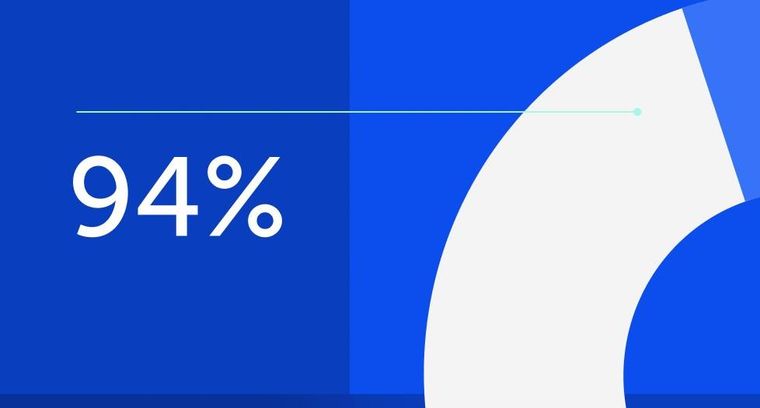
94% of researchers rate our articles as excellent or good
Learn more about the work of our research integrity team to safeguard the quality of each article we publish.
Find out more
SPECIALTY GRAND CHALLENGE article
Front. Arachn. Sci., 07 February 2023
Sec. Arachnid Diversity, Conservation and Biogeography
Volume 2 - 2023 | https://doi.org/10.3389/frchs.2023.1101141
Arachnids, an ancient lineage of chelicerate arthropods, are the largest order of carnivorous animals on the planet. Their diversity and abundance render them the prominent predators of insects and other arthropods in most ecosystems. Yet, they are relatively poorly known, for example, the known diversity of spider around 51,000 species represents but a fraction of their actual diversity that may be as great as 150-200,000 species. Arachnids as a whole may comprise a lineage of over a million species. Most of the yet to be discovered species are likely tropical. Recent years have seen a major growth in the understanding of arachnid biogeography, where they are highly informative due to great variability in dispersal potential. Some spiders are among the first organisms to arrive in any newly formed habitat, such as islands emerging from the sea, other arachnids may move less than a meter in their entire lives. With respect to conservation, research on arachnids is just beginning to emerge. Yet arachnids show great promise in conservation biology due to their diversity, role as key predators, and the highly geographically structured populations of dispersal limited groups. Thus opportunities in research of arachnid diversity, conservation, and biogeography abound.
The Arachnid Diversity, Conservation and Biogeography section of Frontiers in Arachnid Science aims to serve as a forum for new ideas, perspectives, and innovative research approaches utilizing arachnids to enhance our understanding of biodiversity in its broadest sense, including its formation and preservation. It publishes high quality, rigorously peer-reviewed, research, reviews, and commentaries where emphasis will be placed on comparative and integrative studies that place arachnids as eminent research objects to understand global patterns of diversity, historical biogeography, and conservation in the light of ongoing drastic changes in habitat and climate. One key aim is to offer a catalytic venue that synergistically unites research from historically disparate groups of scientists (i.e. acarologists and those studying the remaining arachnological orders), and also to invite articles on other extant or extinct chelicerates. Especially welcome are papers that utilize arachnids to investigate broad biological questions that should be of interest to audience far beyond their taxonomic scope. Grand challenges will include:
● Assess arachnid species richness on local and global scales for comparative biodiversity.
● The distribution of diversity, endemism, origin of species, and fundamental and comparative biogeography addressing how species became to occupy landmasses and the patterns and processes of diversification along broad time scales.
● Estimation of conservation priorities, assessment and conservation status of species, clades, and communities, and research on topics such as habitat degradation and climate change that cause major threats to biodiversity.
All of these will benefit from the rapidly developing genomic techniques where transcriptomic and phylogenomic methodology can aid in diversity estimation, biogeographic reconstruction, and conservation research, ranging from ecological to evolutionary time scales.
Research on biodiversity goes back many centuries but was in some sense formalized with the publication of the 10th edition of Systema Naturae by Linnaeus, 1758. Naturalists were, of course, aware of biodiversity before this time, but the book is the origin of formal classification and tabulation of species that we still recognize today. With one exception: In spiders, cataloging of biodiversity started a year earlier when a student of Linnaeus, Carl Alexander Clerck, published Aranei Svecici (Clerck, 1757). The book describes taxonomically 67 species of spiders found in Sweden. These 67 species are the first described species acknowledged in the currently used synthesis of global biodiversity (World Spider Catalog, 2022).
Perhaps we may attribute this privileged position of spiders to happenstance, however, arachnids continue to play a prominent role in biodiversity research today. Arachnids are impressively diverse with over 110,000 known species (Adis and Harvey, 2000; World Arachnida Catalog, 2022), which is believed to be only a fraction of actual diversity (e.g. Schatz and Behan-Pelletier, 2008; Agnarsson et al., 2013). An important aspect of spiders is that they form the largest clade of exclusively predatory organisms on earth, and the planet’s preeminent consumers of insects (e.g. Coddington and Levi, 1991; Foelix, 2011; Kuntner, 2022). Many other arachnids are also predators, while especially among the Acari, there is enormous richness of key parasites and pests of great economic interest such as ticks or herbivorous mites. The interplay between the diversity of predatory vs prey animals is a topic of broad interest (Shurin et al., 2002; Griffin et al., 2013), but poorly studied in arachnids. Further, the intimate association of parasites and their hosts are starting to reveal enormous gaps in our understanding of diversity among relatively poorly studied parasitic organisms such as Acari (Magalhães et al., 2007; Skoracka and Dabert, 2010; Skoracka et al., 2015). The global distribution of arachnids in practically every terrestrial habitat also renders them ideal to study diversity and diversification in specialized habitats such as mountaintops and caves, characterized by rapid species turnover and extraordinary beta diversity, and where knowledge gaps are particularly large (Arnedo et al., 2007; Cardoso, 2012; Esposito et al., 2015; Hendrixson et al., 2015; Harms, 2018; Mammola, 2019; Mammola et al., 2019; Agnarsson et al., 2023). Challenges and opportunities therefore abound in the study of arachnid biodiversity.
Arachnid biodiversity research, especially on spiders, has featured prominently in the development of species richness inventories. These influences led to improved study design (Coddington et al., 1991; Sorensen et al., 2002; Cardoso, 2009), where arachnologists have helped bringing about progress in empirical and analytical methodology such as estimation of species richness from inventory data (Colwell and Coddington, 1994; Cardoso et al., 2009, Coddington et al., 2009). For arachnids, the use of molecular and integrated data for species identification (Hebert et al., 2003), and for circumscription and diagnosis (Bond and Stockman, 2008; Macias-Hernandez et al., 2010; Hamilton et al., 2011; Bond et al., 2021) are notable. Indeed, through use of integrative approaches in taxonomy, the Acari are emerging as a group where cryptic diversity may be rampant and currently known species richness may be only a fraction of true biodiversity (Skoracka et al., 2015; Dantas-Torres, 2018; Young et al., 2019; Lienhard and Krisper, 2021; Pfingstl et al., 2021). Many arachnids, including spiders, have a complete up to date taxonomic database online (World Arachnida Catalog, 2022) and spiders have featured in the development of improved methods of imaging and referencing images (Ramirez et al., 2007), computer databasing and georeferencing of specimens (e.g. Goblin Spider PBI, http://research.amnh.org/oonopidae/), cyber-informatics (Penev et al., 2009; Penev et al., 2010; Miller et al., 2012), collection digitization and linking of distribution data to public databases such as the Global Biodiversity Information Facility (GBIF http://www.gbif.org/). Another recent progress is the development of less subjective criteria for species delimitation (Bond and Stockman, 2008).
Of course, no consideration of arachnid biodiversity is complete with reference only to their high number of species. Arachnids contribute massively to biodiversity through their function e.g. as major predators and parasites in ecosystems, resulting in great diversity of species interactions and ecosystems. Furthermore, arachnids are prolific producers of biomaterials most notable among which are venoms and silks (Foelix, 2011). The variety of such biomaterials (Sethy and Ahi, 2022), and their genetic underpinnings (Arakawa et al., 2022; Babb et al., 2022)—most of which remain largely unknown (e.g. we know only a handful of the likely hundreds of thousands of silk types produced by tens of thousands of species)—represent underappreciated dimensions of biodiversity. Further, the structures produced, for example, through silk use such as spider webs are both nets and habitats that contribute to habitat diversity. Thus, a great challenge remains in detailed understanding of arachnid biodiversity far beyond their species richness.
In sum, research on arachnid biodiversity is at the forefront of the field as a whole and Frontiers in Arachnid Science aims to provide a venue for studies that continue to demonstrate broad patterns and test key questions relevant to the broadest possible audience in the field. Among prominent challenges are estimates of true species richness of arachnids, the diversity of their ecological interactions, and greatly understudied diversity of biomaterial products and constructs. Filling knowledge gaps both in the largest groups as well as among small, cryptic, specialized, and poorly studied—but likely highly diverse group—may revolutionize our understanding of the biodiversity and role of arachnids in natural and anthropogenic ecosystems.
A critical question in biology is how organisms come to occupy the areas and habitats they are found in, and what factors underlie diversification, persistence, and extinction of lineages. The modern field of biogeography encompasses the distribution of organisms through time, and the history of splitting (diversification) as it relates to geography, geographical changes over millions or hundreds of millions of years, and a variety of geographical barriers that may limit gene flow among populations (e.g. Cox et al., 2023). Furthermore, biogeography aims to marry both historical and current processes, drawing from the massive toolboxes of evolutionary and ecological disciplines (Wiens and Donoghue, 2004; Rominger et al., 2016). Given the multidisciplinary nature of biogeography, and the ancient time scales that can be involved, the field faces some uniquely arduous challenges. First, historical biogeography assumes accurately dated phylogenies. While progress is currently rapid in both data gathering through NGS and methodology to integrate hundreds or thousands of loci to best infer trees, phylogenies remain hypotheses filled with ambiguities due to various issues such as rapid speciation, incomplete lineage sorting, reticulation, horizontal gene transfer, gaps in taxonomic knowledge, incomplete taxon sampling, long branch attraction, and others (Young and Gillung, 2019; Kapli et al., 2020). Layered on top are challenges with accurately dating ambiguous phylogenies with sparse and often speculative fossil evidence and inaccurate molecular clocks. These data then have to be aligned—to our best abilities—with geological history, itself fraught with difficulties, for example, due to ambiguities in existence, position, and timing of landmasses and potential barriers (for bibliography of vicariance biogeography see Agnarsson et al., 2019). Furthermore, obligate hematophagous ectoparasites within Arachnida such as ticks offer a unique challenge to the field of biogeography, as their dispersal and distribution results from the interplay of several factors including host dispersal, vegetation coverage and climate variables which in turn influence the distribution of vector-borne diseases (Estrada-Peña et al., 2012; Estrada-Peña et al., 2014). As biogeographers we like to tell stories and stories attract readership; a major challenge is to curb our narrative to candidly reflect the great ambiguity inherent in our discipline. Thus, we especially welcome work that critically assesses the weight of the evidence favoring one hypothesis over another. Megadiverse lineages, such as arachnids, are perhaps the best suited to study nuances of biogeography in part directly due to their diversity (high resolution tools, redundancies that may compensate for incomplete sampling), and (often) abundance (relative ease of intensive sampling without great impact on populations). The growing emphasis on invertebrate biogeography, where various arachnids play an important role (Boyer et al., 2007; Opatova et al., 2013; Fernández and Giribet, 2015; Chamberland et al., 2018; Nogueira et al., 2019; Pfingstl et al., 2019; Chamberland et al., 2020; Chamberland et al 2022; Cain et al., 2021; Derkarabetian et al., 2021; Santibáñez-López et al., 2021; Monjaraz-Ruedas et al., 2022), demonstrates the opportunities that lie ahead in the field.
One grand biogeographic challenge has long been how to explain occurrence of biota on widely separated landmasses, and on oceanic islands, especially among organisms that lack wings or other obvious means of transoceanic transport. Early in the discipline the field seemed to face an unpleasant choice between unlikely alternative hypotheses, preposterous landbridges lacking geological evidence and nearly unfathomable long-distance dispersal shared by different taxa differing in dispersal abilities. As an early skeptic of hypothetical landbridges created almost at will to explain global biogeography, Darwin went to great lengths testing and demonstrating the ability of different organisms to disperse over water (reviewed in Winkworth, 2010). Dispersal was a convenient and all-encompassing explanation (in principle, all distributions can be explained via ad hoc hypotheses of long-distance dispersal) and was soon favored over poorly founded land-bridge hypotheses. However, with the eventual acceptance of Wegener´s theory of plate tectonics (Wegener, 1924), dispersal hypotheses came under increased scrutiny, with a common criticism of it being a theory that can potentially explain everything, thus in the end explaining nothing. A robust field of biogeography then developed that attempted to explain all distributions with reference to geological change; phylogeny was to recapitulate geography. More recently, the obvious role of dispersal has been reappreciated (de Queiroz, 2005; Gillespie et al., 2012). In fact, modern historical biogeography spends a lot of thinking on the relative importance of geography (vicariance) versus dispersal in shaping biotas and driving diversification. There, arachnids are playing a pivotal role in this discipline. For example, theoretical foundations for testing dispersal hypotheses have been laid down by Rosemary Gillespie and colleagues (Gillespie et al., 2012), an arachnologist that has worked for decades on spiders on islands, especially Hawaiian and Polynesian spiders. Hence, among arachnids we find severely dispersal limited groups that carry the deep imprints of geological history and are excellently suited to test ancient vicariance biogeographical patterns (Boyer et al., 2007; Opatova et al., 2013; Xu et al., 2015; Cain et al., 2021; Giribet et al., 2022) and others that are more prone to movement (among them taxa that are distributed across the globe via jet streams high in the atmosphere) and have helped inspire the modern field of island biogeography (Gillespie, 2002; Hormiga et al., 2003; Gillespie, 2004; Gillespie et al., 2008; Gillespie et al., 2012; Rominger et al., 2016). Yet, a major challenge remains in accurately estimating dispersal probabilities, usually simply inferred from the organism’s biology and e.g. record of ballooning in spiders. Better estimations of dispersal propensities will improve the discipline (Kuntner and Turk, 2022). Thus, the role of arachnids in understanding local and global biogeographical patterns as they reflect the interplay between dispersal and vicariance will likely continue to grow.
Once we have discovered and described species (first section above) and have some understanding of their phylogenetic relationships and processes shaping their distribution and diversification (second section above), we can start looking at questions surrounding the value of biodiversity and the importance and means of its conservation. It is well-known that despite the vast majority of life on earth being invertebrates, little effort is afforded to conservation research and planning and implementation of conservation action for this ´silent majority´. Cardoso et al. (2011) identified seven impediments to effective conservation of invertebrates, among them most have to do with lack of knowledge on the animals and their roles, from lack of scientific knowledge such as true number of species (including lack of funding, species description, distribution etc.), and from knowledge and interest from the public and policy makers. The effort allocated to invertebrate conservation is overshadowed not the least in comparison to popular larger mammals or other ´charismatic´ species (Cardoso et al., 2011; Cardoso et al., 2017; Milano et al., 2021) who receive so much attention as to cast a ´conservation shadow´ over the majority of life on earth—a ´conservation charisma challenge´. For example, while there are over 50,000 known spider species only 148 are currently listed on the IUCN red list (Milano et al., 2021), the lack of information and conservation effort is likely even poorer for other arachnids. As outlined above many arachnids may be cryptic and occur in specialized habitats, can have very short ranges, and high endemism. Species delimitation in such groups can have ´High-stakes´ conservation implications (Hedin, 2015), for example, through identifying important habitats, where charismatic megafauna may be absent. Also, invertebrates may serve as early indicators of localized habitat loss. Jason Bond (Bond, 2012) e.g. described a species of Aptostichus spider subsequent to its extinction from its very restricted habitat patch. Ticks may be an interesting and perhaps controversial example on the ´value´ of biodiversity, as debate exists on viewing parasites as biodiversity or pest, and choosing from using the languages of conservation or control and elimination (Mihalca et al., 2011).
Therefore, great challenges lie ahead in boosting research effort, publication of data, and implementing conservation plans and actions for invertebrate, not the least arachnids. The Diversity, Conservation and Biogeography section of Frontiers in Arachnid Science thus especially invites manuscripts on arachnid conservation assessment, planning, and implementation of conservation action. We aim to be the preeminent outlet for papers tackling the ´conservation charisma challenge´, where the public and decision makers fail to see the value of the many due to the bright light shone by the few. What is the answer to this dilemma? Clearly it lies in 1) detailed understanding of the distribution of biodiversity and the large-scale protection of habitats rather than massive focus on a few species, and 2) in recognizing major threats to biodiversity, primarily among them climate change and habitat destruction, and the role arachnids can play in both detecting change and guiding conservation effort. Utilizing information from arachnids to aid in conservation efforts focused on habitats and regions is a key challenge in the next decades and we invite papers that move the field forward in this direction.
The author confirms being the sole contributor of this work and has approved it for publication.
This research is supported by NSF DEB-1050187, 1050253, 1314749 to IA and G. Binford, and a National Geographic WW-203R-17 grant to IA.
I thank Matjaz Kuntner and the FAS editorial teams for useful suggestions to improve this manuscript.
The author declares that the research was conducted in the absence of any commercial or financial relationships that could be construed as a potential conflict of interest.
All claims expressed in this article are solely those of the authors and do not necessarily represent those of their affiliated organizations, or those of the publisher, the editors and the reviewers. Any product that may be evaluated in this article, or claim that may be made by its manufacturer, is not guaranteed or endorsed by the publisher.
Adis J., Harvey M. S. (2000). How many Arachnida and Myriapoda are there world-wide and in Amazonia? Stud. Neotrop. Fauna. Environ. 35, 129–141. doi: 10.1076/0165-0521(200008)35:2;1-9;FT139
Agnarsson I., Ali J., Barrington D. (2019). Vicariance biogeography. Oxford Bibliog. Ecol. doi: 10.1093/obo/9780199830060-0225
Agnarsson I., Coddington J. A., Caicedo-Quiroga L., May-Collado L. J., Pálsson S. (2023). Deep mtDNA sequence divergences and possible species radiation of whip spiders (Arachnida, amblypygi, phrynus) among Caribbean oceanic and cave islands. (Siri Scientific Press, Manchester, UK)
Agnarsson I., Coddington J. A., Kuntner M. (2013). “Systematics – progress in the study of spider diversity and evolution,” in Spider research in the 21st century, vol. chapter 2 . Ed. Penney D., 58–111.
Arakawa K., Kono N., Malay A. D., Tateishi A., Ifuku N., Masunaga H., et al. (2022). 1000 spider silkomes: linking sequences to silk physical properties. Sci. Adv. 8, eabo6043. doi: 10.1126/sciadv.abo6043
Arnedo M. A., Oromi P., Murria C., Macias-Hernandez N., Ribera C. (2007). The dark side of an island radiation: systematics and evolution of troglobitic spiders of the genus Dysdera latreille (Araneae: Dysderidae) in the canary islands. Invertebrate. Syst. 21, 623–660. doi: 10.1071/IS07015
Babb P. L., Gregorič M., Lahens N. F., Nicholson D. N., Hayashi C. Y., Higgins L., et al. (2022). Characterization of the genome and silk-gland transcriptomes of darwin’s bark spider (Caerostris darwini). PloS One 17, e0268660. doi: 10.1371/journal.pone.0268660
Bond J. (2012). Phylogenetic treatment and taxonomic revision of the trapdoor spider genus Aptostichus Simon (Araneae, mygalomorphae, euctenizidae). ZooKeys 252, 1–209. doi: 10.3897/zookeys.252.3588
Bond J. E., Godwin R. L., Colby J. D., Newton L. G., Zahnle X. J., Agnarsson I., et al. (2021). Improving taxonomic practices and enhancing its extensibility–an example from araneology. Diversity 14 (1), 5. doi: 10.3390/d14010005
Bond J. E., Stockman A. K. (2008). An integrative method for delimiting cohesion species: Finding the population-species interface in a group of Californian trapdoor spiders with extreme genetic divergence and geographic structuring. Syst. Biol. 57, 628–646. doi: 10.1080/10635150802302443
Boyer S. L., Clouse R. M., Benavides L. R., Sharma P., Schwendinger P. J., Karunarathna I., et al. (2007). Biogeography of the world: A case study from cyphophthalmid opiliones, a globally distributed group of arachnids. J. Biogeogr. 34, 2070–2085. doi: 10.1111/j.1365-2699.2007.01755.x
Cain S., Loria S. F., Ben-Shlomo R., Prendini L., Gefen E. (2021). Dated phylogeny and ancestral range estimation of sand scorpions (Buthidae: Buthacus) reveal early Miocene divergence across land bridges connecting Africa and Asia. Mol. Phylogenet. Evol. 164, 107212. doi: 10.1016/j.ympev.2021.107212
Cardoso P. (2009). Standardization and optimization of arthropod inventories – the case of Iberian spiders. Biodivers. Conserv. 18, 3949–3962. doi: 10.1007/s10531-009-9690-7
Cardoso P. (2012). Diversity and community assembly patterns of epigean vs. troglobiont spiders in the Iberian peninsula. Int. J. Speleol. 41, 83−94. doi: 10.5038/1827-806X.41.1.9
Cardoso P., Borges P. A. V., Triantis K. A., Ferrandez M. A., Martin J. L. (2011). Adapting the IUCN red list criteria for invertebrates. Biol. Conserv. 144, 2432–2440. doi: 10.1016/j.biocon.2011.06.020
Cardoso P., Borges P. A. V., Veech J. A. (2009). Testing the performance of beta diversity measures based on incidence data: the robustness to undersampling. Diversity Distributions. 15, 1081–1090. doi: 10.1111/j.1472-4642.2009.00607.x
Cardoso P., Crespo L. C., Silva I., Borges P. A. V., Boieiro M. (2017). Species conservation profiles of endemic spiders (Araneae) from Madeira and selvagens archipelagos, Portugal. Biodivers. Data J. 5, 20810. doi: 10.3897/BDJ.5.e20810
Chamberland L., Agnarsson I., Quayle I. L., Ruddy T., Starrett J., Bond J. E. (2022). Biogeography and eye size evolution of the ogre-faced spiders. Sci. Rep. 12, 17769. doi: 10.1038/s41598-022-22157-5
Chamberland L., Binford G. J., Agnarsson I. (2020). Phylogeography of the widesread Caribbean spiny orb weaver Gasteracantha cancriformis. PeerJ 8, e8976. doi: 10.7717/peerj.8976
Chamberland L., McHugh A., Kechejian S., Binford G. J., Bond J., Coddington J. A., et al. (2018). From gondwana to GAARlandia: global biogeography of ogre-faced spiders (Deinopidae) mirrors geologic history. J. Biogeogr. 45, 2442–2457. doi: 10.1111/jbi.13431
Clerck C. (1757). Svenska Spindlar/Aranei svecici (Stockholm: Laurentius Salvius), [1–8], 1–154, pl. 1–6.
Coddington J. A., Griswold C. E., Silva D., Peñaranda E., Larcher S. (1991). Designing and testing sampling protocols to estimate biodiversity in tropical ecosystems, In The unity of evolutionary biology: Proceedings of the fourth international congress of systematic and evolutionary biology. Ed. Dudley E. C. (Portland, Oregon: Dioscorides Press), 44–60. doi: 10.1111/j.1365-2656.2009.01525.x
Coddington J. A., Levi H. W. (1991). Systematics and evolution of spiders (Araneae). Annu. Rev. Ecol. Syst. 22, 565–592. doi: 10.1146/annurev.es.22.110191.003025
Coddington J. A., Agnarsson I., Miller J., Kuntner M., Hormiga G. (2009). Undersampling bias: the null hypothesis for singleton species in tropical arthropod biodiversity surveys. J. Anim. Ecol. 78, 573–584
Colwell R. K., Coddington J. A. (1994). Estimating terrestrial biodiversity through extrapolation. Philos. Trans. R. Soc. London. Ser. B-Biol. Sci. 345, 101–118. doi: 10.1098/rstb.1994.0091
Cox C. B., Moore P. D., Ladle R. (2023). Biogeography an ecological and evolutionary approach (Wiley Blackwell, Hoboken, New Jersey).
Dantas-Torres F. (2018). Species concepts: What about ticks? Trends Parasitol. 34, 1017–1026. doi: 10.1016/j.pt.2018.09.009
de Queiroz A. (2005). The resurrection of oceanic dispersal in historical biogeography. Trends Ecol. Evol. 20, 68–73. doi: 10.1016/j.tree.2004.11.006
Derkarabetian S., Baker C. M., Giribet G. (2021). Complex patterns of gondwanan biogeography revealed in a dispersal-limited arachnid. J. Biogeogr. 48, 1336–1352. doi: 10.1111/jbi.14080
Esposito L. A., Bloom T., Caicedo L., Alicea-Serrano A., Sánchez-Ruíz J., May-Collado L. J., et al. (2015). Islands within islands: diversification of tailless whip spiders (Amblypygi, phrynus) in Caribbean caves. Mol. Phylogenet. Evol. 93, 107–117. doi: 10.1016/j.ympev.2015.07.005
Estrada-Peña A., Ayllón N., de la Fuente J. (2012). Impact of climate trends on tick-borne pathogen transmission. Front. Physiol. 27, 64. doi: 10.3389/fphys.2012.00064
Estrada-Peña A., Ostfeld R. S., Townsend Peterson A., Poulin R., de la Fuente J. (2014). Effects of environmental change on zoonotic disease risk: an ecological primer. Trends Parasitol. 30, 205–214. doi: 10.1016/j.pt.2014.02.003
Fernández R., Giribet G. (2015). Unnoticed in the tropics: Phylogenomic resolution of the poorly known arachnid order ricinulei (Arachnida). R. Soc. Open Sci. 2. doi: 10.1098/rsos.150065
Gillespie R. G. (2002). Biogeography of spiders on remote oceanic islands of the pacific: archipelagoes as stepping stones? J. Biogeogr. 29, 655–662. doi: 10.1046/j.1365-2699.2002.00714.x
Gillespie R. (2004). Community assembly through adaptive radiation in Hawaiian spiders. Science 303, 356–359. doi: 10.1126/science.1091875
Gillespie R. G., Baldwin B. G., Waters J. M., Fraser C. I., Nikula R., Roderick G. K. (2012). Long-distance dispersal: a framework for hypothesis testing. Trends Ecol. Evol. 27, 47–56. doi: 10.1016/j.tree.2011.08.009
Gillespie R. G., Claridge E. M., Roderick G. K. (2008). Biodiversity dynamics in isolated island communities: Interaction between natural and human-mediated processes. Mol. Ecol. 1717 (1), 45–5745–57.
Giribet G., Shaw M., Lord A., Derkarabetian S. (2022). Closing a biogeographic gap: A new pettalid genus from south Australia (Arachnida: Opiliones: Cyphophthalmi: Pettalidae) with a UCE-based phylogeny of cyphophthalmi. Invertebrate. Syst. 36, 1002–2016. doi: 10.1071/IS22043
Griffin J. N., Byrnes J. E. K., Cardinale B. J. (2013). Effects of predator richness on prey suppression: a meta-analysis. Ecology 94, 2180–2187. doi: 10.1890/13-0179.1
Hamilton C. A., Formanowicz D. R., Bond J. E. (2011). Species delimitation and phylogeography of Aphonopelma hentzi (Araneae, mygalomorphae, theraphosidae): cryptic diversity in north American tarantulas. PloS One 6. doi: 10.1371/journal.pone.0026207
Harms D. (2018). The origins of diversity in ancient landscapes: Deep phylogeographic structuring in a pseudoscorpion (Pseudotyrannochthoniidae: Pseudotyrannochthonius) reflects plio-pleistocene climate fluctuations. Zoologischer. Anzeiger. 273, 112–123. doi: 10.1016/j.jcz.2018.01.001
Hebert P. D. N., Cywinska A., Ball S. L., DeWaard J. R. (2003). Biological identifications through DNA barcodes. Proc. R. Soc. London. Ser. B-Biol. Sci. 270, 313–321. doi: 10.1098/rspb.2002.2218
Hedin M. (2015). High-stakes species delimitation in eyeless cave spiders (Cicurina, dictynidae, araneae) from central Texas. Mol. Ecol. 24, 346–261.
Hendrixson B. E., Guice A. V., Bond J. E. (2015). Integrative species delimitation and conservation of arantulas (Araneae, mygalomorphae, theraphosidae) from a north American biodiversity hotspot. Insect Conserv. Diversity 8, 120–131. doi: 10.1111/icad.12089
Hormiga G., Arnedo M., Gillespie R. G. (2003). Speciation on a conveyor belt: Sequential colonization of the Hawaiian islands by Orsonwelles spiders (Araneae, linyphiidae). Syst. Biol. 52, 70–88. doi: 10.1080/10635150390132786
Kapli P., Yang Z., Telford M. J. (2020). Phylogenetic tree building in the genomic age. Nat. Rev. Genet. 21, 428–444. doi: 10.1038/s41576-020-0233-0
Kuntner M. (2022). The seven grand challenges in arachnid science. Front. Arachnid. Sci. 1, 1082700. doi: 10.3389/frchs.2022.1082700
Kuntner M., Turk E. (2022). Towards better-informed dispersal probabilities in historical biogeography: Arachnids as a model lineage. Front. Arachnid. Sci. 1, 1058676. doi: 10.3389/frchs.2022.1058676
Lienhard A., Krisper G. (2021). Hidden biodiversity in microarthropods (Acari, oribatida, eremaeoidea, caleremaeus). Sci. Rep. 11, 23123. doi: 10.1038/s41598-021-02602-7
Linnaeus C. (1758). Systema naturae per regna tria naturae:secundum classes, ordines, genera, species, cum characteribus, differentiis, synonymis, locis (in Latin). 10th ed (Stockholm: Laurentius Salvius).
Macias-Hernandez N., Oromi P., Arnedo M. A. (2010). Integrative taxonomy uncovers hidden species diversity in woodlouse hunter spiders (Araneae, dysderidae) endemic to the macaronesian archipelagos. Syst. Biodivers. 8, 531–553. doi: 10.1080/14772000.2010.535865
Magalhães S., Forbes M. R., Skoracka A., Osakabe M., Chevillon C., McCoy C. D. (2007). Host race formation in the acari. Experiments. Appl. Acarol. 42, 225–238. doi: 10.1007/s10493-007-9091-0
Mammola S. (2019). Finding answers in the dark: caves as models in ecology fifty years after poulson and white. Ecography 42, 1331–1351. doi: 10.1111/ecog.03905
Mammola S., Cardoso P., Angyal D., Balázs G., Blick T., Brustel H., et al. (2019). Continental data on cave-dwelling spider communities across Europe (Arachnida: Araneae). Biodivers. Data J. 7, e38492. doi: 10.3897/BDJ.7.e38492
Mihalca A. D., Gherman M. C., Cozma V. (2011). Coendangered hard-ticks: threatened or threatening? Rev. Parasit. Vectors 4, 71. doi: 10.1186/1756-3305-4-71
Milano F., Blick T., Cardoso P., Chatzaki M., Fukushima C. S., Gajdos P., et al. (2021). Spider conservation in Europe: a review. Biol. Conserv. 256, 109020. doi: 10.1016/j.biocon.2021.109020
Miller J., Dikow T., Agosti D., Sautter G., Catapano T., Penev L., et al. (2012). From taxonomic literature to cybertaxonomic content. BMC Biol. 10, 87. doi: 10.1186/1741-7007-10-87
Monjaraz-Ruedas R., Francke O. F., Prendini L. (2022). World travelers: parthenogenesis and ecological tolerance enable multiple colonization events by the widespread short-tailed whipscorpion, stenochrus portoricensis (Schizomida: Hubbardiidae). Ins. Syst. Div. Vol. 6, 7.
Nogueira A. A., Bragagnolo C., DaSilva M. B., Martins T. K., Lorenzo E. P., Perbiche-Neves G., et al. (2019). Historical signatures in the alpha and beta diversity patterns of Atlantic forest harvestman communities (Arachnida: Opiliones). Can. J. Zool. 97, 631–643. doi: 10.1139/cjz-2018-0032
Opatova V., Bond J. E., Arnedo M. A. (2013). Ancient origins of the Mediterranean trap-door spiders of the family ctenizidae (Araneae, mygalomorphae). Mol. Phylogenet. Evol. 69, 1135–1145. doi: 10.1016/j.ympev.2013.08.002
Penev L., Agosti D., Georgiev T., Catapano T., Miller J., Blagoderov V., et al. (2010). Semantic tagging of and semantic enhancements to systematics papers: ZooKeys working examples. ZooKeys 50, 1–16. doi: 10.3897/zookeys.50.538
Penev L., Erwin T., Miller J., Chavan V., Moritz T., Griswold C. (2009). Publication and dissemination of datasets in taxonomy: ZooKeys working example. ZooKeys 11, 1–8. doi: 10.3897/zookeys.11.210
Pfingstl T., Lienhard A., Baumann J., Koblmuller S. (2021). A taxonomist’s nightmare-cryptic diversity in Caribbean intertidal arthropods (Arachnida, acari, oribatida). Mol. Phylogenet. Evol. 163, 107240. doi: 10.1016/j.ympev.2021.107240
Pfingstl T., Lienhard A., Petcharad B. (2019). Systematics, genetics, and biogeography of intertidal mites (Acari, oribatida) from the Andaman Sea and strait of malacca. J. Zool. Syst. Evol. Res. 57, 91–112. doi: 10.1111/jzs.12244
Ramirez M. J., Coddington J. A., Maddison W. P., Midford P. E., Prendini L., Miller J., et al. (2007). Linking of digital images to phylogenetic data matrices using a morphological ontology. Syst. Biol. 56, 283–294. doi: 10.1080/10635150701313848
Rominger A. J., Goodman K. R., Lim J. Y., Armstrong E. E., Becking L. E., Bennett G. M., et al. (2016). Community assembly on isolated islands: macroecology meets evolution. Global Ecol. Biogeogr. 25, 769–780. doi: 10.1111/geb.12341
Santibáñez-López C. E., Cushing P. E., Powell A. M., Graham M. R. (2021). Diversification and post-glacial range expansion of giant north American camel spiders in genus Eremocosta (Solifugae: Eremobatidae). Sci. Rep. 11, 22093. doi: 10.1038/s41598-021-01555-1
Schatz H., Behan-Pelletier V. H. (2008). Global diversity of oribatids (Oribatida: Acari: Arachnida). Hydrobiologia 595, 323–328. doi: 10.1007/s10750-007-9027-z
Sethy T. R., Ahi J. (2022). Spider silk and the silk of egg sacs with its astonishing concealed attributes: A review. J. Natural Fibers. 00, 1–15. doi: 10.1080/15440478.2022.2025986
Shurin J. B., Borer E. T., Seabloom E. W., Anderson K., Blanchette C. A., Broitman B., et al (2002). A cross-ecosystem comparison of the strength of trophic cascades. Ecol. Lett. 5, 785–791. doi: 10.1046/j.1461-0248.2002.00381.x
Skoracka A., Dabert M. (2010). The cereal rust mite Abacarus hystrix (Acari: Eriophyoidea) is a complex of species: evidence from mitochondrial and nuclear DNA sequences. Bull. Entomol. Res. 100, 263–272. doi: 10.1017/S0007485309990216
Skoracka A., Magalhães S., Rector B. G., Kaczynski L. (2015). Cryptic speciation in the acari: A function of species lifestyles or our ability to separate species? Exp. Appl. Acarol. 67, 165–182. doi: 10.1007/s10493-015-9954-8
Sorensen L. L., Coddington J. A., Scharff N. (2002). Inventorying and estimating subcanopy spider diversity using semiquantitative sampling methods in an afromontane forest. Environ. Entomol. 31, 319–330. doi: 10.1603/0046-225X-31.2.319
Wegener A. (1924). The origin of continents and oceans 1924. translated by JGA skerl. 4th edition (Dover Publications, Inc. New York, London: Methuen).
Wiens J. J., Donoghue M. J. (2004). Historical biogeography, ecology, and species richness. Trends Ecol. Evol. 19, 639–644. doi: 10.1016/j.tree.2004.09.011
World Arachnida Catalog (2022) Natural history museum Bern. Available at: https://wac.nmbe.ch/ (Accessed 12 November 2022).
World Spider Catalog (2022) World spider catalog. version 23.5. natural history museum Bern. Available at: http://wsc.nmbe.ch (Accessed 12 November 2022).
Xu X., Liu F., Cheng R. C., Chen J., Xu X., Zhang Z., et al. (2015). Extant primitively segmented spiders have recently diversified from an ancient lineage. Proc. R. Soc B.: Biol. Sci. 282, 20142486. doi: 10.1098/rspb.2014.2486
Young A. D., Gillung J. P. (2019). Phylogenomics – principles, opportunities and pitfalls of big-data phylogenetics. Syst. Entomol. 45, 225–247. doi: 10.1111/syen.12406
Keywords: arachnida, diversity, phylogenetics, biogeography, conservation, phylogeography, phylogenomics, taxonomy
Citation: Agnarsson I (2023) Grand challenges in research on arachnid diversity, conservation, and biogeography. Front. Arachn. Sci. 2:1101141. doi: 10.3389/frchs.2023.1101141
Received: 17 November 2022; Accepted: 06 January 2023;
Published: 07 February 2023.
Edited and Reviewed by:
Matjaz Kuntner, National Institute of Biology (NIB), SloveniaCopyright © 2023 Agnarsson. This is an open-access article distributed under the terms of the Creative Commons Attribution License (CC BY). The use, distribution or reproduction in other forums is permitted, provided the original author(s) and the copyright owner(s) are credited and that the original publication in this journal is cited, in accordance with accepted academic practice. No use, distribution or reproduction is permitted which does not comply with these terms.
*Correspondence: Ingi Agnarsson, aWFnbmFyc3NvbkBnbWFpbC5jb20=
Disclaimer: All claims expressed in this article are solely those of the authors and do not necessarily represent those of their affiliated organizations, or those of the publisher, the editors and the reviewers. Any product that may be evaluated in this article or claim that may be made by its manufacturer is not guaranteed or endorsed by the publisher.
Research integrity at Frontiers
Learn more about the work of our research integrity team to safeguard the quality of each article we publish.