- 1Skobeltsyn Institute of Nuclear Physics, Lomonosov Moscow State University, Moscow, Russia
- 2Space Research Institute RAS, Moscow, Russia
One of the main problems of modern magnetospheric physics is the lack of a self-consistent explanation of the main physical processes based on the laws of plasma physics. Among all the traditionally studied phenomena, the polar aurora stands out for being key to our understanding of several magnetospheric processes. In this manuscript, I would like to share with the younger generation my view about main auroral processes which I have developed during my career over the past 50 years.
Introduction
The year 2022 marks the 50th anniversary of my first publication in the field of magnetospheric physics. It was published while I was still a physics student at the Moscow State University. During that time, numerous satellite studies were carried out, and brilliant analytical and numerical models of various magnetospheric processes were developed. However, it was often difficult to achieve a real consensus among groups from different scientific centers or even groups within one center, to the point that until today, some basic magnetospheric processes are still heavily under debate. In this paper, and based on my personal experience and a number of examples, I show how the evolution of our knowledge about the aurora leads to the better understanding of the main magnetospheric phenomena, including the physics of geomagnetic storms and substorms.
The base of the work
My life in space physics began in the Division of Cosmic Rays and Space Physics at the Faculty of Physics of the Lomonosov Moscow State University (MSU) in 1970. I made the decision to attend the MSU when I was a student at a school with advanced teaching of mathematics in 1964, 2 years before my graduation. I had to accomplish a lot to get admitted to MSU. Although the education was free, the admission exams at MSU were hard and extremely competitive. More than eight applicants from all over the country were competing for each place at the Faculty of Physics in the year of my admission. The symbol of the Faculty of Physics was and remains the “root of the factorial” (√!), which means “look at the root.” This principle served as the foundation for all my subsequent work.
Each member of the Laboratory of Theoretical Space Physics dealt with his/her own problems and independently chose approaches and methods for solving them. My advisor Boris Arkadievich Tverskoy believed that it was necessary only to formulate the problem, and the student should come up with its final solution. Therefore, at the beginning, I did not understand what difficulties I would have to face during the initial stages of research. Tverskoy never explained how the problem could be solved and did not provide references to any work. This meant that we had to spend many hours every day in libraries. In addition, I remember well the following recommendation: with all due respect to the authors, do not read the sections containing the interpretation of the experimental results, look only at the figures. This is the only way to get the objective information. Through my career, I encountered numerous times proof of the validity of this approach. While I faced various difficulties at the early stage of my career associated with the almost complete independence provided (I managed to get B.A. Tverskoy attention no more than 5–10 min per month), that very independence has made me a real scientist. The Internet age has fundamentally changed the availability of scientific publications. Orders of magnitude more scientific articles are published now than at the beginning of my career, but almost each article contains way less new information. Here I fully agree with the assessment made in the recently published article by Borovsky (2022).
My goal during the postgraduate study was more straightforward. It was necessary to understand the nature of auroras and the mechanisms of auroral particle acceleration. I have to say, I keep working on this problem even now.
Since 1975, I have been working as a researcher at the Skobeltsyn Institute of Nuclear Physics (SINP). While working in theory, I always appreciated reliable empirical data and did my best to cultivate collaboration with experimentalists. Brilliant instrument designers E.N. Sosnovets, S.N. Kuznetsov, and many others, and excellent data analyzers, such as O.V. Khorosheva, worked at that time at SINP MSU. Olga Khorosheva (1961) compared simultaneous observations of all-sky cameras, and proved the existence of a continuous glow ring surrounding the pole that shifted to the night side, in contrast to the previously statistically obtained auroral zone. It was called the auroral oval (Feldshtein, 1963). Thus, Olga Khorosheva became my first mentor in the field of polar aurora. The well-known scientists Velior Shabansky and Yuri Galperin became my PhD thesis opponents. At numerous national conferences, I met almost all famous space scientists who worked at that time in the USSR and many of their students. It was possible to have discussions with Valeria Troitskaya, the first president of the International Association of Geomagnetism and Aeronomy (IAGA), Viktor Trakhtengerts, Mikhail Pudovkin and other famous scientists. The beginning of the INTERBALL project brought together many scientists from the USSR and abroad and gave me an opportunity to get acquainted with Albert Galeev and his follower Lev Zelenyi. Since then, I, like many my colleagues, have been working part-time at the Space Research Institute of the Russian Academy of Sciences (IKI RAN). A separate page should be dedicated to the enormous efforts of Lev Zeleny (later a member of the Russian Academy of science) in the 90s to support and save local space physicists in this difficult time after the collapse of the Soviet Union. Since then, I have been one of his friends. Anatoly Petrukovich, who replaced Zelenyi as the director of IKI, continued the effort to unite and support all specialists in our field.
I have traveled a lot to scientific conferences, first within my country, and since the late 80s, internationally as well. This provided me with a unique opportunity to meet the world’s leading scientists. Communication with the elite of Space physics community was extremely beneficial to my work. The most fruitful were acquaintances with David Sibeck, Joe Borovsky, Tony Lui, Larry Lyons, Patrick Newell, Maha Ashour-Abdalla and many other well-known scientists in the field of magnetospheric physics. Particularly noteworthy is my acquaintance with sansei Syun-Ichi Akasofu, whose approach (Akasofu, 2022) is very similar to one that I have developed over the years of analyzing magnetospheric processes.
My ideas about the structure of the auroral oval and the persistent patterns of its dynamics changed after I met the unique observer of polar lights Galina Kirillovna Nazarchuk and worked with her for almost a month at the Tiksi geomagnetic observatory of the Institute of Cosmophysical Research and Aeronomy (IKFIA). I have seen with my own eyes what is called a diffuse aurora. In difference to the common view, it consists of thin strips with almost constant thickness of ∼2 km. However, these structures can only be seen when the aurora band is at the magnetic zenith. This phenomenon has been barely studied. I discussed it in (Antonova et al., 1999), where the existing evidence of the non-magnetized nature of the motion of auroral electrons in the region at the top of the magnetic field line was collected. Thin discrete auroral draperies were observed almost every night in Tiksi. A curtain consists of thin beams; the thickness of each is more than two orders of magnitude less than its vertical extent. When such a structure passes through the magnetic zenith, a corona-like aurora is observed due to the foreshortening effect. The main thing that we managed to see is the real projection of magnetospheric turbulence on ionospheric altitudes (rapid movements, appearance and disappearance of structures) during the 10–11 March 1979 magnetic storm.
Acceleration of electrons and ions in the auroral oval as the result of medium scale electric field dynamics
My first two papers, published in 1972, were focused on theoretical analysis of the properties of Pc1 and Pi2 micro pulsations. Therefore, from the start of my career, I have been interested in working on the ring current (source of Pc1) and magnetospheric substorms, leading to the excitation of Pi2 at the time of the substorm onset. It was clear that the processes of the appearance of substorms and the formation of a ring current are very closely related (substorm injections form a ring current). However, the situation began to clear up only in the last decade, when we obtained evidence that the main part of the auroral oval is not mapped into the plasma sheet, but into the outer part of the ring current (Antonova et al., 2014; Antonova et al., 2018a).
Field-aligned acceleration of auroral electrons
My PhD thesis “Passage of strong magnetospheric currents through the ionosphere and acceleration of electrons in high-latitude regions”, defended in 1975, provides a solution to the problem of the dependence of the field-aligned currents flowing from the ionosphere on the field-aligned potential difference. A simple dependence, which later received the name Knigth relation (Knight, 1973) in the literature, was obtained at the very beginning of my study, but was not immediately published since it was required to describe the mechanisms for creating a field-aligned potential drop. I did not like the widely discussed mechanism of generation of a field-aligned electric field due to the appearance of anomalous resistivity, since the occurrence of finite resistivity in a collisionless magnetospheric plasma meant the occurrence of a very rapid heating of the plasma, i.e., the rapid emergence of a non-equilibrium situation. The heated plasma with the development of anomalous resistivity should be quickly transported away from the heating region. Therefore, it was impossible to explain the existence of a relatively stable auroral structure in which electrons were observed to accelerate to energies much higher than thermal ones. The kinetic description, based on the analysis of the motion of particles at a fixed potential drop along the magnetic field lines, for a given distribution function of electrons and ions in the area at the top of the field line and at ionospheric altitudes, led to a violation of plasma quasi-neutrality. Such violation contradicted the definition of plasma described in the first pages of each plasma textbook. The time of postgraduate studies was coming to an end, yet it was impossible to obtain a quasi-neutral solution of the problem. There was an agonizing period of troubleshooting until an article was found in the journal Cosmic Electrodynamics (Carlqvist, 1972) that described the double diode (Langmuir, 1929) and talked about the possibility of violation of quasi-neutrality in plasma. The resulting relation between the value of the field-aligned current and the field-aligned potential drop was used in my PhD thesis to describe the structure of the ionosphere in the auroral region. Articles (Antonova and Tverskoy, 1975a; Antonova and Tverskoy, 1975b) were published and, despite the fact that publications in the journal Geomagnetism and Aeronomy were not translated into English at that time, were cited in a review (Paschmann et al., 2002). It turned out to be really successful. It was possible to make a change in the definition of plasma, from which the quasi-neutrality disappeared. The large-scale double layer model predicted a sharp drop in the plasma density in the acceleration region compared to neighboring regions. The discovery of the magnetospheric cavity (Calvert, 1981) confirmed this prediction of the model. During this period, many models of double layers of different nature and configuration were published. The problem has not been completely solved to date, but the existence of large potential variations along auroral field lines has been fully proven, which greatly limits the applicability of the frozen-in approximation in describing magnetospheric processes. Later, it became clear that the existence of the field-aligned potential drops was recognized by practically all magnetospheric scientists. Other auroral observations (see Chapter 4 of Paschmann et al., 2002) had different controversial explanations and additionally had difficulties in connecting the aurora with the plasma sheet phenomena which is ordinarily considered as the main source of auroral phenomena.
According to Akasofu (1964) and numerous subsequent works, a substorm expansion phase starts with a brightening of the equatorward auroral arc or with the appearance of a new arc. Therefore, it was very interesting to understand how auroral arcs are formed and the factors that contribute to why the arc becomes brighter at the moment of the onset. The theory developed in (Antonova, 1993) considered the stability of the azimuthal distribution of the magnetospheric plasma pressure and large-scale field-aligned currents during magnetosphere-ionosphere interactions. Arc brightening was considered as the result of the penetration of cold ionospheric plasma into the auroral cavity with field-aligned potential drop at its edge. Different studies (Stepanova et al., 2002; Antonova and Tverskoi, 1979; ets.) supported predictions of this theory including the absence of brightening and large magnetic field distortions before the onset. Many other scenarios of onset were developed in this period. However, the mechanism of brightening represents the real interest to date. In the late 1970s and early 1980s, none of the researchers doubted the validity of such a scenario for the appearance of thin auroral arcs observed at the edges of inverted V structures (Antonova, 1979; McFadden et al., 1986). It described the formation of a beam of cold electrons of ionospheric origin accelerated to energies (1–10 keV) with a density of ∼102 cm−3, the transverse energy of which is ∼several eV, less than the field-aligned energy by orders of magnitude. Figure 1 illustrates the mechanism in action. Such beams were observed on the Fast and DMSP auroral satellites (Mende et al., 2003; Shiokawa et al., 2005). Nonetheless, simultaneous fluctuations of oblique Alfvén waves led to the hypothesis of the wave nature of the acceleration of cold ionospheric electrons although the simulation results required also the action of the mechanism of electron penetration into the region of the field-aligned potential drop (Chaston et al., 2002). It was also obvious that the relaxation of the electron beam carrying the field-aligned current leads to the generation of a wide spectrum of waves, including Alfvén waves. Nevertheless, the term Alfvénic aurora has become established in the literature. Acceleration of powerful field-aligned electron beam was recognized as the main acting mechanism of acceleration. The mechanism of ionospheric plasma penetration in the region of field-aligned potential drop had some difficulties and later practically was not discussed as “a 2D simulation of auroral arc structure, with appropriate wave turbulence may be required to solve this problem (Paschmann et al., 2002, p. 165–166)”. Our paper using this mechanism for interpretation of auroral arc splitting and showing that the discussed mechanism difficulties can be illuminated, was rejected. It was only possible to publish an explanation of the generation of multiple arcs by single inverted V structure in our university journal (Antonova et al., 2012). So, in general, the situation should be considered as a failure. However, the situation may be revised in the future with the emergence of new experimental data and a more thorough analysis of existing ones.
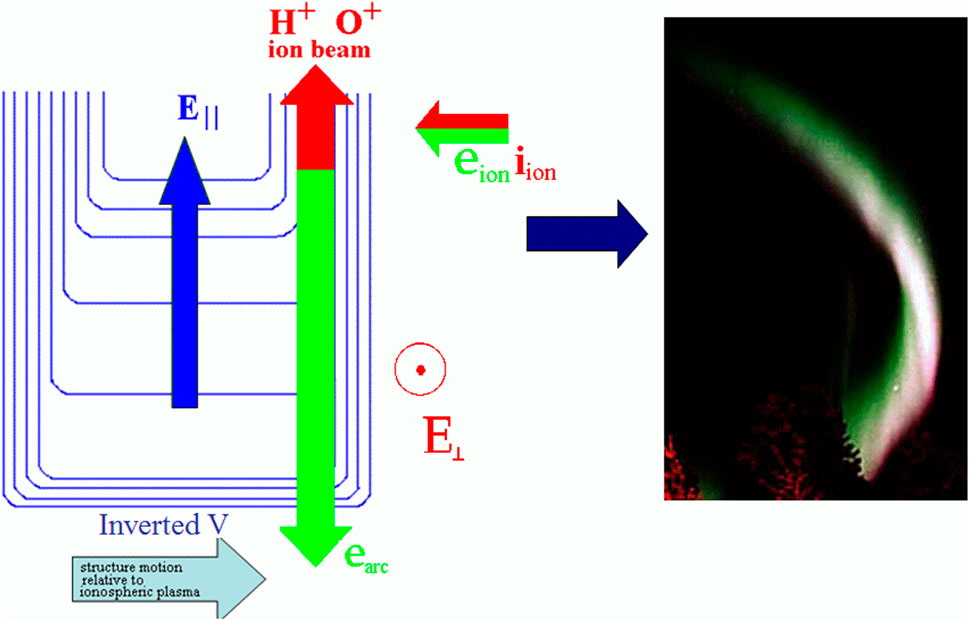
FIGURE 1. Formation of thin bright auroral arc at the boundary of the inverted V/U structure with particle flux J∼1011 cm−2s−1 due to penetration of cold ionospheric plasma inside the plasma cavity [“grader model” in accordance with (Antonova et al., 2012)].
Auroral ion acceleration
The acceleration of auroral electrons from the magnetosphere to the ionosphere resulted in the acceleration of ionospheric ions from the ionosphere to the magnetosphere, the formation of ion beams (Antonova, 1979), and the filling of the magnetosphere with ions of ionospheric origin, which has not been studied in detail until now. However, such a process did not allow one to explain the filling of the magnetosphere with heavy ions of ionospheric origin (mainly, O+ ions were considered) since the formation of ion beams during field-aligned acceleration occurred at sufficiently high altitudes (∼ the radius of the Earth). All-sky observations at Tiksi showed storm time fast motions of structures stretched along the magnetic field, which could lead to the action of a two-dimensional analogue of Fermi acceleration of the second order (Antonova, 1983). The developed mechanism described the formation of ion “conics” with distribution functions f ∼ exp (-V⊥/τ), where τ is the acceleration time. The mechanism explained the predominant acceleration of O+ ions compared to H+ ions up to acceleration to the same value of speed, simultaneous observations of ion-cyclotron waves, and well described the shape of the distribution function (Figure 2), but did not become popular. The dominant version was the acceleration due to interaction with cyclotron waves or broadband ELF waves (Paschmann et al., 2002) in spite of the existence of strong transverse electric fields in the auroral ionosphere and difficulties with the explanation of the predominant acceleration of heavy ions. Therefore, the situation was far from consensus. The well-developed mechanism of particle acceleration by simultaneously observed waves was more popular than the ordinary considered in laboratory plasma: if nonequilibrium distribution function is observed simultaneously with waves which can be generated due to its relaxation, it is necessary to search the mechanism of distribution functions formation different from interaction with observed waves.
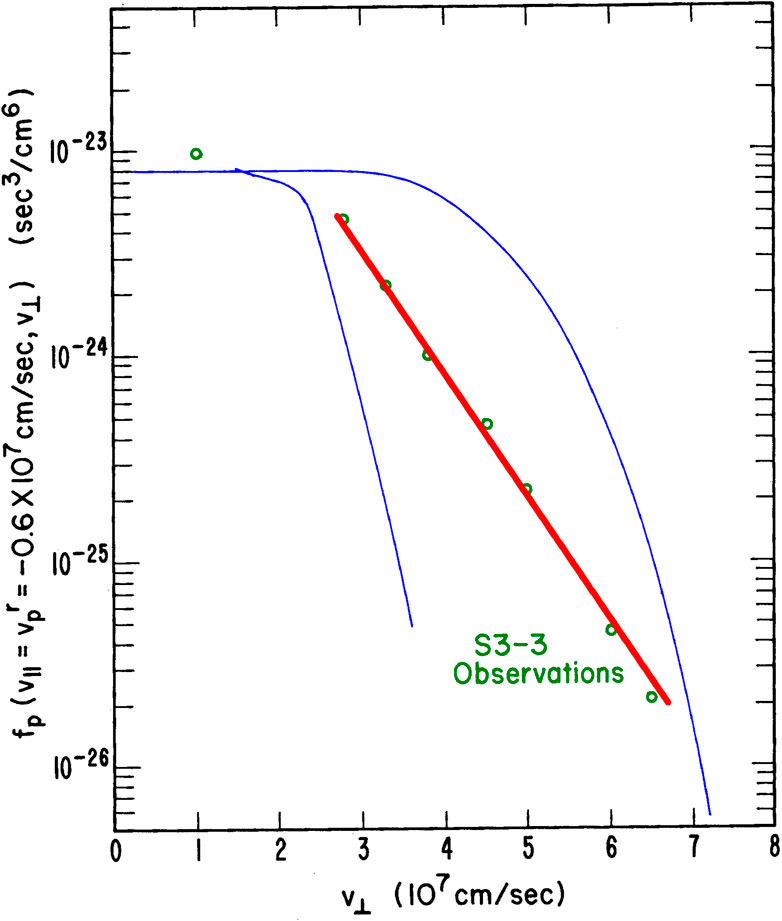
FIGURE 2. Comparison of experimentally measured conic type distribution function on S3-3 satellite on 11 October 1976, at 0046:06 UT (points) with the results of modelling. Blue curve show the results (Dusenbery and Lyons, 1981) model, red curve (Antonova, 1983) model (Antonova, 1987).
Relativistic electron acceleration
The same situation occurs with field-aligned electron beams and conics in the auroral plasma appeared with relativistic electrons of the outer radiation belt (ORB). The resonant wave-particle interaction mechanism was considered as the main acceleration mechanism is the very popular quasilinear mechanism of acceleration of by chorus waves. The mechanism could not explain the acceleration time (it required about a day, but in reality, this time was very short (Foster et al., 2017)) and the existence of magnetic storms, during which the storm ORB fluxes are restored to the pre-storm level (Antonova and Stepanova, 2015; Antonova et al., 2018b). Such situation appeared due to absence of the attention to substorm injections at low latitudes in the region of depressed magnetic field. Therefore we again have no consensus.
The possibility of finding more powerful mechanisms of particle acceleration than interaction with waves in the cases mentioned above is associated with the existence of large electrostatic and electromagnetic medium scale electric fields in a collisionless magnetospheric plasma and the development of magnetospheric turbulence. The obvious inapplicability of the frozen-in condition greatly complicates its description. At the same time, the observed balance of pressures at the magnetopause and across the plasma sheet (see the references in (Antonova and Stepanova, 2021)) makes it possible to obtain adequate solutions to existing problems, to obtain consensus and move towards more accurate Space Weather predictions than the current ones.
Conclusion and discussion
The main conclusion of our discussion, which can be useful for students and scientists at the beginning of their careers, is the possibility of revising even the well-known and analyzed processes and finding fundamentally new and more adequate explanations. Such results will lead to a consistent picture of magnetospheric phenomena and facilitate a consensus among various scientific groups. This will obviously require a lot of effort and a lot of work. Therefore, I want to wish the new generation of young scientists, quoting the famous American writer Mark Twain, “find a job you enjoy doing, and you will never have to work a day in your life.”
Data availability statement
The original contributions presented in the study are included in the article/Supplementary Material, further inquiries can be directed to the corresponding author.
Author contributions
The author confirms being the sole contributor of this work and has approved it for publication.
Acknowledgments
The author is forever grateful to all teaches and former students, coauthors and scientific opponents for attention and fruitful collaboration.
Conflict of interest
The author declares that the research was conducted in the absence of any commercial or financial relationships that could be construed as a potential conflict of interest.
Publisher’s note
All claims expressed in this article are solely those of the authors and do not necessarily represent those of their affiliated organizations, or those of the publisher, the editors and the reviewers. Any product that may be evaluated in this article, or claim that may be made by its manufacturer, is not guaranteed or endorsed by the publisher.
References
Akasofu, S.-I. (2022). When I encountered difficult problems. Front. Astron. Space Sci. 9, 879290. doi:10.3389/fspas.2022.879290
Akasofu, S. I. (1964). The development of the auroral substorm. Planet. Space Sci. 12, 273–282. doi:10.1016/0032-0633(64)90151-5
Antonova, E. E., Kirpichev, I. P., Ryazantseva, M. O., Maryin, B. V., Pulinets, M. S., Znatkovaa, S. S., et al. (2012). Magnetospheric substorms and discrete arcs of the polar aurora. Mosc. Univ. Phys. 67 (6), 500–507. doi:10.3103/S0027134912060033
Antonova, E. E. (1987). Magnetospheric turbulence and magnetospheric particle dynamics. Prepr. SIP MSU 87-005, 32.
Antonova, E. E. (1979). On the formation of the field-aligned potential difference, isotropic and collimated electron fluxes in the evening sector of the auroral magnetosphere. Geomagnetism Aeronomy 19 (6), 1064–1069. Translation.
Antonova, E. E., Stepanova, M., Kirpichev, I. P., Ovchinnikov, I. L., Vorobjev, V. G., Yagodkina, O. I., et al. (2018a). Structure of magnetospheric current systems and mapping of high latitude magnetospheric regions to the ionosphere. J. Atmos. Sol. Terr. Phys. 177, 103–114. doi:10.1016/j.jastp.2017.10.013
Antonova, E. E., Stepanova, M. V., Moya, P. S., Pinto, V. A., Vovchenko, V. V., Ovchinnikov, I. L., et al. (2018b). Processes in auroral oval and outer electron radiation belt. Earth Planets Space 70, 127. doi:10.1186/s40623-018-0898-1
Antonova, E. E., and Stepanova, M. V. (2021). The Impact of turbulence on physics of the geomagnetic tail. Front. Astron. Space Sci. 8, 622570. doi:10.3389/fspas.2021.622570
Antonova, E. E., and Stepanova, M. V. (2015). The problem of the acceleration of electrons of the outer radiation belt and magnetospheric substorms. Earth, Planets, Space 67, 148. doi:10.1186/s40623-015-0319-7
Antonova, E. E., Stepanova, M. V., Vikhreva, E. A., Ovchinnikov, I. L., and Teltsov, M. V. (1999). Generation of unmagnetized motion of plasma sheet electrons and its possible causes. J. Geophys. Res. 104 (A9), 19941–19953. doi:10.1029/1999JA900036
Antonova, E. E. (1993). The development of initial substorm expansive phase disturbance due to generation of localized electric fields in the region of maximum upward field-aligned current. Adv. Space Res. 13 (4), 261–264. doi:10.1016/0273-1177(93)90343-A
Antonova, E. E. (1983). The transverse acceleration of ionospheric ions induced by collisions with moving inhomogeneities. Geomagnetism Aeronomy 23 (5), 609–612. Translation.
Antonova, E. E., and Tverskoi, B. A. (1975a). Nature of the electron precipitation band of the 'inverted V' type and of the Harang discontinuity in the evening sector of the auroral ionosphere. Geomagnetism Aeronomy 15, 85–90. Translation.
Antonova, E. E., and Tverskoi, B. A. (1975b). On acceleration of auroral electrons by a stationary double layer. Geomagnetism Aeronomy 15, 453–454. Translation.
Antonova, E. E., and Tverskoi, B. A. (1979). On the role of the polar ionosphere as source of ions in the magnetosphere. Geomagnetism Aeronomy 19, 118–119. Translation.
Antonova, E. E., Vorobjev, V. G., Kirpichev, I. P., and Yagodkina, O. I. (2014). Comparison of the plasma pressure distributions over the equatorial plane and at low altitudes under magnetically quiet conditions. Geomagn. Aeron. 54, 278–281. doi:10.1134/S0016793214030025
Borovsky, J. E. (2022). Science: Through the years. Perspect. Earth Spac. 3, e2022CN000171. doi:10.1029/2022CN000171
Calvert, W. (1981). The auroral plasma cavity. Geophys. Res. Lett. 8 (8), 919–921. doi:10.1029/GL008i008p00919
Carlqvist, C. E. (1972). On the formation of double layers in plasma. Cosm. Electrodyn. 3 (3), 377–388.
Chaston, C. C., Bonnell, J. W., Peticolas, L. M., Carlson, C. W., and McFadden, J. P. (2002). Driven alfven waves and electron acceleration: A fast case study. Geophys. Res. Lett. 29 (11), 1535. doi:10.1029/2001GL013842
Dusenbery, P. B., and Lyons, R. L. (1981). Generation of ion-conic distribution by upgoing ionospheric electrons. J. Geophys. Res. 86 (A9), 7627–7638. doi:10.1029/JA086iA09p07627
Feldstein, Y. (1963). Some problems concerning the morphology of auroras and magnetic disturbances at high latitudes. Geomagnetism Aeronomy 3, 183–192. Translation.
Foster, J. C., Erickson, P. J., Omura, Y., Baker, D. N., Kletzing, C. A., and Claudepierre, S. G. (2017). Van Allen probes observations of prompt MeV radiation belt electron acceleration in nonlinear interactions with VLF chorus. J. Geophys. Res. Space Phys. 122 (1), 324–339. doi:10.1002/2016JA023429
Khorosheva, O. V. (1961). The space and time distribution of auroras and their relationship with high-latitude geomagnetic disturbances. Geomagnetism Aeronomy 1, 615–620. Translation.
Knight, S. (19731973). Parallel electric fields. Planet. Space Sci. 21 (5), 741–750. doi:10.1016/0032-0633(73)90093-7
Langmuir, I. (1929). The interaction of electron and positive ion space charges in cathode sheaths. Phys. Rev. 33 (6), 954954–954989. doi:10.1103/physrev.33.954
McFadden, J. P., Carlson, C. W., and Boehm, M. H. (1986). Field-aligned electron precipitation at the edge of an arc. J. Geophys. Res. 91, 1723–1730. doi:10.1029/JA091iA02p01723
Mende, S. B., Carlson, C. W., Frey, H. U., Peticolas, L. M., and Østgaard, N. (2003). FAST and IMAGE-FUV observations of a substorm onset. J. Geophys. Res. 108 (A9), 1344. doi:10.1029/2002JA009787
Paschmann, G., Haaland, S., and Treumann, R. (2002). Auroral plasma physics. Space Sci. Rev. 103. doi:10.1023/A:1023030716698
Shiokawa, K., Yago, K., Yumoto, K., Baishev, D. G., Solovyev, S. I., Rich, F. J., et al. (2005). Ground and satellite observations of substorm onset arcs. J. Geophys. Res. 110, A12225. doi:10.1029/2005JA011281
Keywords: magnetosphere, auroral particle acceleration, alfvénic aurora, magnetospheric turbulence, substorm, outer radiation belt
Citation: Antonova EE (2022) From physics of polar aurora to changes of the fundamental approaches to the physics of the magnetospheric processes. Front. Astron. Space Sci. 9:1045689. doi: 10.3389/fspas.2022.1045689
Received: 15 September 2022; Accepted: 21 October 2022;
Published: 01 November 2022.
Edited by:
Maria Usanova, University of Colorado Boulder, United StatesReviewed by:
Colin Forsyth, University College London, United KingdomJoseph E. Borovsky, Space Science Institute, United States
Lev Zelenyi, Space Research Institute (RAS), Russia
Copyright © 2022 Antonova. This is an open-access article distributed under the terms of the Creative Commons Attribution License (CC BY). The use, distribution or reproduction in other forums is permitted, provided the original author(s) and the copyright owner(s) are credited and that the original publication in this journal is cited, in accordance with accepted academic practice. No use, distribution or reproduction is permitted which does not comply with these terms.
*Correspondence: Elizaveta E. Antonova, ZWxpemF2ZXRhLmFudG9ub3ZhQGdtYWlsLmNvbQ==