- 1Research Faculty of Agriculture, Hokkaido University, Sapporo, Japan
- 2Institute of Life and Environmental Sciences/Mountain Science Center, University of Tsukuba, Tsukuba, Japan
Phytophagous spider mites, belonging to the subfamily Tetranychinae, have diverse lifestyles. Life type is an important concept for understanding spider mite diversity. There are three basic life types: little web (LW), non-systematic and complicated web (CW), and woven nest (WN), which are characterised by silk production, silk usage patterns, faeces defaecation patterns, and other behavioural outcomes. In this review, we first address the diversity of life types and then discuss the functions of life types as reported by several studies. The primary function of the CW type is to provide a firm footing for residing on the underside of host leaves and to protect mites from the deleterious effects of solar radiation. Next, we discuss how spider mites have evolved various defence mechanisms through life types to avoid their natural enemies. We observed that the life type can affect the results of interspecies competition. Faeces manipulation behaviours observed in the CW and WN type mites may be an adaptation to keep a clean habitat. The CW species showed a significantly higher intrinsic rate of natural increase (rm) than LW and WN species, and such differences may be associated with host plant stability. Although there was no apparent relationship between sex ratio and life type, the extremely female-biased sex ratio in several WN species appears to contribute to local mate competition. In contrast, WNs strongly affect the aggressiveness of males because they provide a recognisable domain that they should defend. Furthermore, the WN type enhances mite aggregation in nests, and their iteroparous reproduction causes generation overlapping. Such traits may lead some WN species to develop advanced sub-sociality (cooperative sociality).
1 Introduction
The class Arachnida, including spiders, are widely known for their silk production and used in various industries. In the related superorder Acariformes, silk producers are found in several taxa, such as Bdellidae, Cheyletidae, Anystidae, and Tetranychidae (Walter and Proctor, 1999). Spider mites, belonging to the subfamily Tetranychinae, are tiny herbivores that produce silk threads from terminal eupathids (silk glands) present on the tips of their pedipalpi (Jeppson et al., 1975). These silk threads function as lifelines for spider mites, aiding in their movement (Saito, 1977a), as well as in the formation webs and tent-like structures. Saito (1983) recognised three basic lifestyles in spider mites and defined ‘life type’ as a way of living on host-plant leaf surfaces with silk production and usage patterns (threads and webs), defaecation patterns, and other behavioural outcomes such as positions where eggs are placed and where mites enter quiescent phases. Jeffries and Lawton (1984) defined ‘enemy-free space’ as a type of prey strategy that reduces or eliminates vulnerability to natural enemies. They stressed that the struggle for enemy-free space in natural communities is an important component of the divergent evolution of phytophagous species. Thus, we hypothesised that the life types of spider mites reflect an approach to avoid natural enemies. Here, we introduce the diversity of life types of spider mites and the functions of silk threads. We then discuss the relationships between life types and prey-predator interactions, interspecific competition, faeces management to maintain a clean habitat, life history, sex ratio, sexual selection, and social behaviour. The aim of this review is to understand the diversity and evolution of spider mites from the perspective of life types.
2 Diversity of life types
2.1 Little web type
The simplest of the three life types is the little web (LW) type. LW type is found in the genera Aponychus, Eutetranychus, Panonychus, and Yezonychus (Saito, 1983; Mushtaq et al., 2023). Aponychus firminae does not produce silk threads (LW-f hereafter, where the lowercase letter refers to sub-category; see Saito (1983) and Saito (1995b) for more details). Aponychus corpuzae, on the other hand, produces silken webs for covering the eggs, which is the simplest example of a silken structure (LW-j) in Tetranychinae. Panonychus citri and Panonychus ulmi always produce silk threads while walking (Saito, 1977a) but they do not construct any web structures other than ‘guy ropes’ extending from their slender stalks on eggs to the leaf surfaces (Figure 1, LW-c; Beament, 1951).
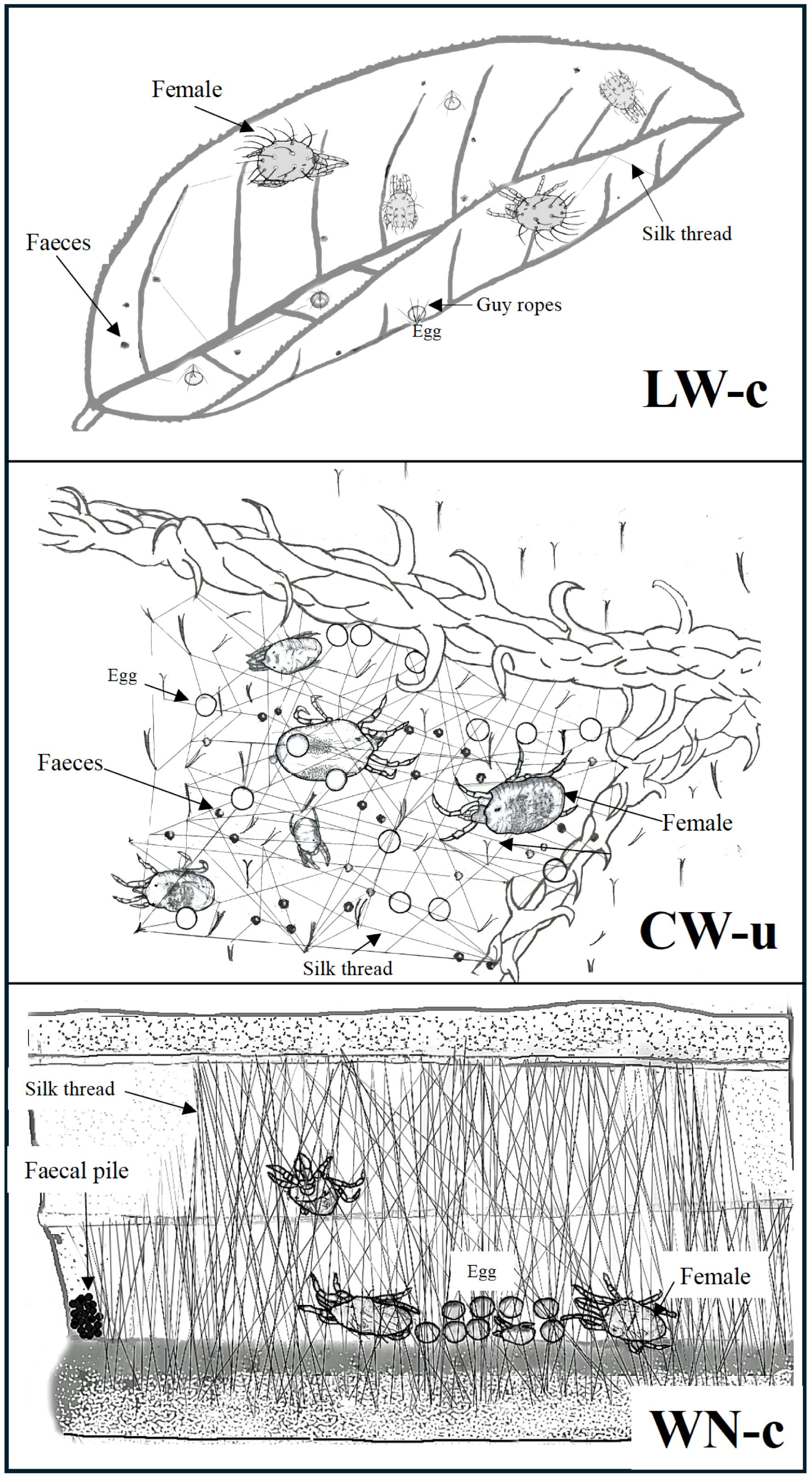
Figure 1. Sketches of three life types in spider mites: LW-c (eg. Panonychus citri) representing LW, CW-u (eg. Tetranychus urtiace) representing CW and WN-c (eg. Stigmaeopsis longus) representing WN. These drawings do not reflect actual body sizes.
2.2 Complicated web type
Most Tetranychus species and some Eotetranychus, Oligonychus, and Schizotetranychus species have another life type called the CW (non-systematic and complicated web) type (Saito, 1983, 1995b, 2010; Mirza et al., 2018; Mushtaq et al., 2021). Saito (1977a) showed that Tetranychus urticae, a well-known pest spider mite in various crops, always spins silk threads while walking. Because females tend to aggregate on leaf depressions (e.g. along leaf midribs), the silk threads produced by them accumulate there and form a web structure. They frequently walk on the web to deposit eggs and faeces (Hazan et al., 1974; Saito, 1983; Gerson, 1985; Mirza et al., 2018) and also use the web for their quiescent phase (CW-u, Figure 1). Leaf depressions over which CW webs accumulate are sometimes created by Tetranychus spp. themselves (Oku et al., 2006; Oku and Yano, 2007).
Sasanychus akitanus forms two to three layers of fine webs over depressions on the leaf undersurfaces of the host plant Sasa senanensis. The webs do not form the complicated structure like CW-u species and rather appear a stratified structure. The mites walk on these layers and deposit eggs and faeces, as well as enter a quiescent phase in these layers (CW-a, Saito, 1983). Thus, CW type is not just a product of accumulated silk threads but is constructed for specific functional reasons.
2.3 Woven nest type
Many species of spider mites build systematic nest-like web structures on the leaf surfaces of their host plants, which is defined as the woven nest (WN) type (Saito, 1983). Eotetranychus suginamensis, Schizotetranychus shii, and S. brevisetosus construct nests with dense webs over leaf depressions and always deposit their faeces on the roofs of WNs; therefore, their nests are coated with faeces (WN-s).
Among the species forming the WN life type, Stigmaeopsis species show the most sophisticated nesting behaviour (before the study by Saito et al. (2004), they were classified as Schizotetranychus). Most species of this genus have a sub-life type, WN-c, which is characterised by solid WNs on leaf undersurfaces with faecal deposits at particular locations near the nest entrances (Figure 1). A similar life type has been reported in Oligonychus perseae infesting avocado trees, although faeces are not always deposited at specific locations (Aponte and McMurtry, 1997).
Neonidulus tereotus forms a WN-n type, in which the faecal deposition pattern is largely different from that of WN-s and WN-c (Saito et al., 2016). The mites build dense WNs alongside the main vein of the leaf undersurfaces and live there gregariously. The faeces deposited within the nest form a concentric circle (similar to a low-clay rampart) around the nest periphery. Most eggs are laid inside the faecal circle, where immature mites also reside (Saito et al., 2016).
In contrast to previous WN types, Schizotetranychus recki with WN-r does not live in groups but instead creates individual nests (like compartments) using two or three leaf hairs on the undersurfaces of the host plant, Sasa spp. The mites make private nests, feed, and enter quiescent stages in these nests. The females also weave dense webs around individual eggs (Saito, 1983, 1995b, 2010).
2.4 Multiple life types
Life types are not always fixed in certain species. As reported by Saito (1983), one species can sometimes exhibit different life types depending on the leaf structure of the host plant. Eotetranychus tiliarium forms a CW-r type on Alnus hirsuta and a WN-t type on Alnus japonica. Additionally, the physical features of the leaf are sometimes pivotal for spider mites, e.g. S. recki to achieve their life types. However, Mushtaq et al. (2023) observed that T. urticae (CW-u), Eutetranychus orientalis (LW-j), and E. palmatus (LW-j) exhibit a consistent life type, even in different host plants. The authors concluded that these spider mites are highly adapted to the resources of different host plants for survival, with slight variations in some important life type characteristics.
3 Functions of life types
3.1 Primary function of CWs and WNs
It is difficult to determine the ‘function’ of a certain character in the evolutionary sense (Williams, 1966). In this review, we adopt a practical usage of the term ‘function’, i.e. if there is evidence that a particular life type aids spider mites in overcoming any adverse conditions, such as gravity, climatic conditions, predators, competitors, and diseases, we consider such a case to be indicative of the function of the life type.
However, before considering life type functions, we should address the fundamental question of why most spider mites prefer to live on the undersurface of host plant leaves (Table 1). This preference is considered an adaptation to avoid rainfall (Jeppson et al., 1975) and ultraviolet B (UVB) radiation (Barcelo, 1981). Ohtsuka and Osakabe (2009) supported the latter idea by demonstrating that egg hatching and juvenile development were significantly suppressed on the upper side of leaves exposed to sunshine or on leaves placed under a UV-transparent film. Plant leaves serve as selective sunscreens for small arthropods on the undersurfaces of leaves (Lavola et al., 1998). Thus, as they lack resistance to solar radiation, spider mites often select leaf undersurfaces to reside (Osakabe, 2021). Sakai et al. (2012) tested the fecundity of T. urticae using kidney bean leaf discs with their adaxial or abaxial sides facing upward or downward under laboratory conditions. They revealed that the adaxial leaf surfaces rather have a nutritional advantage over abaxial surfaces. Then these nutritional factors did not explain the biased distribution of T. urticae to leaf sides. Therefore, the deleterious effects of solar UVB radiation and heat on spider mites may explain why most spider mites live on leaf undersurfaces.
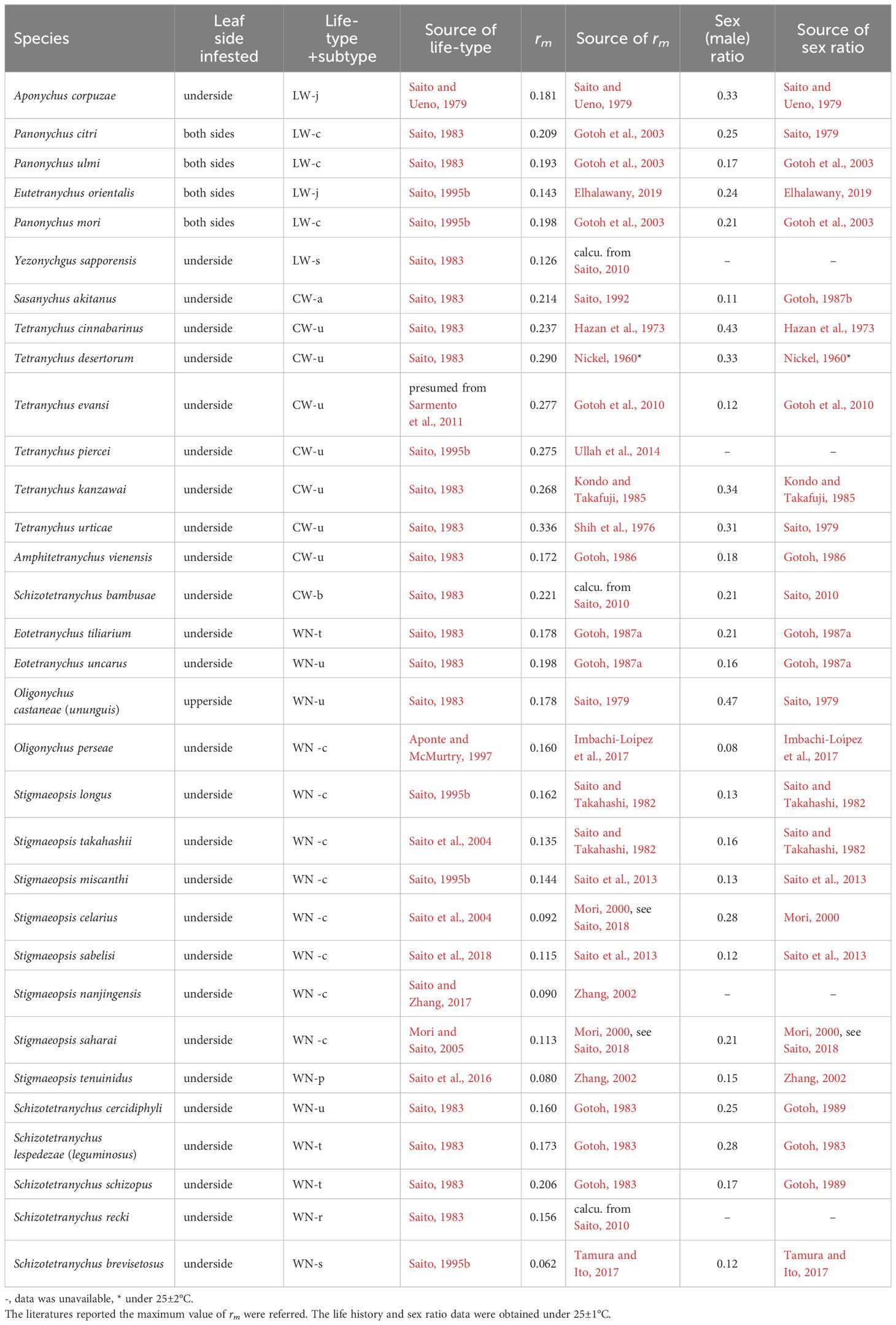
Table 1. Intrinsic rate of natural increase (rm), life type and sex ratio for species of Tetranychinae.
Even if spider mites can thrive equally well on both leaf sides, they may still choose to reside on the underside to escape from the deleterious effects of solar radiation. However, this escape strategy comes with the risk of falling. Saito (1977a) observed that T. urticae occasionally lost their footing while walking on the underside of downward-facing smooth (webless) leaf surfaces. These mites rely on silk threads to suspend themselves and subsequently ascend the leaves by climbing the threads, underscoring the significance of silk threads as lifelines during locomotion (Saito, 1977a). This phenomenon also suggests that T. urticae (and most Tetranychus spp.) has an insufficient structural apparatus (empodia) to walk around smooth leaf undersurfaces that face downward. Saito (2010) stressed the importance of empodium morphology in facilitating the movement of T. urticae on dense CWs. The empodium of T. urticae consists of three pairs of fine hairs (proximoventral hairs) that open radially, which may enable mite legs to firmly grasp the thin and disordered web threads (Gutierrez and Helle, 1985). Conversely, the clawless empodia of this species, with low efficiency for gripping the leaf substratum, may make their movement on the undersides of downward-facing smooth (non-web) host difficult. Consequently, T. urticae and related species (with clawless empodia) should have developed other ways to compensate for such limitations and live stably on the leaf undersides. Saito (1977b) reported that the silk threads (silk segments) of T. urticae females are distributed aggregately on leaf undersides (facing downward) for a day after introduction, indicating that the females first move around frequently in a confined area to accumulate silk threads (see also Saito, 1985).
These results suggest that T. urticae needs to immediately produce dense webs on newly invaded leaf undersides to avoid the negative effects of solar radiation and gravity. Thus, the primary functions of CWs accumulated on the leaf underside include ‘footing’ (Saito, 1985) and/or ‘safety net’. Several studies have reported heterospecific web sharing in Tetranychus spp (Yano, 2012; Sato et al., 2016), and this phenomenon may be explained by the primary function of CW: mites should enter webs pre-produced by either conspecifics or heterospecifics to avoid falling off from the leaves and to avoid solar radiation. Meanwhile, Yano (2008) found that dispersing females of T. urticae follow the trails left by preceding females. This behaviour is thought to be due to the movement of a new colony within a group. However, the phenomenon may also stem from their aversion to walking on webless surfaces and preference to walk on the silk threads left by preceding females, as webless surfaces are considerably dangerous. The WN life type, characterised by nesting behaviour with silk, undoubtedly saves mites from the force of gravity as a safety net, considering that almost all species live on the leaf underside.
3.2 Life types as predator avoidance devices
The CW produced by Tetranychus spp. on leaf surfaces impedes the entry of generalist and specialist predators in different manners (Putman, 1962; McMurtry and Scriven, 1964; McMurtry and Johnson, 1966; McMurtry et al., 1970; Gerson, 1979; Sabelis, 1981; Gerson, 1985; Sabelis and Bakker, 1992; McMurtry and Croft, 1997; Yano, 2012; McMurtry et al., 2013). If specialist predators such as Phytoseiulus persimilis, Neoseiulus womersleyi, and Neoseiulus californicus can cope with the webs (Sabelis and Bakker, 1992; Shimoda et al., 2009), spider mites may change their oviposition and/or quiescent sites from leaf surfaces to webs to avoid (or mitigate) predation (Oku et al., 2003; Otsuki and Yano, 2017). Oku and Yano (2007) observed that female Tetranychus kanzawai (CW-u) and T. urticae tend to deposit their eggs on webs immediately after encountering predators (also see Murase et al., 2017). Furthermore, Lemos et al. (2010) reported that Tetranychus evansi eggs laid on webs were less frequently preyed upon by the predatory mite Phytoseiulus longipes than those laid on leaf surfaces. These results suggest that the shift in oviposition and quiescent sites from leaf surfaces to webs is a strategy to reduce predation risks (Grostal and Dicke, 1999).
Dittmann and Schausberger (2017) showed that T. urticae females aggregate more closely and are more active in the presence of predator cues. The benefit of tighter aggregation arises from the encounter and dilution effects, the combination of which is called attack abatement (Schausberger et al., 2021). Here, the CWs may provide a refuge and landmark for individual mites to aggregate.
However, Okada and Yano (2021) reported that rainstorms had a negative effect on T. kanzawai eggs deposited on webs, as strong rainstorms can easily remove the eggs deposited on CWs. They hypothesised that there is a trade-off in selecting the oviposition sites by T. kanzawai—the eggs on webs are advantageous for escaping predation but disadvantageous for survival under adverse climatic conditions.
To understand the additional functions of the WN life type, ‘removing’ and ‘non-removing (intact)’ life type experiments have proven to be useful. Horita et al. (2004) removed the webs covering S. recki eggs and compared the probability of predation between eggs with and without web protection. The WN-r type nests effectively protected the eggs from four species of sympatric predators (Table 2). An exception was Agistemus summersi, which exhibited a preference for preying on eggs contained within web nests than on those without nests. In this case, the web is likely a cue for the predator to locate the prey eggs. The WN-r type in S. recki functions as a refuge for spider mites from predators; however, in the case of A. summersi, WN exposes spider mites to danger.
Mori and Saito (2004) addressed the functions of the WN-c type by observing three species, Stigmaeopsis longus, Stigmaeopsis takahashii, and Stigmaeopsis saharai, inhabiting Sasa senanensis and having different-sized nests. Several females of each species were introduced onto detached S. senanensis leaves. After the females had constructed their web nests and deposited a sufficient number of eggs within them, the females were removed, and the ‘web-removal’ and ‘web-intact’ treatments were conducted. Several predator species that occur sympatrically on S. senanensis were introduced individually into each experimental arena. The results clearly showed that the nests had strong protective effects on the eggs (Table 2). The key points from these results are that the protective effect of the WN type is positive but varies among nests of different sizes and among predator species.
Another function of the WN-c type has been observed in S. takahashii (and probably S. saharai), which builds many small separate nests during its lifetime. Randomly scattered nests have an indirect effect by creating void nests after predation, effectively decreasing predator-searching efficiency (Saito et al., 2008).
Life types involve not only the web structure, but also the behavioural outcomes of spider mites on host leaves (Saito, 1983, 1995b). Yezonychus sapporensis deposits its eggs and spends its quiescent stages on the tips of leaf hairs (this species is categorised into the LW-s type, Saito, 1983). Yanagida et al. (2001) investigated whether eggs deposited on the tips of leaf hair (S. senanensis) are safer than those deposited on leaf surfaces. Seven predator species which occur on S. senanensis were examined. The feeding rates of several predator species decreased when prey eggs were positioned on the tips of leaf hairs. However, among the predators tested, the feeding rates of Phytoseius tenuiformis and A. summersi were equal regardless of egg position (Table 2).
The spider mite A. corpuzae has an extremely flat body. Furthermore, it feeds in a typical posture (lying flat) and completely spreads its body and legs on S. senanensis leaf surfaces. Chittenden and Saito (2006) used quiescent deutonymphs, which normally assume a flat posture on leaf surfaces. Half of them were tipped on their sides and the other half were left untouched in their natural positions. Additionally, webs covering eggs were removed to determine their function. The feeding abilities of the four predator species that usually co-occur with A. corpuzae were tested. Amblyseius orientalis and Typhlodromus bambusae showed increased egg consumption rates when the egg webs were removed. All tested predators consumed more deutonymphs that were tipped compared with normally positioned deutonymphs. Therefore, the egg-covering webs and quiescent posture with a flat body help A. corpuzae to avoid predation from at least two predator species (Table 2). Osakabe (1988) reported a similar function of egg webs (guy ropes), in which guy ropes surrounding the eggs of P. citri prevented Amblyseius sojaensis from touching the eggs. Furthermore, Yano and Shirotsuka (2013) reported that the dorsal setae and resting posture of P. citri (LW-c type) function as anti-predator defence mechanisms. Adult P. citri females lie on citrus leaf surfaces, with their dorsal setae (hair) oriented upwards. Compared with normally lying females, both manipulated non-lying females and hair-removed females experienced higher predation risks. When a predator approached the body surface of a lying female, the setae inevitably created elasticity upon contact, eventually repelling the predator from the female.
The above three examples show that not only the outcomes of spider mite behaviour but also the morphology itself has a predator avoidance (or elimination) function.
3.3 Function of silk threads
Next, we discuss that the silk threads themselves function as a predator avoidance tool. Even if spider mites with the CW type (e.g. Tetranychus spp.) produce more silk in the presence of predator cues (Oku et al., 2003; Schausberger et al., 2021), it may be challenging to discern whether they actively use silk threads as a defensive tool or whether it is simply a by-product of increased walking activity, because these mites continuously produce silk while walking (Saito, 1977a).
Saito and Zhang (2017) reported that female Stigmaeopsis nanjingensis strongly reinforce their nests after recognising predator cues. Note that this species does not produce silk except for weaving nests or egg covers (Saito and Zhang, 2017). The females heavily reinforce the nest (Figure 2) after considerable spinning movement and then close almost all the nest openings. This robust nest structure force T. bambusae immatures to be locked out, resulting in most of them starving to death outside the nest. Thus, reinforcing web nests with silk in response to predatory cues provides direct evidence that silk protects spider mites from predators.
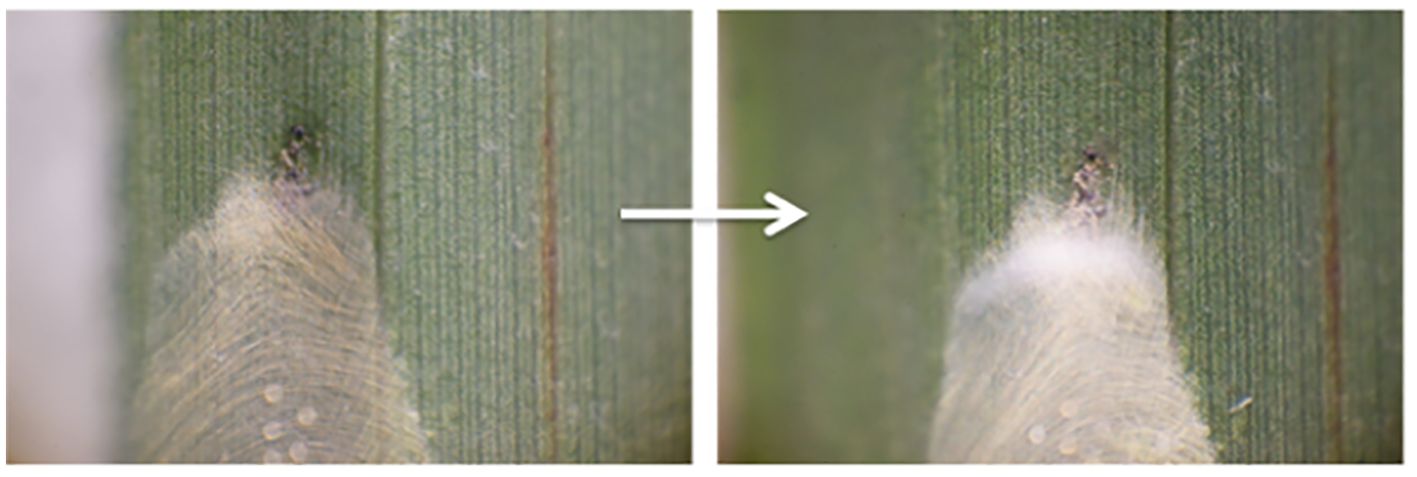
Figure 2. Left, Stigmaeopsis nanjingensis nest before introduction of predator larva. Right, 72 hours after the introduction (larva was already excluded from the nest). From Saito and Zhang (2017).
Kanazawa et al. (2011) used artificial dust to experimentally determine that females of S. longus use adhesive silk threads to perform regular cleaning of the nest space and eggs. They first learned that silk-weaving behaviour is not a by-product of nest building (nest reinforcement); rather, it is actively performed as a function of cleaning the living space and eggs. As such, they have discovered an extraordinary new role of silk threads as devices for cleaning the nest space and/or eggs. These strongly indicate that special adaptations for maintaining clean habitats are essential for animals to evolve aggregative social lives.
3.4 Counterattack and life types
Life types may sometimes become prerequisites for spider mite defence ‘behaviours’, as WN-type nests provide ‘domains’ for predator-prey interactions.
Counterattack behaviours of S. longus, S. miscanthi, and S. nanjingensis against the specific phytoseiid predator T. bambusae have been reported (Figure 3; Saito, 1986a; Mori and Saito, 2005; Yano et al., 2011; Saito and Zhang, 2017). For example, approximately 30 and 80 precent of T. bambusae larvae were killed when they intruded a nest defended by eight S. longus females for two days and two S. longus males, respectively (Saito, 1986b). Ito (2019) also reported a similar phenomenon in another WN-type species, S. brevisetosus. He found biparental counterattack behaviour in this species against insect predators, such as midge (Feltiella sp.), within and around nests. Thus, the WN type appears to play a crucial role in supporting spider mite counterattack behaviour, i.e. WN nest provides sufficient group (family) living space and landmarks (such as a fort or domain) for defence.
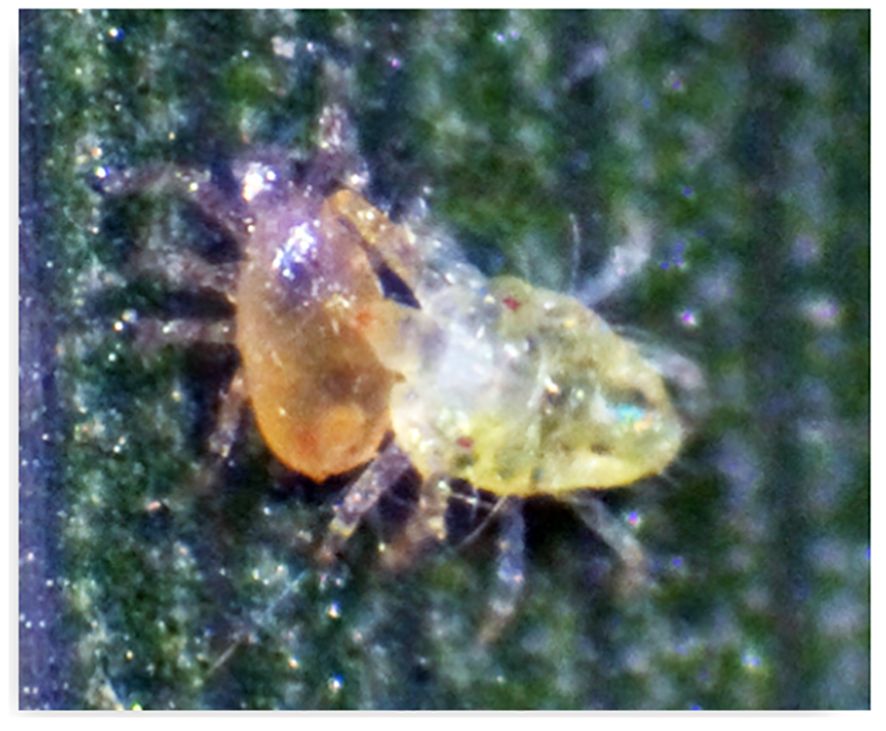
Figure 3. Adult male of Stigmaeopsis nanjingensis counterattacking against adult male of Typhlodromus bambusae.
3.5 Counter response of predators
From the perspective of predators, they must evolve some ways to overcome spider mite defence (life types, counterattacks, and so on) to maintain their ecological niches.
Sabelis and Bakker (1992) investigated whether the morphology of phytoseiid predators aids in coping with the CWs of spider mites. They tested a functional hypothesis on how the seta patterns (chaetotaxy) of phytoseiids contribute to protection in sticky environments, specifically focusing on their impact on the foraging success in the CWs of Tetranychus species. They concluded that the seta patterns of phytoseiids influence their ability to cope with webs (seta protection hypothesis). In line with this, Shimoda et al. (2009) reported that specialist predatory mites P. persimilis, N. womersleyi, and N. californicus cope with the CWs of T. urticae using chelicerae and palps—they can cut the threads and move easily inside the web.
Another interesting example is T. bambusae. As previously stated, S. nanjingensis adults exhibit a strong counterattack against T. bambusae (Saito and Zhang, 2017). Direct counterattacks (killing) and locking out predators using reinforced web nests would be more efficient when both sexes defend their nests together. The mortality of T. bambusae immatures due to S. nanjingensis counterattack is extremely high, suggesting that these immature predators find it difficult to survive alone in nests with several prey adults. However, T. bambusae can still maintain its status as the main predator of S. nanjingensis populations (Zhang et al., 2000, 2004; Tsuji et al., 2011). Adult predators exhibit three types of behaviours (ripping dense nest webs, excluding adult prey, and killing them) to cope with the counterattacks of adult preys. Almost all immature predators can survive and feed on prey eggs when accompanied by their mothers, who can invade nests by ripping the web and killing or purging dangerous prey adults (Saito and Zhang, 2017).
Aponte and McMurtry (1997) observed that phytoseiids such as Galendromus helveolus, Galendromus annectens, and N. californicus can enter nests of Oligonychus perseae exhibiting a WN-u type by ripping webs and then feed on the adults. Other species, such as Euseius hibisci and Iphiseius degenerans, are unable to enter the nests, probably because of their larger size, but feed on mites found outside the nests. Furthermore, Montserrat et al. (2008) reported that N. californicus females can rip open the nests of O. perseae.
3.6 Life type and interspecies competition
Life type can sometimes induce competition among spider mite species. It is well-known that Panonychus ulmi is abundant in apple orchards in the early season and is replaced by T. urticae in the late season (Foott, 1963; Tanigoshi et al., 1979). Morimoto et al. (2006) and Osakabe et al. (2006) confirmed that T. urticae excludes P. ulmi under both experimental and field conditions and suggested that CWs constructed by T. urticae may influence the outcome of their competition. As mentioned earlier, T. urticae has a CW life type, whereas P. ulmi has an LW life type. T. urticae can move on dense and complex webs with the help of their special empodia. In contrast, the hooked empodial claws of P. ulmi are expected to be functional for grasping leaf surfaces but must get hooked on threads when walking on the T. urticae’s CWs (Saito, 2010). P. ulmi may have difficulty living on leaf surfaces with accumulated webs, leading to their displacement by T. urticae.
A similar phenomenon has been observed in two Tetranychus species with CW type infesting tomato plants. Teteranychus evansi can downregulate plant defence, which is usually induced by mite infestation, whereas T. urticae cannot (Sarmento et al., 2011). The plant leaf surface under T. evansi webs is attractive for T. urticae not only in terms of a safety net and protection against predators, but also in terms of suitable feeding areas where plant defences are not induced. However, T. urticae has difficulty reaching the leaf surface to feed when the leaf is covered with dense webs produced by T. evansi, as the webs of T. evansi are much thicker than those of T. urticae. Furthermore, T. evansi produces more silk when exposed to damage or other cues associated with T. urticae. Thus, the silken webs produced by T. evansi prevent competitors from profiting from downregulated plant defences (Sarmento et al., 2011).
3.7 Life type and faeces manipulation
Faeces manipulation is an important behavioural element in life-type recognition (Saito, 1983). Many species with WN and CW life types exhibit faeces manipulation behaviours to different degrees.
Tetranychus urticae lays many faecal pellets on CW webs (Hazan et al., 1974; Gerson, 1985); hence, Hazan et al. (1974) used the pellets on the webs as an indicator of web density. However, little information is available on why Tetranychus spp. deposit their faeces on CW webs. Saito (1995b) suggested that such faecal deposition behaviour may keep living and feeding spaces (leaf surfaces) clean. If one of the functions of the CW type is to enhance group formation and cohesion (Le Goff et al., 2010; Schausberger et al., 2021), the leaf surfaces where mites aggregate and feed should be kept clean.
This hypothesis appears to be true for several WN-type species which deposit faeces on the roof, inner margin of nests, or on other sites (Saito, 1995b). In particular, a relationship between faeces manipulation and group size has been found in the genus Stigmaeopsis. S. miscanthi, S. longus, S. takahashii, and S. saharai share a common feature in that they construct tunnel-like nests and create faecal piles near the nest entrances. However, faecal manipulation is slightly different among these species. Sato et al. (2003) and Sato and Saito (2006) found that S. miscanthi and S. longus defaecate at sites where faecal piles already exist by using volatile chemical cues from faecal piles, whereas S. takahashii and S. saharai defaecate near nest entrances using only tactile cues. S. miscanthi and S. longus build larger nests by extending their nests continuously to live gregariously for a long time; therefore, minimising the locations of faecal piles must be an adaptive strategy to decrease the risk of disease. Volatile chemical cues are convenient for this purpose. Although the exact nature of volatile chemical cue remains unidentified, it is known to be an ether-soluble substance originating from host plants, since mites change their defaecation site in response to these substances (Sato and Saito, 2008).
4 Life type and life history
Sabelis (1991) conducted a comprehensive review on spider mite life history and concluded that interspecific comparisons of life-history data reported to date do not provide evidence for trade-offs between developmental rate, body size, silk production, and egg production.
Since this review, life history studies on various spider mite species have increased, but information about their life types remains scarce. Here, we collected life history data, including life type-related descriptions and analysed the relationship between life history and life type (Table 1). The maximum intrinsic rate of natural increase (rm) of each species under 25 ± 1 °C was considered to be one of the important parameters that describe its life history. We plotted the rm data by life type and detected a clear tendency for the rms of CW-type species to be consistently higher than those of WN-type (Figure 4, P < 0.001) and LW-type species (P < 0.05). The rms values of the LW-type species were intermediate between those of the CW- and WN-type species (Figure 4).
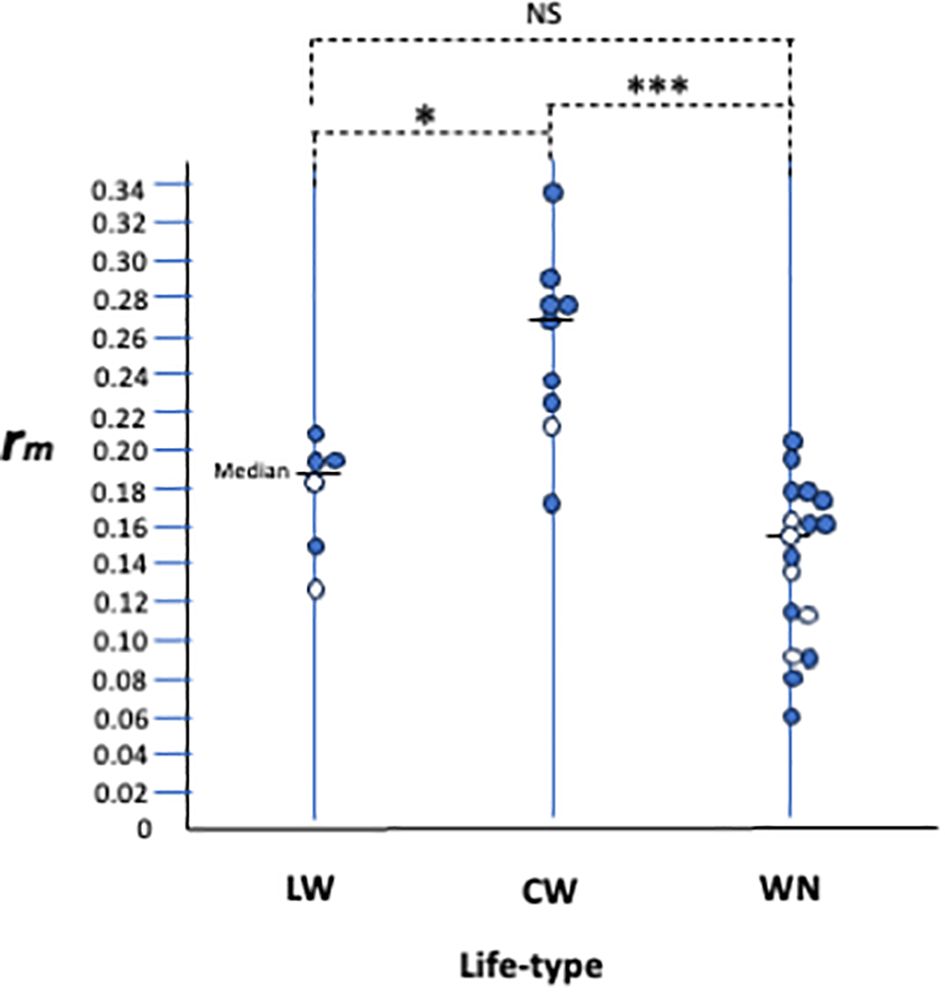
Figure 4. Relationships between life-type and life-history parameter, rm. Each circle represents a single spider mite species. Open circles are species on Sasa senanensis. Life-history and life-type data appear in Table 1. NS, insignificant, *P <0.05, ***P<0.001 by Wilcoxon signed rank test with Rice (1989) adjustment for post hoc multiple comparisons.
Species with higher rm values are supposed to be able to adapt to their relatively unstable habitats (Mitchell, 1973; Southwood, 1977; Saito, 1979). Tetranychus spp. having the CW type showed higher rm and mainly inhabited herbaceous hosts. As mentioned previously, the CW type is supposed to be an outcome of establishing footing webs on the leaf undersides, which are free from solar radiation and gravity. ‘Walking around with silk threads’ is crucial for constructing CW webs, and it must be indispensable for mites, such that it may be performed at a low cost.
In contrast, the habitats of WN-type species, such as bamboo and woody plants, appear to be comparatively stable because of their longevity (stability in time) and large stock (tree) sizes (stability in space). This stability may help the species survive for several generations in the same habitat. The longer a colony persists in the same habitat, the greater the risks associated with the accumulation of natural enemies (predators and diseases, Southwood, 1977; Saito and Ueno, 1979). As described previously, the WN type functions as an effective defence mechanism (shelter) against predators. Thus, the low rm of the WN-type species may be related to their sophisticated defence mechanisms which are inevitably accompanied by higher costs.
However, there is an exception to the above reasoning of host plant stability. As shown in Figure 4, different life type species with various rm values (open circles) occur on the same host plant (S. senanensis). It appears that unstable habitats are only available for CW-type species, but stable habitats may be open for species of all life types. Does a relatively stable host plant allow mite species with different life types and rm s to coexist together, or are there unknown factors at play? In any case, the host plant stability does not entirely explain the relationship between life type and life history. Hence, it remains unclear why there is a relationship between life type and life history.
5 Life type and sex ratio
As a life history parameter, rm is higher in the CW type than in the LW and WN types; thus, some relationship between life type and sex ratio is also expected. In spider mites, the sex ratio is often female-biased (Table 1), with the degree of bias varying largely among species.
Local mate competition (LMC) theory (Hamilton, 1967) has been broadly accepted as an explanation for female-biased sex ratios. In most animals, the sex ratio is 1:1, which is explained by frequency-dependent selection, with the assumption of panmictic populations (Fisher, 1930). However, in animals distributed in patches due to low mobility or other reasons, mating occurs within local patches (Hamilton, 1967). In such animals, intra-male competition for access to females often occurs among brothers. Consequently, mothers may produce more daughters than sons to reduce the unnecessary competition between their sons.
The dispersal of tiny, wingless spider mites is relatively limited; therefore, the occurrence of LMC is highly probable. Indeed, regulating the offspring sex ratio by females according to the number of foundresses, as predicted by the LMC model, has been observed in the CW type, T. urticae (Young et al., 1986; Roeder, 1992; Roeder et al., 1996; Macke et al., 2011) and T. cinnabarinus (Wrensch and Young, 1978), as well as in the WN type, S. longus and S. miscanthi (Sato and Saito, 2007). The CW and WN type species live in groups (colonies) inside webs and rarely leave when there is no habitat deterioration and/or the danger of predation. If mating frequently occurs within a colony, LMC is expected to be stronger in the CW and WN types than in the LW type. In addition, as previously discussed, WN type species may inhabit more stable environments than CW type species. This suggests that LMC is stronger in the WN type than in the CW type. Therefore, the bias in the sex ratio towards females is expected to be highest in the WN type, followed by the CW and LW types.
However, no significant relationship between life type and sex ratio was detected (P > 0.10; Figure 5); in each life type, the sex ratio largely varied among the species. There are several possible explanations for the unclear relationship between life type and sex ratio. First, factors other than LMC, such as temperature (Margolies and Wrensch, 1996; Roy et al., 2003) and changes in population density (Weerawansha et al., 2022), may also influence sex ratio. Life history data are often obtained from individual females; that is, females that are kept alone. Therefore, the sex ratio obtained from life history experiments may not always reflect sex ratios in the field, especially in species with sex-ratio control abilities. Additionally, the ability to control sex ratios varies among species. Macke et al. (2011) reported that the lines of T. urticae exposed to strong LMC lost the ability to control offspring sex ratio and consistently produced offspring with a female-biased sex ratio, whereas those exposed to mild LMC retained the ability to control offspring sex ratio. WN-type species are predicted to be consistently exposed to stronger LMC conditions than LW- and CW-type species. The degree of sex ratio changes in S. longus and S. miscanthi of the WN-c type is smaller than that predicted by LMC (Sato and Saito, 2007). Even though the expected relationship between sex ratio and life type could not statistically supported, a female-biased sex ratio has been consistently observed in the WN type, suggesting that the sex ratio is also related to life type.
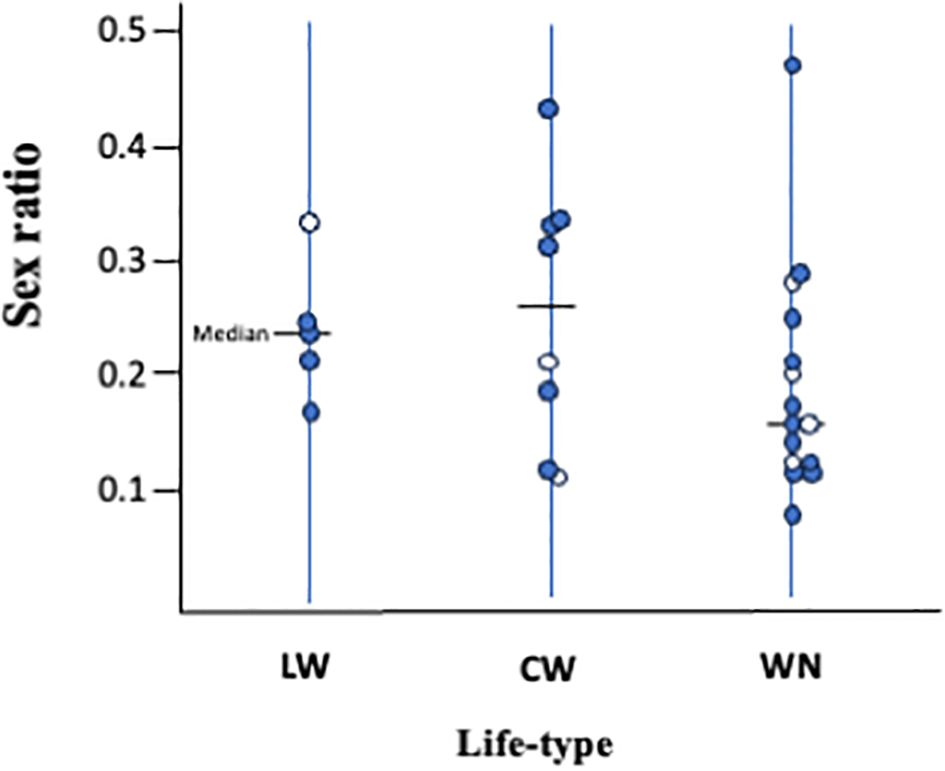
Figure 5. Relationships between life-type and sex ratio (male ratio). Each circle represents a single spider mite species. Open circles are species on Sasa senanensis. Life type and sex ratio data appear in Table 1. There is no significant difference (P > 0.10) between three life types.
6 Life type and sexual selection
Life types inevitably influence the probability of encountering mates, as the distribution of individuals is associated with whether they live alone or in groups. Male spider mites can deliver enough sperm in a single mating to fertilise all the eggs produced by a female during her lifetime (Helle, 1967). In addition, if mating is completed successfully without any interruptions, the sperm of the first male is used to fertilise the eggs, even if the female mates with multiple males (the first male sperm precedence, Helle, 1967; Potter and Wrensch, 1978; Satoh et al., 2001; Oku, 2008; Morita et al., 2020; Rodrigues et al., 2020). In such situations, males actively seek out quiescent females nearing adulthood (and occasionally virgin females) and guard them by mounting on their dorsum (known as precopulatory mate guarding = precopula, Ridley, 1983). If other males disturb the guarding male, they engage in physical combat to get mating priority over the females (Potter et al., 1976a). Then, searching, guarding, and fighting are three basic elements of male mating behaviour in spider mites.
Precopula and fighting behaviours of males have been reported in several spider mite species with different life types (Potter et al., 1976a; Saito, 2010). Although the reproductive behaviours of spider mites have been investigated in many studies, including mating durations (Potter and Wrensch, 1978; Takafuji and Fujimoto, 1985; Ozawa and Takafuji, 1987; Kunimoto et al., 1991; Oku, 2008; Sato et al., 2014a, 2015; Kobayashi et al., 2022), male fights over females (Potter et al., 1976a, 1976b), alternative male reproductive tactics (Sato et al., 2013b, 2014b), female choice (Vala et al., 2004; Oku, 2009; Tien et al., 2011), the cost and benefit of multiple mating (Oku, 2010; Rodrigues et al., 2020), and the influence of predators on reproductive behaviour (Murase et al., 2017; Oku et al., 2018; Rocha et al., 2020), these studies only focused on Tetranychus species and a few other genera. Hence, sufficient data is not available to address the relationship between mating systems and life types.
However, fatal (lethal) male fights have been observed in several WN type species, such as S. miscanthi species group and S. brevisetosus (Saito, 1990a; Masuda et al., 2015). In fatal male fights, a male thrusts its stylets, usually used for sucking the juice of host plants, into the other male’s body, thereby killing the competitor by sucking its body fluids, i.e. cannibalism (Figure 6, Saito, 1990a; Masuda et al., 2015). Intra-sexual competition for access to females is a common phenomenon observed across many animal taxa (Andersson, 1994) and is not restricted to spider mites. However, killing rivals in male fights is rare in the animal kingdom because the cost of such a fight is extremely high relative to the resource (mate) value. Thus, the conditions under which a fatal fight evolves are limited (Maynard Smith and Price, 1973). It is important to note that the males of S. miscanthi engage in fatal fights not only for access to females but also to defend their ‘nests’. Nests of the WN type may prepare recognisable domains that make it easy for males to defend against competitors (and predators). There is evidence that the closely related species S. longus (WN-c type) can recognise the existence of nests (domains) by its dorsal setae (Mori and Saito, 2013). Furthermore, females are packed closely in WN nests (Figure 1), which may make it easy for males to monopolise. These factors appear to be the prerequisites for the evolution of strong male aggression. Thus, we can understand why the males of CW species seldom engage in a fatal fight, even though they often exhibit intra-male competition (Potter et al., 1976a)—because there is no clear border of the domain where the males can recognise the defence. Nevertheless, we could not strictly explain why fatal fights evolved exclusively in males of certain species.
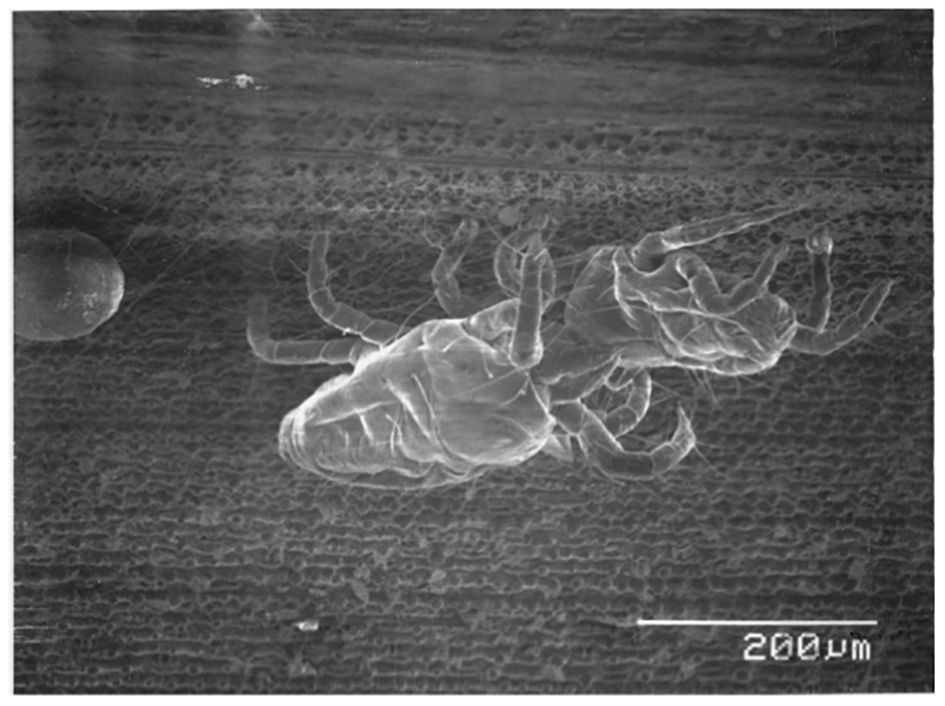
Figure 6. Male-to-male fatal combat in Stigmaeopsis mischanthi. Photographed by Y. Saito under a scanning electron microscope (with cryosystem, Hitachi S-2100A).
Interestingly, S. longus males do not engage in fatal fights, even though they show very strong counterattack behaviour against natural enemies (Saito, 1990a, 2010). The reason for such a difference between closely related species may stem from variation in their distribution patterns, as S. longus is distributed throughout northern Japan, whereas S. miscanthi group (Saito, 1990a) and S. brevisetosus are mainly found in southern Japan. In the S. miscanthi group (two forms of S. miscanthi, Stigmaeopsis sabelisi and Stigmaeopsis continentalis, and Stigmaeopsis formosa), there is geographic variation in the intensity of fatal male fights (Saito, 1995a; Saito and Sahara, 1999; Sato et al., 2013a). Geographical variation corresponds to the difference in average relatedness between nest-mate males which is based on the probability of mother-son mating in early spring through harsh winter conditions (Saito, 1995a, 2010; Sato et al., 2013a). A game-theoretical analysis by Saito and Takada (2009) indicated that the intensity of aggression (frequency of aggressive males) decreases with an increase in relatedness between confronting males when there is sufficient benefit from cooperation. Note that the counterattack of S. longus and S. sabelisi against predators is more effective when there are multiple males (i.e. cooperation of males, Saito, 1986a; Yano et al., 2011). Therefore, not only WN type but also kin structure is thought to be an important cause of male fight variation (Saito, 1995a, 2010; Saito and Sahara, 1999; Saito and Takada, 2009; Sato et al., 2013a).
As mentioned before, S. brevisetosus has the WN-s life type and show male counterattack behaviour (Ito, 2019) and male-to-male fatal combat (Masuda et al., 2015) similar to the species group of S. miscanthi. Recent phylogenetic studies have revealed that S. brevisetosus is distinct from the monophyletic clade of the genus Stigmaeopsis (Matsuda et al., 2018). This means that the fatal male-to-male combat as well as the male counterattack behaviour converged in these two genera (Ito, 2019), again reiterating that WN type provided a strong ‘background’ for such behaviours.
7 Life type and sociality
The cooperative and/or social behaviours of spider mites have been thoroughly reviewed by (Saito, 1997, 2010) and Schausberger et al. (2021). The simplest sub-social behaviour is maternal egg-covering habit in the LW life type (Saito, 1995b). The construction of shelter-like webs to protect themselves and their young against natural enemies in the CW and WN types is considered a more advanced sub-social behaviour. Such constructions may also promote aggregation (Schausberger et al., 2021). Unlike spiders, which exhibit semelparous reproduction leading to the creation of an egg mass, spider mites, being iteroparous, need specific reasons for aggregating their eggs (or other life stages). The necessity for refuge from predators, footing, and a safety net might have promoted the evolution of web formation, which leads to the aggregation of individuals in a confined space. These might provide important traits for the evolution of sociality, i.e. nesting habits lead to mite aggregation and iteroparous reproduction causes generation overlapping to allow interactions between parents and offspring (Saito, 1997, 2010), which have been considered to be the basic elements of sub-sociality (Wilson, 1975). However, aggregation may increase the risk of rapid habitat deterioration and disease outbreaks. There are at least two ways to avoid such risk. The first is to increase the population rapidly (higher rm) and disperse it as quickly as possible before the habitat deteriorates and the disease becomes widespread, as assumed in the CW type. The second is to live in spatiotemporally stable habitats. Mites living in stable habitats should invest more in web construction to protect themselves from predators and develop nest sanitation behaviours (faeces manipulation and nest cleaning by silk), which is observed in the WN type.
There are differences in nest size, nest persistence, and intensity of parent-offspring relationships among Stigmaeopsis spp. with the WN-c type (Saito et al., 2016; Saito, 2018). Larger nests in S. longus, S. miscanthi, and S. nanjingensis can contain a large number of nest members (mostly kin) and persist for a long period, so that the nest defence (counterattack) against natural enemies by the group becomes effective through cooperation (Mori and Saito, 2004). On the other hand, smaller nests of S. takahashii and S. saharai only contain a small number of mites and persist for a short period; therefore, counterattacks are ineffective (Mori and Saito, 2004). However, smaller nests serve as protective refuges that are more effective than larger nests, as they make it difficult for many predator species to search (Saito et al., 2008) and intrude (Mori and Saito, 2004). In short, there might be two strategies against predators, i.e. ‘defence by kin group in large nests’ and ‘protection by smaller and solid nests’ (Mori and Saito, 2004). This must be a reason why there is such variation in nest size of Stigmaeopsis spp. and the former strategy may be synonymous with ‘cooperative sociality’ (Saito, 2010).
8 Epilogue
As discussed above, life types provide a fundamental framework for the diversity and evolution of spider mites. In addition, life types can be useful in identifying spider mite species. Many species of the genus Stigmaeopsis, one of the main groups discussed in this review, have recently been described based on their life-type characteristics. Before Saito (1990b), only one species, Schizotetranychus (at present, Stigmaeopsis) celarius, was known. Subsequently, differences in life type characteristics, including nest size, defaecation sites, and male behaviour, have been recognised (Saito et al., 2016), and 15 species have been described in the reinstated genus Stigmaeopsis to date (Saito et al., 2019). If life types are described in various spider mite species using the method described by Saito (1995b) as a standard method, this will greatly contribute to our understanding of the diversity and evolution of spider mites.
Life type might have evolved through natural selection, as an ‘extended phenotype’ proposed by Dawkins (1982). This review shows that life type is closely related to the defence mechanisms against natural enemies. This result corresponds well with Jeffries and Lawton’s (1984) definition of ‘enemy-free space’. Therefore, we supposed that spider mite life types reflect a way to avoid natural enemies.
Descriptions of diversity and global comparisons between species are necessary steps for the development of evolutionary ecological studies. However, there is a great difficulty in studying evolutionary processes solely by such between-species comparisons without accurate phylogenic information (Harvey and Pagel, 1991). Several recent studies on the molecular phylogeny of spider mites revealed important discrepancies in the morphological classification of genera and molecular phylogenetic relationships (Matsuda et al., 2014; Matsuda et al., 2018). Then, attempts to analyse the evolution of life types along with phylogenetic trees should be made, after the molecular and morphological phylogenetic studies on spider mites attain a reasonable position and more robust and comprehensive data on life types are accumulated.
Author contributions
YutS: Writing – review & editing, Writing – original draft. YukS: Writing – review & editing, Writing – original draft.
Funding
The author(s) declare financial support was received for the research, authorship, and/or publication of this article. The Suzuki Takahisa Memorial Grant, the University of Tsukuba (to YukS).
Acknowledgments
We sincerely thank the late Prof. Sabelis M.W. who supported our work for long periods. We also thank Drs. Lesna I., Sahara K., Takada, T., Mori K., Chittenden A.R., Ito K., Sakagami T., Zhang Y. X., and Lin J. Z. for their help. We also appreciate Schausberger P. who kindly invited us to the present review. This work was supported in part by The Suzuki Takahisa Memorial Grant, the University of Tsukuba (to YukS). Last, we would like to thank Editage (www.editage.jp) for English language editing.
Conflict of interest
The authors declare that the research was conducted in the absence of any commercial or financial relationships that could be construed as a potential conflict of interest.
The author(s) declared that they were an editorial board member of Frontiers, at the time of submission. This had no impact on the peer review process and the final decision.
Publisher’s note
All claims expressed in this article are solely those of the authors and do not necessarily represent those of their affiliated organizations, or those of the publisher, the editors and the reviewers. Any product that may be evaluated in this article, or claim that may be made by its manufacturer, is not guaranteed or endorsed by the publisher.
References
Andersson M. (1994). Sexual selection (Princeton, N.J: Princeton University Press). doi: 10.1515/9780691207278
Aponte O., McMurtry J. A. (1997). Damage on ‘Hass’ avocado leaves, webbing and nesting behaviour of Oligonychus perseae(Acari: Tetranychidae). Exp. Appl. Acarol. 21, 265–272. doi: 10.1023/A:1018451022553
Barcelo J. A. (1981). Photoeffects of visible and ultraviolet radiation on the two-spotted spider mite, Tetranychus urticae. Photochem. Photobiol. 33, 703–706. doi: 10.1111/j.1751-1097.1981.tb05477.x
Beament J. W. L. (1951). The structure and formation of the egg of the fruit tree red spider mite, Metatetranychus ulmi Koch. Ann. Appl. Biol. 38, 1–24. doi: 10.1111/j.1744-7348.1951.tb07787.x
Chittenden A. R., Saito Y. (2006). Tactile crypsis against nonvisual predators in the spider mite, Aponychus corpuzae Rimando(Acari: Tetranychidae). J. Insect Behav. 19, 419–428. doi: 10.1007/s10905-006-9019-2
Dittmann L., Schausberger P. (2017). Adaptive aggregation by spider mites under predation risk. Sci. Rep. 7, 10609. doi: 10.1038/s41598-017-10819-8
Elhalawany A. S. (2019). Influence of some host plants and temperature on biological aspects of the citrus brown mite, Eutetranychus orientalis (Llein) (Acari: Actinedida: Tetranychidae). Ann. Agr. Sci. Moshtohor 57, 745–754.
Fisher R. A. (1930). The genetical theory of natural selection (Oxford: Clarendon Press). doi: 10.5962/bhl.title.27468
Foott W. H. (1963). Competition between two species of mites. II. Factors influencing intensity. Can. Entomol. 95, 45–57. doi: 10.4039/Ent9545-1
Gerson U. (1979). Silk production in tetranychus (Acari: tetranychidae) in Recent advances in acarology, vol. 1 . Ed. Rodriguez J. C. (Academic Press, New York), 177–188.
Gerson U. (1985). Webbing, in spider mites. Their biology, natural enemies and control Vol. 1A. Eds. Helle W., Sabelis M. W. (Amsterdam, The Netherlands: Elsevier Science), 223–232.
Gotoh T. (1983). Life-history parameters of three species of Schizotetranychus on deciduous trees (Acarina: Tetranychidae). Appl. Entomol. Zool. 18, 122–128. doi: 10.1303/aez.18.122
Gotoh T. (1986). Life-history parameters of the hawthorn spider mite, Tetranychus viennensis Zacher (Acarina: Tetranychidae), on deciduous trees. Appl. Entomol. Zool. 21, 389–393. doi: 10.1303/aez.21.389
Gotoh T. (1987a). Life-history parameters of two species of the genus Eotetranychus (Acarina: Tetranychidae) on deciduous trees. Appl. Entomol. Zool. 22, 45–51. doi: 10.1303/aez.22.45
Gotoh T. (1987b). Comparison of life-history parameters of two forms of Panonychus akitanus Ehara (Acarina: Tetranychidae). Appl. Entomol. Zool. 22, 119–124. doi: 10.1303/aez.22.119
Gotoh T. (1989). Seasonal life cycles and diapause in three species of Schizotetranychus (Acarina : Tetranychidae). Appl. Entomol. Zool. 24, 407–414.
Gotoh T., Ishikawa Y., Kitashima Y. (2003). Life-history traits of the six Panonychus species from Japan (acari: tetranychidae). Exp. Appl. Acarol. 29, 241–252. doi: 10.1023/A:1025810731386
Gotoh T., Sugimoto N., Pallini A., Knapp M., Hernandez-Suarez E., Ferragut F., et al. (2010). Reproductive performance of seven strains of the tomato red 4 spider mite Tetranychus evansi (Acari: Tetranychidae) at five 5 temperatures. Exp. Appl. Acarol. 52, 239–259. doi: 10.1007/s10493-010-9362-z
Grostal P., Dicke M. (1999). Direct and indirect cues of predation risk influence behavior and reproduction of prey: a case for acarine interactions. Behav. Ecol. 10, 422–427. doi: 10.1093/beheco/10.4.422
Gutierrez J., Helle W. (1985). Evolutionary changes in the tetranychidae in Spider mites. Their biology, natural enemies and control, vol. 1A . Eds. Helle W., Sabelis M. W. (Elsevier Science, Amsterdam, The Netherlands), 91–107.
Hamilton W. D. (1967). Extraordinary sex ratios. Science 156, 477–488. doi: 10.1126/science.156.3774.477
Harvey P. H., Pagel M. D. (1991). The comparative method in evolutionary biology (Oxford: Oxford University Press). doi: 10.1093/oso/9780198546412.001.0001
Hazan A., Gerson U., Tahori A. S. (1973). Life history and life tables of the carmine spider mite. Acarologia 15, 414–440.
Hazan A., Gerson U., Tahori A. S. (1974). Spider mite webbing. I. The production of webbing under various environmental conditions. Acarologia 16, 68–84.
Helle W. (1967). Fertilization in the two-spotted spider mite (Tetranychus urticae: Acari). Entomol. Exp. Appl. 10, 103–110. doi: 10.1111/j.1570-7458.1967.tb00049.x
Horita M., Chittenden A. R., Sato Y., Saito Y. (2004). Function of web box as an anti-predator barrier in the spider mite, Schizotetranychus recki. J. Ethol 22, 105–108. doi: 10.1007/s10164-003-0101-3
Imbachi-Loípez K., Estrada-Venegas E. G., Equihua-Martiínez A., Mesa-Cobo N. C. (2017). Biologiía de Oligonychus perseae Tuttle, Baker y Abbatiello 1976 (Acari: Tetranychidae) en Persea americana Miller var. Hass bajo condiciones laboratorio Fitosanidad 21, 81–87.
Ito K. (2019). Lethal attack of Schizotetranychus brevisetosus Ehara (Acari: Tetranychidae) on predatory midge larva. Syst. Appl. Acarol. 24, 187–193. doi: 10.11158/saa.24.2
Jeffries M. J., Lawton J. H. (1984). Enemy free space and the structure of ecological communities. Biol. J. Lin. Soc 23, 269–286. doi: 10.1111/j.1095-8312.1984.tb00145.x
Jeppson L. R., Keifer H. H., Baker E. W. (1975). Mites injurious to economic plants (Berkeley: University of California Press). doi: 10.1525/9780520335431
Kanazawa M., Sahara K., Saito Y. (2011). Silk threads function as an A’dhesive cleaner’ for nest space in a social spider mite. Proc. R. Soc B 278, 1653–1660. doi: 10.1098/rspb.2010.1761
Kobayashi H., Sato Y., Egas M. (2022). Males mate with females even after sperm depletion in the two-spotted spider mite. Exp. Appl. Acarol. 86, 465–477. doi: 10.1007/s10493-022-00706-x
Kondo A., Takafuji A. (1985). Resource utilization pattern of two species of tetranychid mites (Acarina: Tetranychidae). Res. Popul. Ecol. 27, 145–157. doi: 10.1007/BF02515487
Kunimoto Y., Shinkaji N., Amano H. (1991). Reproductive isolation between different types of Panonychus citri (McGregor) and P. ulmi (Koch) (Acari: Tetranychidae). Jpn. J. Appl. Entomol. Zool. 35, 103–108. doi: 10.1303/jjaez.35.103
Lavola A., Julkunen-Tiitto R., Roininen H., Aphalo P. (1998). Host-plant preference of an insect herbivore mediated by UV-B and CO2 in relation to plant secondary metabolites. Biochem. Syst. Ecol. 26, 1–12. doi: 10.1016/S0305-1978(97)00104-X
Le Goff G. J., Mailleux A.-C., Detrain C., Deneubourg J.-L., Clotuche G., Hance T. (2010). Group effect on fertility, survival and silk production in the web spinner Tetranychus urticae (Acari: Tetranychidae) during colony foundation. Behaviour 147, 1169–1184. doi: 10.1163/000579510X510980
Lemos F., Sarmento R. A., Pallini A., Dias C. R., Sabelis M. W., Janssen A. (2010). Spider mite web mediates anti-predator behaviour. Exp. Appl. Acarol. 52, 1–10. doi: 10.1007/s10493-010-9344-1
Macke E., Magalhães S., Bach F., Olivieri I. (2011). Experimental evolution of reduced sex ratio adjustment under local mate competition. Science 334, 1127–1129. doi: 10.1126/science.1212177
Margolies D. C., Wrensch D. L. (1996). Temperature-induced changes in spider mite fitness: offsetting effects of development time, fecundity, and sex ratio. Entomol. Exp. Appl. 78, 111–118. doi: 10.1111/j.1570-7458.1996.tb00770.x
Masuda C., Tamura K., Chae Y., Fukuda T., Arakawa R., Ito K., et al. (2015). Lethal male combats in Schizotetranychus brevisetosus(Acari: Tetranychidae)on blue Japanese oak(Quercus glauca). Exp. Appl. Acarol 67, 259–268. doi: 10.1007/s10493-015-9938-8
Matsuda T., Kozaki T., Ishii K., Gotoh T. (2018). Phylogeny of the spider mite sub-family TetranyChinae (Acari: Tetranychidae) inferred from RNA-Seq data. PloS One 13, e0203136. doi: 10.1371/journal.pone.0203136
Matsuda T., Morishita M., Hinomoto N., Gotoh T. (2014). Phylogenetic analysis of the spider mite sub-family Tetranychinae (Acari: Tetranychidae) based on the mitochondrial COI gene and the 18S and the 5' end of the 28S rRNA genes indicates that several genera are polyphyletic. PLoS One 9, e108672. doi: 10.1371/journal.pone.0108672
Maynard Smith J., Price G. R. (1973). The logic of animal conflict. Nature 246, 15–18. doi: 10.1038/246015a0
McMurtry J. A., Croft B. A. (1997). Life-styles of phytoseiid mites and their roles in biological control. Annu. Rev. Entomol. 42, 291–321. doi: 10.1146/annurev.ento.42.1.291
McMurtry J. A., de Moraes G. J., Sorassou N. F. (2013). Revision of the lifestyles of phytoseiid mites (Acari: Phytoseiidae) and implications for biological control strategies. Syst. Appl. Acarol. 18, 297. doi: 10.11158/saa.18.4
McMurtry J. A., Huffaker C. B., van de Vrie M. (1970). Ecology of tetranychid mites and their natural enemies: A review: I. Tetranychid enemies: Their biological characters and the impact of spray practices. Hilgardia 40, 331–390. doi: 10.3733/hilg.v40n11p331
McMurtry J. A., Johnson H. G. (1966). An ecological study of the spider mite Oligonychus punicae (Hirst) and its natural enemies. Hilgardia 37, 363–402. doi: 10.3733/hilg.v37n11p363
McMurtry J. A., Scriven G. T. (1964). Studies on the feeding, reproduction, and development of Amblyseius hibisci (Acarina: Phytoseiidae) on various food substances. Ann. Entomol. Soc Am. 57, 649–655. doi: 10.1093/aesa/57.5.649
Mirza J. H., Kamran M., Alatawi F. J. (2018). Webbing life type and behavioral response of the date palm mite, Oligonychus afrasiaticus, to webbing residues on leaves and fruits of date palm. Exp. Appl. Acarol. 76, 197–207. doi: 10.1007/s10493-018-0297-0
Mitchell R. (1973). Growth and population dynamics of a spider mite (Tetranychus urticae K., Acarina: Tetranychidae). Ecology 54, 1349–1355. doi: 10.2307/1934198
Montserrat M., Peña F. D. L., Hormaza J. I., González-Fernández J. J. (2008). How do Neoseiulus californicus (Acari: Phytoseiidae) females penetrate densely webbed spider mite nests? Exp. Appl. Acarol. 44, 101–106. doi: 10.1007/s10493-008-9137-y
Mori K. (2000). Factors which caused variation in social systems of spider mites, Schizotetranychus celarius species group(Acari: Tetranychidae) (Sapporo: Hokkaido University).
Mori K., Saito Y. (2004). Nest size variation reflecting antipredator strategies in social spider mites of Stigmaeopsis(Acari: Tetranychidae). Behav. Ecol. Sociobiol. 56, 201–206. doi: 10.1007/s00265-004-0776-7
Mori K., Saito Y. (2005). Variation in social behavior within a spider mite genus, Stigmaeopsis (Acari: Tetranychidae). Behav. Ecol. 16, 232–238. doi: 10.1093/beheco/arh157
Mori K., Saito Y. (2013). Genetic basis of woven nest size in subsocial spider mites. Exp. Appl. Acarol. 60, 463–469. doi: 10.1007/s10493-013-9661-2
Morimoto K., Furuichi H., Yano S., Osakabe M. H. (2006). Web mediated interspecific competition among spider mites. J. Econ. Entomol 99, 678–684. doi: 10.1093/jee/99.3.678
Morita A., Ullah M. S., Sera T., Gotoh T. (2020). Effectiveness of second mating in sperm-depleted females in the two-spotted spider mite, Tetranychus urticae (Acari: Tetranychidae). Syst. Appl. Acarol. 25, 1561–1575. doi: 10.11158/saa.25.9.4
Murase A., Fujita K., Yano S. (2017). Behavioural flexibility in spider mites: oviposition site shifts based on past and present stimuli from conspecifics and predators. R. Soc Open Sci. 4, 170328. doi: 10.1098/rsos.170328
Mushtaq H. M. S., Ali H. M. S., Kamran M., Alatawi F. J. (2023). Effect of different host plants on life type characteristics of three spider mite pests (Acari: Prostigmata: Tetranychidae). Animals 13, 3433. doi: 10.3390/ani13223433
Mushtaq H. M. S., Kamran M., Alatawi F. J. (2021). Two new life types and assessment of web−associated behavioral characteristics of some Oligonychus species on various host plants. Exp. Appl. Acarol. 83, 211–27. doi: 10.1007/s10493-020-00579-y
Nickel J. L. (1960). Temperature and humidity relationships of Tetranychus desertorum Banks with special reference to distribution. Hilgardia 30, 41–100. doi: 10.3733/hilg.v30n02p041
Ohtsuka K., Osakabe M. (2009). Deleterious effects of UV-B radiation on herbivorous spider mites: they can avoid it by remaining on lower leaf surfaces. Environ. Entomol. 38, 920–929. doi: 10.1603/022.038.0346
Okada S., Yano S. (2021). Oviposition-site shift in phytophagous mites reflects a trade-off between predator avoidance and rainstorm resistance. Biol. Lett. 17, 20200669. doi: 10.1098/rsbl.2020.0669
Oku K. (2008). Is only the first mating effective for females in the Kanzawa spider mite, Tetranychus kanzawai (Acari: Tetranychidae)? Exp. Appl. Acarol. 45, 53–57. doi: 10.1007/s10493-008-9157-7
Oku K. (2009). Female mating strategy during precopulatory mate guarding in spider mites. Anim. Behav. 77, 207–211. doi: 10.1016/j.anbehav.2008.09.030
Oku K. (2010). Males of the two-spotted spider mite attempt to copulate with mated females: effects of double mating on fitness of either sex. Exp. Appl. Acarol. 50, 107–113. doi: 10.1007/s10493-009-9306-7
Oku K., Poelman E. H., de Jong P. W., Dicke M. (2018). Female response to predation risk alters conspecific male behaviour during pre-copulatory mate guarding. Ethology 124, 122–130. doi: 10.1111/eth.12710
Oku K., Yano S. (2007). Spider mites (Acari: Tetranychidae) deform their host plant leaves: an investigation from the viewpoint of predator avoidance. Ann. Entomol. Soc Am. 100, 69–72. doi: 10.1603/0013-8746(2007)100[69:SMATDT]2.0.CO;2
Oku K., Yano S., Takafuji A. (2003). Spider mite’s use of a refuge during the quiescent stage in the presence of a predator. Entomol. Exp. Appl. 108, 71–74. doi: 10.1046/j.1570-7458.2003.00069.x
Oku K., Yano S., Takafuji A. (2006). Host plant acceptance by the phytophagous mite Tetranychus kanzawai Kishida is affected by the availability of a refuge on the leaf surface. Ecol. Res. 21, 446–452. doi: 10.1007/s11284-005-0141-y
Osakabe M. (1988). Relationships between food substances and developmental success in Amblyseius sojaensis Ehara (Acarina: Phytoseiidae). Appl. Entomol. Zool. 23, 45–51. doi: 10.1303/aez.23.45
Osakabe M. (2021). Biological impact of ultraviolet−B radiation on spider mites and its application in integrated pest management. Appl. Entomol. Zool. 56, 139–1551. doi: 10.1007/s13355-020-00719-1
Osakabe M., Hongo K., Funayama K., Osumi S. (2006). Amensalism via webs causes unidirectional shifts of dominance in spider mite communities. Oecologia 150, 496–505. doi: 10.1007/s00442-006-0560-7
Otsuki H., Yano S. (2017). Within-patch oviposition site shifts by spider mites in response to prior predation risks decrease predator patch exploitation. Ethology 123, 453–459. doi: 10.1111/eth.12615
Ozawa A., Takafuji A. (1987). Inter-Specific crosses between Tetranychus urticae Koch and Tetranychus kanzawai Kishida. Jpn. J. Appl. Entomol. Zool. 31, 51–54. doi: 10.1303/jjaez.31.51
Potter D. A., Wrensch D. L. (1978). Interrupted matings and the effectiveness of second inseminations in the twospotted spider mite. Ann. Entomol. Soc Amer. 71, 882–885. doi: 10.1093/aesa/71.6.882
Potter D. A., Wrensch D. L., Johnston D. E. (1976a). Aggression and mating success in male spider mites. Science 193, 160–161. doi: 10.1126/science.193.4248.160
Potter D. A., Wrensch D. L., Johnston D. E. (1976b). Guarding, aggressive behavior, and mating success in male twospotted spider mites. Ann. Entomol. Soc Amer. 69, 707–711. doi: 10.1093/aesa/69.4.707
Putman W. L. (1962). Life history and behaviour of the predacious mite Typhlodromus (T.) causiglans Schuster (Acari: Phytoseiidae) in Ontario, with notes on the prey of related sopecies. Can. Entomol. 94, 163–177. doi: 10.4039/Ent94163-2
Rice W. R. (1989). Analyzing tables of statistical tests. Evolution 43, 223–225. doi: 10.2307/2409177
Ridley M. (1983). The explanation of organic diversity: The comparative method and adaptations for mating (Oxford: Clarendon Press).
Rocha M. S., Celada L. A., Rodrigues E. N. L., Costa-schmidt L. E. (2020). Under pressure: predation risk defining mating investment in matured spider mite Tetranychus urticae. Syst. Appl. Acarol. 25, 1359–1372. doi: 10.11158/saa.25.8.1
Rodrigues L. R., Figueiredo A. R. T., van Leeuwen T., Olivieri I., Magalhães S. (2020). Costs and benefits of multiple mating in a species with first-male sperm precedence. J. Anim. Ecol. 89, 1045–1054. doi: 10.1111/1365-2656.13171
Roeder C. M. (1992). Sex ratio response of the two-spotted spider mite (Tetranychus urticae Koch) to changes in density under local mate competition. Can. J. Zool. 70, 1965–1967. doi: 10.1139/z92-266
Roeder C., Harmsen R., Mouldey S. (1996). The effects of relatedness on progeny sex ratio in spider mites. J. Evol. Biol. 9, 143–151. doi: 10.1046/j.1420-9101.1996.9020143.x
Roy M., Brodeur J., Cloutier C. (2003). Temperature and sex allocation in a spider mite. Oecologia 135, 322–326. doi: 10.1007/s00442-002-1160-9
Sabelis M. W. (1981). Biological control of two-spotted spider mites using phytoseiid predators. Part I in Modeling the predator-prey interactionat the individual level(Wageningen: Centre for Agricultural Publishing and Documentation). Agricultural Research Reports. No.910. Pudoc.
Sabelis M. W. (1991). Life-history evolution of spider mites in The acari. Eds. Schuster R., Murphy P. W. (Chapman & Hall, London), 23–49.
Sabelis M. W., Bakker F. M. (1992). How predatory mites cope with the web of their tetranychid prey: a functional view on dorsal chaetotaxy in the Phytoseiidae. Exp. Appl. Acarol. 16, 203–255. doi: 10.1007/BF01193804
Saito Y. (1977a). Study on the spinning behavior of the spider mite (Acarina: Tetranychidae). I. Method for quantitative evaluation of mite webbing, and the relationship between webbing and walking. Jpn. J. Appl. Entmol. Zool. 21, 27–34. doi: 10.1303/jjaez.21.27
Saito Y. (1977b). Study on the spinning behavior of the spider mite (Acarina: Tetranychidae). II. The process of silk secretion and the webbing on the various stages of Tetranychus urticae Koch under different environmental conditions. Jpn. J. Appl. Entmol. Zool. 21, 150–157.
Saito Y. (1979). Comparative studies on life histories of three species of spider mites (Acarina: Tetranychidae). Appl. Entomol. Zool. 14, 83–94. doi: 10.1303/aez.14.83
Saito Y. (1983). The concept of “life types” in TetranyChinae. An attempt to classify the spinning behaviour of TetranyChinae. Acarologia 24, 377–391.
Saito Y. (1985). “Life types of spider mites.” in Spider Mites. Their Biology, Natural Enemies andControl, vol. 1A. Eds. Helle W., Sabelis M. W. (Amsterdam, The Netherlands: Elsevier Science), 253–264.
Saito Y. (1986a). Biparental defence in a spider mite (Acari: Tetranychidae)infesting Sasa bamboo. Behav. Ecol. Sociobiol. 18, 377–386. doi: 10.1007/BF00299668
Saito Y. (1986b). Prey kills predator: counter-attack success of a spider mite against its specific phytoseiid predator. Exp. Appl. Acarol. 2, 47–62. doi: 10.1007/BF01193354
Saito Y. (1990a). Harem” and “non-harem” type mating systems in two species of subsocial spider mites (Acari, Tetranychidae). Res. Popul. Ecol. 32, 263–278.
Saito Y. (1990b). Two new spider mite species of the Schizotetranychus celarius complex (Acari: Tetranychidae). Appl. Entomol. Zool. 25, 389–396. doi: 10.1303/aez.25.389
Saito Y. 1992. Subsociality in spider mites -Cooperation and aggression. In Ito Y. ed. Cooperation and aggression (in Japanese). (Tokyo: Toukai Univ. Press).
Saito Y. (1995a). Clinal variation in male-to-male antagonism and weaponry in a subsocial mite. Evolution 49, 413–417. doi: 10.1111/evo.1995.49.issue-3
Saito Y. (1995b). Sociobiological aspects of spider mite life types. J. Acarol. Soc Jpn. 4, 55–67. doi: 10.2300/acari.4.55
Saito Y. (1997). Sociality and kin selection in Acari in The evolution of social behavior in insects and arachnids. Eds. Choe J. C., Crespi B. J. (Cambridge Univ. Press, Cambridge), 443–457.
Saito Y. (2010). Plant mites and sociality. Diversity and evolution (Tokyo: Springer). doi: 10.1007/978-4-431-99456-5
Saito Y. (2018). Biology of Stigmaeopsis spider mites(Acari: Tetranychidae). Jpn. J. Appl. Entomol. Zool. 62, 215–229. doi: 10.1303/jjaez.2018.215
Saito Y., Chittenden A. R., Mori K., Ito K., Yamauchi A. (2008). An overlooked side effect of nest scattering behavior to decrease predation risk(Acari; Tetranychidae, Stigmaeidae). Behav. Ecol. Sociobiol. 63, 33–42. doi: 10.1007/s00265-008-0633-1
Saito Y., Kanazawa M., Sato Y. (2013). Life history differences between two forms of the social spider mite, Stigmaeopsis miscanthi. Exp. Appl. Acarol 60, 313–320. doi: 10.1007/s10493-012-9646-6
Saito Y., Mori K., Sakagami T., Lin J. Z. (2004). Reinstatement of the genus Stigmaeopsis Banks, with descriptions of two new species(Acari, Tetranychidae). Ann. Entomol. Soc Am. 97, 635–646. doi: 10.1603/0013-8746(2004)097[0635:ROTGSB]2.0.CO;2
Saito Y., Sahara K. (1999). Two clinal trends in male-male aggressiveness in a subsocial spider mite (Schizotetranychus miscanthi). Behav.Ecol. Sociobiol. 46, 25–29. doi: 10.1007/s002650050588
Saito Y., Sato Y., Chittenden A.R., Lin J.-Z., Zhang Y.-X. (2018). Description of two new species of Stigmaeopsis, Banks 1917 (Acari, Tetranychidae) inhabiting Miscanthus grasses (Poaceae). Acarologia 58, 414–429. doi: 10.24349/acarologia/20184250
Saito Y., Sato Y., Kongchuensin M., Chao J. T., Sahara K. (2019). New Stigmaeopsis species on Miscanthus grasses in Taiwan and Thailand (Acari, Tetranychidae). Syst. Appl. Acarol. 24, 675–682. doi: 10.11158/saa.24.4.12
Saito Y., Takada T. (2009). Conditions under which male-to-male aggression compromises cooperation in a game without learning. Open Evol. J. 3, 1–8. doi: 10.2174/1874404400903010001
Saito Y., Takahashi K. (1982). Study on variation of Schizotetranychus celarius (Banks) II. Comparison of mode of life between two sympatric forms (Acarina: Tetranychidae). Jpn. J. Ecol. 32, 69–78. doi: 10.18960/seitai.32.1_69
Saito Y., Ueno J. (1979). Life history studies on Schizotetrnychus celarius(Banks)and Aponychus corpuzae Rimando as compared with other tetranychid mite species(Acarina: Tetranychidae). Appl. Entomol. Zool 14, 445–452. doi: 10.1303/aez.14.445
Saito Y., Zhang Y.-X. (2017). Locking out predators by silk, a new counterattack behaviour in a social spider mite. Ecol. Entomol 42, 422–429. doi: 10.1111/een.12402
Saito Y., Zhang Y.-X., Mori K., Ito K., Sato Y., Chittenden A. R., et al. (2016). Variation in nesting behavior of eight species of spider mites, Stigmaeopsis having sociality. Sci. Nat. 103, 87. doi: 10.1007/s00114-016-1408-6
Sakai Y., Sudo M., Osakabe M. (2012). A comparison of the effects of gravity and the nutritional advantage of leaf surfaces on fecundity in the two-spotted spider mite (Acari: Tetranychidae). J. Acarol. Soc Jpn. 21, 1–6. doi: 10.2300/acari.21.1
Sarmento R. A., Lemos F., Dias C. R., Kikuchi W. T., Rodrigues J. C., Pallini A., et al. (2011). A herbivorous mite down-regulates plant defence and produces web to exclude competitors. PloS One 6, e23757. doi: 10.1371/journal.pone.0023757
Sato Y., Alba J. M., Egas M., Sabelis M. W. (2016). The role of web sharing, species recognition and host-plant defence in interspecific competition between two herbivorous mite species. Exp. Appl. Acarol. 70, 261–274. doi: 10.1007/s10493-016-0079-5
Sato Y., Alba J. M., Sabelis M. W. (2014a). Testing for reproductive interference in the population dynamics of two congeneric species of herbivorous mites. Heredity 113, 495–502. doi: 10.1038/hdy.2014.53
Sato Y., Breeuwer J. A. J., Egas M., Sabelis M. W. (2015). Incomplete premating and postmating reproductive barriers between two parapatric populations of a social spider mite. Exp. Appl. Acarol. 65, 277–291. doi: 10.1007/s10493-015-9878-3
Sato Y., Egas M., Sabelis M. W., Mochizuki A. (2013a). Male–male aggression peaks at intermediate relatedness in a social spider mite. Ecol. Evol. 3, 2661–2669. doi: 10.1002/ece3.661
Sato Y., Sabelis M. W., Egas M. (2014b). Alternative male mating behaviour in the two-spotted spider mite: dependence on age and density. Anim. Behav. 92, 125–131. doi: 10.1016/j.anbehav.2014.03.032
Sato Y., Sabelis M. W., Egas M., Faraji F. (2013b). Alternative phenotypes of male mating behaviour in the two-spotted spider mite. Exp. Appl. Acarol. 61, 31–41. doi: 10.1007/s10493-013-9673-y
Sato Y., Saito Y. (2006). Nest sanitation in social spider mites: Interspecific differences in defecation behavior. Ethology 112, 664–669. doi: 10.1111/j.1439-0310.2005.01184.x
Sato Y., Saito Y. (2007). Can the extremely female-biased sex ratio of the social spider mites be explained by Hamilton’s local mate competition model? Ecol. Entomol. 32, 597–602. doi: 10.1111/j.1365-2311.2007.00908.x
Sato Y., Saito Y. (2008). Evolutionary view of waste management behavior using volatile chemical cues in social spider mites. J. Ethol 26, 267–272. doi: 10.1007/s10164-007-0069-5
Sato Y., Saito Y., Sakagami T. (2003). Rules for nest sanitation in a social spider mite, Schizotetranychus miscanthi Saito (Acari: Tetranychidae). Ethology 109, 713–724. doi: 10.1046/j.1439-0310.2003.00905.x
Satoh Y., Yano S., Takafuji A. (2001). Mating strategy of spider mite, Tetranychus urticae (Acari: Tetranychidae) males: postcopulatory guarding to assure paternity. Appl. Entomol. Zool 36, 41–45. doi: 10.1303/aez.2001.41
Schausberger P., Yano S., Sato Y. (2021). Cooperative behaviors in group-living spider mites. Front. Ecol. Evol. 9, 745036. doi: 10.3389/fevo.2021.745036
Shih C. I. T., Poe S. L., Cromroy H. L. (1976). Biology, life table, and intrinsic rate of increase of Tetranychus urticae. Ann. Entomol. Soc Amer. 69, 362–364. doi: 10.1093/aesa/69.2.362
Shimoda T., Kishimoto H., Takabayashi J., Amano H., Dicke M. (2009). Comparison of thread-cutting behavior in three specialist predatory mites to cope with complex webs of Tetranychus spider mites. Exp. Appl. Acarol. 47, 111–120. doi: 10.1007/s10493-008-9205-3
Southwood T. R. E. (1977). Habitat, the template for ecological strategies? J. Anim. Ecol. 46, 337–365. doi: 10.2307/3817
Takafuji A., Fujimoto H. (1985). Reproductive compatibility between populations of the citrus red mite, Panonychus citri (McGregor) (Acarina: Tetranychidae). Res. Popul. Ecol. 27, 361–372. doi: 10.1007/BF02515473
Tamura K., Ito K. (2017). Extremely low fecundity and highly female-biased sex ratio in nest-living spider mite Schizotetranychus brevisetosus (Acari: Tetranychidae). Syst. Appl. Acarol. 22, 170–183. doi: 10.11158/saa.22.2
Tanigoshi L. K., Browne R. W., Hoyt S. C. (1979). Aspects of the relationship between Panonychus ulmi (Koch) and Tetranychus McDanieli McGregor in Simple Ecosystems (Acarina: Tetranychidae). Int. J. Acarol. 5, 285–290. doi: 10.1080/01647957908683174
Tien N. S. H., Massourakis G., Sabelis M. W., Egas M. (2011). Mate choice promotes inbreeding avoidance in the two-spotted spider mite. Exp. Appl. Acarol. 54, 119–124. doi: 10.1007/s10493-011-9431-y
Tsuji N., Chittenden A. R., Ogawa T., Takada T., Zhang Y.-X., Saito Y. (2011). The possibility of sustainable pest management by introducing bio-diversity: simulations of pest mite outbreak and regulation. Sustain. Sci. 6, 97–107. doi: 10.1007/s11625-010-0113-1
Ullah M. S., Gotoh T., Lim U. T. (2014). Life history parameters of three phytophagous spider mites, Tetranychus piercei, T. truncatus and T. bambusae (Acari: Tetranychidae). J. Asia-Pacific Entomol. 17, 767–773. doi: 10.1016/j.aspen.2014.07.008
Vala F., Egas M., Breeuwer J., Sabelis M. W. (2004). Wolbachia affects oviposition and mating behaviour of its spider mite host. J. Evol. Biol. 17, 692–700. doi: 10.1046/j.1420-9101.2003.00679.x
Walter D. E., Proctor H. C. (1999). Mites: ecology, evolution and behaviour (Oxon: CABI Publishing). doi: 10.1079/9780851993751.0000
Weerawansha N., Wang Q., He X. Z. (2022). A haplodiploid mite adjusts fecundity and sex ratio in response to density changes during the reproductive period. Exp. Appl. Acarol. 88, 277–288. doi: 10.1007/s10493-022-00749-0
Williams G. C. (1966). Adaptation and natural selection. A critique of some current evolutionary thought (Princeton: Princeton University Press).
Wrensch D. L., Young S. S. Y. (1978). Effects of density and host quality on rate of development, survivorship, and sex ratio in the carmine spider mite. Environ. Entomol. 7, 499–501. doi: 10.1093/ee/7.4.499
Yanagida H., Saito Y., Mori K., Chittenden A. R. (2001). Egg-depositing behavior as a predator avoidance tactic of Yezonychus sapporoensis. J. Ethol. 19, 63–66. doi: 10.1007/s101640170019
Yano S. (2008). Collective and solitary behaviors of twospotted spider mite (Acari: Tetranychidae) are induced by trail following. Ann. Entomol. Soc Amer. 101, 247–252. doi: 10.1603/0013-8746(2008)101[247:CASBOT]2.0.CO;2
Yano S. (2012). Cooperative web sharing against predators promotes group living in spider mites. Behav. Ecol. Sociobiol 66, 845–853. doi: 10.1007/s00265-012-1332-5
Yano J., Saito Y., Chittenden A. R., Sato Y. (2011). Variation in counterattack success against a phytoseiid predator between two forms of the social spider mite Stigmaeopsis miscanthi. J. Ethol 29, 337–342. doi: 10.1007/s10164-010-0265-6
Yano S., Shirotsuka K. (2013). Lying down with protective setae as an alternative antipredator defence in a non-webbing spider mite. Springer Plus 2, 637. doi: 10.1186/2193-1801-2-637
Young S. S. Y., Wrensch D. L., Kongchuensin M. (1986). Control of sex ratio by female spider mites. Entomol. Exper. Appl. 40, 53–60. doi: 10.1111/j.1570-7458.1986.tb02155.x
Zhang Y.-X. (2002). Studies on factors causing outbreaks of pest mites in bamboo forests in Fujian, China (Sapporo: Hokkaido University). 212 pp.
Zhang Y.-X., Zhang Z.-Q., Saito Y., Liu Q., Ji J. (2004). On the causes of mite pest outbreaks in mono- and poly-cultured moso bamboo forests. Chin. J. Appl. Ecol. 15, 1161–1165.
Keywords: Tetranychinae, Phytoseiidae, Tetranychus, Panonychus, Stigmaeopsis, Typhrodromus
Citation: Saito Y and Sato Y (2024) Diversity in life types of spider mites. Front. Arachn. Sci. 3:1436082. doi: 10.3389/frchs.2024.1436082
Received: 21 May 2024; Accepted: 15 July 2024;
Published: 09 August 2024.
Edited by:
Jason E. Bond, University of California, Davis, United StatesReviewed by:
Everton Nei Lopes Rodrigues, São Paulo State University, BrazilBruno A. Buzatto, Flinders University, Australia
Copyright © 2024 Saito and Sato. This is an open-access article distributed under the terms of the Creative Commons Attribution License (CC BY). The use, distribution or reproduction in other forums is permitted, provided the original author(s) and the copyright owner(s) are credited and that the original publication in this journal is cited, in accordance with accepted academic practice. No use, distribution or reproduction is permitted which does not comply with these terms.
*Correspondence: Yutaka Saito, yutsatmite@sunny.ocn.ne.jp