- 1Center for Space Physics, Boston University, Boston, MA, United States
- 2LATMOS/IPSL, Guyancourt, Paris, France
- 3Space Research Institute of Russian Academy of Sciences, Moscow, Russia
- 4Johns Hopkins University Applied Physics Laboratory, Laurel, MD, United States
- 5University of Colorado Boulder, Boulder, CO, United States
Understanding the role of neutral atoms in the heliospheric interface (between the bow shock and heliopause) is critical to identifying dynamics within our local bubble. This article recommends building upon existing measurements with new, high-spectral resolution observations of H Lyman-α, from a heliospheric mapping mission, to resolve the momentum exchange in reactions between the solar wind and energetic neutral atoms that are key to identifying the most important heliospheric processes.
Introduction
The motion of the Solar System through the interstellar medium (ISM) carves out a cavity in the plasma known as the heliosphere. Voyagers 1 and 2 (V1 and V2) and Interstellar Boundary Explorer (IBEX) (Broadfoot et al., 1977; McComas et al., 2009) missions have revolutionized our understanding of the physics of the heliospheric interface and the interactions of stars with the interstellar medium. V1 observations showed a perplexing region of near-zero radial plasma velocity upstream of the heliopause within 10–15 AU, where the solar wind slows (Krimigis et al., 2011), reaching a “stagnation region.”
There have been several explanations for the existence of the stagnation region, such as momentum loss due to charge–exchange reactions of ions with interstellar H atoms that collectively decelerate the inner heliosheath solar wind (Lallement et al., 2012). An alternative explanation suggests that the solar wind magnetic field reversal alters the flow abruptly as the radial velocity of solar wind becomes zero or negative (Opher et al., 2012). However, current models and theories of observed flows do not reconcile with this (either in magnitude or direction (Opher et al., 2015)), highlighting our lack of understanding of the nature of the heliosheath.
V1 data show a constant Lyman-α intensity measured over ∼90–115 AU from the Sun, where one expects a decreasing brightness with increasing distance due to the decreasing solar flux. Voyager and New Horizons both showed an excess of 20–25 R in the Lyman-α emission not predicted by current models (Katushkina et al., 2017). This excess is not absorbed by interplanetary hydrogen (IPH); therefore, it must consist of high-velocity atoms outside the IPH absorbing spectral range. Such a phenomenon could be attributed to a Doppler-shifted population of H atoms with a position and thickness that are consistent with the stagnation region. One explanation for this is to invoke a dense layer of hydrogen at 115–122 AU. This hydrogen population has not been comprehensively considered in models of the heliosphere (Zank et al., 2013).
The explanation for the excess Lyman-α emission considers a significant contribution from the galactic background (Lallement et al., 2011). The emission can be produced by ionization and subsequent recombination of ISM H atoms either by stellar UV radiation in H+ regions and/or by collisions in interstellar shocks and should be concentrated near the galactic plane. Its spectrum and intensity are key parameters to understand the ionizing radiation field in our galaxy, to understand its application as an indicator of star formation rates that can be extrapolated to inform us of other galaxies, and to understand the amount of shocked gas in the ISM. It is also key to identifying the interaction of H Lyman-α emission with the well-studied H 21 cm radiation through the Wouthuysen–Field effect. Recent work has set an upper limit to the galactic emission intensity of 40 Rayleigh from modeling of various spacecraft data (Quémerais et al., 2003). This emission should exhibit two broad peaks (up to 2–3 Å wide) on either side of a saturated core. This theoretical hydrogen population would create emissions on the edge of the red wing spectrum of the IPH line via the resonant scattering of solar Lyman-α.
Proposed solutions
The inconsistencies in total H emission brightness between observations and models resulted in a theory of the various populations of neutral H atoms in our heliosphere (Figure 1). An IPH emission in the range of 800–1,000 R at 1 AU (lower value near solar minimum) would include multiple spectral components corresponding to a primary interstellar population, with a bulk velocity of 26 km/s and a temperature close to 7,000 K; a secondary population created by charge exchange in the outer heliosheath, with a bulk velocity of 22 km/s and a temperature around 12,000 K; and a tertiary component red-shifted from the main line with a brightness of 25 R.
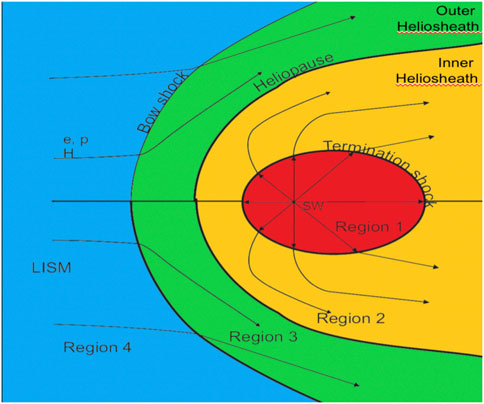
FIGURE 1. Regions of the heliosphere where H atoms are created through the charge exchange reaction with protons include local interstellar medium region (blue) at 26 km/s, 7,000 K, outer heliosheath region (green) at 22 km/s, 12,000 K, inner heliosheath region (yellow) at 180,000 K, and supersonic solar wind region (red) at ∼400 km/s (not observable with UV spectroscopy as they are beyond the Doppler width of solar illuminating flux). Figure adapted from (Izmodenov, 2007).
These inconsistencies around the heliospheric interface can be observationally constrained by new approaches to observations, namely, from high spectral resolution UV measurements that build on knowledge gained from existing UV mapping of H Lyman-α across the sky. This article motivates closing the knowledge gaps by considering a high-resolution spectrometer (∼3 km/s) (Katushkina et al., 2017) to be flown on a mission to map the heliosphere at H Lyman-α. Such a spectrometer would be effective when placed at various locations (e.g., low Earth orbit or the L1 Lagrange point) and would be optimal on a cross-solar system mission, where IPH properties would vary as the measurements progress from inner to outer heliospheric distances. The resulting measurements would be capable of resolving the momentum exchange in the reactions between the solar wind and energetic neutral atoms (ENAs) which are key to identifying the most important processes and how solar wind flow is modified. This can be carried out by measuring the velocity spectrum of interplanetary hydrogen (IPH) atoms in the Lyman-α emission spectral profile. This science is synergistic with IMAP observations of ENAs.
The inflowing ISM neutral atoms can be observed through the resonant scattering of solar H Lyman-α emission to determine both the line of sight speed and the velocity distribution of the inflowing atoms after modification by charge exchange in the interface region (Lallement et al., 1993). These observable characteristics have been used to measure the flow speed of H and He atoms and to identify line broadening associated with the charge exchange reactions in the interface region (Clarke et al., 1995). The inflowing IPH atoms are known to be a combination of unmodified ISM atoms and a second population modified by charge exchange between ISM neutrals and interstellar protons in the interface region.
Science questions
The neutral IPH emissions from the resonant scattering of solar Lyman-α have been mapped across the sky for decades. These maps, coupled with models, have led to valuable findings about the nature of the ISM flow through the heliospheric interface and of interactions with the solar wind. However, the spectral shape of the IPH emission is currently unknown and holds key information about the properties and various interactions of the different populations of H atoms.
- What is the hydrogen atom number density in the local interstellar medium?
- What is the hydrogen distribution in the outer heliosheath?
- What is the hydrogen hot population distribution in the inner heliosheath?
- How is momentum exchanged between these populations?
- What do these properties signify about the interactional dynamics of the three populations?
High-resolution spectra, simulated in Figure 2, would tackle these questions. The H number density from each population (LISM, outer heliosphere, and inner heliosphere) can be separated from the galactic emission with a resolution of 3–5 km/s. The line profile measurements would provide the distribution of atoms from each population, thereby quantifying the number density, velocity distribution, temperature, and, subsequently, the momentum exchange in the reactions that identify how the flow is modified.
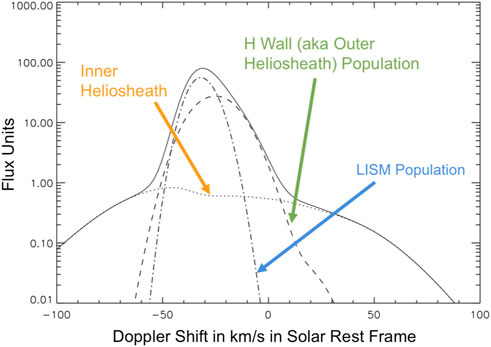
FIGURE 2. High-resolution line profile of IPH, in km/s, for an observer at 1 AU, looking upwind. The populations contributing to the total (solid) line shape are the inner heliosheath atoms (dotted), the outer heliosheath atoms (dash), and the local interstellar medium atoms (dash-dot). Supersonic solar wind H population moves at ∼400 km/s and is not observable with UV spectroscopy as they are Doppler-shifted beyond the width of solar illuminating flux. Figure adapted from Quémerais and Izmodenov (2002).
Conclusion
The recommendation of this article is to support the development and deployment-to-space of a high-resolution spectrograph in order to distinguish the three populations of H atoms that directly interact at the interface of the heliosheath, the region where the solar wind is subsonic. A required resolution of 3 km/s at H Lyman-α would suffice for spectrally resolving the line emissions from the local interstellar medium (LISM) and inner and outer heliosheath populations, allowing characterization of these populations and their interactions from 1–1,000 AU. This new science would be highly suitable for a future interstellar probe mission and would directly complement observations from the two Voyagers, IBEX, IMAP, and New Horizons (Brandt et al., 2022; McNutt et al., 2022). The recommended science yield would directly support NASA’s goals of understanding how the solar wind behaves near Earth, how the heliosphere interacts with the interstellar medium, and determining what the boundaries of the heliosphere look like.
Data availability statement
The original contributions presented in the study are included in the article/Supplementary Material; further inquiries can be directed to the corresponding author.
Author contributions
All authors listed have made a substantial, direct, and intellectual contribution to the work and approved it for publication.
Acknowledgments
The author wishes to acknowledge the contributions of co-authors.
Conflict of interest
The authors declare that the research was conducted in the absence of any commercial or financial relationships that could be construed as a potential conflict of interest.
Publisher’s note
All claims expressed in this article are solely those of the authors and do not necessarily represent those of their affiliated organizations, or those of the publisher, the editors, and the reviewers. Any product that may be evaluated in this article, or claim that may be made by its manufacturer, is not guaranteed or endorsed by the publisher.
References
Brandt, P. C., Provornikova, E. A., Cocoros, A., Turner, D., DeMajistre, R., Runyon, K., et al. (2022). 'Interstellar probe: Humanity's exploration of the galaxy begins. Acta Astronaut. 199, 364–373. doi:10.1016/j.actaastro.2022.07.011
Broadfoot, A. L., Sandel, B. R., Shemansky, D. E., Atreya, S. K., Donahue, T. M., Moos, H. W., et al. (1977). Ultraviolet spectrometer experiment for the voyager mission. Space science reviews, 21experiment for the voyager mission. Space Sci. Rev. 21, 183183–205205.
Clarke, J. T., Lallement, R., Bertaux, J.-L., and Quémerais, E. (1995). HST/GHRS observations of the interplanetary medium downwind and in the inner solar system. Astrophys. J. 448, 893–904. doi:10.1086/176018
Izmodenov, V. (2007). Filtration of interstellar atoms through the Heliospheric Interface. Sp. Sc. Rev. 130, 377–387. doi:10.1007/s11214-007-9203-5
Katushkina, O., Quemerais, E., Izmodenov, V. V., Lallement, R., and Sandel, B. R. (2017). Voyager 1/UVS lyman α measurements at the distant heliosphere (90-130 AU): Unknown source of additional emission. J. Geophys. Res. Space Phys. 122. doi:10.1002/2017JA024205
Krimigis, S. M., Roelof, E. C., Decker, R. B., and Hill, M. E. (2011). Zero outward flow velocity for plasma in a heliosheath transition layer. Nature 474, 359–361. doi:10.1038/nature10115
Lallement, R., Bertaux, J. L., and Clarke, J. T. (1993). Deceleration of interstellar hydrogen at the heliospheric interface. Science 260, 1095–1098. doi:10.1126/science.260.5111.1095
Lallement, R., Bertaux, J. L., Queḿ erais, E., and Sandel, B. R. (2012). Galactic cosmic rays measured by UVS on voyager 1 and the end of the modulation. Is the upwind heliopause a collapsed charge-exchange layer? Astron. Astrophys. 563, A108. doi:10.1051/0004-6361/201322705
Lallement, R., Quemerais, E., Bertaux, J. L., Sandel, B. R., and Izmodenov, V. (2011). Voyager measurements of hydrogen lyman-α diffuse emission from the milky way. Science 334, 1665–1669. doi:10.1126/science.1197340
McComas, D. J., Allegrini, F., , Bochsler, P., Bzowski, M., , Christian, E. R., Crew, G. B., et al. (2009). Global observations of the interstellar interaction from the interstellar boundary explorer (IBEX). Science 326, 959–962. doi:10.1126/science.1180906
McNutt, R. L., Wimmer-Schweingruber, R. F., Gruntman, M., Krimigis, S. M., Roelof, E. C., Pontus, C., et al. (2022). Interstellar probe - destination: Universe!. Acta Astronaut. 196, 13–28. doi:10.1016/j.actaastro.2022.04.001
Opher, M., Drake, J. F., Velli, M., Decker, R. B., and Toth, G. (2012). Near the boundary of the heliosphere: A flow transition region. Astrophys. J. 751, 80. doi:10.1088/0004-637x/751/2/80
Opher, M., Drake, J., Zieger, B., and Gombosi, T. (2015). Magnetized jets driven by the Sun: The structure of the heliosphere, ap. Astrophys. J. 800, L28. doi:10.1088/2041-8205/800/2/L28
Quémerais, E., Bertaux, J.-L., Lallement, R., Sandel, B. R., and Izmodenov, V. (2003). Voyager 1/UVS Lyman α glow data from 1993 to 2003: Hydrogen distribution in the upwind outer heliosphere. J. Geophys. Res. 108 (A10), 8029. doi:10.1029/2003JA009871
Quémerais, E., and Izmodenov, V. (2002). Effects of the heliospheric interface on the interplanetary Lyman-α glow seen at 1 AU from the Sun. Astron. Astrophys. 396, 269–281. doi:10.1051/0004-6361:20021396
Keywords: heliosphere, spectrograph, interplanetary hydrogen, Lyman alpha, Sun
Citation: Mayyasi M, Quémerais E, Katushkina O, Clarke J, Izmodenov V, Brandt P, Sokol J, Linsky J and Baliukin I (2022) Using Lyman-α to probe the interior and edges of the heliosphere. Front. Astron. Space Sci. 9:1063981. doi: 10.3389/fspas.2022.1063981
Received: 07 October 2022; Accepted: 17 October 2022;
Published: 11 November 2022.
Edited by:
Alexa Jean Halford, National Aeronautics and Space Administration, United StatesReviewed by:
Martin Snow, South African National Space Agency, South AfricaCopyright © 2022 Mayyasi, Quémerais, Katushkina, Clarke, Izmodenov, Brandt, Sokol, Linsky and Baliukin. This is an open-access article distributed under the terms of the Creative Commons Attribution License (CC BY). The use, distribution or reproduction in other forums is permitted, provided the original author(s) and the copyright owner(s) are credited and that the original publication in this journal is cited, in accordance with accepted academic practice. No use, distribution or reproduction is permitted which does not comply with these terms.
*Correspondence: Majd Mayyasi, majdm@bu.edu