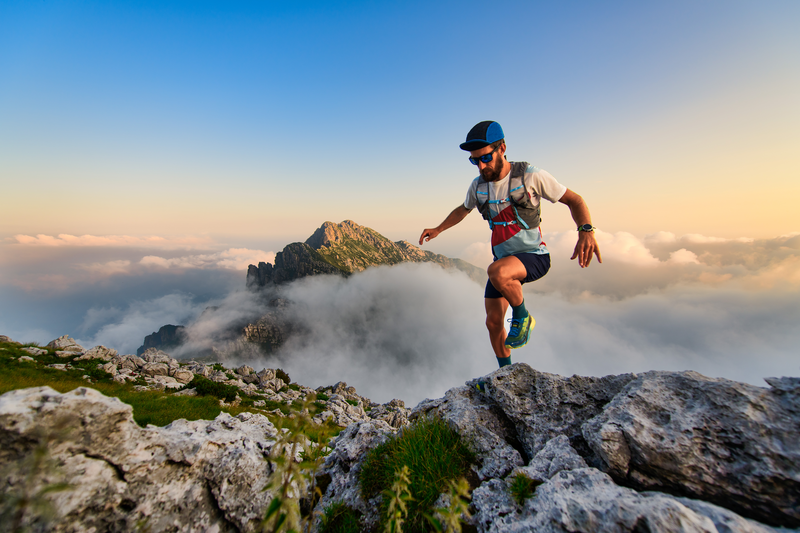
94% of researchers rate our articles as excellent or good
Learn more about the work of our research integrity team to safeguard the quality of each article we publish.
Find out more
SPECIALTY GRAND CHALLENGE article
Front. Arachn. Sci. , 12 October 2023
Sec. Arachnid Toxinology and Biochemistry
Volume 2 - 2023 | https://doi.org/10.3389/frchs.2023.1302040
Venom usage within arachnids is limited to spiders, scorpions, pseudoscorpions, ticks, and maybe even camel spiders if the toxic secretion from their epidermal glands is considered as venom (Aruchami and Sundara Rajulu, 1978; von Reumont et al., 2014). Among the venomous arachnids, spiders and scorpions have received by far the most attention from researchers (von Reumont et al., 2014). Overall, venomous arachnids comprise around 60,000 described extant species, with many more species yet to be characterized (Rein, 2023; World Arachnid Catalog, 2023). Venomous arachnids use their venoms mainly for prey capture and defense (Simone and van der Meijden, 2021; Lüddecke et al., 2022), although some spiders and scorpions use their venom coercively for courtship [males making females more compliant by “sexual bites or stings”; (Sentenska et al., 2020; Olguin-Perez et al., 2021)] and ticks employ venom to facilitate their parasitic lifestyle (Cabezas-Cruz and Valdes, 2014). Arachnid venoms are complex mixtures of metabolites, peptides, proteins and enzymes containing hundreds or even thousands of individual components for each species (Pineda et al., 2020). While arachnids employ these components to provide them with an evolutionary advantage in their respective ecological niche, researchers have realized that the astounding diversity of arachnid venom components can be utilized for the benefit of humanity. For example, applications as therapeutics, bioinsecticides, antiparasitic treatments or for diagnostic purposes are being actively pursued. I therefore hope the realization that arachnids could be beneficial for humans will eventually change their mostly negative public perception as being repulsive and potentially deadly. In the following paragraphs, I will discuss some ongoing and future challenges for toxinological and biochemical research on arachnids which also provides an opportunity to further expand our knowledge on these exciting creatures.
Of the 91,200 to 114,200 known species of arachnids (Schausperger, 2022), around 60,000 species are venomous, but the venoms of only a couple of hundred species have been studied (Pineda et al., 2018). While initially the focus of toxinological research was on the medically important species, this later changed when species that are not harmful to humans were increasingly explored (Herzig, 2021). However, the major limiting factor that remained was the small size of most arachnids and their correspondingly small venom yields. Research to date has therefore been predominantly focused on larger arachnid species (Herzig et al., 2019; Lüddecke et al., 2019). Technical improvements in experimental methods and sensitivity in the various omics fields (e.g. proteomics, transcriptomics, genomics, metabolomics) and in spectrometric (e.g. mass spectrometry, nuclear magnetic resonance spectrometry) and chromatographic (high performance liquid chromatography) techniques have now enabled researchers to study much smaller specimens. The most prominent examples are the recent transcriptomic and proteomic studies of the venom of pseudoscorpions (Santibanez-Lopez et al., 2018; Krämer et al., 2019) that are only a few millimeters in length and yield only few nanoliters of venom. The aim for future research in arachnid toxinology and in arachnid science in general (Kuntner, 2022) should therefore be to expand our knowledge of arachnid venoms into unexplored arachnid families or sub-families to provide a wider taxonomic coverage. Broadening our taxonomic knowledge will facilitate phylogenetic reconstructions of venom evolution in arachnids. With experimental techniques continuously improving, the size of arachnids should become less of a limiting factor preventing research on their venoms. The major bottleneck will then shift to sourcing arachnid specimens for research.
While conservation research into arachnids is still in its infancy (Agnarsson, 2023), international agreements like the Nagoya protocol are already in place in many countries that regulate the protection of natural resources, stipulating the need to acquire permits from the respective countries of origin for utilizing their biological sources (Colmenarez et al., 2023). While the idea behind Nagoya is commendable, i.e. to share financial benefits resulting from research with biological sources with the countries of organismal origin, it is already significantly impeding basic research. While I am in complete support of sharing financial benefits with the countries of origin, only a very small fraction of all basic research will ever produce any financial benefits. Furthermore, providing financial benefits to the countries of origin will not automatically ensure that they will be used towards protection of these species for future generations. A main challenge in the field will therefore be to remove unnecessary and unreasonable bureaucratic hurdles for sourcing arachnids for basic and non-profit research. In times of increasing extinction rates for many organisms caused by mostly human-made factors (Cowie et al., 2022), we should make the most of every opportunity to expand our current knowledge on as many arachnid species as possible before some of them become extinct. This also includes the controversial utilization of specimens already available in the pet trade, in particular if captive-bred specimen can be sourced (Herzig et al., 2023). While research always needs to uphold high moral and ethical standards, common sense should not be entirely disregarded. Trade with exotic arachnid pets will exist irrespective of whether scientists use it for sourcing specimens for their research or not and the overall trade numbers are unlikely to significantly change as a consequence. However, any knowledge obtained from this research could potentially provide lasting global benefits to future generations, and not just local short-lived benefits to a few countries of origin.
The last few decades have seen an immense improvement in our understanding of arachnid venom peptides, with many thousands of peptide toxins already been described (Rodriguez de la Vega et al., 2010; King and Hardy, 2013; Ahmadi et al., 2020). The use of omic methods has further accelerated peptide toxin discovery, with each new venom-gland transcriptome potentially yielding hundreds of new peptide sequences. While there is still much more to discover, particularly in previously neglected arachnid taxa (see Section 2), we should not focus our attention entirely on peptidic venom components. Smaller inorganic and organic components like metabolites, but also larger components like proteins and enzymes, play important ecological roles in the venoms. For example, according to the dual prey inactivation strategy, venom proteins and enzymes are important for the envenomation process by disturbing cellular homeostasis and acting as spreading factors, thereby facilitating the activity of peptide toxins (Kuhn-Nentwig et al., 2019). A wide variety of enzymes and proteins have been reported from scorpion venoms (Delgado-Prudencio et al., 2022), while enzymes and proteins are even dominating pseudoscorpion venoms (Santibanez-Lopez et al., 2018). At the lower end of the size spectrum, small molecules like metabolites have been reported to contribute to the overall activity of arachnid venoms (Wilson et al., 2017; Evans et al., 2020). A holistic approach would therefore be desirable for future research to capture the entire chemical diversity of arachnid venoms. This could also include research into whether particular components found in arachnid venoms are actually produced by the arachnid species itself or by microorganisms inhabiting their venom systems (Ul-Hasan et al., 2019; Esmaeilishirazifard et al., 2022; Cabezas-Cruz, 2023). In addition, a holistic approach should take into account that individual venom components often interact with others to potentiate activity and to provide synergistic effects (Lüddecke et al., 2022). Understanding the underlying mechanisms could therefore be advantageous when employing arachnid venom components for the benefit of humans (see Section 5.).
With research methodologies continuing to improve and techniques becoming ever more sophisticated, the volume of research data continues to expand. In toxinological research, the increasing use of venom-gland transcriptomics and other omics techniques provides us with massive datasets that require specific bioinformatic tools for their analysis. Making the resulting datasets permanently open accessible for everyone will be one of the key challenges in the field. Ideally, those datasets should be integrated into public databases to enable easy data access for non-specialists that do not have a background in bioinformatics or access to the specific bioinformatic tools. In the field of arachnid toxinology, two specific databases have been available. SCORPION2 focused on scorpion toxins but is no longer available (Tan et al., 2006). ArachnoServer is focused on spider toxins, but only a temporary version with limited functionality is currently available (Pineda et al., 2018). As part of the team involved in the development of ArachnoServer, I am now leading efforts to “refurbish” the application to future-proof it for the coming decades. For the new ArachnoServer, we aim to expand the focus from spider toxins into toxins from all venomous arachnids. We also plan to add new functionalities to ArachnoServer and are open to suggestions from the research community about specific new functions they would like to be incorporated. One idea is the incorporation of AlphaFold (Jumper et al., 2021) predicted structures for all toxins that do not yet have an experimentally determined three-dimensional structure.
The emergence of AlphaFold and other Artificial Intelligence (AI)-based programs like ChatGPT illustrates another challenge for the field and for science in general, which is how to best implement AI tools to maximize the gain for scientific discovery, and at the same time, eliminate noise and errors. AI tools might provide a solution for managing the ever-increasing volumes of research data created, but the toxinological research community needs to agree on the standards and boundaries for using AI tools. Another challenge with the increasing number of newly discovered arachnid toxins is their nomenclature. While a rational nomenclature has been implemented for peptide toxins (King et al., 2008), a unified nomenclature for all different venom components would be desirable. In addition, the current rational nomenclature has its own limitations, including the susceptibility to frequently occurring taxonomic changes at the genus and species level. Making toxin nomenclature more resistant to taxonomic changes and expanding it to include proteins, enzymes and metabolites will therefore be another key challenge for the entire toxinological community, not just for those working on arachnid venoms. Given that toxins are stored in the respective databases according to their rational names, a more stable and broadly applicable nomenclature is crucial for enabling permanent storage of arachnid toxin data.
Functional genomics techniques such as RNAi and CRISPR are already being used in related areas (Lau et al., 2019; Lüddecke et al., 2023), but haven’t yet been employed to increase our understanding of the biology of arachnid venom systems. I’m confident that the full utilization of these techniques for arachnid toxinology will provide significant leaps of knowledge for the entire field. Likewise, the biotechnological production of venom peptides, proteins, and enzymes is another area that requires increased attention from researchers in the future to enable a broad range of possible applications of arachnid venom derived leads.
Venomous animals and spiders in particular are often associated with a negative public image (Mammola et al., 2020) which is rarely correlated with the danger they pose to humans (Stuber and Nentwig, 2016; Hauke and Herzig, 2017). By further expanding the research on beneficial aspects of arachnid venoms, I hope that studies published in Frontiers in Arachnid Science will contribute to establishing a more positive public perception of venomous arachnids. In a recent review, I’ve identified six potential fields of application for venom compounds, including as pharmacological tools, drug leads, anti-infectives, insecticides, molecular probes and as tools for drug-target identification (Herzig et al., 2020), which all have been examined for arachnid venom peptides in the last few decades. Given the selectivity and potency of arachnid toxins, they have often been used as pharmacological tools for studying the physiological roles of ion channels (Suchyna et al., 2004; Osteen et al., 2016; Lin King et al., 2019) and as potential drug leads for diseases caused by ion channel dysfunctions [as reviewed in (Saez et al., 2010; Smith et al., 2015; Saez and Herzig, 2019; Ahmadi et al., 2020; Mendes et al., 2023)]. The antibacterial and anti-parasitic properties of some arachnid venom components have also been proposed for controlling bacteria and parasites (Saez and Herzig, 2019; Ageitos et al., 2022; Rincon-Cortes et al., 2022; Salimo et al., 2023). Selective modulators of acid-sensing ion channels (ASICs) including those from spider venoms like PcTx1 (Escoubas et al., 2000) and Hi1a (Chassagnon et al., 2017) have been crucial for understanding the physiological and pathophysiological roles of ASIC channels (Cristofori-Armstrong and Rash, 2017) and for establishing ASIC channels as a drug target for treating stroke and heart attack (Chassagnon et al., 2017; Redd et al., 2021). A striking example of using toxins as molecular probes has been realized with fluorescently tagged versions of chlorotoxin, a peptide from venom of the deathstalker scorpion Leiurus quinquestriatus (Veiseh et al., 2007). Given that chlorotoxin selectively binds to invasive brain tumors, the attached fluorescent probe provides neurosurgeons with a means of intraoperative visualization of tumor tissues to delineate tumor margins (Veiseh et al., 2007; Akcan et al., 2009). The application of arachnid toxins as bioinsecticides has received a boost with the commercial registration of a spider-venom-derived bioinsecticide on the US market in 2014 by US-based company Vestaron Corporation (Bomgardner, 2017; King, 2019). Dozens of novel insecticidal venom peptides have been discovered in recent decades from spiders and scorpions [reviewed in (Gurevitz et al., 2007; Schwartz et al., 2012; Windley et al., 2012; King and Hardy, 2013; King, 2019; Saez and Herzig, 2019)].
Venom research has come a long way from its beginning in the last century of studying only a few medically relevant species. Today’s sophisticated methods provide access to venoms from a wide variety of arachnids ranging from the largest tarantulas to species that are just a few millimeters in length. Nevertheless, the field of Arachnid Toxinology and Biochemistry to which this specialty section in Frontiers in Arachnid Science is dedicated, has barely scratched the surface and many new and exciting discoveries still lie ahead of us. Key challenges in this field include: 1. Increase of taxonomic coverage, which is not only limited by the size of the respective arachnids, but also by the challenges in sourcing sufficient specimens for research; 2. A holistic approach to arachnid venoms, which comprises the understanding of all individual components and their respective function in the venom, including potential synergistic interactions; 3. Utilizing emerging technologies for arachnid toxinology, with research methodologies and AI becoming ever more sophisticated, we need to utilize both in combination to maximize potential gains; 4. Benefits from arachnid venoms, ranging from pharmacological tools for studying ion channels and for identifying drug targets to drug leads, anti-infectives, molecular probes and insecticides.
VH: Writing – original draft, Writing – review & editing.
The author declare financial support was received for the research, authorship, and/or publication of this article. The author acknowledges financial support by the Australian Research Council (FT190100482).
I would like to thank Glenn F. King, Tim Lüddecke and Matjaž Kuntner for their helpful comments on this Specialty Grand Challenge.
The author declares that the research was conducted in the absence of any commercial or financial relationships that could be construed as a potential conflict of interest.
The author VH declared that they were an editorial board member of Frontiers, at the time of submission. This had no impact on the peer review process and the final decision.
All claims expressed in this article are solely those of the authors and do not necessarily represent those of their affiliated organizations, or those of the publisher, the editors and the reviewers. Any product that may be evaluated in this article, or claim that may be made by its manufacturer, is not guaranteed or endorsed by the publisher.
Ageitos L., Torres M. D. T., de la Fuente-Nunez C. (2022). Biologically active peptides from venoms: applications in antibiotic resistance, cancer, and beyond. Int. J. Mol. Sci. 23 (23), 1–17. doi: 10.3390/ijms232315437
Agnarsson I. (2023). Grand challenges in research on arachnid diversity, conservation, and biogeography. Front. Arachn. Sci. 2, 1101141. doi: 10.3389/frchs.2023.1101141
Ahmadi S., Knerr J. M., Argemi L., Bordon K. C. F., Pucca M. B., Cerni F. A., et al. (2020). Scorpion venom: detriments and benefits. Biomedicines 8 (5), 1–31. doi: 10.3390/biomedicines8050118
Akcan M., Stroud M. R., Hansen S. A., Daly N., Craik D., Olson J. (2009). Tumor Paint: a scorpion toxin peptide that targets lluorescent dye to brain tumor cells for real-time intraoperative imaging. Neuro-Oncol 11 (5), 697–697. Available at: https://espace.library.uq.edu.au/view/UQ:248914
Aruchami M., Sundara Rajulu G. (1978). An investigation on the poison glands and the nature of the venom of Rhagodes nicrocinctus (Solifugae: Arachnida). Natl. Acad. Sci. Lett. 1, 191–192.
Bomgardner M. M. (2017). Spider venom: an insecticide whose time has come? Chem. Eng. News 95 (11), 30–31. Available at: https://cen.acs.org/articles/95/i11/Spider-venom-insecticide-whose-time.html.
Cabezas-Cruz A. (2023). Grand challenges in arachnid microbiota and diseases. Front. Arachn. Sci. 2. doi: 10.3389/frchs.2023.1215831
Cabezas-Cruz A., Valdes J. J. (2014). Are ticks venomous animals? Front. Zool. 11, 47. doi: 10.1186/1742-9994-11-47
Chassagnon I. R., McCarthy C. A., Chin Y. K., Pineda S. S., Keramidas A., Mobli M., et al. (2017). Potent neuroprotection after stroke afforded by a double-knot spider-venom peptide that inhibits acid-sensing ion channel 1a. Proc. Natl. Acad. Sci. U.S.A. 114 (14), 3750–3755. doi: 10.1073/pnas.1614728114
Colmenarez Y. C., Smith D., Walsh G. C., France A., Corniani N., Vasquez C. (2023). Regulatory frameworks for the access and use of genetic resources in Latin America. Neotrop. Entomol. 52 (2), 333–344. doi: 10.1007/s13744-022-01017-x
Cowie R. H., Bouchet P., Fontaine B. (2022). The sixth mass extinction: fact, fiction or speculation? Biol. Rev. Camb. Philos. Soc 97 (2), 640–663. doi: 10.1111/brv.12816
Cristofori-Armstrong B., Rash L. D. (2017). Acid-sensing ion channel (ASIC) structure and function: Insights from spider, snake and sea anemone venoms. Neuropharmacol 127, 173–184. doi: 10.1016/j.neuropharm.2017.04.042
Delgado-Prudencio G., Cid-Uribe J. I., Morales J. A., Possani L. D., Ortiz E., Romero-Gutierrez T. (2022). The enzymatic core of scorpion venoms. Toxins 14 (4), 1–25. doi: 10.3390/toxins14040248
Escoubas P., De Weille J. R., Lecoq A., Diochot S., Waldmann R., Champigny G., et al. (2000). Isolation of a tarantula toxin specific for a class of proton-gated Na+ channels. J. Biol. Chem. 275 (33), 25116–25121. doi: 10.1074/jbc.M003643200
Esmaeilishirazifard E., Usher L., Trim C., Denise H., Sangal V., Tyson G. H., et al. (2022). Bacterial adaptation to venom in snakes and arachnida. Microbiol. Spectr. 10 (3), e0240821. doi: 10.1128/spectrum.02408-21
Evans E. R. J., McIntyre L., Northfield T. D., Daly N. L., Wilson D. T. (2020). Small molecules in the venom of the scorpion Hormurus waigiensis. Biomedicines 8 (8), 1–15. doi: 10.3390/biomedicines8080259
Gurevitz M., Karbat I., Cohen L., Ilan N., Kahn R., Turkov M., et al. (2007). The insecticidal potential of scorpion beta-toxins. Toxicon 49 (4), 473–489. doi: 10.1016/j.toxicon.2006.11.015
Hauke T. J., Herzig V. (2017). Dangerous arachnids - fake news or reality? Toxicon 138, 173–183. doi: 10.1016/j.toxicon.2017.08.024
Herzig V. (2021). Animal venoms - curse or cure? Biomedicines 9 (4), 1–8. doi: 10.3390/biomedicines9040413
Herzig V., Cristofori-Armstrong B., Israel M. R., Nixon S. A., Vetter I., King G. F. (2020). Animal toxins - Nature's evolutionary-refined toolkit for basic research and drug discovery. Biochem. Pharmacol. 181, 114096. doi: 10.1016/j.bcp.2020.114096
Herzig V., Hauke T. J., Lüddecke T. (2023). Unmasking trends and drivers of the international arachnid trade. Front. Arachn. Sci. 2, 1161383. doi: 10.3389/frchs.2023.1161383
Herzig V., King G. F., Undheim E. A. B. (2019). Resolving the taxonomic bias in spider venom research. Toxicon: X 100005, 1–4. doi: 10.1016/j.toxcx.2018.100005
Jumper J., Evans R., Pritzel A., Green T., Figurnov M., Ronneberger O., et al. (2021). Highly accurate protein structure prediction with AlphaFold. Nature 596 (7873), 583–589. doi: 10.1038/s41586-021-03819-2
King G. F. (2019). Tying pest insects in knots: the deployment of spider-venom-derived knottins as bioinsecticides. Pest Manage. Sci. 75, 2437–2445. doi: 10.1002/ps.5452
King G. F., Gentz M. C., Escoubas P., Nicholson G. M. (2008). A rational nomenclature for naming peptide toxins from spiders and other venomous animals. Toxicon 52 (2), 264–276. doi: 10.1016/J.Toxicon.2008.05.020
King G. F., Hardy M. C. (2013). Spider-venom peptides: structure, pharmacology, and potential for control of insect pests. Annu. Rev. Entomol. 58, 475–496. doi: 10.1146/annurev-ento-120811-153650
Krämer J., Pohl H., Predel R. (2019). Venom collection and analysis in the pseudoscorpion Chelifer cancroides (Pseudoscorpiones: Cheliferidae). Toxicon 162, 15–23. doi: 10.1016/j.toxicon.2019.02.009
Kuhn-Nentwig L., Langenegger N., Heller M., Koua D., Nentwig W. (2019). The dual prey-inactivation strategy of spiders-In-depth venomic analysis of Cupiennius salei. Toxins 11 (3), 1–37. doi: 10.3390/toxins11030167
Kuntner M. (2022). The seven grand challenges in arachnid science. Front. Arachn. Sci. 1082700. doi: 10.3389/frchs.2022.1082700
Lau M. T., Manion J., Littleboy J. B., Oyston L., Khuong T. M., Wang Q. P., et al. (2019). Molecular dissection of box jellyfish venom cytotoxicity highlights an effective venom antidote. Nat. Commun. 10 (1)1655. doi: 10.1038/s41467-019-09681-1
Lin King J. V., Emrick J. J., Kelly M. J. S., Herzig V., King G. F., Medzihradszky K. F., et al. (2019). A cell-penetrating scorpion toxin enables mode-specific modulation of TRPA1 and pain. Cell 178 (6), 1–13. doi: 10.1016/j.cell.2019.07.014
Lüddecke T., Herzig V., von Reumont B. M., Vilcinskas A. (2022). The biology and evolution of spider venoms. Biol. Rev. Camb. Philos. Soc. 97 (1), 163–178. doi: 10.1111/brv.12793
Lüddecke T., Paas A., Harris R. J., Talmann L., Kirchhoff K. N., Billion A., et al. (2023). Venom biotechnology: casting light on nature's deadliest weapons using synthetic biology. Front. Bioeng. Biotechnol. 11. doi: 10.3389/fbioe.2023.1166601
Lüddecke T., Vilcinskas A., Lemke S. (2019). Phylogeny-guided selection of priority groups for venom bioprospecting: harvesting toxin sequences in tarantulas as a case study. Toxins 11 (9), 1–10. doi: 10.3390/toxins11090488
Mammola S., Nanni V., Pantini P., Isaia M. (2020). Media framing of spiders may exacerbate arachnophobic sentiments. People Nat. 1–13. doi: 10.1002/pan3.10143
Mendes L. C., Viana G. M. M., Nencioni A. L. A., Pimenta D. C., Beraldo-Neto E. (2023). Scorpion peptides and ion channels: an insightful review of mechanisms and drug development. Toxins 15 (4), 1–21. doi: 10.3390/toxins15040238
Olguin-Perez L., Francke O. F., Carbajal-Saucedo A. (2021). Evidence of piercing and sexual differences in venom composition in a sexual stinging scorpion (Scorpiones: Euscorpiidae). J. Arachnol. 49, 98–107. doi: 10.1636/JoA-S-19-056
Osteen J. D., Herzig V., Gilchrist J., Emrick J. J., Zhang C., Wang X., et al. (2016). Selective spider toxins reveal a role for the Nav1.1 channel in mechanical pain. Nature 534 (7608), 494–499. doi: 10.1038/nature17976
Pineda S. S., Chaumeil P. A., Kunert A., Kaas Q., Thang M. W. C., Le L., et al. (2018). ArachnoServer 3.0: an online resource for automated discovery, analysis and annotation of spider toxins. Bioinformatics 34 (6), 1074–1076. doi: 10.1093/bioinformatics/btx661
Pineda S. S., Chin Y. K., Undheim E. A. B., Senff S., Mobli M., Dauly C., et al. (2020). Structural venomics reveals evolution of a complex venom by duplication and diversification of an ancient peptide-encoding gene. Proc. Natl. Acad. Sci. U.S.A. 117 (21), 11399–11408. doi: 10.1073/pnas.1914536117
Redd M. A., Scheuer S. E., Saez N. J., Yoshikawa Y., Chiu H. S., Gao L., et al. (2021). Therapeutic inhibition of acid-sensing ion channel 1a recovers heart function after ischemia-reperfusion injury. Circulation 144 (12), 947–960. doi: 10.1161/CIRCULATIONAHA.121.054360
Rein J. O. (2023) The scorpion files. Available at: https://www.ntnu.no/ub/scorpion-files/.
Rincon-Cortes C. A., Bayona-Rojas M. A., Reyes-Montano E. A., Vega-Castro N. A. (2022). Antimicrobial activity developed by scorpion venoms and its peptide component. Toxins 14 (11), 1–17. doi: 10.3390/toxins14110740
Rodriguez de la Vega R. C., Schwartz E. F., Possani L. D. (2010). Mining on scorpion venom biodiversity. Toxicon 56 (7), 1155–1161. doi: 10.1016/j.toxicon.2009.11.010
Saez N. J., Herzig V. (2019). Versatile spider venom peptides and their medical and agricultural applications. Toxicon 158, 109–126. doi: 10.1016/j.toxicon.2018.11.298
Saez N. J., Senff S., Jensen J. E., Er S. Y., Herzig V., Rash L. D., et al. (2010). Spider-venom peptides as therapeutics. Toxins 2 (12), 2851–2871. doi: 10.3390/toxins2122851
Salimo Z. M., Barros A. L., Adriao A. A. X., Rodrigues A. M., Sartim M. A., de Oliveira I. S., et al. (2023). Toxins from animal venoms as a potential source of antimalarials: a comprehensive review. Toxins 15 (6), 1–26. doi: 10.3390/toxins15060375
Santibanez-Lopez C. E., Ontano A. Z., Harvey M. S., Sharma P. P. (2018). Transcriptomic analysis of pseudoscorpion venom reveals a unique cocktail dominated by enzymes and protease inhibitors. Toxins 10 (5). doi: 10.3390/toxins10050207
Schausperger P. (2022). Grand challenges and bold opportunities in arachnid ecology and behavior. Front. Arachn. Sci. 1. doi: 10.3389/frchs.2022.1097945
Schwartz E. F., Mourao C. B., Moreira K. G., Camargos T. S., Mortari M. R. (2012). Arthropod venoms: a vast arsenal of insecticidal neuropeptides. Biopolymers 98 (4), 385–405. doi: 10.1002/bip.22100
Sentenska L., Sedo O., Pekar S. (2020). Biting and binding: an exclusive coercive mating strategy of males in a philodromid spider. Anim. Behav. 168, 59–68. doi: 10.1016/j.anbehav.2020.08.001
Simone Y., van der Meijden A. (2021). Armed stem to stinger: a review of the ecological roles of scorpion weapons. J. Venom. Anim. Toxins Incl. Trop. Dis. 27, e20210002. doi: 10.1590/1678-9199-JVATITD-2021-0002
Smith J. J., Lau C. H. Y., Herzig V., Ikonomopoulou M., King G. F., Rash L. D., et al. (2015). Chapter 8: “Therapeutic Applications of Spider-Venom Peptides” Venom to Drugs (Cambridge), 221–244. doi: 10.1039/9781849737876-00221
Stuber M., Nentwig W. (2016). How informative are case studies of spider bites in the medical literature? Toxicon 114, 40–44. doi: 10.1016/j.toxicon.2016.02.023
Suchyna T. M., Tape S. E., Koeppe R. E., Andersen O. S., Sachs F., Gottlieb P. A. (2004). Bilayer-dependent inhibition of mechanosensitive channels by neuroactive peptide enantiomers. Nature 430 (6996), 235–240. doi: 10.1038/nature02743
Tan P. T., Veeramani A., Srinivasan K. N., Ranganathan S., Brusic V. (2006). SCORPION2: a database for structure-function analysis of scorpion toxins. Toxicon 47 (3), 356–363. doi: 10.1016/j.toxicon.2005.12.001
Ul-Hasan S., Rodriguez-Roman E., Reitzel A. M., Adams R. M. M., Herzig V., Nobile C. J., et al. (2019). The emerging field of venom-microbiomics for exploring venom as a microenvironment, and the corresponding Initiative for Venom Associated Microbes and Parasites (iVAMP). Toxicon: X 4, 100016. doi: 10.1016/j.toxcx.2019.100016
Veiseh M., Gabikian P., Bahrami S. B., Veiseh O., Zhang M., Hackman R. C., et al. (2007). Tumor paint: a chlorotoxin:Cy5.5 bioconjugate for intraoperative visualization of cancer foci. Cancer Res. 67 (14), 6882–6888. doi: 10.1158/0008-5472.CAN-06-3948
von Reumont B. M., Campbell L. I., Jenner R. A. (2014). Quo vadis venomics? A roadmap to neglected venomous invertebrates. Toxins 6 (12), 3488–3551. doi: 10.3390/toxins6123488
Wilson D., Boyle G. M., McIntyre L., Nolan M. J., Parsons P. G., Smith J. J., et al. (2017). The aromatic head group of spider toxin polyamines influences toxicity to cancer cells. Toxins 9 (11). doi: 10.3390/toxins9110346
Windley M. J., Herzig V., Dziemborowicz S. A., Hardy M. C., King G. F., Nicholson G. M. (2012). Spider-venom peptides as bioinsecticides. Toxins 4 (3), 191–227. doi: 10.3390/Toxins4030191
World Arachnid Catalog (2023). World arachnid catalog (Switzerland: Natural History Museum Bern, Australia: Western Australian Museum Perth, Bonn, Germany: Leibniz Institute for the Analysis of Biodiversity Change). Available at: https://wac.nmbe.ch/.
Keywords: arachnid, venom, toxin, peptides, enzymes, taxonomic coverage, emerging technologies, human benefit
Citation: Herzig V (2023) Grand challenges in arachnid toxinology and biochemistry. Front. Arachn. Sci. 2:1302040. doi: 10.3389/frchs.2023.1302040
Received: 25 September 2023; Accepted: 27 September 2023;
Published: 12 October 2023.
Edited by:
Matjaz Kuntner, National Institute of Biology (NIB), SloveniaCopyright © 2023 Herzig. This is an open-access article distributed under the terms of the Creative Commons Attribution License (CC BY). The use, distribution or reproduction in other forums is permitted, provided the original author(s) and the copyright owner(s) are credited and that the original publication in this journal is cited, in accordance with accepted academic practice. No use, distribution or reproduction is permitted which does not comply with these terms.
*Correspondence: Volker Herzig, dmhlcnppZ0B1c2MuZWR1LmF1
Disclaimer: All claims expressed in this article are solely those of the authors and do not necessarily represent those of their affiliated organizations, or those of the publisher, the editors and the reviewers. Any product that may be evaluated in this article or claim that may be made by its manufacturer is not guaranteed or endorsed by the publisher.
Research integrity at Frontiers
Learn more about the work of our research integrity team to safeguard the quality of each article we publish.