- 1Institute of Molecular Biology and Genetics of NASU, Kyiv, Ukraine
- 2Department of Biochemistry, Centre for Bioinformatics and Computational Biology, Genetics and Microbiology, University of Pretoria, Pretoria, South Africa
- 3Department of Biological Sciences, Universidade Estadual de Santa Cruz (UESC), Ilhéus, Brazil
- 4Departamento de Genetica Ecologia e Evolucao, Instituto de Ciências Biológicas, Universidade Federal de Minas Gerais, Belo Horizonte, Brazil
- 5Centre for Genomics and Applied Gene Technology, Institute of Integrative Omics and AppliedBiotechnology (IIOAB), Purba Medinipur, India
- 6Center of Applied Space Technology and Microgravity, University of Bremen, Bremen, Germany
- 7Laboratory of Astrobiology and Molecular Biology of Cyanobacteria, Department of Biology, University of Rome Tor Vergata, Rome, Italy
- 8Analog Astronaut Training Centre, Rzeszów, Poland
- 9ILEWG EuroMoonMars, Leiden Observatory, VU Amsterdam & ESA ESTEC, Amsterdam, Netherlands
- 10HE Space Operations B.V., Noordwijk, Netherlands
- 11Microgravity User Support Center, German Aerospace Center, Cologne, Germany
Humankind has entered a new era of space exploration: settlements on other planetary bodies are foreseen in the near future. Advanced technologies are being developed to support the adaptation to extraterrestrial environments and, with a view on the longer term, to support the viability of an independent economy. Biological processes will likely play a key role and lead to the production of life-support consumables, and other commodities, in a way that is cheaper and more sustainable than exclusively abiotic processes. Microbial communities could be used to sustain the crews’ health as well as for the production of consumables, for waste recycling, and for biomining. They can self-renew with little resources from Earth, be highly productive on a per-volume basis, and be highly versatile—all of which will be critical in planetary outposts. Well-defined, semi-open, and stress-resistant microecosystems are particularly promising. An instance of it is kombucha, known worldwide as a microbial association that produces an eponymous, widespread soft drink that could be valuable for sustaining crews’ health or as a synbiotic (i.e., probiotic and prebiotic) after a rational assemblage of defined probiotic bacteria and yeasts with endemic or engineered cellulose producers. Bacterial cellulose products offer a wide spectrum of possible functions, from leather-like to innovative smart materials during long-term missions and future activities in extraterrestrial settlements. Cellulose production by kombucha is zero-waste and could be linked to bioregenerative life support system (BLSS) loops. Another advantage of kombucha lies in its ability to mobilize inorganic ions from rocks, which may help feed BLSS from local resources. Besides outlining those applications and others, we discuss needs for knowledge and other obstacles, among which is the biosafety of microbial producers.
Introduction
Long-duration stays on the Moon are scheduled to start this decade (Foing et al., 2018, 2021; Heinicke and Foing, 2021; Silk et al., 2021). One may realistically expect the first steps on Mars to take place within the subsequent few years and to be followed, on a longer term, by settlements on the red planet (Ehrenfreund et al., 2012; Musk, 2017). Advanced biotechnologies could be central to the fulfilment of such plans: they lend themselves to highly efficient use of resources and tend to minimize both waste generation and environmental impact (Camere and Karana, 2018), which will be (or, we argue, should be) priorities of settlements on other celestial bodies. Bioregenerative life-support systems (BLSS; confined self-sustained artificial ecosystems for the generation and recycling of life-support consumables), in particular, may be key to meeting those constraints (Mitchell, 1994). Besides basic BLSS functions, the dependency on Earth could be decreased by the biofabrication of goods such as food, fabrics, construction materials, and medicines. The use of local resources (in situ resource utilization, ISRU) could decrease the reliance of bioprocesses on Earth-imported materials and, thus, improve their cost-effectiveness. Researchers and engineers are, for instance, designing ways of growing plants on lunar or Martian analogue substrates (Lytvynenko et al., 2006; Zaets et al., 2011; Duri et al., 2020), possibly after processing them using microorganisms to release nutritive elements (Auerbach et al., 2019; Castelein et al., 2021).
Here, we argue that the kombucha microbial community (KMC) is worth considering as a multipurpose component of crewed space missions. Kombucha has been consumed for millennia as a health-promoting drink. Its use beyond Earth has been considered for over a decade (Kozyrovska and Foing, 2010; Kozyrovska et al., 2012), and the idea gained momentum in the frame of the BIOMEX project (de Vera et al., 2019; Reva et al., 2015; Zaets et al., 2016; Podolich et al., 2017a,b; 2019; 2020; Góes-Neto et al., 2021; Orlovska et al., 2021; Lee et al., 2021). The applications of KMC we envision can be gathered in two broad categories: 1) prophylaxis of spaceflight-related health disorders, based on both live nutraceuticals and cell-free postbiotics (as well as, perhaps more anecdotally, the psychological benefits of tending cultures), and 2) resource production, which encompasses functions in BLSS/ISRU processes as well as, especially in advanced settlements, the waste-free fabrication of consumer goods, such as comfort drinks and products derived from bacterial cellulose.
The aim of this review is to provide insights into how KMC could be integrated into BLSS for the Moon and Mars, draw attention to obstacles on which research efforts should be focused, and give an overview of the foreseen benefits. Some applications we consider are well-proven and could be implemented at little cost; others may be more speculative and harder to deploy in the foreseeable future. If we elected to include the latter as well, it is with the goal of stimulating constructive discussions, which may help evidence which among them are worth pursuing.
Defining the relevance and feasibility of using KMC beyond Earth will require significant research efforts. We suggest that such efforts are justified, as the benefits may be vast.
Kombucha per Se: Echoes of the Past or Back to the Future
Kombucha as a Multimicrobial Community
KMC is an example of advanced microbial social interactions between representatives of two domains of living beings, based on metabolic cooperation and competition (May et al., 2019). More specifically, it is a mutualistic multiculture of acetic acid bacteria (AAB) and osmophilic yeasts, which produces an acidic medium at the surface of which floats a cellulose-based pellicle biofilm. This community is not found in nature; when it was formed is not precisely known, but it is thought of having existed in anthropic environments (human habitations) for over two millennia. The core bacterial community within KMC is dominated by AAB: representatives of the Komagataeibacter, Acetobacter, and Gluconobacter genera (Jayabalan et al., 2014). The relative dominance of yeast genera, such as Zygosaccharomyces, Brettanomyces/Dekkera, Schizosaccharomyces, Saccharomyces, and Pichia, varies in accordance with the geographical variants of KMC (Jayabalan et al., 2014). Lactic acid bacteria are involved in the fermentation process in some KMC ecotypes (Chakravorty et al., 2016; Coton et al., 2017). The most beneficial genera detected in KMC samples were Bacteroides and Prevotella, which are known as dominant human gut microbiota members (Lavefve et al., 2021). Metagenomics studies also predicted opportunistic bacteria like Bacillus, Pseudomonas, etc. (Arikan et al., 2020; Villarreal-Soto et al., 2020; Barbosa et al., 2021; Harrison and Curtin, 2021), as well as bacteriophages and yeast viruses (Góes-Neto et al., 2021). There is a metabolic interplay between yeasts that convert sugar into monosaccharides and ethanol, in a liquid phase, and AAB that use glucose, fructose, and ethanol to produce organic acids and bacterial cellulose (BC), in a pellicle film phase (Villarreal-Soto et al., 2018). The biofilm, as a three-dimensional microbial hub, supports an evolutionary stable social cooperation between its inhabitants, in a way that is analogous to tissues in multicellular organisms. The biofilm may also optimize oxygen concentrations for the microbial microcolonies, which are stratified in the cellulosic matrix, as well as protect the community from environmental stressors such as UV radiation (Williams and Cannon, 1989). The capability of KMC to generate and tolerate acidic conditions, metabolize ethanol, and produce organic acids, protects the system from invasion by competitor microbes. The bacterially produced cellulose-based pellicle biofilm may also provide protection from contaminants by inhibiting the diffusion of their extracellular metabolites. The KMC as a multipurpose ecosystem has the potential for usage in wide sectors of human activity. The areas of application of kombucha microbial culture products are represented in Figure 1.
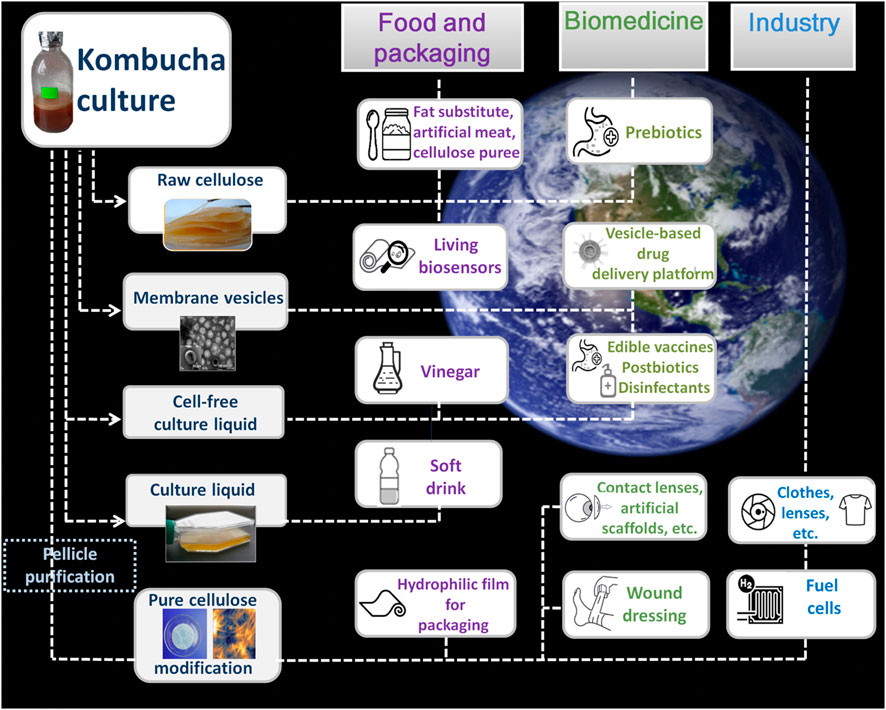
FIGURE 1. Kombucha’s cellulose-based pellicle as a three-dimensional hub for microbial community-member cells and raw material for fermented food and consumer goods fabrication. Background image credits: ESA.
Kombucha as Health-Promoting Products
Home-made drinks and pellicle films have been used for prophylaxis, as well as for the treatment of various ailments, before kombucha was widely commercialized (Danielian, 2005). Cultivation was typically initiated by placing a piece of a kombucha pellicle biofilm in a sweetened tea infusion. Owing to its antimicrobial properties, which were later evidenced (Sreeramulu et al., 2000), and its presumed detoxifying and energizing properties (Jayabalan et al., 2016), kombucha’s products served as medicines for the lack of synthetic drugs. In clinical settings of the former U.S.S.R., planktonic cultures were used against severe alterations of the arterial pressure, various forms of acute tonsillitis, chronic enterocolitis, and bacterial dysentery. The cellulose-based pellicle, which resembles mammal skin, has high biocompatibility and water holding capacity, and contains valuable metabolites, was used as a matrix for skin regeneration and healing (Barbanchik, 1954). Highly acidic solutions obtained by long fermentation times were used to disinfect wounds or household items during epidemics (Danielian, 2005). Such uses were largely abandoned with the rise of the pharmaceutical industry.
In the 90s, kombucha grew as a commercial product and rapidly regained popularity (Kim and Adhikari, 2020), which drew scrutiny on its putative health properties. Reconstructions from kombucha metagenomes (Arıkan et al., 2020; Villarreal-Soto et al., 2020; Góes-Neto et al., 2021) predicted the production of various health-supporting components, such as short-chain fatty acids (SCFA), which influence the gut-brain communication (Silva et al., 2020); polyunsaturated fatty acids, which are essential for brain activity and reduce the risk of heart disease (Freitas et al., 2018); glutamate, a neurotransmitter known for its role in mental health (Zhou and Danbolt, 2014); glucosamine, an amino sugar known to support the function of healthy joints (Leal et al., 2018); and a variety of vitamins (B1, B2, B3, B6, B12, C, K2). Several organic acids, including detoxifying glucuronic acid (Martínez-Leal et al., 2020), sugars, polyols, kombucha-specific theobromine, rutin, quercetin and chlorogenic acid, were detected experimentally (Tran et al., 2020; Villarreal-Soto et al., 2020; Barbosa et al., 2021). In vitro assays and model experiments on animals demonstrated antioxidant, anticancer, antiviral, and anti-inflammatory activities (Mousavi et al., 2020). Nonetheless, in vivo assays remain scarce, and clinical trials are lacking to ascertain the health benefits of drinking kombucha (Morales, 2020). Given the fast-increasing interest in this beverage, one may expect more conclusive evidence in the near future.
Kombucha Multimicrobial Community Are Highly Resilient Under Martian Conditions Simulated Beyond Earth
A common pitfall in BLSS modules or other microbial cultures foreseen for biotechnologies in space is an expected lack of stability under the unusual culture conditions found (or anticipated) in facilities beyond Earth. The resilience of KMC was put to an extreme test in the Biology and Mars Experiment (BIOMEX): biofilm samples of the KMC ecotype IMBG-1 (Ukraine) were exposed at the outer surface of the International Space Station (ISS) to simulated Mars-like conditions for 18 months (de Vera et al., 2019; Podolich et al., 2019). KMC IMBG-1 tolerated those conditions; however, shotgun metagenomic analyses showed that the community was altered with an overall decrease in diversity and richness (the number of species in a community) of microorganisms (Góes-Neto et al., 2021). Among others, the relative diversity of dominant Komagataeibacter spp. increased, suggesting that stress exposure had fostered the development of bacterial species usually found below detection levels. The most striking changes were found in the Komagataeibacter representatives after exposure to the Mars-like UV spectrum: the relative abundance of K. saccharivorans (which is normally dominant) decreased while that of K. hansenii increased. Viral species in KMCs also increased after the exposure experiment. On the other hand, the diversity of the yeast component was not affected significantly by space exposure; however, the dominant genus Schizosaccharomyces was replaced with other yeast genera (Góes-Neto et al., 2021). Results of this metagenomic study will be used for the design of robust, rationally assembled mini-kombuchas composed of both core community members and purposely selected microbial partners. The composition of extracellular membrane vesicles (EMVs), which are thought to mediate communication between cells within and across domains, was altered in the post-flight KMC community members (Podolich et al., 2020). The changed composition of membranes correlated with changes in EMV fitness: the activity of membrane-associated enzymes seemed to be increased by UV radiation.
In spite of those alterations, exposure in space did not lead to significant differences in the community functions, suggesting that kombucha samples were ecologically resilient (Góes-Neto et al., 2021). It should also be noted that conditions outside the ISS were far more challenging than those foreseen for KMC when used in support of crewed missions, where it would be grown mostly in crewed modules and, if cultivated outside, would be contained in a bioreactor (and, thus, would not be exposed to vacuum, UV, or extreme temperatures).
Biosafety of the Kombucha Microbial Community
Biosafety Risks Associated With Microbial Communities
Any crewed mission will be accompanied by microorganisms: they are both necessary and inevitable (see, for instance, Guerrero and Berlanga, 2009; Lloyd-Price et al., 2016). Around half of the microorganisms on inner surfaces of crewed compartments is expected to be represented by the crew’s microbiota (Singh et al., 2018; Checinska et al., 2019; Avila-Herrera et al., 2020), the rest coming from a variety of sources (e.g., imported consumables, various environmental sources, BLSS). The resulting indoor ecosystem should be well-understood: its mismanagement may lead to the emergence of pathogens (or opportunistic pathogens) which would threaten the crew’s health, as well as that of technophiles which might damage equipment.
In order to avoid such a situation in the new indoor environments that will be created by settlers, the philosophy of the interdependence of humans and living microbial beings in the context of co-becoming fates has to go beyond anthropocentrism. The more diverse the microbiota, the better the balance of useful and opportunistic/detrimental counterparts, and the healthier the ecosystem from the standpoint of human health. A reasonable approach to the maintenance of microbiome health is a reconstruction of an Earth-like biosphere and interrelationships with the surrounding biota through a BLSS, as described by Hao et al. (2018) and Chen et al. (2020). The contact with plants for several hours in a day and the consumption of plant fiber diet would increase the relative abundance of friendly microbiota and would reduce proportions of potential pathogens in the crew.
More pragmatically, extensive efforts should be deployed to characterize and, to some extent, design the microbial communities that will accompany crews; in other words, to diversify the microbiota in the indoor ecosystem to reduce the risk of acquired overcompetitiveness. KMC, as a microecosystem organized on metabolic symbiosis and which evolved alongside humans, could contribute to the establishment of an environment free of human pathogens.
Kombucha Microbial Community Biosafety: What Is Known From Our Shared History on Earth
The biosafety of KMC is known from its use over millennia as well as from recent studies (Dutta and Paul, 2019; Martínez-Leal et al., 2020), including some based on metagenomics (Arikan et al., 2020; Villarreal-Soto et al., 2020; Góes-Neto et al., 2021). While adverse health effects have been attributed to the consumption of home-brewed kombucha (Murphy et al., 2018), such cases have been reported very rarely and were probably related to inappropriate cultivation processes, which led to contamination of the product with pathogenic bacteria. Nevertheless, the United States Food and Drug Administration (FDA, 1995) states that kombucha is safe for human consumption when properly prepared, and kombucha, therefore, has been commercialized in a large number of countries (e.g., Kim and Adhikari, 2020).
Komagataeibacter xylinus, a keystone species of KMC, is recognized as a safe microorganism for human consumption. The safety status of K. xylinus was determined by feeding rats with doses of 1016 bacterial cells (Lavasani et al., 2019). Moreover, the toxicological and dietetic aspects of BC have been assessed in a rat model, which led to the conclusion that BC is safe for applications in food technology (Dourado et al., 2017). From a nutritional point of view, BC can be considered a promising low-calorie fiber-rich ingredient or fat substitute. Cellulose generated by Komagataeibacter spp. can be a valuable resource for the food industry in compliance with the current legislation of some countries, e.g., the FDA considers BC as GRAS (Vigentini et al., 2019). Nevertheless, the EFSA (European Food Safety Authority) scientific panel has not decided yet on this issue, in spite of the fact that the chemical structure of BC is well known and identical to that of the vegetable cellulose. Furthermore, AAB that synthesize it are recognized as GRAS, belong to the risk group 1, and are components of fermented foods with a long history of safe human consumption.
Kombucha Microbial Community Biosafety: What Is Known From a Space Experiment
A recent shotgun metagenomic study on the microbial community structure in kombucha exposed to open space and Mars-like conditions on the ISS demonstrated a disturbing effect of abiotic stressors on the KMC relative abundance and taxonomic composition (Góes-Neto et al., 2021). Nonetheless, KMC core microbial community members were preserved, providing the community with needed gene sets for the formation of a cellulose-based pellicle, which allowed for the survival of the community. After 2 years of subculturing in lab conditions on Earth, the returned KMCs displayed vulnerability for contaminations that should be considered as a possible safety risk. It is worth noting that the KMC was exposed to extremely harsh conditions of real space and simulated Martian conditions that never would occur at human habitation where KMC would be cultured.
In addition to the risk of contamination, undesirable changes in the fermentation process and activation of probable pathogenic factors present in genomes of kombucha community inhabitants must be investigated in detail in future studies. In our recent study, despite alterations in membranes, kombucha’s EMVs did not acquire endotoxicity, cytotoxicity, or neurotoxicity (Podolich et al., 2020). Metagenome-assembled genomes did not show the presence of pathogenic microorganisms in KMC IMBG-1, neither before nor after the exposure experiment (Lee et al., 2021). We were also interested in deciphering genomes of core community members in order to analyze them on the presence of genes for toxins and toxin transport systems, extracellular enzymes, genetic mobile elements, and so on. Recently, two dominant bacterial strains of K. oboediens isolated from the dehydrated KMC biofilms (ESA BIOMEX), one maintained on Earth and another sent to space/Mars-like conditions on the ISS, had their genomes sequenced and extensively compared. Despite some differences in phenotypic features (Podolich et al., 2020), the genomic analysis revealed that their genomes were quite similar (de Carvalho et al., 2021; personal communication), which made us consider epigenetic events on the space-flown strain.
It is time to examine both the accumulated experience on human coexistence with the KMC microecosystem and the scientific evidence on its unique properties, and to rigorously assess its value as a potential functional food/edible vaccine and environmentally-friendly materials in the context of crewed missions to other planets. The known advantages of KMC as a companion to astronauts and settlers come from the fact that it is: a self-organized system that can be purposively modified and is resistant to contamination; tolerant to space/Mars-like stressors; simple to cultivate; easy to transport; a multimicrobial multifunctional community; ban immortal bank of probiotic and cellulose-synthesizing bacteria and yeasts of biotechnological importance; a multivariate functional food (as a drink, smoothies, candies, vinegar, etc.); a prophylaxis and healing agent; an indicator of local biosafety; a means of psycho-emotional relaxation (taking care of mother culture, handicrafts, and installations using bacterial cellulose, etc.).
The putative strategies for using KMCs, including purposively upgraded variants, for space exploration are summarized in Figure 2 and outlined below.
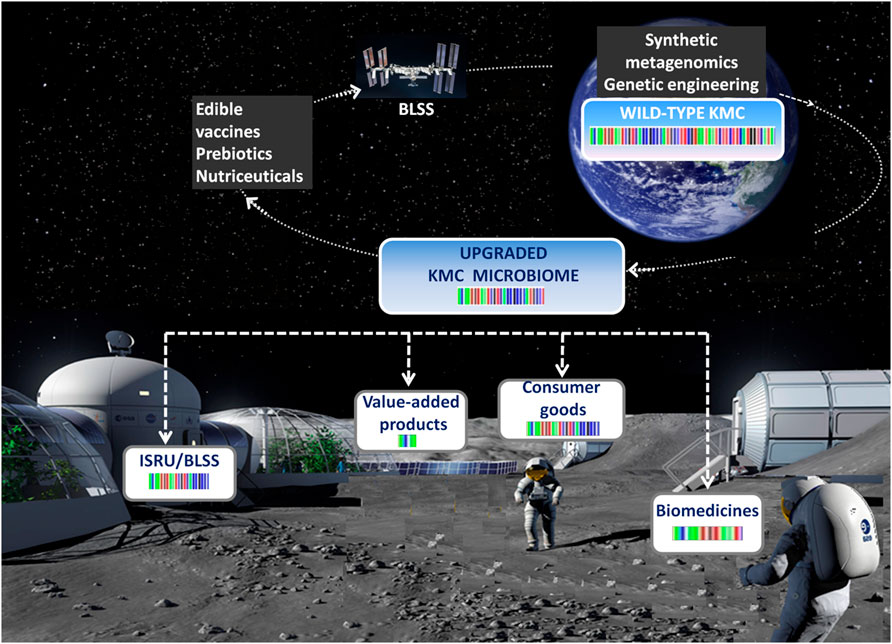
FIGURE 2. A model scheme of the kombucha’s travels from the laboratory to the International Space Station and extraterrestrial settlements.
Rationally Assembled Kombucha Microbial Community Could Support Astronaut Health Long-Term Space Missions
During Missions, the Improvement of Human Health Will Be Profitable Under the Assistance of Health-Promoting Microorganisms
Among the physiological changes undergone by crewmembers in space is a modification of the composition and functionality of the gut microbiome (e.g., Voorhies et al., 2019). The highly comprehensive analyses of the human microbiome using high-throughput “omics”-based technologies evidenced a clear link between gut microorganisms and health problems (Gilbert et al., 2018; Parker et al., 2020). The human intestinal microbiota is essential for microbial homeostasis, regulation of metabolism and immune tolerance (Ahlawat et al., 2020), and a deficit in bacterial products can deregulate brain function, leading to neurobehavioral problems (Cryan et al., 2019). A decrease in the richness of probiotic bacteria in the gut was observed in spaceflight, notably in NASA’s Twins Study (Garrett-Bakelman et al., 2019), alongside a reduction in the number of anti-inflammatory bacteria (Voorhies et al., 2019). Altogether, these results indicated that gut microbiome alterations predispose astronauts to illnesses (reviewed in Turroni et al., 2020). Thus, the maintenance of healthy gut microbiota during interplanetary journeys to Mars or other celestial bodies will require means of mitigation.
There are several countermeasures that might be investigated for mitigating dysbiosis in crews: 1) modification in nutrient supply (David et al., 2014; Fetissov, 2017); 2) non-selective modification of the gut microbiome using fecal microbiota transplantation (Cheng et al., 2019; Guo et al., 2020); 3) semi-selective modification of the gut microbiome using antibiotics (Bajaj et al., 2018); and 4) biological modification of the intestinal barrier. All the aforementioned approaches have pros and contra. Prophylaxis and correction of dysbiosis with microbiome-targeted therapeutics, such as probiotics/synbiotics/postbiotics (Box 1), can reasonably be expected to be beneficial but have not been used so far.
Box 1Probiotics, prebiotics, synbiotics, and postbiotics
Probiotics are viable microorganisms whose health effects are independent of the site of action and route of administration. The potential benefits of probiotics were first proposed over a century ago by the 1908 Nobel Prize winner Elie Metchnikov (Metchnikoff, 1908), and because of him, fermented milk products entered the daily diet. The term “probiotic” was introduced by Lilly and Stillwell (1965). Later, probiotics were officially defined by the FAO and WHO as “live microorganisms that, when administered in adequate amounts, confer a health benefit on the host” (FAO/WHO, 2002; Hill et al., 2014). Probiotics are efficient in treating gut-associated disorders, including allergic disorders and metabolic syndrome (Molina-Tijeras et al., 2019), and improving local immunity. Prebiotics are non-digestible food ingredients resistant to destruction by mammalian body enzymes but metabolized by intestinal microbiota and that stimulate, therefore, the growth and/or activity of intestinal bacteria associated with health (Gibson et al., 2017). This definition will continue to evolve in line with expanding knowledge about dietary fiber. Synbiotics are health-promoting products, containing a combination of prebiotic and probiotic products. Postbiotics are overall cell-free metabolites or separate components, e.g., extracellular membrane vesicles of probiotic bacteria.
In spite of the interest in probiotics for astronauts’ diets (Voorhies et al., 2019; Turroni et al., 2020), the in-flight safety of probiotic microorganisms remains unclear. Currently, neither probiotic/synbiotic products nor its structural components were evaluated on the potential risk for astronauts, except for the first exposure of lactic acid probiotic bacteria preparations during a 1-month trial on the ISS (Sakai et al., 2018), which showed that this probiotic had not lost basic therapeutic properties. Further profound systemic studies will be required to determine the safety and stability of probiotic microorganisms during space missions. Criteria for the scientific substantiation of health claims on foods and issues on probiotics, synbiotics, postbiotics and prebiotics remain to be addressed (Vargas et al., 2021).
Kombucha is a functional food (Dimidi et al., 2019) with features of a probiotic product as it contains lactic acid bacteria (LAB) and probably probiotic yeasts (Shizosaccharomyces, Saccharomyces, and Lachancea), as mentioned above. The main LAB strains isolated from kombucha are the well-known Lactobacillus bulgagricus, Streptococcus thermophilus, and Lactobacillus plantarum (Pei et al., 2020), as well as Pediococcus pentosaceus (Matei et al., 2018). Marsh et al. (2014) reported that Lactobacillus and Lactococcus are dominant in kombucha pellicles, and the content of LAB depends on the kombucha’s origin. LAB isolated from kombucha are considered potential probiotics since they meet most of the probiotics criteria, e.g., bacteriocin production and bile salt tolerance, in according to the FAO/WHO Guidelines for the Evaluation of Probiotics in Food (2002) (Matei et al., 2018; Pei et al., 2020; Vargas et al., 2021). Incoming LAB easily become community members (Zaets et al., 2016; Cvetković et al., 2019; Bueno et al., 2021) and improve kombucha’s biological properties, e.g., antibacterial and antioxidant activities (Nguyen et al., 2015). Another approach is to design rationally assembled (upgraded) kombucha composed of the defined health-promoting bacterial and yeast species capable of kombucha fermentation (Savar et al., 2021).
Kombucha Dietary and Prophylaxis Projects During Spaceflights
Whether Komagataeibacter spp. (and other dominant members of the kombucha community) behavior as probiotics has not been ascertained, these bacteria possess several characteristics which suggest that research on their dietary properties could be fruitful. First of all, their product, cellulose polymer, which is non-digestible in the human gut but can be consumed by cellulolytic bacteria in the distal gut, can be considered a prebiotic (Holscher, 2017; Nsor-Atindana et al., 2020). Low-fiber diets lead to slow gastro-intestinal transit and a microbiome starving in crewmembers. Cellulose is useful for digestion because it stimulates the work of intestines that increases stool bulk and water absorption, and, thereby, decreases the gastrointestinal transit time. Earlier, we proposed KMC as a source of prebiotic for astronauts (Kozyrovska et al., 2012), and most recently, kombucha fermentation was reported to produce SCFAs (Utoiu et al., 2018; Annunziata et al., 2020) or support a significant potential prebiotic effect (Lavefve et al., 2021). The AAB, producing cellulose, are food-grade (Alexandraki et al., 2013) or GRAS bacteria (Gorgieva and Trček, 2019), which gives Komagataeibacter species potential for pro/pre/postbiotic use. Among them, K. xylinus, one of the dominant core kombucha bacteria, exhibited some probiotic features: it was shown to survive in the conditions of a rat gut tract, being resistant to acid, bile, low oxygen pressure, and rat body temperature (Lavasani et al., 2019). Komagataeibacter cells contain valuable antioxidants and lipids which can improve cognitive functions (Fukami et al., 2009) and prevent stratum corneum dryness (Tsuchiya et al., 2020).
During space missions, crewmembers experience nutrient deficiency because of a reduced appetite compared to that on Earth, for many reasons (Turroni et al., 2020). Correction of nutrient and energy deficiency by live kombucha-related products is a reasonable and facile way to enhance energy level and crew’s appetite under specific conditions. Both beverage and pellicle film possess inherently a great collection of various essential and valuable dietary compounds highlighted above, always available fresh and organic, which probably could aid in prophylaxis of health problems in parallel with pharmacological interventions, lowering doses of medicines. Special attention should be given to kombucha’s energizing capability: the formation of the iron and gluconic acid chelating complex increases the level of hemoglobin and stimulates ATP synthesis under the support of energy regeneration by the vitamin B group that promotes enzymatic activation of lipid and protein metabolism (Dufresne and Farnworth, 2000).
In addition to both nutrient and energy deficiency, various health risks (zero- and reduced-gravity effects, ionizing radiation, etc.) affect several crewmembers’ organs and systems during missions. Beyond Earth’s protective magnetosphere, the exposure of astronauts to galactic cosmic radiation could result in degenerative tissue diseases (Thirsk, 2020). A high oxidant and low antioxidant level in the human body is associated with the development of chronic inflammatory diseases, such as cardiovascular and neurologic diseases (Barcellos-Hoff et al., 2015; Vernice et al., 2020) and, thus, lead to deregulation of the immune and metabolic systems (Garrett-Bakelman et al., 2019). Intake of dietary antioxidants may inhibit the formation of peroxides and their absorption in the gastrointestinal tract (Xia et al., 2019). Kombucha-derived products, such as beverage and pellicle-based products, exhibit antioxidant capacity, which has been associated with the presence of polyphenols and derived phenolic compounds, such as gallic acid, caffeic acid, chlorogenic acid, p-coumaric acid, and ferulic acid among many others (Villarreal-Soto et al., 2020). Furthermore, kombucha modulates the immune system in animals, inducing the production of anti-inflammatory cytokines (e.g., IL-4 and TGF-β) and inhibiting the production of pro-inflammatory cytokines, such as IFN-γ and IL-17 (Haghmorad et al., 2020). The microbiome shift is one of the six fundamental changes in human bodies during spaceflights (Afshinnekoo et al., 2020), and it is already known that kombucha brew intake corrects changes in intestinal microbiota promoted by non-alcoholic fatty liver disease (Jung et al., 2019).
An Alternative Approach to the Mitigation of Crew’s Health Dysfunctions: In Situ Biofabricated Acellular Postbiotics
Microorganisms may not be the only players in probiotic products that affect the recipient’s biology and safety; their extracellular membrane vesicles (Box 2) could contribute to health effects under the changed conditions.
BOX 2Extracellular membrane vesicles
EMVs are spherical, nanosized (20–400 nm) bilayered proteolipids that contain different molecular cargo from the cytosol, periplasm, and cellular membranes. EMVs are released by cells of all the three domains of life represented in the KMC, however, depending on their origin, vesicles differ in unicellular and multicellular organisms, but all the EMVs facilitate intercellular communication by shuffling molecular cargo between cells. Proteomic and biochemical analyses have shown that EMVs of Gram-negative bacteria contain a variety of bacterial components from the outer membrane (like lipopolysaccharide and lipoproteins), periplasmic proteins, DNA, and RNA species, which have been suggested to modulate biological processes, such as biofilm formation, survival, competition, defense, etc. Moreover, EMVs of probiotic bacteria mediate communications with the host via molecular pattern molecules, interacting with innate immune receptors (reviewed in Nagakubo et al., 2020). Nano-sized bacterial EMVs withstand long-time circulation through body organs and fluids and are able to penetrate the blood-brain barrier. Eventually, their effect on living systems is comparable to that of the gut microbiota (Muraca et al., 2015; Tulkens et al., 2018).
Several members of the KMC produce EMVs, which could serve a range of health-related purposes. In this case, microbial EMVs could act as a surrogate of probiotics, carrying the same bioactive molecules but limiting the risks associated with the administration of live bacteria (Kozyrovska et al., 2018; Podolich et al., 2020), or act as living edible vaccines.
Hereafter, we report examples of such applications. Some microbial EMVs carry surface immunomodulatory proteins that could be used for protective mucosal immunization through different routes (oral, nasal, rectal, vaginal), to facilitate the restoration of normal immune responses (Gorreja, 2019; Kuhn et al., 2020). The probiotic Propionibacterium freudenreichii produces EMVs that export proteins (e.g., the surface-layer protein B), which interact with the host proteins and attenuate inflammation (Rodovalho et al., 2020). EMVs from various bacteria regulate brain functions by interacting with the peripheral nervous system and delivering neurotransmitters to the central nervous system, which may lead to novel therapeutic approaches to neurodevelopmental and mood disorders (Haas-Neill and Forsythe, 2020). Furthermore, EMVs may also be used to alleviate metabolic dysfunctions (Ashrafian et al., 2019). As the last example, bacterial EMVs are emerging as nanodrugs (Jahromi and Fuhrmann, 2021) and platforms for drug delivery (Li et al., 2020).
Rather than being sent from Earth, EMVs could be produced on-site, which would circumvent issues related to long-term conservation. This could be facilitated by the fact that bacterial EMV production seems to be intensified in space (Zea et al., 2017; Ott et al., 2020). EMVs from ground control and space-flown KMC samples did not exhibit signs of cyto-, endo- or neurotoxicity (Podolich et al., 2019), and neither did EMVs of the dominant kombucha community member Komagataeibacter oboediens (Podolich et al., 2020): membrane alterations occurred but did not lead to toxicity for eukaryotic cells in vitro. This experience suggests that EMVs, originating from carefully selected, non-pathogenic Gram-negative bacteria such as K. oboediens, can be considered as candidates for postbiotics or edible mucosal vaccines produced in situ, beyond Earth.
Kombucha Microbial Community Could Be a Practical Element of the ISRU-Based Bioregenerative Life Support System on Mars and the Moon
The KMC could find application in ISRU-based BLSS, either as a primary goal or via satellite products from other programs (health support, cellulose production, etc.) (Figure 3).
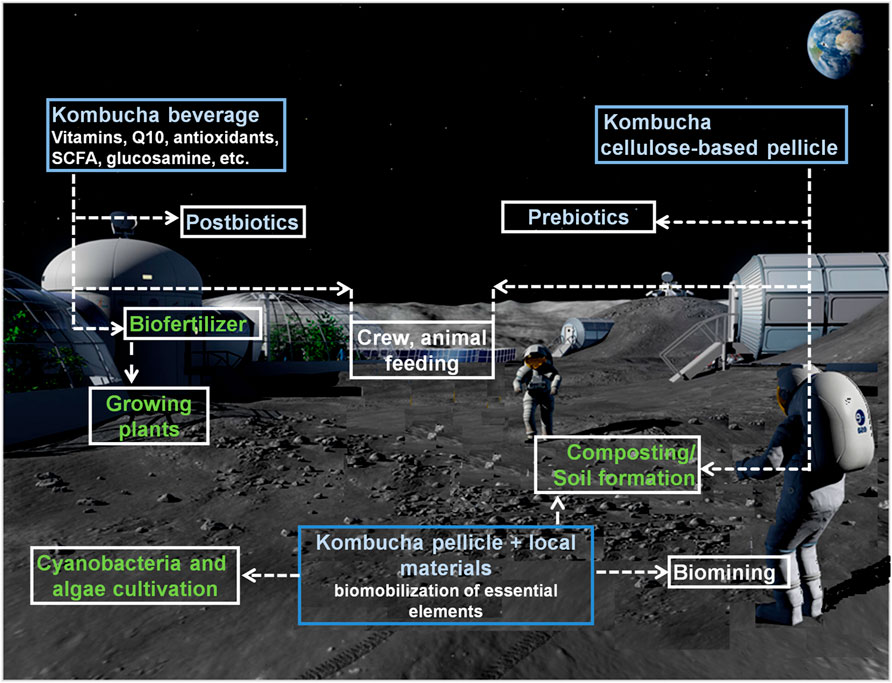
FIGURE 3. A schematic presentation of different approaches for manipulation of the kombucha microbial community to enhance life-support efficacy in extraterrestrial settlements.
Kombucha for Growing Plants in BLSS
The lunar and Martian regoliths are expected to contain all the mineral nutrients required for plant growth (Helmke and Corey, 1989). However, whether either could support plant growth has not been fully demonstrated. The biological tests performed with Moon regolith during and shortly after the Apollo years (reviewed in Ferl and Paul, 2010) mostly aimed at ensuring that the regolith did not present any risk to the Earth’s biosphere. As for Mars, no regolith sample has been brought back so far. Plants were successfully germinated and grown in Mars regolith simulants (Wamelink et al., 2014; Duri et al., 2020), but those differ from the regolith they mimick in ways that are critical to biological experiments. Generally speaking, it is thought that regolith from both bodies would require some physical, chemical, and/or biological modifications (e.g., Ming and Henninger 1994; Maggi and Pallud, 2010; Fackrell et al., 2021) before use as a substrate for plant growth, due to, for instance, poor water retention, low availability (or release up to toxic concentrations) of mineral nutrients, toxins, root damage by abrasive particles, and/or lack of metabolizable nitrogen. These limitations may be addressed by using microorganisms (e.g., Ehrlich, 1989; Ming and Henninger, 1994; Zaets et al., 2011): some may coat the sharp edges of grains, thereby mitigating damage to plant roots (Slenzka and Kempf, 2010); their biomass would enrich the soil in organic compounds, improving both fertility and water retention; and microorganisms capable of bioleaching could foster the release of essential elements (Wamelink et al., 2014). Associated to plant roots in anorthosite, microbial communities were shown to help balance levels of mineral nutrients, favoring release while preventing accumulation to toxic levels (Zaets et al., 2011). Thus, microbial communities, possibly planktonic KMC, could have a positive impact on plant growth, either as an inoculant or by pre-processing the regolith. For the case where such a treatment would not suffice for growing plants of high interest, an approach has been proposed where “first-generation plants” (i.e., plants with higher fitness in such an environment, such as Tagetes patula or Kalanhoe daigremontiana) would be grown first, supported by microbial communities, and composted to enrich a protosoil itself used for plants of direct interest such as calorie-dense crops (e.g., Lytvynenko et al., 2006; Zaets et al., 2011). In line with this, Duri et al. (2020) suggested planting leafy vegetables on Martian regolith ameliorated with organic materials produced in situ. A composted kombucha mat could be an additional source of nutrients and other plant-supporting biologicals.
Another approach may lie in using such communities to mobilize ions from regolith beforehand, for the production of fertilizers. It was shown that in a tea infusion, supplementation with anorthosite led to an accumulation of inorganic ions in a KMC cellulose biofilm matrix: metals concentrations exceeded that in the anorthosite-free matrix by, for instance, 10 times for Ca, and 43–51 times for Mn and Mg (Zaets et al., 2014). Inorganic ions accumulated in the film can readily be extracted. The presence of KMC also increased the concentration of ions in the anorthosite-supplemented tea: after 1.5 months, for instance, the concentrations of Mn, Cu, and Zn increased 5–7 times, and Mg and Fe 12–22 times, compared to a medium with anorthosite but without KMC. The extensive growth of the SiO2 group of secondary minerals on the bottom surface of the pellicle evidenced the primary dissolution of the silica-rich minerals in the anorthosite rock, and its accumulation on and around the pellicle (Podolich et al., 2017a). Kombucha’s leaching activity presumably stems from metabolites (organic acids, siderophores, enzymes) and a proton excess (Hopfe et al., 2017). Increased levels of EMVs production were also noted in the KMC grown with anorthosite, which correlated with the alteration of the ionome in the pellicle. Furthermore, Al and Fe were found in the pure preparations of EMVs isolated from KMC grown in the presence of anorthosite (Podolich et al., 2017a), consistently with observations by Matlakowska et al. (2012), suggesting a role for EMVs in biomineralization reactions during bioweathering of aluminosilicate rocks.
The use of cyanobacteria for the development of ISRU-based BLSS has been under investigation for several years (e.g., Brown et al., 2008; Olsson-Francis and Cockell, 2010; Rothschild, 2016; Verseux et al., 2016). Extremophilic desert strains of Chroococcidiopsis spp. that have been exposed in space and were tested for resistance to high doses of ionizing and ultraviolet radiation (see Billi et al., 2020), as well as nitrogen-fixing, rock-weathering cyanobacteria of the genus Anabaena (or related genera) were shown to be suitable as a source of nutrients for heterotrophic microorganisms (Verseux, 2018; Billi et al., 2021; Verseux et al., 2021). As a proof-of-concept, a desert strain of the cyanobacterium Chroococcidiopsis was grown in a Mars-relevant perchlorate concentration, i.e., 2.4 mM (Billi et al., 2021); perchlorate ions and the cyanobacterial lysate successfully utilized to feed Escherichia coli. Dual cyanobacterium—kombucha cultures are under consideration for processing locally available regolith to make rock-extracted nutrients available for plants of interest. The cyanobacteria-kombucha mat will form a protosoil, also making rock-extracted nutrients available for growing plants.
A challenge for growing KMC in space is the carbon and energy sources. The traditional substrate for KMC, Camellia sinensis or Chinese tea, is a limited resource for space travels, therefore, tea must be replaced by alternative components, considering a self-sustainable production based on green ecology (Kołodziejczyk, 2018). One such possibility is the use of photosynthetic microorganisms, for example, common Chlorella algae, which can grow in bioreactors onboard habitats or spaceships (Suchan, 2020). KMC cultures set on naturally basic Chlorella extract must be reduced to pH = 6. Bacterial nanocellulose produced in green solutions is white, delicate, and glossy (Figure 4A). Other types of algae tested in order to optimize KMC growth in space are extremophilic microorganisms such as endolythic Galdieria sulphuraria and Cyanidioschyzon merolae. These algae are easy to cultivate, and they do not create protective biofilms. Instead of sucrose, there are few options such as using starch or cellulose digested by bacteria and fungi within the BLSS loop. It is important to note that adding a new organism to the consortium takes time and sometimes it is not possible for KMC to accept a new partner and alternatively, yeasts will be modified with genetic engineering tools.
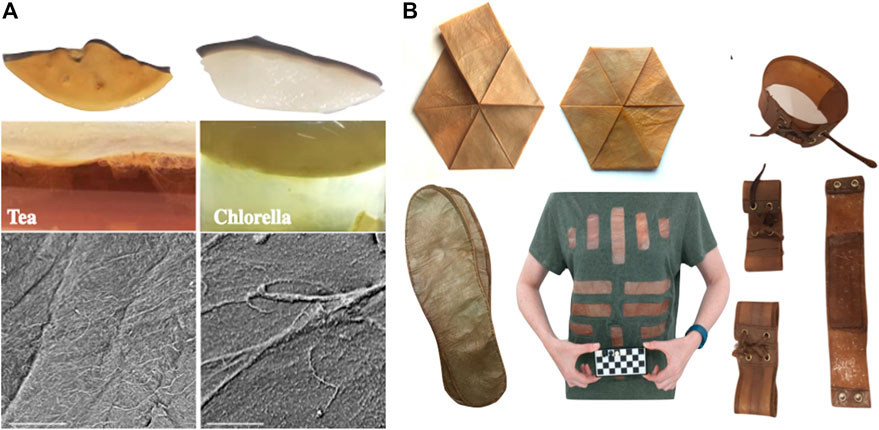
FIGURE 4. Bacterial nanocellulose can be used for clothes in space. (A) The traditional substrate for KMC, a tea, must be replaced by alternative components, e.g., photosynthetic algae, for biofabrication of cellulose. The comparison between two types of KMC cultures: (left) grown on tea, and (right) grown on algae. Hydrogel bacterial cellulose is similar in chemical composition but physical properties are slightly different. Nanocellulose grown on algae extract is much softer and more prone for damage. Scanning electron microscope images reveal the ultrastructure of nanocellulose filaments. Scale bar 50 microns (modified after Suchan, 2020). (B) KMC’s material absorbs odours. It can be easily cleaned and sterilised. It folds gently to very compact volumes. Folding does not implicate changes in structure of the material. Kombucha’s cellulose does not generate allergic responses in direct contact with human skin. It makes skin softer and visibly healthier (Kołodziejczyk, 2018; Kamiński et al., 2020).
Kombucha for Biomining
Biomining describes a process of dissolving ingredients and removing them out by biological agent, e.g., metals of economic interest from rock ores or mine waste (Rawlings and Silver, 1995; Johnson, 2014). When the metal of interest is directly dissolved, the biomining process is called “bioleaching”. The bioleaching of elements occurs directly by electron supply based on the pH difference between the environment and the microbial cytoplasm, which generates a natural proton motive force across the cellular membrane. Another type is indirect leaching by metabolic products of the microorganisms (organic acids, siderophores, enzymes, etc.) (Potysz et al., 2018). Biological technologies offer obvious advantages over the traditional mining procedures due to their low cost and eco-friendliness, and they do not require high amounts of energy. A biomining is also of great advantage since microbes can discard low-grade ores. Moreover, in some cases, bioleaching is significantly more efficient using bacteria when compared to abiotic technology (Auerbach et al., 2019). Microorganisms are increasingly used for metal extraction worldwide, primarily for copper and gold but for other metals as well (see Schippers et al., 2013).
The use of biomining for missions beyond Earth has been considered for over a decade (Dalton and Roberto, 2008; Cockell, 2010; Cockell et al., 2020). Although estimates of the effectiveness and efficiency of such processes on Mars or the Moon can only be tentative, a few assays based on basalts, anorthosite, or slightly more sophisticated simulants were performed for such purposes (e.g., Volger et al., 2020; Olsson-Francis and Cockell, 2010; Navarrete et al., 2012; Castelein et al., 2020), giving insights into the rates of metal release in the liquid phase, metal assimilation in the biomass, and bacterial growth. Besides, an experiment on the ISS showed that microgravity or Martian gravity did not impair biomining (Cockell et al., 2020). Kombucha’s bioleaching abilities could be used for the mining of metals for applications beyond BLSS. KMC has shown to foster the release of the rare earth elements (REEs) from fluorescent tubes (Hopfe et al., 2017) and, as described above, that of various metals from anorthosite. Their use on the Moon on Mars may, thus, be worth considering for leaching small amounts of iron, REEs, and other elements from regolith or waste (Zaets et al., 2011).
Bacterial Cellulose Is a Multipurpose Biodegradable Robust Nanomaterial for Extraterrestrial Bases
Since economic and environmental issues will be critical in the early beginning of extraterrestrial settlements, sustainable bio-based products will be desired. BC is an example of biofabricated multipurpose products, valuable for sustainable eco-friendly extraterrestrial economies.
Bacterial Cellulose Fibrils Are Synthesized by Acetic Acid Bacteria
BC is secreted out of cells as linear polysaccharide polymer, where D-glucose units are linked by β-1,4-glycosidic linkages, similar to plant-derived cellulose (Ross et al., 1991). Nonetheless, in contrast, BC generates structural hydrogel with interconnected ribbons of around 100 µm in length and 100 nm diameter composed of a three-dimensional nanofibrous network (Wang et al., 2020), which is free of both lignin and pectin. Such a peculiar supramolecular structure engineered by nature makes BC stable and robust. The crystallinity of BC is higher than cellulose produced by plants, and this results in higher thermal stability. Being one hundred times thinner than cellulose fibrils obtained from plants, BC possesses high tensile strength. The highly porous structure of the cellulose nanonetwork and controlled shape are attractive for the manufacture of new ultra-light and stable nanomaterials, and various possibilities of the BC-polymer modifications open up the unrestricted development of new cellulose-based nanocomposites.
Bacterial Cellulose has the Potential to Be Used in the Extraterrestrial Economy
The robust cellulose properties, such as high crystallinity, water holding capacity, thermo- and radiation resistance, mechanical properties, biocompatibility with the human body offer a wide range of applications in different fields, including optics, electronics, biomedical and food industry (Blanco Parte et al., 2020; Choi and Shin, 2020; Sriplai and Pinitsoontorn, 2021). The worldwide market for bacterial cellulose is valued at 324.5 million USD in 2020 and is expected to reach 785.1 million USD by the end of 2026 (Global microbial and bacterial cellulose market research report, 2020). Therefore, BC has a high sustainable value and a great potential to be used in the extraterrestrial economy for providing biodegradable and reusable goods and materials. BC’s exceptional bioaffinity will promote the development of BC-based biomedical products for outposts, such as tissue-engineered scaffolds, wound-dressing materials, dental implants, artificial blood vessels and nerves, surgical mesh, bone fillings, heart valve, meniscus, artificial cartilage, etc. (Swingler et al., 2021). When bacterial cellulose is modified with conductive compounds, such as metal nanoparticles or graphite, it naturally becomes conductive of electricity and, therefore, promising in developing materials for electrical applications (Andriani et al., 2020). KMC living cellulose-based materials can sense and respond to their environment. Synthetic biology methods provide tools to create hybrid cellulose materials with predictable novel or enhanced characteristics matching the special purposes of the developing settlements, which cannot be achieved by conventional tools. For example, engineered to secrete enzymes into bacterial cellulose, yeast strains can be taught to sense and respond to chemical and optical stimuli. This means that the modified KMC can be biosensors for many purposes (Mustafa and Andreescu, 2020; Gilbert et al., 2021). Moreover, another especially potential use will be intelligent packaging for foods with short shelf-life, as well as biosensors of microbial contamination and mycotoxins.
Bacterial Cellulose for Clothes in Space
Astronauts must take all clothes for the time of their mission because the supply of water on board is too limited to permit clothes being washed by hand, let alone by a washing machine. The price for each lifted kilogram into space ranges from $10 000 to $25 000, which means that clothes in space are an expensive issue regarding long-term missions (https://www.nasa.gov/vision/space/livinginspace/Astronaut_Laundry.html). There were many discussions about solving the clothing problem in space. One option was proposed to clean dirty clothes using physical methods such as radiation, UV light, or vacuum. Other options suggested using smell-free clothes, for example, Wakata’s clothes developed by Yoshiko Taya designed to be flame-resistant, anti-static, anti-bacterial, to absorb water, insulate the body, and dry quickly, not to mention comfortable and stylish. The last option was to reuse dirty clothing for nourishing plants and bacteria cultivation (Space.com, 2021). Interestingly, KMC-derived bacterial nanocellulose may be easily and ecologically processed to obtain material similar to animal leather (Kołodziejczyk, 2018; Kamiński et al., 2020). It has similar properties to Wakata’s clothes and it is fully biodegradable. The whole process from setting the KMC culture to obtaining material of the desired shape takes around 3 weeks. Transformation of a raw kombucha hydrogel cellulose into a dry and strong anti-flammable material takes another 3 days (Kołodziejczyk, 2018) (Figure 4B). In addition to indoor clothing, e.g., T-shirts shown in Figure 4, the potential use of kombucha nanocellulose as a component of spacesuits is currently being investigated in the Analog Astronaut Training Center (Poland) in collaboration with Jagiellonian University (Krakow, Poland). In particular, post-processed kombucha material reveals new features such as resistance to damage, friction or fire (Kamiński et al., 2020). In support of the fact of cellulose robustness under extreme conditions, Orlovska et al. (2021) provided the first data on the cellulose polymer resilience after exposure of live cellulose-based pellicle films to the simulated Martian environment on the ISS. The ATR-FTIR absorption spectra analysis showed the preservation of its characteristic vibrations. In addition, the characteristic hydroxyl bonding in cellulose structure due to preservation of an Iα crystalline phase may serve as the evidence of structural integrity of cellulose polymer in the space-exposed samples. Notably, after a long-term exposure experiment, the mechanical properties of the de novo synthesized cellulose were slightly changed in the BC-producers, especially, in unprotected KMCs, which exhibited reduced cellulose yield, compared to ground control KMC pellicles. After a long period of permanent culturing, the mechanical properties of cellulose produced by KMCs improved.
Kombucha Microbial Community Is a Hub for the Cellulose-Synthesizing Bacteria
Increasing attention is paid to the cellulose-producing organisms and their appropriate genetic modification as candidates for cellulose biofabrication in earthly and extraterrestrial conditions. For example, the reprogrammed bacterial strains that are capable to produce a high yield of BC in low-cost media with precise control of heterologous cellulose biosynthesis gene expression or metabolic pathway modulation have been designed (Florea et al., 2016; Teh et al., 2019; Ryngajłło et al., 2020; Singh et al., 2020). Our results show that cellulose-producing bacteria of the Komagataeibacter genus are tolerant to spaceflight and Mars-like stressors (Podolich et al., 2019). Nonetheless, the rate of tolerance may depend on the genotype of the species. Among the species of K. saccharivorans, K. hansenii, K. rhaeticus, K. oboediens, K. intermedius and K. europaeus detected in the IMBG-1-ecotype, the relative abundance of K. hansenii was substantially increased in the KMC exposed to Mars-like UV compared to the initial KMC, where K. saccharivorans was dominant. Similarly, K. oboediens, K. intermedius and K. europaeus were enriched in the exposed KMCs. We observed that the Mars-like stressors did not alter the topology or induced mutations in any of the bacterial cellulose synthesis (bcs) genes of K. oboediens, reisolated from the returned KMC (Góes-Neto et al., 2021). Nevertheless, in the revived K. oboediens, the cellulose synthase gene bscA was down-regulated, in spite of the full homology/topology of the bcsABCD to wild type gene cluster, supporting the observation of the lower cellulose output by revived bacteria compared to the ground reference (Orlovska et al., 2021). Since komagataeibacters show a significant potential of survival in extraterrestrial conditions, these bacteria have a chance to be safely delivered within a cellulose-based pellicle matrix to far destinations.
In addition to Komagataeibacter spp., KMC pellicle is a matrix for safe delivery of a miniature collection of microbial organisms, which could later be developed into a real microbial collection hosted by a local research unit. KMC members are co-existed on a principle of cooperation and competition to produce public goods with the endless biotechnological value and myriads of applications. For example, Gluconobacter oxydans could be a candidate for biofabrication of goods for application in pharmaceutical and food industries (vitamin C, a sweetener, co-enzyme Q10, biosensors, etc.) (Moghadami et al., 2019). In extraterrestrial bases, Saccharomyces cerevisiae is of great importance for various biotechnological applications, especially, in such vitally important industries as bread and biofuel production (Parapouli et al., 2020). Schizosaccharomyces pombe is a promising yeast species for the laccase production needed for degrading and detoxifying various synthetic compounds, which inevitably will be accumulated in crewed bases, or CoQ10 as an antioxidant food supplement (Nishida et al., 2019). Moreover, lactic acid bacteria could be an inexhaustible source of probiotics from the kombucha ecosystem (Zaets et al., 2016).
Kombucha and the Zero-Waste Production Philosophy
KMC culturing for the biofabrication of cellulose for industrial purposes lies in the context of eco-friendly, zero-waste production. Importantly, the kombucha-related industry is to operate without wastes and side products. For example, residues of cellulose pellicle in the manufacture of clothes can be used in formation of protosoil in BLSS gardens (Camargo et al., 2020) or as animal feed (Afsharmanesh and Sadaghi, 2014). A sour kombucha drink left could be transformed into vinegar and balms, as well as used in the production of disinfectants (Ryssel et al., 2009). Notably, acetic acid is a cheap and effective measure to eradicate biofilms (Halstead et al., 2015), which would be of great importance in fighting against biofilm-forming bacteria usually competitive in confined systems of crew habitations. Komagataeibacter cells, as a by-product of the BC production, may be reasonably used as a biologically active additive, containing valuable antioxidants and lipids for improving cognitive function (Fukami et al., 2009) and as a product preventing skin dryness (Tsuchiya et al., 2020).
Perspectives
Kombucha culture has evolved over significant periods of time in various artificial environments. An upgraded, rationally assembled, well-defined version could benefit crews, living in the confined space, in long-term missions. It is known as a health-promoting functional food and could provide a wide range of nutrients and other metabolites (including nutraceuticals for prophylaxis, either synbiotic or postbiotic) for crewmembers, animals, and plants. It is also a producer of cellulosic materials, which could find a wide range of applications in human communities beyond Earth, be they small habitats or outposts possessing their own economic systems. A significant advantage of KMC is the zero-waste production in systems ranging from small-scale, ISRU-based BLSS to large-scale cellulose biofabrication. Its organization as a microecosystem provides strong advantages over most microorganisms of biotech value: resistance to contamination, ease of cultivation, and high versatility. As kombucha is a complex microecosystem, it probably complicates operation and its control, especially in alien conditions. The improvement of an advanced toolbox of genetic metabarcoding and shotgun metagenomic technologies (to monitor accurately the microbial populations), would be highly valuable. On the other hand, alternative low-resource tools and novel biological sensing platforms for pathogen monitoring should be designed for easy use by crews. Relying on a combination of systems biology and Meta-Omics methodologies, it is suggested to compose and study different ecotypes of the kombucha microbiota (or related communities) to select an operational, minimal rationally assembled (upgraded) community with defined essential community members for different purposes (health promotion, biosensing, biomining, etc.) (Savar et al., 2021). Our results showed that the diversity within a KMC was not affected significantly by space exposure, suggesting that keystone tolerant species will be maintained and controlled. Minor metagenomes can be customized by including specific transcripts or sequence variants serving specific purposes. Synthetic metagenomics could be used to gather metabolic networks from various members into a single yeast species, then used as a chassis (Belda et al., 2021). Systems and synthetic biology could be used to enhance KMC’s biotechnological features. For example, metabolic engineering could lead to more efficient pathways of carbohydrate utilization from waste plant material produced on-site (rather than imported sugars) by some KMC members (e.g., yeasts).
Even though KMC is quite resistant to contaminating biota, some of the remaining challenges pertain to safety, most importantly with regards to the consumption of fermented products. Studies of the safety of a well-defined yeast-bacterial community, under normal and stressed conditions, as well as the improvement of an advanced toolbox of genetic metabarcoding and shotgun metagenomic technologies (to monitor accurately the microbial populations), would be highly valuable. On the other hand, alternative low-resource tools and novel biological sensing platforms for pathogen monitoring should be designed for easy use by crews. A set of criteria for the scientific substantiation of health claims on foods for consumption beyond Earth are being elaborated, but issues on fermented food, probiotics, synbiotics, postbiotics remain to be addressed (Vargas et al., 2021).
Significant research and development efforts are required to fulfil the potential of KMC beyond Earth. However, those can be provided progressively: from the simplest applications in orbital stations to industrial manufacturing in planetary settlements, incremental developments will reap incremental benefits. Humankind seems to be in the process of expanding its presence beyond Earth: after 2 decades of permanent presence in low Earth orbit, a permanent presence on the Moon is expected to start this decade, followed by crewed missions to Mars. In a more distant future we may, as many have claimed, become a multiplanetary species. KMC-based processes could develop alongside human activities in the Solar System. In any case, the idea of fermentation in space is gaining popularity among scientists from academia, industry, research institutes, and public interest groups, creating—it seems—the required momentum. The evolution of space-related regulations, which may be relaxed as new safety-related knowledge is obtained, could support this initiative.
In short, we argue that KMC applications beyond Earth should be investigated: biotechnological processes may result which could largely benefit human activities, from orbital stations to planetary settlements. On a less tangible side (but nonetheless worthy of consideration), we suggest that the acceptance of microorganisms—such as upgraded KMC—as partners, and of the principle of co-existence, would greatly benefit the creation of a healthy biosphere in alien worlds.
Author Contributions
All authors listed have made a substantial, direct, and intellectual contribution to the work and approved it for publication.
Conflict of Interest
AK was employed by Analog Astronaut Training Centre and RD was employed by HE Space Operations B.V.
The remaining authors declare that the research was conducted in the absence of any commercial or financial relationships that could be construed as a potential conflict of interest.
Publisher’s Note
All claims expressed in this article are solely those of the authors and do not necessarily represent those of their affiliated organizations, or those of the publisher, the editors, and the reviewers. Any product that may be evaluated in this article, or claim that may be made by its manufacturer, is not guaranteed or endorsed by the publisher.
Acknowledgments
The authors are thankful for the research support from the National Academy of Sciences of Ukraine Space Research Program (grant 49/2021). CV’s work is supported by funding from the Alexander von Humboldt Foundation.
Funding
The company Eat Easy (Kyiv, Ukraine), a manufacturer of kombucha, is appreciated for covering the publication fees of this manuscript.
References
Afsharmanesh, M., and Sadaghi, B. (2014). Effects of Dietary Alternatives (Probiotic, green tea Powder, and Kombucha tea) as Antimicrobial Growth Promoters on Growth, Ileal Nutrient Digestibility, Blood Parameters, and Immune Response of Broiler Chickens. Comp. Clin. Pathol. 23, 717–724. doi:10.1007/s00580-013-1676-x
Afshinnekoo, E., Scott, R. T., MacKay, M. J., Pariset, E., Cekanaviciute, E., Barker, R., et al. (2020). Fundamental Biological Features of Spaceflight: Advancing the Field to Enable Deep-Space Exploration. Cell 183, 1162–1184. doi:10.1016/j.cell.2020.10.050
Ahlawat, S., Asha, A., and Sharma, K. K. (2020). Gut-organ axis: a Microbial Outreach and Networking. Lett. Appl. Microbiol. 72, 636–668. doi:10.1111/lam.13333
Alexandraki, V., Tsakalidou, E., Papadimitriou, K., and Holzapfel, W. (2013). Status and Trends of the Conservation and Sustainable Use of Microorganism in Food Processes. Commission on Genetic Resources for Food and Agriculture. Background study paper (FAO) 65, 172.
Andriani, D., Apriyana, A. Y., and Karina, M. (2020). The Optimization of Bacterial Cellulose Production and its Applications: a Review. Cellulose 27, 6747–6766. doi:10.1007/s10570-020-03273-9
Annunziata, G., Arnone, A., Ciampaglia, R., Tenore, G. C., and Novellino, E. (2020). Fermentation of Foods and Beverages as a Tool for Increasing Availability of Bioactive Compounds. Focus on Short-Chain Fatty Acids. Foods 9, 999. doi:10.3390/foods9080999
Arıkan, M., Mitchell, A. L., Finn, R. D., and Gürel, F. (2020). Microbial Composition of Kombucha Determined Using Amplicon Sequencing and Shotgun Metagenomics. J. Food Sci. 85, 455–464. doi:10.1111/1750-3841.14992
Ashrafian, F., Shahriary, A., Behrouzi, A., Moradi, H. R., Keshavarz Azizi Raftar, S., Lari, A., et al. (2019). Akkermansia Muciniphila-Derived Extracellular Vesicles as a Mucosal Delivery Vector for Amelioration of Obesity in Mice. Front. Microbiol. 10, 2155. doi:10.3389/fmicb.2019.02155
Auerbach, R., Ratering, S., Bokelmann, K., Gellermann, C., Brämer, T., Baumann, R., et al. (2019). Bioleaching of Valuable and Hazardous Metals from Dry Discharged Incineration Slag. An Approach for Metal Recycling and Pollutant Elimination. J. Environ. Manage. 232, 428–437. doi:10.1016/j.jenvman.2018.11.028
Avila-Herrera, A., Thissen, J., Urbaniak, C., Be, N. A., Smith, D. J., Karouia, F., et al. (2020). Crewmember Microbiome May Influence Microbial Composition of ISS Habitable Surfaces. PLoS One 15, e0231838. doi:10.1371/journal.pone.0231838
Bajaj, J. S., Barbara, G., DuPont, H. L., Mearin, F., Gasbarrini, A., and Tack, J. (2018). New Concepts on Intestinal Microbiota and the Role of the Non-absorbable Antibiotics with Special Reference to Rifaximin in Digestive Diseases. Dig. Liver Dis. 50, 741–749. doi:10.1016/j.dld.2018.04.020
Barbanchik, G. F. (1954). Kombucha and its Medicinal Properties. Omsk: Omsk Regional Book Publishing House.
Barbosa, C. D., Trovatti Uetanabaro, A. P., Rodrigues Santos, W. C., Caetano, R. G., Albano, H., Kato, R., et al. (2021). Microbial-physicochemical Integrated Analysis of Kombucha Fermentation. LWT 148, 111788. doi:10.1016/j.lwt.2021.111788
Barcellos-Hoff, M. H., Blakely, E. A., Burma, S., Fornace, A. J., Gerson, S., Hlatky, L., et al. (2015). Concepts and Challenges in Cancer Risk Prediction for the Space Radiation Environment. Life Sci. Space Res. 6, 92–103. doi:10.1016/j.lssr.2015.07.006
Belda, I., Williams, T. C., de Celis, M., Paulsen, I. T., and Pretorius, I. S. (2021). Seeding the Idea of Encapsulating a Representative Synthetic Metagenome in a Single Yeast Cell. Nat. Commun. 12, 1599. doi:10.1038/s41467-021-21877-y
Billi, D., Gallego Fernandez, B., Fagliarone, C., Chiavarini, S., and Rothschild, L. J. (2020). Exploiting a Perchlorate-Tolerant Desert Cyanobacterium to Support Bacterial Growth for In Situ Resource Utilization on Mars. Int. J. Astrobiology 20, 29–35. doi:10.1017/S1473550420000300
Billi, D., Gallego Fernandez, B., Fagliarone, C., Chiavarini, S., and Rothschild, L. J. (2021). Exploiting a Perchlorate-Tolerant Desert Cyanobacterium to Support Bacterial Growth for In Situ Resource Utilization on Mars. Int. J. Astrobiology 20, 29–35. doi:10.1017/S1473550420000300
Blanco Parte, F. G., Santoso, S. P., Chou, C.-C., Verma, V., Wang, H.-T., Ismadji, S., et al. (2020). Current Progress on the Production, Modification, and Applications of Bacterial Cellulose. Crit. Rev. Biotechnol. 40, 397–414. doi:10.1080/07388551.2020.1713721
Brown, I., Sarkisova, S. A., Garrison, D. H., Thomas-Keprta1, K., Allen, C. C., Jones, J. A., Galindo, C., and McKay, D. S. (2008). “Bioweathering of Lunarand Martian Rocks by Cyanobacteria: a Resource for Moon and Mars Exploration,” in 39th Lunar and Planetary Science Conference, (Lunar and Planetary Science XXXIX). LPI Contribution No. 1391, League City, TX, March 10–14, 2008, 1673.
Bueno, F., Chouljenko, A., and Sathivel, S. (2021). Development of Coffee Kombucha Containing Lactobacillus Rhamnosus and Lactobacillus Casei: Gastrointestinal Simulations and DNA Microbial Analysis. Lwt 142, 110980. doi:10.1016/j.lwt.2021.110980
Camargo, M. S. A., Cercal, A. P., Silveira, V. F., Mancinelli, K. C. B., Gern, R. M. M., Garcia, M. C. F., et al. (2020). Evaluation of Wet Bacterial Cellulose Degradation in Different Environmental Conditions. Macromol. Symp. 394, 2000149. doi:10.1002/masy.202000149
Camere, S., and Karana, E. (2018). Fabricating Materials from Living Organisms: an Emerging Design Practice. J. Clean. Prod. 186, 570–584. doi:10.1016/j.jclepro.2018.03.081
Castelein, S. M., Aarts, T. F., Schleppi, J., Hendrikx, R., Böttger, A. J., Benz, D., et al. (2021). Iron Can Be Microbially Extracted from Lunar and Martian Regolith Simulants and 3D Printed into Tough Structural Materials. PLoS One 16 (4), e0249962. doi:10.1371/journal.pone.0249962
Chakravorty, S., Bhattacharya, S., Chatzinotas, A., Chakraborty, W., Bhattacharya, D., and Gachhui, R. (2016). Kombucha tea Fermentation: Microbial and Biochemical Dynamics. Int. J. Food Microbiol. 220, 63–72. doi:10.1016/j.ijfoodmicro.2015.12.015
Checinska, A. S., Urbaniak, C., Mohan, G. B. M., Stepanov, V. G., Tran, Q., Wood, J. M., et al. (2019). Characterization of the Total and Viable Bacterial and Fungal Communities Associated with the International Space Station Surfaces. Microbiome 7, 50. doi:10.1186/s40168-019-0666-x
Chen, T., Nomura, K., Wang, X., Sohrabi, R., Xu, J., Yao, L., et al. (2020). A Plant Genetic Network for Preventing Dysbiosis in the Phyllosphere. Nature 580, 653–657. doi:10.1038/s41586-020-2185-0
Cheng, Y.-W., Phelps, E., Ganapini, V., Khan, N., Ouyang, F., Xu, H., et al. (2019). Fecal Microbiota Transplantation for the Treatment of Recurrent and severeClostridium Difficileinfection in Solid Organ Transplant Recipients: A Multicenter Experience. Am. J. Transpl. 19, 501–511. doi:10.1016/j.ijfoodmicro.2015.12.01510.1111/ajt.15058
Choi, S. M., and Shin, E. J. (2020). The Nanofication and Functionalization of Bacterial Cellulose and its Applications. Nanomaterials 10, 406. doi:10.3390/nano10030406
Cockell, C. S. (2010). Geomicrobiology beyond Earth: Microbe-mineral Interactions in Space Exploration and Settlement. Trends Microbiol. 18, 308–314. doi:10.1016/j.tim.2010.03.005
Cockell, C. S., Santomartino, R., Finster, K., Waajen, A. C., Eades, L. J., Moeller, R., et al. (2020). Space Station Biomining experiment Demonstrates Rare Earth Element Extraction in Microgravity and Mars Gravity. Nat. Commun. 11, 5523. doi:10.1038/s41467-020-19276-w
Coton, M., Pawtowski, A., Taminiau, B., Burgaud, G., Deniel, F., Coulloumme-Labarthe, L., et al. (2017). Unraveling Microbial Ecology of Industrial-Scale Kombucha Fermentations by Metabarcoding and Culture-Based Methods. FEMS Microbiol. Ecol. 93 (5), fix048. doi:10.1093/femsec/fix048
Cryan, J. F., O'Riordan, K. J., Cowan, C. S. M., Sandhu, K. V., Bastiaanssen, T. F. S., Boehme, M., et al. (2019). The Microbiota-Gut-Brain axis. Physiol. Rev. 99, 1877–2013. PMID: 31460832. doi:10.1152/physrev.00018.2018
Cvetković, D., Ranitović, A., Savić, D., Joković, N., Vidaković, A., Pezo, L., et al. (2019). Survival of Wild Strains of Lactobacilli during Kombucha Fermentation and Their Contribution to Functional Characteristics of Beverage. Pol. J. Food Nutr. Sci. 69, 407–415. doi:10.31883/pjfns/112276
Dalton, B., and Roberto, F. (2008). Lunar Regolith Biomining: Workshop Report. in: NASA/CP-2008-214564. Available at: http://ntrs.nasa.gov/search.jsp?R=20090010050.
David, L. A., Maurice, C. F., Carmody, R. N., Gootenberg, D. B., Button, J. E., Wolfe, B. E., et al. (2014). Diet Rapidly and Reproducibly Alters the Human Gut Microbiome. Nature 505, 559–563. doi:10.1038/nature12820
de Carvalho, D. S., Trovatti Uetanabaro, A. P., Kato, R., Aburjaile, F., Jaiswal, A. K., Profeta, R., et al. (2021). Characterization Of Space-Exposed Kombucha Microbial Community Member K. Oboediens Shows No Obvious Changes in Its Genome After Reactivation. Front. Microbiol..
de Vera, J.-P., Alawi, M., Backhaus, T., Baqué, M., Billi, D., Böttger, U., et al. (2019). Limits of Life and the Habitability of Mars: The ESA Space Experiment BIOMEX on the ISS. Astrobiology 19, 145–157. doi:10.1089/ast.2018.1897
Dimidi, E., Cox, S. R., Rossi, M., and Whelan, K. (2019). Fermented Foods: Definitions and Characteristics, Impact on the Gut Microbiota and Effects on Gastrointestinal Health and Disease. Nutrients 11, 1806. doi:10.3390/nu11081806
Dourado, F., Gama, M., and Rodrigues, A. C. (2017). A Review on the Toxicology and Dietetic Role of Bacterial Cellulose. Toxicol. Rep. 4, 543–553. doi:10.1016/j.toxrep.2017.09.005
Dufresne, C., and Farnworth, E. (2000). Tea, Kombucha, and Health: a Review. Food Res. Int. 33, 409–421. doi:10.1016/S0963-9969(00)00067-3
Duri, L. G., El-Nakhel, C., Caporale, A. G., Ciriello, M., Graziani, G., Pannico, A., et al. (2020). Mars Regolith Simulant Ameliorated by Compost as In Situ Cultivation Substrate Improves Lettuce Growth and Nutritional Aspects. Plants 9, 628. doi:10.3390/plants9050628
Dutta, H., and Paul, S. K. (2019). Kombucha Drink: Production, Quality, and Safety Aspects. Prod. Manage. Beverages 1, 259–288. doi:10.1016/b978-0-12-815260-7.00008-0
Ehrenfreund, P., McKay, C., Rummel, J. D., Foing, B. H., Neal, C. R., Masson-Zwaan, T., et al. (2012). Toward a Global Space Exploration Program: A Stepping Stone Approach. Adv. Space Res. 49, 2–48. doi:10.1016/j.asr.2011.09.014
Ehrlich, H. L. (1989). “Role of Microbes to Condition Lunar Regolith for Plant Cultivation,” in Lunar Base Agriculture: Soils for Plant Growth. Editors D. W. Ming, and D. L. Henninger (Madison, Wisconsin: Amer Society of Agronomy), 139–144. doi:10.2134/1989.lunarbaseagriculture.c10
Fackrell, L. E., Schroeder, P. A., Thompson, A., Stockstill-Cahill, K., and Hibbitts, C. A. (2021). Development of Martian Regolith and Bedrock Simulants: Potential and Limitations of Martian Regolith as an In-Situ Resource. Icarus 354, 114055. doi:10.1016/j.icarus.2020.114055
FDA (1995). FDA Cautions Consumers on “Kombucha Mushroom Tea” (News Release). Washington, DC: US Department of Health and Human Services, Public Health Service, Food and Drug Administration.
Ferl, R. J., and Paul, A.-L. (2010). Lunar Plant Biology-A Review of the Apollo Era. Astrobiology 10, 261–274. doi:10.1089/ast.2009.0417
Fetissov, S. O. (2017). Role of the Gut Microbiota in Host Appetite Control: Bacterial Growth to Animal Feeding Behaviour. Nat. Rev. Endocrinol. 13, 11–25. doi:10.1038/nrendo.2016.150
Florea, M., Hagemann, H., Santosa, G., Abbott, J., Micklem, C. N., Spencer-Milnes, X., et al. (2016). Engineering Control of Bacterial Cellulose Production Using a Genetic Toolkit and a New Cellulose-Producing Strain. Proc. Natl. Acad. Sci. USA 113, E3431–E3440. doi:10.1073/pnas.1522985113
Foing, B. H., Kolodziejczyk, A. M., Heinicke, C., Lillo, A., Schlacht, I., et al. (2018). “Moon Village Worldwide Activities: Update for LPSC 2018 and Apollo 16/17 Tribute,” in 49th Lunar and Planetary Science Conference. LPI Contribution No. 2083, id.1988, The Woodlands, TX, March 19–23, 2018.
Foing, B. H., Rogers, H., Musilova, M., Weert, A., Mulder, S., Kerber, S., et al. (2021). “Life and Research at South Pole Moonbase: EuroMoonMars Campaigns Results 2019-2020,” in 52nd Lunar and Planetary Science Conference. LPI Contribution No. 2548, id. 25022021LPI.52.2502F, Virtual Conference, March 15–19, 2021.
Freitas, H. R., Isaac, A. R., Malcher-Lopes, R., Diaz, B. L., Trevenzoli, I. H., and De Melo Reis, R. A. (2018). Polyunsaturated Fatty Acids and Endocannabinoids in Health and Disease. Nutr. Neurosci. 21, 695–714. doi:10.1080/1028415X.2017.1347373
Fukami, H., Tachimoto, H., Kishi, M., Kage, T., Tanaka, Y., Koga, Y., et al. (2009). Continuous Ingestion of Acetic Acid Bacteria:Effect on Cognitive Function in Healthy Middle-Aged and Elderly Persons. Anti-aging Med. 6, 60–65. doi:10.3793/jaam.6.60
Garrett-Bakelman, F. E., Darshi, M., Green, S. J., Gur, R. C., Lin, L., Macias, B. R., et al. (2019). The NASA Twins Study: A Multidimensional Analysis of a Year-Long Human Spaceflight. Science 364, eaau8650. doi:10.1126/science.aau8650
Gibson, G. R., Hutkins, R., Sanders, M. E., Prescott, S. L., Reimer, R. A., Salminen, S. J., et al. (2017). Expert Consensus Document: The International Scientific Association for Probiotics and Prebiotics (ISAPP) Consensus Statement on the Definition and Scope of Prebiotics. Nat. Rev. Gastroenterol. Hepatol. 14, 491–502. doi:10.1038/nrgastro.2017.75
Gilbert, C., Tang, T.-C., Ott, W., Dorr, B. A., Shaw, W. M., Sun, G. L., et al. (2021). Living Materials with Programmable Functionalities Grown from Engineered Microbial Co-cultures. Nat. Mater. 20, 691–700. doi:10.1038/s41563-020-00857-5
Gilbert, J. A., Blaser, M. J., Caporaso, J. G., Jansson, J. K., Lynch, S. V., and Knight, R. (2018). Current Understanding of the Human Microbiome. Nat. Med. 24, 392–400. doi:10.1038/nm.4517
Global microbial and bacterial cellulose market research report (2020). SKU ID: QYR-15085121. Available at: https://www.360researchreports.com/global-microbial-and-bacterial-cellulose-market-15085121.
Góes‐Neto, A., Kukharenko, O., Orlovska, I., Podolich, O., Imchen, M., Kumavath, R., et al. (2021). Shotgun Metagenomic Analysis of Kombucha Mutualistic Community Exposed to Mars‐like Environment outside the International Space Station. Environ. Microbiol. 23, 3727–3742. doi:10.1111/1462-2920.15405
Gorgieva, S., and Trček, J. (2019). Bacterial Cellulose: Production, Modification and Perspectives in Biomedical Applications. Nanomaterials 9, 1352. doi:10.3390/nano9101352
Gorreja, F. (2019). Gene Expression Changes as Predictors of the Immune-Modulatory Effects of Probiotics: Towards a Better Understanding of Strain-Disease Specific Interactions. NFS J. 14-15, 1–5. doi:10.1016/j.nfs.2019.02.001
Guerrero, R., and Berlanga, M. (2009). The Evolution of Microbial Life: Paradigm Changesin Microbiology. Contrib. Sci. 5, 55–61. doi:10.2436/20.7010.01.59
Guidelines for the evaluation of probiotics in food (2002). Report of a Joint FAO/WHO Working Group on Drafting Guidelines for the Evaluation of Probiotics in Food. Quebec City, Canada: Food and Agriculture Organization of the United Nations. Available at: https://www.who.int/foodsafety/fs_management/en/probiotic_guidelines.pdf.
Guo, H., Chou, W.-C., Lai, Y., Liang, K., Tam, J. W., Brickey, W. J., et al. (2020). Multi-omics Analyses of Radiation Survivors Identify Radioprotective Microbes and Metabolites. Science 370, eaay9097370. doi:10.1126/science.aay9097
Haas-Neill, S., and Forsythe, P. (2020). A Budding Relationship: Bacterial Extracellular Vesicles in the Microbiota-Gut-Brain axis. Int. J. Mol. Sci. 21, 8899. doi:10.3390/ijms21238899
Haghmorad, D., Yazdanpanah, E., Sadighimoghaddam, B., Yousefi, B., Sahafi, P., Ghorbani, N., et al. (2020). Kombucha Ameliorates Experimental Autoimmune Encephalomyelitis through Activation of Treg and Th2 Cells. Acta Neurol. Belg. doi:10.1007/s13760-020-01475-3
Halstead, F. D., Rauf, M., Moiemen, N. S., Bamford, A., Wearn, C. M., Fraise, A. P., et al. (2015). The Antibacterial Activity of Acetic Acid against Biofilm-Producing Pathogens of Relevance to burns Patients. PLoS One 10 (9), e0136190. doi:10.1371/journal.pone.0136190
Hao, Z., Li, L., Fu, Y., and Liu, H. (2018). The Influence of Bioregenerative Life-Support System Dietary Structure and Lifestyle on the Gut Microbiota: a 105-day Ground-Based Space Simulation in Lunar Palace 1. Environ. Microbiol. 20, 3643–3656. doi:10.1111/1462-2920.14358
Harrison, K., and Curtin, C. (2021). Microbial Composition of SCOBY Starter Cultures Used by Commercial Kombucha Brewers in North America. Microorganisms 9 (5), 1060. doi:10.3390/microorganisms9051060
Heinicke, C., and Foing, B. (2021). Human Habitats: Prospects for Infrastructure Supporting Astronomy from the Moon. Phil. Trans. R. Soc. A. 379, 20190568. doi:10.1098/rsta.2019.0568
Helmke, P. A., and Corey, R. B. (1989). “Physical and Chemical Considerations for the Development of Lunar-Derived Soils,” in Lunar Base Agriculture: Soils for Plant Growth. Editors D. W. Ming, and D. L. Henninger (Madison, Wisconsin: Amer Society of Agronomy), 193–212. doi:10.2134/1989.lunarbaseagriculture.c15
Hill, C., Guarner, F., Reid, G., Gibson, G. R., Merenstein, D. J., Pot, B., et al. (2014). The International Scientific Association for Probiotics and Prebiotics Consensus Statement on the Scope and Appropriate Use of the Term Probiotic. Nat. Rev. Gastroenterol. Hepatol. 11, 506–514. doi:10.1038/nrgastro.2014.66
Holscher, H. D. (2017). Dietary Fiber and Prebiotics and the Gastrointestinal Microbiota. Gut Microbes 8, 172–184. doi:10.1080/19490976.2017.1290756
Hopfe, S., Flemming, K., Lehmann, F., Möckel, R., Kutschke, S., and Pollmann, K. (2017). Leaching of Rare Earth Elements from Fluorescent Powder Using the tea Fungus Kombucha. Waste Manage. 62, 211–221. doi:10.1016/j.wasman.2017.02.005
Jahromi, L. P., and Fuhrmann, G. (2021). Bacterial Extracellular Vesicles: Understanding Biology Promotes Applications as Nanopharmaceuticals. Adv. Drug Deliv. Rev. 173, 125–140. doi:10.1016/j.addr.2021.03.012
Jayabalan, R., Malbaša, R. V., Lončar, E. S., Vitas, J. S., and Sathishkumar, M. (2014). A Review on Kombucha Tea-Microbiology, Composition, Fermentation, Beneficial Effects, Toxicity, and Tea Fungus. Compr. Rev. Food Sci. Food Saf. 13, 538–550. doi:10.1111/1541-4337.12073
Jayabalan, R., Malbaša, R. V., and Sathishkumar, M. (2016). Kombucha. Reference Module in Food Science. Amsterdam, Netherlands: Elsevier. doi:10.1016/B978-0-08-100596-5.03032-8
Johnson, D. B. (2014). Biomining-biotechnologies for Extracting and Recovering Metals from Ores and Waste Materials. Curr. Opin. Biotechnol. 30, 24–31. doi:10.1016/j.copbio.2014.04.008
Jung, Y., Kim, I., Mannaa, M., Kim, J., Wang, S., Park, I., et al. (2019). Effect of Kombucha on Gut-Microbiota in Mouse Having Non-alcoholic Fatty Liver Disease. Food Sci. Biotechnol. 28, 261–267. doi:10.1007/s10068-018-0433-y
Kamiński, K., Jarosz, M., Grudzień, J., Pawlik, J., Zastawnik, F., Pandyra, P., et al. (2020). Hydrogel Bacterial Cellulose: a Path to Improved Materials for New Eco-Friendly Textiles. Cellulose 27, 5353–5365. doi:10.1007/s10570-020-03128-3
Kim, J., and Adhikari, K. (2020). Current Trends in Kombucha: Marketing Perspectives and the Need for Improved Sensory Research. Beverages 6, 15. doi:10.3390/beverages6010015
Kozyrovska, N., Foing, B., Demets, R., and de Vera, J.-P. (2018). “Are Postbiotics a Reasonable Alternative to Probiotics for Astronaut’s Health Support,” in Abstracts of the 18th Ukrainian conference on Space Research, 2018, Kiev, Ukraine, September 17–20, 2018, 65.
Kozyrovska, N., and Foing, B. (2010). “Kombucha Might Be Promising Probiotics for Consumption on the Moon,” in Abstract book COSPAR 38, 3. 38th COSPAR Scientific Assembly (Bibcode 2010cosp. 38.434K), Bremen, Germany, 18–25 July 2010.
Kozyrovska, N. O., Reva, O. M., Goginyan, V. B., and de Vera, J.-P. (2012). Kombucha Microbiome as a Probiotic: a View from the Perspective of post-genomics and Synthetic Ecology. Biopolym. Cel 28, 103–113. doi:10.7124/bc.000034
Kołodziejczyk, A. (2018). “Bacterial Cellulose for Clothes Production in Space Using Kombucha Microbial Consortium,” in 69th International Astronautical Congress (IAC), Bremen, Germany, 1-5 October 2018. IAC-18,A1,7,15,x45657, paper ID: 45657.
Kuhn, T., Koch, M., and Fuhrmann, G. (2020). Probiomimetics-Novel Lactobacillus ‐Mimicking Microparticles Show Anti‐Inflammatory and Barrier‐Protecting Effects in Gastrointestinal Models. Small 16 (40), 2003158. doi:10.1002/smll.202003158
Lavasani, P. S., Motevaseli, E., Sanikhani, N. S., and Modarressi, M. H. (2019). Komagataeibacter Xylinus as a Novel Probiotic Candidate with High Glucose Conversion Rate Properties. Heliyon 5, e01571. doi:10.1016/j.heliyon.2019.e01571
Lavefve, L., Cureau, N., Rodhouse, L., Marasini, D., Walker, L. M., Ashley, D., et al. (2021). Microbiota Profiles and Dynamics in Fermented Plant‐based Products and Preliminary Assessment of their in vitro Gut Microbiota Modulation. Food Front, 1–14. doi:10.1002/fft2.75
Lee, I., Barh, D., Podolich, O., Brenig, B., Tiwari, S., Azevedo, V., et al. (2021). Metagenome-assembled Genome Sequences Obtained from a Reactivated Kombucha Microbial Community Exposed to a Mars-like Environment outside the International Space Station. Microbiol. Resour. Announc 10 (36), e0054921. doi:10.1128/MRA.00549-21
Li, M., Zhou, H., Yang, C., Wu, Y., Zhou, X., Liu, H., et al. (2020). Bacterial Outer Membrane Vesicles as a Platform for Biomedical Applications: An Update. J. Controlled Release 323, 253–268. doi:10.1016/j.jconrel.2020.04.031
Lilly, D. M., and Stillwell, R. H. (1965). Probiotics: Growth-Promoting Factors Produced by Microorganisms. Science 147, 747–748. doi:10.1126/science.147.3659.747
Lloyd-Price, J., Abu-Ali, G., and Huttenhower, C. (2016). The Healthy Human Microbiome. Genome Med. 8, 51. doi:10.1186/s13073-016-0307-y
Lytvynenko, T., Zaetz, I., Voznyuk, T., Kovalchuk, M., Rogutskyy, I., Mytrokhyn, O., et al. (2006). A Rationally Assembled Microbial Community for Growing Tagetes Patula L. In a Lunar Greenhouse. Res. Microbiol. 157, 87–92. doi:10.1016/j.resmic.2005.07.009
Maggi, F., and Pallud, C. (2010). Martian Base Agriculture: The Effect of Low Gravity on Water Flow, Nutrient Cycles, and Microbial Biomass Dynamics. Adv. Space Res. 46, 1257–1265. doi:10.1016/j.asr.2010.07.012
Marsh, A. J., O'Sullivan, O., Hill, C., Ross, R. P., and Cotter, P. D. (2014). Sequence-based Analysis of the Bacterial and Fungal Compositions of Multiple Kombucha (tea Fungus) Samples. Food Microbiol. 38, 171–178. doi:10.1016/j.fm.2013.09.003
Martínez Leal, J., Valenzuela Suárez, L., Jayabalan, R., Huerta Oros, J., and Escalante-Aburto, A. (2018). A Review on Health Benefits of Kombucha Nutritional Compounds and Metabolites. CyTA - J. Food 16 (1), 390–399. doi:10.1080/19476337.2017.1410499
Martínez-Leal, J., Ponce-García, N., and Escalante-Aburto, A. (2020). Recent Evidence of the Beneficial Effects Associated with Glucuronic Acid Contained in Kombucha Beverages. Curr. Nutr. Rep. 9, 163–170. doi:10.1007/s13668-020-00312-6
Matei, B., Salzat, J., Diguta, C. F., Cornea, C. P., Luta, G., Utoıu, E. R., et al. (2018). Lactic Acid Bacteria Strains Isolated from Kombucha with Potential Probiotic Effect. Rom. Biotechnological Lett. 23, 13592–13598. doi:10.26327/RBL2017.133
Matlakowska, R., Skłodowska, A., and Nejbert, K. (2012). Bioweathering of Kupferschiefer Black Shale (Fore-Sudetic Monocline, SW Poland) by Indigenous Bacteria: Implication for Dissolution and Precipitation of Minerals in Deep Underground Mine. FEMS Microbiol. Ecol. 81 (1), 99–110. doi:10.1111/j.1574-6941.2012.01326.x
May, A., Narayanan, S., Alcock, J., Varsani, A., Maley, C., and Aktipis, A. (2019). Kombucha: a Novel Model System for Cooperation and Conflict in a Complex Multi-Species Microbial Ecosystem. PeerJ 7, e7565. doi:10.7717/peerj.7565
Metchnikoff, E. (1908). Quelques remarques sur le lait aigri. Paris: E. Rémy. HAL Id: pasteur-00724105.
Ming, D. W., and Henninger, D. L. (1994). Use of Lunar Regolith as a Substrate for Plant Growth. Adv. Space Res. 14, 435–443. doi:10.1016/0273-1177(94)90333-6
Mitchell, C. A. (1994). Bioregenerative Life-Support Systems. Am. J. Clin. Nutr. 60, 820S–824S. doi:10.1093/ajcn/60.5.820S
Moghadami, F., Fooladi, J., and Hosseini, R. (2019). Introducing a Thermotolerant Gluconobacter Japonicus Strain, Potentially Useful for Coenzyme Q10 Production. Folia Microbiol. 64, 471–479. doi:10.1007/s12223-018-0666-4
Molina-Tijeras, J. A., Gálvez, J., and Rodríguez-Cabezas, M. E. (2019). The Immunomodulatory Properties of Extracellular Vesicles Derived from Probiotics: A Novel Approach for the Management of Gastrointestinal Diseases. Nutrients 11, 1038. doi:10.3390/nu11051038
Morales, D. (2020). Biological Activities of Kombucha Beverages: The Need of Clinical Evidence. Trends Food Sci. Technol. 105, 323–333. doi:10.1016/j.tifs.2020.09.025
Mousavi, S. M., Hashemi, S. A., Zarei, M., Gholami, A., Lai, C. W., Chiang, W. H., et al. (2020). Recent Progress in Chemical Composition, Production, and Pharmaceutical Effects of Kombucha Beverage: A Complementary and Alternative Medicine. Evid Based. Complement. Altern. Med. 2020, 1–14. doi:10.1155/2020/4397543
Muraca, M., Putignani, L., Fierabracci, A., Teti, A., and Perilongo, G. (2015). Gut Microbiota-Derived Outer Membrane Vesicles: Under-recognized Major Players in Health and Disease. Discov. Med. 19, 343–348. PMID: 26105697.
Murphy, T. E., Walia, K., and Farber, J. M. (2018). Safety Aspects and Guidance for Consumers on the Safe Preparation, Handling and Storage of Kombucha — a Fermented tea Beverage. Food Prot. Trends 38, 329–337.
Musk, E. (2017). Making Humans a Multi-Planetary Species. New Space 5 (2), 46–61. doi:10.1089/space.2017.29009.emu
Mustafa, F., and Andreescu, S. (2020). Nanotechnology-based Approaches for Food Sensing and Packaging Applications. RSC Adv. 10, 19309–19336. doi:10.1039/D0RA01084G
Nagakubo, T., Nomura, N., and Toyofuku, M. (2020). Cracking Open Bacterial Membrane Vesicles. Front. Microbiol. 10, 3026. doi:10.3389/fmicb.2019.03026
National Aeronautics and Space Administration (2021). Astronauts' Dirty Laundry. Available at: https://www.nasa.gov/vision/space/livinginspace/Astronaut_Laundry.html.
Navarrete, J. U., Cappelle, I. J., Schnittker, K., and Borrok, D. M. (2012). Bioleaching of Ilmenite and basalt in the Presence of Iron-Oxidizing and Iron-Scavenging Bacteria. Int. J. Astrobiology 12, 123–134. doi:10.1017/S1473550412000493
Nguyen, N. K., Dong, N. T. N., Nguyen, H. T., and Le, P. H. (2015). Lactic Acid Bacteria: Promising Supplements for Enhancing the Biological Activities of Kombucha. Springerplus 4, 91. doi:10.1186/s40064-015-0872-3
Nishida, I., Yokomi, K., Hosono, K., Hayashi, K., Matsuo, Y., Kaino, T., et al. (2019). CoQ10 Production in Schizosaccharomyces pombe Is Increased by Reduction of Glucose Levels or Deletion of Pka1. Appl. Microbiol. Biotechnol. 103, 4899–4915. doi:10.1007/s00253-019-09843-7
Nsor-Atindana, J., Zhou, Y. X., Saqib, M. N., Chen, M., Douglas Goff, H., Ma, J., et al. (2020). Enhancing the Prebiotic Effect of Cellulose Biopolymer in the Gut by Physical Structuring via Particle Size Manipulation. Food Res. Int. 131, 108935. doi:10.1016/j.foodres.2019.108935
Olsson-Francis, K., and Cockell, C. S. (2010). Use of Cyanobacteria for In-Situ Resource Use in Space Applications. Planet. Space ScienceSpace Sci 58, 1279–1285. doi:10.1016/j.pss.2010.05.005
Orlovska, I., Podolich, O., Kukharenko, O., Zaets, I., Reva, O., Khirunenko, L., et al. (2021). Bacterial Cellulose Retains Robustness but its Synthesis Declines after Exposure to a Mars-like Environment Simulated outside the International Space Station. Astrobiology 21, 706–717. doi:10.1089/ast.2020.2332
Ott, E., Kawaguchi, Y., Kölbl, D., Rabbow, E., Rettberg, P., Mora, M., et al. (2020). Molecular Repertoire of Deinococcus Radiodurans after 1 Year of Exposure outside the International Space Station within the Tanpopo mission. Microbiome 8, 150. doi:10.1186/s40168-020-00927-5
Parapouli, M., Vasileiadis, A., Vasileiadi, A., Afendra, A.-S., and Hatziloukas, E. (2020). Saccharomyces cerevisiae and its Industrial Applications. AIMS Microbiol. 6, 1–32. doi:10.3934/microbiol.2020001
Parker, A., Fonseca, S., and Carding, S. R. (2020). Gut Microbes and Metabolites as Modulators of Blood-Brain Barrier Integrity and Brain Health. Gut Microbes 11, 135–157. doi:10.1080/19490976.2019.1638722
Pei, J., Jin, W., Abd El-Aty, A. M., Baranenko, D. A., Gou, X., Zhang, H., et al. (2020). Isolation, Purification, and Structural Identification of a New Bacteriocin Made by Lactobacillus Plantarum Found in Conventional Kombucha. Food Control 110, 106923. doi:10.1016/j.foodcont.2019.106923
Podolich, O., Kukharenko, O., Haidak, A., Zaets, I., Zaika, L., Storozhuk, O., et al. (2019). Multimicrobial Kombucha Culture Tolerates Mars-like Conditions Simulated on Low Earth Orbit. Astrobiology 19, 183–196. doi:10.1089/ast.2017.1746
Podolich, O., Kukharenko, O., Zaets, I., Orlovska, I., Palchykovska, L., Zaika, L., et al. (2020). Fitness of Outer Membrane Vesicles from Komagataeibacter Intermedius Is Altered under the Impact of Simulated Mars-like Stressors outside the International Space Station. Front. Microbiol. 11, 1268. doi:10.3389/fmicb.2020.01268
Podolich, O., Zaets, I., Kukharenko, O., Orlovska, I., Reva, O., Khirunenko, L., et al. (2017b). Kombucha Multimicrobial Community under Simulated Spaceflight and Martian Conditions. Astrobiology 17, 459–469. doi:10.1089/ast.2016.1480
Podolich, O., Zaets, I., Kukharenko, O., Orlovska, I., Reva, O., Khirunenko, L., et al. (2017a). The First Space-Related Study of a Kombucha Multimicrobial Cellulose-Forming Community: Preparatory Laboratory Experiments. Orig Life Evol. Biosph. 47, 169–185. doi:10.1007/s11084-016-9483-4
Potysz, A., van Hullebusch, E. D., and Kierczak, J. (2018). Perspectives Regarding the Use of Metallurgical Slags as Secondary Metal Resources - A Review of Bioleaching Approaches. J. Environ. Manage. 219, 138–152. doi:10.1016/j.jenvman.2018.04.083
Rawlings, D. E., and Silver, S. (1995). Mining with Microbes. Nat. Biotechnol. 13, 773–778. doi:10.1038/nbt0895-773
Reva, O. N., Zaets, I. E., Ovcharenko, L. P., Kukharenko, O. E., Shpylova, S. P., Podolich, O. V., et al. (2015). Metabarcoding of the Kombucha Microbial Community Grown in Different Microenvironments. AMB Expr. 5, 124. doi:10.1186/s13568-015-0124-5
Rodovalho, V. D. R., Luz, B. S. R. D., Rabah, H., do Carmo, F. L. R., Folador, E. L., Nicolas, A., et al. (2020). Extracellular Vesicles Produced by the Probiotic Propionibacterium Freudenreichii CIRM-BIA 129 Mitigate Inflammation by Modulating the NF-κB Pathway. Front. Microbiol. 11, 1544. doi:10.3389/fmicb.2020.01544
Ross, P., Mayer, R., and Benziman, M. (1991). Cellulose Biosynthesis and Function in Bacteria. Microbiol. Rev. 55, 35–58. doi:10.1128/mr.55.1.35-58.1991
Rothschild, L. J. (2016). Synthetic Biology Meets Bioprinting: Enabling Technologies for Humans on Mars (And Earth). Biochem. Soc. Trans. 44, 1158–1164. doi:10.1042/BST20160067
Ryngajłło, M., Jędrzejczak-Krzepkowska, M., Kubiak, K., Ludwicka, K., and Bielecki, S. (2020). Towards Control of Cellulose Biosynthesis by Komagataeibacter Using Systems-Level and Strain Engineering Strategies: Current Progress and Perspectives. Appl. Microbiol. Biotechnol. 104, 6565–6585. doi:10.1007/s00253-020-10671-3
Ryssel, H., Kloeters, O., Germann, G., Schäfer, T., Wiedemann, G., and Oehlbauer, M. (2009). The Antimicrobial Effect of Acetic Acid-An Alternative to Common Local Antiseptics. Burns 35, 695–700. doi:10.1016/j.burns.2008.11.009
Sakai, T., Moteki, Y., Takahashi, T., Shida, K., Kiwaki, M., Shimakawa, Y., et al. (2018). Probiotics into Outer Space: Feasibility Assessments of Encapsulated Freeze-Dried Probiotics during 1 Month's Storage on the International Space Station. Sci. Rep. 8, 10687. doi:10.1038/s41598-018-29094-2
Savary, O., Mounier, J., Thierry, A., Poirier, E., Jourdren, J., Maillard, M.-B., et al. (2021). Tailor-made Microbial Consortium for Kombucha Fermentation: Microbiota-Induced Biochemical Changes and Biofilm Formation. Food Res. Int. 147, 110549. doi:10.1016/j.foodres.2021.110549
Schippers, A., Hedrich, S., Vasters, J., Drobe, M., Sand, W., and Willscher, S. (2013). “Biomining: Metal Recovery from Ores with Microorganisms,” in Geobiotechnology I (Berlin, Germany: Springer), 1–47. doi:10.1007/10_2013_216
Silk, J., Crawford, I., Elvis, M., and Zarnecki, J. (2021). Astronomy from the Moon: the Next Decades. Phil. Trans. R. Soc. A. 379, 20190560. doi:10.1098/rsta.2019.0560
Silva, Y. P., Bernardi, A., and Frozza, R. L. (2020). The Role of Short-Chain Fatty Acids from Gut Microbiota in Gut-Brain Communication. Front. Endocrinol. 11, 25. doi:10.3389/fendo.2020.00025
Singh, A., Walker, K. T., Ledesma-Amaro, R., and Ellis, T. (2020). Engineering Bacterial Cellulose by Synthetic Biology. Int. J. Mol. Sci. 21, 9185. doi:10.3390/ijms21239185
Singh, N. K., Wood, J. M., Karouia, F., and Venkateswaran, K. (2018). Succession and Persistence of Microbial Communities and Antimicrobial Resistance Genes Associated with International Space Station Environmental Surfaces. Microbiome 6, 204. doi:10.1186/s40168-018-0585-2
Slenzka, K., and Kempf, J. (2010). “Bio-ISRU Concepts Using Microorganisms to Release O2 and H2 on Moon and Mars,” in 38th COSPAR Scientific Assembly (Bremen), Bremen, Germany, 18–25 July 2010.
Space.com (2021). Japanese Space Underwear Keeps Stink Out. Available at: https://www.space.com/7077-japanese-space-underwear-stink.html.
Sreeramulu, G., Zhu, Y., and Knol, W. (2000). Kombucha Fermentation and its Antimicrobial Activity. J. Agric. Food Chem. 48, 2589–2594. doi:10.1021/jf991333m
Sriplai, N., and Pinitsoontorn, S. (2021). Bacterial Cellulose-Based Magnetic Nanocomposites: A Review. Carbohydr. Polym. 254, 117228. doi:10.1016/j.carbpol.2020.117228
Suchan, M. (2020). “Optimization of Methods of Obtaining Bacterial Cellulose-Based Fabrics - Research on the Processes of Wetting and Swelling with Water,”. Licenciate thesis.
Swingler, S., Gupta, A., Gibson, H., Kowalczuk, M., Heaselgrave, W., and Radecka, I. (2021). Recent Advances and Applications of Bacterial Cellulose in Biomedicine. Polymers 13, 412. doi:10.3390/polym13030412
Teh, M. Y., Ooi, K. H., Danny Teo, S. X., Bin Mansoor, M. E., Shaun Lim, W. Z., and Tan, M. H. (2019). An Expanded Synthetic Biology Toolkit for Gene Expression Control in Acetobacteraceae. ACS Synth. Biol. 8, 708–723. doi:10.1021/acssynbio.8b00168
Thirsk, R. B. (2020). Health Care for Deep Space Explorers. Ann. ICRP 49, 182–184. doi:10.1177/0146645320935288
Tran, T., Grandvalet, C., Verdier, F., Martin, A., Alexandre, H., and Tourdot-Maréchal, R. (2020). Microbial Dynamics between Yeasts and Acetic Acid Bacteria in Kombucha: Impacts on the Chemical Composition of the Beverage. Foods 9, 963. doi:10.3390/foods9070963
Tsuchiya, Y., Ban, M., Kishi, M., Ono, T., and Masaki, H. (2020). Safety and Efficacy of Oral Intake of Ceramide-Containing Acetic Acid Bacteria for Improving the Stratum Corneum Hydration: A Randomized, Double-Blind, Placebo-Controlled Study over 12 Weeks. J. Oleo Sci. 69, 1497–1508. doi:10.5650/jos.ess20115
Tulkens, J., Vergauwen, G., Van Deun, J., Geeurickx, E., Dhondt, B., Lippens, L., et al. (2018). Increased Levels of Systemic LPS-Positive Bacterial Extracellular Vesicles in Patients with Intestinal Barrier Dysfunction. Gut 69, 191–193. doi:10.1136/gutjnl-2018-317726
Turroni, S., Magnani, M., Kc, P., Lesnik, P., Vidal, H., and Heer, M. (2020). Gut Microbiome and Space Travelers' Health: State of the Art and Possible Pro/Prebiotic Strategies for Long-Term Space Missions. Front. Physiol. 11, 553929. doi:10.3389/fphys.2020.553929
Uțoiu, E., Matei, F., Toma, A., Diguță, C., Ștefan, L., Mănoiu, S., et al. (2018). Bee Collected Pollen with Enhanced Health Benefits, Produced by Fermentation with a Kombucha Consortium. Nutrients 10, 1365. doi:10.3390/nu10101365
Vargas, B. K., Fabricio, M. F., and Záchia Ayub, M. A. (2021). Health Effects and Probiotic and Prebiotic Potential of Kombucha: A Bibliometric and Systematic Review. Food Biosci., 101332. doi:10.1016/j.fbio.2021.101332
Vernice, N. A., Meydan, C., Afshinnekoo, E., and Mason, C. E. (2020). Long-term Spaceflight and the Cardiovascular System. Precision Clin. Med. 3, 284–291. doi:10.1093/pcmedi/pbaa022
Verseux, C., Baqué, M., Lehto, K., de Vera, J.-P. P., Rothschild, L. J., and Billi, D. (2016). Sustainable Life Support on Mars - the Potential Roles of Cyanobacteria. Int. J. Astrobiology 15, 65–92. doi:10.1017/S147355041500021X
Verseux, C., Heinicke, C., Ramalho, T. P., Determann, J., Duckhorn, M., Smagin, M., et al. (2021). A Low-Pressure, N2/CO2 Atmosphere Is Suitable for Cyanobacterium-Based Life-Support Systems on Mars. Front. Microbiol. 12, 67. doi:10.3389/fmicb.2021.611798
Verseux, C. (2018). “Resistance of Cyanobacteria to Space and Mars Environments, in the Frame of the EXPOSE-R2 Space mission and beyond,” (Rome, Italy: University of Rome “Tor Vergata”). Ph.D thesis.
Vigentini, I., Fabrizio, V., Dellacà, F., Rossi, S., Azario, I., Mondin, C., et al. (2019). Set-up of Bacterial Cellulose Production from the Genus Komagataeibacter and its Use in a Gluten-free Bakery Product as a Case Study. Front. Microbiol. 10, 1953. doi:10.3389/fmicb.2019.01953
Villarreal-Soto, S. A., Beaufort, S., Bouajila, J., Souchard, J.-P., and Taillandier, P. (2018). Understanding Kombucha tea Fermentation: A Review. J. Food Sci. 83, 580–588. doi:10.1111/1750-3841.14068
Villarreal-Soto, S. A., Bouajila, J., Pace, M., Leech, J., Cotter, P. D., Souchard, J.-P., et al. (2020). Metabolome-microbiome Signatures in the Fermented Beverage, Kombucha. Int. J. Food Microbiol. 333, 108778. doi:10.1016/j.ijfoodmicro.2020.108778
Volger, R., Pettersson, G. M., Brouns, S. J. J., Rothschild, L. J., Cowley, A., and Lehner, B. A. E. (2020). Mining Moon & mars with Microbes: Biological Approaches to Extract Iron from Lunar and Martian Regolith. Planet. Space Sci. 184, 104850. doi:10.1016/j.pss.2020.104850
Voorhies, A. A., Mark Ott, C., Mehta, S., Pierson, D. L., Crucian, B. E., Feiveson, A., et al. (2019). Study of the Impact of Long-Duration Space Missions at the International Space Station on the Astronaut Microbiome. Sci. Rep. 9, 9911. doi:10.1038/s41598-019-46303-8
Wamelink, G. W. W., Frissel, J. Y., Krijnen, W. H. J., Verwoert, M. R., and Goedhart, P. W. (2014). Can Plants Grow on Mars and the Moon: A Growth experiment on Mars and Moon Soil Simulants. PLoS ONE 9, e103138. doi:10.1371/journal.pone.0103138
Wang, X., Wang, Q., and Xu, C. (2020). Nanocellulose-Based Inks for 3D Bioprinting: Key Aspects in Research Development and Challenging Perspectives in Applications-A Mini Review. Bioengineering 7, 40. doi:10.3390/bioengineering7020040
Williams, W. S., and Cannon, R. E. (1989). Alternative Environmental Roles for Cellulose Produced by Acetobacter Xylinum. Appl. Environ. Microbiol. 55, 2448–2452. PMCID: PMC203103. PMID: 16348023. doi:10.1128/aem.55.10.2448-2452.1989
Xia, T., Zhang, B., Duan, W., Li, Y., Zhang, J., Song, J., et al. (2019). Hepatoprotective Efficacy of Shanxi Aged Vinegar Extract against Oxidative Damage In Vitro and In Vivo. J. Funct. Foods 60, 103448. doi:10.1016/j.jff.2019.103448
Zaets, I., Burlak, O., Rogutskyy, I., Vasilenko, A., Mytrokhyn, O., Lukashov, D., et al. (2011). Bioaugmentation in Growing Plants for Lunar Bases. Adv. Space Res. 47, 1071–1078. doi:10.1016/j.asr.2010.11.014
Zaets, I., Podolich, O., Kukharenko, O., Reshetnyak, G., Shpylova, S., Sosnin, M., et al. (2014). Bacterial Cellulose May Provide the Microbial-Life Biosignature in the Rock Records. Adv. Space Res. 53, 828–835. doi:10.1016/j.asr.2014.01.002
Zaets, I. Y., Podolich, O. V., Reva, O. N., and Kozyrovska, N. O. (2016). DNA Metabarcoding of Microbial Communities for Healthcare. Biopolym. Cel 32, 3–8. doi:10.7124/bc.000906
Zea, L., Larsen, M., Estante, F., Qvortrup, K., Moeller, R., Dias de Oliveira, S., et al. (2017). Phenotypic Changes Exhibited by E. coli Cultured in Space. Front. Microbiol. 8, 1598. doi:10.3389/fmicb.2017.01598
Keywords: kombucha, space exploration, postbiotic, extraterrestrial outposts, microbial technologies, bioregenerative life support system, in situ resource utilization, cellulose biofabrication
Citation: Kozyrovska N, Reva O, Podolich O, Kukharenko O, Orlovska I, Terzova V, Zubova G, Trovatti Uetanabaro AP, Góes-Neto A, Azevedo V, Barh D, Verseux C, Billi D, Kołodziejczyk AM, Foing B, Demets R and Vera J-Pd (2021) To Other Planets With Upgraded Millennial Kombucha in Rhythms of Sustainability and Health Support. Front. Astron. Space Sci. 8:701158. doi: 10.3389/fspas.2021.701158
Received: 27 April 2021; Accepted: 28 September 2021;
Published: 12 October 2021.
Edited by:
Andreas Riedo, University of Bern, SwitzerlandReviewed by:
Jean-Marc Salotti, Inria Bordeaux - Sud-Ouest Research Centre, FranceRicardo Amils, Autonomous University of Madrid, Spain
Copyright © 2021 Kozyrovska, Reva, Podolich, Kukharenko, Orlovska, Terzova, Zubova, Trovatti Uetanabaro, Góes-Neto, Azevedo, Barh, Verseux, Billi, Kołodziejczyk, Foing, Demets and Vera. This is an open-access article distributed under the terms of the Creative Commons Attribution License (CC BY). The use, distribution or reproduction in other forums is permitted, provided the original author(s) and the copyright owner(s) are credited and that the original publication in this journal is cited, in accordance with accepted academic practice. No use, distribution or reproduction is permitted which does not comply with these terms.
*Correspondence: Natalia Kozyrovska, a296eXJuYUB1a3IubmV0; Olga Podolich, cG9kb2xvbGdhQHVrci5uZXQ=