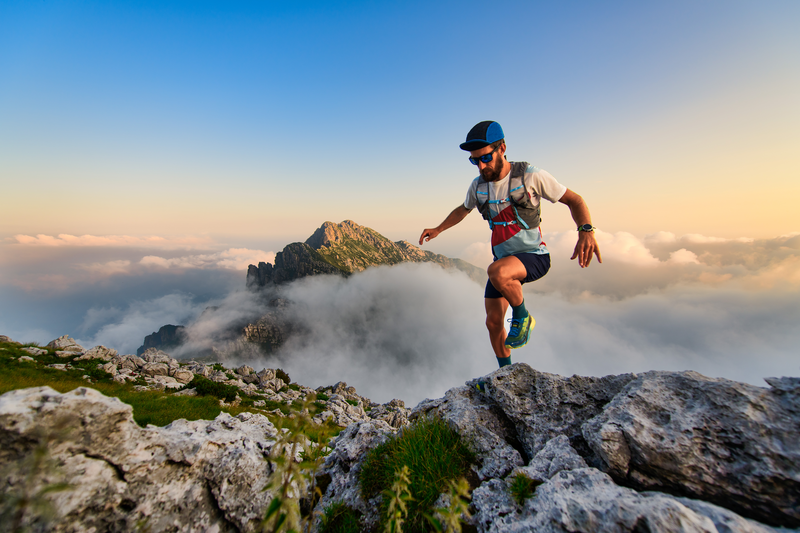
94% of researchers rate our articles as excellent or good
Learn more about the work of our research integrity team to safeguard the quality of each article we publish.
Find out more
EDITORIAL article
Front. Astron. Space Sci. , 01 December 2022
Sec. Nuclear Physics
Volume 9 - 2022 | https://doi.org/10.3389/fspas.2022.1087543
This article is part of the Research Topic Nuclear Physics and Astrophysics in Plasma Traps View all 11 articles
Editorial on the Research Topic
Nuclear physics and astrophysics in plasma traps
β-decay rates are a fundamental ingredient of stellar nucleosynthesis. In particular, elements heavier than iron are produced by a subtle interplay between neutron captures and β-decays, being r-, i- and s-processes mechanisms driving the synthesis of neutron rich nuclei up to the limits imposed by nuclear stability. Therefore, any attempt to reproduce the observed abundances of heavy elements in the Universe calls for an accurate knowledge of neutron capture cross-sections and β-decay rates in a stellar environment as well as the comprehension of any possible mechanism affecting isotope times of life. Even though the β-decay constant has been measured for a wide range of nuclei, a major difference exists between laboratory and stellar conditions. Indeed, temperatures and densities may have very different values, ranging over several orders of magnitude, in the different nucleosynthesis environments. Therefore, stellar plasmas, where nucleosynthesis occurs, are not always fully ionized and when thermodynamical conditions affect the ionization degree of the involved atoms the β-decay process of their nuclei can be strongly modified too. This is particularly true for those nuclei whose β-decay Q value is small or slightly negative in the laboratory. In those cases, half-lives (t1/2) in stellar and terrestrial conditions are predicted to be different for a fixed type of transition at temperatures above a few keV. Such an effect was first shown in the seminal work by Takahashi and Yokoi published in 1987 (Takahashi and Yokoi, 1983; Takahashi and Yokoi, 1987), which investigated the in-plasma effects due to the temperature and the electron density on β-decay rates. Despite the pivotal importance of these data, the cited paper is so far the only one reporting the high temperature β-decay rate of several nuclei and after 35 years the Takashi and Yokoi values are still the most used in calculations of stellar and primordial nucleosynthesis.
The first experimental evidence of the effect on β-decay of the high degree of ionization of atoms was found in Storage Rings (Litvinov and Bosch, 2011), which opened up the possibility of measuring β-decay rates in fully ionized atoms. Among the most impressive results, the fully stripped 187Re75+ ions were found to decay nine orders of magnitude faster than neutral 187Re ones, which have a half-life of 42 Gyr. The reason for this striking variation is rooted in a new decay mechanism called “bound-state β-decay”, typically forbidden in neutral atoms, in which the electron emitted in the decay process is captured in one of the inner shells of the atom.
Storage ring studies can only investigate one charge state at a time, meaning they do not enable the simulation of specific stellar like conditions where ions with broad charge distribution are present, due to the high values and gradients of the temperature of the environment. This limits the comparison of experimental data with model predictions, which typically take into account the average effect due to the ion charge distribution.
A new approach is needed to overcome such limitations and elucidate the possible effects of the charge state distribution (CSD) on the β-decay rate. In the very near future, controlled laboratory plasmas will allow us to investigate the β-decay properties of radioactive nuclei emulating the ionic charge state distributions of several nucleosynthesis environments. This is the main goal of the PANDORA facility (Mascali et al., 2017; Mascali et al., 2022). This approach will shed light on challenging open questions about primordial nucleosynthesis, s-, r- and i-processing (Palmerini et al., 2021), the Solar System formation and its early pollutions with radioactive nuclei, the cosmo-chronometer “calibrations”, etc. At the same time, the plasmas created in laboratory are suited for opacity measurements that are relevant for Kilonovae scenarios, to support and address issues in observations of r-process abundances hours or days after the event. Such attractive opportunities require us to develop suitable theoretical and experimental procedures and methodologies, which are the subject of this Research Topic.
State-of-the-art studies of nucleosynthesis that are beyond Sr for both the s-process and r-process are discussed in contributions by Busso et al. and Spartà et al., respectively. By using up-to-date nuclear inputs, the implications disclosed by a proper knowledge of β-decay rates and neutron cross-sections are discussed in the two papers. New measurements of half-lives in ionized plasmas are suggested to improve the knowledge of both nucleosynthesis scenarios. To achieve this task a trap confining the plasma at temperatures up to about 30 keV and electron densities of 1011–1013 cm−3 is needed. The design and the realization of the plasma trap are complex because some lines of sight have to be created to give access to the diagnostics and the gamma detection system needed to tag the decay events. This setup is described in detail by Mauro et al. The peculiarity of the approach is based on the possibility of operating with magneto-hydrodynamically stable plasmas, whose main features (density, temperature, and ion charge distribution) can be monitored online by a multi-diagnostics system.
The potentialities of some dedicated diagnostics tools to measure electron properties in plasma are discussed by Tsikata et al. and Torrisi et al. While their measurement is fundamental to establishing the thermodynamical condition of the plasma, a priori calculations of plasma electron density and energy distribution in a 3D space are mandatory to predict ion creations and their spatial distribution following plasma neutrality conditions. These arguments are discussed by Galatà et al. who indicate that information coming from the diagnostic system is fundamental to mapping the evolution of the β-decay rates as a function of the plasma thermodynamical conditions. However, the ion distribution in the trap is neither homogeneous nor isotropic and therefore one has to evaluate its implication in the decay rate measurements as well as the non-local thermodynamic equilibrium nature of the system, joining plasma kinetic equations with the expected β-decay rates in ionized and excited ions, a challenging task debated in another contribution to this Research Topic by Mishra et al. The gamma array used to tag the β-decay events will be made of HPGe detectors that work in rather harsh conditions, due to the high background rate coming from the plasma self-emission. Dedicated electronics have been developed to cope with such extreme conditions, making the measurement feasible without any important losses in the array performance. The design of the array, its efficiency, and the simulation of the measurability of a few physic cases are discussed in Naselli et al. while the development of the dedicated electronics and the tests of their performances are presented in the article by Goasduff et al. Finally, the possibility of applying the same experimental setup to an additional physics case is presented in the work of Pidatella et al., which examines the intriguing perspective of measuring the plasma opacities relevant to investigating Kilonovae and multi-messenger astronomy scenarios.
All authors listed have made a substantial, direct, and intellectual contribution to the work and approved it for publication.
The authors declare that the research was conducted in the absence of any commercial or financial relationships that could be construed as a potential conflict of interest.
All claims expressed in this article are solely those of the authors and do not necessarily represent those of their affiliated organizations, or those of the publisher, the editors and the reviewers. Any product that may be evaluated in this article, or claim that may be made by its manufacturer, is not guaranteed or endorsed by the publisher.
Litvinov, Y. A., and Bosch, F. (2011). Beta decay of highly charged ions. Rep. Prog. Phys. 74, 016301. doi:10.1088/0034-4885/74/1/016301
Mascali, D., Musumarra, A., Leone, F., Romano, F. P., Galata, A., Gammino, S., et al. (2017). PANDORA, a new facility for interdisciplinary in-plasma physics. Eur. Phys. J. A 53 (7), 145. doi:10.1140/epja/i2017-12335-1
Mascali, D., Santonocito, D., Amaducci, S., Ando, L., Antonuccio, V., Biri, S., et al. (2022). A novel approach to β-decay: PANDORA, a new experimental setup for future in-plasma measurements. Universe 8, 80. doi:10.3390/universe8020080
Palmerini, S., Busso, M., Vescovi, D., Naselli, E., Pidatella, A., Mucciola, R., et al. (2021). Presolar grain isotopic ratios as constraints to nuclear and stellar parameters of asymptotic giant branch star nucleosynthesis. Astrophys. J. 921 (1), 7. doi:10.3847/1538-4357/ac1786
Takahashi, K., and Yokoi, K. (1987). Beta-decay rates of highly ionized heavy atoms in stellar interiors. Atomic Data Nucl. Data Tables 36 (3), 375–409. doi:10.1016/0092-640X(87)90010-6
Keywords: Beta-decay of highly charged ions, plasma, nucleosynthesis—stars: abundances, s-process nucleosynthesis, r-process, gamma detection, plasma diagnostic measurement
Citation: Mascali D, Santonocito D, de Angelis G, Kratz K-L, Palmerini S and Torrisi G (2022) Editorial: Nuclear physics and astrophysics in plasma traps. Front. Astron. Space Sci. 9:1087543. doi: 10.3389/fspas.2022.1087543
Received: 02 November 2022; Accepted: 09 November 2022;
Published: 01 December 2022.
Edited and reviewed by:
Jie Meng, Peking University, ChinaCopyright © 2022 Mascali, Santonocito, de Angelis, Kratz, Palmerini and Torrisi. This is an open-access article distributed under the terms of the Creative Commons Attribution License (CC BY). The use, distribution or reproduction in other forums is permitted, provided the original author(s) and the copyright owner(s) are credited and that the original publication in this journal is cited, in accordance with accepted academic practice. No use, distribution or reproduction is permitted which does not comply with these terms.
*Correspondence: David Mascali, ZGF2aWRtYXNjYWxpQGxucy5pbmZuLml0
Disclaimer: All claims expressed in this article are solely those of the authors and do not necessarily represent those of their affiliated organizations, or those of the publisher, the editors and the reviewers. Any product that may be evaluated in this article or claim that may be made by its manufacturer is not guaranteed or endorsed by the publisher.
Research integrity at Frontiers
Learn more about the work of our research integrity team to safeguard the quality of each article we publish.