- 1Space Science Division, Naval Research Laboratory, Washington, DC, United States
- 2NASA Goddard Space Flight Center Greenbelt, MD, United States
- 3College of Science, George Mason University 4400 University Drive, Fairfax, VA, United States
- 4Lockheed Martin Solar and Astrophysics Laboratory, Palo Alto, CA, United States
- 5National Center for Atmospheric Research High Altitude Observatory, Boulder, CO, United States
- 6Predictive Science Inc., San Diego, CA, United States
- 7NASA Marshall Space Flight Center, Huntsville, AL, United States
In the context of the recent call for white papers for the Decadal Survey for Solar and Space Physics (Heliophysics) 2024–2033, we present the argument that spatially-resolved spectral diagnostics over the full solar disk should be made a high-priority goal in Heliophysics and lead to the development of a mission concept that has the potential to become a cornerstone of the Heliophysics System Observatory (HSO). We claim that a mission providing consistent full-disk sampling of plasma properties (Doppler-shifts, non-thermal velocities, electron densities, elemental composition, etc.) of the chromosphere-corona system at the dynamic timescales of solar eruptive phenomena will revolutionize the field by adding the spectral diagnostics capability to the highly successful concept of full-disk imaging, everywhere, all the time, represented by the Solar Dynamics Observatory (SDO). We argue for it to be developed by the end of the current Decadal Survey cycle around the lessons learned from spectroscopic missions in the current NASA development pipeline, that include innovative strategies for faster integration of large fields-of-view, and comprehensive temperature coverage.
1 Introduction
Heliophysics is a rapidly evolving field that acknowledges and embraces the importance of global connectivity. Research programs and missions prompt interdisciplinary collaboration and instrument coordination from divergent vantage points. The Sun, source of irradiance and heliospheric disturbances, is an obviously central subject of scrutiny, that has made space missions such as the Solar Dynamics Observatory (SDO; Lemen et al., 2012), with consistent, high-cadence diagnostics of magnetic fields and radiance, a keystone of the Heliophysics System Observatory (HSO). It is hard to envision a future in Heliophysics without a constant, reliable observer of the sources of atmospheric dynamics and space weather. The purpose of the present paper is to present the argument, submitted as a white paper to the Decadal Survey for Solar and Space Physics (Heliophysics) 2024–2033 (Ugarte-Urra et al., 2022), that Heliophysics as a field ought to strategize to maintain such a resource, evolving it into a more powerful diagnostic tool, a spatially resolved full-disk imaging spectrograph.
2 Why full-disk?
There are compelling reasons to prioritize on-disk observations of the solar atmosphere with a small field-of-view (FOV). Fundamental processes, such as coronal heating, magnetic reconnection or solar wind acceleration, take place at often unresolved spatial scales that make ever-increasing high-resolution observations necessary to identify the signatures that can discriminate between competing theories. Since remote sensing is usually performed close to the earth’s orbit, and there are only marginal differences in the angular distance resolved by the different observatories, the trade-off for high-spatial resolution and limited size detectors is to observe with small FOV. Several revolutionary instruments in the current development pipeline will delve into high-resolution fast cadence diagnostics of the chromosphere-corona system (e.g. Solar-C EUVST: Shimizu et al., 2019, MUSE: De Pontieu et al., 2020) and the outcome of those missions is highly anticipated.
However, the high scientific return of EUV and X-ray imagers such as SOHO/EIT (Delaboudinière et al., 1995), Hinode/XRT (Golub et al., 2007), STEREO/EUVI (Wuelser et al., 2004) and the already cited SDO/AIA, makes it equally easy and compelling to advocate for the benefits of full-disk observatories. We have learned that observing the complete solar disk, from earth, but also other perspectives, is critical to our understanding of the Sun and the heliosphere as a connected system where dynamical processes can have large-scale magnetic couplings (e.g. Schrijver et al., 2013). Instruments with small FOV, typically less than 512” squared and 10% of the full-disk area, provide unmatched spectral diagnostics of observed flares and coronal mass ejection (CME) sources, but can observe at best 40% of flare events with our current forecasting abilities and observing strategies (Inglis et al., 2021). They often, therefore, miss the identification and diagnostics of sympathetic activity that can be the trigger in the destabilization of distant regions (Schrijver and Higgins, 2015). That rate of flare sampling is statistically sufficient to accomplish the goals of those missions, but does not maximize our understanding of Earth-directed events monitored by other instruments within the HSO. Full-disk imagers such as those cited above have, on the contrary, provided a continuous watch and characterization of most Earth-side eruptive events and any other phenomena on-disk or just above the limb at different cadences, and with very few gaps. This has constituted a wealth of data that has provided its own intrinsic scientific merit with findings on a variety of phenomena such as coronal holes (e.g. Heinemann et al., 2020), coronal jets (Raouafi et al., 2016) and bright points (Madjarska, 2019), coronal loops (Reale, 2014), flaring active regions (Toriumi and Wang, 2019), coronal mass ejections (Webb and Howard, 2012), prominences (Parenti, 2014), cavities (e.g. Forland et al., 2013) and many others. As important as those discoveries are, full-disk images have multiplied their impact on the field by enhancing the exploitation of data from observatories 1) tracking down the sources for heliospheric activity (ACE, Wind, Solar Probe), 2) observing with focused FOV (e.g. ground based, TRACE, Hinode, RHESSI, IRIS), 3) from different perspectives (STEREO), or 4) a combination of all of them (Solar Orbiter).
The rapid growth and improved fidelity of magneto-hydrodynamic (MHD) global scale computational models of the solar atmosphere (e.g. Mikić et al., 2018; Downs et al., 2021) has also been driven by our ability to test their predictions of the global radiance and connectivity at different solar activity levels. In our path to understanding the Sun as a star among many, but the only one that can be sufficiently resolved to understand the sources of spectral irradiance, it is imperative to continue falsifying these predictions with data at the appropriate global scales and, as we lean into the topic of the next section, with a comprehensive set of diagnostics. This has been routinely the case for computational models representing smaller physical domains (small FOV) that have a matching set of diagnostics (radiance, velocities, densities, non-thermal broadenings), but is not possible yet on global scales because of a lack of diagnostic capabilities in the broadband imagers such as SDO/AIA. NOAA has acknowledged the need of such an observatory deploying the GOES Solar UltraViolet Imager (SUVI: Darnel et al., 2022) that will cover part of those needs. Looking ahead, in the context of the Heliophysics Decadal Survey, we assert that there is a transformative concept, beyond monitoring, that would give us the generational leap forward, that has characterized past major mission success stories, and that would allow us to carry theory and observational data at a similar growing pace. We argue below that the next full-disk observatory should incorporate the spectral diagnostic capabilities of small-FOV instruments benefiting from the best of both approaches.
3 Why spectral diagnostics?
Leaps in our understanding of the solar atmosphere have followed from technology development and new observing strategies. For example, the first EUV and X-ray images of the Sun at the beginning of the space age; novel exploration paths such as the out-of-ecliptic orbit of Ulysses, or the ahead/behind orbits of the STEREO spacecraft; the first comprehensive solar and heliospheric observatory (SOHO); and the first high-cadence, high resolution EUV images from TRACE. SDO delivered its own story by providing EUV radiance and magnetic field boundaries on-demand (meaning everywhere, all the time). We argue that of the multiple possible avenues forward, full-disk sampling of plasma properties at the dynamic timescales of eruptive solar activity will open an observational space with all the ingredients for a follow-up groundbreaking mission and science.
A full-disk solar spectrograph would build on the strengths of its predecessors (SDO/AIA, Hinode/EIS, IRIS and the planned Solar-C (EUVST), MUSE) and provide the plasma diagnostics on-demand that are missing from our current full-disk observatories. Figure 1 shows a representation of the multi-dimensional data products that a spatially resolved full-disk spectrograph would provide. The space of Doppler-shifts, non-thermal widths, densities, and elemental composition changes as a function of time at global scales in the chromosphere-corona system has barely been scratched with full-disk mosaic maps produced by SOHO/SUMER, SOHO/CDS, HINODE/EIS and IRIS. It is constrained by the fact that the current sustained cadence is at most one map per month1. Full-disk velocity and plasma composition maps derived from one of these Hinode/EIS EUV mosaics have already been used in combination with magnetic topology models to make a slow solar wind source map and to identify candidate sources (Brooks et al., 2015), one of the remaining grand open questions in solar physics: where (and how) does the slow solar wind originate. A pilot investigation that has motivated newer missions such as Solar Orbiter to consider full-disk mosaics in their observing plans for their SPICE spectrograph (see Müller et al., 2020), a plan that has already been tested over a fraction of the disk. The study of IRIS mosaics (Bryans et al., 2020) in turn has revealed chromospheric spectral signatures on a solar scale in coronal holes, that are not apparent from the analysis of smaller FOV, nor able to be determined from current full-disk instruments. These new signatures have the potential to address, as additional constraints, the uncertainties in defining the edges of coronal holes, a present challenge for global magnetic field extrapolations (see Linker et al., 2021), that among other uses drive the global MHD models introduced earlier. These topological uncertainties result in a difficulty in unambiguously establishing the connectivity between the solar sources of activity and the space weather counterparts (e.g. Rouillard et al., 2020; Parenti et al., 2021). Besides hindering the interpretation of the observations, it makes challenging to coordinate observatories with diagnostic capabilities on small FOV, e.g., current coordinated observing programs between Hinode, IRIS and Solar Probe and Solar Orbiter. Coordination is not a problem today for SDO/AIA and we advocate this should not be the problem for the next-generation spectrograph. However, SDO/AIA cannot provide the plasma diagnostics for the roots of on-disk activity that small-FOV spectrographs can collect, and we advocate this should not be a limitation for the next-generation full-disk observatory.
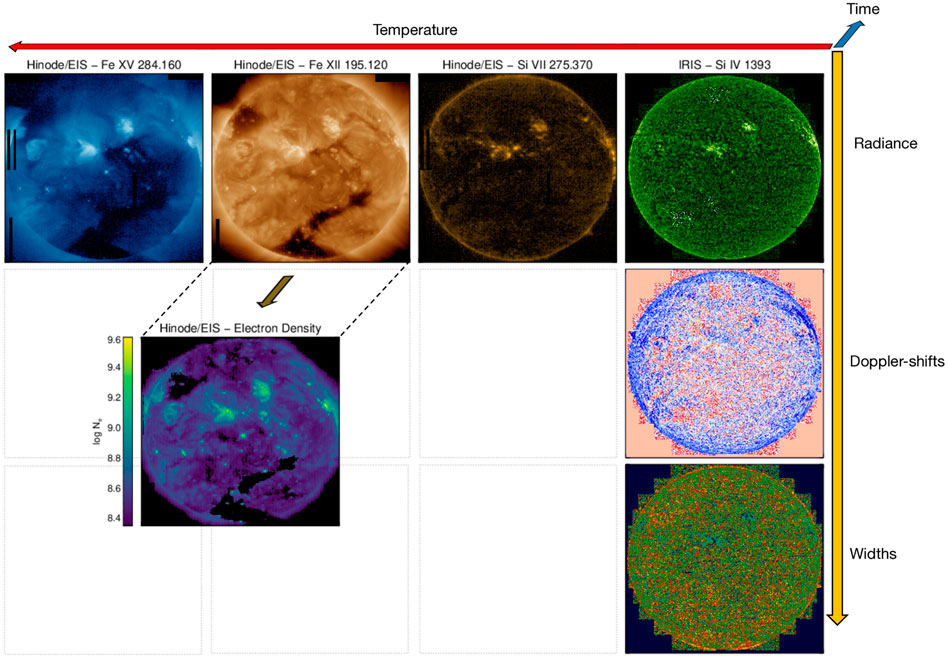
FIGURE 1. Representation of a sample of the multi-dimensional data products that a spatially resolved full-disk spectrograph would provide. The spectral dispersion would sample different spectral lines formed at different temperatures. Analysis of the spectral profiles would return radiance, Doppler velocities and spectral widths. The electron density and element composition would be computed from the ratios of the radiance of selected lines. Finally, consecutive spectra would establish the temporal variability. These images have been generated with Hinode/EIS wide slit (40″) and IRIS (2″) mosaics.
Much of the excitement for a full-disk spectrograph would sit on its ability to improve our understanding of highly dynamic non-local phenomena, e.g., flares and CMEs, particularly if it can serve to predict their occurrence. This is not a far-fetched expectation on a 10-year timescale given our recent findings and the upcoming mission prospects. Signatures of energy build up leading to flares have been identified in the Doppler-shift and non-thermal broadening changes of coronal spectral lines in Hinode/EIS spectral rasters (Harra et al., 2009; Imada et al., 2014). Baker et al. (2022) describe composition changes in a sigmoid prior to eruption as a coronal mass ejection (CME). In IRIS spectra, observations of magnetic flux rope formation exhibit Doppler-shifts and an increase in the non-thermal velocities during the pre-flare phase (Cheng et al., 2015). In the context of a future mission scanning these spectral properties over the full disk, and the anticipation of large quantities of spectral data to search through for signatures of turbulent motions, flows or composition changes, a particularly interesting result comes from machine-learning (ML) data mining studies on IRIS spectra. They show that regions that are about to flare produce spectra that are distinguishable from non-flaring active regions allowing algorithms to be trained to identify them and even forecast activity (Panos et al., 2018; Panos and Kleint, 2020; Woods et al., 2021). This is an area of rapid development that holds much promise with the spectra obtained by missions today, but will also be the subject of intense focus for the upcoming narrowing FOV spectrographs to be launched during solar cycle 25.
Another potential avenue for breakthrough science is the contribution to the development of cross-disciplinary work with stellar observations. A field that now includes space weather in exoplanetary systems and the importance of understanding the ionizing radiation from the host star (Airapetian et al., 2020). Sun-as-a-star EUV spectra show signatures of processes that also occur on solar-like stars. To mention two examples, EUV line profiles show large asymmetries associated with CMEs (Yang et al., 2022), and the elemental composition of the corona evolves on solar-cycle time-scales (Brooks et al., 2017). Full-disk spectra are the only way to realistically disentangle the contributions to the formation of Sun-as-a-star spectra, that may come from multiple sources during periods of high solar activity; particularly relevant for highly active stars whose disks are expected to be covered in active regions.
4 Why next?
Why next? Why not now? The past decade in solar physics has been defined by our ability to obtain multi-temperature images of the solar atmosphere on-demand (everywhere, all the time), including the far-side of the Sun. An unprecedented capability that raises the stakes for what to appeal for from the coming one. Our response to that question is: spatially resolved full-disk spectral diagnostics of the EUV chromosphere-corona system, everywhere, all the time.
It could be argued that the current NASA development pipeline already addresses the need for diagnostics now, with two missions: Solar-C (EUVST) a Japanese mission with US and European participation currently in the Phase-B of development; and MUSE, a Medium-Class explorer, also currently in Phase-B. With both planned to launch around the half-way point (2026–2028) of the current Heliophysics Decadal survey, they will spearhead the investigation of the fundamental processes that lead to the formation of the solar atmosphere and the solar wind, and the processes that drive flares and eruptions. Solar-C (EUVST) is a traditional single slit spectrograph with an unprecedented comprehensive temperature coverage (20 kK–20 MK) at high spatial resolution (.4″ or 300 km) and cadence (≥.5 s). MUSE is an innovative grating spectrograph, with 37-slits, that will obtain EUV spectra and images with high resolution in space (.33–.4”) and time (1–4 s), providing a 100x improvement in spectral raster cadence. These missions will provide a novel understanding of 1) the fundamental processes energizing the atmosphere, and 2) its inter-relation with the observed spectral signatures (e.g., energy build-up, pre-flare reconnection), as well as new capabilities to produce very rapid plasma diagnostics of solar activity, and to data mine and classify that activity.
What the current NASA mission pipeline does not address yet is how it will translate that into an observatory that can provide a detailed characterization of the plasma conditions throughout the low corona to the full network of observatories investigating the heliospheric environment and its space weather consequences. In the white paper submitted to the Decadal Survey for Solar and Space Physics (Heliophysics) 2024–2033 (Ugarte-Urra et al., 2022), we propose that this concept, with the potential to be a cornerstone mission of the HSO, is developed in the current Decadal cycle and around the lessons learned from spectroscopic missions in the current pipeline, that include innovative strategies for faster integration of large FOV and comprehensive temperature coverage. Therefore, with the purpose to drive the community to participate organically in the development of that concept, the white paper requests the Decadal panel to make spatially resolved solar full-disk spectral diagnostics a high priority ranked goal for Heliophysics. Likewise, to facilitate progress in that process the white paper also requests that the panel recommends funding for a technology study to identify the best means for performing high-cadence, spatially-resolved spectroscopy over the full solar disk.
5 Discussion
It was beyond the purpose of the white paper to elaborate a full observational concept in detail. We envision, however, that a full-disk EUV spectrograph can be part of a larger mission concept that can develop from the identified priorities put forward by the community in the Decadal Survey. It is evident that to couple global scale dynamic plasma diagnostics with the global topological connectivity is essential to conceive a companion instrument/observatory that can establish the magnetic topology. If best results are expected from extrapolations from a force-free chromospheric boundary (e.g. Fleishman et al., 2017), full-disk chromospheric magnetograms are a good candidate product for the evolution of what the SDO/HMI photospheric magnetic counterpart is today to SDO/AIA.
The major challenge in a full-disk spectrograph concept is the combination spatial resolution and cadence. Below we describe possible implementations, highlighting two approaches that utilize current technology. The succinct descriptions below focus therefore on the slit choice and the exposure time.
1) A single-slit spectrometer to rapidly scan the disk. A long (full-disk length) and relatively wide slit (5″) coupled with short exposure time and detector readout (1 s) would yield a full-disk scan in around 6–7 min. This is sufficient for studying energy build-up prior to CMEs and large-scale atmospheric dynamics, but not for flare evolution.
2) A multi-slit approach potentially allows full-disk scans in 1 min or less (for 1 s cadence exposures), depending on the number of slits. However, wavelength coverage is limited, potentially compromising diagnostics for density and element composition.
There are other fundamental specifications in the design of a spectrograph, such as the spectral resolution or the spectral line selection. While these are evidently important to the science case of a specific instrument because they establish, among others, the velocity resolution, temperature coverage and diagnostic capabilities (density sensitive lines), the choices are not critical to the case of a full-disk observation. These choices can be made as part of the organic process to develop a full concept.
The two approaches above (see also Table 1) are conservative. The current Solar-C (EUVST) and MUSE definitions include observations at full resolution (.3–.4″) with exposures times of .5–5 s in active region targets. A full-disk spectrograph would address its science goals (spectral diagnostics + connectivity) at a more modest resolution of 1”—5”, with a pixel area (and photon counts) increase of 6–50, not considering the potential technological development in the efficiencies of the optical and electronic components. In the case of the missions above, the improvement in effective area was 10-fold with respect to the predecessors. The effective area factor incorporates contributions from the various design components, including the mirror size and reflectivity, grating efficiency, and quantum efficiency of the detectors.
There is a third approach, slitless spectroscopy, that allows to observe large FOV in a fast single exposure without scanning. The trade-off, as for multi-slit data, is that spatial and spectral information overlap on the detector requiring deconvolution techniques to extract physical parameters (Cheung et al., 2019; Winebarger et al., 2019). Slitless spectroscopy is an area of current interest in the field with multiple concepts in various stages of development: the recently launched sounding rockets ESIS (Kankelborg et al., 2019) and MaGIXS (Champey et al., 2022), CubIXSS/MOXSI a cubesat mission recently selected by NASA (Caspi et al., 2020), and COSIE (Golub et al., 2020), a concept to study coronal events to
A recent review on the future prospects for Solar EUV and Soft X-ray spectroscopy discusses all these and other instruments in further detail (Young, 2021). These efforts confirm the white paper’s thesis that there is a strong interest in the community to obtain spectral diagnostics of coronal phenomena at global scales. A mission concept along the lines described above should be considered a high priority in Heliophysics.
Data availability statement
The datasets presented in this study can be found in online repositories. The names of the repository/repositories and accession number(s) can be found below: IRIS full-disk mosaics: https://iris.lmsal.com/mosaic_index.html Hinode/EIS archive: https://data.darts.isas.jaxa.jp/pub/hinode/eis/level0/.
Author contributions
IU-U and PY contributed to conception of the idea for this manuscript. IU-U wrote the first draft of the manuscript. All authors contributed to manuscript revision, read, and approved the submitted version.
Funding
This material is based upon work supported by the NASA Hinode Program, and by the National Center for Atmospheric Research, which is a major facility sponsored by the National Science Foundation under Cooperative Agreement No. 1852977.
Acknowledgments
We would like to thank the referees for all the suggestions that helped improve the paper. Hinode is a Japanese mission developed and launched by ISAS/JAXA, with NAOJ as domestic partner and NASA and UKSA as international partners. It is operated by these agencies in co-operation with ESA and NSC (Norway). IRIS is a NASA small explorer mission developed and operated by LMSAL with mission operations executed at NASA Ames Research Center and major contributions to downlink communications funded by ESA and the Norwegian Space Centre.
Conflict of interest
Author CD was employed by the company Predictive Science Inc.
The remaining authors declare that the research was conducted in the absence of any commercial or financial relationships that could be construed as a potential conflict of interest.
Publisher’s note
All claims expressed in this article are solely those of the authors and do not necessarily represent those of their affiliated organizations, or those of the publisher, the editors and the reviewers. Any product that may be evaluated in this article, or claim that may be made by its manufacturer, is not guaranteed or endorsed by the publisher.
Footnotes
1IRIS full-disk mosaics: https://iris.lmsal.com/mosaic_index.html.
References
Airapetian, V. S., Barnes, R., Cohen, O., Collinson, G. A., Danchi, W. C., Dong, C. F., et al. (2020). Impact of space weather on climate and habitability of terrestrial-type exoplanets. Int. J. Astrobiol. 19, 136–194. doi:10.1017/S1473550419000132
Baker, D., Green, L. M., Brooks, D. H., Démoulin, P., van Driel-Gesztelyi, L., Mihailescu, T., et al. (2022). Evolution of plasma composition in an eruptive flux rope. ApJ 924, 17. doi:10.3847/1538-4357/ac32d2
Brooks, D. H., Baker, D., van Driel-Gesztelyi, L., and Warren, H. P. (2017). A Solar cycle correlation of coronal element abundances in Sun-as-a-star observations. Nat. Commun. 8, 183. doi:10.1038/s41467-017-00328-7
Brooks, D. H., Ugarte-Urra, I., and Warren, H. P. (2015). Full-Sun observations for identifying the source of the slow solar wind. Nat. Commun. 6, 5947. doi:10.1038/ncomms6947
Bryans, P., McIntosh, S. W., Brooks, D. H., and De Pontieu, B. (2020). Investigating the chromospheric footpoints of the solar wind. ApJ 905, L33. doi:10.3847/2041-8213/abce69
Caspi, A., Shih, A. Y., Warren, H., Winebarger, A. R., Woods, T. N., Cheung, C. M. M., et al. (2020). “The CubeSat imaging X-ray solar spectrometer (CubIXSS),” in AGU Fall Meeting Abstracts, SH048–0007.
Champey, P. R., Winebarger, A. R., Kobayashi, K., Athiray, P. S., Hertz, E., Savage, S., et al. (2022). The marshall grazing incidence X-ray spectrometer (MaGIXS). J. Astronomical Instrum. 11, 2250010. doi:10.1142/S2251171722500106
Cheng, X., Ding, M. D., and Fang, C. (2015). Imaging and spectroscopic diagnostics on the formation of two magnetic flux ropes revealed by SDO/AIA and IRIS. ApJ 804, 82. doi:10.1088/0004-637X/804/2/82
Cheung, M. C. M., De Pontieu, B., Martínez-Sykora, J., Testa, P., Winebarger, A. R., Daw, A., et al. (2019). Multi-component decomposition of astronomical spectra by compressed sensing. ApJ 882, 13. doi:10.3847/1538-4357/ab263d
Darnel, J. M., Seaton, D. B., Bethge, C., Rachmeler, L., Jarvis, A., Hill, S. M., et al. (2022). The GOES-R solar UltraViolet imager. Space weather. 20, e03044. doi:10.1029/2022SW003044
De Pontieu, B., Martínez-Sykora, J., Testa, P., Winebarger, A. R., Daw, A., Hansteen, V., et al. (2020). The multi-slit approach to coronal spectroscopy with the multi-slit solar explorer (MUSE). ApJ 888, 3. doi:10.3847/1538-4357/ab5b03
Delaboudinière, J. P., Artzner, G. E., Brunaud, J., Gabriel, A. H., Hochedez, J. F., Millier, F., et al. (1995). Eit: Extreme-ultraviolet imaging telescope for the SOHO mission. Sol. Phys. 162, 291–312. doi:10.1007/BF00733432
Downs, C., Warmuth, A., Long, D. M., Bloomfield, D. S., Kwon, R.-Y., Veronig, A. M., et al. (2021). Validation of global EUV wave MHD simulations and observational techniques. ApJ 911, 118. doi:10.3847/1538-4357/abea78
Fleishman, G. D., Anfinogentov, S., Loukitcheva, M., Mysh’yakov, I., and Stupishin, A. (2017). Casting the coronal magnetic field reconstruction tools in 3D using the MHD bifrost model. ApJ 839, 30. doi:10.3847/1538-4357/aa6840
Forland, B. C., Gibson, S. E., Dove, J. B., Rachmeler, L. A., and Fan, Y. (2013). Coronal cavity survey: Morphological clues to eruptive magnetic topologies. Sol. Phys. 288, 603–615. doi:10.1007/s11207-013-0361-1
Golub, L., Cheimets, P., DeLuca, E. E., Madsen, C. A., Reeves, K. K., Samra, J., et al. (2020). EUV imaging and spectroscopy for improved space weather forecasting. J. Space Weather Space Clim. 10, 37. doi:10.1051/swsc/2020040
Golub, L., Deluca, E., Austin, G., Bookbinder, J., Caldwell, D., Cheimets, P., et al. (2007). The X-ray telescope (XRT) for the hinode mission. Sol. Phys. 243, 63–86. doi:10.1007/s11207-007-0182-1
Harra, L. K., Williams, D. R., Wallace, A. J., Magara, T., Hara, H., Tsuneta, S., et al. (2009). Coronal nonthermal velocity following helicity injection before an X-class flare. ApJ 691, L99–L102. doi:10.1088/0004-637X/691/2/L99
Heinemann, S. G., Jerčić, V., Temmer, M., Hofmeister, S. J., Dumbović, M., Vennerstrom, S., et al. (2020). A statistical study of the long-term evolution of coronal hole properties as observed by SDO. A&A 638, A68. doi:10.1051/0004-6361/202037613
Imada, S., Bamba, Y., and Kusano, K. (2014). Coronal behavior before the large flare onset. PASJ 66, S17. doi:10.1093/pasj/psu092
Inglis, A. R., Ireland, J., Shih, A. Y., and Christe, S. D. (2021). Evaluating pointing strategies for future solar flare missions. Sol. Phys. 296, 153. doi:10.1007/s11207-021-01896-0
Kankelborg, C. C., Parker, J. D., Smart, R., Winebarger, A. R., Kobayashi, K., Rachmeler, L., et al. (2019). “First flight of the EUV snapshot imaging spectrograph,” in AGU Fall Meeting Abstracts, SH33A–05.
Lemen, J. R., Title, A. M., Akin, D. J., Boerner, P. F., Chou, C., Drake, J. F., et al. (2012). The atmospheric imaging assembly (AIA) on the solar dynamics observatory (SDO). Sol. Phys. 275, 17–40. doi:10.1007/s11207-011-9776-8
Linker, J. A., Heinemann, S. G., Temmer, M., Owens, M. J., Caplan, R. M., Arge, C. N., et al. (2021). Coronal hole detection and open magnetic flux. ApJ 918, 21. doi:10.3847/1538-4357/ac090a
Madjarska, M. S. (2019). Coronal bright points. Living Rev. Sol. Phys. 16, 2. doi:10.1007/s41116-019-0018-8
Mikić, Z., Downs, C., Linker, J. A., Caplan, R. M., Mackay, D. H., Upton, L. A., et al. (2018). Predicting the corona for the 21 August 2017 total solar eclipse. Nat. Astron. 2, 913–921. doi:10.1038/s41550-018-0562-5
Müller, D., Cyr, St.O. C., Zouganelis, I., Gilbert, H. R., Marsden, R., Nieves-Chinchilla, T., et al. (2020). The Solar Orbiter mission. Science overview. A&A 642, A1. doi:10.1051/0004-6361/202038467
Panos, B., Kleint, L., Huwyler, C., Krucker, S., Melchior, M., Ullmann, D., et al. (2018). Identifying typical Mg II flare spectra using machine learning. ApJ 861, 62. doi:10.3847/1538-4357/aac779
Panos, B., and Kleint, L. (2020). Real-time flare prediction based on distinctions between flaring and non-flaring active region spectra. ApJ 891, 17. doi:10.3847/1538-4357/ab700b
Parenti, S., Chifu, I., Del Zanna, G., Edmondson, J., Giunta, A., Hansteen, V. H., et al. (2021). Linking the sun to the heliosphere using composition data and modelling. Space Sci. Rev. 217, 78. doi:10.1007/s11214-021-00856-1
Parenti, S. (2014). Solar prominences: Observations. Living Rev. Sol. Phys. 11, 1. doi:10.12942/lrsp-2014-1
Raouafi, N. E., Patsourakos, S., Pariat, E., Young, P. R., Sterling, A. C., Savcheva, A., et al. (2016). Solar coronal jets: Observations, theory, and modeling. Space Sci. Rev. 201, 1–53. doi:10.1007/s11214-016-0260-5
Reale, F. (2014). Coronal loops: Observations and modeling of confined plasma. Living Rev. Sol. Phys. 11, 4. doi:10.12942/lrsp-2014-4
Rouillard, A. P., Pinto, R. F., Vourlidas, A., De Groof, A., Thompson, W. T., Bemporad, A., et al. (2020). Models and data analysis tools for the Solar Orbiter mission. A&A 642, A2. doi:10.1051/0004-6361/201935305
Schrijver, C. J., and Higgins, P. A. (2015). A statistical study of distant consequences of large solar energetic events. Sol. Phys. 290, 2943–2950. doi:10.1007/s11207-015-0785-x
Schrijver, C. J., Title, A. M., Yeates, A. R., and DeRosa, M. L. (2013). Pathways of large-scale magnetic couplings between solar coronal events. ApJ 773, 93. doi:10.1088/0004-637X/773/2/93
Shimizu, T., Imada, S., Kawate, T., Ichimoto, K., Suematsu, Y., Hara, H., et al. (2019). “The Solar-C_EUVST mission,” in In UV, X-Ray, and Gamma-Ray Space Instrumentation for Astronomy XXI of Society of Photo-Optical Instrumentation Engineers (SPIE) Conference Series, 11118, 1111807. doi:10.1117/12.2528240
Toriumi, S., and Wang, H. (2019). Flare-productive active regions. Living Rev. Sol. Phys. 16, 3. doi:10.1007/s41116-019-0019-7
Ugarte-Urra, I., Young, P. R., Warren, H. P., De Pontieu, B., Bryans, P., Reep, J. W., et al. (2022). The case for solar full-disk spectral diagnostics. Submitted to BAA
Webb, D. F., and Howard, T. A. (2012). Coronal mass ejections: Observations. Living Rev. Sol. Phys. 9, 3. doi:10.12942/lrsp-2012-3
Winebarger, A. R., Weber, M., Bethge, C., Downs, C., Golub, L., DeLuca, E., et al. (2019). Unfolding overlapped slitless imaging spectrometer data for extended sources. ApJ 882, 12. doi:10.3847/1538-4357/ab21db
Woods, M. M., Sainz Dalda, A., and De Pontieu, B. (2021). Unsupervised machine learning for the identification of preflare spectroscopic signatures. ApJ 922, 137. doi:10.3847/1538-4357/ac2667
Wuelser, J.-P., Lemen, J. R., Tarbell, T. D., Wolfson, C. J., Cannon, J. C., Carpenter, B. A., et al. (2004). “Euvi: The STEREO-SECCHI extreme ultraviolet imager,” in Telescopes and instrumentation for solar astrophysics of society of photo-optical instrumentation engineers (SPIE) conference series. Editors S. Fineschi, and M. A. Gummin, 5171, 111–122. doi:10.1117/12.506877
Yang, Z., Tian, H., Bai, X., Chen, Y., Guo, Y., Zhu, Y., et al. (2022). Can we detect coronal mass ejections through asymmetries of sun-as-a-star extreme-ultraviolet spectral line profiles? ApJS 260, 36. doi:10.3847/1538-4365/ac6607
Keywords: Sun, solar corona, instrumentation, extreme ultraviolet, spectroscopy, full-disk
Citation: Ugarte-Urra I, Young PR, Brooks DH, Warren HP, De Pontieu B, Bryans P, Reep JW, Downs C, Winebarger AR and Tun SD (2023) The case for solar full-disk spectral diagnostics: Chromosphere to corona. Front. Astron. Space Sci. 9:1064192. doi: 10.3389/fspas.2022.1064192
Received: 07 October 2022; Accepted: 12 December 2022;
Published: 04 January 2023.
Edited by:
Philip J. Erickson, Massachusetts Institute of Technology, United StatesReviewed by:
R. Gafeira, Instituto de Astrofísica e Ciências do Espaço (IA), PortugalCopyright © 2023 Ugarte-Urra, Young, Brooks, Warren, De Pontieu, Bryans, Reep, Downs, Winebarger and Tun. This is an open-access article distributed under the terms of the Creative Commons Attribution License (CC BY). The use, distribution or reproduction in other forums is permitted, provided the original author(s) and the copyright owner(s) are credited and that the original publication in this journal is cited, in accordance with accepted academic practice. No use, distribution or reproduction is permitted which does not comply with these terms.
*Correspondence: Ignacio Ugarte-Urra, ignacio.ugarte-urra@nrl.navy.mil