science.1123878
Cami, J., Bernard-Salas, J., Peeters, E., and Malek, S. E. (2010). Detection of C 60 and C 70 in a Young Planetary Nebula. Science 329, 1180–1182. doi:10.1126/science.1192035
Cernicharo, J., Heras, A. M., Tielens, A. G. G. M., Pardo, J. R., Herpin, F., Guélin, M., et al. (2001). Infrared Space Observatory's Discovery ofC4H2, C6H2, and Benzene in CRL 618. Astrophys. J. 546, L123–L126. doi:10.1086/318871
Chiar, J. E., Tielens, A. G. G. M., Whittet, D. C. B., Schutte, W. A., Boogert, A. C. A., Lutz, D., et al. (2020). The Composition and Distribution of Dust along the Line of Sight toward the Galactic Center. Astrophys. J. 537, 749–762. doi:10.1086/309047
Cody, G. D., Heying, E., Alexander, C. M., Nittler, L. R., Kilcoyne, A. L., Sandford, S. A., et al. (2001). Establishing a Molecular Relationship between Chondritic and Cometary Organic Solids. Proc. Natl. Acad. Sci. U. S. A. 108, 19171–19176. doi:10.1073/pnas.1015913108
Cody, G. D., Alexander, C. M. O. D., and Tera, F. (2002). Solid-state ( 1 H and 13 C) Nuclear Magnetic Resonance Spectroscopy of Insoluble Organic Residue in the Murchison Meteorite: a Self-Consistent Quantitative Analysis. Geochimica Cosmochimica Acta 66, 1851–1865. doi:10.1016/s0016-7037(01)00888-2
Cohen, M., Allamandola, L., Tielens, A. G. G. M., Bregman, J., Simpson, J. P., Witteborn, F. C., et al. (1986). The Infrared Emission Bands. I - Correlation Studies and the Dependence on C/O Ratio. ApJ 302, 737–749. doi:10.1086/164035
Cohen, M., Tielens, A. G. G. M., Bregman, J., Witteborn, F. C., Rank, D. M., Allamandola, L. J., et al. (1989). The Infrared Emission Bands. III - Southern IRAS Sources. ApJ 341, 246–269. doi:10.1086/167489
Cronin, J. R., Pizzarello, S., and Frye, J. S. (1987). 13C NMR Spectroscopy of the Insoluble Carbon of Carbonaceous Chondrites. Geochimica Cosmochimica Acta 51, 299–303. doi:10.1016/0016-7037(87)90242-0
Cruikshank, D. P., Materese, C. K., Pendleton, Y. J., Boston, P. J., Grundy, W. M., Schmitt, B., et al. (2019). Prebiotic Chemistry of Pluto. Astrobiology 19, 831–848. doi:10.1089/ast.2018.1927
De Muizon, M. J., d’Hendecourt, L. B., and Geballe, T. R. (1990). Polycyclic Aromatic Hydrocarbons in the Near-Infrared Spectra of 24 IRAS Sources. Astron. Astrophys. 227 (2), 526–541.
De Sanctis, M. C., Ammannito, E., McSween, H. Y., Raponi, A., Marchi, S., Capaccioni, F., et al. (2017). Localized Aliphatic Organic Material on the Surface of Ceres. Science 355, 719–722. doi:10.1126/science.aaj2305
Delgado-Inglada, G., and Rodríguez, M. (2014). C/O Abundance Ratios, Iron Depletions, and Infrared Dust Features in Galactic Planetary Nebulae. ApJ 784, 173. doi:10.1088/0004-637x/784/2/173
Dinh, VT, Bujarrabal, V., Castro‐Carrizo, A., Lim, J., and Kwok, S. (2008). Massive Expanding Torus and Fast Outflow in Planetary Nebula NGC 6302. ApJ 673, 934–941. doi:10.1086/524373
Duley, W. W., and Williams, D. A. (1981). The Infrared Spectrum of Interstellar Dust: Surface Functional Groups on Carbon. Mon. Notices R. Astronomical Soc. 196, 269–274. doi:10.1093/mnras/196.2.269
Eigenbrode, J. L., Summons, R. E., Steele, A., Freissinet, C., Millan, M., Navarro-González, R., et al. (2018). Organic Matter Preserved in 3-Billion-Year-Old Mudstones at Gale Crater, Mars. Science 360, 1096–1101. doi:10.1126/science.aas9185
Elsila, J. E., Glavin, D. P., and Dworkin, J. P. (2009). Cometary glycine Detected in Samples Returned by Stardust. Meteorit. Planet. Sci. 44, 1323–1330. doi:10.1111/j.1945-5100.2009.tb01224.x
Endo, I., Sakon, I., Onaka, T., Kimura, Y., Kimura, S., Wada, S., et al. (2021). On the Nature of Organic Dust in Novae. ApJ 917, 103. doi:10.3847/1538-4357/ac0cf1
Flynn, G. J., Keller, L. P., Feser, M., Wirick, S., and Jacobsen, C. (2003). The Origin of Organic Matter in the Solar System: Evidence from the Interplanetary Dust Particles. Geochimica Cosmochimica Acta 67, 4791–4806. doi:10.1016/j.gca.2003.09.001
Fray, N., Bardyn, A., Cottin, H., Altwegg, K., Baklouti, D., Briois, C., et al. (2016). High-molecular-weight Organic Matter in the Particles of Comet 67P/Churyumov-Gerasimenko. Nature 538, 72–74. doi:10.1038/nature19320
García-Hernández, D. A., and Górny, S. K. (2014). Chemical Abundances in Galactic Planetary Nebulae with Spitzer Spectra. Astron. Astrophys. 567, A12.
García-Hernández, D. A., Manchado, A., Garcia-Lario, P., Stanghellini, L., Villaver, E., Shaw, R. A., et al. (2010). Formation of Fullerenes in H-Containing Planetary Nebulae. Astrophys. J. 724, L39–L43. doi:10.1088/2041-8205/724/1/L39
García-Hernández, D. A., Villaver, E., Garcia-Lario, P., Shaw, R. A., Manchado, A., Stanghellini, L., et al. (2012). Infrared Study of Fullerene Planetary Nebulae. Astrophys. J. 760, 107. doi:10.1088/0004-637X/760/2/107
Geballe, T. R., Tielens, A. G. G. M., Kwok, S., and Hrivnak, B. J. (1992). Unusual 3 Micron Emission Features in Three Proto-Planetary Nebulae. ApJ 387, L89–L91. doi:10.1086/186312
Giri, C., McKay, C. P., Goesmann, F., Schäfer, N., Li, X., Steininger, H., et al. (2016). Carbonization in Titan Tholins: Implication for Low Albedo on Surfaces of Centaurs and Trans-neptunian Objects. Int. J. Astrobiol. 15, 231–238. doi:10.1017/s1473550415000439
Goesmann, F., Rosenbauer, H., Bredehöft, J. H., Cabane, M., Ehrenfreund, P., Gautier, T., et al. (2015). Organic Compounds on Comet 67P/Churyumov-Gerasimenko Revealed by COSAC Mass Spectrometry. Science 349. doi:10.1126/science.aab0689
Halfen, D. T., Clouthier, D. J., and Ziurys, L. M. (2008). Detection of the CCP Radical ( X 2 Π R ) in IRC +10216: A New Interstellar Phosphorus-Containing Species. ApJ 677, L101–L104. doi:10.1086/588024
Hrivnak, B. J., Geballe, T. R., and Kwok, S. (2007). A Study of the 3.3 and 3.4 μm Emission Features in Proto-Planetary Nebulae. ApJ 662, 1059–1066. doi:10.1086/518109
Hubble, E. P. (1922). TThe Source of Luminosity in Galactic Nebulae. ApJ 56, 400–438. doi:10.1086/142713
Jäger, C., Molster, F. J., Dorschner, J., Henning, T., Mutschke, H., and Waters, L. (1998). Steps toward Interstellar Silicate Mineralogy - IV. The Crystalline Revolution. Astron. Astrophys. 339 (3), 904–916.
Justtanont, K., Barlow, M. J., Tielens, A. G. C. M., Hollenbach, D., Latter, W. B., Liu, X. W., et al. (2000). ISO-LWS Observations of Rotational CO Lines from C-Rich Objects: AFGL 2688, AFGL 618 and NGC 7027. Astron. Astrophys. 360, 1117.
Keller, L. P., Bajt, S., Baratta, G. A., Borg, J., Bradley, J. P., Brownlee, D. E., et al. (2006). Infrared Spectroscopy of Comet 81P/Wild 2 Samples Returned by Stardust. Science 314, 1728–1731. doi:10.1126/science.1135796
Keller, L. P., Messenger, S., Flynn, G. J., Clemett, S., Wirick, S., and Jacobsen, C. (2004). The Nature of Molecular Cloud Material in Interplanetary Dust. Geochimica Cosmochimica Acta 68, 2577–2589. doi:10.1016/j.gca.2003.10.044
Kwitter, K. B., and Henry, R. B. C. (2022). Planetary Nebulae: Sources of Enlightenment. Pasp 134, 022001. doi:10.1088/1538-3873/ac32b1
Kwok, S., Purton, C. R., and Fitzgerald, P. M. (1978). On the Origin of Planetary Nebulae. ApJ 219, L125–L127. doi:10.1086/182621
Kwok, S., Volk, K., and Bernath, P. (2001). On the Origin of Infrared Plateau Features in Proto-Planetary Nebulae. Astrophys. J. 554, L87–L90. doi:10.1086/320913
Kwok, S., Volk, K., and Bidelman, W. P. (1997). Classification and Identification of IRAS Sources with Low‐Resolution Spectra. Astrophys. J. Suppl. S 112, 557–584. doi:10.1086/313038
Kwok, S., Volk, K., and Hrivnak, B. J. (1999). Chemical Evolution of Carbonaceous Materials in the Last Stages of Stellar Evolution. Astron. Astrophys. 350, L35–L38.
Kwok, S., and Zhang, Y. (2011). Mixed Aromatic-Aliphatic Organic Nanoparticles as Carriers of Unidentified Infrared Emission Features. Nature 479, 80–83. doi:10.1038/nature10542
Kwok, S., and Zhang, Y. (2013). Unidentified Infrared Emission Bands: PAHs or MAONs? ApJ 771, 5. doi:10.1088/0004-637x/771/1/5
Lewis, R. S., Ming, T., Wacker, J. F., Anders, E., and Steel, E. (1987). Interstellar Diamonds in Meteorites. Nature 326, 160–162. doi:10.1038/326160a0
Lorenz, R. D., Karl, L. M., Randolph, L. K., Alexander, G. H., Oded, A., Howard, A., et al. (2008). Titan's Inventory of Organic Surface Materials. Geophys. Res. Lett. 35, 2206. doi:10.1029/2007GL032118
Maciá-Barber, E. (2019). The Chemical Evolution of Phosphorus: An Interdisciplinary Approach to Astrobiology. New York, NY: Apple Academic Press.
McGuire, B. A. (2022). 2021 Census of Interstellar, Circumstellar, Extragalactic, Protoplanetary Disk, and Exoplanetary Molecules. ApJS 259, 30. doi:10.3847/1538-4365/ac2a48
Nakashima, J.-i., Kwok, S., Zhang, Y., and Koning, N. (2010). Three-dimensional Structure of the Central Region of NGC 7027: A Quest for Trails of High-Velocity Jets. Astronomical J. 140, 490–499. doi:10.1088/0004-6256/140/2/490
Nittler, L. R., Alexander, C. M. O. D., Gao, X., Walker, R. M., and Zinner, E. (1997). Stellar Sapphires: The Properties and Origins of Presolar Al2O3in Meteorites. ApJ 483, 475–495. doi:10.1086/304234
Otsuka, M., Kemper, F., Hyung, S., Sargent, B. A., Meixner, M., Tajitsu, A., et al. (2013). The Detection of C60 in the Well-Characterized Planetary Nebula M1-11. ApJ 764, 77. doi:10.1088/0004-637x/764/1/77
Posch, T., Kerschbaum, F., Mutschke, H., Dorschner, J., and Jäger, C. (2002). On the Origin of the 19.5 $\mathsf{\mu}$m featureIdentifying Circumstellar Mg-Fe-Oxides. A&A 393, L7–L10. doi:10.1051/0004-6361:20021127
Posch, T., Kerschbaum, F., Mutschke, H., Fabian, D., Dorschner, J., and Hron, J. (1999). On the Origin of the 13 Μm Feature. A Study of ISO-SWS Spectra of Oxygen-Rich AGB Stars. Astron. Astrophys. 352, 609–618.
Postberg, F., Khawaja, N., Abel, B., Choblet, G., Glein, C. R., Gudipati, M. S., et al. (2018). Macromolecular Organic Compounds from the Depths of Enceladus. Nature 558, 564–568. doi:10.1038/s41586-018-0246-4
Puetter, R. C., Russell, R. W., Willner, S. P., and Soifer, B. T. (1979). Spectrophotometry of Compact H II Regions from 4 to 8 Microns. ApJ 228, 118–122. doi:10.1086/156828
Remusat, L. (2015). Organics in Primitive Meteorites. Eur. Mineralogical Union Notes Mineralogy 15, 33–65. doi:10.1180/emu-notes.15.2
Russell, R. W., Soifer, B. T., and Willner, S. P. (1977). The 4 to 8 Micron Spectrum of NGC 7027. ApJ 217, L149–L153. doi:10.1086/182559
Santander-García, M., Bujarrabal, V., Alcolea, J., Castro-Carrizo, A., Sánchez Contreras, C., Quintana-Lacaci, G., et al. (2017). ALMA High Spatial Resolution Observations of the Dense Molecular Region of NGC 6302. Astron. Astrophys. 597, A27. doi:10.1051/0004-6361/201629288
Santander-García, M., Jones, D., Alcolea, J., Bujarrabal, V., and Wesson, R. (2022). The Ionised and Molecular Mass of Post-common-envelope Planetary Nebulae. The Missing Mass Problem. Astron. Astrophys. 658, A17.
Schmitt-Kopplin, P., Gabelica, Z., Gougeon, R. D., Fekete, A., Kanawati, B., Harir, M., et al. (2010). High Molecular Diversity of Extraterrestrial Organic Matter in Murchison Meteorite Revealed 40 Years after its Fall. Proc. Natl. Acad. Sci. U.S.A. 107, 2763–2768. doi:10.1073/pnas.0912157107
Sellgren, K., Werner, M. W., Ingalls, J. G., Smith, J. D. T., Carleton, T. M., and Joblin, C. (2010). C 60 IN REFLECTION NEBULAE. ApJ 722, L54–L57. doi:10.1088/2041-8205/722/1/l54
Stanghellini, L., Garcia‐Lario, P., Garcia‐Hernandez, D. A., Perea‐Calderon, J. V., Davies, J. E., Manchado, A., et al. (2007). SpitzerInfrared Spectrograph Observations of Magellanic Cloud Planetary Nebulae: The Nature of Dust in Low‐Metallicity Circumstellar Ejecta. ApJ 671, 1669–1684. doi:10.1086/523086
Stanghellini, L., García-Hernández, D. A., García-Lario, P., Davies, J. E., Shaw, R. A., Villaver, E., et al. (2012). THE NATURE OF DUST IN COMPACT GALACTIC PLANETARY NEBULAE FROMSPITZERSPECTRA. ApJ 753, 172. doi:10.1088/0004-637x/753/2/172
Tenenbaum, E. D., Dodd, J. L., Milam, S. N., Woolf, N. J., and Ziurys, L. M. (2010). Comparative Spectra of Oxygen-Rich versus Carbon-Rich Circumstellar Shells: VY Canis Majoris and IRC +10216 at 215-285 GHz. ApJ 720, L102–L107. doi:10.1088/2041-8205/720/1/l102
Tenenbaum, E. D., and Ziurys, L. M. (2008). A Search for Phosphine in Circumstellar Envelopes: PH 3 in IRC +10216 and CRL 2688? ApJ 680, L121–L124. doi:10.1086/589973
Volk, K., Xiong, G. Z., and Kwok, S. (2000). Infrared Space ObservatorySpectroscopy of Extreme Carbon Stars. ApJ 530, 408–417. doi:10.1086/308355
Zanstra, H. (1827). An Application of the Quantum Theory to the Luminosity of Diffuse Nebulae. Astrophys. J. 65, 50.
Zhang, C. Y., and Kwok, S. (1991). Spectral Energy Distribution of Compact Planetary Nebulae. Astron. Astrophys. 250, 179–211.
Zhang, Y., and Kwok, S. (2011). DETECTION OF C60IN THE PROTOPLANETARY NEBULA IRAS 01005+7910. ApJ 730, 126. doi:10.1088/0004-637x/730/2/126
Zhang, Y., and Kwok, S. (2013). On the Detections of C60 and Derivatives in Circumstellar Environments. Earth Planet Sp. 65, 1069–1081. doi:10.5047/eps.2013.06.003
Zinner, E. (1998). Stellar Nucleosynthesis and the Isotopic Composition of Presolar Grains from Primitive Meteorites. Annu. Rev. Earth Planet. Sci. 26, 147–188. doi:10.1146/annurev.earth.26.1.147
Ziurys, L. M., Halfen, D. T., Geppert, W., Aikawa, Y., Geppert, W., and Aikawa, Y. (2016). Following the Interstellar History of Carbon: from the Interiors of Stars to the Surfaces of Planets. Astrobiology 16, 997–1012. doi:10.1089/ast.2016.1484
Ziurys, L. M. (2006). The Chemistry in Circumstellar Envelopes of Evolved Stars: Following the Origin of the Elements to the Origin of Life. Proc. Natl. Acad. Sci. U.S.A. 103, 12274–12279. doi:10.1073/pnas.0602277103
Keywords: planetary nebulae, asymptotic giant branch stars, chemical enrichment, organic synthesis, solar system
Citation: Kwok S (2022) Planetary Nebulae as Sources of Chemical Enrichment of the Galaxy. Front. Astron. Space Sci. 9:893061. doi: 10.3389/fspas.2022.893061
Received: 09 March 2022; Accepted: 25 April 2022;
Published: 10 May 2022.
Edited by:
Miriam Peña, Universidad Nacional Autónoma de México, MexicoReviewed by:
Albert Rimola, Universitat Autònoma de Barcelona, SpainMarcelino Agundez, Spanish National Research Council (CSIC), Spain
Copyright © 2022 Kwok. This is an open-access article distributed under the terms of the Creative Commons Attribution License (CC BY). The use, distribution or reproduction in other forums is permitted, provided the original author(s) and the copyright owner(s) are credited and that the original publication in this journal is cited, in accordance with accepted academic practice. No use, distribution or reproduction is permitted which does not comply with these terms.
*Correspondence: Sun Kwok, c3Vua3dva0Boa3UuaGs=
†ORCID: Sun Kwok, http://orcid.org/0000-0001-7708-081X