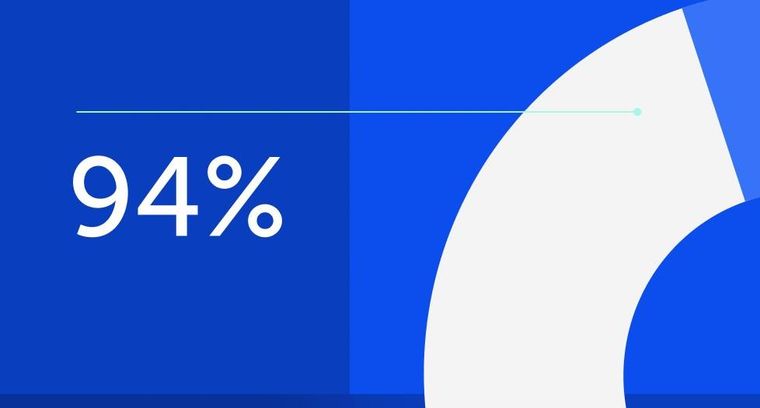
94% of researchers rate our articles as excellent or good
Learn more about the work of our research integrity team to safeguard the quality of each article we publish.
Find out more
REVIEW article
Front. Astron. Space Sci., 25 January 2021
Sec. Nuclear Physics
Volume 7 - 2020 | https://doi.org/10.3389/fspas.2020.609460
This article is part of the Research TopicNuclear reactions of astrophysical interestView all 14 articles
On August 17, 2017, less than two years after the direct detection of gravitational radiation from the merger of two
Although the birth of “multimessenger” astronomy dates back to the detection of the first solar neutrinos in the 1960s and was rejuvenated by the report of MeV neutrinos from SN 1987A in the Large Magellanic Cloud, the detection of gravitational radiation from the binary neutron star merger on August 17, 2017 (GW170817A), marks the transition to maturity of this approach to observational astrophysics, as it is expected to open an effective window into the study of astrophysical sources which is not limited to exceptionally close (the Sun) or rare (Galactic supernova) events. GW170817 is a textbook case for gravitational physics, because, with its accompanying short gamma-ray burst (GRB) and afterglow and its thermal aftermath “kilonova”, it has epitomized the different epiphanies of the coalescence of a binary system of neutron stars and finally allowed us to unify them.
Owing its name to a typical peak luminosity of
The outline of the review is as follows: Section 2 sets the context of binary systems of neutrons stars and describes the predicted outcomes of their coalescences; Section 3 presents the case of GW170817, the only so far confirmed example of double neutron star merger and the multiwavelength features of its electromagnetic counterpart (short GRB and kilonova); Section 4 focusses on the kilonova, elaborates on its observed optical and near-infrared light curves and spectra, draws the link with nucleosynthesis of heavy elements, and outlines the theoretical framework that is necessary to describe the kilonova properties and implications; Section 5 summarizes the results and provides an outlook of this line of research in the near future.
Neutron stars are the endpoints of massive stars evolution and therefore ubiquitous in the Universe: on average, they represent about 0.1% of the total stellar content of a Galaxy. Since massive stars are mostly in binary systems (Sana et al., 2012), neutron star binaries should form readily, if the supernova explosion of either progenitor massive star does not disrupt the system (Renzo et al., 2019). Alternatively, binary neutron star systems can form dynamically in dense environments like stellar clusters (see Ye et al., 2020 and references therein). Binary systems composed by a neutron star and a black hole are also viable, but rare (Pfahl et al., 2005), which may account for the fact that none has so far been detected in our Galaxy.
The prototype binary neutron star system in our Galaxy is PSR B1913 + 16, where one member was detected as a pulsar in a radio survey carried out at the Arecibo Observatory (Hulse and Taylor, 1974), and the presence of its companion was inferred from the periodic changes in the observed pulsation period of 59 ms (Hulse and Taylor, 1975). Among various tests of strong general relativity enabled by the radio monitoring of this binary system, which earned the Nobel Prize for Physics to the discoverers in 1993, was the measurement of the shrinking of the binary system orbit, signaled by the secular decrease of the 7.75 h orbital period, that could be entirely attributed to energy loss via gravitational radiation (Taylor and Weisberg, 1982; Weisberg and Huang, 2016 and references therein).
With an orbital decay rate of
The merger of a binary neutron star system has four predicted outcomes: (1) a gravitational wave signal that is mildly isotropic, with a stronger intensity in the polar direction than in the equatorial plane; (2) a relativistic outflow, which is highly anisotropic and can produce an observable high energy transient; (3) a thermal, radioactive source emitting most of its energy at ultraviolet, optical, and near-infrared wavelengths; and (4) a burst of MeV neutrinos (Eichler et al., 1989; Rosswog and Liebendörfer, 2003) following the formation of the central remnant and possibly of high-energy (>GeV) neutrinos from hadronic interactions within the relativistic jet (Fang and Metzger, 2017; Kimura et al., 2018). While neutrinos are extremely elusive and detectable only from very small distances with present instrumentation (see Section 5), the first three observables have been now all detected, as detailed in the next three subsections.
Coalescing binary systems of degenerate stars and stellar mass black holes are optimal candidates for the generation of gravitational waves detectable from ground-based interferometers as the strong gravity conditions lead to huge velocities and energy losses (Shapiro and Teukolsky, 1983), and the frequency of the emitted gravitational waves reaches several kHz, where the sensitivity of the advanced LIGO, Virgo, and KAGRA interferometers is designed to be maximal (Abbott et al., 2018).
The time behavior of binary systems of compact stars consists of three phases: a first inspiral phase in a close orbit that shrinks as gravitational radiation of frequency proportional to the orbital frequency is emitted, a merger phase where a remnant compact body is produced as a result of the coalescence of the two stars, and a postmerger, or ringdown, phase where the remnant still emits gravitational radiation while settling to its new stable configuration. During the inspiral, the amplitude of the sinusoidal gravitational signal rapidly increases as the distance between the two bodies decreases and the frequency increases (chirp), while in the ringdown phase the signal is an exponentially damped sinusoid. This final phase may encode critical information on the equation of state of the newly formed remnant (a black hole or, in the case of light neutron stars, a massive neutron star or metastable supramassive neutron star). The mathematical tool that is used to describe this evolution is the waveform model that aims at reproducing the dynamics of the system through the application of post-Newtonian corrections of increasing order and at providing the essential parameters that can then be compared with the interferometric observations (Blanchet, 2014; Nakano et al., 2019).
Since the amplitude of gravitational waves depends on the masses of the binary member stars, the signal will be louder and thus detectable from larger distances, for binary systems that involve black holes than those with neutron stars. The current horizon for binary neutron star merger detection with LIGO is
GRBs, flashes of radiation of 100–1,000 keV that outshine the entire Universe in this band, have durations between a fraction of a second and hundreds or even thousands of seconds. However, the duration distribution is bimodal, with a peak around 0.2 s (short or subsecond GRBs) and one around 20 s (long GRBs; Kouveliotou et al., 1993). This bimodality is reflected in the spectral hardness, which is on average larger in short GRBs, and in a physical difference between the two groups. While most long GRBs are associated with core-collapse supernovae (Galama et al., 1998; Woosley and Bloom, 2006; Levan et al., 2016), subsecond GRBs are produced by the merger of two neutron stars or a neutron star and a black hole, as long predicted based on circumstantial evidence (Blinnikov et al., 1984; Eichler et al., 1989; Fong et al., 2010; Berger et al., 2013; Tanvir et al., 2013) and then proven by the detection of GW170817 and of its high energy counterpart GRB170817A (Section 3). The observed relative ratio of long vs. short GRBs depends on the detector sensitivity and effective energy band (e.g., Burns et al., 2016). However, the duration overlap of the two populations is very large, so that the minimum of the distribution has to be regarded as a rather vaguely defined value (Bromberg et al., 2013).
About 140 short GRBs were localized so far to a precision that is better than 10 arc-minutes1; of these,
According to the classical fireball model, both prompt event and multiwavelength afterglow of short GRBs are produced in a highly relativistic jet directed at a small angle with respect to the line of sight, whose aperture can be derived from the achromatic steepening (or “jet break”) of the observed afterglow light curve (Nakar, 2007). In principle, this could be used to reconstruct the collimation-corrected rate of short GRBs, to be compared with predictions of binary neutron star merger rates. However, these estimates proved to be very uncertain, owing to the difficulty of measuring accurately the jet breaks in short GRB afterglows (Wanajo et al., 2002; Fong et al., 2015; Jin et al., 2018; Lamb et al., 2019; Pandey et al., 2019).
Elements heavier than iron cannot form via stellar nucleosynthesis, as no enough neutrons are available for the formation of nuclei and temperatures are not sufficiently high to overcome the repulsive Coulomb barrier that prevents acquisition of further baryons into nuclei (Burbidge et al., 1957). Supernovae (especially the thermonuclear ones) produce large amounts of iron via decay (through 56Co.) of radioactive 56Ni synthesized in the explosion. Heavier nuclei form via four neutron capture processes (Thielemann et al., 2011), the dominant ones being slow and rapid neutron capture, in brief s- and r-process, respectively, where “slow” and “rapid” refer to the timescale of neutron accretion into the nucleus with respect to that of the competing process of
Both s-process and r-process naturally occur in environments that are adequately supplied with large neutron fluxes. For the s-process, these are eminently asymptotic giant branch stars, where neutron captures are driven by the 13C (α, n)16O and 22Ne (α, n)25Mg reactions (Busso et al., 1999). The r-process requires much higher energy and neutron densities, which are only realized in most physically extreme environments. While it can be excluded that big-bang nucleosynthesis can accommodate heavy elements formation in any significant amount (Rauscher et al., 1994), there is currently no consensus on the relative amounts of nucleosynthetic yields in the prime r-process candidate sites: core-collapse supernovae and mergers of binary systems composed by neutron stars or a neutron star and a black hole.
Core-collapse supernovae have been proposed starting many decades ago as sites of r-process nucleosynthesis through various mechanisms and in different parts of the explosion, including dynamical ejecta of prompt explosions of O-Ne-Mg cores (Hillebrandt et al., 1976; Wheeler et al., 1998; Wanajo et al., 2002); C + O layer of O-Ne-Mg-core supernovae (Ning et al., 2007); He-shell exposed to intense neutrino flux (Epstein et al., 1988; Banerjee et al., 2011); re-ejection of fallback material (Fryer et al., 2006); neutrino-driven wind from protoneutron stars (Takahashi et al., 1994; Woosley et al., 1994); and magnetohydrodynamic jets of rare core-collapse SNe (Nishimura et al., 2006; Winteler et al., 2012). Similarly old is the first proposal that the tidal disruption of neutron stars by black holes in close binaries (Lattimer and Schramm, 1974, 1976; Symbalisty and Schramm, 1982; Davies et al., 1994) and coalescences of binary neutron star systems (Eichler et al., 1989) could be at the origin of r-process nucleosynthesis. This should manifest as a thermal optical-infrared source of radioactive nature of much lower luminosity (a factor of 1,000) and shorter duration (rise time of a few days) than supernova (Li & Paczyński, 1998).
The models for r-process elements production in core-collapse supernova all have problems inherent in their physics (mostly related to energy budget and neutron flux density). On the other hand, the binary compact star merger origin may fail to explain observed r-process element abundances in very low metallicities stars, i.e., at very early cosmological epochs, owing to the nonnegligible binary evolution times (see Cowan et al., 2020 for an accurate review of all arguments in favor and against either channel). While the event of August 17, 2017 (Section 3), has now provided incontrovertible evidence that binary neutron star mergers host r-process nucleosynthesis, the role of core-collapse supernovae cannot be dismissed although their relative contribution with respect to the binary compact star channel must be assessed (Ramirez-Ruiz et al., 2015; Ji et al., 2016; Shibagaki et al., 2016; Côté et al., 2019; Safarzadeh et al., 2019; Simonetti et al., 2019). It cannot be excluded that both “weak” and “strong” r-process nucleosyntheses take place, with the former occurring mainly in supernova and possibly failing to produce atoms up to the third peak of r-process elemental abundance distribution (Cowan et al., 2020). The hint that heavy elements may be produced in low-rate events with high yields (Sneden et al., 2008; Wallner et al., 2015; Macias and Ramirez-Ruiz, 2019) points to binary compact star mergers or very energetic (i.e., expansion velocities larger than 20,000 km s−1) core-collapse supernovae as progenitors, rather than regular core-collapse supernovae. Along these lines, it has been proposed that accretion disks of collapsars (the powerful core-collapse supernovae that accompany long GRBs, Woosley and Bloom, 2006) produce neutron-rich outflows that synthesize heavy r-process nuclei (Nakamura et al., 2013; Kajino et al., 2014; Nakamura et al., 2015). Siegel et al. (2019) calculated that collapsars may supply more than 80% of the r-process content and computed synthetic spectra for models of r-process-enriched supernovae corresponding to an MHD supernova and a collapsar disk outflow scenario.
Neutrons are tightly packed together in neutrons stars, but during coalescence of a binary neutron star system the tidal forces disrupt them and the released material forms promptly a disk-like rotating structure (dynamical ejecta, Rosswog et al., 1999; Shibata and Hotokezaka, 2019) where the neutron density rapidly drops to optimal values for r-process occurrence (
On August 17, 2017, the LIGO and Virgo interferometers detected for the first time a gravitational signal that corresponds to the final inspiral and coalescence of a binary neutron star system (Abbott et al., 2017a). The sky uncertainty area associated with the event was 28 square degrees, in principle too large for a uniform search for an electromagnetic counterpart with ground-based and orbiting telescopes. However, its small distance (
Based on the detection of electromagnetic radiation, Bauswein et al. (2017) have argued that the merger remnant may not be a black hole or at least the postmerger collapse to a black hole may be delayed. Since the postmerger phase “ring-down” signal of GW170817 was not detected (Abbott et al., 2017e), this hypothesis cannot be tested directly with gravitational data. Bauswein et al. (2017) also derived lower limits on the radii of the neutron stars.
Notably, while the gravitational data made it possible to set an upper limit on the tidal-deformability parameter of the binary neutron stars (
Independent of LIGO-Virgo detection of the gravitational wave signal, the Gamma-ray Burst Monitor (GBM) onboard the NASA Fermi satellite and the Anticoincidence Shield for the gamma-ray Spectrometer (SPI) of the International Gamma-Ray Astrophysics Laboratory (INTEGRAL) satellite were triggered by a faint short GRB (duration of
About 70 ground-based optical telescopes participated in the hunt and each of them adopted a different pointing sequence. This systematic approach enabled many groups to identify the optical counterpart candidate in a timely manner (with optical magnitude
Rapid follow-up of the gravitational wave and GRB signal in X-rays did not show any source comparable to, or brighter than, a typical afterglow of a short GRB. Since both the gravitational data and the faintness of the prompt GRB emission suggested a jet viewed significantly off axis, this could be expected, as the afterglows from misaligned GRB jets have longer rise times than those of jets observed at small viewing angles (Van Eerten and MacFadyen, 2011). Therefore, X-ray monitoring with Swift/XRT, Chandra, and Nustar continued, and
The Fermi Large Area Telescope covered the sky region of GW170817 starting only 20 min after the merger and did not detect any emission in the energy range 0.1–1 GeV to a limiting flux of
While the radio and X-ray detections are attributed to the afterglow of the short GRB, the ultraviolet, optical, and near-infrared data are dominated by the kilonova at early epochs (with a possible contribution at
The short GRB170817A, with an energy output of
Using milliarcsecond resolution radio VLBI observations at 75 and 230 days, Mooley et al. (2018b) detected superluminal motion with
The optical/near-infrared kilonova component subsided rapidly (see Section 3.1.2) leaving room to the afterglow emission: the HST observations at
The early ground-based optical and near-infrared and space-based (with Swift/UVOT) near-ultraviolet follow-up observations started immediately after identification of the optical counterpart of GW170817, detecting a rapid rise (
Starting the same night when the optical counterpart was detected, low resolution spectroscopy was carried out at the Magellan telescope (Shappee et al., 2017). This spectrum shows that the source is not yet transparent as it is emitting black body radiation, whose maximum lies however blueward of the sampled wavelength range, suggesting that the initial temperature may have been larger than
FIGURE 1. ESO VLT X-Shooter spectra of the counterpart of GW170817 from Pian et al. (2017) and Smartt et al. (2017), at phases indicated in days after merger time, corrected for Galactic extinction E (B-V) = 0.1 mag, deredshifted, and offset in flux by multiples of a
In particular, in the spectrum at day 1.5 an absorption feature extending from
At
HST and Chandra images, combined with VLT MUSE integral field spectroscopy of the optical counterpart of GW170817, show that its host Galaxy, NGC 4993, is a lenticular (S0) Galaxy at
Based only on the gravitational data and the standard siren argument and assuming that the optical counterpart represents the true sky location of the gravitational-wave source instead of marginalizing over a range of potential sky locations, Abbott et al. (2017d) determined a “gravitational” distance of
The unstable isotopes formed during coalescence of a binary neutron star system decay radioactively and the emitted gamma-ray photons are downscattered to the ultraviolet, optical, and infrared thermal radiation that constitutes the kilonova source (Section 3.1.2). Its time decline is determined by the convolution of radioactive decay chain curves of all present unstable nuclei. This is analogous to the supernova phenomenon, where however the vastly dominant radioactive chain is 56Ni decaying into 56Co. and then into 56Fe.
While radioactive nuclei decay, atoms recombine, as the source is cooling, and absorption features are imprinted in the kilonova spectra. Among neutron-rich nuclei, the lanthanides (atomic numbers 57–71) series have full f-shells and therefore numerous atomic transitions that suppress the spectrum at shorter wavelengths (
If the neutron stars coalescence does not produce instantaneously a black hole and a hypermassive neutron star is formed as a transitory remnant, a neutrino wind is emitted that may inhibit the formation of neutrons and reduce the amount of neutron-rich elements (Fernández and Metzger, 2013; Kajino et al., 2014; Kiuchi et al., 2014; Metzger and Fernández, 2014; Perego et al., 2014; Kasen et al., 2015; Lippuner et al., 2017). This “postmerger” kilonova component, of preferentially polar direction, is thus relatively poor in lanthanides and gives rise to a less reddened spectrum (Kasen et al., 2017; Tanaka et al., 2017).
The optical/near-infrared spectral behavior of kilonova is analogous to that of supernovae with the largest kinetic energies (
The gravitational and electromagnetic event of August 17, 2017, provided the long-awaited confirmation that binary neutron star mergers are responsible for well identifiable gravitational signals at kHz frequencies, for short GRBs, and for thermal sources, a. k.a. kilonovae or macronovae, produced by the radioactive decay of unstable heavy elements synthesized via r-process during the coalescence. The intensive and long-term electromagnetic monitoring from ground and space allowed clear detection of the counterpart at all wavelengths. Brief (
Doubtlessly, this series of breakthroughs were made possible by the closeness of the source (40 Mpc), almost unprecedented for GRBs, and by the availability of first-class ground-based and space-borne instruments. The many findings and exceptional new physical insight afforded by GW170817/GRB170817A make it a rosetta stone for future similar events. When a sizable group of sources with good gravitational and electromagnetic detections will be available, the properties of binary systems containing at least one neutron star, of their mergers and their aftermaths, can be mapped. It will then become possible to clarify how the dynamically ejected mass depends on the binary system parameters, mass asymmetry, and neutron stars equation of state (Ruffert and Janka, 2001; Hotokezaka et al., 2013), how the jet forms and evolves, which kinematic regimes and geometry it takes up in time, and how the GRB and afterglow observed phenomenologies can help distinguish the intrinsic properties from viewing angle effects (Janka et al., 2006; Lamb and Kobayashi, 2018; Ioka and Nakamura, 2019), what the detailed chemical content of kilonova ejecta is and how the r-process abundance pattern inferred from kilonova spectra compares with the history of heavy elements cosmic enrichment (Rosswog et al., 2018), how kilonovae can help constrain the binary neutron star rates and how the parent population of short GRBs evolves (Guetta and Stella, 2009; Yang et al., 2017; Belczynski et al., 2018; Artale et al., 2019; Matsumoto and Piran, 2020), and how gravitational and electromagnetic data can be used jointly to determine the cosmological parameters (Schutz, 1986; Del Pozzo, 2012; Abbott et al., 2017d), to mention only some fundamental open problems. Comparison of the optical and near-infrared light curves of GW170817 kilonova with those of short GRBs with known redshift suggests infact significant diversity in the kilonova component luminosities (Gompertz et al., 2018; Rossi et al., 2020).
Regrettably, short GRBs viewed at random angles, and not pole on, are relativistically beamed away from the observer direction and kilonovae are intrinsically weak. These circumstances make electromagnetic detections very difficult if the sources lie at more than
The search for electromagnetic counterparts of gravitational radiation signals is currently thwarted primarily by the large uncertainty of their localization in the sky, which is usually no more accurate than several dozens of square degrees. Much smaller error boxes are expected to be available when the KAGRA (which had already joined LIGO-Virgo in the last months of the 2019–2020 observing run) and the INDIGO interferometers will operate at full regime as part of the network during the next observing run (Abbott et al., 2018). Observing modes, strategies, and simulations are being implemented to optimize the electromagnetic multiwavelength search and follow-up (Bartos et al., 2016; Patricelli et al., 2018; Cowperthwaite et al., 2019; Graham et al., 2019; Artale et al., 2020), and new dedicated space-based facilities are designed with critical capabilities of large sky area coverage and rapid turnaround (e.g., ULTRASAT, Sagiv et al., 2014; THESEUS, Amati et al., 2018, Stratta et al., 2018; DORADO, Cenko, 2019), to maximize the chance of detection of dim, fast-declining transients.
Finally, the possible detection of elusive MeV and
The author confirms being the sole contributor of this work and has approved it for publication.
The author declares that the research was conducted in the absence of any commercial or financial relationships that could be construed as a potential conflict of interest.
The author is indebted to T. Belloni, S. Cristallo, Th. Janka, P. Mazzali, A. Possenti, M. Tanaka, and F. Thielemann for discussion. She acknowledges hospitality from Liverpool John Moores University, Weizmann Institute of Science, Rehovot, and the Hebrew University of Jerusalem, Israel; National Astronomical Observatory of Japan, Tokyo; Beihang University, Beijing; Yunnan National Astronomical Observatory, Kunming, China; and Max-Planck Institute for Astrophysics and Munich Institute for Astro- and Particle Physics, Garching, Germany, where part of this work was accomplished.
1http://www.mpe.mpg.de/jcg/grbgen.html
Aartsen, M. G., Ackermann, M., Adams, J., Aguilar, J. A., Ahlers, M., Ahrens, M., et al. (2020). IceCube search for neutrinos coincident with compact binary mergers from LIGO-virgo’s first gravitational-wave transient catalog. Astrophys. J. 898, L10. doi:10.3847/2041-8213/ab9d24
Abbott, B. P., Abbott, R., Abbott, T. D., Abernathy, M. R., Acernese, F., Ackley, K., et al. (2016). Properties of the binary black hole merger GW150914. Phys. Rev. Lett. 116, 241102. doi:10.1103/PhysRevLett.116.241102
Abbott, B. P., Abbott, R., Abbott, T. D., Acernese, F., Ackley, K., Adams, C., et al. (2017a). GW170817: observation of gravitational waves from a binary neutron star inspiral. Phys. Rev. Lett. 119, 161101. doi:10.1103/PhysRevLett.119.161101
Abbott, B. P., Abbott, R., Abbott, T. D., Acernese, F., Ackley, K., Adams, C., et al. (2017b). Gravitational waves and gamma-rays from a binary neutron star merger: GW170817 and GRB 170817A. Astrophys. J. 848, L13. doi:10.3847/2041-8213/aa920c
Abbott, B. P., Abbott, R., Abbott, T. D., Acernese, F., Ackley, K., Adams, C., et al. (2017c). Multi-messenger observations of a binary neutron star merger. Astrophys. J. 848, L12. doi:10.3847/2041-8213/aa91c9
Abbott, B. P., Abbott, R., Abbott, T. D., Acernese, F., Ackley, K., Adams, C., et al. (2017d). A gravitational-wave standard siren measurement of the Hubble constant. Nature 551, 85. doi:10.1038/nature24471
Abbott, B. P., Abbott, R., Abbott, T. D., Acernese, F., Ackley, K., Adams, C., et al. (2017e). Search for post-merger gravitational waves from the remnant of the binary neutron star merger GW170817. Astrophys. J. 851, L16. doi:10.3847/2041-8213/aa9a35
Abbott, B. P., Abbott, R., Abbott, T. D., Abernathy, M. R., Acernese, F., Ackley, K., et al. (2018). Prospects for observing and localizing gravitational-wave transients with Advanced LIGO, Advanced Virgo and KAGRA. Living Rev. Relat. 21, 3. doi:10.1007/s41114-018-0012-9
Abbott, B. P., Abbott, R., Abbott, T. D., Abraham, S., Acernese, F., Ackley, K., et al. (2019). GWTC-1: a gravitational-wave transient catalog of compact binary mergers observed by LIGO and Virgo during the first and second observing runs. Phys. Rev. X 9, 031040. doi:10.1103/PhysRevX.9.031040
Abbott, B. P., Abbott, R., Abbott, T. D., Abraham, S., Acernese, F., Ackley, K., et al. (2020a). Observation of a compact binary coalescence with total mass
Abbott, B. P., Abbott, T. D., Abraham, S., Acernese, F., Ackley, K., Adams, C., et al. (2020b). GW190814: gravitational waves from the coalescence of a 23 solar mass black hole with a 2.6 solar mass compact object. Astrophys. J. 896, L44. doi:10.3847/2041-8213/ab960f
Abdalla, H., Abramowski, A., Aharonian, F., Ait Benkhali, F., Angüner, E. O., Arakawa, M., et al. (2017). TeV gamma-ray observations of the binary neutron star merger GW170817 with H. E.S.S.. Astrophys. J. 850, L22. doi:10.3847/2041-8213/aa97d2
Abe, K., Bronner, C., Hayato, Y., Ikeda, M., Iyogi, K., and Kameda, J. (2018). Search for neutrinos in super-kamiokande associated with the GW170817 neutron-star merger. Astrophys. J. 857, L4. doi:10.3847/2041-8213/aabaca
Ackley, K., Amati, L., Barbieri, C., Bauer, F. E., Benetti, S., and Bernardini, M. G., (Forthcoming 2020). Observational constraints on the optical and near-infrared emission from the neutron star-black hole binary merger S190814bv. Astr. Astrophys Available at: https://arxiv.org/abs/2002.01950 (Accessed February 5, 2020).
Ajello, M., Allafort, A., Axelsson, M., Baldini, L., Barbiellini, G., Baring, M. G., et al. (2018). Fermi-LAT observations of LIGO/Virgo event GW170817. Astrophys. J. 861, 85. doi:10.3847/1538-4357/aac515
Albert, A., Andre, M., Anghinolfi, M., Ardid, M., Aubert, J.-J., Aublin, J., et al. (2017). Search for high-energy neutrinos from binary neutron star merger GW170817 with ANTARES, IceCube, and the Pierre auger observatory. Astrophys. J. 850, L35. doi:10.3847/2041-8213/aa9aed
Alexander, K. D., Berger, E., Fong, W., Williams, P. K. G., Guidorzi, C., Margutti, R., et al. (2017). The electromagnetic counterpart of the binary neutron star merger LIGO/Virgo GW170817. VI. Radio constraints on a relativistic jet and predictions for late-time emission from the kilonova ejecta. Astrophys. J. 848, L21. doi:10.3847/2041-8213/aa905d
Amati, L., O’Brien, P., Götz, D., Bozzo, E., Tenzer, C., Frontera, F., et al. (2018). The THESEUS space mission concept: science case, design and expected performances. Adv. Space Res. 62, 191. doi:10.1016/j.asr.2018.03.010
Andreoni, I., Ackley, K., Cooke, J., Acharyya, A., Allison, J. R., Anderson, G. E., et al. (2017). Follow up of GW170817 and its electromagnetic counterpart by Australian-led observing programmes. Pub. Astron. Soc. Au. 34, 69. doi:10.1017/pasa.2017.65
Andreoni, I., Goldstein, D. A., Kasliwal, M. M., Nugent, P. E., Zhou, R., Newman, J. A., et al. (2020). GROWTH on S190814bv: deep synoptic limits on the optical/near-infrared counterpart to a neutron star-black hole merger. Astrophys. J. 890, 131. doi:10.3847/1538-4357/ab6a1b
Annala, E., Gorda, T., Kurkela, A., and Vuorinen, A. (2018). Gravitational-wave constraints on the neutron-star-matter equation of state. Phys. Rev. Lett. 120, 172703. doi:10.1103/PhysRevLett.120.172703
Antier, S., Agayeva, S., Almualla, M., Awiphan, S., Baransky, K., Barynova, S., et al. (2020). GRANDMA observations of advanced LIGO’s and advanced Virgo’s third observational campaign. Mon. Not. Roy. Astron. Soc. 497, 5518. doi:10.1093/mnras/staa1846
Antonelli, L. A., D’Avanzo, P., Perna, R., Amati, L., Covino, S., Cutini, S., et al. (2009). Grb 090426: the farthest short gamma-ray burst? Astron. Astrophys. 507, L45. doi:10.1051/0004-6361/200913062
Arcavi, I., Hosseinzadeh, G., Andrew Howell, D., McCully, C., Poznanski, D., Kasen, D., et al. (2017). Optical emission from a kilonova following a gravitational-wave-detected neutron-star merger. Nature 551, 64. doi:10.1038/nature24291
Artale, M. C., Michela, M., Nicola, G., Sabha, N. B., Mario, S., Santoliquido, F., et al. (2019). Host galaxies of merging compact objects: mass, star formation rate, metallicity, and colours. Mon. Not. Roy. Astron. Soc. 487, 1675. doi:10.1093/mnras/stz1382
Artale, M. C., Bouffanais, Y., Mapelli, M., Giacobbo, N., Sabha, N. B., Santoliquido, F., et al. (2020). An astrophysically motivated ranking criterion for low-latency electromagnetic follow-up of gravitational wave events. Mon. Not. Roy. Astron. Soc. 495, 1841. doi:10.1093/mnras/staa1252
Ashall, C., and Mazzali, P. (2020). Extracting high-level information from gamma-ray burst supernova spectra. Mon. Not. Roy. Astron. Soc. 492, 5956. doi:10.1093/mnras/staa212
Banerjee, P., Haxton, W. C., and Qian, Y.-Z. (2011). Long, cold, early r process? Neutrino-Induced nucleosynthesis in He shells revisited. Phys. Rev. Lett. 106, 201104. doi:10.1103/PhysRevLett.106.201104
Barnes, J., and Kasen, D. (2013). Effect of a high opacity on the light curves of radioactively powered transients from compact. object mergers. Astrophys. J. 775, 18. doi:10.1088/0004-637X/775/1/18
Bartos, I., Huard, T. L., and Márka, S. (2016). James webb space telescope can detect kilonovae in gravitational wave follow-up search. Astrophys. J. 816, 61. doi:10.3847/0004-637X/816/2/61
Bartos, I., Veske, D., Keivani, A., Marka, Z., Countryman, S., Blaufuss, E., et al. (2019). Bayesian multimessenger search method for common sources of gravitational waves and high-energy neutrinos. Phys. Rev. D 100, 083017. doi:10.1103/PhysRevD.100.083017
Bauswein, A., Just, O., Janka, H.-J., and Stergioulas, N. (2017). Neutron-star radius constraints from GW170817 and future detections. Astrophys. J. 850, L34. doi:10.3847/2041-8213/aa9994
Belczynski, K., Askar, A., Arca-Sedda, M., Chruslinska, M., Donnari, M., Giersz, M., et al. (2018). The origin of the first neutron star - neutron star merger. Astron. Astrophys. 615, A91. doi:10.1051/0004-6361/201732428
Beniamini, P., Duran, R. B., Petropoulou, M., and Giannios, D. (2020). Ready, set, launch: time interval between a binary neutron star merger and short gamma-ray burst jet formation. Astrophys. J. 895, L33. doi:10.3847/2041-8213/ab9223
Berger, E., Fong, W., and Chornock, R. (2013). An r-process kilonova associated with the short-hard GRB 130603B. Astrophys. J. 774, L23. doi:10.1088/2041-8205/774/2/L23
Berger, E. (2014). Short-duration gamma-ray bursts. Annu. Rev. Astron. Astrophys. 52, 43. doi:10.1146/annurev-astro-081913-035926
Blanchard, P. K., Berger, E., Fong, W., Nicholl, M., Leja, J., Conroy, C., et al. (2017). The electromagnetic counterpart of the binary neutron star merger LIGO/Virgo GW170817. VII. Properties of the host galaxy and constraints on the merger timescale. Astrophys. J. 848, L22. doi:10.3847/2041-8213/aa9055
Blanchet, L. (2014). Gravitational radiation from post-Newtonian sources and inspiralling compact binaries. Living Rev. Relat. 17, 2. doi:10.12942/lrr-2014-2
Blinnikov, S. I., Novikov, I. D., Perevodchikova, T. V., and Polnarev, A. G. (1984). Exploding neutron stars in close binaries. Sov. Astron. Lett. 10, 177.
Bromberg, O., Nakar, E., Piran, T., and Sari, R. (2018). The γ-rays that accompanied GW170817 and the observational signature of a magnetic jet breaking out of NS merger ejecta. Mon. Not. Roy. Astron. Soc. 475, 2971. doi:10.1093/mnras/stx3316
Bromberg, O., Nakar, E., Piran, T., and Sari, R. (2013). Short versus long and collapsars versus non-collapsars: a quantitative classification of gamma-ray bursts. Astrophys. J. 764, 179. doi:10.1088/0004-637X/764/2/179
Burbidge, E. M., Burbidge, G. R., Fowler, W. A., and Hoyle, F. (1957). Synthesis of the elements in stars. Rev. Mod. Phys. 29, 547. doi:10.1103/RevModPhys.29.547
Burgay, M., D’Amico, N., Possenti, A., Manchester, R. N., Lyne, A. G., and Joshi, B. C. (2003). An increased estimate of the merger rate of double neutron stars from observations of a highly relativistic system. Nature. 426, 531. doi:10.1038/nature02124
Burgio, G. F., Drago, A., Pagliara, G., Schulze, H.-J., and Wei, J.-B. (2018). Are small radii of compact stars ruled out by GW170817/AT2017gfo?. Astrophys. J. 860, 139. doi:10.3847/1538-4357/aac6ee
Burns, E., Connaughton, V., Zhang, B.-B., Lien, A., Briggs, M. S., Goldstein, A., et al. (2016). Do the Fermi gamma-ray burst monitor and Swift burst alert telescope see the same short gamma-ray bursts?. Astrophys. J. 818, 110. doi:10.3847/0004-637X/818/2/110
Busso, M., Gallino, R., and Wasserburg, G. J. (1999). Nucleosynthesis in asymptotic giant branch stars: relevance for galactic enrichment and solar system formation. Annu. Rev. Astron. Astrophys. 37, 239. doi:10.1146/annurev.astro.37.1.239
Cantiello, M., Jensen, J. B., Blakeslee, J. P., Berger, E., Levan, A. J., Tanvir, N. R., et al. (2018). A precise distance to the host galaxy of the binary neutron star merger GW170817 using surface brightness fluctuations. Astrophys. J. 854, L31. doi:10.3847/2041-8213/aaad64
Cenko, S. B. (2019). The gravitational-wave ultraviolet counterpart imager (GUCI) network. American Astronomical Society Meeting Abstracts 234, 212.
Chornock, R., Berger, E., Kasen, D., Cowperthwaite, P. S., Nicholl, M., Villar, V. A., et al. (2017). The electromagnetic counterpart of the binary neutron star merger LIGO/Virgo GW170817. IV. Detection of near-infrared signatures of r-process nucleosynthesis with gemini-south. Astrophys. J. 848, L19. doi:10.3847/2041-8213/aa905c
Chruslinska, M., Belczynski, K., Klencki, J., and Benacquista, M. (2018). Double neutron stars: merger rates revisited. Mon. Not. Roy. Astron. Soc. 474, 2937. doi:10.1093/mnras/stx2923
Côté, B., Fryer, C. L., Belczynski, K., Korobkin, O., Chruślińska, M., Vassh, N., et al. (2018). The origin of r-process elements in the milky way. Astrophys. J. 855, 99. doi:10.3847/1538-4357/aaad67
Côté, B., Eichler, M., Arcones, A., Hansen, C. J., Simonetti, P., Frebel, A., et al. (2019). Neutron star mergers might not Be the only source of r-process elements in the milky way. Astrophys. J. 875, 106. doi:10.3847/1538-4357/ab10db
Coughlin, M. W., Ahumada, T., Anand, S., De, K., Hankins, M. J., Kasliwal, M. M., et al. (2019). GROWTH on S190425z: searching thousands of square degrees to identify an optical or infrared counterpart to a binary neutron star merger with the zwicky transient facility and palomar gattini-IR. Astrophys. J. 885, L19. doi:10.3847/2041-8213/ab4ad8
Coulter, D. A., Foley, R. J., Kilpatrick, C. D., Drout, M. R., Piro, A. L., Shappee, B. J., et al. (2017). Swope Supernova Survey 2017a (SSS17a), the optical counterpart to a gravitational wave source. Science 358, 1556. doi:10.1126/science.aap9811
Covino, S., Wiersema, K., Fan, Y. Z., Toma, K., Higgins, A. B., Melandri, A., et al. (2017). The unpolarized macronova associated with the gravitational wave event GW 170817. Nature Astr 1, 791. doi:10.1038/s41550-017-0285-z
Cowan, J. J., Sneden, C., Lawler, J. E., Aprahamian, A., Weischer, M., Langanke, M., et al. (Forthcoming 2020). Origin of the heaviest elements: the rapid neutron-capture process. Rev. Mod. Phys Available at: https://arxiv.org/abs/1901.01410 (Accessed January 5, 2020).
Cowperthwaite, P. S., Berger, E., Villar, V. A., Metzger, B. D., Nicholl, M., Chornock, R., et al. (2017). The electromagnetic counterpart of the binary neutron star merger LIGO/Virgo GW170817. II. UV, optical, and near-infrared light curves and comparison to kilonova models. Astrophys. J. 848, L17. doi:10.3847/2041-8213/aa8fc7
Cowperthwaite, P. S., Villar, V. A., Scolnic, D. M., and Berger, E. (2019). LSST target-of-opportunity observations of gravitational-wave events: essential and efficient. Astrophys. J. 874, 88. doi:10.3847/1538-4357/ab07b6
D’Avanzo, P., Campana, S., Salafia, O. S., Ghirlanda, G., Ghisellini, G., Melandri, A., et al. (2018). The evolution of the X-ray afterglow emission of GW 170817/GRB 170817A in XMM-Newton observations. Astron. Astrophys. 613, L1. doi:10.1051/0004-6361/201832664
Davies, M. B., Benz, W., Piran, T., and Thielemann, F. K. (1994). Merging neutron stars. I. Initial results for coalescence of noncorotating systems. Astrophys. J. 431, 742. doi:10.1086/174525
Del Pozzo, W. (2012). Inference of the cosmological parameters from gravitational waves: application to second generation interferometers. Phys. Rev. D 86, 043011. doi:10.1103/PhysRevD.86.043011
Díaz, M. C., Macri, L. M., Garcia Lambas, D., Mendes de Oliveira, C., Nilo Castellon, L., Ribeiro, T., et al. (2017). Observations of the first electromagnetic counterpart to a gravitational-wave source by the TOROS collaboration. Astrophys. J. 848, L29. doi:10.3847/2041-8213/aa9060
Drout, M. R., Piro, A. L., Shappee, B. J., Kilpatrick, C. D., Simon, J. D., Contreras, C., et al. (2017). Light curves of the neutron star merger GW170817/SSS17a: implications for r-process nucleosynthesis. Science. 358, 1570. doi:10.1126/science.aaq0049
Eichler, D., Livio, M., Piran, T., and Schramm, D. N. (1989). Nucleosynthesis, neutrino bursts and gamma-rays from coalescing neutron stars. Nature 340, 126. doi:10.1038/340126a0
Epstein, R. I., Colgate, S. A., and Haxton, W. C. (1988). Neutrino-induced r-process nucleosynthesis. Phys. Rev. Lett. 61, 2038. doi:10.1103/PhysRevLett.61.2038
Ergon, M., Fransson, C., Jerkstrand, A., Kozma, C., Kromer, M., and Spricer, K. (2018). Monte-Carlo methods for NLTE spectral synthesis of supernovae. Astron. Astrophys. 620, A156. doi:10.1051/0004-6361/201833043
Evans, P. A., Cenko, S. B., Kennea, J. A., Emrey, S. W. K., Kuin, N. P. M., Korobkin, O., et al. (2017). Swift and NuSTAR observations of GW170817: detection of a blue kilonova. Science. 358, 1565. doi:10.1126/science.aap9580
Fang, K., and Metzger, B. D. (2017). High-energy neutrinos from millisecond magnetars formed from the merger of binary neutron stars. Astrophys. J 849, 153. doi:10.3847/1538-4357/aa8b6a
Fernández, R., and Metzger, B. D. (2013). Delayed outflows from black hole accretion tori following neutron star binary coalescence. Mon. Not. Roy. Astron. Soc. 435, 502. doi:10.1093/mnras/stt1312
Fernández, R., and Metzger, B. D. (2016). Electromagnetic signatures of neutron star mergers in the advanced LIGO era. Annu. Rev. Nucl. Part Sci. 66, 23. doi:10.1146/annurev-nucl-102115-044819
Fong, W., Berger, E., and Fox, D. B. (2010). Hubble space telescope observations of short gamma-ray burst host galaxies: morphologies, offsets, and local environments. Astrophys. J. 708, 9. doi:10.1088/0004-637X/708/1/9
Fong, W., Berger, E., Margutti, R., and Zauderer, B. A. (2015). A decade of short-duration gamma-ray burst broadband Afterglows: energetics, circumburst densities, and jet opening angles. Astrophys. J. 815, 102. doi:10.1088/0004-637X/815/2/102
Fong, W., Blanchard, P. K., Alexander, K. D., Strader, J., Margutti, R., Hajela, A., et al. (2019). The optical afterglow of GW170817: an off-axis structured jet and deep constraints on a globular cluster origin. Astrophys. J. 883, L1. doi:10.3847/2041-8213/ab3d9e
Fontes, C. J., Fryer, C. L., Hungerford, A. L., Wollaeger, R. T., and Korobkin, O. (2020). A line-binned treatment of opacities for the spectra and light curves from neutron star mergers. Mon. Not. Roy. Astron. Soc. 493, 4143. doi:10.1093/mnras/staa485
Freiburghaus, C., Rosswog, S., and Thielemann, F. (1999). R-process in neutron star mergers. Astrophys. J. 525, L121. doi:10.1086/312343
Fryer, C. L., Herwig, F., Hungerford, A., and Timmes, F. X. (2006). Supernova fallback: a possible site for the r-process. Astrophys. J. 646, L131. doi:10.1086/507071
Galama, T. J., Vreeswjik, P. M., van Paradijs, J., Kouveliotou, C., Augusteijn, T., and Bohnhardt, H. (1998). An unusual supernova in the error box of the γ-ray burst of 25 April 1998. Nature 395, 670. doi:10.1038/27150
Gehrels, N., Cannizzo, J. K., Kanner, J., Kasliwal, M. M., Nissanke, S., and Singer, L. P. (2016). Galaxy strategy for LIGO-virgo gravitational wave counterpart searches. Astrophys. J. 820, 136. doi:10.3847/0004-637X/820/2/136
Ghirlanda, G., Salafia, O. S., Paragi, Z., Giroletti, M., Yang, J., Marcote, B., et al. (2019). Compact radio emission indicates a structured jet was produced by a binary neutron star merger. Science. 363, 968. doi:10.1126/science.aau8815
Goldstein, A., Veres, P., Burns, E., Briggs, M. S., Hamburg, R., Kocevski, D., et al. (2017). An ordinary short gamma-ray burst with extraordinary implications: fermi-GBM detection of GRB 170817A. Astrophys. J. 848, L14. doi:10.3847/2041-8213/aa8f41
Gomez, S., Hosseinzadeh, G., Cowperthwaite, P. S., Villar, V. A., Berger, E., Gardner, T., et al. (2019). A galaxy-targeted search for the optical counterpart of the candidate NS-BH merger S190814bv with magellan. Astrophys. J. 884, L55. doi:10.3847/2041-8213/ab4ad5
Gompertz, B. P., Levan, A. J., Tanvir, N. R., Hjorth, J., Covino, S., Evans, P. A., et al. (2018). The diversity of kilonova emission in short gamma-ray bursts. Astrophys. J. 860, 62. doi:10.3847/1538-4357/aac206
Gottlieb, O., Nakar, E., Piran, T., and Hotokezaka, K. (2018). A cocoon shock breakout as the origin of the γ-ray emission in GW170817. Mon. Not. Roy. Astron. Soc. 479, 588. doi:10.1093/mnras/sty1462
Graham, M. J., Kulkarni, S. R., Bellm, E. C., Adams, S. M., Barbarino, C., Blagorodnova, N., et al. (2019). The zwicky transient facility: science objectives. Publ. Astron. Soc. Pac. 131, 078001. doi:10.1088/1538-3873/ab006c
Guetta, D., and Stella, L. (2009). Short γ-ray bursts and gravitational waves from dynamically formed merging binaries. Astron. Astrophys. 498, 329. doi:10.1051/0004-6361:200810493
Hajela, A., Margutti, R., Alexander, K. D., Kathirgamaraju, A., Baldeschi, A., Guidorzi, C., et al. (2019). Two years of nonthermal emission from the binary neutron star merger GW170817: rapid fading of the jet afterglow and first constraints on the kilonova fastest ejecta. Astrophys. J. 886, L17. doi:10.3847/2041-8213/ab5226
Hallinan, G., Corsi, A., Mooley, K. P., Hotokezaka, K., Nakar, E., Kasliwal, M. M., et al. (2017). A radio counterpart to a neutron star merger. Science 358, 1579. doi:10.1126/science.aap9855
Hillebrandt, W., Takahaski, K., and Kodama, T. (1976). R-process nucleosynthesis: a dynamical model. Astron. Astrophys. 52, 63.
Hillier, D. J., and Dessart, L. (2019). Photometric and spectroscopic diversity of Type II supernovae. Astron. Astrophys. 631, A8. doi:10.1051/0004-6361/201935100
Hjorth, J., Levan, A. J., Tanvir, N. R., Lyman, J. D., Wojtak, R., Schrøder, S. L., et al. (2017). The distance to NGC 4993: the host galaxy of the gravitational-wave event GW170817. Astrophys. J. 848, L31. doi:10.3847/2041-8213/aa9110
Hoeflich, P., Hsiao, E. Y., Ashall, C., Burns, C. R., Diamond, T. R., Phillips, M. M., et al. (2017). Light and color curve properties of type ia supernovae: theory versus observations. Astrophys. J. 846, 58. doi:10.3847/1538-4357/aa84b2
Horowitz, C. J., Arcones, A., Côté, B., Dillmann, I., Nazarewicz, W., Roederer, I. U., et al. (2019). R-process nucleosynthesis: connecting rare-isotope beam facilities with the cosmos. J. Phys. G Nucl. Part. Phys. 46, 083001. doi:10.1088/1361-6471/ab0849
Hotokezaka, K., Kuichi, K., Kyutoku, K., Okawa, H., Sekiguchi, Y.-i., Shibata, M., et al. (2013). Mass ejection from the merger of binary neutron stars. Phys. Rev. D 87, 024001. doi:10.1103/PhysRevD.87.024001
Hotokezaka, K., Nakar, E., Gottlieb, O., Nissanke, S., Masuda, K., Hallinan, G., et al. (2019). A Hubble constant measurement from superluminal motion of the jet in GW170817. Nature Astr 3, 940. doi:10.1038/s41550-019-0820-1
Hulse, R. A., and Taylor, J. H. (1974). A high-sensitivity pulsar survey. Astrophys. J. 191, L59. doi:10.1086/181548
Hulse, R. A., and Taylor, J. H. (1975). Discovery of a pulsar in a binary system. Astrophys. J. 195, L51. doi:10.1086/181708
Im, M., Yoon, Y., Lee, S. J., Lee, H. M., Kim, J., Lee, C.-U., et al. (2017). Distance and properties of NGC 4993 as the host galaxy of the gravitational-wave source GW170817. Astrophys. J. 849, L16. doi:10.3847/2041-8213/aa9367
Ioka, K., and Nakamura, T. (2019). Spectral puzzle of the off-axis gamma-ray burst in GW170817. Mon. Not. Roy. Astron. Soc 487, 4884. doi:10.1093/mnras/stz1650
Iwamoto, K., Mazzali, P. A., Nomoto, K., Umeda, H., Nakamura, T., Patat, F., et al. (1998). A hypernova model for the supernova associated with the γ-ray burst of 25 April 1998. Nature. 395, 672. doi:10.1038/27155
Janka, H. -Th., Aloy, M.-A., Mazzali, P. A., and Pian, E. (2006). Off-Axis properties of short gamma-ray bursts. Astrophys. J. 645, 1305. doi:10.1086/504580
Ji, A. P., Frebel, A., Chiti, A., and Simon, J. D. (2016). R-process enrichment from a single event in an ancient dwarf galaxy. Nature 531, 610. doi:10.1038/nature17425
Jin, Z.-P., Li, X., Cano, Z., Covino, S., Fan, Y.-Z., Wei, D.-M., et al. (2015). The light curve of the macronova associated with the long-short burst GRB 060614. Astrophys. J. 811, L22. doi:10.1088/2041-8205/811/2/L22
Jin, Z.-P., Hotokezaka, K., Li, X., Tanaka, M., D’Avanzo, P., Fan, Y.-Z., et al. (2016). The Macronova in GRB 050709 and the GRB-macronova connection. Nat. Commun. 7, 12898. doi:10.1038/ncomms12898
Jin, Z.-P., Xiang, L., Hao, W., Yuan, Z.-W., Hao, N.-H., Yuan, Q., et al. (2018). Short GRBs: opening angles, local neutron star merger rate, and off-axis events for GRB/GW association. Astrophys. J. 857, 128. doi:10.3847/1538-4357/aab76d
Kajino, T., Grant, J. M., and Takehito, H. (2014). Neutrinos in core-collapse supernovae and nucleosynthesis. J. Phys. G Nucl. Phys. 41, 044007. doi:10.1088/0954-3899/41/4/044007
Kajino, T., Aoki, W., Balantekin, A. B., Diehl, R., Famiano, M. A., Mathews, G J., et al. (2019). Current status of r-process nucleosynthesis. Prog. Part. Nucl. Phys. 107, 109. doi:10.1016/j.ppnp.2019.02.008
Kasen, D., Badnell, N. R., and Barnes, J. (2013). Opacities and spectra of the r-process ejecta from neutron star mergers. Astrophys. J. 774, 25. doi:10.1088/0004-637X/774/1/25
Kasen, D., Rodrigo, F., and Brian, D. M. (2015). Kilonova light curves from the disc wind outflows of compact object mergers. Mon. Not. Roy. Astron. Soc. 450, 1777. doi:10.1093/mnras/stv721
Kasen, D., Metzger, B., Barnes, J., Quataeart, E., and Ramirez-Ruiz, E. (2017). Origin of the heavy elements in binary neutron-star mergers from a gravitational-wave event. Nature 551, 80. doi:10.1038/nature24453
Kasliwal, M. M., Nakar, E., Singer, L. P., Kaplan, D. L., Cook, D. O., Van Sistine, A., et al. (2017). Illuminating gravitational waves: a concordant picture of photons from a neutron star merger. Science 358, 1559. doi:10.1126/science.aap9455
Kasliwal, M. M., Anand, S., Ahumada, T., Stein, R., Carracedo, A. S., Andreoni, I., et al. (Forthcoming 2020). Kilonova luminosity function constraints based on zwicky transient facility searches for 13 neutron star mergers. Astrophys. J Available at: https://arxiv.org/abs/2006.11306 (Accessed June 19, 2020).
Kathirgamaraju, A., Giannios, D., and Beniamini, P. (2019). Observable features of GW170817 kilonova afterglow. Mon. Not. Roy. Astron. Soc. 487, 3914. doi:10.1093/mnras/stz1564
Kim, S., Schulze, S., Resmi, L., González-López, J., Higgins, A. B., Ishwara-Chandra, C. H., et al. (2017). ALMA and GMRT constraints on the off-axis gamma-ray burst 170817A from the binary neutron star merger GW170817. Astrophys. J. 850, L21. doi:10.3847/2041-8213/aa970b
Kimura, S. S., Murase, K., Bartos, I., Ioka, K., Heng, I. S., and Mészáros, P. (2018). Transejecta high-energy neutrino emission from binary neutron star mergers. Phys. Rev. D 98, 043020. doi:10.1103/PhysRevD.98.043020
Kiuchi, K., Kyutoku, K., Sekiduchi, Y., Shibata, M., and Wada, T. (2014). High resolution numerical relativity simulations for the merger of binary magnetized neutron stars. Phys. Rev. D. 90, 041502. doi:10.1103/PhysRevD.90.041502
Kouveliotou, C., Meegan, C. A., Fishman, G. J., Bhat, N. P., Briggs, M. S., Koshut, T. M., et al. (1993). Identification of two classes of gamma-ray bursts. Astrophys. J. 413, L101. doi:10.1086/186969
Kyutoku, K., and Kashiyama, K. (2018). Detectability of thermal neutrinos from binary neutron-star mergers and implications for neutrino physics. Phys. Rev. D 97, 103001. doi:10.1103/PhysRevD.97.103001
Lamb, G. P., and Kobayashi, S. (2018). GRB 170817A as a jet counterpart to gravitational wave triggerGW 170817. Mon. Not. Roy. Astron. Soc. 478, 733. doi:10.1093/mnras/sty1108
Lamb, G. P., Tanvir, N., Levan, A., Postigo, A. d. U., Kawaguchi, K., Corsi, A., et al. (2019). Short GRB 160821B: a reverse shock, a refreshed shock, and a well-sampled kilonova. Astrophys. J. 883, 48. doi:10.3847/1538-4357/ab38bb
Lattimer, J. M., and Schramm, D. N. (1974). Black-hole-neutron-star collisions. Astrophys. J. 192, L145. doi:10.1086/181612
Lattimer, J. M., and Schramm, D. N. (1976). The tidal disruption of neutron stars by black holes in close binaries. Astrophys. J. 210, 549. doi:10.1086/154860
Lazzati, D., Deich, A., Morsony, B. J., and Workman, J. C. (2017a). Off-axis emission of short gamma-ray bursts and the detectability of electromagnetic counterparts of gravitational-wave-detected binary mergers. Mon. Not. Roy. Astron. Soc. 471, 1652. doi:10.1093/mnras/stx1683
Lazzati, D., Lopez-Camara, D., Cantiello, M., Morsony, B. J., Perna, R., and Workman, J. C. (2017b). Off-axis prompt X-ray transients from the cocoon of short gamma-ray bursts. Astrophys. J. 848, L6. doi:10.3847/2041-8213/aa8f3d
Lazzati, D., Perna, R., Morsony, B. J., Lopez-Camara, D., Cantiello, M., Ciolfi, R., et al. (2018). Late time afterglow observations reveal a collimated relativistic jet in the ejecta of the binary neutron star merger GW170817. Phys. Rev. Lett. 120, 241103. doi:10.1103/PhysRevLett.120.241103
Levan, A., Crowther, P., Grijs, R. d., Langer, N., Xu, D., and Yoon, S.-C. (2016). Gamma-ray burst progenitors. Space Sci. Rev. 202, 33. doi:10.1007/s11214-016-0312-x
Levan, A., Lyman, J. D., Tanvir, N. R., Hjorth, J., Mandel, I., Stanway, E. R., et al. (2017). The environment of the binary neutron star merger GW170817. Astrophys. J. 848, L28. doi:10.3847/2041-8213/aa905f
Li, L.-X., and Paczyński, B. (1998). Transient events from neutron star mergers. Astrophys. J. 507, L59. doi:10.1086/311680
Lim, Y., and Holt, J. W. (2018). Neutron star tidal deformabilities constrained by nuclear theory and experiment. Phys. Rev. Lett. 121, 062701. doi:10.1103/PhysRevLett.121.062701
Lippuner, J., Fernandez, R., Roberts, L. F., Foucart, F., Rasen, D., Metzger, B. D., et al. (2017). Signatures of hypermassive neutron star lifetimes on r-process nucleosynthesis in the disc ejecta from neutron star mergers. Mon. Not. Roy. Astron. Soc. 472, 904. doi:10.1093/mnras/stx1987
Lipunov, V. M., Gorbovskoy, E., Kornilov, V. G., Tyurina, N., Balanutsa, P., Kuznetsov, A., et al. (2017). MASTER optical detection of the first LIGO/Virgo neutron star binary merger GW170817. Astrophys. J. 850, L1. doi:10.3847/2041-8213/aa92c0
Lyman, J. D., Lamb, G. P., Levan, A. J., Mandel, I., Tanvir, N. R., Kobayashi, S., et al. (2018). The optical afterglow of the short gamma-ray burst associated with GW170817. Nature Astr 2, 751. doi:10.1038/s41550-018-0511-3
Macias, P., and Ramirez-Ruiz, E. (2019). Constraining collapsar r-process models through stellar abundances. Astrophys. J. 877, L24. doi:10.3847/2041-8213/ab2049
Margutti, R., Berger, E., Fong, W., Guidorzi, C., Alexander, K. D., Metzger, B. D., et al. (2017). The electromagnetic counterpart of the binary neutron star merger LIGO/Virgo GW170817. V. Rising X-ray emission from an off-axis jet. Astrophys. J. 848, L20. doi:10.3847/2041-8213/aa9057
Martinez, J. G., Stovall, K., Freire, P. C. C., Deneva, J. S., Tauris, T. M., Ridolfi, A., et al. (2017). Pulsar J1411+2551: a low-mass double neutron star system. Astrophys. J. 851, L29. doi:10.3847/2041-8213/aa9d87
Matsumoto, T., and Piran, T. (2020). On short GRBs similar to GRB 170817A detected by Fermi-GBM. Mon. Not. Roy. Astron. Soc. 492, 4283. doi:10.1093/mnras/staa050
Mazzali, P. A., Iwamoto, K., and Nomoto, K. (2000). A spectroscopic analysis of the energetic type ic hypernova SN 1997ef. Astrophys. J. 545, 407. doi:10.1086/317808
Mazzali, P. A., Sullivan, M., Pian, E., Greiner, J., and Kann, D. A. (2016). Spectrum formation in superluminous supernovae (Type I). Mon. Not. Roy. Astron. Soc. 458, 3455. doi:10.1093/mnras/stw512
McCully, C., Hiramatsu, D., Howell, D. A., Hosseinzadeh, G., Arcavi, I., Kasen, D., et al. (2017). The rapid reddening and featureless optical spectra of the optical counterpart of GW170817, at 2017gfo, during the first four days. Astrophys. J. 848, L32. doi:10.3847/2041-8213/aa9111
Mennekens, N., and Vanbeveren, D. (2014). Massive double compact object mergers: gravitational wave sources and r-process element production sites. Astron. Astrophys. 564, A134. doi:10.1051/0004-6361/201322198
Metzger, B. D., and Fernández, R. (2014). Red or blue? A potential kilonova imprint of the delay until black hole formation following a neutron star merger. Mon. Not. Roy. Astron. Soc. 441, 3444. doi:10.1093/mnras/stu802
Metzger, B. D. (2019). Kilonovae. Living Reviews in Relativity. 23, 1. doi:10.1007/s41114-019-0024-0
Mooley, K., Nakar, E., Hotokezaka, K., Hallinan, G., Corsi, A., Frail, D. A., et al. (2018a). A mildly relativistic wide-angle outflow in the neutron-star merger event GW170817. Nature 554, 207. doi:10.1038/nature25452
Mooley, K., Deller, A. T., Gottlieb, O., Nakar, E., Hallinan, G., Bourke, S., et al. (2018b). Superluminal motion of a relativistic jet in the neutron-star merger GW170817. Nature 561, 355. doi:10.1038/s41586-018-0486-3
Mooley, K., Frail, D. A., Dobie, D., Lenc, E., Corsi, A., De, K., et al. (2018c). A strong jet signature in the late-time light curve of GW170817. Astrophys. J. 868, L11. doi:10.3847/2041-8213/aaeda7
Most, E. R., Lukas, R. W., Rezzolla, L., and Schaffner-Bielich, J. (2018). New constraints on radii and tidal deformabilities of neutron stars from GW170817. Phys. Rev. Lett. 120, 261103. doi:10.1103/PhysRevLett.120.261103
Nakamura, T., Mazzali, P. A., Nomoto, K., and Iwamoto, K. (2001). Light curve and spectral models for the hypernova SN 1998BW associated with GRB 980425. Astrophys. J. 550, 991. doi:10.1086/319784
Nakamura, K., Kajino, T., Mathews, G. J., Sato, S., and Harikae, S. (2013). A review of R-PROCESS nucleosynthesis in the collapsar jet. Int. J. Mod. Phys. E. 22, 1330022. doi:10.1142/S0218301313300221
Nakamura, K., Kajino, T., Mathews, G. J., Sato, S., and Harikae, A. (2015). r-process nucleosynthesis in the MHD+neutrino-heated collapsar jet. Astron. Astrophys. 582, A34. doi:10.1051/0004-6361/201526110
Nakano, H., Narikawa, T., Oohara, K.-I., Sakai, K., Shinkai, H.-A., Takahashi, H., et al. (2019). Comparison of various methods to extract ringdown frequency from gravitational wave data. Phys. Rev. D 99, 124032. doi:10.1103/PhysRevD.99.124032
Nakar, E., and Piran, T. (2017). The observable signatures of GRB cocoons. Astrophys. J. 834, 28. doi:10.3847/1538-4357/834/1/28
Nakar, E. (2007). Short-hard gamma-ray bursts. Phys. Rep. 442, 166. doi:10.1016/j.physrep.2007.02.005
Nicholl, M., Berger, E., Kasen, D., Metzger, B. D., Elias, J., Briceno, C., et al. (2017). The electromagnetic counterpart of the binary neutron star merger LIGO/Virgo GW170817. III. Optical and UV spectra of a blue kilonova from fast polar ejecta. Astrophys. J. 848, L18. doi:10.3847/2041-8213/aa9029
Ning, H., Qian, Y.-Z., and Meyer, B. S. (2007). R-Process nucleosynthesis in shocked surface layers of O-Ne-Mg cores. Astrophys. J. 667, L159. doi:10.1086/522372
Nishimura, S., Kotake, K., Hashimoto, M.-A., Yamada, S., Nishimura, N., Fujimoto, S., et al. (2006). R-Process nucleosynthesis in magnetohydrodynamic jet explosions of core-collapse supernovae. Astrophys. J. 642, 410. doi:10.1086/500786
Nissanke, S., Holz, D. E., Hughes, S. A., Dalal, N., and Sievers, J. L. (2010). Exploring short gamma-ray bursts as gravitational-wave standard sirens. Astrophys. J. 725, 496. doi:10.1088/0004-637X/725/1/496
Özel, F., and Freire, P. (2016). Masses, radii, and the equation of state of neutron stars. Annu. Rev. Astron. Astrophys. 54, 401. doi:10.1146/annurev-astro-081915-023322
Palmese, A., Hartley, W., Tarsitano, F., Conselice, C., Lahav, O., Allam, S. S., et al. (2017). Evidence for dynamically driven formation of the GW170817 neutron star binary in NGC 4993. Astrophys. J. 849, L34. doi:10.3847/2041-8213/aa9660
Pan, Y. -C., Kilpatrick, C. D., Simon, J. D., Xhakaj, E., Boutsia, K., Coulter, D.A., et al. (2017). The old host-galaxy environment of SSS17a, the first electromagnetic counterpart to a gravitational-wave source. Astrophys. J 848, L30. doi:10.3847/2041-8213/aa9116
Pandey, S. B., Hu, Y., Tirado, A. J. C., Pozanenko, A. S., Sanchez-Ramirez, R., Gorosabel, J., et al. (2019). A multiwavelength analysis of a collection of short-duration GRBs observed between 2012 and 2015. Mon. Not. Roy. Astron. Soc. 485, 5294. doi:10.1093/mnras/stz530
Patricelli, B., Stamerra, A., Razzano, M., Pian, E., and Cella, G. (2018). Searching for gamma-ray counterparts to gravitational waves from merging binary neutron stars with the Cherenkov Telescope Array. J. Cosmol. Astropart. Phys. 5, 056. doi:10.1088/1475-7516/2018/05/056
Perego, A., Rosswog, S., Cabezon, R., Korobkin, O., Kaeppeli, R., Arcones, A., et al. (2014). Neutrino-driven winds from neutron star merger remnants. Mon. Not. Roy. Astron. Soc. 443, 3134. doi:10.1093/mnras/stu1352
Pfahl, E., Podsiadlowski, P., and Rappaport, S. (2005). Relativistic binary pulsars with black hole companions. Astrophys. J. 628, 343. doi:10.1086/430515
Pian, E., D’Avanzo, P., Benetti, S., Branchesi, M., Brocato, E., Campana, S., et al. (2017). Spectroscopic identification of r-process nucleosynthesis in a double neutron-star merger. Nature. 551, 67. doi:10.1038/nature24298
Piro, A. L., and Kollmeier, J. A. (2018). Evidence for cocoon emission from the early light curve of SSS17a. Astrophys. J. 855, 103. doi:10.3847/1538-4357/aaaab3
Pozanenko, A. S., Barkov, M. V., Minaev, P. V., Volnova, A. A., Mazaeva, E. D., Moskvitin, A. S., et al. (2018). GRB 170817A associated with GW170817: multi-frequency observations and modeling of prompt gamma-ray emission. Astrophys. J. 852, L30. doi:10.3847/2041-8213/aaa2f6
Pozanenko, A. S., Minaev, P. Y., Grebenev, S. A., and Chelovekov, I. V. (2019). Observation of the second LIGO/Virgo event connected with a binary neutron star merger S190425z in the gamma-ray range. Astrophys. Lett. 45, 710. doi:10.1134/S1063773719110057
Radice, D., Perego, A., Zappa, F., and Bernuzzi, S. (2018). GW170817: joint constraint on the neutron star equation of state from multimessenger observations. Astrophys. J. 852, L29. doi:10.3847/2041-8213/aaa402
Ramirez-Ruiz, E., Trenti, M., Macleod, M., Roberts, L. F., Lee, W. H., Saladino-Rosas, M. I., et al. (2015). Compact stellar binary assembly in the first nuclear star clusters and r-process synthesis in the early Universe. Astrophys. J. 802, L22. doi:10.1088/2041-8205/802/2/L22
Rauscher, T., Applegate, J. M., Cowan, J. J., and Weischer, M. (1994). Production of heavy elements in inhomogeneous cosmologies. Astrophys. J. 429, 499. doi:10.1086/174339
Renzo, M., Zapartas, E., de Mink, S. E., Gotberg, Y., Justham, S., Farmer, R. J., et al. (2019). Massive runaway and walkaway stars. A study of the kinematical imprints of the physical processes governing the evolution and explosion of their binary progenitors. Astron. Astrophys. 624, A66. doi:10.1051/0004-6361/201833297
Rossi, A., Stratta, G., Maiorano, E., Spighi, D., Masetti, N., Palazzi, E., et al. (2020). A comparison between short GRB afterglows and kilonova AT2017gfo: shedding light on kilonovae properties. Mon. Not. Roy. Astron. Soc. 493, 3379. doi:10.1093/mnras/staa4794
Rosswog, S., and Liebendörfer, M. (2003). High-resolution calculations of merging neutron stars - II. Neutrino emission. Mon. Not. Roy. Astron. Soc. 342, 673. doi:10.1046/j.1365-8711.2003.06579.x
Rosswog, S., Liebendorfer, M., Thielemann, F.-K., Davies, M. B., Benz, W., and Piran, T. (1999). Mass ejection in neutron star mergers. Astron. Astrophys. 341, 499.
Rosswog, S., Sollerman, J., Feindt, U., Goobar, A., Korobkin, O., Wollaeger, R., et al. (2018). The first direct double neutron star merger detection: implications for cosmic nucleosynthesis. Astron. Astrophys. 615, A132. doi:10.1051/0004-6361/201732117
Ruffert, M., and Janka, H. -Th. (2001). Coalescing neutron stars - a step towards physical models. III. Improved numerics and different neutron star masses and spins. Astron. Astrophys. 380, 544. doi:10.1051/0004-6361:20011453
Safarzadeh, M., Sarmento, R., and Scannapieco, E. (2019). On neutron star mergers as the source of r-process-enhanced metal-poor stars in the milky way. Astrophys. J. 876, 28. doi:10.1088/2041-8205/802/2/L22
Sagiv, I., Gal-Yam, A., Ofek, E. O., Waxman, E., Aharonson, O., Kulkarni, S. R., et al. (2014). Science with a wide-field UV transient explorer. Astrophys. J. 147, 79. doi:10.1088/0004-6256/147/4/79
Salafia, O. S., Ghisellini, G., Ghirlanda, G., and Colpi, M. (2018). Interpreting GRB170817A as a giant flare from a jet-less double neutron star merger. Astron. Astrophys. 619, A18. doi:10.1051/0004-6361/201732259
Sana, H., Mink, S. E. d., Koter, A. d., Langer, N., Evans, C. J., Geiles, M., et al. (2012). Binary interaction dominates the evolution of massive stars. Science. 337, 444. doi:10.1126/science.1223344
Savchenko, V., Ferrigno, C., Kuulkers, E., Bazzano, A., Bozzo, E., Brandt, S., et al. (2017). Integral detection of the first prompt gamma-ray signal coincident with the gravitational-wave event GW170817. Astrophys. J. 848, L15. doi:10.3847/2041-8213/aa8f94
Schutz, B. F. (1986). Determining the Hubble constant from gravitational wave observations. Nature 323, 310. doi:10.1038/323310a0
Shapiro, S. L., and Teukolsky, S. A. (1983). Black holes, white dwarfs, and neutron stars. The Physics of Compact Objects. doi:10.1002/9783527617661
Shappee, B. J., Simon, J. D., Drout, M. R., Piro, A. L., Morrell, N., Prieto, J. L., et al. (2017). Early spectra of the gravitational wave source GW170817: evolution of a neutron star merger. Science 358, 1574. doi:10.1126/science.aaq0186
Shibagaki, S., Kajino, T., Mathews, G. J., Chiba, S., Nishimura, S., Lorusso, G., et al. (2016). Relative contributions of the weak, main, and fission-recycling r-process. Astrophys. J. 816, 79. doi:10.3847/0004-637X/816/2/79
Shibata, M., and Hotokezaka, K. (2019). Neutron star collisions and the r-process. Annu. Rev. Nucl. Part Sci. 69, 41. doi:10.1146/annurev-nucl-101918-023625
Shingles, L. J., Sim, S. A., Kromer, M., Maguire, K., Bulla, M., Collins, C., et al. (2020). Monte Carlo radiative transfer for the nebular phase of Type Ia supernovae. Mon. Not. Roy. Astron. Soc. 492, 2029. doi:10.1093/mnras/stz3412
Siegel, D. M., Barnes, J., and Metzger, B. D. (2019). Collapsars as a major source of r-process elements. Nature. 569, 241. doi:10.1038/s41586-019-1136-0
Simonetti, P., Matteucci, F., Greggio, L., and Cescutti, G. (2019). A new delay time distribution for merging neutron stars tested against Galactic and cosmic data. Mon. Not. Roy. Astron. Soc. 486, 2896. doi:10.1093/mnras/stz991
Smartt, S. J., Chen, T.-W., Jerkstrand, A., Coughlin, M., Kankare, E., Sim, S. A., et al. (2017). A kilonova as the electromagnetic counterpart to a gravitational-wave source. Nature. 551, 75. doi:10.1038/nature24303
Sneden, C., Cowan, J. J., and Gallino, R. (2008). Neutron-capture elements in the early galaxy. Annu. Rev. Astron. Astrophys. 46, 241. doi:10.1146/annurev.astro.46.060407.145207
Soares-Santos, M., Holz, D. E., Annis, J., Chornock, R., Herner, K., Berger, E., et al. (2017). The electromagnetic counterpart of the binary neutron star merger LIGO/Virgo GW170817. I. Discovery of the optical counterpart using the dark energy camera. Astrophys. J. 848, L16. doi:10.3847/2041-8213/aa9059
Stratta, G., Ciolfi, R., Amati, L., Bozzo, E., Ghirlanda, G., Maiorano, E., et al. (2018). Theseus: a key space mission concept for Multi-Messenger Astrophysics. Adv. Space Res. 62, 662. doi:10.1016/j.asr.2018.04.013
Symbalisty, E., and Schramm, D. N. (1982). Neutron star collisions and the r-process. Astrophys. Lett. 22, 143.
Takahashi, K., Witti, J., and Janka, H.-T. (1994). Nucleosynthesis in neutrino-driven winds from protoneutron stars II. The r-process. Astron. Astrophys. 286, 857.
Tanaka, M., and Hotokezaka, K. (2013). Radiative transfer simulations of neutron star merger ejecta. Astrophys. J. 775, 113. doi:10.1088/0004-637X/775/2/113
Tanaka, M., Utsumi, Y., Mazzali, P. A., Tominaga, N., Yoshida, M., Sekiguchi, Y., et al. (2017). Kilonova from post-merger ejecta as an optical and near-Infrared counterpart of GW170817. Publ. Astron. Soc. Jpn. 69, 102. doi:10.1093/pasj/psx121
Tanaka, M., Kato, D., Gaigalas, G., Rynkun, P., Radziute, L., Wanajo, S., et al. (2018). Properties of kilonovae from dynamical and post-merger ejecta of neutron star mergers. Astrophys. J. 852, 109. doi:10.3847/1538-4357/aaa0cb
Tanaka, M., Kato, D., Gaigalas, G., and Kawaguchi, K. (2020). Systematic opacity calculations for kilonovae. Mon. Not. Roy. Astron. Soc. 496, 1369. doi:10.1093/mnras/staa1576
Tanaka, M. (2016). Kilonova/Macronova emission from compact binary mergers. Advances in Astronomy, 634197. doi:10.1155/2016/6341974
Tanvir, N. R., Levan, A. J., Fruchter, A. S., Hjorth, J., Hounsell, R. A., Weirsema, K., et al. (2013). A “kilonova” associated with the short-duration γ-ray burst GRB 130603B. Nature. 500, 547. doi:10.1038/nature12505
Tanvir, N. R., Levan, A. J., Gonzalez-Fernandez, C., Korobkin, O., Mandel, I., Rosswog, S., et al. (2017). The emergence of a lanthanide-rich kilonova following the merger of two neutron stars. Astrophys. J. 848, L27. doi:10.3847/2041-8213/aa90b6
Tauris, M. T., Kramer, M., Freire, P. C. C., Wex, N., Janka, H.-T., Langer, N., et al. (2017). Formation of double neutron star systems. Astrophys. J. 846, 170. doi:10.3847/1538-4357/aa7e89
Taylor, J. H., and Weisberg, J. M. (1982). A new test of general relativity - gravitational radiation and the binary pulsar PSR 1913+16. Astrophys. J. 253, 908. doi:10.1086/159690
Thielemann, F.-K., Arcones, A., Kappeli, R., Liebendorfer, M., Rauscher, T., Winteler, C., et al. (2011). What are the astrophysical sites for the r-process and the production of heavy elements? Prog. Part. Nucl. Phys. 66, 346. doi:10.1016/j.ppnp.2011.01.032
Thielemann, F.-K., Eichler, M., Panov, I. V., and Wehmeyer, B. (2017). Neutron star mergers and nucleosynthesis of heavy elements. Annu. Rev. Nucl. Part Sci. 67, 253. doi:10.1146/annurev-nucl-101916-123246
Tominaga, N., Tanaka, M., Morokuma, T., Utsumi, Y., Yamaguchi, M. S., Yasuda, N., et al. (2018). Subaru hyper suprime-cam survey for an optical counterpart of GW170817. Publ. Astron. Soc. Jpn. 70, 28. doi:10.1093/pasj/psy007
Troja, E., Piro, L., van Eerten, H., Wollaeger, R. T., Im, M., Fox, O. D., et al. (2017). The X-ray counterpart to the gravitational-wave event GW170817. Nature 551, 71. doi:10.1038/nature24290
Troja, E., van Eerten, H., Zhang, B., Ryan, G., Piro, L., Ricci, R., et al. (Forthcoming 2020). A thousand days after the merger: continued X-ray emission from GW170817. Mon. Not. Roy. Astron. Soc Available at: https://arxiv.org/abs/2006.01150 (Accessed June 1, 2020).
Utsumi, Y., Tanaka, M., Tominaga, N., Yoshida, M., Barway, S., Nagayama, T., et al. (2017). J-GEM observations of an electromagnetic counterpart to the neutron star merger GW170817. Publ. Astron. Soc. Jpn. 69, 101. doi:10.1093/pasj/psx118
Valenti, S., David, J. S., Yang, S., Cappellaro, E., Tartaglia, L., Corsi, A., et al. (2017). The discovery of the electromagnetic counterpart of GW170817: kilonova at 2017gfo/DLT17ck. Astrophys. J. 848, L24. doi:10.3847/2041-8213/aa8edf
van Eerten, H. J., and MacFadyen, A. I. (2011). Synthetic off-axis light curves for low-energy gamma-ray bursts. Astrophys. J. 733, L37. doi:10.1088/2041-8205/733/2/L37
Villar, V. A., Guillochon, J., Berger, E., Metzger, B. D., Cowperthwaite, P. S., Nicholl, M., et al. (2017). The combined ultraviolet, optical, and near-infrared light curves of the kilonova associated with the binary neutron star merger GW170817: unified data set, analytic models, and physical implications. Astrophys. J. 851, L21. doi:10.3847/2041-8213/aa9c84
Villar, V. A., Cowperthwaite, P. S., Berger, E., Blanchard, P. K., Gomez, S., Alexander, K. D., et al. (2018). Spitzer space telescope infrared observations of the binary neutron star merger GW170817. Astrophys. J. 862, L11. doi:10.3847/2041-8213/aad281
Wallner, A., Faestermann, T., Feige, J., Feldstein, C., Knie, K., Korschinek, G., et al. (2015). Abundance of live Pu in deep-sea reservoirs on Earth points to rarity of actinide nucleosynthesis. Nat. Commun. 6, 5956. doi:10.1086/ncomms6956
Wanajo, S., Itoh, N., Ishimaru, Y., Nozawa, S., and Beers, T. C. (2002). The r-process in the neutrino winds of core-collapse supernovae and U-Th cosmochronology. Astrophys. J. 577, 853. doi:10.1086/342230
Wang, X.-G., Zhang, B., Liang, E.-W., Lu, R.-J., Lin, D.-B., Li, J., et al. (2018). Gamma-ray burst jet breaks revisited. Astrophys. J. 859, 160. doi:10.3847/1538-4357/aabc13
Watson, D., Hansen, C. J., Selsing, J., Koch, A., Malesani, D. B., Anderson, A. C., et al. (2019). Identification of strontium in the merger of two neutron stars. Nature 574, 497. doi:10.1038/s41586-019-1676-3
Waxman, E., Ofek, E. O., Doron, K., and Avishay, G.-Y. (2018). Constraints on the ejecta of the GW170817 neutron star merger from its electromagnetic emission. Mon. Not. Roy. Astron. Soc. 481, 3423. doi:10.1093/mnras/sty2441
Weisberg, J. M., and Huang, Y. (2016). Relativistic measurements from timing the binary pulsar PSR B1913+16. Astrophys. J. 829, 55. doi:10.3847/0004-637X/829/1/55
Wheeler, J. C., Cowan, J. J., and Hillebrandt, W. (1998). The r-process in collapsing O/Ne/Mg cores. Astrophys. J. 493, L101. doi:10.1086/311133
Winteler, C., Kappeli, R., Perego, A., Arcones, A., Vasset, N., Nishimura, N., et al. (2012). Magnetorotationally driven supernovae as the origin of early galaxy r-process elements? Astrophys. J. 750, L22. doi:10.1088/2041-8205/750/1/L22
Wollaeger, R. T., Korobkin, O., Fontes, C. J., Rosswog, S. K., Even, W. P., Fryer, C. L., et al. (2018). Impact of ejecta morphology and composition on the electromagnetic signatures of neutron star mergers. Mon. Not. Roy. Astron. Soc. 478, 3298. doi:10.1093/mnras/sty1018
Wolszczan, A. (1991). A nearby 37.9-ms radio pulsar in a relativistic binary system. Nature 350, 688. doi:10.1038/350688a0
Woosley, S. E., and Bloom, J. S. (2006). The supernova gamma-ray burst connection. Annu. Rev. Astron. Astrophys. 44, 507. doi:10.1146/annurev.astro.43.072103.150558
Woosley, S. E., Wilson, J. R., Mathews, G. J., Hoffman, R. D., and Meyer, B. S. (1994). The r-process and neutrino-heated supernova ejecta. Astrophys. J. 433, 229. doi:10.1086/174638
Xie, X., Zrake, J., and MacFadyen, A. (2018). Numerical simulations of the jet dynamics and synchrotron radiation of binary neutron star merger event GW170817/GRB 170817A. Astrophys. J. 863, 58. doi:10.3847/1538-4357/aacf9c
Yang, S., Valenti, S., Cappellaro, E., Sand, D. J., Tartaglia, L., Corsi, A., et al. (2017). An empirical limit on the kilonova rate from the DLT40 one day cadence supernova survey. Astrophys. J. 851, L48. doi:10.3847/2041-8213/aaa07d
Yaron, O., and Gal-Yam, A. (2012). WISeREP—an interactive supernova data repository. Publ. Astr. Soc. Pacific 124, 668. doi:10.1086/666656
Keywords: gamma-ray burst, gravitational waves, neutron star, nucleosynthesis, r-process, kilonova
Citation: Pian E (2021) Mergers of Binary Neutron Star Systems: A Multimessenger Revolution. Front. Astron. Space Sci. 7:609460. doi: 10.3389/fspas.2020.609460
Received: 23 September 2020; Accepted: 02 December 2020;
Published: 25 January 2021.
Edited by:
Livio Lamia, University of Catania, ItalyReviewed by:
Toshitaka Kajino, The University of Tokyo, JapanCopyright © 2021 Pian. This is an open-access article distributed under the terms of the Creative Commons Attribution License (CC BY). The use, distribution or reproduction in other forums is permitted, provided the original author(s) and the copyright owner(s) are credited and that the original publication in this journal is cited, in accordance with accepted academic practice. No use, distribution or reproduction is permitted which does not comply with these terms.
*Correspondence: Elena Pian, ZWxlbmEucGlhbkBpbmFmLml0
Disclaimer: All claims expressed in this article are solely those of the authors and do not necessarily represent those of their affiliated organizations, or those of the publisher, the editors and the reviewers. Any product that may be evaluated in this article or claim that may be made by its manufacturer is not guaranteed or endorsed by the publisher.
Research integrity at Frontiers
Learn more about the work of our research integrity team to safeguard the quality of each article we publish.