- 1University of Chicago Department of the Geophysical Sciences, Chicago, IL, United States
- 2Department of Astronomy and Steward Observatory, University of Arizona, Tucson, AZ, United States
- 3Niels Bohr Institute, University of Copenhagen, Copenhagen, Denmark
- 4Department of Chemistry, Massachusetts Institute of Technology, Cambridge, MA, United States
- 5National Radio Astronomy Observatory, Charlottesville, VA, United States
- 6Department of Space, Earth and Environment with Onsala Space Observatory, Chalmers University of Technology, Göteborg, Sweden
- 7NASA Goddard Space Flight Center, Greenbelt, MD, United States
- 8LERMA, Observatoire de Paris, PSL Research University, CNRS, Sorbonne Université, Paris, France
- 9Max Planck Institute for Solar System Research, Goettingen, Germany
- 10Wyant College of Optical Sciences, University of Arizona, Tucson, AZ, United States
- 11NSF’s NOIRLab, Tucson, AZ, United States
- 12Max-Planck-Institut für Astronomie, Heidelberg, Germany
- 13Astronomy Department, University of Maryland, College Park, MD, United States
- 14Leiden Observatory, University of Leiden, Leiden, Netherlands
- 15Center for Astrophysics, Harvard and Smithsonian, Cambridge, MA, United States
Chemistry along the star- and planet-formation sequence regulates how prebiotic building blocks—carriers of the elements CHNOPS—are incorporated into nascent planetesimals and planets. Spectral line observations across the electromagnetic spectrum are needed to fully characterize interstellar CHNOPS chemistry, yet to date there are only limited astrochemical constraints at THz frequencies. Here, we highlight advances to the study of CHNOPS astrochemistry that will be possible with the Orbiting Astronomical Satellite for Investigating Stellar Systems (OASIS). OASIS is a NASA mission concept for a space-based observatory that will utilize an inflatable 14-m reflector along with a heterodyne receiver system to observe at THz frequencies with unprecedented sensitivity and angular resolution. As part of a survey of H2O and HD toward ∼100 protostellar and protoplanetary disk systems, OASIS will also obtain statistical constraints on the emission of complex organics from protostellar hot corinos and envelopes as well as light hydrides including NH3 and H2S toward protoplanetary disks. Line surveys of high-mass hot cores, protostellar outflow shocks, and prestellar cores will also leverage the unique capabilities of OASIS to probe high-excitation organics and small hydrides, as is needed to fully understand the chemistry of these objects.
1 Introduction
The elements carbon, hydrogen, nitrogen, oxygen, phosphorus, and sulfur (CHNOPS) are considered the main biogenic elements on Earth, as they are found universally in all life forms. Studying the chemistry of these elements along the star- and planet-formation sequence provides crucial insight into how they are incorporated into nascent planetesimals and planets (see review by Öberg and Bergin, 2021). Besides regulating the bulk inventories of CHNOPS in planet-forming gas and solids, interstellar chemistry can also convert simple CHNOPS carriers into more complex organic molecules. If this chemically complex material is incorporated into icy bodies such as asteroids and comets, then it may be delivered to planetary surfaces via impact and potentially play a role in jump-starting origins-of-life chemistry (e.g., Rubin et al., 2019). Indeed, icy bodies within the Solar System appear to be composed in part of material inherited from the early stages of star formation (e.g., Alexander et al., 2017; Altwegg et al., 2017; Rubin et al., 2020) and are also implicated in the delivery of volatiles to Earth’s surface (e.g., Alexander et al., 2012; Marty et al., 2017). Thus, understanding the formation and inheritance of simple and complex CHNOPS carriers along the star-formation sequence is a major aim of astrochemistry.
The Orbiting Astronomical Satellite for Investigating Stellar Systems (OASIS) observatory is a NASA Medium Explorer (MIDEX) space mission concept designed to “follow the water trail” from galaxies to oceans, by covering key rotational lines of H2O and HD at submillimeter wavelengths with unprecedented sensitivity and angular resolution (Arenberg et al., 2021). With its broad frequency coverage and tunability between 455 and 4,745 GHz, OASIS will also cover multitudes of spectral lines from CHNOPS carriers. As much of our current understanding of astrochemical complexity is based on observations at millimeter wavelengths (McGuire, 2018), OASIS will open a new window for studying the volatile chemistry along the star- and planet-formation sequence. In particular, OASIS will transform our understanding of the astrochemistry of hydrides (e.g., NHx, CHx, and OHx) and high-excitation lines of organic molecules.
In this work, our aim is to highlight knowledge gaps that OASIS will uniquely fill in our understanding of organic/prebiotic astrochemistry. For a discussion of HD and H2O science applications, we refer the reader to Walker et al. (2021). Section 2 presents a brief overview of the optical and technical capabilities of OASIS, how it compares to other state-of-the-art observing facilities, and the galactic star-forming regions it will observe. In Section 3, we describe how OASIS’s unique observational capabilities will advance the study of CHNOPS astrochemistry along the star- and planet-formation sequence, from prestellar cores to protoplanetary disks. Section 4 presents our summary and conclusions.
2 OASIS Overview
The OASIS mission concept is detailed in Walker et al. (2021). Here, we present a brief overview of the mission features most salient to the astrochemistry science use cases.
2.1 Technical Specifications
OASIS is a space-based observatory, which will be in a Sun–Earth L1 halo orbit. In the current design, OASIS utilizes an inflatable 14-m reflector, followed by aberration corrector optics achieving diffraction-limited optical performance, coupled to a state-of-the-art terahertz (THz) heterodyne receiver system (Takashima et al., 2021). This enables high sensitivity and high spectral resolving power (
2.2 Complementarity to Other Facilities
Figure 1 shows a comparison of the line sensitivity, spectral resolution, and angular resolution that OASIS will achieve, compared to those of other previous, existing, and future observational facilities. The spectral line sensitivity of OASIS is comparable to that of the state-of-the-art facilities ALMA and JWST and
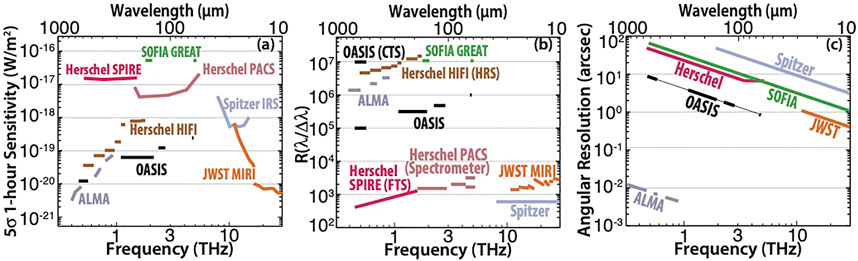
FIGURE 1. Comparison of (A) spectral line sensitivity, (B) spectral resolving power, and (C) angular resolution of OASIS with other state-of-the-art telescopes.
OASIS also has spectral coverage overlapping with the Origins Space Telescope, a potential future facility–class mission. As proposed, Origins is a 5.9-m cryogenic telescope with three scientific instruments operating in the wavelength range 2.8–588 μm (Leisawitz et al., 2021). The Origins Survey Spectrometer (OSS) would make far-IR spectroscopic measurements with a maximum spectral resolving power R ∼ 3 × 105 (Bradford et al., 2021). A fourth instrument, the Heterodyne Receiver for Origins (HERO), was also studied as a way to provide higher spectral resolving power than OSS ∼106—107; (Wiedner et al., 2021). However, HERO was not included in the baseline mission concept because heterodyne detection, limited by receiver quantum noise, does not require a very cold (4.5 K) telescope such as Origins. OASIS will accomplish Origins/HERO science in a much less expensive mission.
In summary, OASIS will outperform other far-infrared facilities (Herschel, SOFIA) and complement near/mid-infrared (JWST) and (sub-) millimeter (ALMA) facilities as well as the future mid-/far-infrared Origins Space Telescope. These capabilities will make OASIS a powerful instrument for astrochemical studies in star- and planet-forming regions. In Section 3, we provide more detailed discussions of OASIS performance relative to other observatories in the context of specific science use cases.
2.3 Observations of Galactic Star-Forming Regions
As part of its aim to “follow the water trail,” OASIS will observe galaxies, nearby star-forming regions, and Solar System bodies. Here, we focus on science applications in galactic star-forming regions. OASIS will observe various classes of objects along the star-formation sequence: prestellar cores, low- and high-mass protostars, and protoplanetary disks. In particular, disk systems will be a key focus of the OASIS mission, with the goal of measuring the H2O content and HD-derived disk mass toward
Measurements of the HD and
3 CHNOPS Astrochemistry With OASIS
Here, we highlight impactful astrochemical contributions that OASIS will make with Band 1 and Band 2 observations of various dense star-forming regions. We begin with objects targeted by the OASIS disk survey: Class II disks (Section 3.1) and Class 0/I protostars (Section 3.2). We next consider additional star-forming regions where line surveys with OASIS will provide novel constraints on the chemistry and physics: protostellar outflows (Section 3.3), high-mass hot cores (Section 3.4), and prestellar cores (Section 3.5).
3.1 Protoplanetary Disks (Class II)
Given the small angular scales and cold temperatures of mature (Class II) disks, the detection of complex molecules
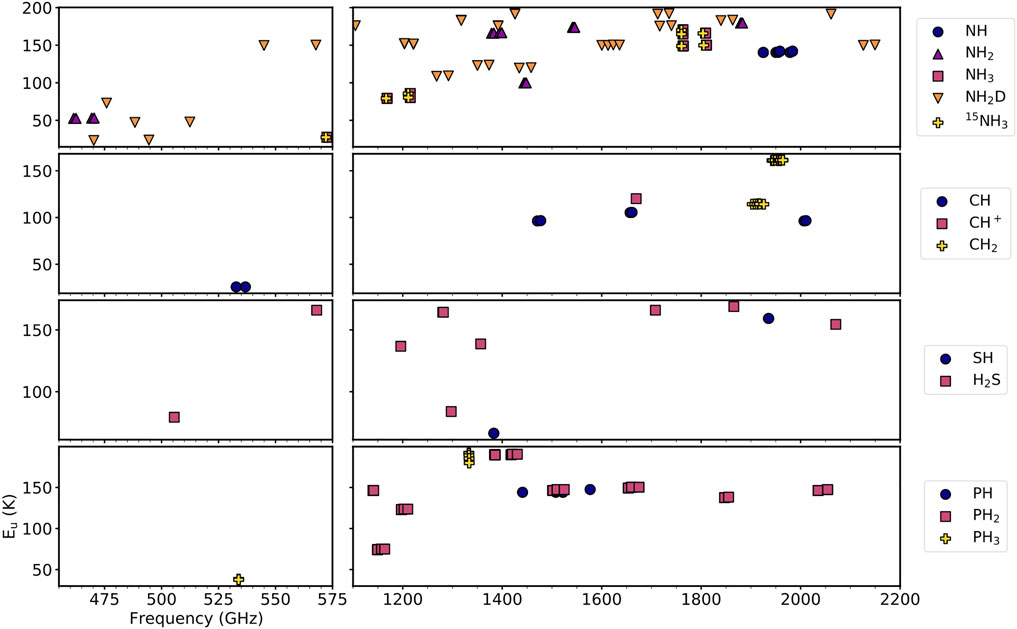
FIGURE 2. Summary of the frequencies and upper-state energies of selected N, C, S, and P hydrides with transitions observable by OASIS Bands 1 and 2. Only lines with Einstein coefficients
3.1.1 NH3 and Its Isotopologues
Of the light hydrides covered by OASIS Bands 1 and 2 (Figure 2), perhaps the most exciting science will be enabled by observations of NH3. Indeed, the N budget in disks is poorly constrained given that the dominant N carrier, N2, cannot be observed. Ice spectroscopy toward low-mass protostars, the evolutionary progenitors of disks, has revealed that NH3 is an important N carrier in the ice, with a relative abundance of ∼5% with respect to H2O compared to
Bands 1 and 2 of OASIS will cover multiple strong transitions tracing cool NH3 (upper state energies from 27 to 170 K). While the 572 GHz NH3 line toward TW Hya was detected with low SNR with Herschel, the same flux is over an order of magnitude above the OASIS 5σ detection threshold. To further assess the prospects of detecting NH3 with OASIS, we created a toy disk model based on TW Hya. We adopt a physical structure based on the models of Cleeves et al. (2015), Zhang et al. (2017), and Huang et al. (2018). Informed by the constraints on o-NH3 from Salinas et al. (2016), we assume a power-law abundance profile X(r) = 8 × 10–11 (r/100au). We assume a freeze-out temperature of 65 K (e.g., Kruczkiewicz et al., 2021), below which we attenuated the NH3 abundance by two orders of magnitude. We use RADMC-3D (Dullemond et al., 2012) to simulate fluxes of the three o-NH3 lines observable by OASIS and recover a comparable flux of the 572 GHz transition compared to the Herschel observations of TW Hya. We emphasize that this is a toy model that reproduces the observations with a reasonable physical and chemical structure, but other distributions of NH3 in TW Hya are of course possible.
Figure 3 shows the predicted o-NH3 fluxes for three distances representative of the source targets in the OASIS disk survey. For relatively nearby sources (
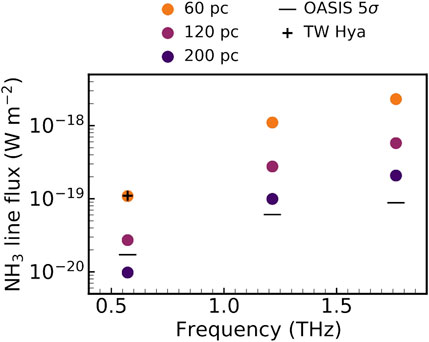
FIGURE 3. Simulated o-NH3 line fluxes for a TW Hya-like disk at 60, 120, and 200 pc. Horizontal bars represent the OASIS 5σ detection threshold for each line. The 572 GHz o-NH3 flux observed by Salinas et al. (2016) is shown with a “+”.
Excitingly, detection of multiple lines with OASIS will allow for the first excitation analysis of NH3 in the outer disk. Additionally, the high spectral resolution provided by OASIS will enable a kinematic analysis of the NH3 line profiles in sources with high SNR, providing constraints on the spatial origin of emission. Auxiliary constraints on the disk structures, provided by the OASIS observations of CO isotopologues and HD and H2O, will permit robust NH3 abundance retrievals. The NH3/H2O abundance ratio is of particular interest, as it can be directly compared with the ratio measured in comets to provide insights into how N is inherited by Solar System bodies.
While we focused our toy model on o-NH3 for simplicity, OASIS will also cover six transitions of p-NH3 with comparable line intensities to the modeled o-NH3 transitions. While the statistical NH3 ortho-to-para ratio (OPR) is 1, values
Numerous transitions of NH3 isotopologues (e.g., 15NH3 and NH2D) are also observable by OASIS, raising the possibility of measuring isotopic fractionation levels in NH3. The case of 15NH3 is particularly interesting since, to date, the 14N/15N ratio in disks has only been measured via nitriles (i.e., CN and HCN; Guzmán et al., 2017; Hily-Blant et al., 2017), which form through a distinct chemistry compared to NH3 (e.g., Visser et al., 2018). 15NH3 transitions are close in frequency, upper-state energy, and intrinsic line strength to the analogous NH3 transitions (Figure 2). Detection of these lines will likely be challenging: based on our predicted o-NH3 line fluxes and given that the 14N/15N ratio measured in disk nitriles is ≳100, 15NH3 would not be detected toward TW Hya. Still, given the large number of disks in the OASIS survey, a detection of 15NH3 is plausible and would be of high impact.
3.1.2 Other Light Hydride Science
Another promising avenue for disk science with OASIS is S hydrides. Sulfur is commonly very depleted from the gas in dense star-forming regions, though several S carriers (CS, SO, H2S, and H2CS) have now been detected in disks (Dutrey et al., 1997; Guilloteau et al., 2013; Phuong et al., 2018; Le Gal et al., 2019). H2S was only recently detected in Class II disks: first toward GG Tau A (Phuong et al., 2018), followed by UY Aur (Rivière-Marichalar et al., 2021). Toward other well-known disks, deep searches for H2S have only produced upper limits (Dutrey et al., 2011). To date, only the 11,0–10,1 line (168.73 GHz) has been targeted, which is readily observable by using ground-based telescopes but also intrinsically quite weak compared to the higher frequency lines covered by OASIS. The H2S lines at 1865.6 and 1,281.7 GHz appear particularly promising for detection in disks with OASIS, particularly if the emission originates in a somewhat warm environment. For instance, assuming a comparable source-average column density (1.3 × 1012 cm−2) and emitting area (∼ 3″ radius) to GG Tau A, we expect to detect these lines given a rotational temperature ≳35 K. With warmer temperatures, closer/larger sources, or higher H2S column densities, seven additional H2S lines in OASIS Bands 1 and 2 (Eu 79–168 K) will also become good candidates for detection, enabling a multiline excitation analysis.
It is important to highlight that H2S is the dominant sulfur carrier in cometary comae (Calmonte et al., 2016). The lack of constraints on H2S abundances and distributions in protoplanetary disks has made it challenging to contextualize these measurements of cometary sulfur chemistry. By providing constraints or strong upper limits on the H2S inventory in a physically diverse sample of ∼100 disks, OASIS will greatly advance our understanding of the volatile sulfur reservoir in planet-forming disks and our own Solar System.
CH+ was previously detected toward one disk with Herschel and was found to probe warm emission from the disk surface and inner rim (Thi et al., 2011). Prospects for detecting other light carbon hydrides (CH and CH2) in disks are unclear given that there is no precedent in the literature. Similarly, the unsaturated nitrogen and sulfur hydrides (NH, NH2, and SH) have not been previously observed toward disks. OASIS will cover numerous strong lines of each of these molecules (Figure 2), so it remains a possible avenue of discovery science. If detected, these lines would provide yet further constraints on the volatile C/N/S chemistry in disks. We note that phosphorus has yet to be detected in any form in a disk, and so the detection prospects for phosphorus hydrides are even less certain.
3.2 Low-Mass Protostars (Class 0/I)
3.2.1 Hot Corino Emission
A subset of low-mass protostars exhibits a rich gas-phase organic chemistry near the protostellar core and have been termed “hot corinos” in analogy to the high-mass hot cores described in Section 3.4 (Cazaux et al., 2003; Bottinelli et al., 2004). Because this chemical richness originates from the sublimation of icy molecules, the inventory of organic molecules in the gas should reflect, at least in part, the composition of the parent ices (e.g., Garrod and Widicus Weaver, 2013).
Within the protostellar environment, a hot corino occupies a similar spatial scale as the nascent protoplanetary disk (e.g., Maury et al., 2014; Maret et al., 2020). Constraints on the organic inventories in hot corinos, therefore, provide a view of the complex organic material that is present in the ices where disk formation, and ultimately planet/planetesimal formation, is taking place. Indeed, similarities in the organic compositions of hot corinos compared to cometary ices support the idea that comets formed at least in part from the icy material with a pre/protostellar origin (Drozdovskaya et al., 2019). Moreover, probing isotopic fractionation levels in these organics provides powerful constraints on their formation conditions (e.g., Coutens et al., 2016; Jørgensen et al., 2018). This is key to determining where along the star-formation sequence organic complexity is built up.
The archetypical hot corino source IRAS 16293–2422 (hereafter IRAS 16293) was observed as part of the Herschel key project CHESS (Ceccarelli et al., 2010). In the 555–636 GHz range, comparable to OASIS Band 1, numerous lines of small organics (e.g., HCN, H2CO, and CH3OH) were detected. However, neither rarer isotopologues of CH3OH nor organics larger than CH3OH were detected. With a beam size around 35″ at these frequencies and a hot corino emitting size of 0.5″, these observations suffered a beam dilution factor of nearly 5,000. Thus, previous submillimeter and THz observations of hot corinos offered virtually no constraints on the organic molecule emission.
The smaller beam size of OASIS (∼ 10″ at Band 1) will provide a huge improvement in this regard. In Figure 4A, we show predicted spectra of four common oxygen-bearing organics toward IRAS 16293, generated using the physical and chemical model constrained by the ALMA-PILS survey (Jørgensen et al., 2016). Although the OASIS beam will still be larger than the source size, thousands of organic molecule transitions will be detected at high SNR. Coverage of numerous lines per molecule will permit a detailed analysis of their excitation conditions. Moreover, with dozens of protostellar targets, the OASIS mission will deliver demographic constraints on the complex organic inventories across hot corinos.
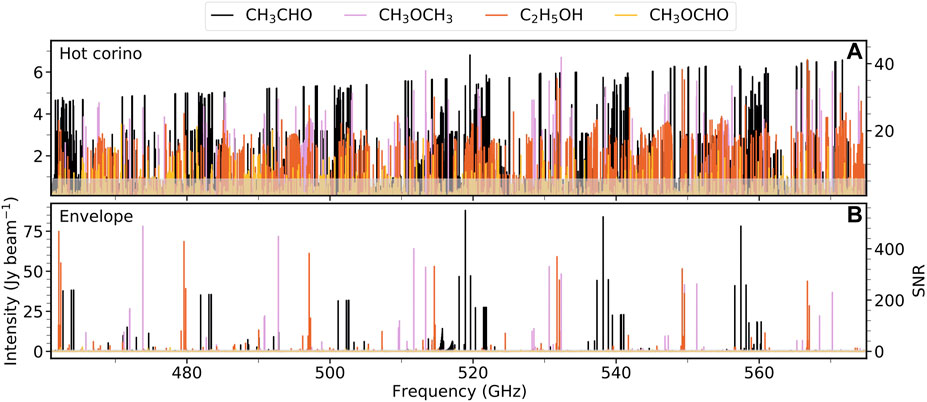
FIGURE 4. Simulated spectra of common O-bearing organics in OASIS Band 1. Physical models are based on a hot corino (A) and protostellar envelope (B). In both panels, the 5σ detection threshold for a 1-h integration of OASIS Band 1 is shown as a gray shaded region, assuming a 1 km s−1 line width.
3.2.2 Organics in Protostellar Envelopes
Not all Class 0/I protostars are hosts to hot corino emission, yet due to non-thermal desorption mechanisms, protostellar envelopes still host detectable abundances of gas-phase emission from organic molecules (e.g., Öberg et al., 2011b; Bergner et al., 2017). Figure 4B shows simulated spectra assuming the same chemical model as the hot corino IRAS 16293 but adopting abundances scaled down by two orders of magnitude and a cool (30 K) gas temperature. For this simulation, we assume that the emission fills the OASIS beam, as is appropriate for a protostellar envelope. Multitudes of transitions are detectable by OASIS, with even higher SNR compared to those by hot corinos due to the larger emitting area. Interferometers are not well suited for probing this diffuse envelope emission since they are mainly sensitive to emission on smaller spatial scales and resolve out emission on larger scales. OASIS, therefore, offers a unique advantage for studying the emission of non-thermally desorbed molecules in lukewarm (∼30 K) protostellar envelopes of Class 0/I sources. These constraints will provide novel insights into how organic complexity evolves during the epoch when dust and gas of the cold envelope collapse toward the central protostar and can help benchmark chemical models of such evolution (e.g., Garrod and Herbst, 2006). This avenue is also highly complementary to forthcoming JWST ice observations: ice maps constructed from observations of extincted background stars will probe similar spatial scales, enabling direct comparisons between the ice and gas compositions of the envelope (e.g., Perotti et al., 2020, 2021).
3.2.3 Deuterium Fractionation
In both hot corino and non-hot corino sources, OASIS should have sufficient sensitivity to detect deuterated isotopologues of common complex organics. Figure 5 shows the simulated spectra for singly and doubly deuterated dimethyl ether (CH3OCH3) and singly deuterated ethanol (C2H5OH), assuming the same hot corino and envelope conditions as for Figure 4. For clarity, different isotopomers are combined to produce a single spectrum for each substituted molecule. While deuterated ethanol lines are only slightly above the detection threshold in the hot corino simulation, both singly and doubly deuterated dimethyl ethers should be readily detectable. The envelope scenario is even more promising, with all three molecules exhibiting numerous lines well above the detection threshold.
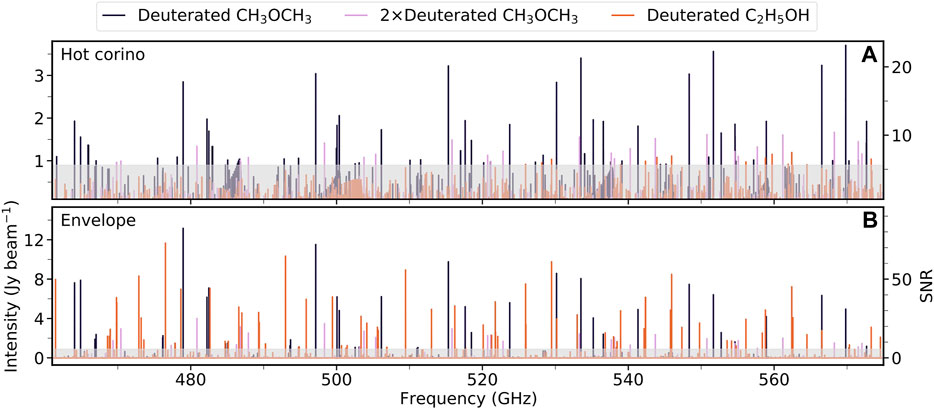
FIGURE 5. Simulated spectra of deuterated O-bearing organics in OASIS Band 1. Physical models are based on a hot corino (A) and protostellar envelope (B). In both panels, the 5σ detection threshold for a 1-h integration of OASIS Band 1 is shown as a gray shaded region.
3.2.4 Comparison to ALMA
It is important to consider how the performance of OASIS will compare to ALMA, the current state-of-the-art facility for studying complex chemistry in hot corinos. Given its sub-arcsecond spatial resolution, ALMA is better beam-matched to hot corinos than OASIS and is capable of detecting lower abundance species such as even larger molecules (e.g., glycolaldehyde; Jørgensen et al., 2016) and rarer isotopologues (Coutens et al., 2016; Jørgensen et al., 2018). Still, OASIS has several unique advantages. 1) Bandwidth: In order to obtain sufficient SNR on the HD line in Band 3, OASIS will observe many sources for ∼12 h. Because Bands 1 and 2 are independently tunable, simultaneous spectral scans can cover up to 200 GHz in bandwidth assuming 1 h per scan. This spectral coverage is far beyond what is practically achievable with ALMA and will provide a detailed and unbiased picture of the inventories and excitation conditions of moderately abundant complex organics. 2) Source statistics: The OASIS mission will observe numerous Class 0/I protostars as part of its baseline mission. While surveys of organic chemistry in low-mass protostars are beginning to be undertaken with ALMA and NOEMA (e.g., Bergner et al., 2019a; Belloche et al., 2020; Yang et al., 2021), our understanding of the chemical demographics in these objects is still in its infancy. Given also that ALMA is heavily over-subscribed, OASIS will provide much-needed statistical constraints on the complex chemistry in Class 0/I protostars. 3) Maximum resolvable scale: As noted previously, due to its larger beam size, OASIS will be able to probe organic molecule emission originating in the diffuse envelope of protostars, to which ALMA observations are not sensitive. This will provide novel constraints on chemical evolution during protostellar infall. 4) Far-IR spectral coverage: While ALMA can access far-IR wavelengths with Bands 8–10, these observations require exceptional weather conditions. By contrast, there are no obstacles for observing at these wavelengths using a space telescope. Higher frequency observations generally cover lines with higher upper-state energies and are thus well suited for studying the hot emission originating close to a protostar. Additionally, for some molecules, the spectral intensity will peak in the far-IR under hot corino conditions. We highlight the case of E-C-cyanomethanamine, a HCN dimer implicated in prebiotic chemical schemes: at a temperature of 100 K, the strongest spectral lines occur around 900–1,000 GHz (Melosso et al., 2018). Thus, coverage at THz frequencies may be uniquely suited for detecting certain organics compared to lower-frequency observations.
3.3 Protostellar Outflows
The early (Class 0 and Class I) stages of low-mass protostellar evolution are often accompanied by the launching of an outflow, which promotes accretion onto the protostar by carrying away angular momentum. Encounters between the outflow and the ambient envelope material produce shocks, which can dramatically alter the local chemistry through heating and grain sputtering. In some “chemically rich” outflows, the gas-phase abundances of molecules normally associated with the ice phase (H2CO, CH3OH, and CH3OCHO) are enhanced by orders of magnitude due to shock-induced ice sputtering (e.g., Garay et al., 1998; Codella and Bachiller, 1999; Requena-Torres et al., 2007; Arce et al., 2008). Thus, as for hot cores and hot corinos, chemically rich outflows offer a valuable window to probe the organic composition of interstellar ices. Moreover, studies of outflow shock physics and chemistry inform our understanding of the same processes that take place on smaller, disk-forming scales within the protostellar core.
The archetypical chemically rich outflow shock, L1157-B1, was observed as part of the Herschel CHESS survey (Ceccarelli et al., 2010). The 555–636 GHz spectrum contained emission lines from high-excitation transitions of grain tracers such as NH3, H2CO, and CH3OH (Codella et al., 2010). An excitation analysis of these lines revealed that they emit with temperatures ≥200 K, intermediate between the cold emission observed by longer wavelength transitions and the very hot gas traced by H2 emission. Thus, observations of higher excitation organics toward outflow shocks can help to link these different emission regimes and disentangle how the shock chemistry and physics progresses. These insights can in turn be used to tune models of shock astrochemistry, which are needed to connect observed gas-phase abundances to the underlying grain compositions (e.g., Burkhardt et al., 2019).
Herschel observations as part of the WISH program also provided detailed constraints on the physics and chemistry toward numerous star-forming regions including protostellar outflows (van Dishoeck et al., 2011). While H2O chemistry is not our focus, it is worth highlighting that WISH obtained extensive constraints on the abundances and origin (e.g., ice sputtering vs. gas-phase chemistry) of H2O within outflows (van Dishoeck et al., 2021). Additionally, light hydride lines measured through the WISH program provided powerful constraints on the UV and X-ray fields around young protostars and the physics of shock propagation (Benz et al., 2016).
OASIS will offer several improvements for observations of outflows compared to Herschel (Figure 1). The improved OASIS sensitivity raises the possibility of detecting more complex species toward chemically rich shocks and characterizing their excitation in detail. With higher angular resolution, the OASIS beam will encompass a smaller range of physical environments within the outflow, leading to less spectral blending of different shock components. Last, the high-resolution mode of OASIS Band 1 has a higher spectral resolution than that of Herschel, allowing different velocity components within the outflow to be clearly distinguished. Thus, we expect significant improvements in the shock chemistry and physics that can be probed with OASIS compared to Herschel. While ALMA similarly offers improvements in sensitivity and spectral/spatial resolution compared to Herschel, the higher frequencies covered by OASIS are better suited for accessing the warm post-shocked material compared to ALMA.
It is also worth noting that chemically rich outflow shocks are, to date, the only low-mass star forming regions where phosphorus carriers have been detected (Yamaguchi et al., 2011; Bergner et al., 2019c). In shock chemistry models, PH3 and smaller P hydrides are predicted to be at least as abundant as the detected P carriers PN and PO (Jiménez-Serra et al., 2018). However, PH3 has only one strong transition observable below 500 GHz and has yet to be detected in a star-forming region. OASIS will cover multiple strong lines of P hydrides (Figure 2), opening the door for obtaining a more complete inventory of the volatile phosphorus carriers in star-forming regions.
3.4 High-Mass Hot Cores
The regions of hot molecular line emission within massive star-forming regions are termed hot cores. These regions are typically characterized by high temperatures (hundreds of K) and densities (∼107 cm−2) (van der Tak et al., 2000). Originally identified based on the detection of hot NH3 toward Orion-KL (Morris et al., 1980), hot cores were subsequently found to host an incredibly rich gas-phase organic chemistry (Blake et al., 1987). Ice mantles are the main sites of astrochemical complex organic molecule formation, and sublimation of these ices is responsible for the wealth of chemical complexity detected in hot cores (e.g., Garrod and Herbst, 2006; Herbst and van Dishoeck, 2009). The molecular line emission in hot cores provides powerful constraints on both the physical and chemical conditions in these regions (see, for e.g., Garrod and Widicus Weaver, 2013). With a rich organic chemistry, high temperatures, and large emitting areas, high-mass hot cores represent ideal targets to exploit the unique capabilities of OASIS.
Pioneering work in the submillimeter study of high-mass hot cores was performed by the Herschel observations of EXtra-Ordinary Sources (HEXOS) guaranteed-time program. HEXOS targeted Orion KL and Sgr B2 (N + M) with
HEXOS provided a similar wealth of insight into the chemistry of simple molecules during high-mass star formation, including constraints on H2O deuteration levels (Bergin et al., 2010), the H2O abundance and ortho-to-para ratio (Melnick et al., 2010), and the CO2 abundance as traced by HOCO+ (Neill et al., 2014). Additionally, many of the thousands of lines detected in the HEXOS scans correspond to complex organic molecules. Coverage of lines with a wide range in upper-state energies allowed unprecedented constraints on the column densities and excitation conditions of dozens of organic molecules toward Orion KL and Sgr B2 (Crockett et al., 2010, 2014b; Neill et al., 2014). Intriguingly, HEXOS revealed a chemical differentiation in the complex organic inventories between Orion KL and Sgr B2(N), which could arise from evolutionary differences between the high-mass hot cores (Neill et al., 2014). This underscores the potential for such surveys to probe demographics in high-mass star formation.
While Herschel greatly expanded our understanding of high-mass hot cores, there is an ample room for improvement with OASIS. Notably, the OASIS beam will be nearly a factor of four smaller than the Herschel beam at a given frequency, mitigating loss of sensitivity due to beam dilution. For instance, in OASIS Bands 1 and 2, the OASIS beam will be 10.3 and 3.2″ compared to 40.4 and 12.8″ in the corresponding Herschel bands, respectively. Depending on the emitting source size, this could translate to a factor of up to 16 higher line sensitivity with OASIS compared to Herschel.
OASIS’s improved line sensitivity at THz wavelengths will open a new window into studying complex organic molecules in hot cores. Figure 6, adapted from McGuire et al. (2018), clearly illustrates how a massive star-forming region can appear line-poor when observed by Herschel but harbor hundreds of spectral lines when observed with a higher sensitivity and higher resolution observatory, in this case ALMA Band 10. With OASIS, we similarly expect higher line densities of organic molecules than Herschel. While the increase in sensitivity will be more modest with OASIS compared to ALMA, we note that Band 9 and 10 observations with ALMA require exceptional weather conditions and also do not extend to frequencies higher than 950 GHz, whereas OASIS will provide weather-independent access to wavelengths up to 3.6 THz.
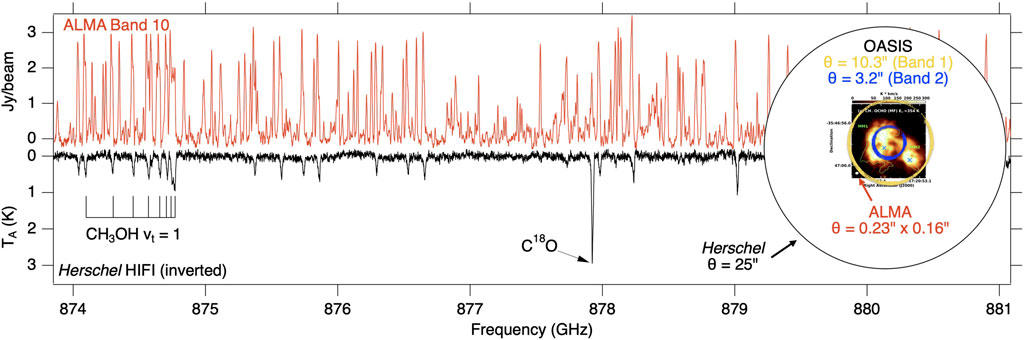
FIGURE 6. Spectra of the high-mass star-forming region NGC 6334I as observed by ALMA (red) compared to that of Herschel (black, inverted). Inset shows the Herschel, ALMA, and OASIS Band 1 and Band 2 beam sizes overlaid on the source structure, as traced by methyl formate emission. Figure adapted from McGuire et al. (2018).
While many complex organics can be detected via lines at lower frequencies, there are several advantages to obtaining observations at far-IR wavelengths. First, the lines covered by OASIS typically probe higher upper-state energies than millimeter-wavelength lines, which can provide a powerful lever arm for constraining excitation conditions. This is especially important for high-mass hot cores, in which organics often have excitation temperatures of a few hundred K (e.g., Crockett et al., 2014b; Neill et al., 2014). Good constraints on organic molecule excitation temperatures are required to interpret the physical conditions of the emitting regions as well as the chemical relationships between different classes of molecules. Also, as noted in Section 3.2.4, observations at higher frequencies may enable the detection of organic molecules with intensity peaks in the far-IR.
3.5 Prestellar Cores
Prestellar cores are the gravitationally bound phase of star formation immediately prior to the formation of a protostar (Bergin and Tafalla, 2007; André et al., 2014). OASIS will provide a unique opportunity to probe transitions that are difficult or impossible to observe from the ground in prestellar cores. It is during this phase that the initial conditions are set for the chemistry of the disk and subsequent planet formation. The direct chemical inheritance from the prestellar phase to the protostellar disk has been established, for instance reflected in the D/H ratio from ALMA observations of deuterated water (Jensen et al., 2021).
The central region of a prestellar core is a cold (TK < 10 K), dense (n > 105 cm−3) environment that is well shielded from the surrounding interstellar radiation field (AV > 10 mag). Many molecules, such as CO, deplete onto dust grains at these densities and temperatures (Willacy et al., 1998; Bacmann et al., 2002) eliminating them as probes of these regions. Deuterium fractionation of molecules via reactions with H2D+ becomes important (Millar et al., 1989; Roberts et al., 2003). The ground state rotational transitions of para-H2D+ (1.370 THz) and ortho-D2H+ (1.477 THz) are within OASIS Band 2; however, these lines are extremely difficult to detect toward prestellar cores due to the large energy difference between rotational energy levels (i.e., Eu/k = 65.7 K for the upper energy level of the 1.370 THz 10,1–00,0 transition of para-H2D+). NH2D is an excellent tracer of these coldest, densest regions of prestellar cores where deuterium fractionation enhances (NH2D)/(NH3) by more than four orders of magnitude above the ISM D/H ratio (Tiné et al., 2000; Ceccarelli et al., 2014; Harju et al., 2017). OASIS will observe the high critical density (ncrit > 107 cm−3) transitions of NH2D that probe the very centers of the cores where a disk will eventually form. In Band 1, the 11,0–00,0 ground state rotation-inversion transitions of both ortho and para spin-species of NH2D are observable at 470 and 494 GHz, respectively (Figure 2). These transitions may be used in combination with the 86 and 110 GHz transitions, which are observable from the ground, to constrain the physical conditions of the innermost regions. The 86/470 line ratio of ortho-NH2D is very sensitive to the temperature in the center of the core for n (H2) > 106 cm−3 (Figure 7). Observations of the 470 GHz transition are difficult from the ground because the atmospheric transmission is less than 25% with 1 mm of water vapor at a high altitude site, and subthermal energy level populations result in a low-excitation temperature and therefore weak lines. The detectability of these lines from space was demonstrated with Herschel observations of the prestellar cores IRAS16293E and L1544 (Bacmann et al., 2012). The OASIS beam will better couple to the source emission for high critical density lines in prestellar cores than the Herschel beam permitting surveys of the densest prestellar cores in nearby molecular clouds.
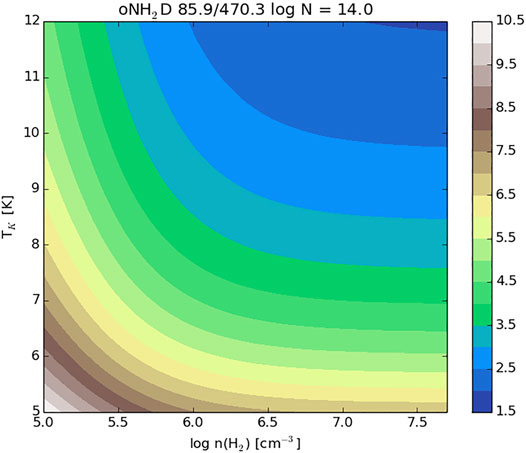
FIGURE 7. RADEX (van der Tak et al., 2007) models of the line ratio of the 85.9 and 470.3 GHz transitions of o-NH2D. Plotted line ratios vary from 1.5 to 10.5 for different values of the H2 density and gas kinetic temperature. The o-NH2D column density is assumed to be 1014 cm−3.
Water observations of prestellar cores with OASIS will also play an important role in constraining the temperature profile in the outer part of the cores. Most of the water in a prestellar core is found in the solid state on the icy surfaces of dust grains (Bergin and Snell, 2002). However, photodesorption by UV photons can liberate some water molecules into the gas phase at abundances that are typically
OASIS observations of light hydride molecules will also play an important role in constraining the kinematics of prestellar cores. The dynamical motions onto and within a prestellar core are difficult to constrain with current observational techniques. The density profile of a hydrostatic core is very similar to the density profile of a collapsing core until very late in the collapse history (Myers, 2005). Optically thick emission lines, such as HCN 1–0, can probe the internal kinematics through blue asymmetric self-absorbed emission profiles. The creation of this line profile requires very specific kinematic and molecular excitation conditions along the line of sight that are often difficult to achieve. Surveys searching for blue asymmetric line profiles generally find only a small fraction of prestellar cores with this signature (Sohn et al., 2007; Campbell et al., 2016; Seo et al., 2019). An alternative strategy is to observe red-shifted absorption lines from molecules that trace the outer portions of the core and compare to the velocities of emission lines that probe the inner regions of the core. This will be possible with OASIS observations of light hydride molecules and their deuterated isotopologues as well as with observations of H2O. Tracers that have a wide range of critical densities probe the kinematics at different depths in the core. An example of this technique was demonstrated with Herschel observations of the ground state rotational transitions of ND (in emission) and NH (in absorption) toward the prestellar core IRAS 16293E (Bacmann et al., 2016).
4 Summary and Conclusion
The OASIS observatory is a NASA MIDEX space mission concept designed to observe at THz frequencies with unprecedented sensitivity and angular resolution. Compared to other state-of-the-art telescopes, OASIS will outperform other far-IR observatories (Herschel and SOFIA) and complement near/mid-IR (JWST) and (sub-) millimeter (ALMA) facilities. With its unique capacity to probe light hydrides and high-excitation organic lines, OASIS will open a new window for studying organic/prebiotic astrochemistry along the star- and planet-formation sequence. Key science use cases include:
1 OASIS will cover multiple strong transitions of NH3 and H2S, both of which are expected to be important volatiles in disks but are detected in only two sources to date. With ∼100 source targets in the OASIS disk survey, OASIS will provide statistical constraints on the NH3 and H2S content in disks. It will also enable, for the first time, analyses of the excitation conditions, ortho-to-para ratios, and isotopic fractionation levels of these molecules in disks.
2. With broad spectral scans, OASIS will provide a detailed and unbiased view of the organic inventories and excitation conditions of hot corinos, lukewarm protostellar envelopes, protostellar outflows, and hot cores. Compared to ALMA, OASIS offers important advantages in its capacity to cover wide spectral bandwidths, to obtain robust source statistics, to sample diffuse large-scale emission, and to access high-excitation lines in the far-infrared.
3. OASIS coverage of light hydrides will provide novel constraints on the physical conditions in the dense inner regions of prestellar cores, thus probing the initial conditions of protostellar and disk physics and chemistry.
Importantly, these efforts directly address high-priority science questions posed by the 2020 Decadal Survey (National Academies of Sciences, E., and Medicine, 2021), including: How are potentially habitable environments formed (E-Q3a)? And, what generates the observed chemical complexity of molecular gas (F-Q2c)?
Auxiliary laboratory efforts in THz spectroscopy will be critical for maximizing the scientific output of OASIS. Indeed, ∼10% of lines in the Herschel spectra of Sgr B2(N) and Orion-KL were unidentified (Crockett et al., 2014b; Neill et al., 2014). The spectral line properties of many interstellar molecules are not well characterized above ∼300 GHz, and some classes of molecules (e.g., isotopologues, vibrationally excited states, and unstable molecules) are particularly under-studied, though there are promising avenues for filling in these gaps (Widicus Weaver, 2019). Along with laboratory efforts, astrochemical modeling will greatly enrich the interpretation of OASIS observations. We expect OASIS to be particularly powerful for testing various mechanisms for ice-phase organic molecule production (e.g., Garrod & Herbst, 2006; Shingledecker et al., 2018; Jin & Garrod, 2020; Simons et al., 2020; Carder et al., 2021) given the detailed constraints on organic molecule inventories, abundances, and excitation conditions that will be obtained for low- and high-mass protostars and outflow shocks.
In summary, light hydrides and high-excitation organics are key players in the chemistry at various stages throughout the entire star- and planet-formation sequence. Our current understanding of these chemical regimes is limited due to the challenges of observing at THz frequencies. The OASIS mission will provide an unparalleled view of this astrochemistry, thus advancing our understanding of how and in what form the prebiotically important material is incorporated into nascent planets and planetesimals.
Software: Matplotlib (Hunter 2007), NumPy (van der Walt et al., 2011), RADEX (van der Tak et al., 2007), and RADMC-3D (Dullemond et al., 2012).
Data Availability Statement
The raw data supporting the conclusion of this article will be made available by the authors, without undue reservation.
Author Contributions
JB led the analysis and writing of the study. YS provided sections of the manuscript. JK and BM contributed figures and data. All authors contributed to manuscript editing and revision.
Conflict of Interest
The authors declare that the research was conducted in the absence of any commercial or financial relationships that could be construed as a potential conflict of interest.
Publisher’s Note
All claims expressed in this article are solely those of the authors and do not necessarily represent those of their affiliated organizations, or those of the publisher, the editors, and the reviewers. Any product that may be evaluated in this article, or claim that may be made by its manufacturer, is not guaranteed or endorsed by the publisher.
Acknowledgments
We are grateful to the reviewers for helpful feedback on this manuscript. J.B.B. acknowledges support from NASA through the NASA Hubble Fellowship grant #HST-HF2-51 429.001-A awarded by the Space Telescope Science Institute, which is operated by the Association of Universities for Research in Astronomy, Incorporated under NASA contract NAS5-26 555. S.A. gratefully acknowledges funding from the European Research Council (ERC) under the European Union’s Horizon 2020 Research and Innovation Programme (grant agreement no: 789 410).
References
Alexander, C. M. O. D., Bowden, R., Fogel, M. L., Howard, K. T., Herd, C. D. K., and Nittler, L. R. (2012). The Provenances of Asteroids, and Their Contributions to the Volatile Inventories of the Terrestrial Planets. Science 337, 721–723. doi:10.1126/science.1223474
Alexander, C. M. O. D., Nittler, L. R., Davidson, J., and Ciesla, F. J. (2017). Measuring the Level of Interstellar Inheritance in the Solar Protoplanetary Disk. Meteorit Planet. Sci. 52, 1797–1821. doi:10.1111/maps.12891
Altwegg, K., Balsiger, H., Berthelier, J. J., Bieler, A., Calmonte, U., De Keyser, J., et al. (2017). D 2 O and HDS in the Coma of 67P/Churyumov-Gerasimenko. Phil. Trans. R. Soc. A. 375, 20160253. doi:10.1098/rsta.2016.0253
André, P., Di Francesco, J., Ward-Thompson, D., Inutsuka, S.-I., Pudritz, R. E., and Pineda, J. (2014). “From Filamentary Networks to Dense Cores in Molecular Clouds: Toward a New Paradigm for Star Formation,” in Protostars and Planets VI. Editors H. Beuther, R. S. Klessen, C. P. Dullemond, and T. Henning, 27. doi:10.2458/azu_uapress_9780816531240-ch002
Arce, H. G., Santiago-García, J., Jørgensen, J. K., Tafalla, M., and Bachiller, R. (2008). Complex Molecules in the L1157 Molecular Outflow. ApJ 681, L21–L24. doi:10.1086/590110
Arenberg, J. W., Villareal, M. N., Yamane, J., Yu, T., Lazear, J., Pohner, J., et al. (2021). “OASIS Architecture: Key Features,”. Astronomical Optics: Design, Manufacture, and Test of Space and Ground Systems III. Editors T. B. Hull, D. Kim, P. Hallibert, and F. Keller (International Society for Optics and Photonics SPIE), 11820, 264–283. doi:10.1117/12.2594681
Bacmann, A., Caselli, P., Ceccarelli, C., Pagani, L., and Vastel, C. (2012). “ESA Science & Technology,” in From Atoms to Pebbles: Herschel’s View of Star and Planet Formation. 15.
Bacmann, A., Daniel, F., Caselli, P., Ceccarelli, C., Lis, D., Vastel, C., et al. (2016). Stratified NH and ND Emission in the Prestellar Core 16293E in L1689N. A&A 587, 587A26. doi:10.1051/0004-6361/201526084
Bacmann, A., Lefloch, B., Ceccarelli, C., Castets, A., Steinacker, J., and Loinard, L. (2002). The Degree of CO Depletion in Pre-stellar Cores. A&A 389, 389L6–L10. doi:10.1051/0004-6361:20020652
Belloche, A., Maury, A. J., Maret, S., Anderl, S., Bacmann, A., André, P., et al. (2020). Questioning the Spatial Origin of Complex Organic Molecules in Young Protostars with the CALYPSO Survey. A&A 635, A198. doi:10.1051/0004-6361/201937352
Benz, A. O., Bruderer, S., van Dishoeck, E. F., Melchior, M., Wampfler, S. F., van der Tak, F., et al. (2016). Water in star-forming Regions withHerschel(WISH). A&A 590, 590A105. doi:10.1051/0004-6361/201525835
Bergin, E. A., Phillips, T. G., Comito, C., Crockett, N. R., Lis, D. C., Schilke, P., et al. (2010). Herschel Observations of EXtra-ordinary Sources (HEXOS): The Present and Future of Spectral Surveys with Herschel/HIFI. A&A 521, L20. doi:10.1051/0004-6361/201015071
Bergin, E. A., and Snell, R. L. (2002). Sensitive Limits on the Water Abundance in Cold Low-Mass Molecular Cores. ApJL 581, 581L105–L108. doi:10.1086/346014
Bergin, E. A., and Tafalla, M. (2007). Cold Dark Clouds: The Initial Conditions for Star Formation. Annu. Rev. Astron. Astrophys. 45, 339–396. doi:10.1146/annurev.astro.45.071206.100404
Bergner, J. B., Martín-Doménech, R., Öberg, K. I., Jørgensen, J. K., de la Villarmois, E. A., and Brinch, C. (2019a). Organic Complexity in Protostellar Disk Candidates. ACS Earth Space Chem. 3, 1564. doi:10.1021/acsearthspacechem.9b00059
Bergner, J. B., Öberg, K. I., Bergin, E. A., Loomis, R. A., Pegues, J., and Qi, C. (2019b). A Survey of C2H, HCN, and C18O in Protoplanetary Disks. ApJ 876, 25. doi:10.3847/1538-4357/ab141e
Bergner, J. B., Öberg, K. I., Garrod, R. T., and Graninger, D. M. (2017). Complex Organic Molecules toward Embedded Low-Mass Protostars. ApJ 841, 120. doi:10.3847/1538-4357/aa72f6
Bergner, J. B., Öberg, K. I., Walker, S., Guzmán, V. V., Rice, T. S., and Bergin, E. A. (2019c). Detection of Phosphorus-Bearing Molecules toward a Solar-type Protostar. ApJ 884, L36. doi:10.3847/2041-8213/ab48f9
Blake, G. A., Sutton, E. C., Masson, C. R., and Phillips, T. G. (1987). Molecular Abundances in OMC-1 - the Chemical Composition of Interstellar Molecular Clouds and the Influence of Massive star Formation. ApJ 315, 621. doi:10.1086/165165
Bottinelli, S., Ceccarelli, C., Lefloch, B., Williams, J. P., Castets, A., Caux, E., et al. (2004). Complex Molecules in the Hot Core of the Low‐Mass Protostar NGC 1333 IRAS 4A. ApJ 615, 354–358. doi:10.1086/423952
Bradford, C. M., Cameron, B., Moore, B., Hailey-Dunsheath, S., Amatucci, E., Bradley, D., et al. (2021). Origins Survey Spectrometer: Revealing the Hearts of Distant Galaxies and Forming Planetary Systems with Far-IR Spectroscopy. J. Astron. Telesc. Instrum. Syst. 7, 011017. doi:10.1117/1.JATIS.7.1.011017
Burkhardt, A. M., Shingledecker, C. N., Gal, R. L., McGuire, B. A., Remijan, A. J., and Herbst, E. (2019). Modeling C-Shock Chemistry in Isolated Molecular Outflows. ApJ 881, 32. doi:10.3847/1538-4357/ab2be8
Calmonte, U., Altwegg, K., Balsiger, H., Berthelier, J. J., Bieler, A., Cessateur, G., et al. (2016). Sulphur-bearing Species in the Coma of Comet 67P/Churyumov-Gerasimenko. Mon. Not. R. Astron. Soc. 462, S253–S273. doi:10.1093/mnras/stw2601
Campbell, J. L., Friesen, R. K., Martin, P. G., Caselli, P., Kauffmann, J., and Pineda, J. E. (2016). Contraction Signatures toward Dense Cores in the Perseus Molecular Cloud. ApJ 819, 143. doi:10.3847/0004-637X/819/2/143
Carder, J. T., Ochs, W., and Herbst, E. (2021). Modelling the Insertion of O(1D) into Methane on the Surface of Interstellar Ice Mantles. MNRAS 508, 1526–1532. doi:10.1093/mnras/stab2619
Caselli, P., Keto, E., Bergin, E. A., Tafalla, M., Aikawa, Y., Douglas, T., et al. (2012). First Detection of Water Vapor in a Pre-stellar Core. ApJ 759, L37. doi:10.1088/2041-8205/759/2/L37
Cazaux, S., Tielens, A. G. G. M., Ceccarelli, C., Castets, A., Wakelam, V., Caux, E., et al. (2003). The Hot Core Around the Low-Mass Protostar IRAS 16293-2422: Scoundrels Rule!. ApJ 593, L51–L55. doi:10.1086/378038
Ceccarelli, C., Bacmann, A., Boogert, A., Caux, E., Dominik, C., Lefloch, B., et al. (2010). Herschel Spectral Surveys of star- Forming Regions Overview of the 555-636 GHz Range. A&A 521, L22. doi:10.1051/0004-6361/201015081
Ceccarelli, C., Caselli, P., Bockelée-Morvan, D., Mousis, O., Pizzarello, S., Robert, F., et al. (2014). “Deuterium Fractionation: The Ariadne's Thread from the Precollapse Phase to Meteorites and Comets Today,” in Protostars and Planets VI. Editors H. Beuther, R. S. Klessen, C. P. Dullemond, and T. Henning, 859. doi:10.2458/azu_uapress_9780816531240-ch037
Cleeves, L. I., Bergin, E. A., Qi, C., Adams, F. C., and Öberg, K. I. (2015). CONSTRAINING the X-RAY and COSMIC-RAY IONIZATION CHEMISTRY of the TW Hya PROTOPLANETARY DISK: EVIDENCE for A SUB-INTERSTELLAR COSMIC-RAY RATE. ApJ 799, 204. doi:10.1088/0004-637X/799/2/204
Codella, C., Lefloch, B., Ceccarelli, C., Cernicharo, J., Caux, E., Lorenzani, A., et al. (2010). The CHESS Spectral Survey of star Forming Regions: Peering into the Protostellar Shock L1157-B1. I. Shock Chemical Complexity. A&A 518, L112. doi:10.1051/0004-6361/201014582
Coutens, A., Jørgensen, J. K., van der Wiel, M. H. D., Müller, H. S. P., Lykke, J. M., Bjerkeli, P., et al. (2016). The ALMA-PILS Survey: First Detections of Deuterated Formamide and Deuterated Isocyanic Acid in the Interstellar Medium. A&A 590, 590L6. doi:10.1051/0004-6361/201628612
Crockett, N. R., Bergin, E. A., Neill, J. L., Black, J. H., Blake, G. A., and Kleshcheva, M. (2014a). Herschelobservations of Extra-ordinary Sources: H2S as a Probe of Dense Gas and Possibly Hidden Luminosity toward the Orion Kl Hot Core. ApJ 781, 114. doi:10.1088/0004-637X/781/2/114
Crockett, N. R., Bergin, E. A., Neill, J. L., Favre, C., Schilke, P., Lis, D. C., et al. (2014b). HERSCHELOBSERVATIONS OF EXTRAORDINARY SOURCES: ANALYSIS OF THE HIFI 1.2 THz WIDE SPECTRAL SURVEY TOWARD ORION KL. I. METHODS. ApJ 787, 112. doi:10.1088/0004-637X/787/2/112
Crockett, N. R., Bergin, E. A., Wang, S., Lis, D. C., Bell, T. A., Blake, G. A., et al. (2010). Herschel Observations of EXtra-ordinary Sources (HEXOS): The Terahertz Spectrum of Orion KL Seen at High Spectral Resolution. A&A 521, L21. doi:10.1051/0004-6361/201015116
Drozdovskaya, M. N., van Dishoeck, E. F., Rubin, M., Jørgensen, J. K., and Altwegg, K. (2019). Ingredients for Solar-like Systems: Protostar IRAS 16293-2422 B versus Comet 67P/Churyumov-Gerasimenko. MNRAS 490, 50–79. doi:10.1093/mnras/stz2430
Dullemond, C. P., Juhasz, A., Pohl, A., Sereshti, F., Shetty, R., Peters, T., et al. (2012). RADMC-3D: A Multi-Purpose Radiative Transfer Tool. Available at: http://ascl.net/1202.015.
Dutrey, A., Wakelam, V., Boehler, Y., Guilloteau, S., Hersant, F., Semenov, D., et al. (2011). Chemistry in Disks. A&A 535, 535A104. doi:10.1051/0004-6361/201116931
Evans II, N. J., Rawlings, J. M. C., Shirley, Y. L., and Mundy, L. G. (2001). Tracing the Mass during Low‐Mass Star Formation. II. Modeling the Submillimeter Emission from Preprotostellar Cores. ApJ 557, 193–208. doi:10.1086/321639
Faure, A., Hily-Blant, P., Le Gal, R., Rist, C., and Pineau des Forêts, G. (2013). Ortho-para Selection Rules in the Gas-phase Chemistry of Interstellar Ammonia. ApJ 770, 770L2. doi:10.1088/2041-8205/770/1/L2
Garay, G., Kohnenkamp, I., Bourke, T. L., Rodriguez, L. F., and Lehtinen, K. K. (1998). Molecular Abundance Enhancements in the Highly Collimated Bipolar Outflow BHR 71. ApJ 509, 768–784. doi:10.1086/306534
Garrod, R. T., and Herbst, E. (2006). Formation of Methyl Formate and Other Organic Species in the Warm-Up Phase of Hot Molecular Cores. A&A 457, 927–936. doi:10.1051/0004-6361:20065560
Garrod, R. T., and Widicus Weaver, S. L. (2013). Simulations of Hot-Core Chemistry. Chem. Rev. 113, 8939–8960. doi:10.1021/cr400147g
Guilloteau, S., Di Folco, E., Dutrey, A., Simon, M., Grosso, N., and Piétu, V. (2013). A Sensitive Survey for13CO, CN, H2CO, and SO in the Disks of T Tauri and Herbig Ae Stars. A&A 549, A92. doi:10.1051/0004-6361/201220298
Guzmán, V. V., Öberg, K. I., Huang, J., Loomis, R., and Qi, C. (2017). Nitrogen Fractionation in Protoplanetary Disks from the H13CN/HC15N Ratio. ApJ 836, 30. doi:10.3847/1538-4357/836/1/30
Harju, J., Daniel, F., Sipilä, O., Caselli, P., Pineda, J. E., Friesen, R. K., et al. (2017). Deuteration of Ammonia in the Starless Core Ophiuchus/H-MM1. A&A 600, 600A61. doi:10.1051/0004-6361/201628463
Herbst, E., and van Dishoeck, E. F. (2009). Complex Organic Interstellar Molecules. Annu. Rev. Astron. Astrophys. 47, 427–480. doi:10.1146/annurev-astro-082708-101654
Hily-Blant, P., Magalhaes, V., Kastner, J., Faure, A., Forveille, T., and Qi, C. (2017). Direct Evidence of Multiple Reservoirs of Volatile Nitrogen in a Protosolar Nebula Analogue. A&A 603, 603L6. doi:10.1051/0004-6361/201730524
Huang, J., Andrews, S. M., Cleeves, L. I., Öberg, K. I., Wilner, D. J., Bai, X., et al. (2018). CO and Dust Properties in the TW Hya Disk from High-Resolution ALMA Observations. ApJ 852, 122. doi:10.3847/1538-4357/aaa1e7
Hunter, J. D. (2007). Matplotlib: A 2D Graphics Environment. Comput. Sci. Eng. 9, 90–95. doi:10.1109/MCSE.2007.55
Jensen, S. S., Jørgensen, J. K., Kristensen, L. E., Coutens, A., van Dishoeck, E. F., Furuya, K., et al. (2021). ALMA Observations of Doubly Deuterated Water: Inheritance of Water from the Prestellar Environment. A&A 650, 650A172. doi:10.1051/0004-6361/202140560
Jiménez-Serra, I., Viti, S., Quénard, D., and Holdship, J. (2018). The Chemistry of Phosphorus-Bearing Molecules under Energetic Phenomena. JApJ 862, 128. doi:10.3847/1538-4357/aacdf2
Jin, M., and Garrod, R. T. (2020). Formation of Complex Organic Molecules in Cold Interstellar Environments through Nondiffusive Grain-Surface and Ice-Mantle Chemistry. ApJs 249, 26. doi:10.3847/1538-4365/ab9ec8
Jørgensen, J. K., Müller, H. S. P., Calcutt, H., Coutens, A., Drozdovskaya, M. N., Öberg, K. I., et al. (2018). The ALMA-PILS Survey: Isotopic Composition of Oxygen-Containing Complex Organic Molecules toward IRAS 16293-2422B. A&A 620, A170. doi:10.1051/0004-6361/201731667
Jørgensen, J. K., van der Wiel, M. H. D., Coutens, A., Lykke, J. M., Müller, H. S. P., van Dishoeck, E. F., et al. (2016). The ALMA Protostellar Interferometric Line Survey (PILS). A&A 595, A117. doi:10.1051/0004-6361/201628648
Kruczkiewicz, F., Vitorino, J., Congiu, E., Theulé, P., and Dulieu, F. (2021). Ammonia Snow Lines and Ammonium Salts Desorption. A&A 652, A29. doi:10.1051/0004-6361/202140579
Le Gal, R., Öberg, K. I., Loomis, R. A., Pegues, J., and Bergner, J. B. (2019). Sulfur Chemistry in Protoplanetary Disks: CS and H2CS. ApJ 876, 72. doi:10.3847/1538-4357/ab1416
Leisawitz, D., Amatucci, E., Allen, L., Arenberg, J. W., Armus, L., Battersby, C., et al. (2021). Origins Space Telescope: Baseline mission Concep. tJournal Astronomical Telescopes, Instr. Syst. 7, 011002. doi:10.1117/1.JATIS.7.1.011002
Maret, S., Maury, A. J., Belloche, A., Gaudel, M., André, P., Cabrit, S., et al. (2020). Searching for Kinematic Evidence of Keplerian Disks Around Class 0 Protostars with CALYPSO. A&A 635, 635A15. doi:10.1051/0004-6361/201936798
Marty, B., Altwegg, K., Balsiger, H., Bar-Nun, A., Bekaert, D. V., Berthelier, J-J., et al. (2017). Xenon Isotopes in 67P/Churyumov-Gerasimenko Show that Comets Contributed to Earth's Atmosphere. Science 356, 1069. doi:10.1126/science.aal3496
Maury, A. J., Belloche, A., André, P., Maret, S., Gueth, F., Codella, C., et al. (2014). First Results from the CALYPSO IRAM-PdBI Survey. A&A 563, L2. doi:10.1051/0004-6361/201323033
McGuire, B. A. (2018). 2018 Census of Interstellar, Circumstellar, Extragalactic, Protoplanetary Disk, and Exoplanetary Molecules. ApJs 239, 17. doi:10.3847/1538-4365/aae5d2
McGuire, B. A., Brogan, C. L., Hunter, T. R., Remijan, A. J., Blake, G. A., Burkhardt, A. M., et al. (2018). First Results of an ALMA Band 10 Spectral Line Survey of NGC 6334I: Detections of Glycolaldehyde (HC(O)CH2OH) and a New Compact Bipolar Outflow in HDO and CS. ApJ 863, L35. doi:10.3847/2041-8213/aad7bb
Melnick, G. J., Tolls, V., Neufeld, D. A., Phillips, T. G., Wang, S., Crockett, N. R., et al. (2010). Herschel Observations of EXtra-ordinary Sources (HEXOS): Observations of H2O and its Isotopologues towards Orion KL. A&A 521, L27. doi:10.1051/0004-6361/201015085
Melosso, M., Melli, A., Puzzarini, C., Codella, C., Spada, L., Dore, L., et al. (2018). Laboratory Measurements and Astronomical Search for Cyanomethanimine. A&A 609, A121. doi:10.1051/0004-6361/201731972
Millar, T. J., Bennett, A., and Herbst, E. (1989). Deuterium Fractionation in Dense Interstellar Clouds. ApJ 340, 906. doi:10.1086/167444
Morris, M., Palmer, P., and Zuckerman, B. (1980). Hot Ammonia in Orion. ApJ 237, 1. doi:10.1086/157837
Myers, P. C. (2005). Centrally Condensed Collapse of Starless Cores. ApJ 623, 280–290. doi:10.1086/428386
Najita, J. R., Carr, J. S., Brittain, S. D., Lacy, J. H., Richter, M. J., and Doppmann, G. W. (2021). High-resolution Mid-infrared Spectroscopy of GV Tau N: Surface Accretion and Detection of NH3 in a Young Protoplanetary Disk. ApJ 908, 171. doi:10.3847/1538-4357/abcfc6
National Academies of Sciences, E., and Medicine (2021). Pathways to Discovery in Astronomy and Astrophysics for the 2020s. Washington, DC: The National Academies Press. doi:10.17226/26141
Neill, J. L., Bergin, E. A., Lis, D. C., Schilke, P., Crockett, N. R., Favre, C., et al. (2014). Herschelobservations of Extraordinary Sources: Analysis of the Fullherschel/hifi Molecular Line Survey of Sagittarius B2(N). ApJ 789, 8. doi:10.1088/0004-637X/789/1/8
Öberg, K. I., and Bergin, E. A. (2021). Astrochemistry and Compositions of Planetary Systems. Phys. Rep. 893, 1–48. doi:10.1016/j.physrep.2020.09.004
Öberg, K. I., Boogert, A. C. A., Pontoppidan, K. M., van den Broek, S., van Dishoeck, E. F., Bottinelli, S., et al. (2011a). Thespitzerice Legacy: Ice Evolution from Cores to Protostars. ApJ 740, 109. doi:10.1088/0004-637X/740/2/109
Öberg, K. I., Guzmán, V. V., Furuya, K., Qi, C., Aikawa, Y., Andrews, S. M., et al. (2015). The Comet-like Composition of a Protoplanetary Disk as Revealed by Complex Cyanides. Nature 520, 198. doi:10.1038/nature14276
Perotti, G., Jørgensen, J. K., Fraser, H. J., Suutarinen, A. N., Kristensen, L. E., Rocha, W. R. M., et al. (2021). Linking Ice and Gas in the Lambda Orionis Barnard 35A Cloud. A&A 650, A168. doi:10.1051/0004-6361/202039669
Perotti, G., Rocha, W. R. M., Jørgensen, J. K., Kristensen, L. E., Fraser, H. J., and Pontoppidan, K. M. (2020). Linking Ice and Gas in the Serpens Low-Mass star-forming Region. A&A 643, A48. doi:10.1051/0004-6361/202038102
Persson, C. M., De Luca, M., Mookerjea, B., Olofsson, A. O. H., Black, J. H., Gerin, M., et al. (2012). Nitrogen Hydrides in Interstellar Gas. A&A 543, A145. doi:10.1051/0004-6361/201118686
Phillips, T. G., Bergin, E. A., Lis, D. C., Neufeld, D. A., Bell, T. A., Wang, S., et al. (2010). Herschel Observations of Extra-ordinary Sources (HEXOS): Detection of Hydrogen Fluoride in Absorption towards Orion KL. A&A 518, L109. doi:10.1051/0004-6361/201014570
Phuong, N. T., Chapillon, E., Majumdar, L., Dutrey, A., Guilloteau, S., Piétu, V., et al. (2018). First Detection of H2S in a Protoplanetary Disk. A&A 616, L5. doi:10.1051/0004-6361/201833766
Prasad, S. S., and Tarafdar, S. P. (1983). UV Radiation Field inside Dense Clouds - its Possible Existence and Chemical Implications. ApJ 267, 603. doi:10.1086/160896
Requena-Torres, M. A., Marcelino, N., Jiménez-Serra, I., Martín-Pintado, J., Martín, S., and Mauersberger, R. (2007). Organic Chemistry in the Dark Clouds L1448 and L183: A Unique Grain Mantle Composition. ApJ 655, L37–L40. doi:10.1086/511677
Rivière-Marichalar, P., Fuente, A., Le Gal, R., Arabhavi, A. M., Cazaux, S., Navarro-Almaida, D., et al. (2021). H2S Observations in Young Stellar Disks in Taurus. A&A 652, 652A46. doi:10.1051/0004-6361/202140470
Roberts, H., Herbst, E., and Millar, T. J. (2003). Enhanced Deuterium Fractionation in Dense Interstellar Cores Resulting from Multiply Deuterated H[FORMULA][F][SUP]+[/SUP][INF]3[/INF][/F][/FORMULA]. ApJApJL 591, L41–L44. doi:10.1086/376962
Rolffs, R., Schilke, P., Comito, C., Bergin, E. A., van der Tak, F. F. S., Lis, D. C., et al. (2010). Reversal of Infall in SgrB2(M) Revealed by Herschel/HIFI Observations of HCN Lines at THz Frequencies. A&A 521, L46. doi:10.1051/0004-6361/201015106
Rubin, M., Bekaert, D. V., Broadley, M. W., Drozdovskaya, M. N., and Wampfler, S. F. (2019). Volatile Species in Comet 67P/Churyumov-Gerasimenko: Investigating the Link from the ISM to the Terrestrial Planets. ACS Earth Space Chem. 3, 1792–1811. doi:10.1021/acsearthspacechem.9b00096
Rubin, M., Engrand, C., Snodgrass, C., Weissman, P., Altwegg, K., Busemann, H., et al. (2020). On the Origin and Evolution of the Material in 67P/Churyumov-Gerasimenko. Space Sci. Rev. 216, 102. doi:10.1007/s11214-020-00718-2
Salinas, V. N., Hogerheijde, M. R., Bergin, E. A., Ilsedore Cleeves, L., Brinch, C., Blake, G. A., et al. (2016). First Detection of Gas-phase Ammonia in a Planet-Forming Disk. A&A 591, A122. doi:10.1051/0004-6361/201628172
Seo, Y. M., Majumdar, L., Goldsmith, P. F., Shirley, Y. L., Willacy, K., Ward-Thompson, D., et al. (2019). An Ammonia Spectral Map of the L1495-B218 Filaments in the Taurus Molecular Cloud. II. CCS and HC 7 N Chemistry and Three Modes of star Formation in the Filaments. ApJ 871, 134. doi:10.3847/1538-4357/aaf887
Shingledecker, C. N., Tennis, J., Gal, R. L., and Herbst, E. (2018). On Cosmic-Ray-driven Grain Chemistry in Cold Core Models. ApJ 861, 20. doi:10.3847/1538-4357/aac5ee
Simons, M. A. J., Lamberts, T., and Cuppen, H. M. (2020). Formation of COMs through CO Hydrogenation on Interstellar Grains. A&A 634, 634A52. doi:10.1051/0004-6361/201936522
Sohn, J., Lee, C. W., Park, Y. S., Lee, H. M., Myers, P. C., and Lee, Y. (2007). Probing Inward Motions in Starless Cores Using the HCN(J= 1-0) Hyperfine Transitions: A Pointing Survey toward Central Regions. ApJ 664, 928–941. doi:10.1086/519159
Takashima, Y., Sirsi, S., Choi, H., Arenberg, J. W., Kim, D. W., and Walker, C. K. (2021). “All Reflective THz Telescope Design with an Inflatable Primary Antenna for Orbiting Astronomical Satellite for Investigating Stellar Systems (OASIS) mission,” in Astronomical Optics: Design, Manufacture, and Test of Space and Ground Systems III. Editors T. B. Hull, D. Kim, P. Hallibert, and F. Keller (International Society for Optics and Photonics SPIE), 11820, 233–241. doi:10.1117/12.2594610
Thi, W.-F., Ménard, F., Meeus, G., Martin-Zaïdi, C., Woitke, P., Tatulli, E., et al. (2011). Detection of CH+emission from the Disc Around HD 100546. A&A 530, 530L2. doi:10.1051/0004-6361/201116678
Tiné, S., Roueff, E., Falgarone, E., Gerin, M., and Pineau des Forêts, G. (2000). Detection of Doubly Deuterated Ammonia in L134N. A&A 354, L63–L66.
Umemoto, T., Mikami, H., Yamamoto, S., and Hirano, N. (1999). The Ortho-To-Para Ratio of Ammonia in the L1157 Outflow. ApJL 525, L105–L108. doi:10.1086/312337
van der Marel, N., Kristensen, L. E., van Dishoeck, E. F., and van Dishoeck, E. F. (2011b). Complex Molecules toward Low-Mass Protostars: the Serpens Core. ApJ 740, 14. doi:10.1088/0004-637X/740/1/14
van der Tak, F. F. S., Black, J. H., Schöier, F. L., Jansen, D. J., and van Dishoeck, E. F. (2007). A Computer Program for Fast Non-LTE Analysis of Interstellar Line Spectra. A&A 468, 627–635. doi:10.1051/0004-6361:20066820
van der Tak, F. F. S., van Dishoeck, E. F., Evans II, N. J., and Blake, G. A. (2000). Structure and Evolution of the Envelopes of Deeply Embedded Massive Young Stars. ApJ 537, 283–303. doi:10.1086/309011
van der Walt, S., Colbert, S. C., and Varoquaux, G. (2011). The NumPy Array: A Structure for Efficient Numerical Computation. Comput. Sci. Eng. 13, 22–30. doi:10.1109/MCSE.2011.37
van Dishoeck, E. F., Kristensen, L. E., Benz, A. O., Bergin, E. A., Caselli, P., Cernicharo, J., et al. (2011). Water in Star-forming Regions with the Herschel Space Observatory (WISH): I. Overview of Key Program and First Results. PASP 123, 138. doi:10.1086/658676
van Dishoeck, E. F., Kristensen, L. E., Mottram, J. C., Benz, A. O., Bergin, E. A., Caselli, P., et al. (2021). Water in star-forming Regions: Physics and Chemistry from Clouds to Disks as Probed by Herschel Spectroscopy. A&A 648, A24. doi:10.1051/0004-6361/202039084
van Terwisga, S. E., van Dishoeck, E. F., Cazzoletti, P., Facchini, S., Trapman, L., Williams, J. P., et al. (2019). The ALMA Lupus Protoplanetary Disk Survey: Evidence for Compact Gas Disks and Molecular Rings from CN. A&A 623, 623A150. doi:10.1051/0004-6361/201834257
Visser, R., Bruderer, S., Cazzoletti, P., Facchini, S., Heays, A. N., and van Dishoeck, E. F. (2018). Nitrogen Isotope Fractionation in Protoplanetary Disks. A&A 615, A75. doi:10.1051/0004-6361/201731898
Walker, C. K., Chin, G., and Aalto, S. (2021). “Astronomical Optics,”. Astronomical Optics: Design, Manufacture, and Test of Space and Ground Systems III. Editors T. B. Hull, D. Kim, P. Hallibert, and F. Keller (International Society for Optics and Photonics SPIE), 11820, 181–232. doi:10.1117/12.2594847
Walsh, C., Loomis, R. A., Öberg, K. I., Kama, M., van ’t Hoff, M. L. R., Millar, T. J., et al. (2016). First Detection of Gas-phase Methanol in a Protoplanetary Disk. ApJ 823, L10. doi:10.3847/2041-8205/823/1/L10
Wang, S., Bergin, E. A., Crockett, N. R., Goldsmith, P. F., Lis, D. C., Pearson, J. C., et al. (2011). Herschel Observations of EXtra-ordinary Sources (HEXOS): Methanol as a Probe of Physical Conditions in Orion KL. A&A 527, A95. doi:10.1051/0004-6361/201015079
Widicus Weaver, S. L. (2019). Millimeterwave and Submillimeterwave Laboratory Spectroscopy in Support of Observational Astronomy. Annu. Rev. Astron. Astrophys. 57, 79–112. doi:10.1146/annurev-astro-091918-104438
Wiedner, M. C., Aalto, S., Amatucci, E. G., Baryshev, A. M., Battersby, C., Belitsky, V. Y., et al. (2021). Heterodyne Receiver for Origins. J. Astronomical Telescopes, Instr. Syst. 7, 011007. doi:10.1117/1.JATIS.7.1.011007
Willacy, K., Langer, W. D., and Velusamy, T. (1998). Dust Emission and Molecular Depletion in L1498. ApJL 507, L171–L175. doi:10.1086/311695
Yamaguchi, T., Takano, S., Sakai, N., Sakai, T., Liu, S.-Y., Su, Y.-N., et al. (2011). Detection of Phosphorus Nitride in the Lynds 1157 B1 Shocked Region. Publ. Astron. Soc. Jpn. 63, L37–L41. doi:10.1093/pasj/63.5.L37
Yang, Y.-L., Sakai, N., Zhang, Y., Murillo, N. M., Zhang, Z. E., Higuchi, A. E., et al. (2021). Erratum: The Perseus ALMA Chemistry Survey (PEACHES). I: The Complex Organic Molecules in Perseus Embedded Protostars. ApJ 910, 20. doi:10.3847/1538-4357/abdfd6
Young, K. E., Lee, J. E., Evans II, N. J., Goldsmith, P. F., and Doty, S. D. (2004). Probing Pre‐Protostellar Cores with Formaldehyde. ApJ 614, 252–266. doi:10.1086/423609
Keywords: astrochemistry, interstellar molecules, star-forming regions, far-infrared astronomy, space telescopes
Citation: Bergner JB, Shirley YL, Jørgensen JK, McGuire B, Aalto S, Anderson CM, Chin G, Gerin M, Hartogh P, Kim D, Leisawitz D, Najita J, Schwarz KR, Tielens AGGM, Walker CK, Wilner DJ and Wollack EJ (2022) Astrochemistry With the Orbiting Astronomical Satellite for Investigating Stellar Systems. Front. Astron. Space Sci. 8:793922. doi: 10.3389/fspas.2021.793922
Received: 12 October 2021; Accepted: 09 December 2021;
Published: 02 February 2022.
Edited by:
Ryan C. Fortenberry, University of Mississippi, United StatesReviewed by:
Luca Fossati, Austrian Academy of Sciences, AustriaNigel John Mason, University of Kent, United Kingdom
Copyright © 2022 Bergner, Shirley, Jørgensen, McGuire, Aalto, Anderson, Chin, Gerin, Hartogh, Kim, Leisawitz, Najita, Schwarz, Tielens, Walker, Wilner and Wollack. This is an open-access article distributed under the terms of the Creative Commons Attribution License (CC BY). The use, distribution or reproduction in other forums is permitted, provided the original author(s) and the copyright owner(s) are credited and that the original publication in this journal is cited, in accordance with accepted academic practice. No use, distribution or reproduction is permitted which does not comply with these terms.
*Correspondence: Jennifer B. Bergner, amJlcmduZXJAdWNoaWNhZ28uZWR1
†NASA Sagan Fellow