- 1Department of Organisms and Ecosystems Research, National Institute of Biology, Ljubljana, Slovenia
- 2Department of Biology, Biotechnical Faculty, University of Ljubljana, Ljubljana, Slovenia
- 3Te Aka Mātuatua School of Science, University of Waikato, Hamilton, New Zealand
- 4School of Biological Sciences, University of Nebraska-Lincoln, Lincoln, NE, United States
- 5Department of Pest Management and Conservation, Lincoln University, Lincoln, New Zealand
- 6School of Mathematical and Natural Sciences, New College of Interdisciplinary Arts and Sciences, Arizona State University, Phoenix, AZ, United States
- 7Research Centre of the Slovenian Academy of Sciences and Arts, Jovan Hadži Institute of Biology, Ljubljana, Slovenia
- 8Faculty of Mathematics, Natural Sciences and Information Technologies, University of Primorska, Koper, Slovenia
- 9Department of Entomology, National Museum of Natural History, Smithsonian Institution, Washington, DC, United States
- 10State Key Laboratory of Biocatalysis and Enzyme Engineering, and Centre for Behavioral Ecology and Evolution, School of Life Sciences, Hubei University, Wuhan, Hubei, China
- 11Te Pūnaha Matatini, Centre of Research Excellence, Auckland, New Zealand
Dolomedes may easily be considered to be among the most charismatic spider taxa. Known colloquially as fishing or raft spiders, this clade of dolomedid cursorial hunters is speciose with about 100 valid species names. Most Dolomedes are large spiders that inhabit water bodies across all continents except Antarctica and, interestingly, South America. Dolomedes have captured the attention of researchers and the public alike for their ability to walk on and submerge under water, fish for prey (including small vertebrates), and for their often-bizarre mating behavior that includes examples of male spontaneous death and sexual cannibalism. In this review, we critically evaluate what is known of Dolomedes biology, focusing on their systematics and morphology, ecology, behavior, and conservation. Given their close association with water, Dolomedes may be particularly vulnerable to the impacts of anthropogenic change and provide an important group of indicator species for understanding the effect of pollution, habitat loss and climate change. We outline a roadmap for future studies that, in our view, will consolidate Dolomedes as an ideal model lineage among spiders for addressing a vast array of questions across multiple fields of biology.
1 Introduction
Spiders are estimated to kill a staggering 400–800 million tons of prey per year globally and serve as generalist predators in terrestrial ecosystems where they contribute significantly to ecosystem function (Nyffeler and Birkhofer, 2017; Michalko et al., 2019). Spiders also comprise important food sources for reptiles (e.g., James, 1991; Manicom and Schwarzkopf, 2011), birds (e.g., Gunnarsson, 2007; Pagani-Núñez et al., 2011), and mammals (e.g., Schulz, 2000; Alves-Costa et al., 2004). In a world increasingly impacted by anthropogenic change, spiders can act as environmental indicators due to their sensitivity to habitat changes and pollution (Pearson, 1994; Milano et al., 2021). Ultimately, the diversity, biomass, and abundance of spiders can reflect ecosystem stability and condition (Büchs, 2003; Oxbrough et al., 2005; Buchholz, 2010).
Although freshwater wetlands cover only 1% of the earth’s surface, they are important biomes that harbor more than 40% of global biodiversity (Mitra et al., 2003). A group of spiders that inhabits freshwater bodies and terrestrial habitats is Dolomedes Latreille, 1804, commonly known as fishing or raft spiders (Figure 1), a genus comprising over a hundred species found across most continents (Figure 2). Behaviorally and morphologically, Dolomedes provide unique opportunities to explore evolutionary adaptations to life on and near water (e.g., waterborne locomotion, diving and ‘fishing’ behavior, etc.). Dolomedes have also been the subject of numerous behavioral studies that place them in the center of theoretical and empirical research aimed at interrogating evolutionary puzzles such as the evolution of extreme mating behaviors including sexual cannibalism and spontaneous male death, and mating system diversification. The limited observations of reproductive behavior across the genus provide a snapshot of intriguing species-specific variability in sexual cannibalism, female and male mating rates, and more. As species-specific studies accumulate, it is important to synthesize them in a manner that facilitates the recognition of patterns and that enables the testing of general hypotheses. We aim to provide just such a synthesis.
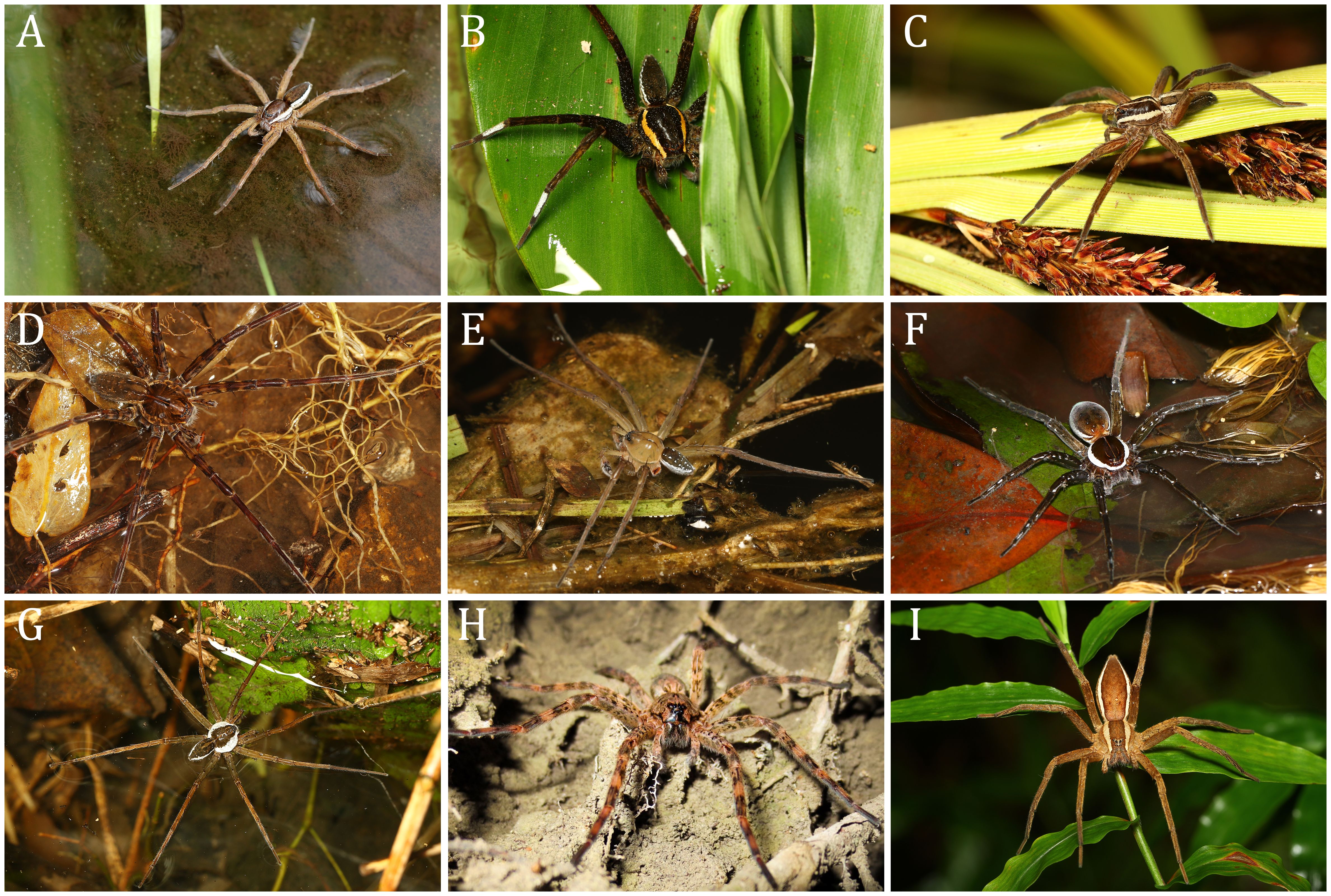
Figure 1. A glimpse into diversity of fishing spiders, genus Dolomedes Latreille, 1804 in their natural environments, except H: (A) male D. fimbriatus (Clerck, 1757); (B) female D. raptor Bösenberg and Strand, 1906; (C) female D. minor L. Koch, 1876; (D) female D. bedjanic Yu and Kuntner, 2024; (E) male of an undescribed Dolomedes species from Madagascar; (F) female D. horishanus Kishida, 1936; (G) male D. mizhoanus Kishida, 1936; (H) female D. tenebrosus Hentz, 1844; (I) female D. sulfureus L. Koch, 1878.
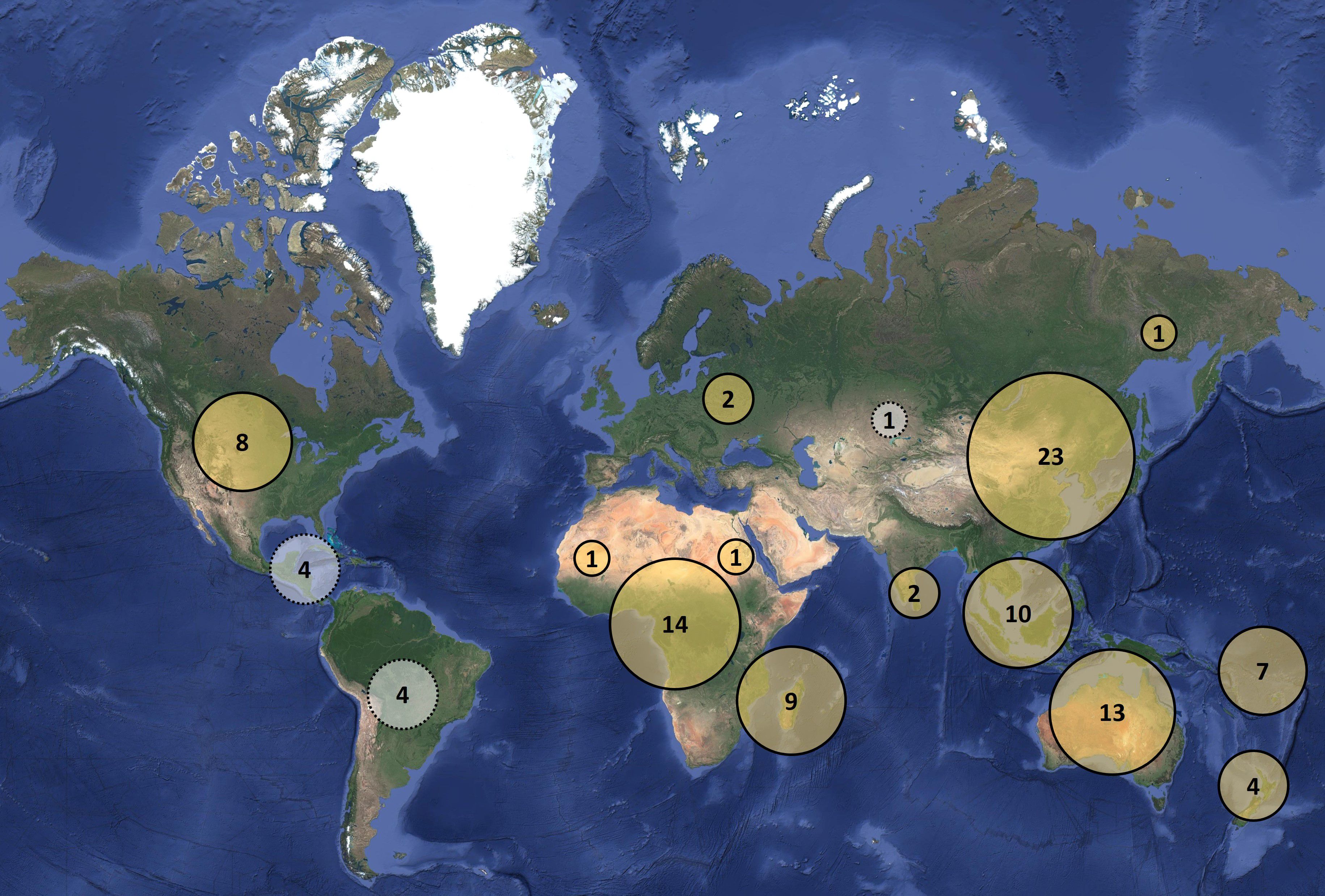
Figure 2. Contemporary distribution pattern of the known Dolomedes species. Each number represents the number of valid species in the region; yellow circles with black borders represent regions with confirmed Dolomedes species; gray circles with dotted line borders denote regions with historic, but doubtful, Dolomedes species records.
Our authorship team encompasses a group of researchers with diverse interests in Dolomedes and thus with distinct knowledge and expertise. Through new and ongoing collaborations, we are surprised by just how frequently Dolomedes has featured in studies across a wide range of biological fields. We have each appreciated how knowledge gained in one field of study may be directly or indirectly connected to our own area of research. Additionally, we have identified areas of research for which Dolomedes are particularly well suited. Given the expanding literature around various aspects of Dolomedes biology and the exciting opportunities for future conceptual contributions, a review of Dolomedes biology is not only timely, but also necessary.
Over the last 30 years, much of the research on Dolomedes has focused on their behavior and ecology, with a resurgence in systematics and morphology work in the early 2000s (Figure 3A). The number of publications on Dolomedes has steadily increased over time, although largely dominated by studies coming out of Europe and North America (Figure 3B). The few studies from Oceania are all from New Zealand, with a clear gap in research from Australia, and a similar lack of research in Africa.
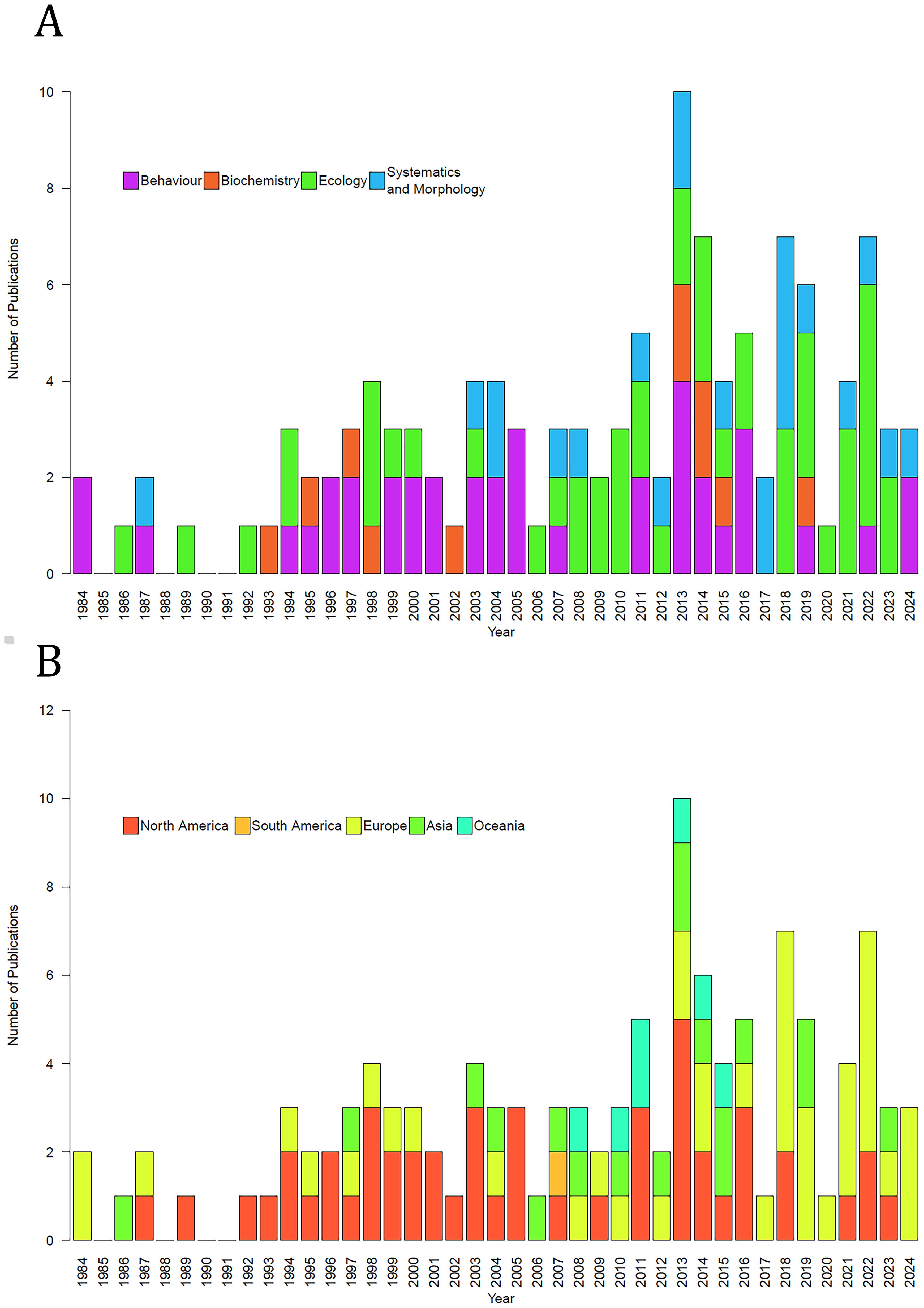
Figure 3. Number of Dolomedes related research by years, from 1964 to present; with color codon highlighting different (A) research fields and (B) continents where the research was carried out.
Ray and Lyn Forster, the acclaimed New Zealand arachnologists, capture the beauty of Dolomedes with their quote “She is a magnificent creature whose body seems clothed with the finest velvet” (Forster and Forster, 1973, page 95). While we agree with the Forsters, wider society tends to lack appreciation of spiders, where arachnophobia (Gerdes et al., 2009) and misinformation (Mammola et al., 2022) abound. Mainstream media stories on Dolomedes tend to use hyperbole, focusing on their ability to walk on water and capture fish as prey – not necessarily in a positive light (“horrifying” and “creepiest” are among the sensationalist headings). However, given these large spiders have an ability to engage public audiences they have significant storytelling potential if we can craft narratives of their natural history and ecological importance that move beyond negative clickbait.
The aim of this review is to synthesize the current state of knowledge on the biology of Dolomedes. The manuscript composes four key sections. We begin by first asking the question – what is a Dolomedes? – and answer this by reviewing the systematics and morphology of the genus, which has recently been reclassified in the family Dolomedidae Simon, 1876. Second, we explore the ecology of Dolomedes, including their habitat use, phenology, predators and parasitoids, and conservation. Thirdly, we delve into Dolomedes behavior, ranging from sensory adaptations to their predatory, reproductive and parental behavior. Lastly, we shine a spotlight on the many mysteries still surrounding this spider genus and offer a plethora of future avenues to explore. Our aspiration is for the review to become the go-to guide for researchers interested in this captivating group of arachnids.
2 Systematics and morphology
2.1 Taxonomic history and diversity
Dolomedes is a diverse genus containing 105 species worldwide (World Spider Catalog, 2024). With Clerck’s (1757) descriptions of “Araneus fimbriatus Clerck, 1757” and “A. plantarius Clerck, 1757” the earliest taxonomic discovery of any species of Dolomedes predates Linnaeus’ system of nomenclature by a year. The genus name Dolomedes dates back to Latreille (1804) who established it for the “wolf spiders” with a second eye arrangement differing from Lycosa Latreille, 1804. According to Latreille (1804), Dolomedes was equivalent to “Les coureuses” of Walckenaer (1802), a group of spiders that contained “Araneus mirabilis Clerck, 1757” = Pisaura mirabilis (Clerck, 1757) and “Aranea marginata De Geer, 1778” = Dolomedes fimbriatus (Clerck, 1757). Although not specified in Latreille’s catalogue, it is generally believed that D. fimbriatus is the type species of Dolomedes.
Subsequent authors have described new Dolomedes species in a non-linear fashion (Figure 4A). Two bursts of taxonomic discovery in Dolomedes are evident, one between 1850 and 1950, and another from 2000 onwards. The leading taxonomists are Carl Friedrich Roewer (Roewer, 1955), Robert J. Raven, and Wendy Hebron (Raven and Hebron, 2018). The result of taxonomic discoveries is that Dolomedes species diversity is well documented in North America, Europe, Japan, Australia, and New Zealand, but remains poorly known in regions like Africa and Southeast Asia (Figure 4B). Thus, despite recent species discovery, many unknown pockets of species diversity are likely yet to be uncovered, particularly in the Old-World tropics (Yu and Kuntner, 2024). Depending on location, Dolomedes are commonly referred to as fishing or raft spiders. Herein, we use the colloquial names suggested in Yu et al. (2024), where the dolomedid family are the raft spiders, while Dolomedes specifically are called fishing spiders.
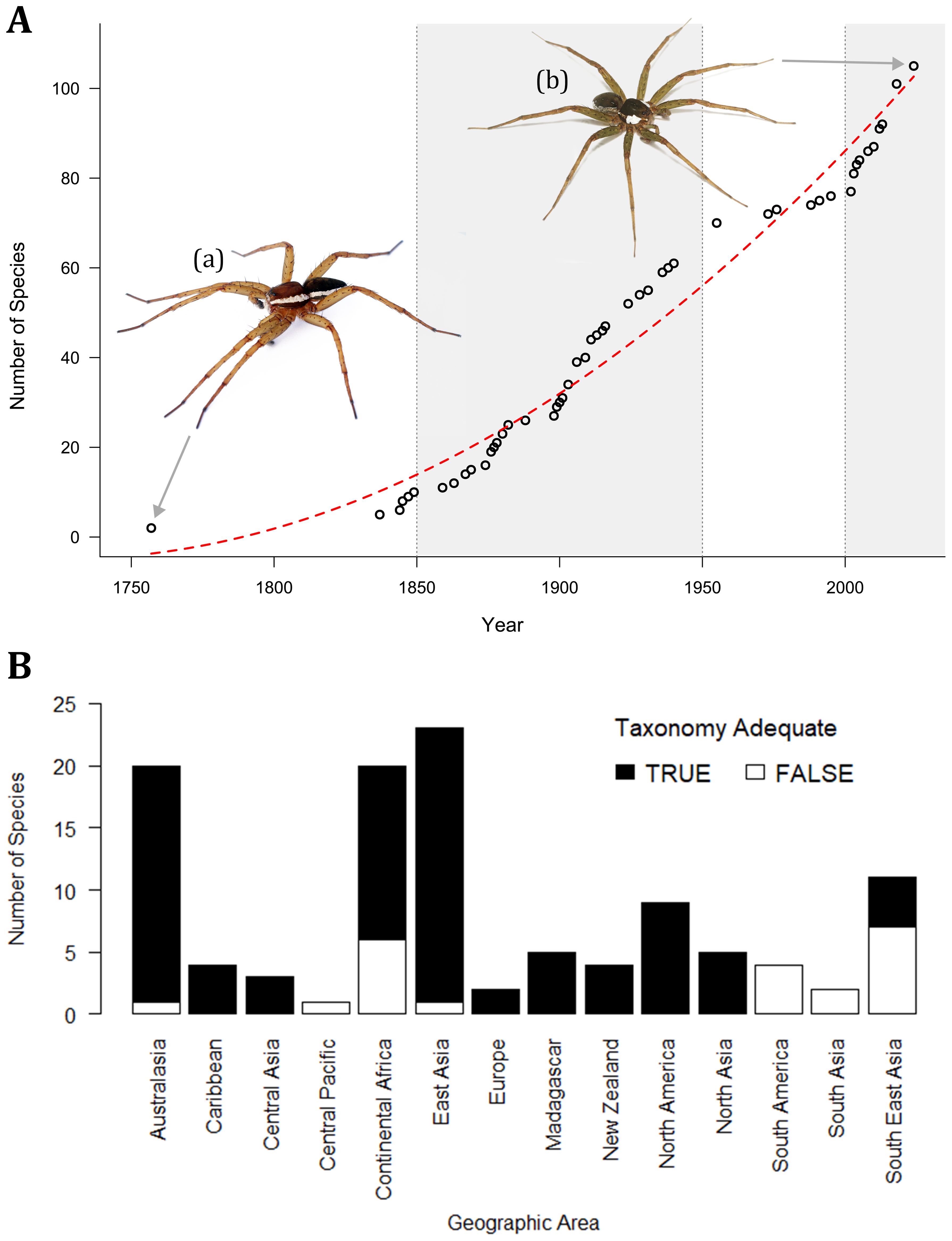
Figure 4. History and pace of Dolomedes species discovery (A) as well as spatial pattern of Dolomedes taxonomic adequacy for species identification among geographic regions (B). Open circles represent cumulative number of valid species from 1750 to the present (World Spider Catalog, 2024); red line represents the general trend of increasing known species diversity; grey areas highlight two major bursts of taxonomic discovery. (A) D. fimbriatus, the first described Dolomedes species; (B) D. rotundus Yu and Kuntner, 2024, currently the latest described Dolomedes species.
2.2 General morphology
For a species-rich genus, the morphology of Dolomedes is remarkably conserved. Dolomedes has a carapace that is longer than wide (Supplementary Figure S1A) with the posterior half slightly higher than the eye region (Supplementary Figure S1B). Dolomedes have eight eyes in two rows, with the posterior eye row strongly recurved while the anterior row is straight or weakly re/procurved (Supplementary Figure S1C). The posterior lateral eyes are fully separated from the anterior lateral eyes (Supplementary Figure S1C). The abdomen of Dolomedes is oval with no modifications. Dolomedes legs are prograde and usually unmodified (but see Supplementary Figure S2). The fourth leg is the longest, followed by the second or the first leg while the third leg is the shortest.
Dolomedes are well known for their iconic body coloration with distinct white lateral bands, patches, or spots on carapace and/or abdomen over a dark background. This color pattern is uniform in some species (e.g., D. mizhoanus Kishida, 1936, D. hydatostella Yu and Kuntner, 2024, D. rotundus Yu and Kuntner, 2024) but can also show intraspecific variation in females (e.g., D. raptor Bösenberg and Strand, 1906, and D. horishanus Kishida, 1936) or in both sexes (e.g., D. fimbriatus, D. plantarius (Clerck, 1757), D. kalanoro Silva and Griswold, 2013, D. pegasus Tanikawa, 2012, and D. sulfureus L. Koch, 1878; Supplementary Figure S3) (Tanikawa, 2003, Tanikawa, 2012; Tanikawa and Miyashita, 2008; Baillie et al., 2019; Serita, 2019; Yu and Kuntner, 2024). Several species uniformly lack this typical Dolomedes coloration in both sexes (e.g., D. bedjanic Yu and Kuntner, 2024). In D. sulfureus, three coloration morphs are known (Supplementary Figure S3; after Tanikawa and Miyashita, 2008). Although the function of the typical Dolomedes white lateral bands is unexplored, studies in D. raptor have linked them to foraging (Lin et al., 2015; Tso et al., 2016) (see Diet & Predation Behavior) and male mating success (Lin et al., 2015) (see Reproductive Behavior). The mechanism(s) behind Dolomedes color variation has only been studied in D. plantarius. Baillie et al. (2019) investigated the proportion of banded and non-banded offspring from 47 broods with their parents of different color phenotypes. They showed that presence/absence of white lateral bands was controlled by a single-gene system where the banded allele was dominant.
All Dolomedes species exhibit moderate female-biased sexual size dimorphism (SSD) with the ratio of female to male linear size (= SSD) between 1.00 and 1.88 (see Supplementary Table S1). Exceptions are D. tenebrosus Hentz, 1844, D. okefinokensis Bishop, 1924, and D. raptoroides Zhang et al., 2004 with SSD ratios 2.40, 2.46, and 2.46, respectively (Carico, 1973; Zhang et al., 2004; Silva et al., 2015). The extreme SSD (eSSD; Kuntner and Coddington, 2020) in these species may be indicative of phenotypic adaptations in males that relate to reproduction (see Reproductive Behavior).
2.3 Reproductive morphology
In spiders, genital anatomy provides critical taxonomic evidence to define species boundaries (Eberhard and Huber, 2010; Foelix, 2010). However, genital anatomy is conserved across Dolomedes, providing only limited species diagnostics. As the degrees of intraspecific variation vary in different groups of Dolomedes, combinations of diagnostic characteristics change even among closely related species (Yu and Kuntner, 2024). In this section, we describe the general anatomy of female epigynum and male pedipalp partially following Sierwald’s (1989, 1990) nomenclature. However, our knowledge of the precise interactions of anatomical parts of male and female genitals is currently too preliminary to allow speculation of their precise reproductive function.
The female epigyne of Dolomedes is either round, triangular, or pentagonal, but some Australian species have lateral extensions (Raven and Hebron, 2018). The epigyne is highly sclerotized and separated into two lateral lobes by the middle field with usually two membranous windows (Figure 5A). The median field windows in some species (e.g., D. plantarius) merge into a larger transparent part while in others (e.g., D. scriptus Hentz, 1845) are small and indistinct. Some species from Africa (D. actaeon Pocock, 1903 and D. straeleni Roewer, 1955), Madagascar (D. kalanoro), and Australia (e.g., D. briangreenei Raven and Hebron, 2018) have one or two ventral protrusions on their median field (Roewer, 1955; Raven and Hebron, 2018; Yu and Kuntner, 2024). The margins of the median field and lateral lobes together form two longitudinal epigynal folds that posteriorly lead to the copulatory openings (Figures 5A–C). A looped copulatory duct inserts into a spermathecal base, which connects to a small knob-, horn-, or bulb-shaped head of spermatheca via an indistinct stem. The remainder of spermathecal bases are long, curved, or spiraled, ending with short and flat fertilization ducts (Figures 5B–D). Two species, D. tenebrosus and D. okefinokensis have unique epigyna (Carico and Holt, 1964) with their median fields lacking membranous windows and their copulatory openings distinctly wider (Figure 5E) (Carico, 1973; Sierwald, 1989). However, their vulvae nonetheless share the common Dolomedes gestalt (Figure 5F; see Carico, 1973; Sierwald, 1989). Considering that D. tenebrosus and D. okefinokensis are both eSSD, their unique epigynal anatomy could determine their mating behavior (Schwartz et al., 2013; see also Reproductive Behavior).
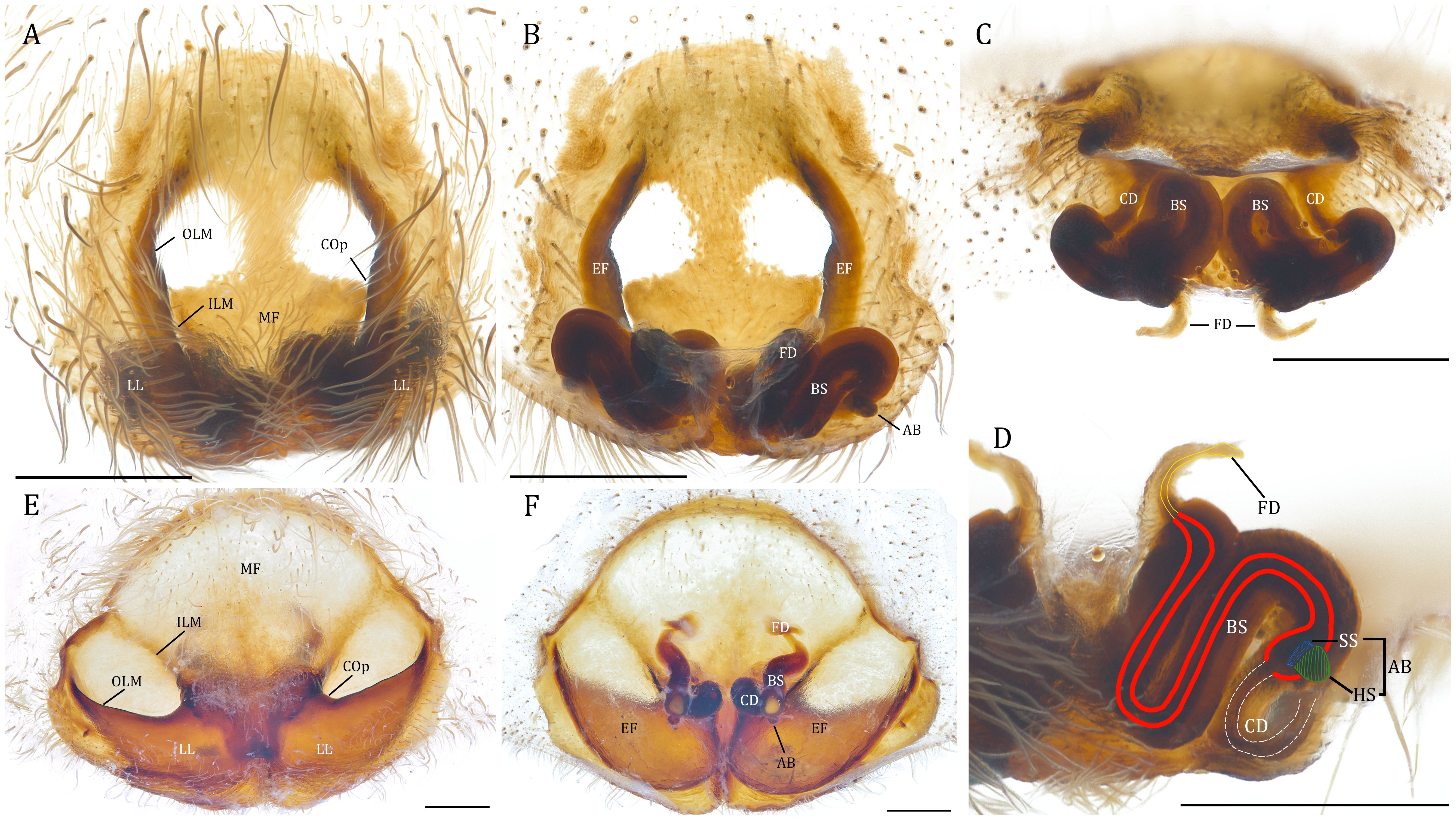
Figure 5. (A–D), Female genitalia of Dolomedes fimbriatus, typical of Dolomedes: (A) epigyne, ventral view; (B) idem, dorsal view; (C) vulva, anterior view; (D) idem, posterior view with anatomic structures highlighted in colors: white dot lines, copulatory duct; red bold lines, base of spermatheca; blue dotted region, stem of spermatheca; green lined region, accessory bulb; yellow line, fertilization duct. (E, F), Female genital anatomy of Dolomedes tenebrosus Hentz, 1844, representing a unique genital morphology among Dolomedes: (E) epigyne, ventral view; (F) idem, dorsal view. AB, accessory bulb; BS, base of spermatheca; CD, copulatory duct; COp, copulatory opening; EF, epigynal fold; FD, fertilization duct; HS, head of spermatheca; ILM, interior margin of epigynal fold; LL, lateral lobe; MF, middle field; OLM, outer lateral margin of epigynal fold; SS, stem of spermathecae. Scale bars: 0.5 mm.
The Dolomedes male pedipalp has a U-shaped tegular ring consisting of the tegulum, its distal projection, and a membranous conductor (Figures 6A–C). Unlike pisaurids, Dolomedes does not have a distal tegular apophysis; instead, a round, sclerotized saddle sits at the lower center of the tegular ring and connects the tegulum and the subtegulum (Figure 6B). Retrolateral to the saddle sits the highly sclerotized median apophysis which can be hooked (but see D. tenebrosus and D. okefinokensis; Carico, 1973). A distal sclerotized tube of the apical division that attaches to the embolus, fulcrum, and the lateral subterminal apophysis is a dolomedid feature (Figure 6D; Sierwald, 1990; Yu et al., 2024). Dolomedes can be separated from other dolomedids by the embolus with one simple circular or semi-circular loop (but see D. bistylus Roewer, 1955) that neither passes the dorsal part of the palp nor extends to the tip of the cymbium (Raven and Hebron, 2018; Yu and Kuntner, 2024). Dolomedes palps feature an oval or triangular basal cymbial apophysis. Palpal tibia has a ventral and a retrolateral apophysis (Figures 6A–C). The former is highly conserved across the genus while the latter readily distinguishes species (but, see D. minor L. Koch, 1876: Vink and Dupérré, 2010; and D. tenebrosus: Carico, 1973). Lengths of the papal tibia and the whole palp can additionally diagnose species (Tanikawa and Miyashita, 2008; Yu and Kuntner, 2024). When expanded, the left palpal organ rotates clockwise in ventral view. In D. tenebrosus the distal sclerotized tube rotates to the position between the tibia and the retrolateral tibial apophysis during mating (Sierwald and Coddington, 1988).
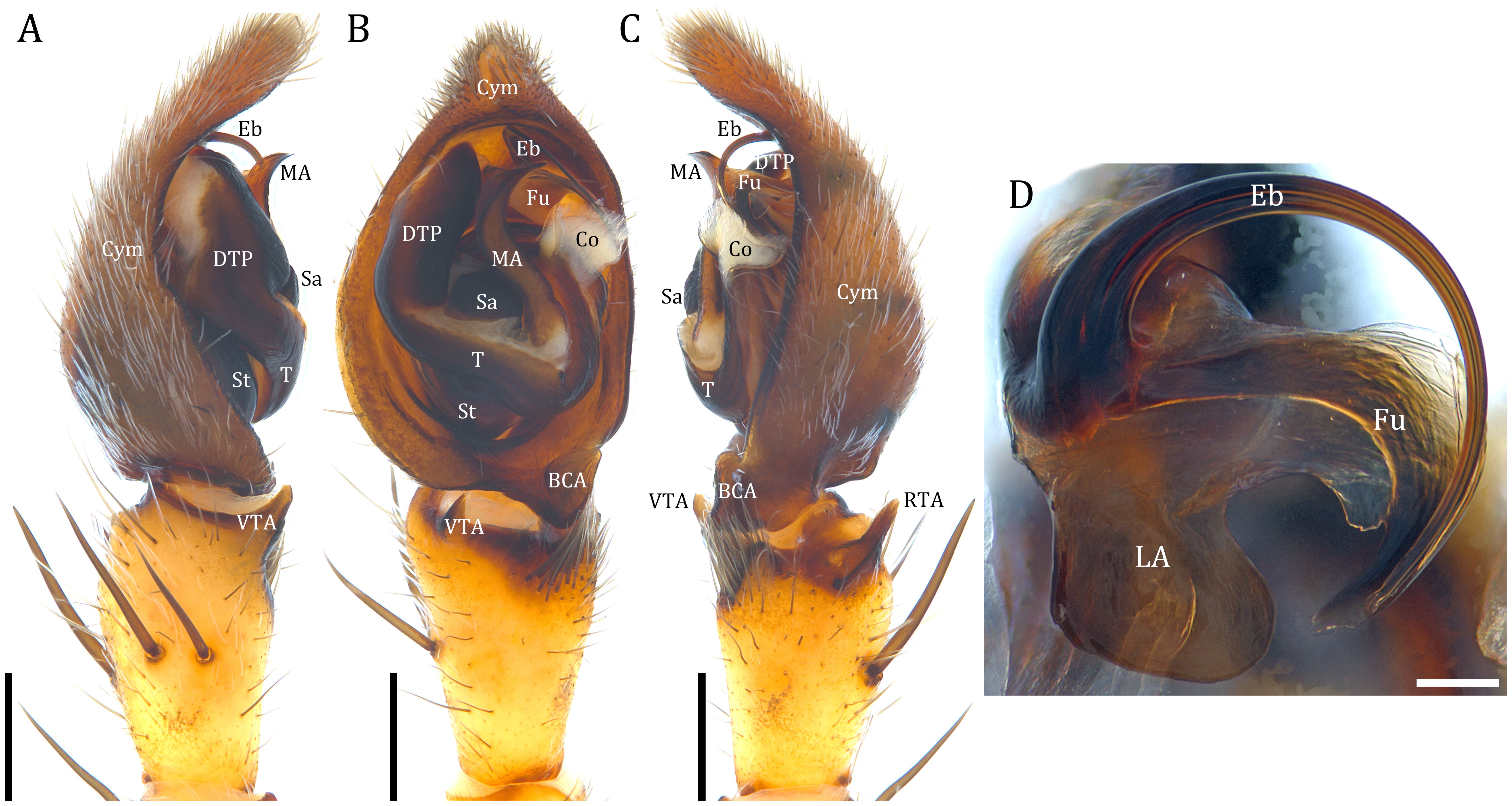
Figure 6. Male pedipalp of Dolomedes fimbriatus, typical of Dolomedes: (A) left palp, prolateral view; (B) idem, ventral view; (C) idem, retrolateral view; (D) distal sclerotized tube of the apical division of the expanded right palp. BCA, basal cymbium apophysis; Co, conductor; Cym, cymbium; DTP, distal tegular projection; Eb, embolus; Fu, fulcrum; LA, lateral subterminal apophysis; MA, median apophysis; RTA, retrolateral tibial apophysis; Sa, saddle; St, subtegulum; T, tegulum; VTA, ventral tibial apophysis. Scale bars: A–C: 0.5 mm, D: 0.1 mm.
2.4 Phylogeny, evolution and genomics
Although Dolomedes has been traditionally classified in Pisauridae Simon, 1890 (World Spider Catalog, 2024), these clades are not each other’s closest relatives. Instead, Dolomedes with related Oceanian genera has now been reclassified in Dolomedidae (Yu et al., 2024). In a pre-phylogenetic argumentation, Lehtinen (1967) already proposed the use of Dolomedidae, however, the family status for the clade has not been generally accepted due to conflicting topologies (Sierwald, 1990; Griswold, 1993; Zhang et al., 2004; Santos, 2007; Wheeler et al., 2017; Piacentini and Ramírez, 2019) but has recently regained phylogenetic attention (Albo et al., 2017; Hazzi and Hormiga, 2023; Kulkarni et al., 2023). Dolomedidae is now supported through a phylogenomic analysis of over half of Dolomedes species and pisaurid genera (Yu et al., 2024). This phylogeny vastly expands the prior understanding of phylogenetic relationships among Dolomedes species that has been limited to regional analyses focused on New Zealand (Vink and Dupérré, 2010), Japan (Ono, 2002; Tanikawa, 2003, Tanikawa, 2012; Tanikawa and Miyashita, 2008), and Madagascar (Yu and Kuntner, 2024). Currently, no fossil Dolomedes are known (Wunderlich, 2008; Magalhaes et al., 2020).
Among the most well-known semi-aquatic spiders, Dolomedes species are common model organisms in many study fields (see sections below). However, incomplete and conflicting Dolomedes phylogenies (see citations above) have hampered further studying the evolution of their remarkable lifestyles and related traits until very recently. Based on the phylogenomic data of more than half of the pisaurids genera and Dolomedes species, Yu et al. (2024) investigate the evolutionary shifts of lifestyles and the presence of a capture web, as well as the morphological traits accompanying a semi-aquatic lifestyle. Their results suggest that Dolomedes and dolomedids are ancestrally semi-aquatic with several independent reversals to a terrestrial lifestyle (see also Microhabitat use and preference and Locomotion & Dispersal); and ancestrally lacking a capture web without any reversals. Yu et al. (2024) also found that Dolomedes and other semi-aquatic dolomedid and pisaurid genera have wider carapaces than the terrestrial genera but with no differences in their legs. They proposed that semi-aquatic spiders need to be large enough to break through the water surface tension to forage under water.
A reference genome is currently available only for D. plantarius (GenBank GCA_907164885.2). At 2.8 Gb, its size is among the largest sequenced arachnid genomes (reviewed in Kuntner, 2022). The complete mitochondrial genome of D. angustivirgatus Kishida, 1933 has been sequenced with gene arrangement typical of mitochondrial genomes of Entelegynae spiders (Wang et al., 2020). Ten polymorphic microsatellite DNA loci were developed for D. plantarius for use in paternity studies and for analysis of population genetics (Ji et al., 2004). The newly available subgenomic data with ultraconserved elements of Dolomedes worldwide (Yu et al., 2024) will be useful, beyond phylogenomics, in efforts to generate new sets of microsatellites (Raposo do Amaral et al., 2015).
2.5 Biogeography
Extant Dolomedes species are distributed globally (Figure 2). It is noteworthy, however, that South America seems to lack any Dolomedes diversity (the few catalogued names are ambiguous or refer to other spider groups), making it the only major continent, in addition to Antarctica, that is thought to lack Dolomedes. Furthermore, while some species such as D. triton (Walckenaer, 1837), D. fimbriatus, and D. plantarius are widespread across continents, others such as D. orion Tanikawa, 2003 (Okinawa Island) and D. schauinslandi Simon, 1899 (Chatham Island archipelago) are narrow island endemics.
Dolomedes is a relatively distal clade on the spider tree of life (Wheeler et al., 2017; Kulkarni et al., 2023). The origin of the genus is hypothesized in the Cenozoic, between 16 and 9 (mid-Miocene) million years ago (Yu et al., 2024). This relatively recent origin of Dolomedes implies that climate oscillations in the Cenozoic (Zachos et al., 2001) may have driven its diversification. Considering that the current distribution patterns of Dolomedes include most continents, glacial cycles and land bridges might also have shaped their distribution patterns. Although the origin and the biogeographic history of Dolomedes have not been directly tested, preliminary hypotheses can be derived. Considering i) the known sister relationship with the New Caledonian Bradystichus Simon, 1884 (Wheeler et al., 2017; Piacentini and Ramírez, 2019; Kulkarni et al., 2023); ii) the monophyly of Dolomedidae containing Dolomedes and Australian relatives (Raven and Hebron, 2018; Yu et al., 2024); and iii) the highest Dolomedes contemporary species richness in East Asia, one can hypothesize that Dolomedes might have originated from either Australasia or Eastern Eurasia.
3 Ecology
3.1 Microhabitat use and preference
Dolomedes inhabit most freshwater-related habitats, each species preferring specific microhabitats with varying flexibility (Figure 7) (Carico, 1973; Jordan et al., 1994; Tanikawa and Miyashita, 2008; Ono, 2009; Vink and Dupérré, 2010; Dickel et al., 2022; Yu and Kuntner, 2024). In regions where multiple species cohabit, they distinctly differentiate their habitat usage (Carico, 1973; Vink and Dupérré, 2010; Dickel et al., 2022). By summarizing the literature, the major differences in habitat preferences among cohabiting Dolomedes are: 1) vegetation structure near and above water bodies, 2) velocity and depth of water bodies, and 3) distance to the water bodies. Aside from picking different aquatic microhabitats, we found at least eight species that do not engage water bodies frequently and can inhabit terrestrial habitats away from water (e.g., forest understory, open bushes, or tree trunks; see Supplementary Table S1). These more terrestrial species are found on separate landmasses, including New Zealand (D. minor (Figure 1C) and D. schauinslandi: Vink and Dupérré, 2010), North America (D. tenebrosus (Figure 1H) and D. albineus Hentz, 1845: Carico, 1973; Guarisco, 2010), and East Asia (D. sulfureus (Figure 1I), D. silvicola Tanikawa and Miyashita, 2008, D. nigrimaculatus Song and Chen, 1991, and D. zatsun Tanikawa, 2003: Tanikawa, 2003; Tanikawa and Miyashita, 2008; Ono, 2009; Chae et al., 2023). These instances of terrestrial lifestyle have, according to the phylogeny, evolved independently (Yu et al., 2024).
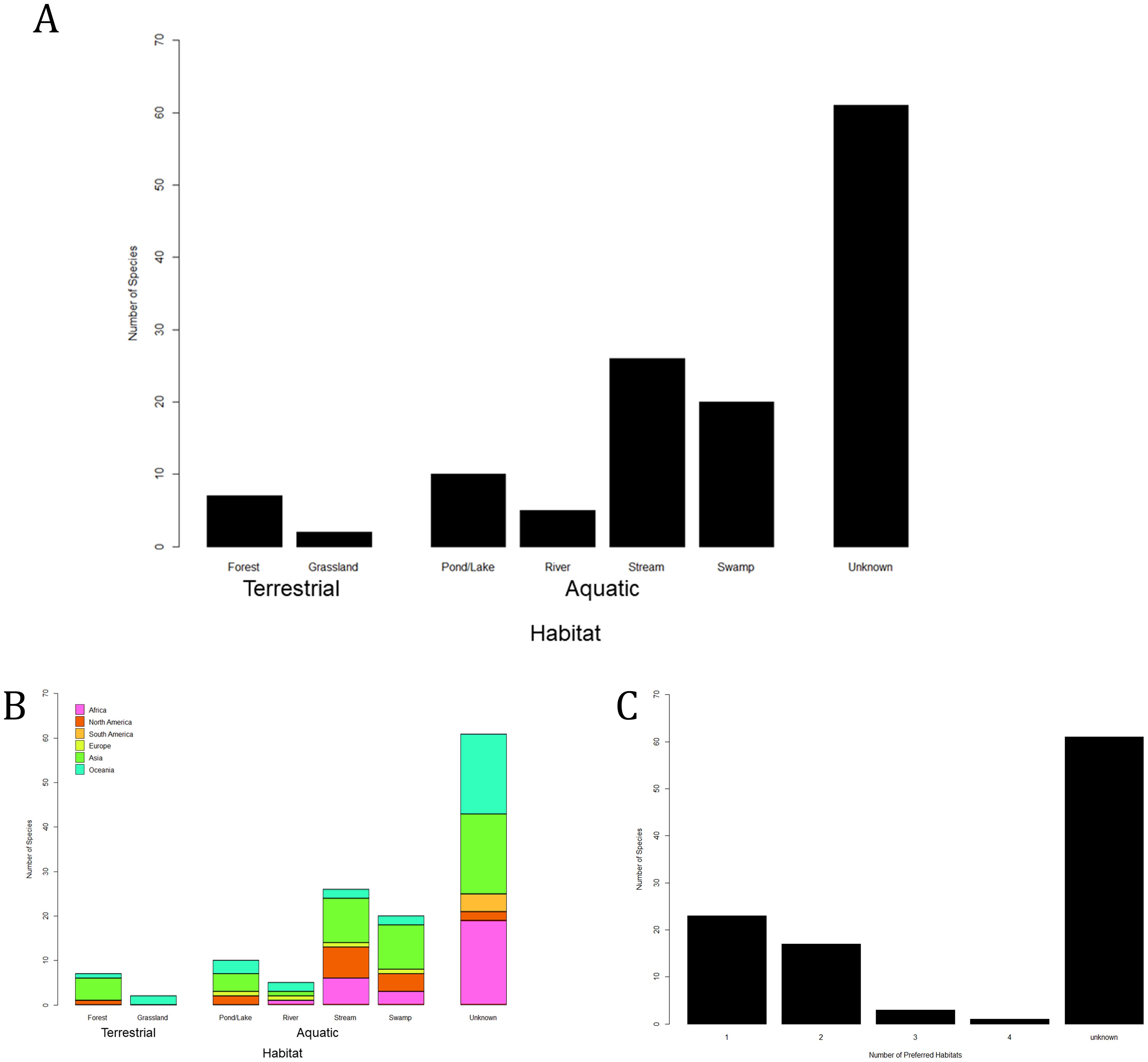
Figure 7. Documented six major habitat types in two terrestrial and four aquatic categories inhabited by Dolomedes species (see also Supplementary Table S1): (A) number of Dolomedes species of each habitat category; (B) idem, with color codon showing different geographic regions; (C) number of Dolomedes species with different numbers of preferred habitat category/categories.
The apparent high degree of microhabitat specialization may play a role in limiting heterospecific interactions and matings, even in regions where multiple species are common. Indeed, introgression has only been recorded once between two New Zealand species (Vink and Dupérré, 2010; Lattimore et al., 2011). Cytochrome c oxidase subunit I (COI) haplotypes clearly assignable to D. aquaticus Goyen, 1888 were present in specimens of D. minor, however, the reverse situation is unknown (Vink and Dupérré, 2010; Lattimore et al., 2011). This introgression has only been identified from the southern quarter of the South Island of New Zealand despite the two species also occurring sympatrically elsewhere. It is unknown why introgression only occurs one way in these two species and why it appears to be geographically limited, but it may have something to do with species-specific microhabitat use and/or flexibility. Studies examining microhabitat use and mating behavior in these species will surely shed light on this intriguing pattern.
As a genus well known for its semi-aquatic lifestyle, terrestrial Dolomedes species raise questions about adaptations to land versus water. Tanikawa and Miyashita (2008) compared two terrestrial species – D. sulfureus and D. silvicola – to their semi-aquatic sisters and found that the terrestrial species have relatively longer first legs. A comparative analysis over the breath of Dolomedes phylogeny, however, has rejected an overall validity of this hypothesis but instead found that semi-aquatic spiders at higher hierarchical levels are larger-bodied (Yu et al., 2024). Empirical studies that focus on hydrophobic structures, mechanisms, and behavior related to locomotion on and under water (e.g., claw tuft functional morphology, the ability to dive across species) as well as resilience to dehydration are now needed to elucidate the differences between semi-aquatic and terrestrial species.
3.2 Phenology
Although there is information on the population dynamics across seasons for a few Dolomedes species (e.g., D. triton: Zimmermann and Spence, 1998), most species accounts of phenology can only be estimated according to notes on collections or from anecdotal evidence. Although Dolomedes can be found throughout the year, they are most commonly observed during the mating season. Most Dolomedes seem to be nocturnal (D. minor, D. aquaticus: Williams, 1979a; D. orion: Baba et al., 2019; D. raptor: Tso et al., 2016), however, North American and European species are active during the day (e.g. D. scriptus: Scott et al., 2016; D. fimbriatus and D. plantarius: Heldingen, 1993; Dolomedes sp.: Nyffeler and Pusey, 2014).
The reproductive season for northern hemisphere species typically spans May to October (Carico, 1973; Guarisco, 2010; Nakajo, 2024), while southern hemisphere species reproduce between September to May, peaking in December and January (Vink and Dupérré, 2010). Many species overwinter as juveniles, though adults have also been found during these months (Carico, 1973; Zimmermann and Spence, 1998; Guarisco, 2010; Nakajo, 2024; Miyashita, 1986).
Many species require one to two years of development and live for several seasons (Schmidt, 1957; Zimmermann and Spence, 1998; Nakajo, 2024). This varies not only between species, but also within species. For example, in D. sulfureus, juveniles will overwinter once or twice to reach maturity depending on hatching time. Such differences in overwintering strategy might relate to the cessation of juvenile growth under short daylight conditions (Miyashita, 1986). Maturation time can also differ between the sexes, which may relate to variation in SSD. For example, Nakajo (2024) suggests male D. raptor require a year to mature, while the much larger females may need two additional years. Sex ratios in Dolomedes can fluctuate over the season, shifting from male-biased to female-biased, likely due to mating behavior, including sexual cannibalism and spontaneous male death (Zimmermann and Spence, 1992; Schwartz et al., 2013). Furthermore, protandry, or the patterns of males maturing before females, appears common (Dolomedes tenebrosus: Schwartz et al., 2013; D. triton: Johnson, 2004, Johnson, 2005). We lack data on population sex-ratio and seasonality for most species, yet this information is crucial for understanding aspects of their biology, especially as it relates to reproduction and mating systems.
3.3 Predators and parasitoids
Dolomedes are known to be important to nutrient flow in riparian systems (Collier et al., 2002), through their role as predators of aquatic invertebrates and vertebrates. However, their role as prey, and therefore as nutrient transfer to higher trophic levels is poorly documented.
There are sporadic references to Dolomedes as prey to generalist predators. These include little blue heron (Egretta caerulea) (Carico, 1973), frogs (Suter, 2003; Loc-Barragán et al., 2017), fish (Figiel and Miller, 1994), owls (Lindsay and Ordish, 1964), and Parasteatoda tepidariorum cobweb spiders, documented with D. tenebrosus and D. albineus specimens in their webs (Guarisco, 2010). Dolomedes fimbriatus and D. striatus Giebel, 1869 occasionally fall prey to the purple pitcher plant (Sarracenia purpurea) when using it as a refuge and hunting ground, although even newly emerged spiderlings can avoid pitcher plant predation (Leech and Buckle, 1987; Zander, 2016). Carico (1973) suggested that sphecoid wasps hunt Dolomedes, but this predation appears rare, with only occasional instances by generalist species (Krombein, 1979; Polidori et al., 2007). Furthermore, Carico (1973) speculated that visual predators must be important to multiple Dolomedes species given their cryptic coloration which helps them blend into their respective habitats.
The New Zealand fernbird (Megalurus punctatus) is a notable predator of Dolomedes, feeding on all three mainland New Zealand species (Harris, 1986; Parker, 2002). Fernbirds partially specialize in Dolomedes, taking spiderlings from nursery webs for their nestlings and consuming adult females (Forster and Forster, 1999; Parker, 2002). Bird predation is assumed to influence habitat selection in Dolomedes triton, with higher population densities forming in habitats with more potential refuges from bird and fish predators (Jordan et al., 1994). This suggests bird predation affects the behavior and habitat selection of many Dolomedes species, driving them to remain inconspicuous. The impact of bird predation on spiders varies by spider family (Gunnarsson, 2007), presenting the need for more research on its effects on Dolomedes. Future studies should examine how bird predation influences Dolomedes microhabitat selection and activity cycles to better understand its role in shaping their behavior.
Predatory fish also indirectly affect Dolomedes. While anecdotal evidence found fish eating semi-aquatic spiders (Jordan et al., 1994), experimental evidence shows that direct effects of fish predation are limited. In experimental pools, bluegill sunfish presence reduced average D. triton body size but not population size, suggesting avoidance strategies or competition for prey (Figiel and Miller, 1994). Dolomedes triton escape behavior is ineffective against simulated trout (Oncorhynchus mykiss) attacks (Suter and Gruenwald, 2000a) implying fish predation on Dolomedes is a relatively minor evolutionary factor. Similar studies, however, are needed in other Dolomedes species.
Dolomedes have numerous defense mechanisms against predation. Touch and vibration are most important in threat detection, with vision being used only secondarily, if at all (Williams, 1979a; Suter, 2003). When under threat, Dolomedes can use their rapid locomotion to escape. Williams (1979a) found that New Zealand Dolomedes tend to escape by either submerging under water or dropping to the ground (see also Locomotion & Dispersal). However, D. dondalei Vink and Dupérré, 2010 will run onto the surface of rivers, even allowing the current to take it further downstream. This species is also more difficult to disturb, indicating it could rely more on crypsis than escape behavior (Williams, 1979a). Dolomedes triton also has specialized escape behavior against frog attack, involving leaping away from the surface of the water. This behavior was tested against two frog species under laboratory conditions, and when the Dolomedes deployed this behavior, they mostly escaped predation (Suter, 2003). The behavior is effective in the wild, as Krupa (2002) found Dolomedes make up only a small proportion of frog gut contents.
Dolomedes are also preyed upon by parasitoid pompilid wasps, as evidenced by prey records in North America, Europe, New Zealand and Eastern Russia (Richards and Hamm, 1939; Harris, 1999; Kurczewski and Edwards, 2012; Kurczewski and Kiernan, 2015; Kurczewski et al., 2017; Kochetkov and Loktionov, 2019), laboratory evidence of pompilid predation on Dolomedes in Japan (Shimizu, 1992), as well as assumed interactions in Ireland (O’Hanlon and O’Connor, 2021) and India (Rajmohana, 2017). Pompilids often rob nests of other species, leading to Dolomedes becoming prey for pompilids that do not hunt them directly (Harris, 1999). Wasps hunt Dolomedes predominantly by visual cues (Shimizu, 1992) but can also utilize their antennae to follow scent trails left by spiders (Harris, 1987; Harris, 1999), and then paralyze the spider with venom. At least one case is noted of a Dolomedes resisting capture by biting a wasp (Kurczewski and Edwards, 2012). After paralysis, the spider is dragged back to the wasp’s nest. The hydrophobic nature of Dolomedes can be used by wasps to ride them as rafts, sometimes propelling themselves along the water using their wings (Evans and Yoshimoto, 1962; Shimizu, 1992; Kurczewski and Edwards, 2012). Eggs are laid on the spider, after which Dolomedes can be large enough to sustain a wasp larva through several early instars (Harris, 1999).
Generally, Pompilidae target spiders based on their ecology, but there is also evidence of specialization on Dolomedes. In New Zealand, nests of the introduced Australian Cryptochelius australis were found to have large numbers of Dolomedes. However, there are also reports of this species preying upon Miturga and Ulidon spiders (Harris, 1999; Martin, 2012). In North America, Anoplius depressipes is a specialist on Dolomedidae and Pisauridae, predominantly targeting Dolomedes, though in some cases hunting Pisaurina mira (Kurczewski and Edwards, 2012; Kurczewski and Kiernan, 2015; Kurczewski et al., 2017). Anoplius depressipes are also adapted to walk across water and dive to capture their prey (Evans and Yoshimoto, 1962; Roble, 1985; Kurczewski and Edwards, 2012). These traits are shared with Anoplius eous, however, while laboratory data shows this species to specialize on Dolomedes, it seems to hunt Pardosa pseudoannulata (Lycosidae) in the wild (Iwata, 1939; Shimizu, 1992). Details of pompilid predation and other predation on Dolomedes can be found in Table 1.
Mantis lacewings (Mantispidae) are also spider-specific parasitoids (Kaston, 1938). Unlike the above-mentioned wasps, mantispid larvae target spider eggs by “hitchhiking” on spiders then entering their egg sacs when the female spiders are laying eggs (Haug et al., 2018). So far, direct record of Mantispidae parasitizing Dolomedes is only known from a female D. bedjanic from Madagascar, where Yu and Kuntner (2024) found a mantispid larva in the spider’s epigastric furrow.
3.4 Conservation
Despite their ecological importance, spiders are rarely the focus of conservation programmes (Milano et al., 2021) but wetlands, where many Dolomedes are located, are estimated to have decreased between 33% and 87% since the 18th Century (Davidson, 2014; Hu et al., 2017). Wetlands provide vital ecological services, including temperature regulation, pollution filtering, and surface runoff control (Zedler and Kercher, 2005). Unfortunately, these vital habitats face significant degradation from urbanization, agriculture, pollution, and climate change (Davidson, 2014; Hu et al., 2017). Monitoring organisms like spiders can help gauge the impact of human activities on these crucial habitats.
Two species of Dolomedes are of current conservation interest; D. plantarius from Europe and D. schauinslandi from the Chatham Islands of New Zealand. The former is rated as “vulnerable” on the IUCN Red List (World Conservation Monitoring Centre, 1996) while the latter is classified as “At Risk: Relict” in New Zealand (Sirvid et al., 2021).
Dolomedes plantarius is one of the most widespread Dolomedes species, distributed from Siberia to Britain and from the Apennines to Scandinavia (World Spider Catalog, 2024). However, the species prefers very specific habitats which are in general well-vegetated open water bodies with low velocity (Dickel et al., 2022), such as lowland rivers, bogs, fens, and oxbows (Smith, 2000; Van Helsdingen, 2005; Duffey, 2012). Dolomedes plantarius is considered threatened by habitat loss and degradation caused by human activities (Smith, 2000; Duffey, 2012; Milano et al., 2021). Although our knowledge of their distribution ranges remains largely incomplete, recent estimations and modeling of their suitable habitats (Leroy et al., 2013, Leroy et al., 2014; Monsimet et al., 2020; Milano et al., 2022) show that D. plantarius is under more pressure from climate and land use changes than D. fimbriatus. Compared to D. fimbriatus, D. plantarius exhibits narrower habitat preferences as well as poorer ability of both waterborne and airborne long-distance dispersal (Monsimet et al., 2020, Monsimet et al., 2022; see also Locomotion & Dispersal). Overall, the distribution ranges of D. plantarius are estimated to decrease and shift northward following the trends of global temperature rising (Leroy et al., 2013, Leroy et al., 2014; Monsimet et al., 2020; Milano et al., 2022).
Dolomedes plantarius is listed in the national Red Lists of 13 European countries with nine of them protecting the species and its habitats by law (see Milano et al., 2021). The United Kingdom (UK) is the only country that applies further actions in protecting D. plantarius (Smith, 1996, Smith, 2000, Smith, 20051; Duffey, 2012; Smith et al., 2013). There are only three disjunct natural populations of D. plantairus left in the UK (Duffey, 2012). Therefore, the species is suggested to be highly vulnerable with urgent need of conservation action to prevent local extinction. Habitat restoration work and regular census of D. plantarius at Lopham Fen National Nature Reserve has occurred since 1991 to prevent degradation of current water bodies and create new habitats (Smith, 1996, Smith, 2000, Smith, 20051). Although long-term monitoring suggests that the population sizes of D. plantarius vary drastically across years, progressive vegetation restoration at the site has allowed considerable expansion of the population over the last decade (Smith, 20051; Smith, 2020). In addition, the conservation framework of D. plantarius in the UK also includes translocation. Starting in 2010, Smith et al. (2013) launched the translocation project of D. plantarius aiming to expand its populations from three to 12 in the UK following the IUCN protocols. By 2021, the translocation project had successfully increased the D. plantarius populations from three to seven (Milano et al., 2021).
Unlike D. plantarius, the degradation of wetlands does not explain the decline of D. schauinslandi, found in forest and scrublands away from waterways on three small islands in the Chatham Island archipelago (Hokorereoro/Rangatira/South East, Maung’Re/Mangere, and Houruakopara) in New Zealand. It was previously found on Rangihaute/Rangiauria/Pitt Island before going extinct in the early 1900s (Vink and Dupérré, 2010) and was likely also found on Rēkohu/Wharekauri/Chatham Island. Despite being a threatened species, little is known about its biology, making it an obvious subject for conservation genomics and ecology research. In particular, understanding the interacting effects of dispersal behavior, impacts of invasive predators, habitat availability and quality, prey availability, and climate change are essential for the future of this species.
It is unlikely that these two species are the only Dolomedes affected by global change, but understanding the impacts of anthropogenic pressures is difficult when we lack diagnosis of threat status for most species. A relatively new research avenue involves studying the effects of heavy metals and pharmaceuticals on aquatic spiders as bioindicators of waterway pollutants. For example, Ortega-Rodriguez et al. (2019) found that an unidentified Dolomedes had the highest methylmercury concentration among numerous shoreline spiders, likely reflecting their aquatic prey diet. Given their close proximity to water and ease of observation, using Dolomedes as bioindicators of a range of anthropogenic impacts provides a fruitful avenue for future research.
4 Behavior
4.1 Sensory physiology
Given that many Dolomedes species hunt on water, a strong focus of their sensory physiology has been the detection of waterborne prey. Prey detection and stimulus discrimination has been well-investigated in D. triton (Bleckmann and Barth, 1984; Bleckmann and Rovner, 1984; Bleckmann and Bender, 1987; Bleckmann and Lotz, 1987; Bleckmann et al., 1994; Suter, 2003) as well as in D. okefinokensis (Bleckmann et al., 1994). Dolomedes can locate prey using different environmental cues, with artificially generated water surface waves showing the highest spider responsiveness, followed by airborne vibrations (Bleckmann and Rovner, 1984; Bleckmann and Barth, 1984). Notably, visual stimuli were shown to trigger spider reactions in a few cases as well (Bleckmann and Rovner, 1984).
Dolomedes are likely to detect water surface waves using lyriform organs (a slit organ on the metatarsus of the legs; Figure 8A) and airborne vibrations using trichobothria (long, thick sensilla; Figure 8B). Studies have shown that spiders are attracted to stimuli with an irregular mix of low and high frequencies, including those about 30–40 Hz (Bleckmann and Barth, 1984; Bleckmann and Lotz, 1987). In comparison, wind generated surface waves rarely exceed 10 Hz (Bleckmann and Rovner, 1984). Sensory abilities and reactions to wave sources also seem to differ between species, as shown in a comparative study on D. fimbriatus and D. triton (Bleckmann and Barth, 1984). Dolomedes fimbriatus has a larger error angle (i.e., the spider is less accurate in targeting the wave source) and is slower than D. triton (Bleckmann and Barth, 1984). Both the lyriform organ and trichobothria were shown to be crucial to minimize the error angle when spiders move towards or escape from a wave source (Bleckmann and Rovner, 1984; Suter, 2003). The morphology and ultrastructure of Dolomedes sensory structures is only briefly touched on by a few studies. For New Zealand species mechanoreceptive sensilla and contact-chemoreceptors have been discussed (Williams, 1979a), but never described in further detail. There is much room for comparative studies on sensory systems across Dolomedes, especially focused on terrestrial versus water-associated species.
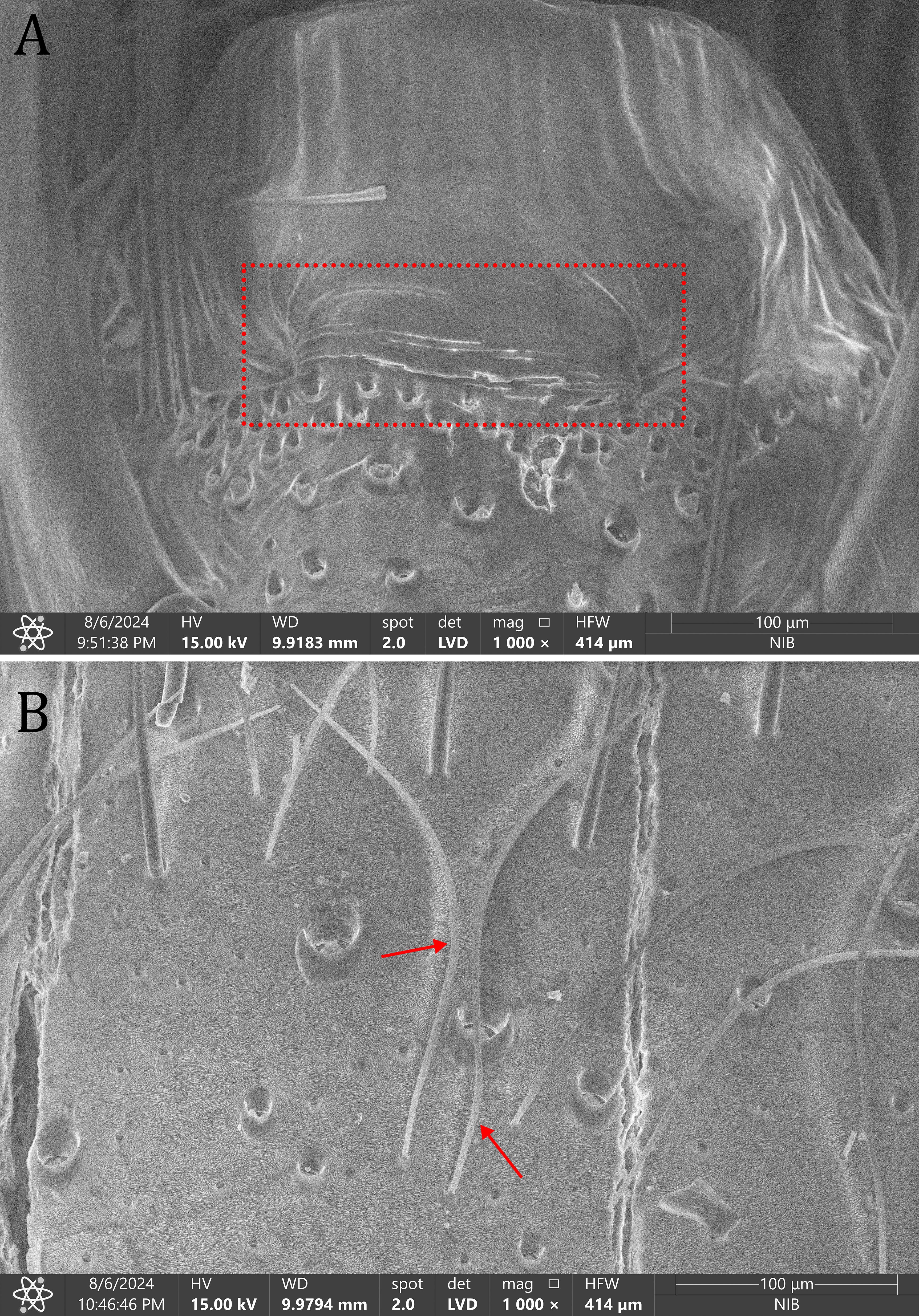
Figure 8. Vibration sensing organs in Dolomedes: (A) a lyriform organ of Dolomedes fimbriatus (highlighted in red) on the apical dorsal part of metatarsus of leg I; (B) trichobothria of Dolomedes angustivirgatus Kishida, 1933 (red arrows) on the basal ventral part of metatarsus of leg IV.
Dolomedes, like the majority of spider groups, are thought to have rather poor vision. Nonetheless, Dolomedes use visual cues during predation and presumably also during courtship (Roland and Rovner, 1983; Bleckmann and Barth, 1984). Dolomedes triton, for example, was found to run slower and shorter distances towards prey when blinded (Bleckmann and Barth, 1984). They also appear able to focus light under water, because the surrounding body hairs capture a thin air layer (Williams, 1979a). However, the extent to which they might use vision underwater, and whether this varies across species, has yet to be explored.
There are detailed descriptions of the architecture of the eyes of D. aquaticus and other New Zealand Dolomedes species (Blest and Day, 1978; Williams, 1979b) as well as the tapetum lucidum (a light-reflecting layer inside the eye) of D. tenebrosus, D. triton, D. scriptus and D. vittatus Walckenaer, 1837 (Benson and Suter, 2013). But apart from those studies, there has been little work done on the anatomy of the visual system. To our knowledge, no studies have explored the processing pathways of the primary and/or secondary eyes of any Dolomedes species. Such a study would be interesting, as major differences were recently discovered in the central nervous system of primarily web building versus cursorial spiders. While cursorial species possess prominent higher order processing centers (mushroom bodies) and visual neuropils, web builders have those regions reduced or absent, however their leg neuropils are proportionally larger (Steinhoff et al., 2023). We would predict Dolomedes central nervous system patterns to be like those described in other cursorial species, but detailed neuroanatomical studies are needed.
4.2 Locomotion and dispersal
Given their often-close connection with water, Dolomedes are highly capable of moving across its surface (Figure 9A). Early research explored their patterns of locomotion across terrestrial versus water surfaces (multiple species: Ehlers, 1939; D. fimbriatus: Barnes and Barth, 1991; D. triton: Shultz, 1987) and comparative studies suggest that Dolomedes are specialized for water locomotion (Shultz, 1987; Barnes and Barth, 1991; Stratton et al., 2004).
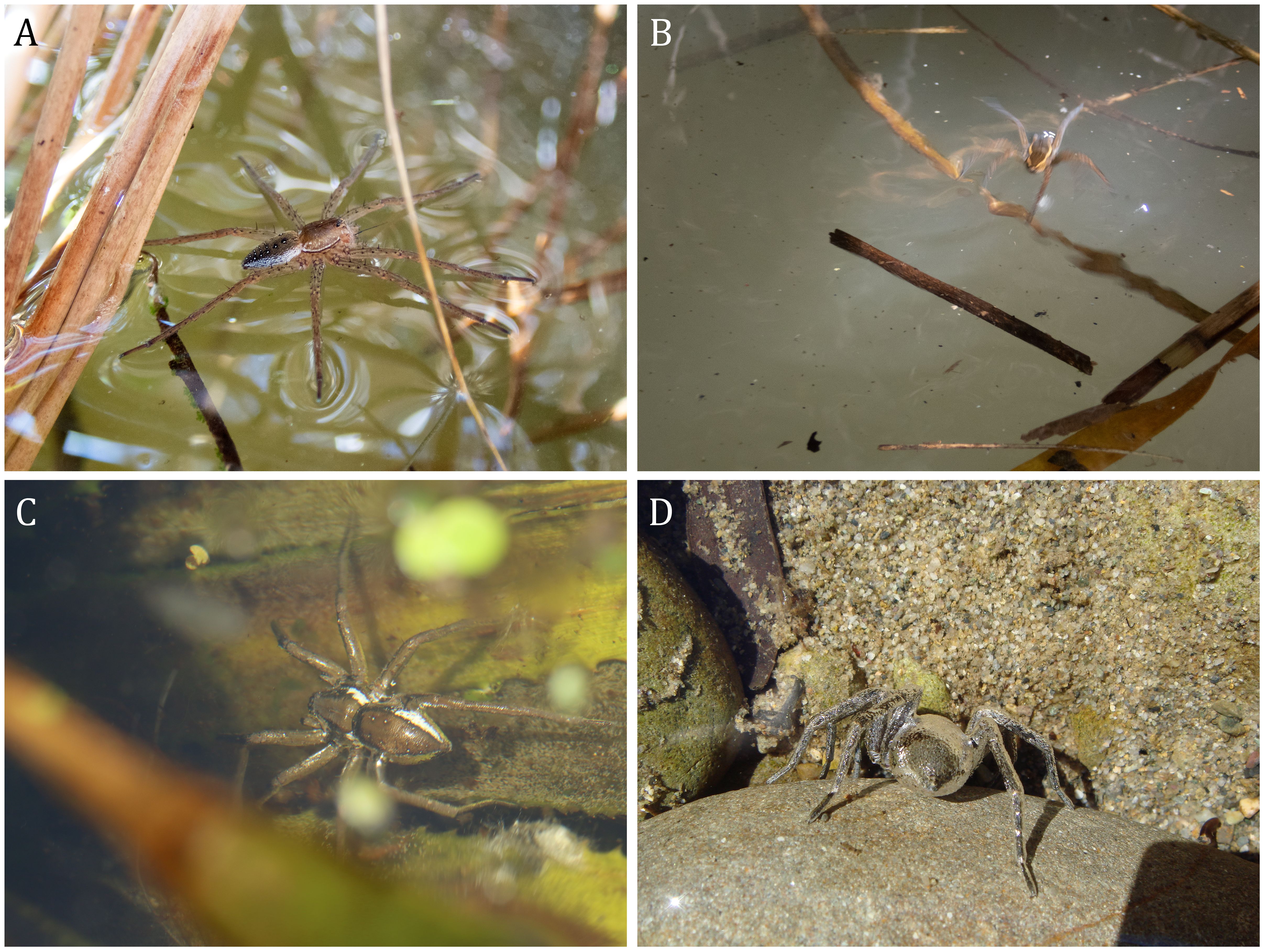
Figure 9. Aquatic locomotion in Dolomedes: (A) D. plantarius (Clerck, 1757) floating on water; (B) D. plantarius “running” on water; (C) D. plantarius diving under water; (D) D. aquaticus Goyen, 1888 diving under water.
Two types of water surface gaits have been described – rowing (D. triton: McAlister, 1960; Shultz, 1987; D. plantarius: Gorb and Barth, 1994; Suter and Gruenwald, 2000a, Suter and Gruenwald, 2000b) and galloping or running (Figure 9B; D. triton: Suter and Wildman, 1999; D. plantarius: Gorb and Barth, 1994); the latter of which tends to be associated with prey capture (D. plantarius: Gorb and Barth, 1994). Rowing Dolomedes can reach speeds of <0.27m/s (reviewed in Suter, 1999) and involves the use of leg pairs II and III in synchrony while legs I and IV are motionless and held parallel to the direction of movement. Galloping is much faster, with individuals moving more than 0.4m/s,and involves leg pairs I, II, and III moved in synchrony (reviewed in Stratton et al., 2004). Numerous studies have explored the posture, gait, and rowing behavior of Dolomedes on different substrates and/or on water with different viscosity and depth (e.g., D. aquaticus: Campbell et al., 2014; D. triton: Shultz, 1987; D. fimbriatus: Barnes and Barth, 1991) while others have explored their hydrophobicity and escape responses across water (D. aquaticus and D. minor: Williams, 1979a).
In 2004, Stratton and colleagues greatly expanded our understanding of spider locomotion on water by conducting a comparative study exploring water-surface locomotion from 249 spider species across 42 families. Trechaleidae Simon, 1890 and Pisauridae (at the time, containing Dolomedes) were the only focal families to show a monomorphy for both a hydrophobic surface (remaining dry and completely above the water surface) and movement by rowing. All five Dolomedes species tested (D. albineus, D. tenebrosus, D. triton, D. gertschi Carico, 1973, and D. vittatus) demonstrated rowing and the genus was used as the standard against which other species were compared (Stratton et al., 2004). A specialized rowing gait was hypothesized to have evolved at least 4 times independently, with hydrophobicity suggested to be a preadaptation to aquatic gaits. Rowing behavior, however, was hypothesized to have evolved once at the base of the clade that includes Pisauridae (containing Dolomedes), Lycosidae and Trechaleidae. The specialized gait of pisaurids is facilitated by dimple distortion, drag, generation of vortices, and the hydrophpilic hairs (Suter, 2013). The results of Stratton et al. (2004) aligned with the latest study (Yu et al., 2024) suggesting that the semi-aquatic lifestyle is ancestral to Dolomedidae, Pisauridae, Lycosidae, and Trechaleidae. Considering our knowledge of the aquatic movement patterns is limited to a few Dolomedes species, an expanded investigation across a broader range of Dolomedes species would allow a more nuanced understanding of the evolution of water-surface locomotory gaits and associated traits.
Dolomedes triton has also been observed “sailing” by extending and elevating its anterior pair of legs and letting the wind carry it across the water’s surface (Deshefy, 1981). Another distinct form of sailing involves the spider lifting its body above the water surface by extending and depressing all of its legs (Suter, 1999). Controlled studies of this elevated posture reveal that it is a cheap form of locomotion but comes at the cost of reduced control of directionality (Suter, 1999). Expanding biomechanical and behavioral studies of sailing to other Dolomedes species, potentially with a focus on locomotion across distinct bodies of water (e.g., fast flowing versus stagnant) could provide information regarding the evolution of mechanisms of unique locomotion.
In addition to moving across water, many Dolomedes species can submerge underwater (Figures 9C, D) in response to predation risk, by exploiting air bubbles created by hydrophobic hairs spread across their body. Submergence tests revealed that D. triton individuals would dive under water and remain there voluntarily from 4–30 minutes, with at least one individual remaining responsive underwater for more than 3 hours (McAlister, 1960). Similarly, D. aquaticus can stay submerged for up to 30 minutes (Forster and Forster, 1973). Dolomedes triton appeared to require solid support structures to break through the water surface both on entry and exit (McAlister, 1960; Williams, 1979b) and similar observations of the requirement of a substrate for submergence were seen in New Zealand’s Dolomedes (presumably D. minor, D. aquaticus, and D. dondalei: Williams, 1979a). Interestingly, McAlister noted that D. vitattus (referred to as D. urinator Hentz, 1845) exhibits a different exit behavior (McAlister, 1960), which sounds similar to some of the feeding positions of D. dondalei (Williams, 1979a) – remaining largely submerged with one or two legs protruding above the water. Given observations of distinct exit behavior, comparative work across species exploring the biomechanics of submergence and re-emergence might provide insights into the evolution of related morphologies – e.g., size variation.
To disperse, spiders can actively walk over short-distances or passively travel over longer-distances by ballooning, like many spiderlings do after their first molts in their nursery web (Bell et al., 2005; Vink and Dupérré, 2010; Frost et al., 2013; Monsimet et al., 2022). Species-level differences have been found too: for example, Dolomedes fimbriatus, a species with less habitat specialization, demonstrated a higher propensity for long-distance dispersal (both airborne and waterborne), while the more habitat specialized D. plantarius was more likely to engage in waterborne rowing (Monsimet et al., 2022). In the field, quantified distances of dispersal are lacking for any species. Studies aimed at Dolomedes dispersal distance and patterns could provide insight into the likelihood of establishment for species of conservation concern, such as D. plantarius and D. schauinslandi, in newly restored habitats or for translocation. Such studies could also inform hypotheses about the global distribution of Dolomedes.
Little is known about individual movement patterns in most Dolomedes species. In D. triton, a field survey using marked individuals found that adult females moved more than juveniles, but their movement reduced again once they produced egg sacs (Kreiter and Wise, 1996). This increase in movement with adulthood was presumed to be associated with more active, versus passive, hunting in adult females, but could also relate to distinct age or size-related predation pressure. To fully understand the natural history of Dolomedes, we require additional information on species-specific movement and dispersal patterns.
4.3 Diet and predation behavior
Like most spiders, Dolomedes are opportunistic predators with broad diets (Figure 10), although invertebrates seem to make up most of their catch (Figures 10A, B). In D. triton, semi-aquatic and aquatic insects and spiders form most of their diet, dominated by Hemiptera, Odonata and Diptera (Zimmermann and Spence, 1989). Similarly, D. dondalei and D. aquaticus primarily consume aquatic insects, especially Diptera (mostly tipulids), Trichoptera and Ephemeroptera. An isotope analysis confirmed that aquatic insects are a key component of Dolomedes diet, although this varied between sites (Collier et al., 2002). Cannibalism can also form a significant portion of Dolomedes diet (Figure 10C; e.g. Greenwood et al., 2010). For example, in D. triton conspecifics formed about 5% of their diet (Zimmermann and Spence, 1989). The diet of terrestrial Dolomedes species (e.g. D. schauinslandi; Figure 10A) is less known, but presumably comprises of terrestrial invertebrates. Even in semiaquatic species, small juveniles may forage on vegetation away from the water that larger juveniles and adults rely on (Zimmermann and Spence, 1998). The extent to which Dolomedes feed on aquatic versus terrestrial prey may be important in their roles as bioindicators as well as in their susceptibility to distinct forms of environmental contamination.
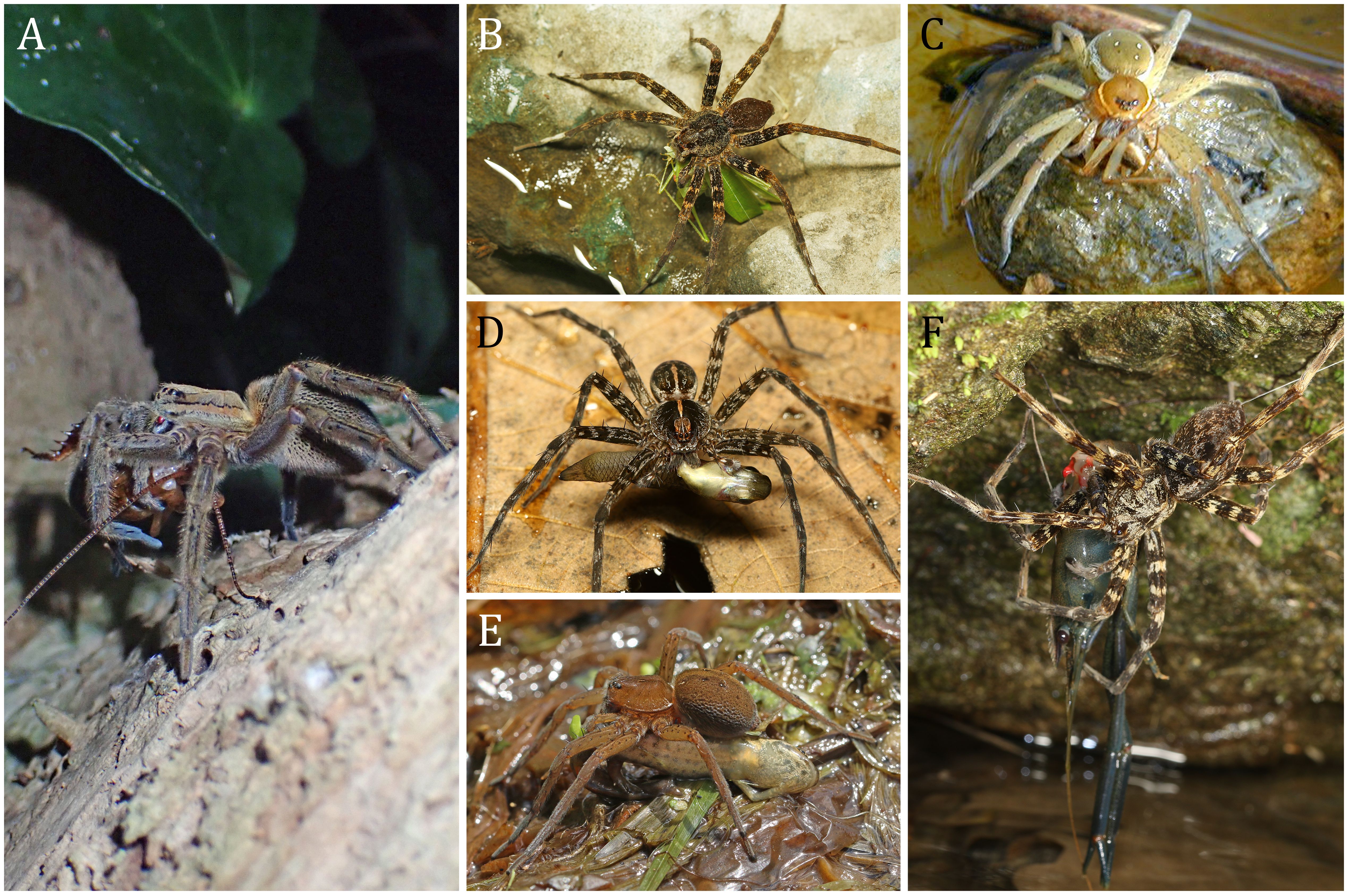
Figure 10. Diet of Dolomedes: (A) D. schauinslandi Simon, 1899 feeding on a Wētā; (B) D. raptor eating a katydid; (C) female D. triton (Walckenaer, 1837) cannibalizing a male; (D) D. mizhoanus Kishida, 1936 eating a mosquito fish; (E) D. plantarius having captured a newt; (F) D. raptor feeding on a freshwater prawn.
Dolomedes do not limit their diet to small invertebrates, capturing the attention of biologists and arachnophobes alike with their predation of vertebrates, including fish (Figure 10D; Williams, 1979a; Nyffeler and Pusey, 2014), lizards (Eversole, 2022), amphibians (frogs, tadpoles, toads, and newts; Figure 10E; Moore and Townsend, 1998; Cabrera-Guzmán et al., 2015), bats (Leivers et al., 2021), as well as large freshwater crustaceans (Figure 10F; Kosuge and Sasaki, 2002; Baba et al., 2019). A review by Nyffeler and Pusey (2014) reports observations of fish predation in the wild across 11 Dolomedes species. It seems appropriate to refer to Dolomedes as fishing spiders given widespread fish predation in the genus.
Adult Dolomedes spiders forego capture webs, opting instead to position themselves motionless to sit and wait for their prey to walk or float by. A characteristic behavior of semi-aquatic Dolomedes is to dangle their anterior legs over water surfaces in anticipation of prey floating past (Williams, 1979a). They will pursue prey, but only after initial detection (Williams, 1979a) and they do not actively hunt throughout the landscape, in contrast to roaming predators. Dolomedes use their chelicerae to inject venom, which immobilizes and kills prey, followed by extraintestinal digestion, some species transport prey to land after capture (Forster and Forster, 1973; Williams, 1979a; Uzenbaev and Lyabzina, 2009). Meal completion in D. minor can range from 10 to 30 minutes from prey capture, depending on prey size (Forster and Forster, 1973).
The use of body coloration in prey capture has also been suggested to help Dolomedes forage. For example, female D. raptor use distinct white patches of hairs on their legs to lure prey (Tso et al., 2016), while bright white stripes on male cephalothorax provide a similar function for prey attraction (Lin et al., 2015). Several other Dolomedes similarly possess such distinct leg patches in females (e.g. D. horishanus and D. hydatostella) or cephalothorax stripes in males (e.g. D. fimbriatus, D. rotundus), with related species lacking them, offering a valuable system to determine the prevalence of luring and compare prey capture techniques in this group.
Given that Dolomedes are able to take down vertebrates that can be many times larger than the spider itself, there has been significant interest in the function and biochemical properties of the venom for a handful of species – D. fimbriatus (Uzenbaev and Lyabzina, 2009; Kozlov et al., 2014) D. mizhoanus (Jiang et al., 2013; Li et al., 2014; Xu et al., 2015), D. sulfureus (Wang et al., 2013; Xu et al., 2015), and D. okefinokensis (McCormick et al., 1993; Meinwaldt and Eisnert, 1995). Bioactivity assays have shown that Dolomedes venom has a neurotoxic effect, causing disorientation, altered movement, and ultimately the death of prey (Li et al., 2014). Venoms of several Dolomedes species have been analyzed using mass spectrometry (McCormick et al., 1993; Meinwaldt and Eisnert, 1995; Wang et al., 2013; Li et al., 2014) and transcriptomics (Kozlov et al., 2014; Xu et al., 2015; Jiang et al., 2013). These studies document the diversity and structure of venom polypeptides, aid in reconstructing the evolutionary history of spider venom, and contribute to our understanding of venom function. Dolomedes venom seems to be of special interest in the potential for neurochemical and neurotherapeutic drug development, particularly because they can prey upon vertebrates, which suggests their venom contains neurotoxins that are targeted for vertebrate nervous systems (Li et al., 2014).
4.4 Reproductive behavior
Dolomedes courtship behavior (Figure 11A) has received less attention compared to other spider groups that show conspicuous behavior (see for a review: Huber, 2005) – e.g., the numerous colorful jumping spiders that engage in complex dances (reviews: Richman and Jackson, 1992; Elias et al., 2012; Nelson, 2023) or wolf spiders like the genus Schizocosa Chamberlin, 1904 which have conspicuous ornaments combined with complex songs and dances and whose study has contributed significantly to our understanding of complex multimodal signaling (Starrett et al., 2022 and references therein). Dolomedes lack obvious secondary sexual traits, sensational courtship or extensive silk use during courtship, all traits which have been observed in other spider taxa (e.g., some nephilids (Zhang et al., 2011) and pisaurids (Anderson and Hebets, 2016) (reviewed in Scott et al., 2018). As such, there has been little research on the relationship(s) between reproductive behavior such as courtship and mating success in Dolomedes.
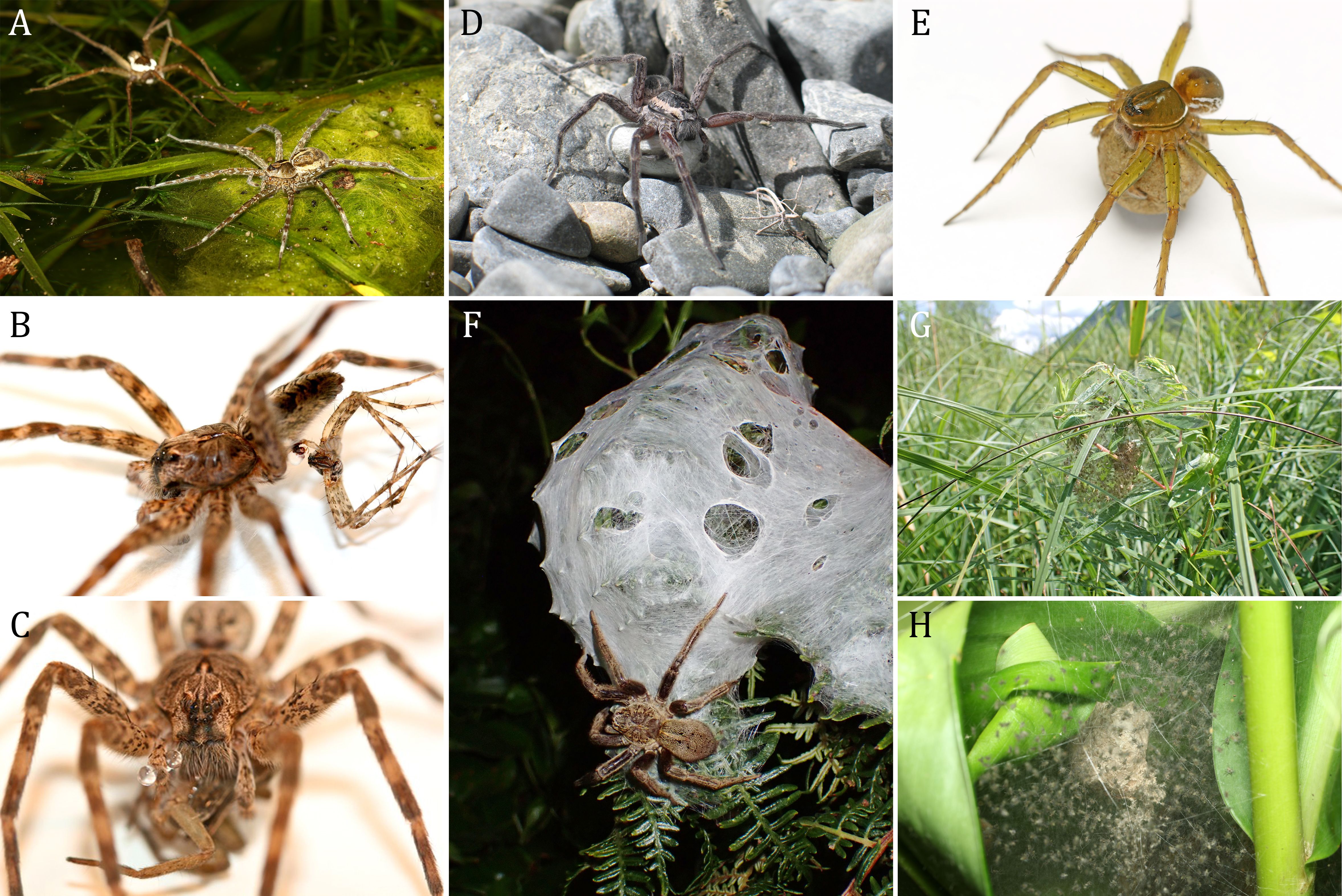
Figure 11. Reproductive behavior of Dolomedes: (A) male D. mizhoanus (left) approaching a female (right); (B) copulation in D. tenebrosus, showing a male (right) having spontaneously died after inserting his left palp; (C) female D. tenebrosus cannibalizing a male; (D) female D. aquaticus carrying her egg sac; (E) female of an unknown Dolomedes species from Madagascar carrying her egg sac; (F) female D. schauinslandi guarding her nursery web; (G) nursery web of D. fimbriatus housing the spiderlings; (H) spiderlings of D. raptor in the nursery web.
Early studies on D. scriptus and D. triton showed that female silk contains sex pheromones, which aid as chemical cues for males who follow female draglines (Kaston, 1936; Roland and Rovner, 1983), inducing courtship displays. These pheromones are probably emitted from the female’s integument to the surrounding water, which may help males locate potential mating partners (Roland and Rovner, 1983). The species-specificity of pheromones and/or the potential for airborne signals/cues remain unexplored in Dolomedes.
Though courtship behavior likely varies in intensity, duration and specific expression between species, all investigated species share common features. The first phase of male courtship usually includes a series of leg movements (visual and vibratory modalities), which are often referred to as “leg-waving”, “tapping” and “jerking” (Kralj-Fišer et al., 2016). Next, males touch the female on their legs and abdomen (Carico, 1973; Roland and Rovner, 1983; Sierwald and Coddington, 1988; Arnqvist, 1992; Lin et al., 2015). In D. fimbriatus, male courtship was found to vary with female traits – females with lower body weight received less intense male courtship and were more likely to remain unmated (Kralj-Fišer et al., 2016). To date, however, no information exists regarding the relationship between courtship duration and/or complexity and male mating success. Male coloration appears to play a significant role in female mating decisions. For instance, in D. raptor, females are more likely to reject and even attack males that lack the species-typical white stripes (Lin et al., 2015). Female mate choice based on other visual or vibratory cues/signals has not received much attention in other Dolomedes, but they are worth investigating further, especially in conjunction with studies on their sensory ecology and physiology.
Female responses to courting males are quite variable among species. In D. scriptus and D. triton, females may respond to courting males with their own courtship behavior, such as “drumming” and “leg waving” (Roland and Rovner, 1983; Schoenberg et al., 2022), while females of D. fimbriatus react either by attacking the male or staying motionless in a receptive body position (Arnqvist, 1992), similar to the motionless female D. tenebrosus (Sierwald and Coddington, 1988). Males mount females usually facing the opposite direction and start inserting their pedipalps for sperm transfer (Sierwald and Coddington, 1988; Arnqvist, 1992; Schoenberg et al., 2022). The motionless state of females is reminiscent of the quiescence described in other spiders (reviewed in Cargnelutti et al., 2023) and would be interesting to explore further.
The duration of copulation as well as the number of pedipalps used for insertions varies across Dolomedes species. Copulation duration (male mounting female and inserting pedipalp/s) can be rather short (e.g., a few seconds in D. vittatus, D. triton, and D. fimbriatus: Carico, 1973; Arnqvist, 1992) or relatively long (on average 22 minutes in D. tenebrosus: Schwartz et al., 2014; though most of this time involves the male’s body hanging from the female as his heart slowly stops beating). The pattern of pedipalp use (one or both) varies between but also within species (Schmidt, 1957; Carico, 1973; Johnson, 2001; Schoenberg et al., 2022; Sierwald and Coddington, 1988; Arnqvist, 1992; Schwartz et al., 2013). Quantifying the relationship between copulation duration, number of pedipalps being used and sperm transfer, as well as disentangling the roles of each sex in copulation duration, will help us understand the observed variation and the potential role of sexual selection and sperm competition in influencing these evolutionary patterns.
4.5 Mating systems
In Dolomedes we observe great variation in species-specific patterns of female and male mating rates (i.e. mating systems; Table 2). At one extreme end of the spectrum we find D. tenebrosus, a species in which females will mate with up to three males while males will die in 100% of first matings (spontaneous male death; Figure 11B), making them strictly monogynous (Schwartz et al., 2013, Schwartz et al., 2014). At the other end of the spectrum, D. fimbriatus, D. scriptus and D. triton males mate with multiple females, making them polygynous (Arnqvist and Henriksson, 1997; Johnson, 2001; Kralj-Fišer et al., 2016; Schoenberg et al., 2022). Since both sexes have paired genitalia, males can potentially inseminate the same female twice, inserting both pedipalps in different openings. There is also evidence of high individual variation within the same population in a female’s number of mates (Arnqvist and Henriksson, 1997; Johnson, 2001; Kralj-Fišer et al., 2016; Schoenberg et al., 2022). Details of mating and cannibalism rates for previously studied species are summarized in Table 2.
The spontaneous death by male D. tenebrosus provides an intriguing example of monogyny (including terminal investment strategies) (for examples from other spiders and social insects see: Boomsma et al., 2005; Schneider and Fromhage, 2010; Jaffé et al., 2014) and provides a system in which to test hypotheses of the evolution of this mating system. In D. tenebrosus, the male’s death coincides with his consumption by the female, which has been shown to benefit both sexes through higher offspring quantity and quality (Schwartz et al., 2016). Various life history traits linked to monogyny are also observed in D. tenebrosus – i.e., a male-biased sex ratio and eSSD (Fromhage et al., 2007; Miller, 2007; Wilder and Rypstra, 2008; Schwartz et al., 2013). To fully leverage Dolomedes mating system evolution, more data on the mating systems and life history traits of additional species is needed for comparative analyses. Research on D. scriptus (Schoenberg et al., 2022), D. tenebrosus (Schwartz et al., 2013, Schwartz et al., 2014) and D. triton (Wojcicki, 1992) provide a good start, but such a diverse genus offers a wealth of further species to contribute to a comprehensive comparative study.
4.6 Sexual cannibalism and female aggression
Female aggression, often resulting in precopulatory and postcopulatory sexual cannibalism (consuming a mate before, during or after copulation; reviewed in Burke, 2024), is present in many Dolomedes species and significantly impacts mating rates and population dynamics (D. fimbriatus: Arnqvist, 1992; D. triton: Johnson, 2005b; Johnson and Sih, 2005; D. scriptus: Fisher and Price, 2019; Schoenberg et al., 2022). Numerous hypotheses have been proposed to explain sexual cannibalism (Figures 10C, 11C; reviewed in Burke, 2024), and a few have been tested with Dolomedes. Using D. fimbriatus, for example, Arnqvist and Henriksson (1997) found no evidence for Newman and Elgar (1991) idea that pre-copulatory sexual cannibalism represents a female’s assessment of a male’s value as a sperm donor versus a prey item, as female foraging history did not influence likelihood of cannibalism. An alternative hypothesis – the “aggressive spillover hypothesis” – was discussed in the same publication by Arnqvist and Henriksson (1997). They proposed that high female aggression towards males may stem from selection for a general predatory aggressive syndrome that is beneficial in a foraging context but potentially costly in a mating context. The aggressive spillover hypothesis has been tested in two Dolomedes species that showed mixed results. While a study on D. fimbriatus did not find evidence of a correlation between female foraging aggression and aggression toward courting males (Kralj-Fišer et al., 2016), a study on D. triton showed that foraging aggression was positively correlated with increased sexual cannibalism, feeding rate, larger adult size, boldness towards a threat and higher fecundity (Johnson and Sih, 2005). Further research exploring relationships between aggression and reproductive behavior are necessary to discern the potential role of female aggression in influencing reproductive strategies across Dolomedes and the potential for sexual cannibalism to be a sexually selected trait (Burke, 2024).
There are also interesting first insights into the existence of consistent among-individual differences in aggressiveness (i.e. “personality” traits) in Dolomedes and their correlation to sexual behaviors. In D. fimbriatus, for example, female voracity towards prey might be considered an intrinsic personality trait, but not aggression towards mates, as females adjusted their aggressive responses towards courting males based on the male’s size relative to their own (Kralj-Fišer et al., 2016). In contrast, D. triton female aggression levels were consistent across contexts, with D. triton females showing positive behavioral correlations between foraging voracity, sexual cannibalism tendency, and boldness in response to predation risk (Johnson and Sih, 2005, Johnson and Sih, 2007). Given the heightened interest in animal personality and behavioral syndromes in the last decades (see for example: Réale et al., 2007; Sih et al., 2015; MacKinlay and Shaw, 2023), Dolomedes could be a useful taxon to address questions related to the extent to which certain behavior is fixed per individual (i.e., a personal trait) or context dependent.
Previous experience also influences cannibalistic behavior in Dolomedes. Specifically, works on D. triton, D. scriptus, and D. fimbriatus found females to be significantly more aggressive to courting males if already mated (Zimmermann and Spence, 1989, Zimmermann and Spence, 1992; Johnson, 2001; Kralj-Fišer et al., 2016; Schoenberg et al., 2022). In D. triton, females that cohabited with adult males were more likely to subsequently cannibalize males during courtship encounters later in life (Johnson, 2004). Furthermore, levels of SSD also impact the probability of a female cannibalizing a male – i.e., when size differences were minimal, male D. fimbriatus and D. triton had a higher chance of evading female attacks (Johnson, 2005; Johnson and Sih, 2005; Kralj-Fišer et al., 2016). These latter results are consistent with the idea that female aggression towards males is non-selective with cannibalism being contingent upon the female’s physical power relative to the male’s defensive capability (Wilder and Rypstra, 2008; Roggenbuck et al., 2011).
In contrast to pre-copulatory sexual cannibalism, post-copulatory sexual cannibalism occurs after sperm transfer, making it possible for males to receive a fitness benefit from being cannibalized. Such a benefit was observed for both sexes in D. tenebrosus as females that cannibalized males after copulation produced more offspring that were higher in mass and survived longer than the offspring of females who consumed a similarly sized cricket (Schwartz et al., 2016). To date, it is unknown whether this benefit is specific to the consumption of male D. tenebrosus, or simply to the consumption of a new prey type. It is also unknown whether similar fitness benefits are present from post-copulatory sexual cannibalism in other Dolomedes species. Dolomedes tenebrosus is an eSSD species and has received attention because the male’s terminal investment strategy of obligate death following sperm transfer makes the males complicit in their own cannibalism (Schwartz et al., 2013, Schwartz et al., 2014). Complicity in sexual cannibalism has not yet been observed in other Dolomedes species. It remains unclear what, if any, mechanisms of post-copulatory sexual selection are at play in this system, but future studies exploring the potential for sperm competition and cryptic female choice are likely to reveal interesting patterns across species.
4.7 Parental investment
Dolomedes parental care is strictly maternal. Upon oviposition, female Dolomedes create a silken egg sac, holding it tight in their chelicerae, with their pedipalps extended over the front, and a silk dragline attached to their spinnerets (Figures 11D, E; Comstock, 1912). The egg sacs of D. triton have a unique, highly hydrophobic outer layer, allowing them to submerge their egg sacs (Correa-Garhwal et al., 2019). The egg sac exudes a buoyant force while submerged, resulting in the female exerting more pressure to remain underwater (McAlister, 1960), away from potential threats. Egg sacs are highly protected by the female and difficult to remove without tearing the lining of the egg sac (Johnson and Sih, 2007). Even unmated females have been observed guarding unfertilized egg sacs, however unfertilized egg sacks are also frequently consumed (Schmidt, 1957; Arnqvist, 1992; Fisher and Price, 2019). The only time a female will let go of her egg sac is to forage, after which she will resume carrying the egg sac (Forster and Forster, 1973). A study by Johnson and Sih (2007) found a significant negative correlation between a female’s boldness in guarding her egg sac and her body condition, denoting the importance of foraging to the cost of parental care. As with most topics discussed thus far, data on parental investment come from only a handful of species, leaving open the possibility of variation across the genus in parental investment.
A general timeline of reproduction for some common Dolomedes species is within Table 3. Female Dolomedes will travel with their egg sacs until close to hatching (Comstock, 1912). Spiderlings hatch within the egg sac as they are being carried (Forster and Forster, 1973). Near the time of the spiderlings’ first molt, the female constructs her nursery web and deposits the egg sac (Carico, 1973; Forster and Forster, 1973; Vink and Dupérré, 2010). She will tear open the egg sac using her chelicerae, releasing her offspring into the nursery web (Figures 11F–H; Comstock, 1912; Nicholas et al., 2011). In New Zealand, nursery webs of D. minor are more easily seen than the spiders. These distinctive nursery webs appear as little white purses, often on the tips of shrubs (Forster and Forster, 1973). On the other hand, in some species like D. fimbriatus nursery webs are less distinct, being made of sparser silk (Figure 11G) and persist for a shorter time. The placement of nursery webs by some female Dolomedes is purposeful and habitat specific. A study by Kreiter and Wise (1996) found a preference for placing nursery webs in Juncus effusus and structurally similar Juncus-like vegetation. Similarly, Dickel et al. (2022) found that D. plantarius showed a strong association with Carex sp. and that distance to water was a significant factor of web placement.
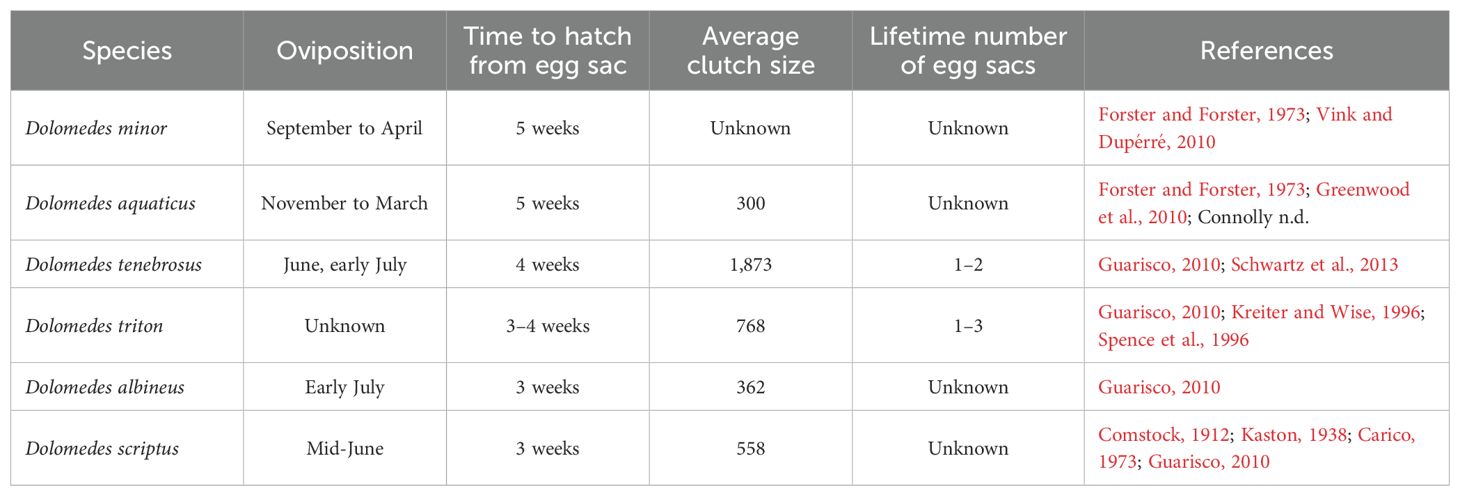
Table 3. General timelines for Dolomedes parental care behaviors and reproductive output. Some oviposition times may differ slightly depending on region.
Parental care in Dolomedes does not end at hatching, as females are infamous for fiercely guarding their young. If a threat approaches the nursery web, the female will aggressively approach and may be prompted to bite (Comstock, 1912). Much of the research on female aggression has addressed its benefits to female fecundity, but no studies have investigated the benefits of female aggression to offspring survival post-hatching.
5 Discussion
Although the genus Dolomedes boasts over 100 species, our review has revealed that detailed studies on most species are scarce. Our current understanding is predominantly shaped by knowledge of just four species from Europe and North America – D. fimbriatus, D. plantarius, D. triton, D. tenebrosus, although studies on several Asian (e.g., D. sulfurerus) and New Zealand (e.g., D. aquaticus) species are expanding (Figure 12). We particularly noticed an absence of research in Africa and Australia, where there is clearly much to learn. Regardless, our review has compiled rich insight into the biology of this genus. Our key conclusion is that Dolomedes spiders are an exceptional model group for exploring a wide range of ecological, evolutionary and conservation questions. With their near-global distribution and highly diverse ecology and behavior, Dolomedes make themselves available for collaborative and comparative research opportunities. Furthermore, their impressive size, ease of collection, and straightforward handling and rearing enhance their appeal to researchers.
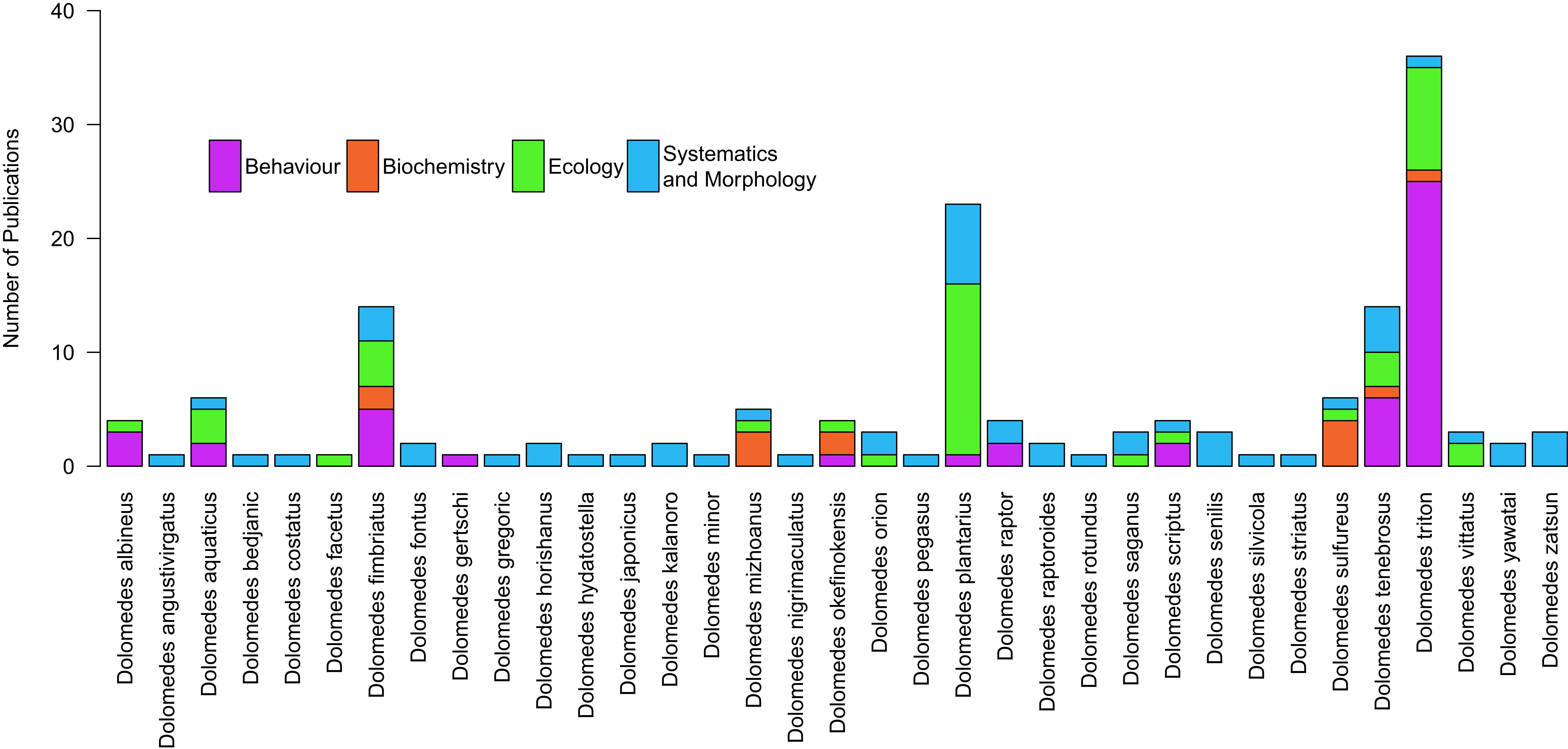
Figure 12. Number of research papers focusing on different Dolomedes species with color codon highlighting different research fields.
5.1 Systematics and morphology
We uncovered numerous avenues for further investigation regarding the systematics and morphology of Dolomedes. The recent placement of Dolomedes within the newly resurrected Dolomedidae family (Yu et al., 2024) marks a significant advancement in our understanding of their systematics, but many questions remain. Prioritizing the phylogenetic testing of the monophyly and the nomenclatural validity of Dolomedidae with increased taxon sampling is essential, along with clarifying their biogeographic history. If Miocene climatic oscillations have shaped their trait evolution and diversification, then Dolomedes can inform us of future biotic responses to global change. Expanding taxonomic descriptions to understudied regions (central Africa, Madagascar, India, Southeast Asia, and Oceania) is crucial for a more balanced understanding of their diversity. Further taxonomic discoveries will facilitate reconstruction of a more complete species-level phylogeny, strengthening evolutionary analyses, classification decisions and biogeographic reconstruction. Furthermore, the few South American Dolomedes are likely misplaced, leaving a continent curiously devoid of these almost globally distributed creatures – a biogeographic puzzle possibly explained by competition from earlier-arriving, semi-aquatic spiders.
We identified several unknowns regarding Dolomedes morphology. One key question is the adaptive function of color pattern variation within and among species. A comparative study mapping the occurrence of white lateral bands and their functions across species would provide valuable insights into the selective pressures driving and maintaining this variation. Studies on D. raptor suggest these bands play roles in both foraging and mate choice (Lin et al., 2015; Tso et al., 2016), but the extent to which this applies to other species is unknown. Furthermore, studies incorporating within-species variation would be powerful for untangling proximate and ultimate causes of polymorphism in body color patterns. Another set of morphological questions concerns the functional implications of divergent genital morphology in species, such as D. tenebrosus and D. okefinokensis, which also exhibit other traits such as eSSD and unusual mating systems. We suspect that this variation is driven by sexual conflict over mating optima, including gametic competition and choice, and look forward to future studies exploring these possibilities.
5.2 Ecology
The ecology of Dolomedes offers opportunities for insight into the interface between aquatic and terrestrial ecosystems and associated adaptations. Apparent microhabitat specialization coincident with indications of recent divergence (Yu et al., 2024) suggests that microhabitat specificity may have influenced diversification in Dolomedes; a hypothesis that requires testing. Numerous opportunities also exist to explore potential adaptations to aquatic versus terrestrial lifestyles, especially studies that focus on populations that vary in their aquatic/terrestrial lifestyle and/or species that change their microhabitat use throughout their life.
Often found at the boundary between freshwater and terrestrial ecosystems, Dolomedes are ideal for testing the effects of anthropogenic pressures on behavior, ecology and morphology. Early evidence suggests Dolomedes can serve as bioindicators of heavy metals in waterways (Ortega-Rodriguez et al., 2019) but there is significant potential to explore the impacts of a wider range of pollutants, including agrichemicals, pharmaceuticals and excess nutrients, on these key ecosystem predators and their prey. Additionally, many Dolomedes are found along urban-rural gradients, offering a natural transect to tease apart the pollutant impacts. Furthermore, the effects of global change on the behavioral ecology of animals are a rapidly growing area of research (Wong and Candolin, 2015). One area of interest has been the effect of pollutants on aquatic animal behavior, especially signaling and communication (reviewed in Saaristo et al., 2018). Although Dolomedes have yet to be studied from this perspective, observable courtship and reproductive behavior make them ideal candidates. They would also be a good taxon to explore for targeted citizen science data projects, like iNaturalist, for documenting distributions and diets (see Powell et al., 2021).
Seasonal variation in maturation times and sex ratios across Dolomedes makes them a potential model system for exploring the relationship between life history and reproductive traits. Sex ratios, for example, are theoretically and empirically linked to SSD across animals and often, to extreme reproductive behavior (Kuntner and Coddington, 2020). The causes and consequences of these relationships, however, remain obscure and require testing in a system with a strong hypothesis of evolutionary relationships coincident with species-level variation. We now have the former requirement (Yu et al., 2024), but unfortunately, the number of species for which we have behavioral and ecological data remains dismally low.
The often-high abundance and large size of Dolomedes make them inviting prey for birds and hosts for the growing larvae of pompilid wasps. The pattern of generalists and specialists in pompilid wasp parasitism observed in New Zealand and the USA likely varies in other regions, especially areas of high Dolomedes diversity such as Africa or Asia. Knowledge of prey for many pompilid species in Britain and Ireland are missing (O’Hanlon and O’Connor, 2021), and in New Zealand, there is call for more thorough documentation of pompilid prey (Thompson, 2020) as it may provide insight into species-level divergence in morphology, microhabitat use, and other behavior. Furthermore, the importance of predation pressure in microhabitat choice, phenology, activity cycles, and more remain to be explored. Such information will be especially informative when elucidating the conservation status of species and populations and when developing interventions that might mitigate their conservation risk.
These large spiders are not only prey, but are presumably important predators in their aquatic and terrestrial environments as well. Future directions related to their importance as predators could include molecular gut content analyses through metabarcoding to examine their role in ecosystems, including their potential role in controlling pest species.
5.3 Behavior
Fishing spiders have fascinated scientists for decades with their ability to move on water and detect surface vibrations. The literature on Dolomedes sensory capabilities is extensive, yet incomplete and provides a great basis for follow-up studies on sensory physiology, especially studies that compare across species with different lifestyles and those that focus explicitly on vision as well as sensory processing. Future research could also explore the biomechanics of submerging and re-emerging from water, and biomimetic studies of artificial water-surface locomotion may inspire innovative robotic designs.
Many opportunities exist to further investigate parental care behavior in Dolomedes. Studies could explore the metabolic or biomechanical costs of taking an egg sac underwater and how the tradeoff between reduced foraging and increased offspring survival during nursery web guarding varies by female size and species. Spence et al. (1996), for example, found that food availability increasingly restricts fecundity in D. triton as size increases.
Given our collective expertise in behavioral ecology, we identify Dolomedes as an excellent taxon for studying reproductive behavior, particularly sexual cannibalism. Investigating more species could help test hypotheses about the evolution and function of both pre- and post-copulatory cannibalism (Burke, 2024). While the role of aggressive spillover in pre-copulatory cannibalism remains uncertain (Johnson and Sih, 2005; Kralj-Fišer et al., 2013, Kralj-Fišer et al., 2016), there is substantial evidence that post-copulatory sexual cannibalism is adaptive, even for males (Schwartz et al., 2016). It would be valuable to address how sexual selection relates to sexual cannibalism rates and whether any other Dolomedes species shows male complicity in cannibalism. Dolomedes have also been at the forefront of personality research, as female aggression in foraging is highly repeatable and sometimes correlated with sexual aggressiveness. Additional research topics could include the role of sexual selection on sperm competition, paternal investment, and mating systems. Most current studies on reproductive behavior of spiders focus on web-building families. Studying Dolomedes, a free-roaming predator, will broaden our understanding of mating behavior and its dependence on other behavioral ecology and life-history traits.
Author contributions
K-PY: Writing – original draft, Writing – review & editing. ZR: Writing – original draft, Writing – review & editing. JK: Writing – original draft, Writing – review & editing. SC: Writing – original draft, Writing – review & editing. CV: Writing – original draft, Writing – review & editing. JJ: Writing – original draft, Writing – review & editing. SK-F: Writing – original draft, Writing – review & editing. MK: Writing – original draft, Writing – review & editing. EH: Writing – original draft, Writing – review & editing. CP: Writing – original draft, Writing – review & editing.
Funding
The author(s) declare financial support was received for the research, authorship, and/or publication of this article. CP was supported by the Marsden Fund, managed by the Royal Society Te Apārangi New Zealand (MFP-UOW2201). K-PY and MK have been supported by the Slovenian Research and Innovation Agency, grants P1-0255 and J1-50015 and Helse Vest (F-13096 / 10541-PSY Forskingsprosjekt).
Acknowledgments
We thank the editors of Frontiers in Arachnid Science for the invitation to the Horizons review collection. Thank you also to our various institute library staff for helping us track down some of the older publications.
Conflict of interest
The authors declare that the research was conducted in the absence of any commercial or financial relationships that could be construed as a potential conflict of interest.
Publisher’s note
All claims expressed in this article are solely those of the authors and do not necessarily represent those of their affiliated organizations, or those of the publisher, the editors and the reviewers. Any product that may be evaluated in this article, or claim that may be made by its manufacturer, is not guaranteed or endorsed by the publisher.
Supplementary material
The Supplementary Material for this article can be found online at: https://www.frontiersin.org/articles/10.3389/frchs.2024.1501653/full#supplementary-material
Supplementary Figure S1 | General somatic characteristics of Dolomedes featuring female D. fimbriatus (A) habitus, dorsal view; (B) idem, lateral view, red arrows showing the height differences between posterior carapace and eye region; (C) eye region, anterior view, white dot lines showing curvature of the eye rows and red arrow showing the separation between PER and AER. Scale bar: (A, B), 3 mm; (C), 1 mm. AER, anterior eye row; ALE, anterior lateral eye; AME, anterior median eye; PER, posterior eye row; PLE, posterior lateral eye; PME, posterior median eye.
Supplementary Figure S2 | Leg modifications and extra bristles (red arrows) in male Dolomedes: (A) D. triton, leg IV; (B) D. vittatus Walckenaer, 1837, leg IV; and (C) D. horishanus, leg IV. Scale bars: 1 mm.
Supplementary Figure S3 | Intraspecific variation of body coloration in Dolomedes sulfureus: (A) female, white banded morph; (B) female, dark/white band absent morph; (C) female, mottled brown morph; (D) male, white banded morph; (E) male, dark/white band absent morph.
Supplementary Table S1 | Valid Dolomedes species with known distribution ranges, habitat preferences, and SSD (as female to male size ratio). See attached excel file.
Footnotes
- ^ Smith, H. (2005). Fen raft spider recovery project: report for redgrave and lopham fen 2001–2005 (Unpublished report to Natural England). Available at: https://www.dolomedes.org.uk/conservation/Redgrave_Lopham_Fen.
References
Albo M. J., Bidegaray-Batista L., Bechsgaard J., Silva E. L. C., Bilde T., Pérez-Miles F. (2017). Molecular phylogenetic analyses show that Trechaleidae and Lycosidae are sister groups. Arachnology 17, 169–176. doi: 10.13156/arac.2017.17.4.169
Alves-Costa C. P., Da Fonseca G. A. B., Christófaro C. (2004). Variation in the diet of the brown-nosed coati (Nasua nasua) in southeastern Brazil. J. Mammalogy 85, 478–482. doi: 10.1644/1545-1542(2004)085<0478:VITDOT>2.0.CO;2
Anderson A. G., Hebets E. A. (2016). Benefits of size dimorphism and copulatory silk wrapping in the sexually cannibalistic nursery web spider. Pisaurina mira. Biol. Lett. 12, 20150957. doi: 10.1098/rsbl.2015.0957
Arnqvist G. (1992). Courtship behavior and sexual cannibalism in the semi-aquatic fishing spider, Dolomedes fimbriatus (Clerck) (Araneae: Pisauridae). J. Arachnology 20, 222–226. Available at: http://www.jstor.org/stable/3705884.
Arnqvist G., Henriksson S. (1997). Sexual cannibalism in the fishing spider and a model for the evolution of sexual cannibalism based on genetic constraints. Evolutionary Ecol. 11, 255–273. doi: 10.1023/A:1018412302621
Baba Y. G., Watari Y., Nishi M., Sasaki T. (2019). Notes on the feeding habits of the Okinawan fishing spider, Dolomedes orion (Araneae: Pisauridae), in the southwestern islands of Japan. J. Arachnology 47, 154–158. doi: 10.1636/0161-8202-47.1.154
Baillie A. L., Baillie S. R., Smith H. (2019). The heritability of lateral banding in. Dolomedes plantarius. Arachnology 18, 237–244. doi: 10.13156/arac.2019.18.3.237
Barnes W. J. P., Barth F. G. (1991). “Sensory control of locomotor mode in semi-aquatic spiders,” in Locomotor neural mechanisms in Arthropods and Vertebrates. Eds. Armstong D. M., Bush B. M. H. (Manchester University Press, Manchester), 105–116.
Bell J. R., Bohan D. A., Shaw E. M., Weyman G. S. (2005). Ballooning dispersal using silk: World fauna, phylogenies, genetics and models. Bull. Entomological Res. 95, 69–114. doi: 10.1079/ber2004350
Benson K., Suter R. B. (2013). Reflections on the tapetum lucidum and eyeshine in lycosoid spiders. J. Arachnology 41, 43–52. doi: 10.1636/B12-35.1
Bishop S. C. (1924). A revision of the pisauridae of the United States. New York State Museum Bull. 252, 1–140.
Bleckmann H., Barth F. G. (1984). Sensory ecology of a semi-aquatic spider (Dolomedes triton): II. The release of predatory behavior by water surface waves. Sociobiology 14, 303–312. doi: 10.1007/BF00299502
Bleckmann H., Bender M. (1987). Water surface waves generated by the male pisaurid spider Dolomedes triton (Walckenaer) during courtship behavior. Source: J. Arachnology 15, 363–369. Available at: https://www.jstor.org/stable/3705852.
Bleckmann H., Borchardt M., Horn P., Görner P. (1994). Stimulus discrimination and wave source localization in fishing spiders (Dolomedes triton and D. okefinokensis). J. Comp. Physiol. A 174, 305–316. doi: 10.1007/BF00240213
Bleckmann H., Lotz T. (1987). The vertebrate-catching behaviour of the fishing spider Dolomedes triton (Araneae, Pisauridae). Anim. Behav. 35, 641–651. doi: 10.1016/S0003-3472(87)80100-8
Bleckmann H., Rovner J. S. (1984). Behavioral ecology and sociobiology sensory ecology of a semi-aquatic spider (Dolomedes triton) I. Roles of vegetation and wind-generated waves in site selection. Behav. Ecol. Sociobiology 14, 297–301. doi: 10.1007/BF00299501
Blest A. D., Day W. A. (1978). The rhabdomere organization of some nocturnal pisaurid spiders in light and darkness. Philos. Trans. R. Soc. London. Ser. B Biol. Sci. 283, 1–23. doi: 10.1098/rstb.1978.0016
Boomsma J. J., Baer B., Heinze J. (2005). The evolution of male traits in social insects. Annu. Rev. Entomology 50, 395–420. doi: 10.1146/annurev.ento.50.071803.130416
Bösenberg W., Strand E. (1906). Japanische spinnen. Abhandlungen der Senckenbergischen Naturforschenden Gesellschaft 30, 93–422.
Buchholz S. (2010). Ground spider assemblages as indicators for habitat structure in inland sand ecosystems. Biodiversity Conserv. 19, 2565–2595. doi: 10.1007/s10531-010-9860-7
Büchs W. (2003). Biodiversity and agri-environmental indicators - General scopes and skills with special reference to the habitat level. Agriculture Ecosyst. Environ. 98, 35–78. doi: 10.1016/S0167-8809(03)00070-7
Burke N. W. (2024). Sexual cannibalism as a female resistance trait: a new hypothesis. Evolution 78, 612–623. doi: 10.1093/evolut/qpae017
Cabrera-Guzmán E., Crossland M. R., Shine R. (2015). Invasive cane toads as prey for native arthropod predators in tropical Australia. Herpetological Monogr. 29, 28–39. doi: 10.1655/HERPMONOGRAPHS-D-13-00007
Campbell J., Mills S., Paulin M. (2014). “Kinematic spider tracking with PoseCut,” in IVCNZ ‘14: proceedings of the 29th international conference on image and vision computing New Zealand (Association for Computing Machinery, New York). doi: 10.1145/2683405.2683441
Cargnelutti F., Bollatti F., Izquierdo M. A., Abregú D., Oviedo-Diego M., Vrech D., et al. (2023). Waking beauties: Mating quiescence in arachnid females. Adv. Study Behav. 55, 55–159. doi: 10.1016/bs.asb.2023.03.001
Carico J. E. (1973). The nearctic species of the genus dolomedes (Araneae: pisauridae). Bull. Museum Comp. Zoology 144, 435–488. Available at: https://biostor.org/reference/688.
Carico J. E., Holt P. C. (1964). A comparative study of the female copulatory apparatus of certain species in the spider genus Dolomedes (Pisauridae: Araneae). Terchnical Bull. 172, 1–27.
Chae J. H., Lee J. G., Lee J. H., Kim S. K. (2023). Taxonomic notes on Dolomedes nigrimaculatus (Araneae: Pisauridae) from Korea. Acta Arachnologica 72, 29–37. doi: 10.2476/asjaa.72.29
Chamberlin R. V. (1904). Notes on generic characters in the Lycosidae. Can. Entomologist 36, 145–148. doi: 10.4039/Ent36145-5
Clerck C. (1757). Svenska spindlar: uti sina hufvud-slaggter indelte samt under naigra och sextio sarskildte arter beskrefne: och med illuminerade figurer uplyste (Stockholmiae: Literis Laur. Salvii, MDCCLVII).
Collier K. J., Bury S., Gibbs M. (2002). A stable isotope study of linkages between stream and terrestrial food webs through spider predation. Freshw. Biol. 47, 1651–1659. doi: 10.1046/j.1365-2427.2002.00903.x
Comstock J. H. (1912). The spider book; a manual for the study of the spiders and their near relatives, the scorpions, pseudoscorpions, whipscorpions, harvestmen and other members of the class Arachnida, found in America north of Mexico, with analytical keys for their classification and popular accounts of their habits (New York: Garden City).
Correa-Garhwal S. M., Chaw R. C., Dugger T., Clarke T. H., Chea K. H., Kisailus D., et al. (2019). Semi-aquatic spider silks: Transcripts, proteins, and silk fibers of the fishing spider, Dolomedes triton (Pisauridae). Insect Mol. Biol. 28, 35–51. doi: 10.1111/imb.12527
Davidson N. C. (2014). How much wetland has the world lost? Long-term and recent trends in global wetland area. Mar. Freshw. Res. 65, 934–941. doi: 10.1071/MF14173
De Geer C. (1778). “Des araignées,” in Mémoires pour servir à l’histoire des insectes. Tome septième (Pierre Hesselberg, Stockholm), 176–324.
Deshefy G. S. (1981). Sailing behaviour in the fishing spider, Dolomedes triton (Walckenaer). Anim. Behav. 29, 965–966. doi: 10.1016/S0003-3472(81)80042-5
Dickel L., Monsimet J., Lafage D., Devineau O. (2022). Characterization of habitat requirements of European fishing spiders. PeerJ 10, e12806. doi: 10.1101/2020.12.13.422580
Duffey E. (2012). Dolomedes plantarius (Clerck 1757) (Araneae: Pisauridae): A reassessment of its ecology and distribution in Europe, with comments on its history at Redgrave and Lopham Fen, England. Bull. Br. Arachnological Soc. 15, 285–292. doi: 10.13156/arac.2012.15.1.285
Eberhard W. G., Huber B. A. (2010). “Spider genitalia,” in The evolution of primary sexual characters in animals. Eds. Leonard J., Cordoba A. (Oxford University Press, Oxford), 249–284.
Ehlers M. (1939). Untersuchungen uber Formen ak- tiver Lokomotion bei Spinnen. Zoologische Jahrbücher 72, 373–499.
Elias D. O., Maddison W. P., Peckmezian C., Girard M. B., Mason A. C. (2012). Orchestrating the score: Complex multimodal courtship in the Habronattus coecatus group of Habronattus jumping spiders (Araneae: Salticidae). Biol. J. Linn. Soc. 105, 522–547. doi: 10.1111/j.1095-8312.2011.01817.x
Evans H. E., Yoshimoto C. M. (1962). The ecology and nesting behavior of the Pompilidae (Hymenoptera) of the Northeastern United States. Miscellaneous Publications Entomological Soc. America 3, 67–119. doi: 10.4182/BWJG6430.3-3.67
Eversole C. (2022). Predation by a white banded fishing spider, Dolomedes albineus (Araneae: Pisauridae), on the green anole, Anolis carolinensis (Squamata: Dactyloidae), in southeastern Texas, USA. Herpetology Notes 15, 821–822.
Figiel C. R., Miller G. L. (1994). Effects of fish on the growth and survival of two fishing spider populations (Dolomedes triton; Araneae, Pisauridae). J. Arachnology 22, 185–189. Available at: https://www.jstor.org/stable/3705420.
Fisher A. M., Price T. A. R. (2019). Density-dependent aggression, courtship, and sex ratio in a fishing spider. Arachnology 18, 295–300. doi: 10.13156/arac.2019.18.3.295
Forster R., Forster L. (1973). New Zealand spiders - an introduction (Auckland and London: Collins).
Forster R., Forster L. (1999). Spiders of New Zealand and their worldwide kin (Dunedin, New Zealand: Otago University of Otago Press, in association with Otago Museum).
Fromhage L., Jacobs K., Schneider J. M. (2007). Monogynous mating behaviour and its ecological basis in the golden orb spider. Nephila fenestrata. Ethology 113, 813–820. doi: 10.1111/j.1439-0310.2007.01383.x
Frost C. M., Graham A. K., Spence J. R. (2013). Abiotic conditions rather than resource availability cues determine aerial dispersal behaviour in spiderlings of Dolomedes triton (Araneae: Pisauridae). Can. Entomologist 145, 29–39. doi: 10.4039/tce.2012.92
Gerdes A. B. M., Uhl G., Alpers G. W. (2009). Spiders are special: Fear and disgust evoked by pictures of arthropods. Evol. Hum. Behav. 30, 66–73. doi: 10.1016/j.evolhumbehav.2008.08.005
Giebel C. G. (1869). Über einige Spinnen aus Illinois. Z. für die Gesammten Naturwissenschaften 33, 248–253.
Gorb S. N., Barth F. G. (1994). Locomotor behavior during prey-capture of a fishing spider, Dolomedes plantarius (Araneae; Araneidae): Galloping and stopping. J. Arachnology 22, 89–93. Available at: https://biostor.org/reference/214588.
Goyen P. (1888). Descriptions of new species of New Zealand Araneae. Trans. Proc. New Z. Institute 20, 133–139.
Greenwood M. J., McIntosh A. R., Harding J. S. (2010). Disturbance across an ecosystem boundary drives cannibalism propensity in a riparian consumer. Behav. Ecol. 21, 1227–1235. doi: 10.1093/beheco/arq140
Griswold C. E. (1993). Investigations into the phylogeny of the lycosoid spiders and their kin (Arachnida: Araneae: Lycosoidea). Smithsonian Contributions to Zoology 539, 1–39. doi: 10.5479/si.00810282.539
Guarisco H. (2010). The fishing spider genus Dolomedes (Araneae: Pisauridae) in Kansas. Trans. Kansas Acad. Sci. 113, 35–43. doi: 10.1660/062.113.0202
Gunnarsson B. (2007). Bird predation on spiders: Ecological mechanisms and evolutionary consequences. J. Arachnology 35, 509–529. doi: 10.1636/RT07-64.1
Harris W. (1986). The breeding ecology of the South Island Fernbird in Otago wetlands. (Thesis). University of Otago.
Harris A. C. (1987). Pompilidae (Insecta: hymenoptera). Fauna N. Z. 12, 1–160. doi: 10.7931/J2/FNZ.12
Harris A. C. (1999). The life histories and nesting behaviour of the Pompilidae (Hymenoptera) in New Zealand: A comparative study. Species Diversity 4, 143–235. doi: 10.12782/specdiv.4.143
Haug J. T., Müller P., Haug C. (2018). The ride of the parasite: a 100-million-year old mantis lacewing larva captured while mounting its spider host. Zoological Lett. 4, 1–8. doi: 10.1186/s40851-018-0116-9
Hazzi N. A., Hormiga G. (2023). Molecular phylogeny of the tropical wandering spiders (Araneae, Ctenidae) and the evolution of eye conformation in the RTA clade. Cladistics 39, 18–42. doi: 10.1111/cla.12518
Heldingen P. J. (1993). Ecology and distribution of dolomedes in europe (Araneida: dolomedidae). Bolletino dell’Accademia Gioenia di Sci. Naturali 26, 181–187.
Hentz N. M. (1844). Descriptions and figures of the araneides of the United States. Boston J. Natural History 4, 386–396. doi: 10.5962/bhl.part.16998
Hentz N. M. (1845). Descriptions and figures of the araneides of the United States. Boston J. Natural History 5, 189–202.
Hu S., Niu Z., Chen Y., Li L., Zhang H. (2017). Global wetlands: Potential distribution, wetland loss, and status. Sci. Total Environ. 586, 319–327. doi: 10.1016/j.scitotenv.2017.02.001
Huber B. A. (2005). Sexual selection research on spiders: Progress and biases. Biol. Rev. Cambridge Philos. Soc. 80, 363–385. doi: 10.1017/S1464793104006700
Jaffé R., Pioker-Hara F. C., Dos Santos C. F., Santiago L. R., Alves D. A., De M. P., et al. (2014). Monogamy in large bee societies: A stingless paradox. Naturwissenschaften 101, 261–264. doi: 10.1007/s00114-014-1149-3
James C. D. (1991). Temporal variation in diets and trophic partitioning by coexisting lizards (Ctenotus: Scincidae) in central Australia. Oecologia 85, 553–561. doi: 10.1007/BF00323768
Ji Y. J., Smith H., Zhang D. X., Hewitt G. M. (2004). Ten polymorphic microsatellite DNA loci for paternity and population genetics analysis in the fen raft spider (Dolomedes plantarius). Mol. Ecol. Notes 4, 274–276. doi: 10.1111/j.1471-8286.2004.00639.x
Jiang L., Liu C., Duan Z., Deng M., Tang X., Liang S. (2013). Transcriptome analysis of venom glands from a single fishing spider. Dolomedes mizhoanus. Toxicon 73, 23–32. doi: 10.1016/j.toxicon.2013.07.005
Johnson J. C. (2001). Sexual cannibalism in fishing spiders (Dolomedes triton): An evaluation of two explanations for female aggression towards potential mates. Anim. Behav. 61, 905–914. doi: 10.1006/anbe.2000.1679
Johnson J. C. (2004). Cohabitation of juvenile females with mature males promotes sexual cannibalism in fishing spiders. Behav. Ecol. 16, 269–273. doi: 10.1093/beheco/arh152
Johnson J. C. (2005). The role of body size in mating interactions of the sexually cannibalistic fishing spider Dolomedes triton. Ethology 111, 51–61. doi: 10.1111/j.1439-0310.2004.01042.x
Johnson J. C., Sih A. (2005). Precopulatory sexual cannibalism in fishing spiders (Dolomedes triton): A role for behavioral syndromes. Behav. Ecol. Sociobiology 58, 390–396. doi: 10.1007/s00265-005-0943-5
Johnson J. C., Sih A. (2007). Fear, food, sex and parental care: a syndrome of boldness in the fishing spider. Dolomedes triton. Anim. Behav. 74, 1131–1138. doi: 10.1016/j.anbehav.2007.02.006
Jordan F., Jelks H. L., Kitchens W. M. (1994). ). Habitat use by the fishing spider Dolomedes triton in a northern Everglades wetland. Wetlands 14, 239–242. doi: 10.1007/BF03160661
Kaston B. J. (1936). The senses involved in the courtship of some vagabond spiders. Entomologica Americana 16, 97–166.
Kaston B. J. (1938). Mantispidae parasitic on spider egg sacs. J. New York Entomological Soc. 46, 147–153. Available at: https://www.jstor.org/stable/25004740.
Kishida K. (1936). A synopsis Japanese spiders genus Dolomedes. Acta Arachnologica 1, 114–127. doi: 10.2476/asjaa.1.114
Koch L. (1876). Die Arachniden Australiens, nach der Natur beschrieben und abgebildet (Nürnberg: Bauer & Raspe). Available at: https://biostor.org/reference/57305.
Koch L. (1878). Japanesische arachniden und myriapoden. Verhandlungen der Kaiserlich-Königlichen Zoologisch-Botanischen Gesellschaft Wien 27, 735–798.
Kochetkov D. N., Loktionov V. M. (2019). New and little known species of spider wasps (Hymenoptera: Pompilidae) from the Russian Far East. Far Eastern Entomologist 382, 1–9. doi: 10.25221/fee.382.1
Kosuge T., Sasaki T. (2002). Predation of the freshwater crab Geothelphusa minei, by the spider, Dolomedes raptor. Biol. Magazine Okinawa 40, 51–52.
Kozlov S. A., Lazarev V. N., Kostryukova E. S., Selezneva O. V., Ospanova E. A., Alexeev D. G., et al. (2014). Comprehensive analysis of the venom gland transcriptome of the spider Dolomedes fimbriatus. Sci. Data 1, 140023. doi: 10.1038/sdata.2014.23
Kralj-Fišer S., Čandek K., Lokovšek T., Čelik T., Cheng R. C., Elgar M. A., et al. (2016). Mate choice and sexual size dimorphism, not personality, explain female aggression and sexual cannibalism in raft spiders. Anim. Behav. 111, 49–55. doi: 10.1016/j.anbehav.2015.10.013
Kralj-Fišer S., Schneider J. M., Kuntner M. (2013). Challenging the aggressive spillover hypothesis: Is pre-copulatory sexual cannibalism a part of a behavioural syndrome? Ethology 119, 615–623. doi: 10.1111/eth.12111
Kreiter N., Wise D. H. (1996). Age-related changes in movement patterns in the fishing spider, Dolomedes triton (Araneae, Pisauridae). J. Arachnology 24, 24–33. Available at: https://www.jstor.org/stable/3705863.
Krombein K. V. (1979). “Family pompilidae,” in Catalog of hymenoptera in America north of Mexico (Washington: Smithsonian Institution Press), 1523–1570. doi: 10.5962/bhl.title.5074
Krupa J. J. (2002). Temporal shift in diet in a population of American bullfrog (Rana catesbeiana) in Carlsbad Caverns National Park. Southwestern Nat. 47, 461–467. doi: 10.2307/3672506
Kulkarni S., Wood H. M., Hormiga G. (2023). Advances in the reconstruction of the spider tree of life: A roadmap for spider systematics and comparative studies. Cladistics 39, 479–532. doi: 10.1111/cla.12557
Kuntner M. (2022). The seven grand challenges in arachnid science. Front. Arachnid Sci. 1. doi: 10.3389/frchs.2022.1082700
Kuntner M., Coddington J. A. (2020). Sexual size dimorphism: Evolution and perils of extreme phenotypes in spiders. Annu. Rev. Entomology 65, 57–80. doi: 10.1146/annurev-ento-011019-025032
Kurczewski F. E., Edwards G. B. (2012). Hosts, nesting behavior, and ecology of some North American spider wasps (Hymenoptera: Pompilidae). Southeastern Nat. 11, 1–71. doi: 10.1656/058.011.m401
Kurczewski F. E., Edwards G. B., Pitts J. P. (2017). Hosts, nesting behavior, and ecology of some North American spider wasps (Hymenoptera: Pompilidae), II. Southeastern Nat. 16, 1–82. doi: 10.1656/058.016.m901
Kurczewski F. E., Kiernan D. H. (2015). Analysis of spider wasp host selection in the Eastern Great Lakes Region (Hymenoptera: Pompilidae). Northeastern Nat. 22, 1–88. doi: 10.1656/045.022.m1101
Kurczewski F. E., Kurczewski E. J. (1972). Host records for some North American Pompilidae (Hymenoptera), Second Supplement. Tribe Pepsini. J. Kansas Entomol. Soc. 45, 181–193.
Latreille P. A. (1804). Tablau methodique des Insectes. Nouveau Dictionnaire d’Histoire Naturelle Paris 24, 129–295.
Lattimore V. L., Vink C. J., Paterson A. M., Cruickshank R. H. (2011). Unidirectional introgression within the genus Dolomedes (Araneae: Pisauridae) in Southern New Zealand. Invertebrate Systematics 25, 70–79. doi: 10.1071/IS11001
Leech R., Buckle D. J. (1987). The first records of Dolomedes striatus Giebel in Alberta and Saskatchewan (Araneida: Pisauridae). Can. Entomologist 119, 1143–1144. doi: 10.4039/Ent1191143-12
Lehtinen P. T. (1967). Classification of the cribellate spiders and some allied families, with notes on the evolution of the suborder Araneomorpha. Annales Zoologici Fennici 4, 199–468. Available at: https://www.jstor.org/stable/23731560.
Leivers S. J., Lee E. H., Fuller N. W. (2021). Tri-colored bat (Perimyotis subflavus) predation by a dark fishing spider (Dolomedes tenebrosus) in East Texas. Southeastern Nat. 20, N98–N101. doi: 10.1656/058.020.0312
Leroy B., Bellard C., Dubos N., Colliot A., Vasseur M., Courtial C., et al. (2014). Forecasted climate and land use changes, and protected areas: The contrasting case of spiders. Diversity Distributions 20, 686–697. doi: 10.1111/ddi.12191
Leroy B., Paschetta M., Canard A., Bakkenes M., Isaia M., Ysnel F. (2013). First assessment of effects of global change on threatened spiders: Potential impacts on Dolomedes plantarius (Clerck) and its conservation plans. Biol. Conserv. 161, 155–163. doi: 10.1016/j.biocon.2013.03.022
Li J., Li D., Zhang F., Wang H., Yu H., Liu Z., et al. (2014). A comparative study of the molecular composition and electrophysiological activity of the venoms from two fishing spiders Dolomedes mizhoanus and Dolomedes sulfurous. Toxicon 83, 35–42. doi: 10.1016/j.toxicon.2014.02.014
Lin T. S., Zhang S., Liao C. P., Hebets E. A., Tso I. M. (2015). A dual function of white coloration in a nocturnal spider Dolomedes raptor (Araneae: Pisauridae). Anim. Behav. 108, 25–32. doi: 10.1016/j.anbehav.2015.07.001
Loc-Barragán J. L., Carbajal-Márquez R. A., Domínguez de la Riva M. A. (2017). Other contributions: Nature notes: Amphibia: Anura. Lithobates forreri (Boulenge). Diet. Mesoamerican Herpetology 4, 622–623.
MacKinlay R. D., Shaw R. C. (2023). A systematic review of animal personality in conservation science. Conserv. Biol. 37, e13935. doi: 10.1111/cobi.13935
Magalhaes I. L. F., Azevedo G. H. F., Michalik P., Ramírez M. J. (2020). The fossil record of spiders revisited: implications for calibrating trees and evidence for a major faunal turnover since the Mesozoic. Biol. Rev. 95, 184–217. doi: 10.1111/brv.12559
Mammola S., Malumbres-Olarte J., Arabesky V., Barrales-Alcalá D. A., Barrion-Dupo A. L., Benamú M. A., et al. (2022). The global spread of misinformation on spiders. Curr. Biol. 32, R871–R873. doi: 10.1016/j.cub.2022.07.026
Manicom C., Schwarzkopf L. (2011). Diet and prey selection of sympatric tropical skinks. Austral Ecol. 36, 485–496. doi: 10.1111/j.1442-9993.2010.02181.x
Martin N. (2012). Cryptocheilus australis (Guéri) (Hymenoptera: Pompilidae): A new spider host. Wētā 43, 29–32.
McAlister W. H. (1960). The diving and surface-walking behavior of Dolomedes triton sexpunctatus (Araneida: Pisauridae). Anim. Behav. 8, 109–111. doi: 10.1016/0003-3472(60)90017-8
McCormick K. D., Kobayashi K., Goldin S. M., Laxma Reddy N., Meinwalda J. (1993). Characterization and synthesis of a new calcium from the venom of a fishing spider. Tetrahedron 49, 11155–11168. doi: 10.1016/S0040-4020(01)81803-2
Meinwaldt J., Eisnert T. (1995). Chemical ecology: The chemistry of biotic interaction. PNAS 92, 14–18.
Michalko R., Pekár S., Entling M. H. (2019). An updated perspective on spiders as generalist predators in biological control. Oecologia 189, 21–36. doi: 10.2307/48702285
Milano F., Blick T., Cardoso P., Chatzaki M., Fukushima C. S., Gajdoš P., et al. (2021). Spider conservation in Europe: a review. Biol. Conserv. 256, 109020. doi: 10.1016/j.biocon.2021.109020
Milano F., Cardoso P., Mammola S., Smith H., Isaia M. (2022). Trends in habitat suitability and conservation status of aquatic spiders in Europe. Biol. Conserv. 275, 109767. doi: 10.1016/j.biocon.2022.109767
Miller J. A. (2007). Repeated evolution of male sacrifice behavior in spiders correlated with genital mutilation. Evolution 61, 1301–1315. doi: 10.1111/j.1558-5646.2007.00115.x
Mitra S., Wassmann R., Vlek P. L. G. (2003). “Global inventory of Wetlands and their role in the carbon cycle,” in ZEF discussion papers on development policy (Zentrum für Entwicklungsforschung (ZEF) Center for Development Research, Bonn), 1–44. doi: 10.22004/ag.econ.18771
Miyashita K. (1986). Developmental retardation in Dolomedes sulfureus L. Koch (Araneae: Pisauridae) under short photoperiod. Acta Arachnologica 35, 11–14. doi: 10.2476/asjaa.35.11
Monsimet J., Devineau O., Pétillon J., Lafage D. (2020). Explicit integration of dispersal-related metrics improves predictions of SDM in predatory arthropods. Sci. Rep. 10, 16668. doi: 10.1101/2020.06.05.136044
Monsimet J., Pétillon J., Devineau O., Gardoni N., Bataillard L., Lafage D. (2022). Contrasted propensity for waterborne and airborne dispersal between two closely related semi-aquatic spider species. Insect Conserv. Diversity 15, 704–713. doi: 10.1111/icad.12596
Moore M. K., Townsend V. R. (1998). The interaction of temperature, dissolved oxygen and predation pressure in an aquatic predator-prey system. Oikos 81, 329–336. doi: 10.2307/3547053
Nakajo R. (2024). Phenology of dolomedes in the hills of bōsō Peninsula. Bull. Tokyo Spider Study Group 124, 7–11.
Nelson X. J. (2023). A road map of jumping spider behavior. J. Arachnology 51, 139–154. doi: 10.1636/JoA-S-22-011
Newman J. A., Elgar M. A. (1991). Sexual cannibalism in orb-weaving spiders: An economic model. Am. Nat. 138, 1372–1395. doi: 10.1086/285292
Nicholas A. C., Stratton G. E., Reed D. H. (2011). Determinants of differential reproductive allocation in wolf and nursery-web spiders. J. Arachnology 39, 139–146. doi: 10.1636/Hi10-62.1
Nyffeler M., Birkhofer K. (2017). An estimated 400-800 million tons of prey are annually killed by the global spider community. Sci. Nat. 104, 30. doi: 10.1007/s00114-017-1440-1
Nyffeler M., Pusey B. J. (2014). Fish predation by semi-aquatic spiders: A global pattern. PloS One 9, e99459. doi: 10.1371/journal.pone.0099459
O’Hanlon A., O’Connor J. P. (2021). The spider-hunting wasps of Ireland (Hymenoptera: Pompilidae). A review of the species, their natural history and recorded distribution. Biol. Environ. 121B, 61–82. doi: 10.3318/BIOE.2021.06
Ono H. (2002). New and remarkable spiders of the families Liphistiidae, Argyronetidae, Pisauridae, Theridiidae and Araneidae (Arachnida) from Japan. Bull. Natl. Museum Nat. Sci. Tokyo 28, 51–60.
Ono H. (2009). The spiders of Japan with keys to the families and genera and illustrations of the species (Kanagawa: Tokai University Press).
Ortega-Rodriguez C. L., Chumchal M. M., Drenner R. W., Kennedy J. H., Nowlin W. H., Barst B. D., et al. (2019). Relationship between Methylmercury contamination and proportion of aquatic and terrestrial prey in diets of shoreline spiders. Environ. Toxicol. Chem. 38, 2503–2508. doi: 10.1002/etc.4579
Oxbrough A. G., Gittings T., O’Halloran J., Giller P. S., Smith G. (2005). Structural indicators of spider communities across the forest plantation cycle. F. For. Ecol. Manage. 212, 171–183. doi: 10.1016/j.foreco.2005.03.040
Pagani-Núñez E., Ruiz Í., Quesada J., Negro J. J., Senar J. C. (2011). The diet of Great Tit Parus major nestlings in a Mediterranean Iberian forest: the important role of spiders. Anim. Biodiversity Conserv. 34, 355–361. doi: 10.32800/abc.2011.34.0355
Parker K. A. (2002). Ecology and management of North Island fernbird (Bowdleria punctata vealeae). (Masters of Science thesis). Auckland, New Zealand: The University of Auckland.
Pearson D. L. (1994). Selecting indicator taxa for the quantitative assessment of biodiversity. Philos. Trans. R. Soc. B: Biol. Sci. 345, 75–79. doi: 10.1098/rstb.1994.0088
Piacentini L. N., Ramírez M. J. (2019). Hunting the wolf: A molecular phylogeny of the wolf spiders (Araneae, Lycosidae). Mol. Phylogenet. Evol. 136, 227–240. doi: 10.1016/j.ympev.2019.04.004
Pocock R. I. (1903). Some new spiders from the Camaroons collected by Mr. G. L. Bates. Ann. Magazine Natural History 11, 258–264. doi: 10.1080/00222930308678762
Polidori C., Federici M., Pesarini C., Andrietti F. (2007). Factors affecting spider prey selection by Sceliphron mud-dauber wasps (Hymenoptera: Sphecidae) in Northern Italy. Anim. Biol. 57, 11–28. doi: 10.1163/157075607780002005
Powell E. C., Painting C. J., Hickey A. J., MaChado G., Howell G. (2021). Diet, predators, and defensive behaviors of New Zealand harvestmen (Opiliones: Neopilionidae). I. J. Arachnology 49, 122–140. doi: 10.1636/joa-s-20-002
Rajmohana K. (2017). “Insecta: hymenoptera (Aquatic),” in Current status of freshwater faunal diversity in India. Eds. Chandra K., Gopi K. C., Rao D. V., Valarmathi K., Alfred J. R. B. (Zoological Survey of India, Kolkata), 487–499.
Raposo do Amaral F., Neves L. G., Resende M. F. R., Mobili F., Miyaki C. Y., Pellegrino K. C. M., et al. (2015). Ultraconserved elements sequencing as a low-cost source of complete mitochondrial genomes and microsatellite markers in non-model amniotes. PloS One 10, e0138446. doi: 10.1371/journal.pone.0138446
Raven R., Hebron W. (2018). A review of the water spider family Pisauridae in Australia and New Caledonia with descriptions of four new genera and 23 new species. Memoirs Queensland Museum Nat. 60, 233–381. doi: 10.17082/j.2204-1478.60.2018.2017-06
Réale D., Reader S. M., Sol D., McDougall P. T., Dingemanse N. J. (2007). Integrating animal temperament within ecology and evolution. Biol. Rev. 82, 291–318. doi: 10.1111/j.1469-185X.2007.00010.x
Richards O. W., Hamm A. H. (1939). The biology of the british pompilidae (Hymenoptera). Trans. Soc. Br. Entomology 6, 51–114.
Richman D. B., Jackson R. (1992). A review of the ethology of jumping spiders (Araneae, Salticidae). Bull. Br. arachnological Soc. 9, 33–37.
Roble S. (1985). Submergent capture of Dolomedes triton (Araneae, Pisauridae) by Anoplius depressipes (Hymenoptera, Pompilidae). J. Arachnology 13, 391–392. Available at: https://www.jstor.org/stable/3705324.
Roewer C. F. (1955). Araneae Lycosaeformia I. (Agelenidae, Hahniidae, Pisauridae) mit Berücksichtigung aller Arten der äthiopischen Region. Explor. du Parc Natl. l’Upemba Mission G. F. Witte 30, 1–420.
Roggenbuck H., Pekár S., Schneider J. M. (2011). Sexual cannibalism in the European garden spider Araneus diadematus: The roles of female hunger and mate size dimorphism. Anim. Behav. 81, 749–755. doi: 10.1016/j.anbehav.2011.01.005
Roland C., Rovner J. S. (1983). Chemical and vibratory communication in the aquatic pisaurid spider Dolomedes triton. J. Arachnology 11, 77–85. Available at: https://www.jstor.org/stable/3705268.
Saaristo M., Brodin T., Balshine S., Bertram M. G., Brooks B. W., Ehlman S. M., et al. (2018). Direct and indirect effects of chemical contaminants on the behaviour, ecology and evolution of wildlife. Proc. R. Soc. B: Biol. Sci. 285, 20181297. doi: 10.1098/rspb.2018.1297
Santos A. J. (2007). A phylogenetic analysis of the nursery-web spider family Pisauridae, with emphasis on the genera Architis and Staberius (Araneae: Lycosoidea). Zoologica Scripta 36, 489–507. doi: 10.1111/j.1463-6409.2007.00291.x
Schmidt G. (1957). Einige notizen über Dolomedes fimbriatus (Cl.). Zoologischer Anzeiger 158, 83–97.
Schneider J., Fromhage L. (2010). “Monogynous mating strategies in spiders,” in Animal behaviour: evolution and mechanisms (Springer Berlin Heidelberg, Berlin, Heidelberg). doi: 10.1007/978-3-642-02624-9
Schoenberg D., Hebets E. A., Sullivan-Beckers L. (2022). Mating and cannibalism dynamics of the fishing spider Dolomedes scriptus Hent(Araneae: pisauridae). J. Arachnology 50, 56–66. doi: 10.1636/JoA-S-20-024
Schulz M. (2000). Diet and foraging behavior of the golden-tipped bat, Kerivoula papuensis: A spider specialist? J. Mammalogy 81, 948–957. doi: 10.1644/1545-1542(2000)081<0948:DAFBOT>2.0.CO;2
Schwartz S. K., Wagner W. E., Hebets E. A. (2013). Spontaneous male death and monogyny in the dark fishing spider. Biol. Lett. 9. doi: 10.1098/rsbl.2013.0113
Schwartz S. K., Wagner W. E., Hebets E. A. (2014). Obligate male death and sexual cannibalism in dark fishing spiders. Anim. Behav. 93, 151–156. doi: 10.1016/j.anbehav.2014.04.037
Schwartz S. K., Wagner W. E., Hebets E. A. (2016). Males can benefit from sexual cannibalism facilitated by self-sacrifice. Curr. Biol. 26, 2794–2799. doi: 10.1016/j.cub.2016.08.010
Scott C. E., Anderson A. G., Andrade M. C. B. (2018). A review of the mechanisms and functional roles of male silk use in spider courtship and mating. J. Arachnology 46, 173–206. doi: 10.1636/JoA-S-17-093.1
Scott K. R., Dillard Z. W., Foltz D. A., Loughman Z. J. (2016). Predation of the freshwater crayfish Orconectes cristavarius by the fishing spider. Dolomedes scriptus. Southeastern Nat. 15, N1–N3. doi: 10.1656/058.015.0317
Shimizu A. (1992). Nesting behavior of the semi-aquatic spider wasp, Anoplius eous, which transports its prey on the surface film of water (Hymenoptera, Pompilidae). J. Ethology 10, 85–102. doi: 10.1007/BF02350113
Shultz J. W. (1987). Walking and surface film locomotion in terrestrial and semi-aquatic spiders. J. Exp. Biol. 128, 427–444. doi: 10.1242/jeb.128.1.427
Sierwald P. (1989). Morphology and ontogeny of female copulatory organs in American Pisauridae, with special reference to homologous features (Arachnida: Araneae). Smithsonian Contributions to Zoology 484, 1–24. doi: 10.5479/si.00810282.484
Sierwald P. (1990). Morphology and homologous features in the male palpal organ in Pisauridae and other spider families, with notes on the taxonomy of Pisauridae (Arachnida: Araneae). Nemouria Occasional Papers Delaware Museum Natural History 35, 1–59. Available at: https://biostor.org/reference/143506.
Sierwald P., Coddington J. A. (1988). Functional aspects of the male palpal organs in Dolomedes tenebrosus, with notes on the mating behavior (Araneae, Pisauridae). J. Arachnology 16, 262–265. Available at: https://www.jstor.org/stable/3705762.
Sih A., Mathot K. J., Moirón M., Montiglio P. O., Wolf M., Dingemanse N. J. (2015). Animal personality and state-behaviour feedbacks: A review and guide for empiricists. Trends Ecol. Evol. 30, 50–60. doi: 10.1016/j.tree.2014.11.004
Silva E. L. C., Gibbons A., Sierwald P. (2015). Description of the male of Dolomedes raptoroides Zhang, Zhu & Son(Araneae: lycosoidea: pisauridae). Zootaxa 3946, 139–145. doi: 10.11646/zootaxa.3946.1.8
Silva E. L. C., Griswold C. E. (2013). A new species of Dolomedes Latreill(Araneae: lycosoidea: pisauridae) from Madagascar. Zootaxa 3691, 461–466. doi: 10.11646/zootaxa.3691.4.5
Simon E. (1884). Description d’une nouvelle famille de l’ordre des Araneae (Bradystichidae). Annales la Société Entomologique Belgique 28, 297–301.
Simon E. (1890). Etudes arachnologiques. 22e Mémoire. XXXIV. Etude sur les arachnides de l’Yemen. Annales la Société Entomologique France 10, 77–124.
Simon E. (1899). Ergebnisse einer Reise nach dem Pacific (Schauinsland 1896-1897). Arachnoideen. Zoologische JahrbücherAbtheilung für Systematik Geographie und Biologie der Thiere 12, 411–437. doi: 10.5962/bhl.part.2034
Sirvid P. J., Vink C. J., Fitzgerald B. M., Wakelin M. D., Rolfe J., Michel P. (2021). Conservation status of New Zealand araneae (spiders), 2020. New Z. Threat Classification Ser. 33, 1–33. doi: 10.1080/00222933.2020.1781950
Smith H. (1996). “The status and management of Dolomedes plantarius on Redgrave and Lopham Fen National Nature Reserve in 1996,” in English nature research reports 214 (English Nature, Peterborough).
Smith H. (2000). The status and conservation of the fen raft spider (Dolomedes plantarius) at Redgrave and Lopham Fen National Nature Reserve, England. Biol. Conserv. 95, 153–164. doi: 10.1016/S0006-3207(00)00030-6
Smith H. (2020). The Fen Raft Spider – from unknown past to uncertain future. Br. Wildlife 32, 98–109.
Smith H., Clarke D., Heaver D., Hughes I., Pearce-Kelly P., Sainsbury T. (2013). “Translocation and augmentation of the fen raft spider populations in the UK,” in Global Re-introduction Perspectives: 2013. Further case studies from around the globe. Ed. Soorae P. (Gland: IUCN/SSC Re-introduction Specialist Group and Abu Dhabi, UAE: Environment Agency-Abu Dhabi), 1–6.
Song D. X., Chen Z. F. (1991). A new species of the genus Dolomedes from Zhejiang, China. Acta Zootaxonomica Sin. 16, 15–17.
Spence J. R., Zimmermann M., Wojcicki J. P. (1996). Effects of food limitation and sexual cannibalism on reproductive output of the nursery web spider Dolomedes triton (Araneae: Pisauridae). Oikos 75, 373–382. doi: 10.2307/3545877
Starrett J., McGinley R. H., Hebets E. A., Bond J. E. (2022). Phylogeny and secondary sexual trait evolution in Schizocosa wolf spiders (Araneae, Lycosidae) shows evidence for multiple gains and losses of ornamentation and species delimitation uncertainty. Mol. Phylogenet. Evol. 169, 107397. doi: 10.1016/j.ympev.2022.107397
Steinhoff P. O. M., Harzsch S., Uhl G. (2023). Comparative neuroanatomy of the central nervous system in web-building and cursorial hunting spiders. J. Comp. Neurol. 532, e25554. doi: 10.1002/cne.25554
Stratton G. E., Suter R. B., Miller P. R. (2004). Evolution of water surface locomotion by spiders: a comparative approach. Biol. J. Linn. Soc. 81, 63–78. doi: 10.1111/j.1095-8312.2004.00269.x
Suter R. B. (1999). Cheap transport for fishing spiders (Araneae, Pisauridae): The physics of sailing on the water surface. J. Arachnology 27, 489–496. Available at: https://www.jstor.org/stable/3706047.
Suter R. B. (2003). Trichobothrial mediation of an aquatic escape response: Directional jumps by the fishing spider, Dolomedes triton, foil frog attacks. J. Insect Sci. 3, 19. doi: 10.1673/031.003.1901
Suter R. B. (2013). Spider locomotion on the water surface: Biomechanics and diversity. J. Arachnology 41, 93–101. doi: 10.1636/M13-14
Suter R. B., Gruenwald J. (2000a). Predator avoidance on the water surface? Kinematics and efficacy of vertical jumping by Dolomedes (Araneae, Pisauridae). J. Arachnology 28, 201–210. doi: 10.1636/0161-8202(2000)028[0201:PAOTWS]2.0.CO;2
Suter R. B., Gruenwald J. (2000b). Spider size and locomotion on the water surface (Araneae, Pisauridae). J. Arachnology 28, 300–308. doi: 10.1636/0161-8202(2000)028[0300:SSALOT]2.0.CO;2
Suter R. B., Wildman H. (1999). Locomotion on the water surface: Hydrodynamic constraints on rowing velocity require a gait change. J. Exp. Biol. 202, 2771–2785. doi: 10.1242/jeb.202.20.2771
Tanikawa A. (2003). Two new species and two newly recorded species of the spider family Pisauridae (Arachnida: Araneae) from Japan. Acta Arachnologica 52, 35–42. doi: 10.2476/asjaa.52.35
Tanikawa A. (2012). Further notes on the spiders of the genus Dolomedes (Araneae: Pisauridae) from Japan. Acta Arachnologica 61, 11–17. doi: 10.2476/asjaa.61.11
Tanikawa A., Miyashita T. (2008). A revision of Japanese spiders of the genus Dolomedes (Araneae: Pisauridae) with its phylogeny based on mt-DNA. Acta Arachnologica 57, 19–35. doi: 10.2476/asjaa.57.19
Tso I. M., Zhang S., Tan W. L., Peng P., Blamires S. J. (2016). Prey luring coloration of a nocturnal semi-aquatic predator. Ethology 122, 671–681. doi: 10.1111/eth.12512
Uzenbaev S. D., Lyabzina S. N. (2009). An experimental study of the effects of spider venom on animals. Entomological Rev. 89, 479–486. doi: 10.1134/S0013873809040125
Van Helsdingen P. J. (2005). “Characteristic spider species of peat bog fenlands in the Netherlands (Araneae),” in European arachnology 2005. Eds. Deltshev C., Stoev P. (Acta zoologica bulgarica, Blagoevgrad), 115–124.
Vink C. J., Dupérré N. (2010). Pisauridae (Arachnida: araneae). Fauna New Z. 64, 1–60. doi: 10.7931/J2/FNZ.64
Walckenaer C. A. (1802). Faune parisienne. Insectes. Ou histoire abrégée des insectes de environs de Paris, classés d’après le système de Fabricius (Paris: Dentu).
Walckenaer C. A. (1837). Histoire naturelle des insectes. Aptères (Paris: Librairie encyclopédique de Roret).
Wang Z. L., Huang J., Li M. Y., Yu X. P. (2020). The complete mitochondrial genome of a nursery-web spider Dolomedes angustivirgatus (Araneae: Pisauridae). Mitochondrial DNA Part B 5, 1695–1696. doi: 10.1080/23802359.2020.1748548
Wang H., Zhang F., Li D., Xu S., He J., Yu H., et al. (2013). The venom of the fishing spider Dolomedes sulfurous contains various neurotoxins acting on voltage-activated ion channels in rat dorsal root ganglion neurons. Toxicon 65, 68–75. doi: 10.1016/j.toxicon.2013.01.014
Wheeler W. C., Coddington J. A., Crowley L. M., Dimitrov D., Goloboff P. A., Griswold C. E., et al. (2017). The spider tree of life: phylogeny of Araneae based on target-gene analyses from an extensive taxon sampling. Cladistics 33, 574–616. doi: 10.1111/cla.12182
Wilder S. M., Rypstra A. L. (2008). Sexual size dimorphism predicts the frequency of sexual cannibalism within and among species of spiders. Am. Nat. 172, 431–440. doi: 10.1086/589518
Williams D. S. (1979a). The feeding behaviour of New Zealand Dolomedes species (Araneae: Pisauridae). N Z J. Zool 6, 95–105. doi: 10.1080/03014223.1979.10428352
Williams D. S. (1979b). The physiological optics of a nocturnal semi-aquatic spider, Dolomedes aquaticus (Pisauridae). Z. fur Naturforschung C 34, 463–469. doi: 10.1515/znc-1979-5-625
Wojcicki J. P. (1992). A description of the reproductive biology of the fishing spider Dolomedes triton (Walck.) (Araneae: Pisuridae) in central Alberta: University of Alberta).
Wong B. B. M., Candolin U. (2015). Behavioral responses to changing environments. Behav. Ecol. 26, 665–673. doi: 10.1093/beheco/aru183
World Conservation Monitoring Centre (1996). olomedes plantarius. The IUCN Red List of Threatened Species 1996. Available online at: https://dx.doi.org/10.2305/IUCN.UK.1996.RLTS.T6790A12806270.en (Accessed August 13, 2024).
World Spider Catalog (2024). World spider catalog. Version 25.5 (Bern, Switzerland: Natural History Museum Bern). doi: 10.24436/2
Xu X., Wang H., Zhang F., Hu Z., Liang S., Liu Z. (2015). A comparative analysis of the venom gland transcriptomes of the fishing spiders Dolomedes mizhoanus and Dolomedes sulfurous. PloS One 10, e0139908. doi: 10.1371/journal.pone.0139908
Yu K. P., Cheng R. C., Haddad C. R., Tanikawa A., Pett B. L., Piacentini L. N., et al. (2024). Phylogenomics, classification, and lifestyle evolution in raft- and nursery web- spiders (Araneae: dolomedidae and pisauridae). bioRxiv. [Preprint]. doi: 10.1101/2024.08.23.609317
Yu K.-P., Kuntner M. (2024). Discovering unknown Madagascar biodiversity: integrative taxonomy of raft spiders (Pisauridae: Dolomedes). PeerJ 12, e16781. doi: 10.7717/peerj.16781
Zachos J., Pagani M., Sloan L., Thomas E., Billups K. (2001). Trends, rhythms, and aberrations in global climate 65 Ma to present. Sci. (1979) 292, 686–693. doi: 10.1126/science.1059412
Zander A. (2016). Interactions of “Sarracenia purpurea” and its inquilines: Comparing Europe and North America (Fribourg, Switzerland: Université de Fribourg).
Zedler J. B., Kercher S. (2005). Wetland resources: Status, trends, ecosystem services, and restorability. Annu. Rev. Environ. Resour. 30, 39–74. doi: 10.1146/annurev.energy.30.050504.144248
Zhang S., Kuntner M., Li D. (2011). Mate binding: Male adaptation to sexual conflict in the golden orb-web spider (Nephilidae: Nephila pilipes). Anim. Behav. 82, 1299–1304. doi: 10.1016/j.anbehav.2011.09.010
Zhang J. X., Zhu M. S., Song D. X. (2004). A review of the Chinese nursery-web spiders (Araneae: Pisauridae). J. Arachnology 32, 353–417. doi: 10.1636/M02-58
Zimmermann M., Spence J. R. (1989). Prey use of the fishing spider Dolomedes triton (Pisauridae, Araneae): An important predator of the neuston community. Oecologia 80, 187–194. doi: 10.1007/BF00380149
Zimmermann M., Spence J. R. (1992). Adult population dynamics and reproductive effort of the fishing spider Dolomedes triton (Araneae, Pisauridae) in central Alberta. Can. J. Zoology 70, 2224–2233. doi: 10.1139/z92-299
Keywords: raft spiders, behavioral ecology, diversity, physiology, evolution, conservation, Dolomedidae
Citation: Yu K-P, Roithmair Z, Kurovski J, Connolly SJ, Vink CJ, Johnson JC, Kralj-Fišer S, Kuntner M, Hebets EA and Painting CJ (2024) Dolomedes fishing spider biology: gaps and opportunities for future research. Front. Arachn. Sci. 3:1501653. doi: 10.3389/frchs.2024.1501653
Received: 25 September 2024; Accepted: 28 October 2024;
Published: 25 November 2024.
Edited by:
Yukie Sato, University of Tsukuba, JapanReviewed by:
Jimmy Cabra-García, University of the Valley, ColombiaHelen Smith, British Arachnological Society, United Kingdom
Copyright © 2024 Yu, Roithmair, Kurovski, Connolly, Vink, Johnson, Kralj-Fišer, Kuntner, Hebets and Painting. This is an open-access article distributed under the terms of the Creative Commons Attribution License (CC BY). The use, distribution or reproduction in other forums is permitted, provided the original author(s) and the copyright owner(s) are credited and that the original publication in this journal is cited, in accordance with accepted academic practice. No use, distribution or reproduction is permitted which does not comply with these terms.
*Correspondence: Christina J. Painting, Y2hyaXNzaWUucGFpbnRpbmdAd2Fpa2F0by5hYy5ueg==
†These authors have contributed equally to this work and share first authorship