- 1Department of Biology, The University of Akron, Akron, OH, United States
- 2School of Polymer Science and Polymer Engineering, The University of Akron, Akron, OH, United States
Introduction: The design of biological structures and the materials composing those structures are intimately connected to performance in biological systems. Spider webs present an excellent example of how design and materials interact during their function in capturing prey. Major shifts in how spider webs capture prey have occurred due to evolutionary changes in both web architecture and silk properties. However, these shifts are mostly described for long timescales deep within the spider’s tree of life. Hawaiian Tetragnatha presents an opportunity to ask if such shifts can occur at much shorter timescales because web design diverges significantly among closely related species on the same island while also converging with more distant relatives on other islands. Here, we provide an initial test of whether or not silk properties diversified during the recent adaptive radiation of Hawaiian Tetragnatha.
Methods: We obtained radial and capture spiral silk from orb webs for spiders on two islands and tested their tensile and adhesive properties. We also used solution-state NMR to compare the composition of low molecular weight compounds in the glue because of their influence on capture spiral stickiness.
Results: Results showed differences in the stiffness of radial silk among four populations of Hawaiian Tetragnatha, while extensibility remained unchanged. Although not statistically different, radial strength and toughness varied twofold among species. Stickiness varied threefold among the four populations of orb weavers. No conspicuous qualitative differences in the low molecular weight compound composition of aggregate glue were found, suggesting that differences in capture spiral stickiness were due to the amount or arrangement of glue droplets on threads.
Discussion: While our sampling is modest, our data provide the first evidence that silk properties can evolve measurably over the relatively short timescales of the adaptive radiation of Hawaiian Tetragnatha spiders.
1 Introduction
Adaptive radiations are ideal systems to study how evolution generates the diversity of life. Closely related species often diversify in their use of resources during adaptive radiation by diverging in key traits, termed character displacement (Darwin, 1859). The evolution of limb morphology in Anolis lizards from the Caribbean Islands (Losos, 1990), jaw structure in cichlid fishes in Africa (Schluter, 1993), and beak shape in Darwin’s finches in the Galapagos Islands (Lack, 1945) are well-studied examples. However, the degree to which the underlying materials from which those structures are built also coevolve with function is typically unknown. Do biomaterials remain largely unchanged during character displacement, or can they undergo the same rapid evolutionary changes seen in other types of traits?
Hawaiian Tetragnatha offers an excellent model system to test the diversification of biomaterials in adaptive radiation because the Hawaiian Islands present a “natural laboratory” for the study of evolution, where their isolation allowed repeated and explosive taxonomic diversification, generating endemism (Simon, 1987). Tetragnatha is the only endemic nocturnal orb-weaving spider in the archipelago and shows significant diversification of behaviors, morphology, physiology, and gene expression (Binford, 2001; Blackledge and Gillespie, 2004; Gillespie, 2016; Kennedy et al., 2019; Berger et al., 2021). Orb web architecture has diverged among sympatric species of Tetragnatha within each island, often showing convergent evolution of web shapes with species on other islands (Blackledge and Gillespie, 2004). However, whether or not silk material properties also change as web architecture evolves remains unknown.
Orb webs are stereotypical structures built primarily from two types of silk fibers: the major ampullate (MA) silk composes the outer frame and radii, while the glue-coated flagelliform silk forms the adhesive capture spirals. Major ampullate silk is most important for stopping flying prey due to its toughness (Swanson et al., 2006) and high energy damping (Kelly et al., 2011). Next, the adhesive capture spiral must hold insects long enough for spiders to attack their prey, which often struggle free within seconds after hitting an orb web (Rypstra, 1982; Blackledge and Zevenbergen, 2006). The flagelliform silk forms the core fiber of the capture spiral and is weaker than major ampullate but highly extensible, deforming over 500% of its original length (Blackledge and Hayashi, 2006). Aggregate glue coating the flagelliform silk is composed of glycoproteins and hygroscopic low molecular weight compounds (LMWCs) that are important in maintaining silk stickiness (Vollrath et al., 1990; Sahni et al., 2011; Amarpuri et al., 2015a). LMWCs in the droplets solvate and stabilize the glycoproteins (Sahni et al., 2014) and also aid in the absorption of water from the environment (Jain et al., 2018). By sequestering water from the environment, glue droplets can change glue viscosity, altering how the droplets spread on surfaces and deform during detachment (Opell et al., 2011). Variations in LMWC profiles can change glue viscosity and hence the adhesion of glue among different species of spiders (Amarpuri et al., 2015b). Capture spiral stickiness is also enhanced by structural interactions between the glue droplets and flagelliform axial fiber. The high extensibility of both components results in a suspension bridge morphology that recruits multiple glue droplets that simultaneously resist detachment (Opell and Hendricks, 2007). Therefore, adhesion is also affected by glue droplets’ size and the spacing between them. Additionally, variations in the environment [e.g., wind (Wu et al., 2013), humidity (Opell et al., 2017), UV radiation (Stellwagen et al., 2015), and spider diet (Blamires et al., 2017)], could have an effect in the spiders’ glue droplet properties with corresponding variability in adhesion. In this study, we looked at the material and chemical properties of capture spiral and major ampullate silk of three species of Hawaiian Tetragnatha as an initial test for evidence of diversification of silk properties in this young radiation of Hawaiian spiders.
2 Materials and methods
2.1 Spiders and silk collection
Webs from orb-weaving spiders (Tetragnatha) were collected in July 2015 at two sites in the Hawaiian archipelago: Waikamoi Nature Conservancy Preserve, Maui Island and Upper Waiakea Forest Reserve, Hawaii Island. Three species (Tetragnatha stela robusta Gillespie 1992, Tetragnatha trituberculata Gillespie 1992, and Tetragnatha hawaiensis Simon 1900) were commonly found at Waikamoi Preserve. Two other species (T. filiciphilia Gillespie 1992 and T. acuta Gillespie 1992) were found cohabiting in the same area but were not as abundant and thus were not included because of the low sample size. At Upper Waiakea Forest Reserve, only webs from T. hawaiensis were collected. With the assumption that no gene flow is occurring between Tetragnatha hawaiensis collected at Waikamoi Nature Conservancy Forest Preserve, Maui and T. hawaiensis collected at Upper Waiakea Forest Reserve, Hawaii Island (Roderick and Gillespie, 1998), these will be treated as distinct populations, respectively, called T. hawaiensis (Maui) and T. hawaiensis (Big Island) from this point forward. Although the Hawaiian Tetragnatha comprises more than these species, these were the only species found during fieldwork in these two locations. Dry weather during field season may have impacted the diversity and abundance of spiders found.
Hawaiian Tetragnatha are largely nocturnal and were located in the field from 20:00 until 02:00 hours. Whole webs were collected using plastic circle frames (15 cm in diameter) covered in double-sided tape. The frames were adhered to the webs, and the radial threads were then cut outside the frames using a butane lighter to retain the natural tension of the webs. On average, webs from the three species included in this study had diameters of 12–15 cm (Blackledge et al., 2003; Blackledge and Gillespie, 2004). When webs were larger than the circle frame, only the bottom half of the webs were collected because orb-web spiders can allocate different amounts of resources to upper and lower orb-halves (Blackledge et al., 2011). Webs were carried back to the field station using a waterproof box. Up to six samples of radii (major ampullate silk) and 20 samples of capture spiral were obtained from each web, suspended across 12.58 mm gaps in cardboard holders. Radial threads were secured with cyanoacrylate glue (Superglue©). Capture spiral threads were secured with water-soluble glue (Elmers©) because cyanoacrylate desiccates the glue droplets. Threads were kept in a dark box to prevent damage from UV light (Lai and Goh, 2015) until they were shipped to and tested at the University of Akron. The remains of the web after thread collection were gathered using a clean glass pipette for chemical composition analysis of the glue, with all webs pooled within each population. All insects or debris stuck to the web were removed prior to the collection of capture spirals. For some webs, capture threads were stuck together, and it was not possible to get good thread samples for mechanical testing, so these webs were only collected for chemical composition purposes.
2.2 Material properties
Radial threads were tested 3 months after collection. Even after just a month, radial silk could show higher stiffness and less strain and stress due to aging (Lepore et al., 2016). However, because silk samples were collected in the field over the course of a month and mailed to the University of Akron before any testing could occur, this time effect could not be avoided. Stress–strain curves were generated for major ampullate radial silk using a Nano Bionix test system (Agilent Technologies, Oak Ridge, TN, USA) following previously described methods (Blackledge et al., 2005; Sensenig et al., 2010). Fibers were extended at a strain rate of 0.015 s−1. A minimum of three threads per web were tested at room humidity [~40%–50% relative humidity (RH)]. Diameters of threads were measured from threads viewed under a Leica DM LB2 polarized light microscope (Leica Microsystems CMS GmbH, Wetzlar, Germany) at ×100 magnification. Photographs of the suspended radial threads were taken using a 5-Megapixel Olympus Q-Color5TM imaging system (Olympus America Inc., Melville, NY, USA) attached to the microscope. Measurements were taken at three points using Image-Pro Plus 6.2 software (Media Cybernetics, Inc., Bethesda, MD, USA) and averaged for one axial fiber per double-stranded thread. Material properties (tensile strength, extensibility, toughness, and stiffness) were obtained as previously described (Vollrath et al., 2001; Sensenig et al., 2010). Tensile strength, or true breaking stress, was calculated as the amount of force required to break a fiber relative to the instantaneous cross-sectional area. Extensibility, or true breaking strain, was calculated as the natural log of one plus the ratio of breaking length to the original length and refers to how much the material has stretched normalized to the instantaneous length. Toughness, or the energy absorbed by a fiber prior to rupture, was calculated as the area under the stress–strain curve. Stiffness, or Young’s modulus, was obtained from the initial slope of the stress–strain curve, in which the relationship of stress and strain was almost linear.
The adhesiveness of the capture spiral silk was measured 3 months after collection. Older capture spirals lose adhesion as they dry out (Agnarsson et al., 2008); however, adhesive testing was performed at high humidities, which rehydrates samples, and therefore little effect of aging is expected (Opell and Schwend, 2008). Capture silk threads were brought in contact with a clean, 5-mm-wide glass surface and pressed until they generated 15 µN. Samples were then held for 6 s and pulled off at an extension of 0.1 mm s−1 using the Nano Bionix test system (Agilent Technologies, Inc., Santa Clara, CA, USA) to generate force-extension curves (Agnarsson and Blackledge, 2009). The work to release was calculated from the area under the force-extension curve and represents the energy required to separate the thread from the glass substrate. Peak load was the maximum force of adhesion achieved by the system, and extensibility was the distance the capture spiral displaced until reaching the maximum force of adhesion. This distance includes both the stretching of the flagelliform fiber and the stretching of the glue droplets themselves. Three capture spiral threads were tested per web. To control for humidity, adhesion tests were performed inside a humidity chamber at 85% RH to mimic the natural conditions of the spiders’ habitat. Samples were placed inside the humidity chamber and allowed to acclimate for 2–3 min prior to testing, enough time for droplets to swell or contract to equilibrium. Prior to testing, the glue droplet length and width were measured from images of the suspended capture silk under a Leica DM LB2 polarized light microscope (Leica Microsystems) at ×100 magnification and the space between glue droplets at ×10magnification. Photographs of the suspended capture silk were taken at room humidity (~ 46% RH) using a 5-Megapixel Olympus Q-Color5TM imaging system (Olympus America Inc., Melville, NY, USA) attached to the microscope. Glue morphology measurements were done using Image-Pro Plus 6.2 software (Media Cybernetics Inc., Bethesda, MD, USA). The glue droplet volume, the distance between the droplets, and the glue volume per millimeter were calculated as in Liao et al. (2015).
2.3 Low molecular weight compound composition
We used 1H solution-state nuclear magnetic resonance (NMR) to compare the chemical composition of LMWCs present in the glue of each species (Sahni et al., 2014; Jain et al., 2015). The collated samples of webs for each species (T. hawaiensis (Big Island) ~ 22, T. hawaiensis (Maui) ~13, T. trituberculata ~ 9, and T. stelarobusta ~ 14) were first washed with deionized water (~10 mL) to remove the soluble LMWC components. Next, the soluble residue was lyophilized for about 10 h to remove the water and obtain the dried water-soluble LMWCs components. Finally, the dried extracts were dissolved in about 1 ml of 99.96% deuterated water (Sigma-Aldrich Solutions Merck KGaA, Darmstadt, Germany) and placed in 5-mm NMR glass tubes (Norell, Morganton, NC, USA). All experiments were performed on a Varian 300 MHz spectrometer at 298 K. The spectra were recorded with a scan size of ~ 512, acquisition time of ~3 s, a 900 pulse width of 15–16 µs, and a sweep width of 5 ppm in the 1H dimension. Although there may be additional peaks beyond the 5-ppm chemical shift (Hu et al., 2007; Wolff et al., 2023), this was beyond the scope of our study due to the limitations of the NMR equipment, leading to lower resolution and sensitivity, and the limited silk material that we could collect from webs. Once the spectra were processed, different peaks were assigned to known LMWCs using data reported in the literature (Anderson and Tillinghast, 1980; Tillinghast and Christenson, 1984; Tillinghast et al., 1987; Townley et al., 1991; Townley and Tillinghast, 2013; Sahni et al., 2014; Jain et al., 2015, 2006, 2012; Vollrath et al., 1990) and an unpublished database provided by Townley. We compared the LMWC profiles of Hawaiian Tetragnatha spiders to those of a mainland species, Tetragnatha laboriosa Hentz, 1850, using data from Jain et al. (2018).
2.4 Data analysis
All data were analyzed, and graphs were built using JMP Pro 17 (JMP Statistical Discovery LLC., SAS Institute Inc., Cary, NC, USA). Values were averaged across all threads tested for an individual spider. The data were checked for normality by the Shapiro–Wilk’s test. While major ampullate silk followed a normal distribution, adhesion test results and capture silk morphology did not, so those values were log-transformed. A Wilk’s Lambda multivariate analysis of variance (MANOVA) was used to see if the mechanical properties of the major ampullate silk and the capture spiral threads differed between the populations. Individual ANOVAs were used to compare each of the material properties (strength, extensibility, toughness, stiffness, and silk diameter) of major ampullate threads, the adhesiveness of capture spiral threads (ln work of adhesion, ln peak force of adhesion, and ln extension), and the morphology of capture threads (volume per droplet, spacing between droplets, and glue volume per mm) between the four populations of Tetragnatha: T. stelarobusta, T. trituberculata, T. hawaiensis (Maui), and T. hawaiensis (Big Island). Tukey’s honest significant difference tests were used post hoc when needed to identify which pairwise comparisons were significant among the populations. Individual spiders were used as a random variable in all tests.
3 Results
3.1 Material properties
The material properties of radial threads differed among the species (F(15, 58.37) = 3.87, p < 0.0001; Wilk’s Λ = 0.15, Figure 1). One-way analysis of variance showed that T. stelarobusta had a significantly stiffer silk than T. hawaiensis (Big Island) (21.75 GPa ± 4.54 GPa and 10.42 GPa ± 1.55 GPa, respectively) (F(3, 25) = 4.24, p = 0.015; Figure 1D). Even though no statistical differences were found for toughness (F(3, 25) = 2.45, p = 0.087, Figure 1A), true stain (F(3, 25) = 2.08, p = 0.128, Figure 1B), and true stress (F(3, 25) = 1.74, p = 0.185; Figure 1C), a trend can be observed with T. trituberculata having stronger, stretchier, and tougher silk (785.58 MPa ± 120.76 MPa, 0.19 mm/mm ± 0.04 mm/mm, and 85.09 MPa ± 21.51 MPa, respectively) and T. hawaiensis (Big Island) having the least strong, least stretchy, and least tough radial silk (523.85 MPa ± 76.99 MPa, 0.16 mm/mm ± 0.01 mm/mm, and 45.81 MPa ± 6.77 MPa, respectively). Radial thread diameter differed between the four populations of Tetragnatha spiders, following the same trend as strength, extensibility, and toughness (F(3, 25) = 0.18.15, p < 0.01; Figure 1F). The diameters of T. trituberculata silk were larger than T. hawaiensis (Big Island) (1.31 µm ± 0.05 µm vs. 1.05 µm ± 0.02 µm, respectively).
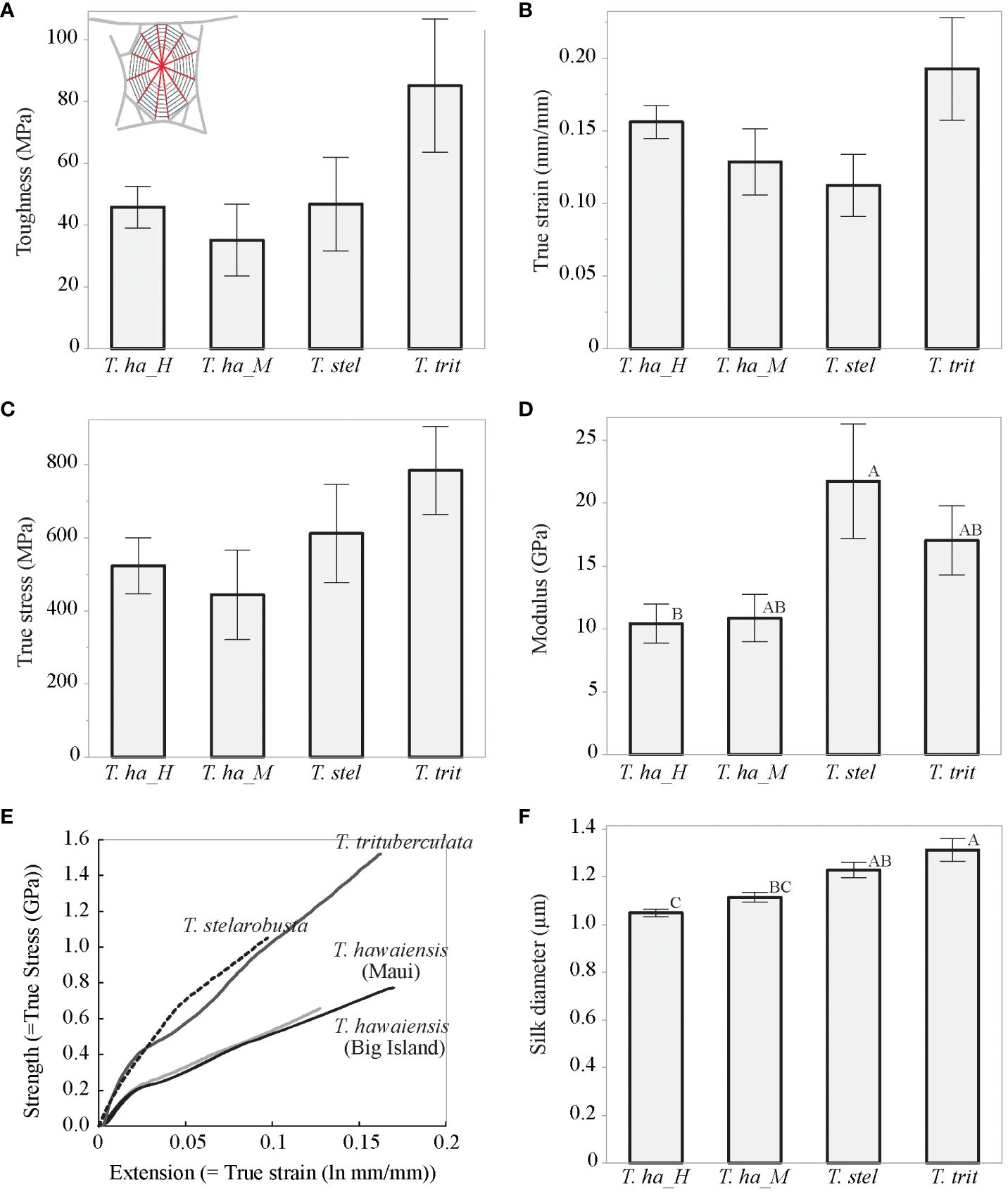
Figure 1 Material properties of radial threads for T. hawaiensis (Maui) (T. ha_M), T. hawaiensis (Big Island) (T. ha_H), T. stelarobusta (T. stel), and T. trituberculata (T. trit). Different letters represent significant differences (p < 0.05). Bars represent mean values with a standard error from the mean. (A–D) Mechanical properties. (E) Representative stress–strain curves for each of the groups. (F) Single fiber diameters. Tests were performed at room humidity (40%–50% RH).
Adhesive properties of capture silk differed among the different populations of spiders (F(9, 68.30) = 3.24, p = 0.003; Wilk’s Λ = 0.42, Figure 2). Work of adhesion (F(3, 30) = 5.53, p = 0.004) and peak force (F(3, 30) = 4.79, p = 0.008) differed between species, while extension remained similar (F(3, 30) = 0.69, p = 0.56). Tetragnatha trituberculata capture silk had a higher work of adhesion (0.79 µJ ± 0.12 µJ) and higher force of adhesion (170.9 µN ± 23.6 µN), followed by T. stelarobusta (0.67 µJ ± 0.21 µJ and 157.64 µN ± 38.37 µN, respectively), while T. hawaiensis (Maui) and T. hawaiensis (Big Island) showed the lowest stickiness with a work of adhesion of 0.33 µJ ± 0.1 µJ and 0.27 µJ ± 0.06 µJ and a force of adhesion of 89.35 µN ± 19.91 µN and 77.89 µN ± 12.68 µN.
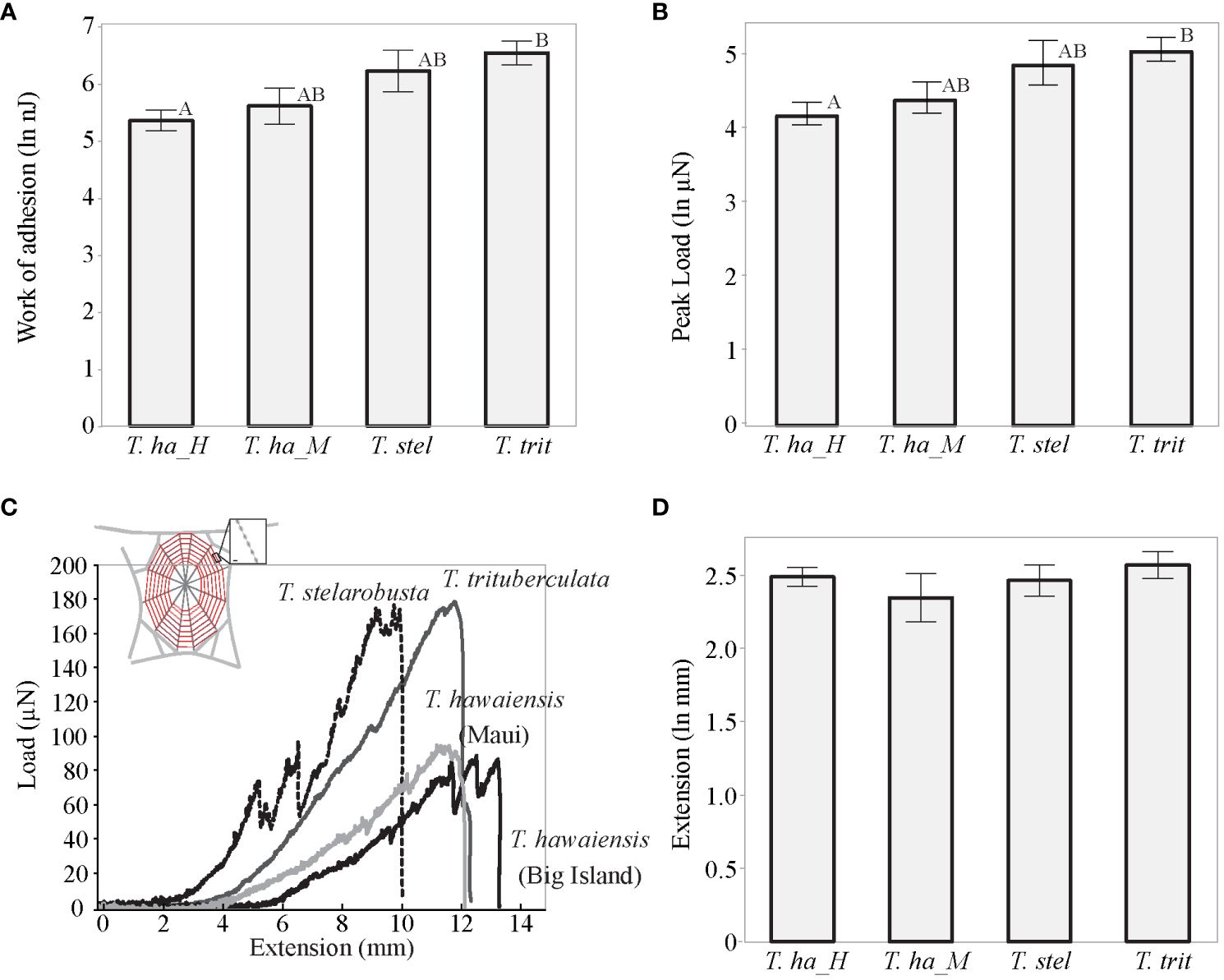
Figure 2 Adhesive properties of capture silk for T. hawaiensis (Maui) (T. ha_M), T. hawaiensis (Big Island) (T. ha_H), T. stelarobusta (T. stel), and T. trituberculata (T. trit). Different letters represent significant differences (p < 0.05). Bars represent mean values with a standard error from the mean. (A, B, D) Stickiness parameters: work of adhesion, peak force of adhesion, and extension. (C) Representative force-extension curves for each of the groups. Tests were performed at a relative humidity of ~85%.
Capture thread stickiness can be affected by the size and spacing of the glue droplet (Opell and Hendricks, 2007), which varied among the four groups in this study (F(9, 78.03) = 2.91, p = 0.005; Wilk’s Λ = 0.50, Table 1). Each structural property measured for glue varied among the four groups (glue droplet volume: F(3, 34) = 10.66, p < 0.001; spacing: F(3, 34) = 6.29, p = 0.002; glue volume per mm: F(3, 34) = 8.14, p = 0.0003). Tetragnatha stelarobusta had on average the highest glue volume and the largest spacing between glue droplets, which means that glue is getting packed in larger droplets that are relatively farther apart from each other. Tetragnatha trituberculata had the second-largest volume of glue per mm of thread, followed by T. hawaiensis (Maui). Tetragnatha hawaiensis (Big Island) had, in contrast, the lowest glue volume and the least space in between the droplets (Table 1).
3.2 Low molecular weight compound composition
Figure 3 shows the 1H NMR spectra illustrating different small molecules present in the glues of Tetragnatha spiders. The major components include betaine, choline, glycine, and alanine. The quantitative comparison of LMWCs among species was challenging due to the small samples, which led to a poor signal-to-noise ratio. However, we compared the LMWC profiles of Hawaiian Tetragnatha spiders to those of mainland (Ohio, USA) Tetragnatha laboriosa and found no major differences except for the lack of N-acetyl taurine in Hawaiian spiders. While the absolute lack of N-acetyl taurine in Hawaiian species is not clear because the starred peaks around 2 ppm and 3 ppm in T. trituberculata and T. hawaiensis (Big Island and Maui) may represent low concentrations of the compound, these unidentified peaks are clearly absent in the better-resolved spectrum of the glue of T. stelarobusta. LMWCs found in the glues of other local US species, such as T. versicolor Walckenaer 1841, and T. elongata Walckenaer 1841 also shows the presence of N-acetyl taurine, and in some cases, proline (Townley unpublished data), in addition to our reported LMWCs for Tetragnatha from the Hawaiian archipelago.
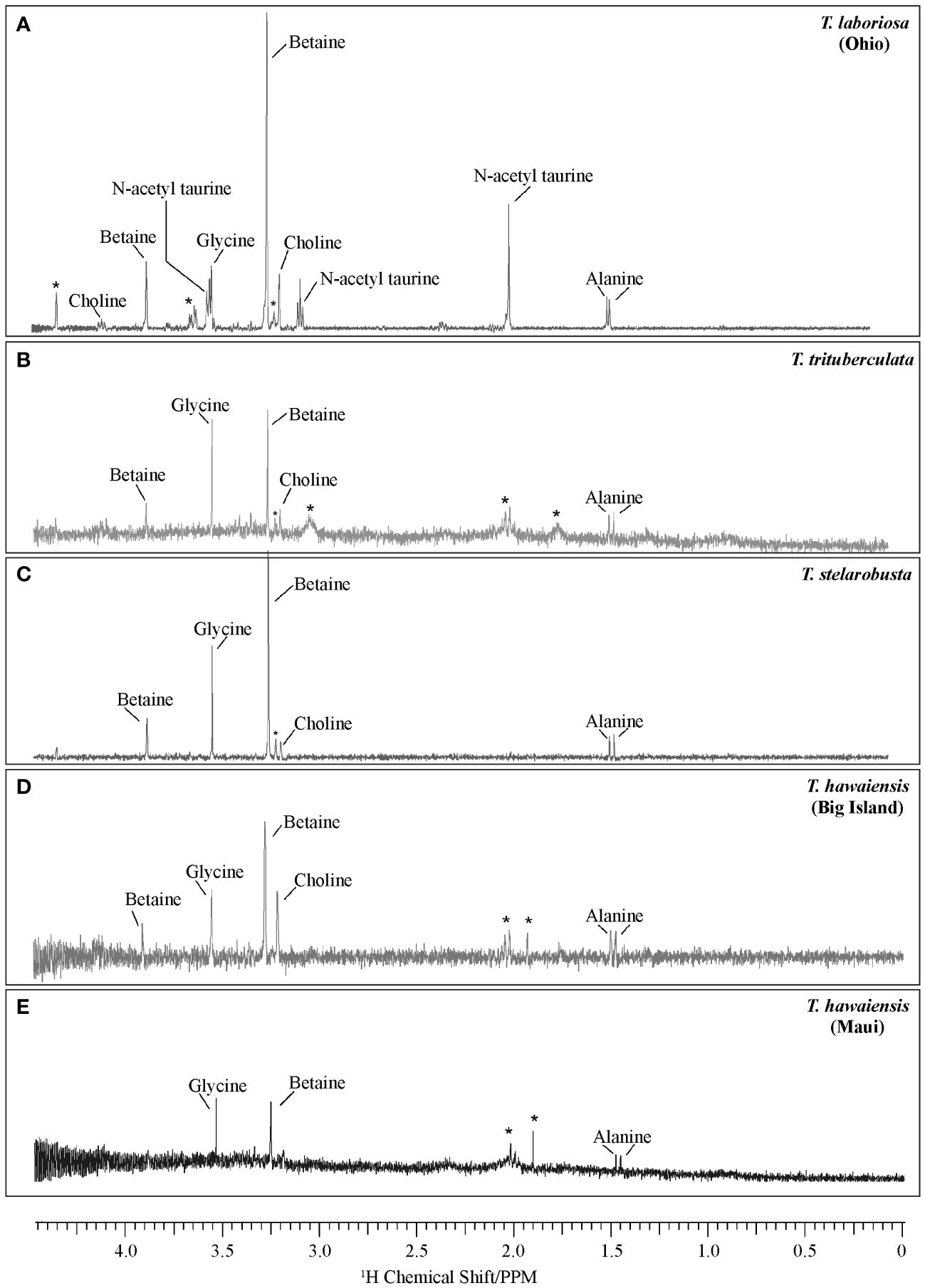
Figure 3 1H solution-state NMR results for soluble molecules of aggregate glue in capture silk of (A) T. laboriosa (Ohio), (B) T. trituberculata, (C) T. stelarobusta, (D) T. hawaiensis (Big Island), and (E) T. hawaiensis (Maui). Asterisk indicates ambiguity of the presence of molecules. Results for T. laboriosa (Ohio) are from Jain et al. (2018).
4 Discussion
We examined the silk properties of closely related species Hawaiian Tetragnatha to test if materials can diversify as other traits, like web-spinning behaviors, during adaptive radiations. We found evidence for evolutionary diversification in the properties of both the dry radial silk and viscid capture silk used to construct orb webs (Figures 1, 2, Table 1). These results suggest that the diversification of prey capture among sympatric Hawaiian Tetragnatha (Blackledge et al., 2003) is driven by changes in both the shapes of orb webs (Blackledge and Gillespie, 2004) and the silks from which those orb webs are constructed. While our sampling only provides an initial look at a small number of endemic Hawaiian Tetragnatha, we found tensile properties of radial silk varied almost twofold among species, with statistically significant differences in stiffness (Figure 1). The adhesion force for the stickiness of capture silk also varied twofold among species, while the work of adhesion varied threefold (Figures 2A, B).
Capture silk stickiness is affected by both the total volume of glue adhering to a surface and by the recruitment of multiple glue droplets during thread pull-off, with the total number of droplets simultaneously deforming determined by the size and spacing of glue droplets on the underlying axial thread (Opell and Hendricks, 2007). Big Island T. hawaiensis placed the lowest volume of glue per millimeter of capture spiral in their webs and also showed the lowest stickiness (both force and work). Neither the structure nor stickiness of capture threads varied significantly among other species. This may be due in part to the limitations of our testing. For instance, T. stelarobusta had the largest glue droplets with the widest distance between them (Table 1), allowing for only a few points of contact with the 5-mm-width substrate. This allowed only about 12 droplets per millimeter to simultaneously resist pull-off, while a fully formed “suspension bridge” can generate adhesion from as many as 20 droplets (Opell and Hendricks, 2009).
Tetragnatha stelarobusta primarily consumes moths, while the other species of Tetragnatha consume mostly small Drosophila and Tipulidae (Gillespie et al., 1997; Blackledge et al., 2003). Moths are not a common food for orb weavers because their scales rub off easily, allowing the moths to pull free from webs (Stowe, 1986). We speculate that the larger glue droplets of T. stelarobusta might help overcome anti-adhesive moth scales, as seen in other moth specialist spiders, like Cyrtarachne (Diaz et al., 2018) and Mastophora (Eberhard, 1980), that both use exceptionally large glue droplets. The composition of low-molecular-weight compounds in glue droplets was mostly identical to that of a mainland species, T. laboriosa. However, T. stelarobusta clearly lacked n-acetyl taurine, which was conspicuous in the glue of mainland T. laboriosa and corresponded to a set of ambiguously assigned peaks in all other Hawaiian Tetragnatha (Figure 3). Divergence of low molecular weight compounds is also seen in the glues of better-studied moth specialists and may relate to the glue’s unusually low viscosity that allows it to flow under moth scales (Diaz et al., 2018). While largely speculative, Tetragnatha stelarobusta may present a set of traits for its capture silk that are similar to those of other moth-specialist spiders and not easily recognized by just looking at web architecture. NMR signals being noisy, relative to other studies (e.g., Wolff et al., 2023), limited our ability to identify fine-scale differences and less abundant compounds for Hawaiian Tetragnatha. For starters, the strength of the NMR machine used in this study may have contributed to the low signal-to-noise ratio obtained. Additionally, this is, to our knowledge, the first study of LMWCs from glue collected in the wild, and challenges arise when using this type of samples, like the limited silk that we could collect from the webs, additional impurities introduced during web collection in the wild (e.g., pollen or prey getting caught in webs), and an uncontrolled spider diet. Using high-strength NMR supported by mass spectrometry techniques would be required for further understanding.
Our study is a modest first step in answering the question of how rapidly silk properties can evolve in spiders. Regardless, our results show that spider silk can diverge among closely related species over very short timescales (~1.2 MYA). The differences that we found in silk properties could result from diverse mechanisms that include changes in silk gene sequences (Hayashi et al., 1999) and expression (Marhabaie et al., 2014), as well as spinning conditions like shearing and reeling speed during silk production (Ortlepp and Gosline, 2004). Investigating these mechanisms, along with increased sampling and inclusion of more species from the Hawaiian archipelago, could reveal insight into how form and function evolve over short timescales in nature, like Caribbean anoles (Losos, 1990) or African cichlids (Lack, 1945).
Data availability statement
The original contributions presented in the study are included in the article/Supplementary Material. Further inquiries can be directed to the corresponding author.
Ethics statement
The manuscript presents research on animals that do not require ethical approval for their study.
Author contributions
AA-S: Conceptualization, Data curation, Formal analysis, Funding acquisition, Investigation, Methodology, Project administration, Software, Writing – original draft, Writing – review & editing. DJ: Investigation, Methodology, Writing – review & editing. AD: Funding acquisition, Resources, Supervision, Writing – review & editing. TB: Conceptualization, Funding acquisition, Methodology, Project administration, Resources, Supervision, Validation, Writing – review & editing.
Funding
The author(s) declare financial support was received for the research, authorship, and/or publication of this article. This work was partially funded by the American Arachnological Society Research Fund (2015) and the National Science Foundation.
Acknowledgments
We thank Pat Bily from The Nature Conservancy of Hawaii, as well as Joey S. Mello, Raymond McGuire, and Cynthia B. King from the Department of Forestry and Wildlife of Hawaii, for all their help in logistics and fieldwork. Thanks to Susan Kennedy for all her input and web pictures, and to Bricey Kepner, who did all the capture silk measurements. We would also like to thank the reviewers for their helpful comments.
Conflict of interest
The authors declare that the research was conducted in the absence of any commercial or financial relationships that could be construed as a potential conflict of interest.
Publisher’s note
All claims expressed in this article are solely those of the authors and do not necessarily represent those of their affiliated organizations, or those of the publisher, the editors and the reviewers. Any product that may be evaluated in this article, or claim that may be made by its manufacturer, is not guaranteed or endorsed by the publisher.
Supplementary material
The Supplementary Material for this article can be found online at: https://www.frontiersin.org/articles/10.3389/frchs.2024.1386353/full#supplementary-material.
References
Agnarsson I., Blackledge T. A. (2009). Can a spider web be too sticky? Tensile mechanics constrains the evolution of capture spiral stickiness in orb-weaving spiders. J. Zool. 278, 134–140. doi: 10.1111/j.1469-7998.2009.00558.x
Agnarsson I., Boutry C., Blackledge T. A. (2008). Spider silk aging: Initial improvement in a high-performance material followed by slow degradation. J. Exp. Zool A Ecol. Genet. Physiol. 309A, 494–504. doi: 10.1002/jez.480
Amarpuri G., Chaurasia V., Jain D., Blackledge T. A., Dhinojwala A. (2015a). Ubiquitous distribution of salts and proteins in spider glue enhances spider silk adhesion. Sci. Rep. 5, 1–6. doi: 10.1038/srep09030
Amarpuri G., Zhang C., Diaz C., Opell B. D., Blackledge T. A., Dhinojwala A. (2015b). Spiders tune glue viscosity to maximize adhesion. ACS Nano. 9, 11472–11478. doi: 10.1021/acsnano.5b05658
Anderson C. M., Tillinghast E. K. (1980). GABA and taurine derivatives on the adhesive spiral of the orb web of Argiope spiders, and their possible behavioural significance. Physiol. Entomol. 5, 101–106. doi: 10.1111/j.1365-3032.1980.tb00216.x
Berger C. A., Brewer M. S., Kono N., Nakamura H., Arakawa K., Kennedy S., et al. (2021). Shifts in morphology, gene expression, and selection underlie web loss in Hawaiian Tetragnatha spiders. BMC Ecol. Evol. 21, 1–17. doi: 10.1186/s12862-021-01779-9
Binford G. J. (2001). Differences in venom composition between orb-weaving and wandering Hawaiian Tetragnatha (Araneae). Biol. J. Linn. Soc 74, 581–595. doi: 10.1111/j.1095-8312.2001.tb01415.x
Blackledge T. A., Binford G. J., Gillespie R. G. (2003). Resource use within a community of Hawaiian spiders (Araneae : Tetragnathidae). Ann. Zool Fennici. 40, 293–303.
Blackledge T. A., Cardullo R. A., Hayashi C. Y. (2005). Polarized light microscopy, variability in spider silk diameters, and the mechanical characterization of spider silk. Invertebr. Biol. 124, 165–173. doi: 10.1111/j.1744-7410.2005.00016.x
Blackledge T. A., Gillespie R. G. (2004). Convergent evolution of behavior in an adaptive radiation of Hawaiian web-building spiders. Proc. Natl. Acad. Sci. U S A. 101, 16228–16233. doi: 10.1073/pnas.0407395101
Blackledge T. A., Hayashi C. Y. (2006). Silken toolkits: biomechanics of silk fibers spun by the orb web spider Argiope argentata (Fabricius 1775). J. Exp. Biol. 209, 2452–2461. doi: 10.1242/jeb.02275
Blackledge T. A., Kuntner M. M., Agnarsson I. (2011). “The form and function of spider orb webs: Evolution from silk to ecosystems,” in Advances in insect physiology (Cambridge, Massachusetts, USA: Academic Press) , 41st, vol. 41 . Ed. Casas J., 175–262).
Blackledge T. A., Zevenbergen J. M. (2006). Mesh width influences prey retention in spider orb webs. Ethology. 112, 1194–1201. doi: 10.1111/j.1439-0310.2006.01277.x
Blamires S. J., Hasemore M., Martens P. J., Kasumovic M. M. (2017). Diet-induced co-variation between architectural and physicochemical plasticity in an extended phenotype. J. Exp. Biol. 220, 876–884. doi: 10.1242/jeb.150029
Darwin C. (1859). On the origin of species by means of natural selection, or, the preservation of favoured races in the struggle for life (London: Cambridge University Press). doi: 10.5962/bhl.title.68064
Diaz C., Tanikawa A., Miyashita T., Amarpuri G., Jain D., Dhinojwala A., et al. (2018). Supersaturation with water explains the unusual adhesion of aggregate glue in the webs of the moth-specialist spider, Cyrtarachne akirai. R Soc. Open Sci. 5, 1–16. doi: 10.1098/rsos.181296
Eberhard W. G. (1980). The natural history and behavior of the bolas spider Mastophora dizzydeani Sp. N. (Araneidae). Psyche 87, 143–169. doi: 10.1155/1980/81062
Gillespie R. G. (2016). Island time and the interplay between ecology and evolution in species diversification. Evol. Appl. 9, 53–73. doi: 10.1111/eva.12302
Gillespie R. G., Croomt H. B., Hasty G. L. (1997). Phylogenetic relationships and adaptive shifts among major clades of Tetragnatha spiders (Araneae: Tetragnathidae) in Hawai’i. Pac Sci. 51, 380–394.
Hayashi C. Y., Shipley N. H., Lewis R. V. (1999). Hypotheses that correlate the sequence, structure, and mechanical properties of spider silk proteins. Int. J. Biol. Macromol. 24, 271–275. doi: 10.1016/S0141-8130(98)00089-0
Hu X., Yuan J., Wang X., Vasanthavada K., Falick A. M., Jones P. R., et al. (2007). Analysis of aqueous glue coating proteins on the silk fibers of the cob weaver, Latrodectus hesperus. Biochem. 46, 3294–3303. doi: 10.1021/bi602507e
Jain D., Amarpuri G., Fitch J., Blackledge T. A., Dhinojwala A. (2018). Role of hygroscopic low molecular weight compounds in humidity responsive adhesion of spider’s capture silk. Biomacromolecules. 19, 3048–3057. doi: 10.1021/acs.biomac.8b00602
Jain D., Zhang C., Cool L. R., Blackledge T. A., Wesdemiotis C., Miyoshi T., et al. (2015). Composition and function of spider glues maintained during the evolution of cobwebs. Biomacromolecules. 16, 3373–3380. doi: 10.1021/acs.biomac.5b01040
Kelly S. P., Sensenig A., Lorentz K. A., Blackledge T. A. (2011). Damping capacity is evolutionarily conserved in the radial silk of orb-weaving spiders. Zoology. 114, 233–238. doi: 10.1016/j.zool.2011.02.001
Kennedy S., Lim J. Y., Clavel J., Krehenwinkel H., Gillespie R. G. (2019). Spider webs, stable isotopes and molecular gut content analysis: Multiple lines of evidence support trophic niche differentiation in a community of Hawaiian spiders. Funct. Ecol. 33, 1722–1733. doi: 10.1111/1365-2435.13361
Lack D. (1945). The Galápagos finches (Geospizinae): a study in variation. Occas. pap. Calif. Acad. Sci. 21, 1–159.
Lai W. L., Goh K. L. (2015). Consequences of Ultra-Violet irradiation on the mechanical properties of spider silk. J. Funct. Biomater. 6, 901–916. doi: 10.3390/jfb6030901
Lepore E., Isaia M., Mammola S., Pugno N. (2016). The effect of ageing on the mechanical properties of the silk of the bridge spider Larinioides cornutus (Clerck 1757). Sci. Rep. 6, 24699. doi: 10.1038/srep24699
Liao C.-P., Blamires S. J., Hendricks M. L., Opell B. D. (2015). A re-evaluation of the formula to estimate the volume of orb web glue droplets. J. Arachnol. 43, 97–100. doi: 10.1636/M14-50.1
Losos J. B. (1990). The evolution of form and function - morphology and locomotor performance in West-Indian Anolis lizards. Evol. (N Y). 44, 1189–1203. doi: 10.2307/2409282
Marhabaie M., Leeper T. C., Blackledge T. A. (2014). Protein composition correlates with the mechanical properties of spider (Argiope trifasciata) dragline silk. Biomacromolecules 15, 20–29. doi: 10.1021/bm401110b
Opell B. D., Buccella K. E., Godwin M. K., Rivas M. X., Hendricks M. L. (2017). Humidity-mediated changes in an orb spider’s glycoprotein adhesive impact prey retention time. J. Exp. Biol. 220, 1313–1321. doi: 10.1242/jeb.148080
Opell B. D., Hendricks M. L. (2007). Adhesive recruitment by the viscous capture threads of araneoid orb-weaving spiders. J. Exp. Biol. 210, 553–560. doi: 10.1242/jeb.02682
Opell B. D., Hendricks M. L. (2009). The adhesive delivery system of viscous capture threads spun by orb-weaving spiders. J. Exp. Biol. 212, 3026–3034. doi: 10.1242/jeb.030064
Opell B. D., Karinshak S. E., Sigler M. A. (2011). Humidity affects the extensibility of an orb-weaving spider’s viscous thread droplets. J. Exp. Biol. 214, 2988–2993. doi: 10.1242/jeb.055996
Opell B. S., Schwend H. S. (2008). Persistent stickiness of viscous capture threads produced by araneoid orb-weaving spiders. J. Exp. Zool. 309A, 11–16. doi: 10.1002/jez.426
Ortlepp C. S., Gosline J. M. (2004). Consequences of forced silking. Biomacromolecules 5, 727–731. doi: 10.1021/bm034269x
Roderick G. K., Gillespie R. G. (1998). Speciation and phylogeography of Hawaiian terrestrial arthropods. Mol. Ecol. 7, 519–531. doi: 10.1046/j.1365-294x.1998.00309.x
Rypstra A. L. (1982). Building a better insect trap; An experimental investigation of prey capture in a variety of spider webs. Source: Oecologia 52, 31–36. doi: 10.1007/BF00349008
Sahni V., Blackledge T. A., Dhinojwala A. (2011). A review on spider silk adhesion. J. Adhes. 87, 595–614. doi: 10.1080/00218464.2011.583588
Sahni V., Miyoshi T., Chen K., Jain D., Blamires S. J., Blackledge T. A., et al. (2014). Direct solvation of glycoproteins by salts in spider silk glues enhances adhesion and helps to explain the evolution of modern spider orb webs. Biomacromolecules. 15, 1225–1232. doi: 10.1021/bm401800y
Schluter D. (1993). Adaptive radiation in sticklebacks - size, shape, and habitat use efficiency. Ecology. 74, 699–709. doi: 10.2307/1940797
Sensenig A., Agnarsson I., Blackledge T. A. (2010). Behavioural and biomaterial coevolution in spider orb webs. J. Evol. Biol. 23, 1839–1856. doi: 10.1111/j.1420-9101.2010.02048.x
Simon C. (1987). Hawaiian evolutionary biology - an introduction. Trends Ecol. Evol. 2, 175–178. doi: 10.1016/0169-5347(87)90015-2
Stellwagen S. D., Opell B. D., Clouse M. E. (2015). The impact of UVB radiation on the glycoprotein glue of orb-weaving spider capture thread. J. Exp. Biol. 218, 2675–2684. doi: 10.1242/jeb.123067
Stowe M. K. (1986). “Prey specialization in the araneidae,” in Spiders: webs, behavior, and evolution. Ed. Shear W. A. (Stanford University Press, Stanford), 101.
Swanson B. O., Blackledge T. A., Summers A. P., Hayashi C. Y. (2006). Spider dragline silk: correlated and mosaic evolution in high-performance biological materials. Evol. (N Y). 60, 2539–2551. doi: 10.1111/j.0014-3820.2006.tb01888.x
Tillinghast E. K., Christenson T. (1984). Observations on the chemical composition of the web of Nephila clavipes (Araneae, Araneidae). J. Arachnol. 12, 69–74.
Tillinghast E. K., Huxtable RJ W. H. W., Townley M. A. (1987). Evidence for the presence of gabamide on the web of orb weaving spiders. Comp. Biochem. Physiol. B. 88, 457–460. doi: 10.1016/0305-0491(87)90326-9
Townley M. A., Bernstein D. T., Gallagher K. S., Tillinghast E. K. (1991). Comparative study of orb web hygroscopicity and ahesive spiral composition in three araneid spiders. J. Exp. Zool A Ecol. Genet. Physiol. 259, 154–165. doi: 10.1002/jez.1402590203
Townley M. A., Pu Q., Zercher C. K., Neefus C. D., Tillinghast E. K. (2012). Small organic solutes in sticky droplets from orb webs of the spider Zygiella atrica (Araneae; Araneidae): β-Alaninamide is a novel and abundant component. Chem. Biodivers. 9, 2159–2174. doi: 10.1002/cbdv.201200077
Townley M. A., Tillinghast E. K. (2013). “Aggregate silk gland secretions of Araneoid spiders,” in Spider ecophysiology. Ed. Nentwig W. (Springer-Verlag, Berlin Heidelberg), 283–302.
Townley M. A., Tillinghast E. K., Neefus C. D. (2006). Changes in composition of spider orb web sticky droplets with starvation and web removal, and synthesis of sticky droplet compounds. J. Exp. Biol. 209, 1463–1486. doi: 10.1242/jeb.02147
Vollrath F., Fairbrother W. J., Williams R. J. P., Tillinghast E. K., Bernstein D. T., Gallagher K. S., et al. (1990). Compounds in the droplets of the orb spider’s viscid spiral. Nature. 345, 526–528. doi: 10.1038/345526a0
Vollrath F., Madsen B., Shao Z. Z. (2001). The effect of spinning conditions on the mechanics of a spider’s dragline silk. Proc. R. Soc B. 268, 2339–2346. doi: 10.1098/rspb.2001.1590
Wolff J. O., Cherry B. R., Yarger J. L., Adler L., Thomas D. S., Hook J. M., et al. (2023). Organic salt composition of pressure sensitive adhesives produced by spiders. Front. Ecol. Evol. 11. doi: 10.3389/fevo.2023.1123614
Keywords: adaptive radiation, major ampullate, aggregate silk, diversification, material properties
Citation: Alicea-Serrano AM, Jain D, Dhinojwala A and Blackledge TA (2024) Diversification of spider silk properties in an adaptive radiation of Hawaiian orb-weaving spiders. Front. Arachn. Sci. 3:1386353. doi: 10.3389/frchs.2024.1386353
Received: 15 February 2024; Accepted: 07 May 2024;
Published: 24 May 2024.
Edited by:
André Walter, Aarhus University, DenmarkReviewed by:
Sean J. Blamires, University of New South Wales, AustraliaGreta J. Binford, Lewis & Clark College, United States
Jeffery Lynn Yarger, Arizona State University, United States
Copyright © 2024 Alicea-Serrano, Jain, Dhinojwala and Blackledge. This is an open-access article distributed under the terms of the Creative Commons Attribution License (CC BY). The use, distribution or reproduction in other forums is permitted, provided the original author(s) and the copyright owner(s) are credited and that the original publication in this journal is cited, in accordance with accepted academic practice. No use, distribution or reproduction is permitted which does not comply with these terms.
*Correspondence: Angela M. Alicea-Serrano, ama251@uakron.edu
†Present address: Angela M. Alicea-Serrano, School of Polymer Science and Polymer Engineering, The University of Akron, Akron, OH, United States; Department of Biological Sciences, University of Massachusetts Lowell, Lowell, MA, United States
Dharamdeep Jain, Department of Biological Sciences, University of Massachusetts Lowell, Lowell, MA, United States
Todd A. Blackledge, Department of Biology, Integrated Bioscience Program, The University of Akron, Akron, OH, United States