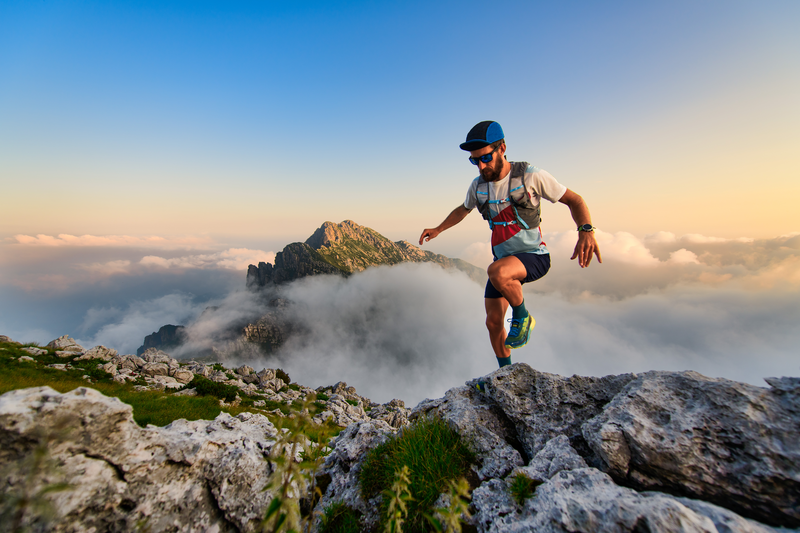
94% of researchers rate our articles as excellent or good
Learn more about the work of our research integrity team to safeguard the quality of each article we publish.
Find out more
SYSTEMATIC REVIEW article
Front. Arachn. Sci.
Sec. Arachnid Morphology, Systematics and Evolution
Volume 4 - 2025 | doi: 10.3389/frchs.2025.1544428
This article is part of the Research Topic Horizons in Arachnid Science View all 7 articles
The final, formatted version of the article will be published soon.
You have multiple emails registered with Frontiers:
Please enter your email address:
If you already have an account, please login
You don't have a Frontiers account ? You can register here
Kleptoparasitism, broadly defined, is the theft of extrinsic resources resulting in potential cost to the host. The stealing of resources, often food gathered by another, is perhaps best known in birds and mammals, but is even more common and widespread in arthropods like ants, bees, flies and spiders. Spiders are involved in myriad kleptoparasitic interactions, perhaps best studied as obligatory kleptoparasites of other spiders. However, less attention has been paid to the critical role of spiders as "superhosts" to commensal and kleptoparasitic organisms, and their variety of facultative kleptoparasitic strategies. To understand obligatory kleptoparasitism in spiders, it is first necessary to examine their role and characteristics as hosts and as facultative kleptoparasites. Most spider kleptoparasites utilize other spiders as hosts, a link that is not coincidental, and facultative resource stealing, in its many forms, is generally assumed to provide an evolutionary bridge to obligate kleptoparasitism. Here, I provide a brief review of these two roles through a summary of literature on all kleptoparasitic spiders and over 200 hosts. The phylogenetic distribution of spider hosts is distinctly non-random, involving about 200 species, in 86 genera, and 23 families (Table 1). The vast majority of argyrodine hosts belong to four Araneoidea families (Araneidae, Nephilidae, Theridiidae, Linyphiidae), while the majority of hosts of mysmenid kleptoparasites are mygalomorphs, mostly Dipluridae and Ischnothelidae. Key spider hosts like Nephila, Trichonephila, Argiope, Cyrtophora, and Linothele, build large, often structurally complex, and persistent webs. Three-dimensionality, often in the form of auxiliary webbing, provides safe refuges for kleptoparasites, and the abundant prey and extended prey handing time of large spiders provide resources and opportunities for theft. Many of the favored hosts either interlink webs or are social. Key host traits to counter kleptoparasitism include web takedown and relocation, food concealment, and direct aggression. Facultative resource stealing in spiders includes web takeover, male kleptoparasitism of females in webs, and opportunistic prey theft. Among these, kleptotany, the facultative abandonment of own web and the invasion of a larger host web, to steal it and/or to prey on the host (araneophagy) are the most likely to link to obligatory kleptoparasitism.
Keywords: Klepto parasitism, commensal, Theridiidae, Spider webs, Kleptotany
Received: 12 Dec 2024; Accepted: 19 Feb 2025.
Copyright: © 2025 Agnarsson. This is an open-access article distributed under the terms of the Creative Commons Attribution License (CC BY). The use, distribution or reproduction in other forums is permitted, provided the original author(s) or licensor are credited and that the original publication in this journal is cited, in accordance with accepted academic practice. No use, distribution or reproduction is permitted which does not comply with these terms.
* Correspondence:
Ingi Agnarsson, Biology, University of Iceland, Reykjavik, Iceland
Disclaimer: All claims expressed in this article are solely those of the authors and do not necessarily represent those of their affiliated organizations, or those of the publisher, the editors and the reviewers. Any product that may be evaluated in this article or claim that may be made by its manufacturer is not guaranteed or endorsed by the publisher.
Research integrity at Frontiers
Learn more about the work of our research integrity team to safeguard the quality of each article we publish.