- 1DST Sponsored Fish Nutrition Research Laboratory, Department of Zoology, University of Kashmir, Srinagar, India
- 2Department of Nursing, Jazan University, Jazan, Saudi Arabia
Exhaustive work on bacterial fish diseases provides a significant knowledge about the influence of bacterial infections in economically noteworthy freshwater fish species farmed around the world. However, it is still difficult to exactly pinpoint the causes of bacterial infections among wide diversity of fish species, although significant strides in this direction have been achieved with respect to different fish species. Various studies have demonstrated the growing prevalence of bacterial infections, concurrent with the rapid development of aquaculture worldwide, yet information regarding these infections, their influence on the host species, consequences of numerous environmental variables as well as social-economic impacts are still lacking, which warrant for a desperate need of detailed studies. Despite various efforts, the pathogenesis, appropriate diagnostic techniques and treatment measures of various diseases remains unclear and thus responsible for huge losses both in natural as well as aquaculture conditions. In addition, progress in control and prevention of these diseases is hampered by the lack of adequate epidemiological data on bacterial diseases in many countries. In the present review, an attempt has been made to bring together the scattered information available about the diverse features of most economically significant bacterial diseases affecting fresh water salmonids worldwide including: bacterial gill disease, columnaris disease, bacterial kidney disease, enteric red mouth disease, lactococcosis, furunculosis, bacterial coldwater disease, mycobacteriosis and photobacteriosis. Besides, we also tried to highlight a brief overview of the traditional techniques for isolating bacteria from their hosts along with the serological and/or genetic diagnostic tools. The development of immunisation techniques to stop certain bacterial infections is also discussed in this review paper. The study focuses on the latest fundamental knowledge linked to bacterial infections in freshwater systems covering epidemiology, clinical signs/pathogenesis, transmission, diagnosis and treatment measures while the pathogenesis data emphasizes the area calling for further investigation.
1 Introduction
Food and nutritional security are one of the major threats both in developing and undeveloped countries due to the exponential rise in world population. Aquaculture has the potential to feed the world's rising population. As an emerging industrial sector, aquaculture needs substantial advances in science and technology. The 2024 edition of the State of World Fisheries and Aquaculture reports that worldwide fisheries and aquaculture production reached 223.2 million tonnes in 2022, a 4.4% increase over 2020. 37.8 million tonnes of algae and 185.4 million tonnes of aquatic creatures were produced (FAO, 2024). It is apparent that fresh water fish dominate the worldwide aquaculture production sector, as their share of global fish production was 83.6%, contributing 44.6 million metric tons in 2017 putting them well ahead of the other sectors (FAO, 2018). While, contribution of fish produced is about 54.1 million tonnes of finfish (US$ 138.5 billion). The recent studies indicated that aquaculture could provide an immediate answer to tackle the problem of increasing nutritional demands of worldwide population. It has also the capability to provide steady work and wages for many people associated with this sector all around the world. However, unwarranted environmental changes and emerging diseases are known to negatively impact this industry worldwide. Due to its elevated protein content, less saturated fat, and abundance of omega fatty acids, fish fetch the attraction of almost all the sectors associated with food industry especially essential in the aquatic food production business during the past few decades (FAO, 2006). Improving production efficiency is a continual priority for the world's water resources since it allows for a beneficial improvement in profit. Aquatic meals are becoming increasingly popular around the world, but this trend is most pronounced in Asia, where 90% of the world's aquaculture is located (FAO, 2020).
In aquaculture, a significant loss of output has been reported in terms of food insecurity, job loss, revenue reduction, which negatively affected the livelihoods of farmers owing to a variety of reasons, among them disease holds a vital place. Like other organisms, fish is also susceptible to number of diseases. Various studies have demonstrated that diseases in aquaculture mostly found in underdeveloped countries contribute to about 50% production loss. It has been reported that an estimated $ 6.5 billion of revenue is lost every year due to the outbreak of diseases. For instance, reduced production rates and decrease exports due to acute hepatopancreatic necrosis diseases (AHPND) has resulted in losses of USD 12 billion in Thailand over the period of 2010–2017 and more than USD 26 million in Viet Nam in 2015 (Shinn et al., 2018). The extensive loss in aquaculture due to the outbreak of diseases have largely been on rise in major fish producing countries like China, the largest producer of world aquaculture products (BOF et al., 2019) where an estimated loss of about 15% of the total fish production due to disease has been reported (Assefa and Abunna, 2018). Disease loses in China amounted to over USD 6.5 billion in 2018 which was USD 1.2 billion over 2017 and USD 2.4 billon over 2016 losses (Bondad-Reantaso et al., 2021). Chile, one of the important fish producing country also reported that cost of infective salmon anaemia alone is $2 billion, and it has led to the dismissal of about 20000 workers (Leung and Bates, 2013; Assefa and Abunna, 2018).
Live production always carries the danger of infection-related losses with farmed fish having increased susceptibility than wild fish to diseases by a variety of bacterial, viral, parasitic, and fungal pathogens (Barber, 2007). The trend towards more intensive production systems, disturbance in ecological balance caused by pollution and changes in climate, and the anticipated rise in global aquaculture trade has affected interactions between fish hosts, disease agents and humans. This affects the rates of pathogen replication and proliferation, which expands the geographic and host range of pathogenic agents and also raises the number of species that are affected by disease outbreaks (Brugere et al., 2017; Chintagari et al., 2018). The outbreaks of diseases have a considerable influence on the quantity, quality, and safety of fish produced globally (Hill, 2005; Adams and Thompson, 2006; Iwama, 2007; Shinn et al., 2015) and can prevent producers from accessing markets, which causes significant constraint on the aquaculture business (Trienekens and Zuurbier, 2008; Aung and Chang, 2014).
The increased severity of bacterial diseases has a tremendous impact on aquaculture production globally to a large extent. The current trend of high intensity practices lack recognition of delicate balance between host, pathogen and the environment, which greatly contributes to diseases. This includes the expansion of intensive and semi-intensive aquaculture practices that have enhanced production (Bondad-Reantaso et al., 2005). Expanding intensive and semi-intensive aquaculture practices have contributed to enhancing the production through aquaculture. The culture practices including high stocking densities, cause stress conditions that in turn makes them more susceptible to economic losses due to disease outbreaks on their farms (Das and Mishra, 2014). Lack of fundamental knowledge about the suitable fish culture techniques especially among the rural fish farmers makes them the worst sufferers in terms of economic loss occurring due to different disease outbreaks in fish farms (Bagum et al., 2013). For the development of diseases in aquaculture systems, host, pathogen and the environment must interact in sufficiently complex manner (Chong et al., 2023). These days whole aquaculture systems face a unique challenge when it comes to disease management, as pathogens always look for new opportunities where they gain entry into the host during compromised conditions (Vijayan and Sanil, 2012).
One of the challenging problems to deal within the aquaculture disease outbreaks is the occurrence of bacterial fish diseases, as bacteria are abundantly found in all aquatic ecosystems. Generally nutritional or the physiological imbalance among the fishes paves the way for these bacteria to become pathogenic in addition to other stressors such as overstocking, deteriorating water quality that facilities growth, infectivity and pathogenicity of these bacterial pathogens (Sandeep et al., 2016). Fish especially the juvenile or larval stages are particularly susceptible to bacterial diseases and suffer high mortalities causing severe economic losses.
Besides affecting fisheries sector to a large extent, fish diseases are known to pose significant health challenges to human beings. There has been a trend of using certain substances and antibiotics for effective treatment of fish diseases but these substances potentially threaten the environment along with humans directly and indirectly through the food safety route (Sapkota et al., 2008). Sizeable portion of population will come into contact to certain diseases after eating farmed fish which resulted in spread of certain diseases to human populations, referring to fish related zoonoses (Haenen et al., 2013). There have been claims that many bacterial infections found in water are indeed zoonotic, however proof beyond isolated cases is generally inadequate. Many studies indicated that bacterial zoonosis from aquaculture has been patients which were already immunocompromised due to another disease (Weir et al., 2012). As was previously stated, the risk spread of bacterial diseases from aquaculture is modest because few aquatic diseases can induce true zoonosis due to their low thermal activity. Presently only few diseases such as: Mycobacterium species, Streptococcus iniae, Clostridium botulinum, and Vibrio vulnificus are considered proven aquatic zoonotic agents (Gauthier, 2015). The risk of acquiring an aquatic zoonotic illness is greater than previously thought but this is more likely due to topical exposure (Haenen et al., 2013). Using genomics specifically transcriptomics to validate the pathogenicity and transmission route, aquatic bacterial zoonosis is one of the active topics of research. Confirming actual zoonotic status of a particular pathogen requires combining results of the identification tests with active epidemiological surveillance and risk analysis (Gauthier, 2015). Globalization, rising aquaculture output, microbial adaptability and climate change all had an impact on the international trade, making it harder to estimate the disease situation in aquaculture (Subasinghe et al., 2004; Bondad-Reantaso et al., 2018; Woo et al., 2020). The economic and social progress of many countries has been obstructed due to the prevalence of diseases and its impact on aquaculture (FAO/NACA, 2000; Bondad-Reantaso et al., 2005). Besides these, co-infections involving multiple pathogens, are generally underreported, which have been linked to higher fatality rates that resulted in higher economic loss (Wise et al., 2021). However, there is insufficient reliable data on the incidence and mechanisms of such bacterial coinfections. Therefore, it is vital to understand disease aetiology and the available treatment choices in order to make an accurate diagnosis and study of co-infective factors that affect bacterial infections. In order to combat the disease issue in aquaculture, many preventive and therapeutic approaches have been utilised over time. Non judicious use of antibiotics to manage fish diseases in the early days of aquaculture has led to the development of antibiotic resistance (Schar et al., 2021). Now efforts are being made to try innovative antimicrobial medication development in order to overcome the failure of current management strategies.
A detailed account of the frequently encountered bacterial fish diseases has been discussed in Table 1.
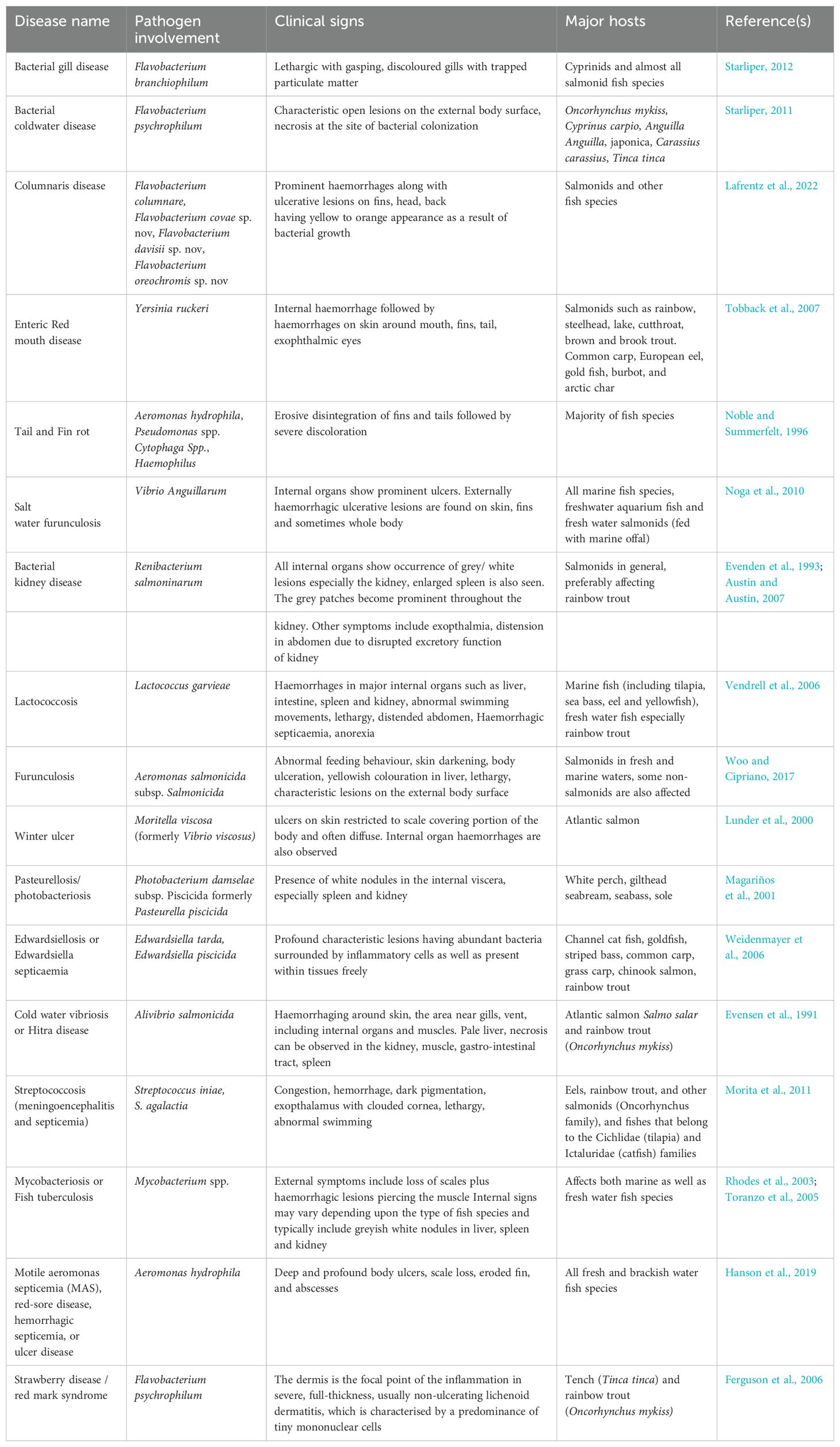
Table 1. Commonly encountered bacterial fish diseases, causative agents and clinical signs found in fresh water fish fauna.
2 Bacterial diseases commonly encountered in freshwater salmonids
2.1 Bacterial gill disease
2.1.1 Epidemiology
A common bacterial disease known as gill disease mainly affects young hatchery-raised and farmed salmonids to a significant extent. Davis was the first to describe the BGD (Davis, 1926). The etiological agent was named Flavobacterium branchiophilum (Kimura et al., 1978). The causative organism F. branchiophilum was previously called as Flavobacterium branchiophila (Von Graevenitz, 1990). F. branchiophilum was isolated, described by Wakabayashi et al (1989). However, F. branchiophilum was suggested as the correct name by Von Graevenitz (1990). Later on, it was experimentally produced in fingerlings, causing disease (Wakabayashi et al., 1989). When trout were raised under controlled settings, it was observed that a spontaneous outbreak of BGD occurs which is thought to be prevalent in fish that live in low-temperature, posing a serious hazard to young salmonids raised in hatcheries (Bullock, 1972; Daoust and Ferguson, 1983; Schachte and Carey, 1983; Farkas, 1985). Although this pathogen has not caused any serious threats in natural habitats. In general, BGD is a pathological disorder whose progression depends on a number of interdependent bacteria, the prevalence of which rises proportionately with the quantity and quality of different metabolic waste products. The presence or absence of these bacteria depends heavily on the ecological conditions. BGD causes a significant number of fatalities and is characterised by the abundance of filamentous bacteria on the gill surface of affected organism (Snieszko, 1981). The fish's gills are crucial to several physiological activities, including respiration, excretion, and the filtration of food particles. Although the patho-physiological impacts of BGD have not yet been investigated, they are affected in this disease because they depend on the healthy functioning of the gill epithelial tissue, which is severely affected during the course of this infection. The occurrence of this disease results in around 25% mortality (Speare et al., 1991; Zamparo et al., 2024). Environmental variables including depleted oxygen levels, elevated turbidity, high ammonia levels and overcrowding among others, not only increase disease prevalence, but also make the disease worse (Leung and Bates, 2013). The patterns of pathogenicity are unaffected by temperature changes. Salmonids and cyprinids experience BGD outbreaks at temperatures of almost 20 °C. Water is recognised to be a source of BGD transmission (Starliper, 2012).
2.1.2 Clinical signs/pathogenesis
The gills comprise the major organ affected by BGD. Observable indications of BGD in fish include lethargic movements, irregular feeding behaviour due to loss of appetite, movement near the water’s surface with swollen operculum, mostly seen gasping at the water's surface (Warren, 1991). The white coloured gill tips and an increase in mucus secretion are considered as characteristic signs of this disease. While lesions on the surface of the body are not noticeable at the onset of the outbreak of disease (Starliper, 2012). The distortion of the gill tips, dyspnea, coughing, flaring of opercula, and respiratory impairment are further clinical signs helpful in identifying the disease (Figure 1). Primary lamellae are prominently enlarged during the early stages of the disease, which includes hyperaemic gills. Increased mucus secretion causes particles to become trapped in the gill filaments which leads to impairment of respiratory functions. In Indian major carp, catla and rohu the occurrence of BGD has most prominently been recognised in the appearance of dark body colour associated with gill necrosis and eye congestion (Swain et al., 2007). Proliferative bronchitis is an epithelial disease reported in fishes, the pathogen first colonises the terminals of the secondary lamellae before moving inside and causing tissue growth by excessive cell division (hyperplasia). Due to the secondary lamellae merging at the distal ends, the bacteria forms a sac-like structure with deformed epithelial cells and a lot of surrounding mucus appears. Under extreme circumstances, hyperplasia may result in the destruction of the interlamellar space and the fusion of adjacent primary lamellae (Noga, 2010). Diagnosticians for fish health do not typically try to grow F. branchiophilum as a primary bacterial culture. When the typically high mortality rates necessitate an immediate response, the bacterium's slow growth makes it difficult to identify the pathogen using conventional biochemical characterizations. This chance for prompt action is also made possible if it is correctly diagnosed using microscopy and clinical signs (Starliper, 2012).
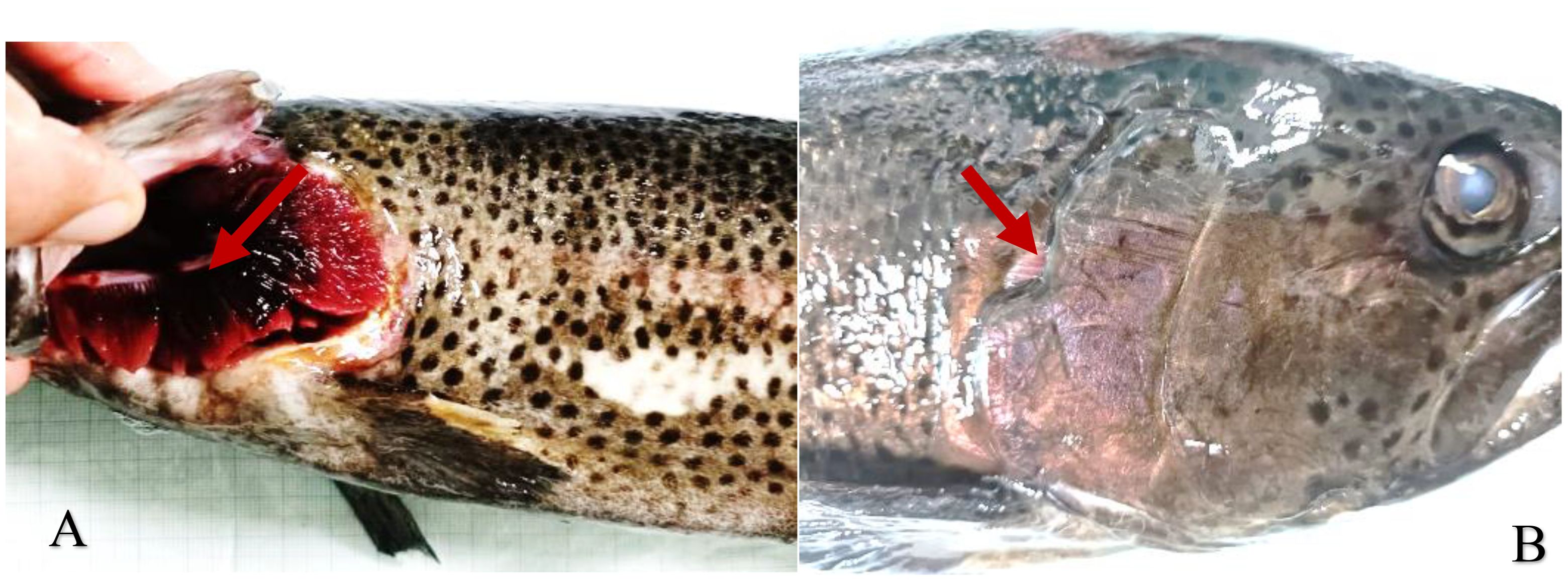
Figure 1. Rainbow trout with bacterial gill disease infection, showing characteristic bleeding of gills (A) and flared opercula (B).
2.1.3 Diagnosis
Wet mounts or histopathological studies can be used to quickly make a presumptive identification of the F. branchiophilum, similar technique used for the identification process of Flavobacterium columnare, when the lesion has long, thin rods on the surface. Loss of bacteria during tissue processing can make it a challenging task for histopathologists to detect early lesions. If the examination calls for it, the pathogen can be cultured. It can be challenging to isolate bacteria without clinical signs (Good et al., 2014). A description of the diagnostic parameters of the BGD is given in Table 2.
2.1.4 Treatment
Antiseptic baths, diquat bath, chloramine-T bath, quaternary ammonium bath, are considered as successful tools in treating BGD while providing enough oxygen (Noga, 2010). In order to control the condition or treat it in the first place, overcrowded culture conditions should be avoided. Besides, maintaining the optimal water quality, removal of severely diseased fish could be useful preventive measure for the prevention of the spread of disease to the healthy individuals from the culture points (Ostevik et al., 2022).
2.2 Bacterial coldwater disease or rainbow trout fry syndrome
2.2.1 Epidemiology
This disease is also called as peduncle disease. Salmonid hatcheries including rainbow trout face a serious problem with bacterial cold water disease. It is probably an endemic strain of pathogen found in salmonid culture. The disease is caused by Flavobacterium psychrophilum earlier known as Cytophaga psychrophila and Flexibacter psychreophilus (Noga, 2010). The pathogen has been isolated and recovered from variety of cultured salmonid fish species along with non-salmonid fish hosts with a wide geographic range. Many coldwater fish species are affected by cold water disease and high mortality rate cause significant loss in trout and other salmon populations. A bacterial cold water fish disease epidemic develops at water temperatures of around 16 °C and at 10 °C and lower, a significant prevalence and damage has been observed (Lee et al., 2010). As a result, the sickness is known to be more prevalent throughout the spring. Almost all age groups of fish are susceptible to this disease; however, fry and fingerling are known to sustain the most damage as a result of BCW disease. It has been observed that when temperature is above 15 °C a declining trend in mortalities has been observed. After infection, mortality typically takes 5–10 days to reach its peak. The average fatality rate is between 5 and 10% but in some epidemics, that number can rise up to 90% (Starliper, 2011). The disease may recur due to some environmental stressors such as handling, turbid waters, toxicity, etc.
2.2.2 Clinical signs/pathogenesis
The disease is characterized by large number of profound open lesions on the epidermal surface of the fish. At the onset of disease, the lesions are characterized as rough skin patches or fin tip fraying (Figure 2). However, at later stages of infection, skin necrosis is observed at the site of bacterial colonization, characterized by pathological changes in dorsal and adipose fin (LaFrentz et al, 2004). Along with epithelial erosions and necrosis of external tissue, distended abdomen, severe anaemia, and bilateral exophthalmia are the clinical symptoms of the affected fish. Although, in severe cases internal haemorrhages are also being observed. The pathogen is usually present in abundant numbers in secondary lamellar capillaries, kidney, heart, and spleen (Knupp, 2023). Moderate type of inflammation is observed in clinical cases or inflammation is absent in many cases.
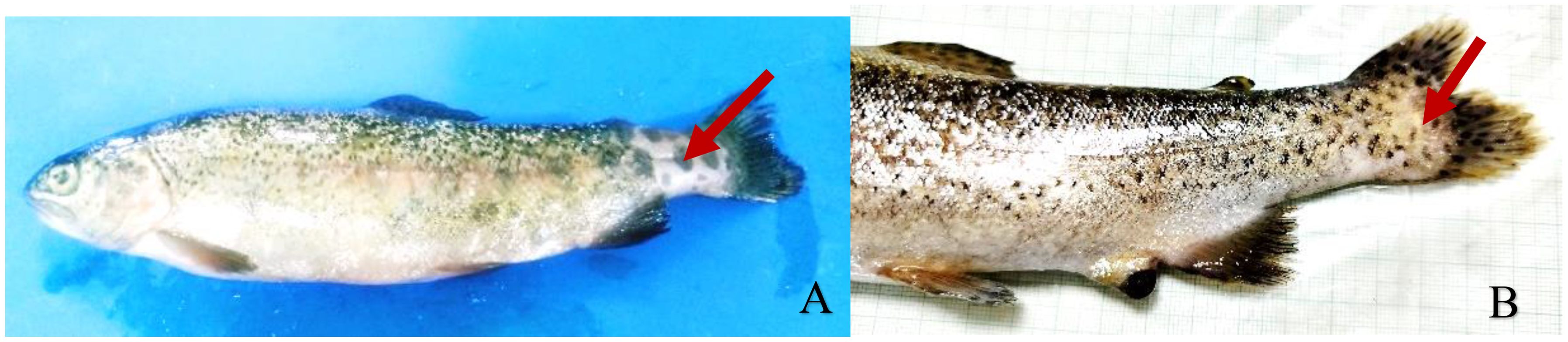
Figure 2. Rainbow trout with rainbow trout fry syndrome, showing epithelial erosion of caudal peduncle (A) and spinal compression (B).
2.2.3 Diagnosis
Histopathological examination reveals enormous pathological signs in diseased fish with focal necrosis in spleen, liver, kidneys, enhanced vacuolar degeneration, increased eosinophilia and haemosiderin in the kidneys, necrosis, pyknosis and infiltration of lymphocyte in the dermis and underlying lateral musculature of skin lesions. The wet mounts of the damaged skin and internal organs, followed by examination of the thin long rods (0.5–1.0 × 4–10µm) which flexes and glides in a characteristic manner, can act as a basic tool for diagnosis of the pathogen in a rapid and presumptive manner (Park et al., 2023). On the other hand, culture of the pathogen is necessary for confirmatory diagnosis of this disease. Flavobacterium species are known to exhibit slow or no growth in routine standardized bacteriological media but require specific media for separation and testing the antibiotic sensitivity. Usually, tryptone yeast extract agar containing 5% foetal bovine serum is used for the growth of the pathogen (Noga, 2010; Starliper, 2011).
In addition to bacterial culture, more sensitive diagnostic methods have been employed to find out F. psychrophilum in water, fish, as well as to identify or support conventional culture diagnostics for bacterial cold water disease. In the immunofluorescence antibody technique (Amita et al., 2000; Vatsos et al., 2006; Lindstrom et al., 2009) and for immunohistochemistry (Madetoja et al., 2002) large number of scientists have employed antisera produced against F. psychrophilum. For the purpose of detecting the pathogen in fish, enzyme-linked immunosorbent techniques have been devised utilising antibodies for F. psychrophilum cell surface components (Crump et al., 2004; Lindstrom et al., 2009). Chum salmon, Oncorhynchus keta kidneys and ovarian fluids were employed by Misaka et al (2008) to quantify live F. psychrophilum using nitrocellulose blotting of bacterial colony culture media plates followed by immunostaining. Nucleic acid genotype-based assays are being used and relied upon more frequently by fish disease diagnosticians to identify fish infection caused by F. psychrophilum, or to confirm the identifications done using other techniques, such as conventional phenotypic characterizations. Numerous approaches have been employed to understand the mechanism of this disease including the utilization of nested polymerase chain reaction assays (PCR), which are considered extra precise, and have been described earlier by many scientists (Taylor, 2004; Crumlish et al., 2007; Lönnström et al., 2008). A description of the diagnostic parameters of the bacterial cold water disease is given in Table 3.
2.2.4 Treatment
Antiseptic baths such as quaternary ammonium, chloramine-T or copper sulphate constant flow for one hour can be used for preliminary surface skin cases of bacterial cold water disease (Noga, 2010). Povidone-iodine is frequently been used to sanitise the surface of fertilised and eyed fish eggs (Wedemeyer, 2001). Even though F. psychrophilum cannot always be eliminated with this treatment, however, it does lessen the spread of egg-associated pathogens. Antibiotics such as oxytetracycline, oxolinic acid, sarafloxacin, ormetoprim-sulfadimthoxine, florfenicol and amoxicillin have frequently been employed which have shown a varying degree of success (Van Vliet et al., 2017). This pathogen has been shown to develop resistance to various antibiotics very quickly hence there is a dire need of determining the antibiotic sensitivity of the isolate. At the alevin stage the treatment of bacterial coldwater disease is very difficult because the feeding is completely seized until all of the yolk sac is utilized (LaFrentz et al, 2004). Currently no vaccine is commercially available for the effective treatment and management of this disease prevalent in freshwater aquaculture systems.aW5mb0BzdXN0YWluYWJsZWFxdWFjdWx0dXJlLmNvbQ==
2.3 Columnaris disease/saddle back disease
2.3.1 Epidemiology
Flavobacterium columnare, belonging to family Flavobactericeae is the causative agent of columnaris disease and is regarded as one of the most dreadful bacterial diseases of freshwater fish species across the globe. Other species of Flavobacterium known to cause disease include Flavobacterium oreochromis sp. nov, Flavobacterium covae sp. nov, Flavobacterium davisii, sp. nov. Davis described the columnaris disease in fish species residing in warm waters and found that numerous slim and motile bacteria were present in the lesions but was unable to successfully culture the etiological agent (Declercq et al., 2013a). Bacteria produced columnar structures within these lesions, which were visible in a wet mount preparation. Thus, the pathogen was given the name Bacillus columnaris, and the condition was dubbed columnar sickness. Since Davis's seminal work, various studies were made about the taxonomic position of this pathogen over the years. Columnaris disease in sockeye salmon, Oncorhynchus nerka was first discovered and isolated by Ordal and Rucker in 1944 (Declercq et al., 2013b). The bacterium was grown in a diluted culture media and was determined to be a myxobacterium based on its characteristic cell shape (Bullock, 1986). The infection is known to spread very quickly within a fish population and cause large number of mortalities. Having the capacity to respond efficiently to fluctuating ecological variables by being living and non-dividing for a very long time, this bacterium can live outside its host for a very long time. As a result of its widespread and rapid mortality rate the disease has a significant economic impact on fish production worldwide (Arias et al., 2012).The direct correlation of disease severity with water temperature and strain virulence has been reported (Noga, 2010).At 15 °C water temperature, Flavobacterium columnare becomes active. Pathogenicity and water temperature being directly corelated shows that pathogens increase with the increase of water temperature which in turn leads to elevated disease severity hence results in higher number of mortalities. Study also shows that mineral content of water has a significant role in determining the virulency of the isolate. In soft water having about 10mg/L CaCO3 especially with low organic content, Flavobacterium shows decreased pathogenicity. One of the studies revealed that some isolates were found to be non-pathogenic at optimum hardness of about 70mg/l in distilled water. Calcium, magnesium, potassium and sodium ions all are vital for continued survival of F. columnare in water (Fijan, 1969; Pulkkinen et al., 2010) Organic pollution, Oxygen deficiency, high nitrite, physical injury (e.g., net damage) are some of the vital aspects for the progression of the columnaris disease (Fijan, 1969). Left out feed at the culture sites promotes the growth of this bacterium hence, is considered as a source of infection clearly indicating that cleaning the culture sites properly is a key step in stopping the growth of the bacterium hence preventing the disease outbreak (Kunttu et al., 2012).
2.3.2 Clinical signs/pathogenesis
Gills, skin, and fins are the main body portions affected during the columnaris infection. The virulence of the strain determines the severity of the clinical signs of columnaris disease. Low virulence strains brought delayed progressive infections at water temperatures over 21 °C resulting in extensive tissue impairment prior to death. It has been reported that Atlantic Salmon (Salmo salar) juveniles infected with highly virulent strains died from their fulminating diseases within 12 to 24 hours when kept at 20 °C. Upon death, these fish typically showed no signs of severe tissue injury (Rucker et al., 1954; Declercq et al., 2013b).
Being an epithelial disease, the onset of the disease occurs with disruptive erosion of dorsal and tail fins which ultimately leads to eruption of grey and yellowish spots on the external surface of head, back and fin areas having a red coloured boundary prominently at the caudal fin region. A prominent necrosis of epidermis is also observed in these areas affecting significantly the integument, dermis, and the fish muscle. As opposed to typical fin rot, the degradation of the fin begins at the lesion's site around the base of the fin and works its way towards the fin tip. After starting at the dorsal fin base, the lesions spread sideways and eventually enfold the fish like a “saddle-back” (Declercq et al., 2013b). Thus, the name “saddle-back disease” is being used to describe the condition. The fin rays are damaged to such an extent that only the fragmented portions of the fin rays are present as the epithelium is sloughed down during infection leaving a characteristic ragged appearance of the surface. Yellow or orange appearing ulcers are also developed at the sites of lesions due to the immense growth of the pigmented bacteria at the affected sites. These ulcers penetrate the deeper layers of the skin leading to a condition called as bacteraemia. In columnaris disease, gill infections when present give rise to a serious condition, lesions often start at the tips of the gills and extend towards the gill arch causing the distortion of the whole gill structure. It is possible for the lesions to spread both cranially and caudally, penetrating deeper layers of skin and exposing the musculature, which can then cause severe ulcers (Figure 3) (Declercq et al., 2015). Yellowish white mucus often covers the lesions. Additionally, oral mucosal ulceration leads to mouth rot. It is thought that oral lesions are more likely to cause death than dermal lesions due to hampered feed consumption leading to anorexia. Additionally, the mandible and maxilla are easily affected by the condition. Along with filamentous bacteria, secondary infections with fungi or other bacteria might worsen the condition. The filaments composed of numerous bacterial cells emerge from the lesions in case of tropical fish hence known as “cotton wool disease” or “mouth fungus” (Bernardet and Bowman, 2006).

Figure 3. Rainbow trout with columnaris disease, showing cotton wool-like growth (A) and ulceration on lateral body wall (B).
2.3.3 Diagnosis
Preparing the wet mounts of the F. columnare from the lesion reveal thin long rods (0.50–1.0 × 4–10 micrometres) having characteristic flexing or gliding motion and forms the basis of the quick presumptive identification of this bacterium. The bacteria form a column or haystack like structure when the wet mount of the lesion is left undisturbed for a while and there are times that there is no haystack formation even when examination of the wet mount is delayed (Declercq et al., 2013b). Standard bacteriological media does not grow F. columnare, thus special type of media is required for both isolation as well as antibiotic sensitivity testing. In case of bacteriological culture low nutritional content media supplemented with high moisture content is recommended (Noga, 2010). Cytophaga agar (Anacker and Ordal, 1959) or a 1:10 dilution of nutrient broth in 1% agar has extensively been employed, but specific media containing antibiotics significantly enhance isolation. Besides isolation other techniques may also be employed to find out this pathogen. The enzyme-linked immunosorbent assay (Shoemaker et al., 2003; Darwish et al., 2008) and the fluorescent antibody test (Speare et al., 1995; Panangala et al., 2006) are the best examples of diagnostic serological techniques that fall within this category. Both have shown to be quick and effective in detecting columnaris disease. Channel catfish were used to develop a loop-mediated isothermal amplification method (LAMP) for the quick identification of F. columnare from infected fish organs such as gills, skin, and head kidneys (Yeh et al., 2006; Mabrok et al., 2021). The benefit of PCR is its capacity to identify very low levels of F. columnare therefore acts as a promising diagnostic tool (Darwish et al., 2004; Welker et al., 2005). Additionally, Panangala et al., 2007 have created a TaqMan-based real-time PCR that specifically targets a 113 base pair nucleotide sequence of F. columnare chondroitin AC lyase gene. To detect and quantify the presence of F. columnare in tissues (blood, gills, and kidney) of infected fish, the PCR is specific, sensitive, and repeatable. Real-time PCR-based techniques are also noticeably more favourable than traditional PCR techniques since they do not require the detection of amplified products by gel electrophoresis, which saves money, time, and labour (Panangala et al., 2007). A description of the diagnostic parameters of the columnaris disease is given in Table 4.
2.3.4 Treatment
At the beginning of the outbreak antiseptic baths or continued dip in potassium permanganate or copper sulphate can be successfully used in the treatment of columnaris infection. However, in severe cases i.e., persistent lesions having exposed muscle or when 5% of the surface of body is covered by lesions, systemic antibiotics are required (Farmer et al., 2012). Different antimicrobial treatments for columnaris disease have had varying degrees of efficacy. Only in the preliminary phases of the disease, when the infection is still superficial, are external treatments effective (Bullock, 1986). Chloramphenicol (Snieszko, 1958), nifurpirinol (Amend and Ross, 1970), nifurprazine and oxolinic acid (Soltani et al., 1995) are medications that have been used successfully in bath therapy. Recently, it has been recommended to deliver antimicrobials in the feed during the early onset of the disease or when septicaemia symptoms are seen. Both early and severe outbreaks of columnaris disease in Pacific salmon, Salmo salar were successfully treated with oxytetracycline when given orally up to 10 days (Wood, 1974; Bullock, 1986) Sulfonamides, including sulfamerazine and sulfamethazine can be used orally but are less potent than other medications. Additionally, nitrofuran may be given orally for 3 to 5 days (Bullock et al., 1986). The effectiveness of florfenicol in the feed against columnaris disease in channel catfish has been demonstrated (Gaunt et al., 2010). Many of the F. columnare isolates are resistant to ormetoprim-sulfadimethoxine (Declercq et al., 2013a). Recent advancements include the development of F. columnare vaccine which has been successfully employed for the treatment of columnaris disease in cat fish (Guo et al., 2023).
2.4 Lactococcosis
2.4.1 Epidemiology
Globally, Lactococcus garvieae (formerly known as Enterococcus seriolicida) is alleged for charge of substantial loss of economy in global aquaculture by triggering lactococcosis generating haemorrhagic septicaemia (Austin and Austin, 2012b) and meningoencephalitis like variety of diseases among diversity of fish species (Evans et al., 2009; Didinen et al., 2014). It is considered as a growing zoonotic pathogenic bacterium that is held responsible for serious infections among humans as well as animals (Vendrell et al., 2006). Despite the fact that this bacterium can infect a huge diversity of fish species including the most of marine fishes, however reports of its high pathogenicity have also been found in rainbow trout (Austin and Austin, 2007, Austin and Austin, 2016; Meyburgh et al., 2017; Chapela et al., 2018). Due to its high pathogenicity, the evolution of many strains resistant to antibiotics, the deficiency of efficient control methods, and the migration of diseased fish between different stations, this bacterium has spread to a number of nations since the 1980s. L. garvieae is not exclusively aquatic, it can also live on a variety of hosts. Additionally, it has identified in raw cow's milk, chickens, water buffaloes, as well in cows with asymptomatic intramammary infections. L. garvieae has also been recovered from humans on numerous occasions, signifying that it may be considered a possible zoonotic agent (Xie et al., 2023).
2.4.2 Clinical signs/pathogenesis
Two categories of bacterial serotypes have been identified: non-agglutinating (KG) and agglutinating (KG+) phenotypes, which are determined by the capacity to agglutinate serum produced against L. garvieae (Soltani et al., 2021b). The disease fish are initially lethargic and display aberrant behaviour such as asymmetrical and twisting movements, and darkening of the skin (Soltani et al., 2008; Austin and Austin, 2016). The affected fish also had an enlarged abdomen, anal prolapse, unilateral or bilateral exophthalmia, cataracts, as well as splenomegaly and hepatomegaly, accumulation of turbid ascitic fluid in the coelomic cavity, and the presence of exudates in the brain in the final stages of the disease (Figure 4). The infected fish may also exhibit acute hyperaemia, extensive bleeding, petechiae of the organs, including the intestine's mucosal layers (Leung and Bates, 2013; Meyburgh et al., 2017). Gram stain preparations of hematopoietic tissue imprints, obtained from the kidney and spleen, can reveal massive numbers of Gram-positive coccoid cells in single or chain formations in more severe diseased conditions (Soltani et al., 2021a).
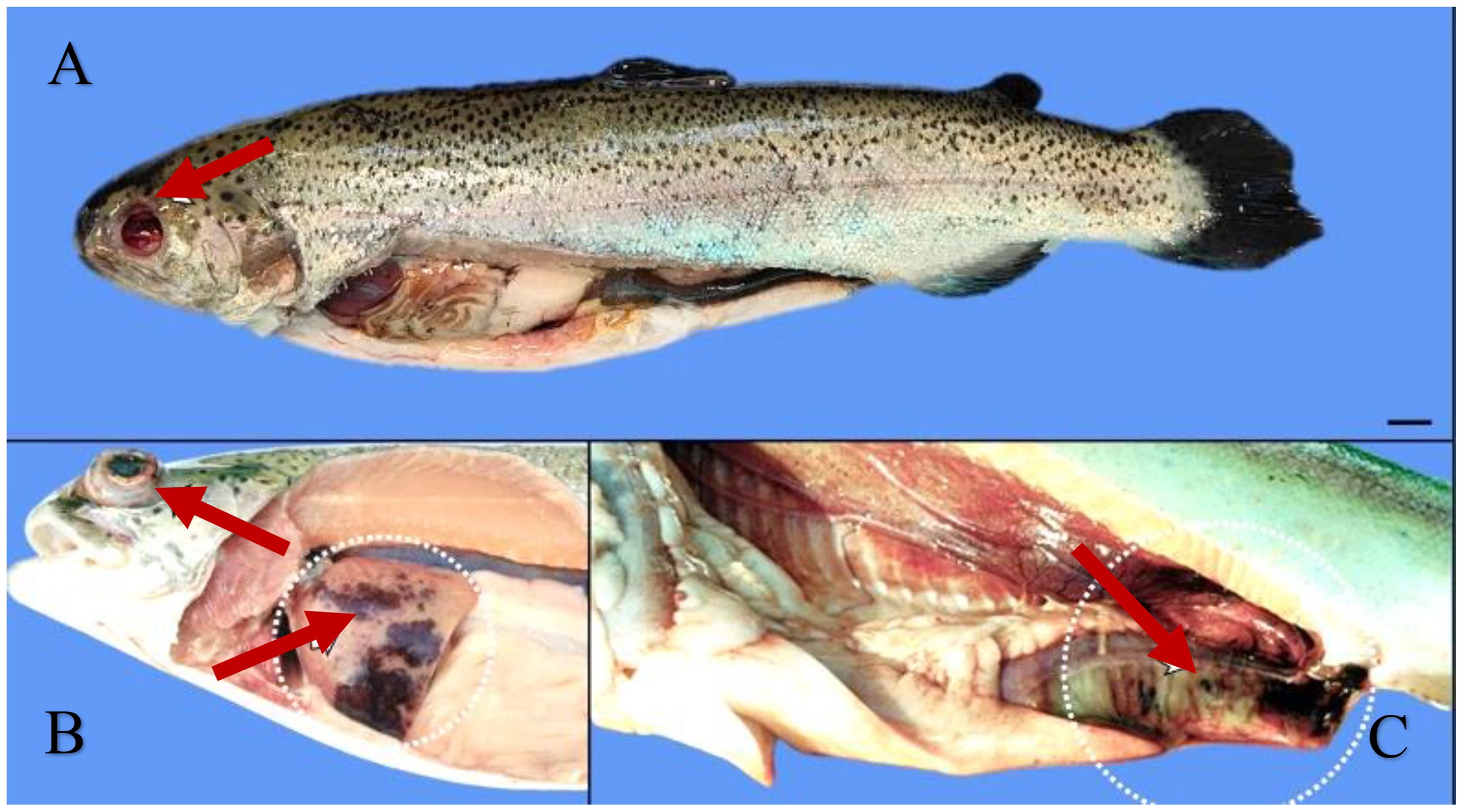
Figure 4. Rainbow trout with lactococcosis, showing eye enucleation (A), unilateral exophthalmia with the presence of haemorrhages in the liver (B), and heavily haemorrhagic intestine (C). Source: picture courtesy Esposito et al. (2024).
Although lactococcosis has been described as a hyperacute and haemorrhagic septicaemia in fishes (Bercovier et al., 1997). The progression of the disease is largely dependent on the environmental factors in fish keeping facilities, particularly the temperature and microbiological conditions of water (Prieta, 1993). It has been reported that when lactococcosis is present in fish species, such as yellowtail or grey mullet, the typical clinical indications are remarkably comparable to those seen in salmonids. It is crucial to stress upon the fact that the disease causes significant economic losses due to raised mortality rates of about 50%, and responsible for decreased growth rates, and unpresentable fish look. Numerous experimental studies have shown that the disease incubation period is quite brief and the bacterium is highly virulent. Intramuscular infection in grey mullet, M. cephalus produced initial signs and 100% death two days after inoculation, while intraperitoneal infection in yellowtail induced symptoms 2–3 days after inoculation. The first symptoms and mortality following an experimental intraperitoneal infection in rainbow trout occurred three days after inoculation (Nakai et al., 1999; Vendrell et al., 2006; Khalil et al., 2024). There are various amounts of haemorrhages found within air bladder, kidney, gut, liver, peritoneum, and spleen, as well as macroscopically visible lesions that are indicative of an acute systemic disease in affected fish. Additionally, the isolated areas of liver and spleen necrosis, pericarditis, haemorrhagic effusion in the intestines, and yellowish fluid coating the surface of the brain are frequently been seen due to the involvement of this disease (Bekker et al., 2011).
2.4.3 Diagnosis
L. garvieae may typically be grown on the traditional culture media that are used to recover pathogens in aquaculture, such as TSA, BHIA, BA, or nutrient agar (Vendrell et al., 2006). It is occasionally possible to utilise selective media with inhibitors, such as bile esculin agar. 37 °C is the ideal temperature for L. garvieae growth. When compared to other fish bacterial infections that cannot develop at high temperatures, this temperature can be used as preliminary step in the differential diagnostic procedures. The most common techniques for identifying this pathogen are traditional biochemical assays, which are based on its biochemical properties allowing the phenotypic characterization of the pathogen. The popular serological methods for diagnosing L. garvieae include slide agglutination, which uses a pure culture of the pathogen as an antigen fluorescent antibody staining (Vendrell et al., 2006), and more recently, a rapid flow cytometry-based technique that can be used to find the pathogen in mixed cultures (Kim et al., 2025). Samples for the histological diagnosis of L. garvieae are taken from visceral organs, including the encephalon, liver and kidney. The haematoxylin and eosin procedure must be used to stain them. The study of plasma samples from sick fish served as evidence of the effectiveness of PCR method for identifying L. garvieae. The assay, however, was unable to identify the pathogen in ambient water samples from ponds where lactococcosis was active (Huang et al., 2024). A description of the diagnostic parameters of the lactococcosis disease is given in Table 5.
2.4.4 Treatment
Repeated re-infection and short-term disease brought on by L. garvieae in fish farms has been treated with a number of medications, including erythromycin, spiramycin, kitasamycin, josamycin (macrolide antibiotics), oxytetracycline, doxycycline, enrofloxacin, florfenicol, lincomycin, and amoxicillin (Kawanishi et al., 2005; Soltani et al., 2008). The issue with the use of antibacterial drugs is that, despite having good in vitro activity against L. garvieae, their use in fish farms is limited by the fish's loss of appetite or perhaps by the diseased fish's inefficient antibiotic metabolism (Romero et al., 2012; Huang et al., 2024).
2.5 Bacterial kidney disease
2.5.1 Epidemiology
Bacterial kidney disease (BKD), mainly infects salmonid fish worldwide and the causative agent is Renibacterium salmoninarum (Murray et al., 2012; Bayliss et al., 2018). The causal agent might affect the fish both horizontally and vertically (Boerlage et al., 2018). The bacterium can damage salmonids both in wild and in captivity at any stage of life, which has a negative effect on the salmonid business (Johansen et al., 2011; Wiens, 2011). Mortalities up to 80% has been recorded in Pacific salmon, Oncorhynchus spp. and 40% in Atlantic salmon, Salmo salar which are linked to R. salmoninarum (Evenden et al., 1993) While, daily deaths are as high as 5% and have been reported in some extreme situations (Boerlage et al., 2017) especially at juvenile stage and pre-spawning adults (Wiens, 2011). Ecological characteristics, geographic location, habitat, food quality, fish size and age are a few risk variables that can affect bacterial growth and the onset of this disease. The sickness is more common during the summer months. It has been observed that fish supplied in the spring and summer are noticeably more likely to get BKD, indicating that the time of year during which they were transferred out of the hatchery also affects their probability of having R. salmoninarum infection. This might be described by the fact that the fish were younger and more vulnerable during the peak time when the bacterium was most active (Boerlage et al., 2018). The first mention of BKD in wild Atlantic salmon was reported during 1930s in Scotland. A related disease was discovered in brook trout, Salvelinus fontinalis brown trout, Salmo trutta and rainbow trout, Oncorhynchus mykiss in 1935 (Delghandi et al., 2020c) Salmonid stock culture from Japan, South America, North America and Europe have all been reported to have BKD. Since this disease is caused by a freshwater infection, there have not been any reports of it from Ireland, Australia or New Zealand. Like other bacterial disease the coldwater pathogen R. salmoninarum is mostly been active at temperatures of 15 °C or lower. Purcell et al. (2016) showed that the mortality, total bacterial load in the kidney tissue, and the rate of shedding the bacteria were highest at lower temperature, while making a comparison in severity of the disease in chinook salmon at different temperatures of about 8, 12 and 15 °C (Purcell et al., 2016). They also observed a rise in the frequency of breakouts during periods of low temperature. The severity of the condition is significantly influenced by water temperature, with lower temperatures being linked to a severe demonstration (Plumb, 2018). There is no need for an intermediary host for BKD transmission, as it can spread both horizontally and vertically. The gastrointestinal tract, skin diseases and the eyes are all potential portals of entry for horizontal transmission (Hoar et al., 1997) While in case of salmon it is transmitted through feed and water, cannibalism among diseased fish, and the consumption of contaminated raw viscera of fish (Plumb, 2018). Nevertheless, this bacterium does not survive during processing (Cunningham, 2002). In seawater net-pens, BKD is sometimes transmitted by the excrement of sick fish (Balfry et al., 1996). Vertical transmission is another feature of this disease as the pathogen has been recovered from coelomic fluid (Suzuki et al., 2017) and is known to enter the egg yolk during fertilisation hence, disinfecting the whole surface of egg does not result in the removal of the bacteria (Evelyn et al., 1986).
2.5.2 Clinical signs/pathogenesis
BKD is a chronic disease that is frequently accompanied by low levels of mortality, making it a challenging task to identify an issue. R. salmoninarum infected fish typically lose their balance and exhibit erratic swimming behaviour from the outside. Ocular lesions (Richards et al., 2017) and unilateral or bilateral exophthalmia (Eissa and Elsayed, 2006) are additional external symptoms. There have also been reports of discoloration of skin surface, darkly pigmented areas, distension of the abdomen, pale gills, anal vent haemorrhage and corneal opacity. Blebs and blisters on the epidermis with white or yellowish haemorrhagic fluid have also been reported which are linked with BKD (Delghandi et al., 2020a) also haemorrhages or petechiae near the fin areas and lateral line surface have been observed (Richards et al., 2017). Adult infected fish have muscle cavitation and a belly rash on the skin (Buller, 2004; Plumb, 2018). Internal symptoms include liver, spleen, kidney, and heart enlargement as well as leucocyte, bacterial, and host cell debris-filled white-creamish and grey appearing granulomatous lesions on the visceral surface (Figure 5) (Evenden et al., 1993). More infrequently reported symptoms include bleeding into the muscles and internal organs (Eissa and Elsayed, 2006) bloody ascites fluid accumulation in the abdominal cavity, and covering internal organs with a false membrane, especially in fish kept at low temperatures, raising the possibility indicating that the disease is more chronic form of the disease (Evenden et al., 1993; Fryer and Sanders, 1981). Rainbow trout, brook trout, Atlantic, Chinook, and Coho salmon have been documented having brain lesions as a result of the central nervous system (CNS) being invaded by the posterior ocular canal (Buller, 2004; Plumb, 2018). Histologically, BKD is characterised by the emergence of granulomas, non-encapsulated granulomatous lesions, chronic inflammation, caseation in the centres, proliferative division of epithelioid macrophages (melano-macrophages) in the kidney, liver and spleen along with glomerulus degeneration and alteration of renal corpuscles and tubules. Hematopoietic tissues such as macrophages, lymphocytes, neutrophils, and eosinophils surround the uriniferous tubules and glomeruli. In rare cases, this disease has been associated with adhesion in the bowman's capsule (Elliott, 2017). R. salmoninarum also causes focal tissue injury in the spleen by invading the lympho-haemopoietic and splenic parenchyma. Barrier cells, infected macrophages, and monocyte-like cells have all been observed (Delghandi et al., 2020a). Hepatocyte separation and the presence of fibrin within sinusoids are two examples of histopathological changes in the liver (Kent et al., 2013). It has also been suggested that condition like encephalitis and meningitis in Atlantic salmon and chinook salmon due to R. salmoninarum might be connected to neurological disease in the brain (Kent et al., 2013; Elliott, 2017). Heart being another target organ in infected fish develops granulomatous myocarditis with eosinophils, karyorrhectic debris, macrophages, and neutrophils, as well as spongy and necrotic myocardium (Kent et al., 2013).
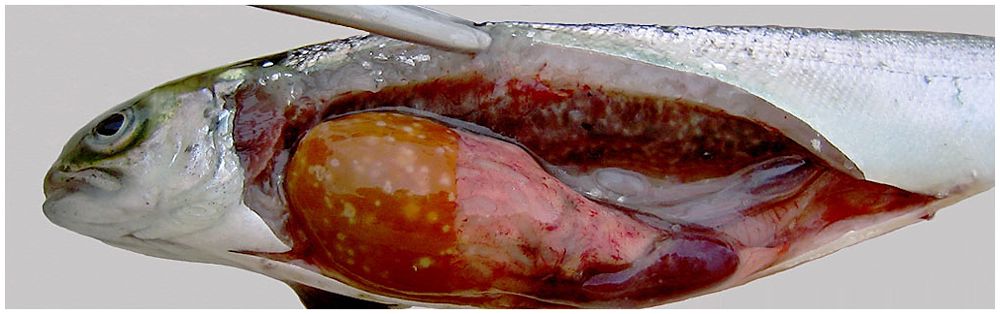
Figure 5. Atlantic salmon with bacterial kidney disease, showing profound kidney and liver granulomas. Source: picture courtesy Jaime Santana.
2.5.3 Diagnosis
Specific media, such as kidney disease medium-1 (KDM-1), KDM-2, KDM-C (containing charcoal), and selective kidney disease medium have been created for the slow-growing, fastidious pathogen R. salmoninarum (SKDM) (Hirvelä-Koski et al., 2006).The bacterium is reported to grow on Ten-M medium (Chambers and Barker, 2006) and generates creamy, smooth, and shiny colonies, when grown on KDM-2 (Hirvelä-Koski et al., 2006). Likewise, studies have proven that this organism is capable of growing in KDM-2 medium containing culture-spent medium of R. salmoninarum (SMRs) instead of foetal bovine serum (FBS) (Matsui and Nishizawa, 2009). Because R. salmoninarum is slow growing, overgrowth by heterotrophic bacteria is likely. A description of the diagnostic parameters of the bacterial kidney disease is given in Table 6.
2.5.4 Treatment
Antimicrobial agents such as cycloheximide, D-cycloserine, polymyxin B sulphate, or oxolinic acid can be used for the protection from contaminants (Bethke et al., 2017) also the enrichment with an antibiotic may decrease the risk of contamination by fungal or other non-specific bacterial agents. As per existing literature, it has been observed that BKD is considered as one among the challenging diseases of fish to manage. There are presently no effective vaccines available, wild fish are recognised to operate as a pool and a vector for the disease (Wiens et al., 2008; Delghandi et al., 2020a). Also, there are no therapeutic methods available to entirely eradicate R. salmoninarum from the salmonid fish (Noga, 2010). The first course of antibiotic therapy against R. salmoninarum was used in Blueback salmon, Oncorhynchus nerka by using sulfadiazine (264 mg/kg) for 7 days, followed by a lower dose of 132 mg/kg for the next 21 days (Rucker et al., 1951; Delghandi et al., 2020a). Since then, numerous more antibiotics have been used to treat BKD, including erythromycin, azithromycin and enrofloxacin (Hsu et al., 1994; Austin et al., 2007). Remarkably, the practice of erythromycin as a medication in fish is not being permitted in United States. However, some other countries including India, erythromycin is being used as antibiotic agent for the management of BKD in fishes (Bethke et al., 2017).
Antibiotic therapy necessitates lengthy treatment of about 28 or 30 days due to the bacterium's low susceptibility (Rhodes et al., 2008). Additionally, antibiotic therapy is not very successful due to R. salmoninarum's intracellular position. The reported occurrences of antibiotic resistance are more significant (Wiens, 2011). For instance, analysis of seven R. salmoninarum strains revealed decreased sensitivity to macrolide antibiotics. On the genome of R. salmoninarum, several classes of macrolide resistance genes have been discovered, including the genes for two 23S rRNA methyltransferases (rlmA and sopU), the macrolide efflux factor (mefA), the multidrug resistance efflux pump (pvsC), and the 16S rRNA dimethylase (ksgA) (Rhodes et al., 2008; Wiens et al., 2008; Montforts and Keen, 2011). Although, the most widely used strategy for containing bacterial disease outbreaks is still the usage of antibiotics, however, the excessive use of antibiotics has negative effects on both the environment and public health. In this situation, it is crucial to limit the use of antibiotics and, in specific, to identify trustworthy substitutes for these overused medications. Hence the management of the disease poses a serious challenge to the aquaculture industry.
2.6 Enteric red mouth disease
2.6.1 Epidemiology
A devastating septicaemic bacterial disease found mostly in salmonid species is called as enteric red mouth disease which is caused due to the gram-negative rod-shaped enterobacterium Yersinia ruckeri. It affects extensive number of hosts and is broadly dispersed geologically. The appearance of this disease was noted in rainbow trout, O. mykiss, from a trout farm in Idaho, USA during in the early 1950s It was later discovered in Europe, Australia, Africa and Asia (Zorriehzahra et al., 2017). Fish of all age groups can be impacted by ERM, however young fish are most severely affected (fry and fingerlings). In older or large sized fish, the sickness manifests a more severe disorder. Due to the outbreak of this disease initially only a few deaths occur but with the passage of time, substantial cumulative stock losses has been reported (Horne and Barnes, 1999; Tobback et al., 2007). Infections caused by Yersinia ruckeri can disseminate via direct contact between infected and healthy fish. A carrier condition has been established, wherein infected fish can endure up to two months after both experimental and natural exposure (Tobback et al., 2007). It has also been established that as much as 25% of a rainbow trout population may include Y. ruckeri in their lower intestine. Bacteria are released when carrier fish undergo stress and were found to transmit Y. ruckeri to clinically healthy fish at an increased temperature of 25 °C, but no transmission was detected from unstressed carriers (Busch and Lingg, 1975; Kumar et al., 2015). Since Y. ruckeri can survive for at least four months outside of its host, it is likely that faecal shedding contributes significantly to its spread. In order to adhere to other bacteria and aid in the establishment or growth of microcolonies, bacterial cells use pili or flagella to move across surfaces (Coquet et al., 2002). Initiating the formation of biofilms requires the overexpression of flagellar proteins, a phenotypic characteristic associated with enhanced adhesiveness. It is now commonly acknowledged that one of the most important survival strategies for bacteria on the surface of fish body and in sediments of aquatic environments is the creation of biofilms (Kumar et al., 2015). Potential vectors like birds and aquatic invertebrates have been linked to the spread of Y. ruckeri. The possibility of vertical transmission of the infection is suggested by the recent discovery of Yersinia ruckeri DNA in Chinook salmon ovarian fluid and unfertilised eggs. However, this study was unable to verify if bacterial cells were present in the egg chorion, though (Glenn et al., 2015).
2.6.2 Clinical signs/pathogenesis
Fish may exhibit altered behaviour, such as abnormal movements on the water surface with characteristic sluggish movements and hunger reduction. Exophthalmia, skin darkening, subcutaneous haemorrhages usually found prominently in and around the mouth and throat, could be the other symptoms of the ERM disease. Petechial haemorrhages can happen in the lateral muscles including the surfaces of the liver, pancreas, pyloric caeca, and swim bladder. Besides, lower intestine becoming prominently red coloured containing an opaque, yellow appearing fluid with frequently enlarged spleen, could be the other symptoms that indicate the appearance of ERM (Figure 6) (Horne and Barnes, 1999; Tobback et al., 2007). Histopathological analysis reveals systemic septicaemia with chronic inflammation seen in most of the organs, especially the kidney, spleen, liver, heart, gills along with petechial haemorrhage. Hyperaemia, oedema and desquamation of the epithelial cells of the secondary lamellae have all been characterised as pathological alterations in the gills. The liver, kidney and spleen can all have focal regions of necrosis. Degenerated renal tubules, glomerular nephritis and a noticeable rise in melano-macrophages can all be seen in the kidney (Tobback et al., 2009). It has been observed that rainbow trout experimentally infected with Y. ruckeri showed that the gills provide a key portal of entry for this pathogen. Recent research used immunohistochemical and optical projection techniques to observe and understand the mechanism of spreading of ERM in rainbow trout (Ohtani et al., 2014). According to the study, it has been suggested that Y. ruckeri at the onset of infection infects the secondary gill lamellae and within one minute of infection it enters to the circulatory system through the gill pavement cells. At about 30 minutes post infection the bacteria spreads to the gut lumen, after 3 days it enters to the kidney tissues and at 7 days post infection major organs are known to be affected. However, after 21 days of infection no visible signs of Y. ruckeri in the liver, spleen, brain or heart can be observed (Waine et al., 2023).
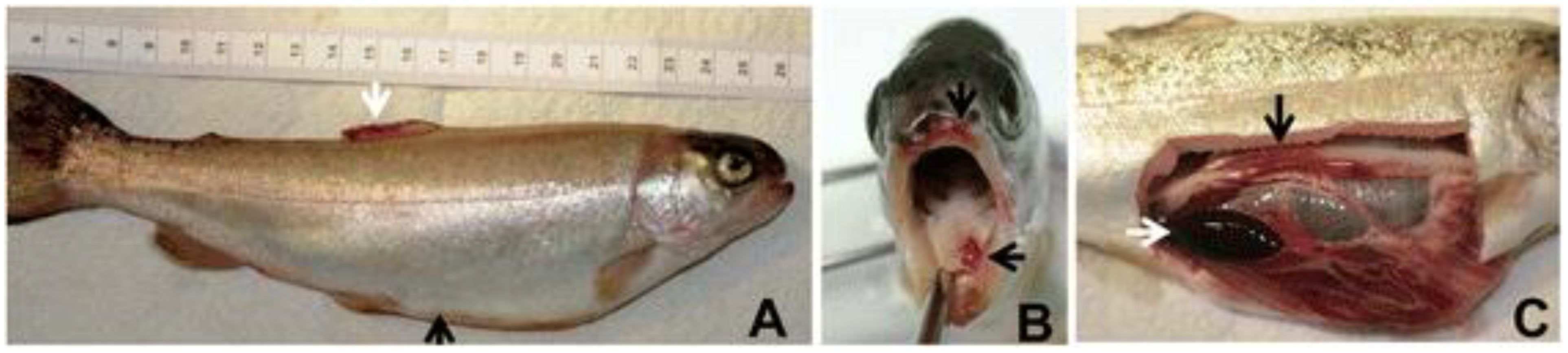
Figure 6. Rainbow trout with enteric red mouth disease, showing enlarged abdominal valley (black arrow) and haemorrhages in the dorsal fin (white arrow) (A), haemorrhages in and around the mouth (B), and enlarged and black spleen (white arrow) and reddened intestine (black arrow) (C). Source: picture courtesy Kumar et al. (2015).
2.6.3 Diagnosis
Several diagnostic techniques have been created, including molecular biology methods, serological testing and culturing of the causative agent (Tobback et al., 2007). Different types of culture media specifically that of Tryptic soy agar, Columbia blood agar and MacConkey agar have been used to identify Y. ruckeri (Horne and Barnes, 1999; Gibello et al., 1999; Austin et al., 2003; Tobback et al., 2007). Though Y. ruckeri may thrive at a variety of temperatures, its ideal range is between 20 and 28 °C (Tobback et al., 2007; Horne and Barnes, 1999). A large variety of immunodiagnostic techniques have already been employed for the recognition and identification of Y. ruckeri among which ELISA, agglutination, and immunofluorescence antibodies have all been widely used. Of the molecular diagnostic techniques, the most widely employed ones include polymerase chain reaction (PCR) (Gibello et al., 1999; LeJeune and Rurangirwa, 2000; Altinok et al., 2001), Loop-mediated isothermal amplification (LAMP) (Saleh et al., 2008) and restriction fragment length polymorphism (RFLP) (Garcia et al., 1998). Y. ruckeri can be found in the tissues of infected fish using PCR-based amplification of the 16S rRNA gene (Gibello et al., 1999; Wiens et al., 2008; Saleh et al., 2008). Altinok et al., 2001 developed a LAMP assay that enhances the Y. ruckeri quorum sensing system's yruI/yruR gene. Visual inspection or gel electrophoresis can be used to identify the amplification products in this ERM-LAMP assay, which is sensitive and quick. A description of the diagnostic parameters of the enteric red mouth disease is given in Table 7.
2.6.4 Treatment/control
A crucial step in preventing the spread of ERM involves the identification of asymptomatic carriers and this has been achieved through the use of PCR technique, which is capable of identifying the causative agent at specifically low concentrations (Gibello et al., 1999; Gohari et al., 2010). Culturing of faecal samples and head kidney biopsy by dorso-caudal aspiration of the kidney tissues, blood samples are non-lethal techniques used in the identification process of the causative agent of disease (Noga et al., 1988; Altinok et al., 2001). Specificity of traditional and quantitative PCR have been enhanced using other specific potential genes (Glenn et al., 2011). A novel qPCR technique based on the recombinant protein A (recA) gene was described by Bastardo et al., 2012. Emerging technologies like gold nanoparticle-based assays have the potential to be effective tools for the quick, accurate, and sensitive identification of Y. ruckeri nucleic acids in clinical samples. DNA or RNA hybridises with pathogen-specific probes that are affixed to the surface of the gold nanoparticles in nanoparticle-based tests. The nanoparticles aggregate and change colour from red to blue as a result of hybridization, which takes place at a specific temperature and duration (Saleh et al., 2015).
Post-infection care mostly makes use of antibiotics, as is typical with fish bacterial infections. Unfortunately, only a small number of different antibioticsare frequently utilised (Alderman and Hastings, 1998). Some of the widely used antibiotics involve the use of amoxicillin, oxolonic acid, oxytetracycline, sulphadiazine combined with trimethroprim, and more recently, florfenicol has also been used to cure this disease (Michel et al., 2003). This limited selection of choices can encourage the development of antibiotic resistance (Defoirdt et al., 2007). Only one out of fifty Y. ruckeri isolates was found to be florfenicol resistant by screening (Michel et al., 2003). Similar to this, a more recent assessment claimed that the majority of European isolates continue to exhibit widespread resistance to antibiotics (Calvez et al., 2014). Despite the fact that a lactamase gene for Y. ruckeri has been found on its chromosome (Mammeri et al., 2006) there is evidence to suggest that this gene is not likely to be produced at high levels (Stock et al., 2002). Oxolinic acid, oxytetracycline and potentiated sulphonamide has indicated resistance against Y. ruckeri (Rodgers, 2001). Finally, and more anecdotally, holomycin resistance and production by Y. ruckeri are both natural processes (Qin et al., 2013). Among the earliest fish diseases for which a reliable commercial vaccination was created is ERM (Horne and Barnes, 1999; Villumsen et al., 2014). The vaccine uses whole cell suspensions of monovalent, inactivated Y. ruckeri serotype O1 biotype 1, which can be given to fish orally, intravenously, orally by immersion and it offers adequate defences against Y. ruckeri biotype 1 strains (Villumsen et al., 2014). Monovalent vaccines fail to produce protection against biotype 2 infection because Y. ruckeri biotype 2 strains are more difficult to treat and have been implicated in disease epidemics in fish that had been immunised against biotype 1 strains (Austin et al., 2003; Arias et al., 2007). A bivalent vaccination was created utilising Y. ruckeri biotypes 1 and 2 that had been formalin inactivated, but offers effective defence against the biotype 2 strains (Tinsley et al., 2011a; Deshmukh et al., 2012). Atlantic salmon, Salmo salar challenged with Y. ruckeri O1b biotype 1 using cDNA microarray analysis of gill tissues have revealed the presence of genes and biosignatures that may serve as predictors of vaccination efficacy.
2.7 Furunculosis
2.7.1 Epidemiology
Furunculosis is a serious, severe, infectious disease reported from both fresh and saline environment and is considered as economically devastating fish disease. Emmerich and Weibel of Germany were the first to identify and characterise this sickness in hatchery fish (Cain and Polinski, 2014). They identified a bacterium which is responsible for this disease and named it as salmonicida. Later, the disease which had previously only been suspected in German trout hatcheries, became known worldwide, debunked the notion that furunculosis was limited to hatcheries by showing that it could be found in wild trout as well. Furunculosis quickly spread beyond of its original home in Germany, showing up first in neighbouring Austria, then Belgium, Ireland, France, Switzerland, and the United Kingdom (Kar, 2015). Caused by Aeromonas salmonicida presently known as septicemic disease can be found all over the globe. Many species of farmed and wild stock fish are susceptible to this crippling and ultimately fatal disease, including salmonids and cyprinids, with the family Salmonidae being the hardest hit (Noga, 2010). Several studies (Toranzo and Barja, 1992; Hiney and Olivier, 1999) suggested that furunculosis is known to exhibit a large effect on non-salmonid fish also. The occurrence of typical furunculosis may even constitute a restraining feature in the sustained existence of few fragile fish populations including the wild Atlantic salmon of USA and Spain. Several studies have shown that Atlantic salmon carry hidden A. salmonicida infections once they return from ocean during their routine annual migration (Cipriano et al., 1996). The disease got its name due to the fact that it causes the characteristic red spots or furuncles leading to darkening of skin all along the body. Besides A. salmonicida subspecies salmonicida, three other Aeromonas spp. (Aeromonas caviae, A. hydrophila and A. sobria) have been isolated from fish with furunculosis but none of these 3 bacteria are responsible for the disease. Only non-motile, rod shaped, facultatively anaerobic, non-sporulated psychrophilic A. salmonicida bacterium having size of about 0.8 to 1.3 mm responsible for disease in freshwater salmonids, cyprinids etc (Austin and Austin, 2012a). The furunculosis-causing pathogen thrives in warm temperatures, but hibernates in cooler ones. While furunculosis is more common when water temperatures are higher it can still occur even when it is cold outside. The optimal temperature range for furunculosis is 12.8 to 21.1 °C, but A. salmonicida is always present at temperatures between 0.5 and 1.6 °C (Schachte, 2002; Perry et al., 2004). Even though furunculosis is a year-round problem, it produces the most severe losses in spring and fall at seawater farms. Salmonids can spread disease to one another through the water column, direct contact between fish, polluted water, and the help of animal vectors like birds and insects. However, non-salmonids can be harmed, if they eat sick fish (Dar et al., 2016; Khoo and Taylor, 2019). The crowded stocked varieties are especially vulnerable to infection. Stress factors including overcrowding, high temperatures, physical trauma, and contaminated water can all amplify furunculosis epidemics and fatality rates. It has been studied that pollution has a noteworthy part in the development of furunculosis. Industrial chemical farms, petroleum and petrochemical plants, paper mills, electric utilities, pesticides, sewage treatment plants, and overfishing are just a few of the many polluting activities that affect the environment (Kumari and Teacher, 2020).
2.7.2 Clinical signs/pathogenesis
A chronic or acute haemorrhagic septicaemia with widespread liquefactive necrosis is the hallmark of typical furunculosis. The clinical indications of an acute critical case in fish are a change/darkening in colour, red patches and furuncles/ulcers around the fins which indicates that the fish only has a few days to live (Figure 7). Chronically unwell fish, on the other hand, show symptoms including a swollen spleen and inflamed lower intestine (Austin and Austin, 2007; Dar et al., 2016, Dar et al., 2020). The type of infection, character of the disease, and the death rate are all related to the quality of the environmental factors that are further influenced by the host's age and natural immunity of the organism for the infection. Fingerlings are most frequently affected by acute infections, which can cause them to turn darker and eventually die without exhibiting obvious clinical symptoms of disease. There may only be a very minor exophthalmia visible on the affected fish. Young and adult fish that blacken in colour and bleed at fin base and within their mouths frequently have acute diseases. Affected fish may have internal haemorrhages visible in their heart, viscera, and abdomen walls (Izadi and Vajargah, 2022). The spleen enlarges, and the liver may show localised necrosis of parenchymatous tissue or subcapsular haemorrhages. Affected fish may swim erratically, become lethargic and stop feeding. As a result, there is typically no food in the stomach and intestines, and the lumen may comprise blood, mucus, and degenerated epithelial cells. Large sized fish have become more susceptible to the condition or some species with higher inborn resistance to infection by A. salmonicida, the chronic form of furunculosis typically manifests itself. The dermis may have one or more furuncle-like lesions, and ulcers may penetrate deeply into the musculature. Salmonids that have been infected for a long-time exhibit peritonitis and overall visceral congestion within. The liver and pylorus may bleed, and the kidneys may be soft or friable (Cipriano and Bullock, 2001). Gill lamellae fusion, epithelial cell death and eosinophilic inflammation are all signs of this disease at a microscopic level. Collapsing of the epithelial lining of the renal tubes and intestines, as well as the development of bacterial colonies in various tissues, are additional significant signs of the disease (Dar et al., 2020).
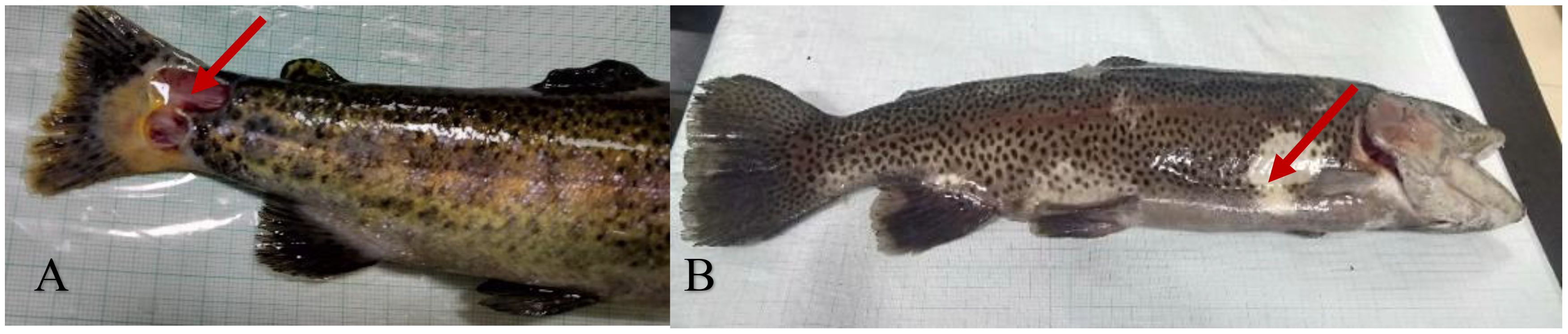
Figure 7. Rainbow trout with furunculosis, showing deep ulcerative skin lesions (A) and profound body discolouration (B).
2.7.3 Diagnosis
Although A. salmonicida sub spp. salmonicida can be recovered in standard microbiological media, it usually takes more than 48 hours for the distinctive brown coloured colonies to form. It may be isolated from bodily fluids, wounds, and even whole organs. Within 72 hours of incubation, the diameter of the colonies can vary from 0.5 to 3.0 mm, and the optimum growth temperature is 18 °C to 22 °C. The diagnosis of furunculosis has been approached from a number of different angles (Austin and Austin, 2012a). A pre-enrichment of the samples in culture broth is advised for the initial recovery from fish tissues, particularly in the case of carrier fish. It has been shown that the mucus of asymptomatic fish can be used to non-lethally detect the presence of A. salmonicida (Cipriano et al., 1994) While, the use of selective medium Coomassie Brilliant Blue (CBB) agar is advised to recover A. salmonicida from mucus samples where, mixed populations of bacterial species typically exist (Cipriano et al., 1992). Over the past few decades, tremendous progress has been made in the field of rapid diagnostics, which has had a major impact on the ability to correctly identify bacteria. Bioassays that measure the immunology of A. salmonicida, in addition to genetic-based assays, have been developed over the past few decades. Nonetheless, current investigations have yielded a number of methods for clinical diagnosis of furunculosis that do not use the culture of bacteria for the identification of A. salmonicida. These methods can be broadly divided as immune based techniques and genome-based techniques (Austin, 2019). Although salmonids are the primary hosts, furunculosis can also infect a widespread diversity of animals in a varied range of environments (Hofer et al., 2023). It is generally accepted that A. salmonicida must be treated as an omnipresent yet insidious disease. As a result, aqua culturists, fish growers, and resource managers need to be aware of the dangers it poses. Indeed, host, pathogen, and environment all play important roles in the conceptualization of the development of the disease (Menanteau-Ledouble, 2016) A description of the diagnostic parameters of the furunculosis disease is given in Table 8.
2.7.4 Treatment/control
Eliminating furunculosis is crucial for maintaining healthy fish populations. The anadromous fish population spreads disease to fish farms by entering their water supply. Such factors are difficult to prevent, yet under the managers' control (Cipriano and Bullock, 2001) The most effective method of addressing the major possible contamination sources and preventing sickness is to educate the workforce. The presence of A. salmonicida in the water should be monitored regularly as part of a comprehensive management plan. The staff must remain vigilant in the event of such a scenario. Only fertilised eggs or A. salmonicida free fish stocks should be sent to hatcheries and culture stations. Disinfecting the culture stations before stocking, removing sources of stress, segregating fish by age, using water that has not encountered wild or cultured fish, removing infected fish beforehand, and vaccinations are all effective preventative methods. It is imperative to quarantine any infected fish before the start of treatment (Weis et al., 2017). Adherence to biosecurity measures is crucial in preventing the spread of deadly fish infections. Although numerous furunculosis bacterins have been developed and commercialised during the 1980s, immersion or oral route and their usefulness has been questioned because of the deficiency of repeating outcomes plus very short protection time. Protective efficacy from vaccinations adjuvanted with mineral oil in salmonids has been reported to be highest. However, vaccination may result in granulomatous lesions, adhesions of the viscera and decrease weight gain. To circumvent these problems, new non-mineral oil-adjuvanted vaccinations have been lately produced and are presently available in the market for commercial use (Toranzo et al., 2005; Hoare et al., 2019).
2.8 Photobacteriosis or pasteurellosis
2.8.1 Epidemiology
The gram-negative, halophilic bacteria Photobacterium damselae sub spp. piscicida, is the causative agent of photobacteriosis, also known as fish pasteurellosis (Osorio et al., 2000). Owing to its extensive dispersal, high death rate and capacity to infect a wide variety of hosts, photobacteriosis is among the most concerning bacterial infections affecting aquaculture globally (Barnes et al., 2005). Besides, the disease is also known to affect a large number of marine species including Yellowtail, Seriola quinqueradiata, gilthead sea bream, Sparus aurata, sea bass, Dicentrarchus labrax, and sole, Solea senegalensis and Solea solea in Europe, striped bass, Morone saxatilis, white perch, Morone americana and hybrid striped bass (cross between white bass, M. chrysops and striped bass, M. saxatilis in America (Romalde, 2002; Wang et al., 2013). Fish pasteurellosis was formerly known as pseudotuberculosis because of the presence of white nodules found in the spleen and kidneys of infected fish. In general, water temperatures between 18 and 20 °C are fatal. Below this temperature range, fish can remain latently infected and serve as reservoirs of infection (Magariños et al., 2001). Different ages of fish are being uniquely vulnerable to the sickness. Acute photobacteriosis infection can cause 90–100% mortality in juvenile sea bream, but fish of above 50 g are more resistant because neutrophils and macrophages are better able to phagocytose and kill the bacteria (Santos et al., 2022). Acute form of photobacteriosis are both caused by bacteria that live in various tissues and inside of phagocytes. In acute phase, the liver, spleen and kidney all show signs of multifocal necrosis and bacteria populate phagocytes, capillaries and interstitial spaces with abundance. White tubercles of 0.3–0.5 mm in diameter are diagnostic of chronic diseases in the internal organs (Andreoni and Magnani, 2014). The behavioural symptoms of photobacteriosis in fish are highly linked upon the type of subspecies involved during infection. Fish infected with P. damselae sub spp. piscicida develop acute septicemia, become restless and float lethargically at the surface before eventually sinking to the bottom and die. Symptoms may include rapid breathing and inability to maintain equilibrium (Hawke, 2012). However, the behavioural symptoms of P. damselae sub spp. damselae infection in fish have not been well characterised. Diseased Australian snapper, Pagrus auratus float on the surface in lateral recumbency for several hours before they expire (Stephens et al., 2006). Acute bacterial septicemia is the hallmark of photobacteriosis induced by P. damselae sub spp. piscicida. It has been reported that when fish are kept at temperatures that are too high or too low, or when antibiotics are used, the sickness almost never becomes chronic (Thune et al., 1993; Hawke, 2012). Regardless of the species, relatively little gross pathology has also been reported in the past in the acute form (Toranzo et al., 1991). Affected striped bass and hybrid striped bass look normal other than having darker than normal pigmentation, pale gills and petechiae in the opercular region. The sole externally apparent gross clinical symptom is a spleen that is enlarged and friable. Except for the liver, which may be slightly mottled, and the kidney, which may be haemorrhagic, all other organs and physical features appear normal (Gupta et al., 2013). In addition to oedema, yellowtail display signs of dysregulated pigmentation and fluid accumulation. Bleeding at the operculum and fin base is minimal and reported in infected white perch. Some sick gilthead seabream shows visible clinical indications like bleeding around the head and opercular region, but this is quite uncommon. Disease persistence can look different depending on host species, environmental conditions, and the use of antibiotics in feed. An enlarged spleen and kidney may be accompanied by little white miliary lesions in striped bass and white perch. Antibiotic treatment of hybrid striped bass in captivity also result in similar lesions (Aswal et al., 2020). Yellowtails can develop chronic lesions that resemble granulomas and measure between 1 - 2 millimetres in diameter. These lesions are made up of clusters of the causative bacterium, epithelial cells, and fibroblasts. Japanese yellowtail suffers from a disease sometimes known as “pseudotuberculosis” because the lesions superficially mimic granulomas (Hawke, 2012).
2.8.2 Clinical signs/pathogenesis
In the early stages of an infection, the capability to stick to surfaces and invade surrounding tissue is crucial for the disease to develop successfully (Figure 8) (Romalde, 2002). In contrast to its low to moderate adhesion and invasion in fish cell lines, P. damselae sub spp. piscicida has been established to have a high binding capacity to fish intestines (Magariños et al., 1996; Andreoni and Magnani, 2014). Internalization of the bacteria happens via an actin microfilament-dependent mechanism and adherence appears to be mediated by a protein or glycoprotein receptor of the bacterial cell surface. Although adhesion and contact with host cell receptors and virulence factors are well known to contribute to the invasion of fish non-phagocytic cells however, the precise nature of these processes is not yet understood (Acosta et al., 2009; Andreoni and Magnani, 2014). P. damselae sub spp. piscicida has multiple virulence mechanisms that have been characterised. Fish mortality is increased and resistance to serum death is conferred by the polysaccharide capsular material of the bacteria hence playing a significant role in the pathogenicity of the bacterium (Magariños et al., 1996; Baseggio et al., 2022). Pathogens that are able to live inside of cells are likely to be protected from both specific and non-specific host immune responses and antibiotic-based antimicrobial defences (Magariños et al., 1996). Damage to infected cells, the subsequent release of microbes and the invasion of neighbouring cells may all be attributable to extracellular products having phospholipase, cytotoxic and haemolytic activities. In particular, the plasmid-encoded apoptosis-inducing protein of 56 kDa (AIP56) is an exotoxin that is secreted in high amounts by virulent strains of P. damselae subsp. piscicida and is responsible for apoptogenic activity against sea bass macrophages and neutrophils during acute fish photobacteriosis (Do Vale et al., 2005; Silva et al., 2010). In order to effectively manage and control fish photobacteriosis epidemics in aquaculture, a rapid diagnosis is required. Standard microbiological procedures, based on the growing and isolation of pathogens are typically used for disease diagnosis. To fully define the bacterium and distinguish between the piscicida and damselae sub species of P. damselae, more biochemical and serological confirmation is also required (Andreoni and Magnani, 2014).
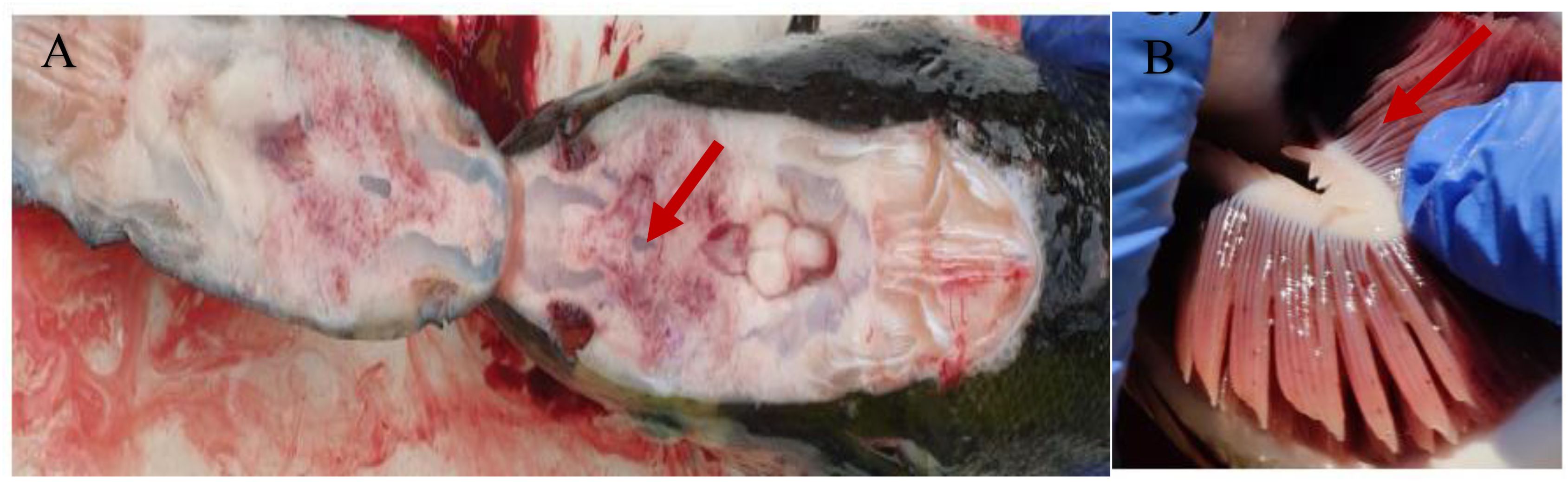
Figure 8. Atlantic salmon with photobacteriosis, showing petechial bleeding in the cranium (A) and petechial bleeding on the gill filaments (B). Source: picture courtesy Strøm and Nilsen (2021).
2.8.3 Diagnosis
In recent years, molecular approaches have been established to enable a precise and explicit identification of P. damselae subsp. piscicida and a speedy diagnosis of photobacteriosis, thereby overcoming the problem of time-consuming and arduous procedures. The difficulty is that identifying sequences helpful for constructing a subspecies-specific approach is made more difficult by the high degree of similarity between the two subspecies at the DNA level (Romalde, 2002). Although rRNA sequences have been considered for this purpose, high levels of similarity (>99%) have been found between the two P. damselae sub spp in 16S, 23S and 5S regions, as well as the intergenic spacer regions (98–99.5%). In addition, the latter are not useful for diagnosis because of their mosaic-like nature. Using the 16S sequences, only a PCR-based approach at the species level has been established. To produce PCR products, universal primers to 16S rRNA are available. By sequencing the PCR products and comparing them to the sequences stored in GenBank via NCBI BLAST, it is possible to verify that the results are really from P. damselae (Zappulli et al., 2005). A description of the diagnostic parameters of the photobacteriosis disease is given in Table 9.
2.8.4 Treatment
It has been reported that in fish farming, antibiotics have been considered as the first line of defence against photobacteriosis outbreaks, but after just a few years, the pathogen developed resistance to different antibiotics. It has been proven that P. damselae subsp. piscicida has many transposable genetic elements (R plasmids) bearing genes for resistance against kanamycin, sulphonamide, tetracycline (Kim and Aoki, 1993; Del Castillo et al., 2013), ampicillin (Morii et al., 2004), chloramphenicol (Morii et al., 2003; Del Castillo et al., 2013), florfenicol (Kim and Aoki, 1996) and erythromycin (Morii and Ishikawa, 2012). Several strains obtained in Japan and the United States show variations in the geographic distribution of multidrug transferrable components (Kim et al., 2008). Chemotherapy has also been tested to cure this disease; however, it shows less successful results due to the intracellular parasitism nature of P. damselae subspp. piscicida in macrophages.
To protect fish from P. damselae subspp. piscicida, a number of commercial vaccinations have been developed, but their effectiveness varies greatly depending on a variety of variables, including the type and size of the fish, the formulation of the vaccine, and the use of immunostimulants (Su and Chen, 2022). The effectiveness of the vaccination was shown in 50-day-old gilthead seabream larvae the commercially available licenced bacterin (DI vaccine) patented by the University of Santiago (Spain). A vaccination regimen that includes a first dip immunisation at the larval stage and a booster vaccination when fish reach a size of around 1–2 g is indicated to prevent substantial economic losses that can result from pasteurellosis epidemics, which typically affect fingerlings of 10 to 30 g. Different stable siderophore deficient and aro-A deletion mutant strains that were recently created via an allelic replacement technique were experimentally tested, and the results showed that they were suitable candidates for live vaccines (Thune et al., 2003; Chin and Anderson, 2024). But due to the very limited use of recombinant DNA technology and biotechnological techniques in the development of fish bacterial vaccines, effective preventative treatments against fish pasteurellosis are still unavailable.
2.9 Mycobacteriosis (fish tuberculosis)
2.9.1 Epidemiology
Mycobacterium marinum, is regarded as the primary pathogen responsible for causing fish tuberculosis. Besides other mycobacterium species allied with tubercle granulomas in fish have been identified such as M. fortuitum, M. chelonae, M. smegmatis, M. abscessus, M. neonarum, M. scrofulaceum and M. poriferae (Diamant et al., 2000; Aubry et al., 2017). The human population is also vulnerable to infection from any of these species (Decostere et al., 2004). Although Mycobacterium spp. are ubiquitious, tropical and subtropical areas seem to have higher concentrations of them. These organisms have also been found in diversity of aquatic environments, including fresh, brackish, and salt water (Prearo et al., 2004; Chang and Whipps, 2015) as well as soil, biofilms, and sediments (Beran et al., 2006) including the sediment in decorative aquaria and fish breeding facilities. This disease affects a wide variety of aquatic creatures, including amphibians, oysters, and more than 150 species of marine and freshwater fish as hosts (Hashish et al., 2018). Besides, 28 mycobacterial positive infections have been identified in 3880 different species of reptiles, birds and mammals (Delghandi et al., 2020b).
Mycobacteriosis is a typically chronic disease, and is known to affect the aquarium fish more severely as they are frequently set aside for longer time periods compared with fish raised for commercial purposes. Mycobacterial organisms flourish in warm water with decreased dissolved oxygen levels, acidic pH, higher concentration of soluble zinc, fulvic acid and high humic acid. In intensive aquaculture systems, circumstances including low dissolved oxygen, low pH, and an organically rich environment are common place thus paving the way for this infection to occur (Slany et al., 2014). Reducing the amount of time, it takes for host fish to become sick after being exposed is crucial in disease prevention. It is more likely that fish in the system will become infected if conditions are ideal for the organism's proliferation. Mycobacteriosis manifests internally in fish in a numerous ways depending on the species, but the most common manifestations are nodules (granulomas) that range in colour from grey to white in the spleen, kidneys, and liver. Scale loss and deep haemorrhagic sores on the skin and muscles are identified as external symptoms of this disease (Delghandi et al., 2020b).
Actively infecting mycobacterial agents are the most serious threat to aquaculture. Fish with the infection shed the organism from its digestive system and from lesions on its skin and gills. The infected fish discharges harmful bacteria into the environment as they die and decay. Fish can contract an infection through the contact with communicable material or through ingestion of infected tissue (Mutoji, 2011). The best example of this may be seen in the salmon industry, which faced a widespread problem with mycobacteriosis because it fed unpasteurized fish offal to its fish at an early stage in its growth. However, no cases of this disease have been recorded in salmon hatcheries after the same feeding was stopped. Any dead animals in a collection or culture system should be disposed of to prevent the spread of mycobacteriosis (Delghandi et al., 2020a). During mycobacteriosis vertical transmission from mother to offspring also happens in addition to horizontal transmission between fish species (Gauthier and Rhodes, 2009). Although it has been well established that the organism is present in gonadal tissue, the mechanisms by which it is transmitted vertically in live fish are more understood than those by which it is transmitted in egg-laying species. In the absence of contrary evidence, it is prudent to presume vertical transmission, hence it is suggested that mycobacterium-infected brood stock be eradicated (Francis- Floyd, 2011).
2.9.2 Clinical signs/pathogenesis
The disease begins with the pathogens attaching themselves to the base of the fins or to skin sores, as is frequently the case with other bacterial diseases in fish. Furthermore, degraded fins and tail rot are frequently been reported among the early visible symptoms. Additionally, non-specific exterior symptoms that are relatively alike to those of other diseases, such as enlarged abdomen, scale loss, pigmentary changes and inflammatory cutaneous necrosis, as well as a heavy mucus coating on the body's surface have also been reported. Besides these, shallow irregular ulcers and external red lesions on the lateral line have also been seen (Figure 9) (Swaim et al., 2006; Evely et al., 2011; Keller et al., 2018). Further, exophthalmia, blindness, ascites, pale gills, aberrant behaviour, including lethargy and loss of appetite, as well as skeletal malformations such as spine curvature or stunted growth, can all be seen in the diseased fish (Marzouk et al., 2009; Wiens, 2011). Mycobacterial organisms can be discovered in most tissues and organs, including the eyes, gills, visceral organs and muscles after infection. The infection spreads throughout the entire fish body via the circulatory and lymphatic systems. The spleen, kidneys and liver can swell and develop characteristic grey or white nodules as visible internal indications of infection (Chinabut, 1999). In rare cases, elevated bacterial loads are linked to the onset of acute disease (Whipps et al., 2007). In case of wild species, mycobacteriosis does not usually cause widespread mortality, but it has been linked to substantial economic losses in the aquaculture industry (Hedrick et al., 1987). The typical histological sign of piscine mycobacteriosis is granulomatous inflammation (Noga, 2000). Concentric layers of epithelioid cells make up granulomas, which are discrete spherical lesions. Multiple organs and tissues may develop granulomas as a result of the systemic nature of piscine mycobacteriosis. Granulomas range from highly structured lesions with dense epithelioid layers to poorly organised inflammation with low epithelioid cell production, both in terms of size and structural organisation. In contrast to chronic infections, which were characterised by the presence of granulomas in various internal organs and the ability of infected fish to survive for 4 to 8 weeks, acute disease is characterised by a rapid progression of the infection, uncontrolled growth of the pathogen, and death of infected fish within 16 days (Lehane and Rawlln, 2000; Van Der Sar et al., 2004; Delghandi et al., 2020a). There are currently no commonly used therapies for fish mycobacteriosis. Humans infected with M. tuberculosis and opportunistic mycobacteria often require lengthy treatment, lasting up to 24 months, and a variety of medications. Rarely are fish isolates tested for antibiotic susceptibility, and it appears that resistance is strongly been influenced by the species and strain that is infected. It has been demonstrated that rifampicin, streptomycin and erythromycin have some activity against this pathogen in fish. With few exceptions, nothing is known about the pharmacokinetics of anti-mycobacterial drugs in fish (Brown et al., 1990). Although it has been discovered that M. marinum is quickly eliminated from water by employing ethanol, benzyl-4-chlorophenol-phenylphenol (Lysol) and sodium chlorite, other widely used disinfectants like N-alkyl dimethyl benzyl ammonium chloride (Roccal-D, Micronex) and potassium peroxymonosulfate (Virkon-S), are ineffective even after prolonged contact times. As long as the contact period was greater than 10 minutes, sodium hypochlorite was a reliable sterilising agent (Mainous and Smith, 2005).
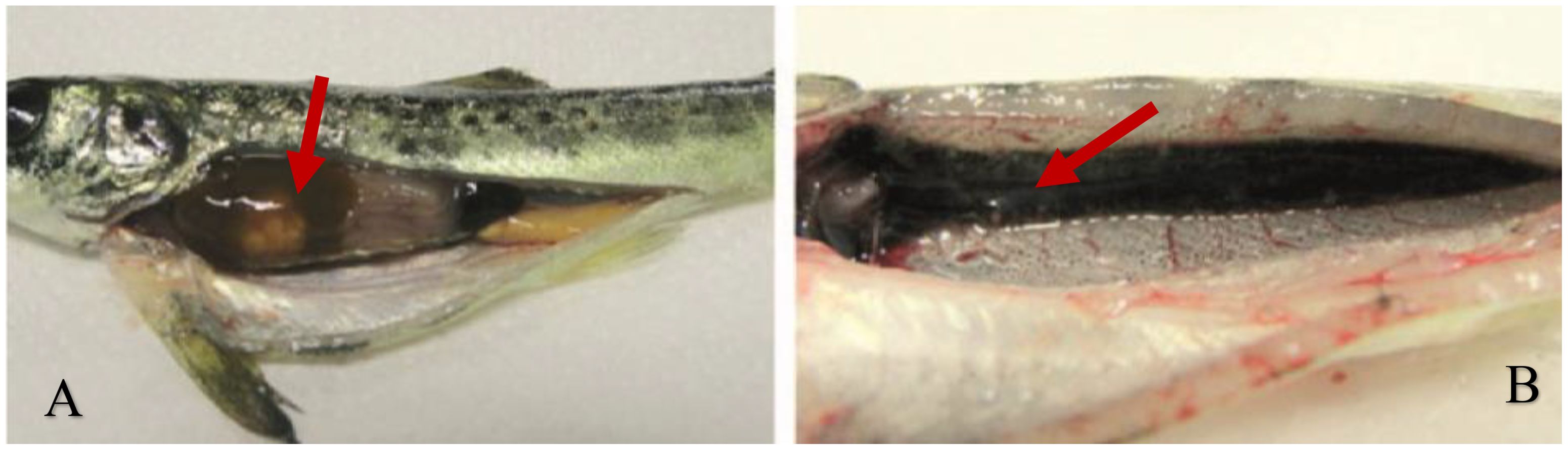
Figure 9. Atlantic salmon with mycobacteriosis, showing multiple whitish nodules in the liver (A) and nodules in the kidney (B). Source: picture courtesy Aro et al. (2014).
2.9.3 Diagnosis
The Ziehl-Neelsen acid-fast stain, which is based on the resistance, or “acid-fastness,” of the mycobacterial cell wall to acid-alcohol decolorization following staining with carbolfuchsin, is the best way to see mycobacteria in tissue sections. On non-selective medium, mycobacteria are meticulous and slow-growing, and they are likely to be outcompeted by organisms that develop more quickly. These media include growth petragnani agar, dorset egg media, middlebrook 7H10 lowenstein-Jensen (solid medium), and middlebrook 7H9 (broth medium). In addition, Mycobacterium can tolerate treatment with basic and acidic chemicals as well as other mixtures including benzalkonium chloride and hypochlorite (Hashish et al., 2018) which have been utilised to isolate Mycobacterium from a microbial background. Several selective liquid and agar media have been developed for the efficient cultivation of mycobacteria (Chinabut, 1999; Rhodes et al., 2004). Mycobacteria can be isolated from non-mycobacterial pollutants by plating on solid medium. Mycobacterial densities can be measured by using the spread-plating of homogenates from weighted tissue and dilutions thereof (Talaat et al., 1999; Rhodes et al., 2004). Colony morphology can also show if an infection is caused by both mycobacteria and something else (Rhodes et al., 2004). Pathogens should ideally be cultured at room temperature or at the ambient temperature similar to the conditions from where fishes are collected. While some bacteria, like M. marinum can develop at high temperature (30 °C) others, like M. shottsii and M. pseudoshottsii can grow best at 23 °C and poorly or not at all above 30 °C (Rhodes et al., 2003, Rhodes et al., 2005). Besides using specific media as well as phenotypical tests identification should be confirmed through 16S ribosomal gene DNA sequencing (Liu et al., 2024). Mycobacteria can be detected from fish tissues and blood by using previously reported polymerase chain reaction (PCR) methods that employ the 16S rDNA as the target gene and are followed by restriction enzyme analysis of the amplified fragment (Rodriíguez-Nava et al., 2006). This approach has strong potential to serve as a valuable, non-destructive means of screening brood stock. A description of the diagnostic parameters of the mycobacteriosis disease is given in Table 10.
2.9.4 Treatment/control
For the prevention and management of this disease, vaccination against fish mycobacteriosis would be extremely helpful. The Bacillus Calmette and Guerin (BCG) was found to stimulate the expression of a number of immune genes including IL-1, IL-6, IFN-, and TNF. In some fish species this vaccine was linked to improved survival after challenge, though surprisingly, no antibodies were reported by the authors.
Author contributions
IA: Conceptualization, Supervision, Writing – review & editing. SI: Data curation, Writing – original draft, Writing – review & editing. SS: Supervision, Writing – review & editing.
Funding
The author(s) declare that no financial support was received for the research and/or publication of this article.
Conflict of interest
The authors declare that the research was conducted in the absence of any commercial or financial relationships that could be construed as a potential conflict of interest.
The author(s) declared that they were an editorial board member of Frontiers, at the time of submission. This had no impact on the peer review process and the final decision.
Generative AI statement
The author(s) declare that no Generative AI was used in the creation of this manuscript.
Publisher’s note
All claims expressed in this article are solely those of the authors and do not necessarily represent those of their affiliated organizations, or those of the publisher, the editors and the reviewers. Any product that may be evaluated in this article, or claim that may be made by its manufacturer, is not guaranteed or endorsed by the publisher.
References
Acosta F., Vivas J., Padilla D., Vega J., Bravo J., Grasso V., et al. (2009). Invasion and survival of Photobacterium damselae subsp. piscicida in non-phagocytic cells of gilthead sea bream, Sparus aurata L. J. Fish Dis. 32, 535–541. doi: 10.1111/j.1365-2761.2009.01023.x
Adams A., Thompson K. D. (2006). Biotechnology offers revolution to fish health management. Trends Biotechnol. 24, 201–205. doi: 10.1016/j.tibtech.2006.03.004
Alderman D. J., Hastings T. S. (1998). Antibiotic use in aquaculture: development of antibiotic resistance–potential for consumer health risks. Int. J. Food Sci. &Technology 33, 139–155. doi: 10.1046/j.1365-2621.1998.3320139.x
Altinok I., Grizzle J. M., Liu Z. (2001). Detection of Yersinia ruckeri in rainbow trout blood by use of the polymerase chain reaction. Dis. Aquat. Organisms 44, 29–34. doi: 10.3354/dao044029
Amend D. F., Ross A. J. (1970). Experimental control of columnaris disease with a new nitrofuran drug, P-7138. Progressive Fish-Culturist 32, 19–25. doi: 10.1577/1548-8640(1970)32[19:ECOCDW]2.0.CO;2
Amita K., Hoshino M., Honma T., Wakabayashi H. (2000). An investigation on the distribution of Flavobacterium psychrophilum in the Umikawa River. Fish Pathol. 35, 193–197. doi: 10.3147/jsfp.35.193
Anacker R. L., Ordal E. J. (1959). Studies on the myxobacterium Chondrococcus columnaris: II. Bacteriocins. J. Bacteriology 78, 33–40. doi: 10.1128/jb.78.1.33-40.1959
Andreoni F., Magnani M. (2014). Photobacteriosis: prevention and diagnosis. J. Immunol. Res. 1. doi: 10.1155/2014/793817
Arias C. R., LaFrentz S., Cai W., Olivares-Fuster O. (2012). Adaptive response to starvation in the fish pathogen Flavobacterium columnare: cell viability and ultrastructural changes. BMC Microbiol. 12, 1–12. doi: 10.1186%2F1471-2180-12-266
Arias C. R., Olivares-Fuster O., Hayden K., Shoemaker C. A., Grizzle J. M., Klesius P. H. (2007). First report of Yersinia ruckeri biotype 2 in the USA. J. Aquat. Anim. Health 19, 35–40. doi: 10.1577/H06-011.1
Aro L., Correa K., Martínez A., Ildefonso R. Y. J. M., Yanez J. M. (2014). Characterization of Mycobacterium salmoniphilum as causal agent of mycobacteriosis in Atlantic salmon, Salmo salar L., from a freshwater recirculation system. J. Fish Dis. 37, 341–348. doi: 10.1111/jfd.12108
Assefa A., Abunna F. (2018). Maintenance of fish health in aquaculture: review of epidemiological approaches for prevention and control of infectious disease of fish. Veterinary Med. Int. 1. doi: 10.1155/2018/5432497
Aswal M., Garg A., Singhal N., Kumar M. (2020). Comparative in-silico proteomic analysis discerns potential granuloma proteins of Yersinia pseudotuberculosis. Sci. Rep. 10, 3036. doi: 10.1038/s41598-020-59924-1
Aubry A., Mougari F., Reibel F., Cambau E. (2017). Mycobacterium marinum. Microbiol. Spectr. 5, 735–752. doi: 10.1128/microbiolspec.tnmi7-0038-2016
Aung M. M., Chang Y. S. (2014). Traceability in a food supply chain: Safety and quality perspectives. Food Control 39, 172–184. doi: 10.1016/j.foodcont.2013.11.007
Austin B. (2019). “Methods for the diagnosis of bacterial fish diseases,” in Marine life science & Technology, vol. 1, 41–49. Springer Nature, Ocean University of China. doi: 10.1007/s42995-019-00002-5
Austin B., Austin D. A. (2007). Bacterial fish pathogens: diseases of farmed and wild fish. 5th ed (Chichester: Springer publishers). doi: 10.1007/978-1-4020-6069-4
Austin B., Austin D. A. (2012a). Bacterial fish pathogens: diseases of farmed and wild fish. 5th ed (Chichester: Springer publishers). doi: 10.1007/978-94-007-4884-2
Austin B., Austin D. A. (2012b). “Aeromonadaceae representatives (motile aeromonads),” in Bacterial fish pathogens: disease of farmed and wild fish, 119–146. Springer, Dordrecht. doi: 10.1007/978-94-007-4884-2
Austin B., Austin D. A. (2016). Bacterial fish pathogens, diseases of farmed and wild fish. 2nd Ed (Chichester, UK: Springer, Cham), 732. doi: 10.1007/978-3-319-32674-0
Austin B., Austin D. A., Munn C. B. (2007). Bacterial fish pathogens: disease of farmed and wild fish Vol. 26 (Dordrecht: The Netherlands: Springer), 552.
Austin D. A., Robertson P. A. W., Austin B. (2003). Recovery of a new biogroup of Yersinia ruckeri from diseased rainbow trout (Oncorhynchus mykiss, Walbaum). Systematic Appl. Microbiol. 26, 127–131. doi: 10.1078/072320203322337416
Bagum N., Monir M. S., Khan M. H. (2013). Present status of fish diseases and economic losses due to incidence of disease in rural freshwater aquaculture of Bangladesh. J. Innovative Dev. Strategy 7, 48–53.
Balfry S. K., Albright L. J., Evelyn T. P. T. (1996). Horizontal transfer of Renibacterium salmoninarum among farmed salmonids via the fecal-oral route. Dis. Aquat. Organisms 25, 63–69. doi: 10.3354/dao025063
Barber I. (2007). Parasites, behaviour and welfare in fish. Appl. Anim. Behav. Sci. 104, 251–264. doi: 10.1016/j.applanim.2006.09.005
Barnes A. C., Dos Santos N. M., Ellis A. E. (2005). Update on bacterial vaccines: Photobacterium damselae subsp. piscicida. Developments Biologicals 121, 75–84. Available online: https://pubmed.ncbi.nlm.nih.gov/15962471/.
Baseggio L., Rudenko O., Engelstädter J., Barnes A. C. (2022). The evolution of a specialized, highly virulent fish pathogen through gene loss and acquisition of host-specific survival mechanisms. Appl. Environ. Microbiol. 88, e00222–e00222. doi: 10.1128/aem.00222-22
Bastardo A., Ravelo C., Romalde J. L. (2012). Highly sensitive detection and quantification of the pathogen Yersinia ruckeri in fish tissues by using real-time PCR. Appl. Microbiol. Biotechnol. 96, 511–520. doi: 10.1007/s00253-012-4328-1
Bayliss S. C., Verner-Jeffreys D. W., Ryder D., Suarez R., Ramirez R., Romero J., et al. (2018). Genomic epidemiology of the commercially important pathogen Renibacterium salmoninarum within the Chilean salmon industry. Microbial Genomics 4. doi: 10.1099/mgen.0.000201
Beran V., Matlova L., Dvorska L., Svastova P., Pavlik I. (2006). Distribution of mycobacteria in clinically healthy ornamental fish and their aquarium environment. J. Fish Dis. 29, 383–393. doi: 10.1111/j.1365-2761.2006.00729.x
Bercovier H., Ghittino C., Eldar A. (1997). Immunization with bacterial antigens: infections with streptococci and related organisms. Developments Biol. Standardization 90, 153–160. Available online: https://pubmed.ncbi.nlm.nih.gov/9270844/.
Bernardet J. F., Bowman J. P. (2006). The genus flavobacterium. Prokaryotes 7, 481–531. doi: 10.1007/0-387-30747-8_17
Bethke J., Quezada J., Poblete-Morales M., Irgang R., Yáňez A. (2017). Biochemical, serological, and genetic characterisation of Renibacterium salmoninarum isolates recovered from salmonids in Chile. Available online at: http://repositorio.unab.cl/xmlui/handle/ria/5230 (Accessed March 15, 2024).
Bekker A., Hugo C., Albertyn J., Boucher C. E., Bragg R. R. (2011). Pathogenic Gram-positive cocci in South African rainbow trout, Oncorhynchus mykiss (Walbaum). J. Fish Diseases 34 (6).
Boerlage A. S., Elghafghuf A., Stryhn H., Sanchez J., Hammell K. L. (2018). Risk factors associated with time to first clinical case of Bacterial Kidney Disease (BKD) in farmed Atlantic Salmon (Salmo salar L.) in New Brunswick, Canada. Prev. Veterinary Med. 149, 98–106. doi: 10.1016/j.prevetmed.2017.11.014
Boerlage A. S., Stryhn H., Sanchez J., Hammell K. L. (2017). Case definition for clinical and subclinical bacterial kidney disease (BKD) in Atlantic Salmon (Salmo salar L.) in New Brunswick, Canada. J. Fish Dis. 40, 395–409. doi: 10.1111/jfd.1252
BOF (Bureau of Fisheries under Ministry of Agriculture and Rural Affairs of China), NFTEC (National Fisheries Technology Extension Center), CSF (China Society of Fisheries) (2019). Aquatic animal health in China (Beijing, China: China Agriculture Press).
Bondad-Reantaso M. G., Fejzic N., MacKinnon B., Huchzermeyer D., Seric-Haracic S., Mardones F. O., et al. (2021). A 12-point checklist for surveillance of diseases of aquatic organisms: a novel approach to assist multidisciplinary teams in developing countries. Rev. Aquaculture 13, 1469–1487. doi: 10.1111/raq.12530
Bondad-Reantaso M. G., Garrido-Gamarro E., McGladdery S. E. (2018). “Climate change-driven hazards on food safety and aquatic animal health,” in Impacts of climate change on fisheries and aquaculture. Food and Agriculture Organization of the United Nations, Rome, 2018, 517–533.
Bondad-Reantaso M. G., Subasinghe R. P., Arthur J. R., Ogawa K., Chinabut S., Adlard R., et al. (2005). Disease and health management in Asian aquaculture. Veterinary Parasitol. 132, 249–272. doi: 10.1016/j.vetpar.2005.07.005
Brown L. L., Albright L. J., Evelyn T. P. T. (1990). Control of vertical transmission of Renibacterium salmoninarum by injection of antibiotics into maturing female coho salmon Oncorhynchus kisutch. Dis. Aquat. Organisms 9, 127–131. doi: 10.3354/dao009127
Brugere C., Onuigbo D. M., Morgan K. L. (2017). People matter in animal disease surveillance: challenges and opportunities for the aquaculture sector. Aquaculture 467, 158–169. doi: 10.1016/j.aquaculture.2016.04.012
Buller N. B. (2004). “Biochemical identification tables,” in Bacteria from Fish and Other Aquatic Animals: a practical identification manual (CABI Publishing, Wallingford UK), 137–221.
Bullock G. L. (1972). Studies on selected myxobacteria pathogenic for fishes and on bacterial gill disease in hatchery-reared salmonids (Vol. 60) (US Department of the Interior, Fish and Wildlife Service, Bureau of Sport Fisheries and Wildlife, United States).
Bullock G. L. (1986). Columnaris disease of fishes (Vol. 72) (US Department of the Interior, Fish and Wildlife Service, Division of Fisheries and Wetlands Research, Washington).
Busch R. A., Lingg A. J. (1975). Establishment of an asymptomatic carrier state infection of enteric redmouth disease in rainbow trout (Salmo gairdneri). J. Fisheries Board Canada 32, 2429–2432. doi: 10.1139/f75-279
Cain K. D., Polinski M. P. (2014). “Infectious diseases of coldwater fish in fresh water,” in Diseases and disorders of finfish in cage culture (CABI, Wallingford UK), 60–113. doi: 10.1079/9781780642079.0060
Calvez S., Gantelet H., Blanc G., Douet D. G., Daniel P. (2014). Yersinia ruckeri biotypes 1 and 2 in France: presence and antibiotic susceptibility. Dis. Aquat. Organisms 109, 117–126. doi: 10.3354/dao02725
Chambers E., Barker G. (2006). Comparison of culture media for the isolation of Renibacterium salmoninarum from naturally infected rainbow trout (Oncorhynchus mykiss). Bull. Eur. Assoc. Fish Pathologists 26, 137.
Chang C. T., Whipps C. M. (2015). Activity of antibiotics against Mycobacterium species commonly found in laboratory zebrafish. J. Aquat. Anim. Health 27, 88–95. doi: 10.1080/08997659.2015.1007176
Chapela M. J., Ferreira M., Varela C., Arregui L., Garrido-Maestu A. (2018). Development of a multiplex real-time PCR method for early diagnosis of three bacterial diseases in fish: a real-case study in trout aquaculture. Aquaculture 496, 255–261. doi: 10.1016/J.AQUACULTURE.2018.07.003
Chin P., Anderson C. J. (2024). New insights on an old friend: AroA linked to iron-dependent outer membrane stability. mBio 15, e02799–e02724. doi: 10.1128/mbio.02799-24
Chinabut S. (1999). Fish disease and disorders: Viral, bacterial, and fungal infections (CAB International, Nosworthy Way, Wallingford, Oxfordshire, OX10 8DE, UK.), 319–340.
Chintagari S., Hazard N., Edwards G., Jadeja R., Janes M. (2018). Risks associated with fish and seafood. Preharvest Food Saf., 123–142. doi: 10.1128/microbiolspec.PFS-0013-2016
Chong C. M., Lee P. T., Rakus K., Wangkahart E. (2023). The epidemiologic triads in aquaculture: host, pathogen and environment. Front. Immunol. 14. doi: 10.3389/fimmu.2023.1305784
Cipriano R. C., Bullock G. L. (2001). “Furunculosis and other diseases caused by Aeromonas salmonicida,” (National Fish Health Research Laboratory, Charles Town, WV).
Cipriano R. C., Ford L. A., Schachte J. H., Petrie C. (1994). Evaluation of mucus as a valid site to isolate Aeromonas salmonicida among asymptomatic populations of lake trout (Salvelinus namaycush). Biomed. Lett. 49, 229–233.
Cipriano R. C., Ford L. A., Teska J. D., Hale L. E. (1992). Detection of Aeromonas salmonicida in the mucus of salmonid fishes. J. Aquat. Anim. Health 4, 114–118. doi: 10.1577/1548-8667(1992)004<0114:DOASIT>2.3.CO;2
Cipriano R. C., Ford L. A., Teska J. D., Schachte J. H., Petrie C., Novak B. M., et al. (1996). Use of non-lethal procedures to detect and monitor Aeromonas salmonicida in potentially endangered or threatened populations of migrating and post-spawning salmon. Dis. Aquat. Organisms 27, 233–236. doi: 10.3354/dao027233
Coquet L., Cosette P., Junter G. A., Beucher E., Saiter J. M., Jouenne T. (2002). Adhesion of Yersinia ruckeri to fish farm materials: influence of cell and material surface properties. Colloids surfaces B: Biointerfaces 26, 373–378. doi: 10.1016/S0927-7765(02)00023-1
Crumlish M., Diab A. M., George S., Ferguson H. W. (2007). Detection of the bacterium Flavobacterium psychrophilum from a natural infection in rainbow trout, Oncorhynchus mykiss (Walbaum), using formalin-fixed, wax-embedded fish tissues. J. Fish Dis. 30, 37–41. doi: 10.1111/j.1365-2761.2007.00779.x
Crump E. M., Perry M. B., Gale S., Crawford E., Kay W. W. (2004). Lipopolysaccharide O-antigen antibody-based detection of the fish pathogen Flavobacterium psychrophilum. Microbial Physiol. 6, 182–190. doi: 10.1159/000077249
Cunningham C. O. (2002). Molecular diagnosis of fish and shellfish diseases: present status and potential use in disease control. Aquaculture 206, 19–55. doi: 10.1016/S0044-8486(01)00864-X
Daoust P. Y., Ferguson H. W. (1983). Gill diseases of cultured salmonids in Ontario. Can. J. Comp. Med. 47, 358. Availble online: https://pmc.ncbi.nlm.nih.gov/articles/PMC1235953/.
Dar G. H., Bhat R. A., Kamili A. N., Chishti M. Z., Qadri H., Dar R., et al. (2020). “Correlation between pollution trends of freshwater bodies and bacterial disease of fish fauna,” in Fresh water pollution dynamics and remediation, 51–67. Springer, Singapore. doi: 10.1007/978-981-13-8277-2_4
Dar G. H., Dar S. A., Kamili A. N., Chishti M. Z., Ahmad F. (2016). Detection and characterization of potentially pathogenic Aeromonas sobria isolated from fish Hypophthalmichthys molitrix (Cypriniformes: Cyprinidae). Microbial Pathogenesis 91, 136–140. doi: 10.1016/j.micpath.2015.10.017
Darwish A. M., Farmer B. D., Hawke J. P. (2008). Improved method for determining antibiotic susceptibility of Flavobacterium columnare isolates by broth microdilution. J. Aquat. Anim. Health 20, 185–191. doi: 10.1577/H07-047.1
Darwish A. M., Ismaiel A. A., Newton J. C., Tang J. (2004). Identification of Flavobacterium columnare by a species-specific polymerase chain reaction and renaming of ATCC43622 strain to Flavobacterium johnsoniae. Mol. Cell. Probes 18, 421–427. doi: 10.1016/j.mcp.2004.07.002
Das B. K., Mishra S. S. (2014). Diseases in Freshwater aquaculture. Training Manual on Model training course on Preventive health management practices in freshwater aquaculture (Bhubaneswar, Odisha, India: ICAR-Central Institute of Freshwater aquaculture).
Davis H. S. (1926). A new gill disease of trout. Trans. Am. Fisheries Soc. 56, 156–160. doi: 10.1577/15488659(1926)56[156:ANGDOT]2.0.CO;2
Declercq A. M., Boyen F., Van Den Broeck W., Bossier P., Karsi A., Haesebrouck F., et al. (2013a). Antimicrobial susceptibility pattern of Flavobacterium columnare isolates collected worldwide from 17 fish species. J. Fish Dis. 36, 45–55. doi: 10.1111/j.1365-2761.2012.01410.x
Declercq A. M., Haesebrouck F., Van den Broeck W., Bossier P., Decostere A. (2013b). Columnaris disease in fish: a review with emphasis on bacterium-host interactions. Veterinary Res. 44, 1–17. doi: 10.1186/1297-9716-44-27
Declercq A. M., Chiers K., Haesebrouck F., Van Den Broeck W., Dewulf J., Cornelissen M., et al. (2015). Gill infection model for columnaris disease in common carp and rainbow trout. J. Aquat. Anim. Health 27, 1–11. doi: 10.1080/08997659.2014.953265
Decostere A., Hermans K., Haesebrouck F. (2004). Piscine mycobacteriosis: a literature review covering the agent and the disease it causes in fish and humans. Veterinary Microbiol. 99, 159–166. doi: 10.1016/j.vetmic.2003.07.011
Defoirdt T., Boon N., Sorgeloos P., Verstraete W., Bossier P. (2007). Alternatives to antibiotics to control bacterial infections: luminescent vibriosis in aquaculture as an example. Trends Biotechnol. 25, 472–479. doi: 10.1016/j.tibtech.2007.08.001
Del Castillo C. S., Jang H. B., Hikima J. I., Jung T. S., Morii H., Hirono I., et al (2013). Comparative analysis and distribution of pP9014, a novel drug resistance IncP-1 plasmid from Photobacterium damselae subsp. piscicida. Int. J. Antimicrobial Agents 42, 10–18. doi: 10.1016/j.ijantimicag.2013.02.027
Delghandi M. R., El-Matbouli M., Menanteau-Ledouble S. (2020a). Mycobacteriosis and infections with non-tuberculous mycobacteria in aquatic organisms: A review. Microorganisms 8, 1368. doi: 10.3390/microorganisms8091368
Delghandi M. R., El-Matbouli M., Menanteau-Ledouble S. (2020b). Renibacterium salmoninarum—the causative agent of bacterial kidney disease in salmonid fish. Pathogens 9, 845. doi: 10.3390/pathogens9100845
Delghandi M. R., Menanteau-Ledouble S., Waldner K., El-Matbouli M. (2020c). Renibacterium salmoninarum and Mycobacterium spp.: Two bacterial pathogens present at low levels in wild brown trout (Salmo trutta fario) populations in Austrian rivers. BMC Veterinary Res. 16, 1–12. doi: 10.1186/s12917-020-2260-7
Deshmukh S., Raida M. K., Dalsgaard I., Chettri J. K., Kania P. W., Buchmann K. (2012). Comparative protection of two different commercial vaccines against Yersinia ruckeri serotype O1 and biotype 2 in rainbow trout (Oncorhynchus mykiss). Veterinary Immunol. Immunopathology 145, 379–385. doi: 10.1016/j.vetimm.2011.12.014
Diamant A., Banet A., Ucko M., Colorni A., Knibb W., Kvitt H. (2000). Mycobacteriosis in wild rabbitfish Siganus rivulatus associated with cage farming in the Gulf of Eilat, Red Sea. Dis. Aquat. Organisms 39, 211–219. doi: 10.3354/dao039211
Didinen B. I., Yardimci B., Onuk E. E., Metin S., Yildirim P. (2014). Naturally Lactococcus garvieae infection in rainbow trout (Oncorhyncus mykiss Walbau): new histopathological observations, phenotypic and molecular identification. Rev. Med. Vet-Toulouse 165, 12–19.
Do Vale A., Silva M. T., dos Santos N. M., Nascimento D. S., Reis-Rodrigues P., Costa-Ramos C., et al. (2005). AIP56, a novel plasmid-encoded virulence factor of Photobacterium damselae subsp. piscicida with apoptogenic activity against sea bass macrophages and neutrophils. Mol. Microbiol. 58, 1025–1038. doi: 10.1111/j.1365-2958.2005.04893.x
Elliott D. G. (2017). “Renibacterium salmoninarum,” in Fish viruses and bacteria: pathobiology and protection (CABI, Wallingford UK), 286–297. doi: 10.1079/9781780647784.0286
Evans J. J., Klesius P. H., Shoemaker C. A. (2009). First isolation and characterization of Lactococcus garvieae from Brazilian Nile tilapia, Oreochromis niloticus (L.), and pintado, Pseudoplathystoma corruscans (Spix & Agassiz). J. Fish Dis. 32, 943–951. doi: 10.1111/j.1365-2761.2009.01075.x
Evely M. M., Donahue J. M., Sells S. F., Loynachan A. T. (2011). Ocular mycobacteriosis in a red-bellied piranha, Pygocentrus nattereri Kner. J. Fish Dis. 34, 323–326. doi: 10.1111/j.1365-2761.2011.01243.x
Evelyn T. P. T., Prosperi-Porta L., Ketcheson J. E. (1986). Experimental intra-ovum infection of salmonid eggs with Renibacterium salmoninarum and vertical transmission of the pathogen with such eggs despite their treatment with erythromycin. Dis. Aquat. Organisms 1, 197–202. doi: 10.3354/dao001197
Evenden A. J., Grayson T. H., Gilpin M. L., Munn C. B. (1993). Renibacterium salmoninarum and bacterial kidney disease—the unfinished jigsaw. Annu. Rev. Fish Dis. 3, 87–104. doi: 10.1016/0959-8030(93)90030-F
Evensen Ø., Espelid S., Håstein T. (1991). Immunohistochemical identification of vibrio salmonicida in stored tissues of atlantic salmon salmo salar from the first known outbreak of cold-water vibriosis ('Hitra disease'). doi: 10.5555/19922262488
FAO (2018).The state of world fisheries and aquaculture 2018. In: Meeting the sustainable development goals. Available online at: https://www.fao.org/3/i9540en/i9540en.pdf (Accessed September 5, 2022).
FAO (2020). “The state of world fisheries and aquaculture 2020,” in Sustainability in action. (FAO, United Nations). Available at: https://www.fao.org/3/ca9229en/ca9229en.pdf (Accessed June 5, 2023).
FAO/NACA (2000). Asia regional technical guidelines on health management for the responsible movement of live aquatic animals and the beijing consensus and implementation strategy, in FAO fisheries technical Paper no. 402 (FAO, Rome, Italy), 53.
FAO Regional Office for Asia (2006). Rapid growth of selected asian economies: lessons and implications for agriculture and food security (Vol. 1) (Food & Agriculture Organisation, Regional office for the Asia and Pacific, Bangkok, Thailand).
Farkas J. (1985). Filamentous Flavobacterium sp. isolated from fish with gill diseases in cold water. Aquaculture 44, 1–10. doi: 10.1016/0044-8486(85)90037-7
Farmer B. D., Beck B. H., Straus D. L. (2012). Effectiveness of copper sulfate and potassium permanganate on Channel Catfish infected with Flavobacterium columnare. North Am. J. Aquaculture 74, 320–329. doi: 10.1080/15222055.2012.676000
Ferguson H. W., Girons A., Rizgalla G., LaPatra S., Branson E. J., MacKenzie K. (2006). Strawberry disease in rainbow trout in Scotland: pathology and association with Flavobacterium psychrophilum. 630–632.
Fijan N. N. (1969). Antibiotic additives for the isolation of Chondrococcus columnaris from fish. Appl. Microbiol. 17, 333. doi: 10.1128%2Fam.17.2.333-334.1969
Fisheries, F. A. O (2024). The state of world fisheries and aquaculture 2024 blue transformation in action. Rome, Italy.
Francis-Floyd R. (2011). Mycobacterial Infections of Fish. Stoneville, MS, USA: SRAC Publisher, pp. 1–12.
Fryer J. L., Sanders J. E. (1981). Bacterial kidney disease of salmonid fish. Annu. Rev. Microbiol. 35, 273–298. doi: 10.1146/annurev.mi.35.100181.001421
Garcia J. A., Dominguez L., Larsen J. L., Pedersen K. (1998). Ribotyping and plasmid profiling of Yersinia ruckeri. J. Appl. Microbiol. 85, 949–955. doi: 10.1111/j.1365-2672.1998.tb05258.x
Gaunt P. S., Gao D., Sun F., Endris R. (2010). Efficacy of florfenicol for control of mortality caused by flavobacterium columnare infection in channel catfish. J. Aquat. Anim. Health 22 (2), 115–122. doi: 10.1577/H09-057.1
Gauthier D. T. (2015). Bacterial zoonoses of fishes: a review and appraisal of evidence for linkages between fish and human infections. Veterinary J. 203, 27–35. doi: 10.1016/j.tvjl.2014.10.028
Gauthier D. T., Rhodes M. W. (2009). Mycobacteriosis in fishes: a review. Veterinary J. 180, 33–47. doi: 10.1016/j.tvjl.2008.05.012
Gibello A., Blanco M. M., Moreno M. A., Cutuli M. T., Domenech A., Domínguez L., et al. (1999). Development of a PCR assay for detection of Yersinia ruckeri in tissues of inoculated and naturally infected trout. Appl. Environ. Microbiol. 65, 346–350. doi: 10.1128/AEM.65.1.346-350.1999
Glenn R. A., Taylor P. W., Hanson K. C. (2011). The use of a real-time PCR primer/probe set to observe infectivity of Yersinia ruckeri in Chinook salmon, Oncorhynchus tshawytscha (Walbaum), and steelhead trout, Oncorhynchus mykiss (Walbaum. ). J. Fish Dis. 34, 783–791. doi: 10.1111/j.1365-2761.2011.01294.x
Glenn R. A., Taylor P. W., Pelton E. H., Gutenberger S. K., Ahrens M. A., Marchant L. M., et al. (2015). Genetic evidence of vertical transmission and cycling of Yersinia ruckeri in hatchery-origin fall chinook salmon Oncorhynchus tshawytscha. J. Fish Wildlife Manage. 6, 44–54. doi: 10.3996/012014-JFWM-010
Gohari M., Sharifiyazdi H., Akhlaghi M. (2010). Detection of Yersinia ruckeri in rainbow trout (Oncorhynchus mykiss) fry tissues, using bacterial culture, simple PCR and nested PCR. Bulletian Eur. Assoc. Fish Pathologists 30, 177–184.
Good C., Davidson J., Wiens G. D., Welch T. J., Summerfelt S. (2014). Flavobacterium branchiophilum and F. succinicans associated with bacterial gill disease in rainbow trout Oncorhynchus mykiss (Walbaum) in water recirculation aquaculture systems. J. fish Dis. 38, 409. doi: 10.1111/jfd.12249
Guo W., Han R., Xu W., Lu Z., Li Y., Dan X., et al. (2023). The protective effect of inactivated Flavobacterium columnare vaccine in grass carp (Ctenopharyngodon idellus). Front. Immunol. 14. doi: 10.3389/fimmu.2023.1162975
Gupta T., Fine-Coulson K., Karls R., Gauthier D., Quinn F. (2013). Internalization of Mycobacterium shottsii and Mycobacterium pseudoshottsii by Acanthamoeba polyphaga. Can. J. Microbiol. 59, 570–576. doi: 10.1139/cjm-2013-0079
Haenen O. L., Evans J. J., Berthe F. (2013). Bacterial infections from aquatic species: potential for and prevention of contact zoonoses. Rev. Scientifique Technique (International Office Epizootics) 32, 497–507. doi: 10.20506/rst.32.2.2245
Hashish E., Merwad A., Elgaml S., Amer A., Kamal H., Elsadek A., et al. (2018). Mycobacterium marinum infection in fish and man: epidemiology, pathophysiology and management; a review. Veterinary Q. 38, 35–46. doi: 10.1080/01652176.2018.1447171
Hedrick R. P., McDowell T., Groff J. (1987). Mycobacteriosis in cultured striped bass from California. J. Wildlife Dis. 23, 391–395. doi: 10.7589/0090-3558-23.3.391
Hill B. J. (2005). The need for effective disease control in international aquaculture. Developments Biologicals 121, 3–12. Available online: https://pubmed.ncbi.nlm.nih.gov/15962465/
Hiney M., Olivier G. (1999). Furunculosis (Aeromonas salmonicida). In: Woo PTK, Bruno DW (eds) Fish diseases and disorders, Vol 3. Viral, bacterial and fungal infections. CABI Publishing, New York, p 341–425.
Hirvelä‐Koski V., Pohjanvirta T., Koski P., Sukura A. (2006). Atypical growth of Renibacterium salmoninarum in subclinical infections. Journal of Fish Diseases 29 (1), 21–29. doi: 10.1111/j.1365-2761.2005.00677.x
Hoar W. S., Randall D. J., Iwama G., Nakanishi T. (1997). The fish immune system: organism, pathogen, and environment (Academic press, United States of America).
Hoare R., Jung S. J., Ngo T. P., Bartie K., Bailey J., Thompson K. D., et al. (2019). Efficacy and safety of a non-mineral oil adjuvanted injectabl vaccine for the protection of Atlantic salmon (Salmo salar L.) against Flavobacterium psychrophilum. Fish shellfish Immunol. 85, 44–51. doi: 10.1016/j.fsi.2017.10.005
Hofer R. N., Lin A., House B. C., Purvis C. N., Harris B. J., Symes S. J., et al. (2023). Exogenous polyunsaturated fatty acids (PUFAs) influence permeability, antimicrobial peptide resistance, biofilm formation and membrane phospholipid structure in an A-layer and non-A-layer strain of Aeromonas salmonicida. J. Fish Dis. 46, 31–45. doi: 10.1111/jfd.13715
Horne M. T., Barnes A. C. (1999). Enteric redmouth disease (Yersinia ruckeri). In: Woo PTK, Bruno DW(eds) Fish diseases and disorders, Vol 3. Viral, bacterial and fungal infections. CABI Publishing, Wallingford, p 455–477.
Hsu H. M., Wooster G. A., Bowser P. R. (1994). Efficacy of enrofloxacin for the treatment of salmonids with bacterial kidney disease, caused by Renibacterium salmoninarum. J. Aquat. Anim. Health 6, 220–223. doi: 10.1577/1548-8667(1994)006%3C0220:EOEFTT%3E2.3.CO;2
Huang Y., Sun H., Dong J., Zhang J., Wang H., Yang L., et al. (2024). Novel identification of mixed infection of Lactococcus garvieae and Cryptocaryon irritans isolated from cultured Trachinotus ovatus in China. PloS One 19, e0301674. doi: 10.1371/journal.pone.0301674
Izadi F., Vajargah M. F. (2022). An overview of furunculosis. J. Biomed. Res. Environ. Sci. ISSN 2766, 2276. doi: 10.37871/jbres1507
Johansen L. H., Jensen I., Mikkelsen H., Bjørn P. A., Jansen P. A., Bergh Ø. (2011). Disease interaction and pathogens exchange between wild and farmed fish populations with special reference to Norway. Aquaculture 315, 167–186. doi: 10.1016/j.aquaculture.2011.02.014
Kawanishi M., Kojima A., Ishihara K., Esaki H., Kijima M., Takahashi T., et al. (2005). Drug resistance and pulsed-field gel electrophoresis patterns of lactococcus garvieae isolates from cultured seriola (yellowtail, amberjack and kingfish) in japan. Lett. Appl. Microbiol. 40 (5), 322–328. doi: 10.1111/j.1472-765X.2005.01690.x
Keller C., Wenker C., Jermann T., Hirschi R., Schildger B., Meier R., et al. (2018). Piscine mycobacteriosis–Involvement of bacterial species and reflection in pathology. Schweizer Archiv für Tierheilkunde 160, 385–393. doi: 10.17236/sat00165
Kent M. L., Benda S., St-Hilaire S., Schreck C. B. (2013). Sensitivity and specificity of histology for diagnoses of four common pathogens and detection of nontarget pathogens in adult Chinook salmon (Oncorhynchus tshawytscha) in fresh water. J. Veterinary Diagn. Invest. 25, 341–351. doi: 10.1177/1040638713482124\
Khalil S. M. I., Orioles M., Tomé P., Galeotti M., Volpatti D. (2024). Current knowledge of lactococcosis in rainbow trout: Pathogenesis, immune response and prevention tools. Aquaculture 580, 740363. doi: 10.1016/j.aquaculture.2023.740363
Khoo L., Taylor & Francis Group (2019). “Renal diseases and disorders,” in Fish diseases and medicine (CRC Press, 6000 Broken Sound Parkway NW, Suite 300, Boca Raton, FL 33487-2742), 211–229.
Kim E. H., Aoki T. (1993). Drug resistance and broad geographical distribution of identical R plasmids of Pasteurella piscicida isolated from cultured yellowtail in Japan. Microbiol. Immunol. 37, 103–109. doi: 10.1111/j.1348-0421.1993.tb03186.x
Kim E. H., Aoki T. (1996). Sulfonamide resistance gene in a transferable R plasmid of Pasteurella piscicida. Microbiol. Immunol. 40, 397–399. doi: 10.1111/j.1348-0421.1996.tb01085.x
Kim M. J., Hirono I., Kurokawa K., Maki T., Hawke J., Kondo H., et al. (2008). Complete DNA sequence and analysis of the transferable multiple-drug resistance plasmids (R plasmids) from Photobacterium damselae subsp. piscicida isolates collected in Japan and the United States. Antimicrobial Agents Chemotherapy 52, 606–611. doi: 10.1128/AAC.01216-07
Kim C. S., Lee S. H., Kim M. S., Kong K. H., Kim H. J., Shin G. W., et al. (2025). Development of a lateral flow immunochromatographic assay for the detection of Lactococcus garvieae in grey mullet (Mugil cephalus). Aquaculture Int. 33, 1–16. doi: 10.2139/ssrn.5007560
Kimura N., Wakabayashi H., Kudo S. (1978). Studies on bacterial gill disease in salmonids—I selection of bacterium transmitting gill disease. Fish Pathol. 12, 233–242. doi: 10.3147/jsfp.12.233
Knupp C. K. (2023). Intraspecific flavobacterium psychrophilum diversity as a factor in bacterial coldwater disease ecology and management (Michigan State University, United States of America). doi: 10.25335/j659-1k18
Kumar G., Menanteau-Ledouble S., Saleh M., El-Matbouli M. (2015). Yersinia ruckeri, the causative agent of enteric redmouth disease in fish. Veterinary Res. 46, 1–10. doi: 10.1186/s13567-015-0238-4
Kumari S., Teacher A. (2020). Freshwater and marine water fish diseases: a review. Int. J. Fisheries Aquat. Stud. 8, 65–68.
Kunttu H. M., Sundberg L. R., Pulkkinen K., Valtonen E. T. (2012). Environment may be the source of Flavobacterium columnare outbreaks at fish farms. Environ. Microbiol. Rep. 4, 398–402. doi: 10.1111/j.1758-2229.2012.00342.x
LaFrentz B. R., Cain K. D., Department of Fish and Wildlife Resources and the Aquaculture Research Institute (2004). Bacterial coldwater disease (University of Idaho, Moscow).
LaFrentz B. R., Králová S., Burbick C. R., Alexander T. L., Phillips C. W., Griffin M. J., et al. (2022). The fish pathogen flavobacterium columnare represents four distinct species: Flavobacterium columnare, flavobacterium covae sp. nov., flavobacterium davisii sp. nov. and flavobacterium oreochromis sp. nov., and emended description of flavobacterium columnare. Systematic Appl. Microbiol. 45 (2), 126293. doi: 10.1016/j.syapm.2021.126293
Lee S. H., Kim J. M., Lee J. R., Park W., Jeon C. O. (2010). Flavobacterium fluvii sp. nov., isolated from stream sediment. Int. J. Systematic Evolutionary Microbiol. 60, 353–357. doi: 10.1099/ijs.0.010850-0
Lehane L., Rawlln G. T. (2000). Topically acquired bacterial zoonoses from fish: a review. Med. J. Aust. 173, 256–259. doi: 10.5694/j.1326-5377.2000.tb125632.x
LeJeune J. T., Rurangirwa F. R. (2000). Polymerase chain reaction for definitive identification of Yersinia ruckeri. J. Veterinary Diagn. Invest. 12, 558–561. doi: 10.1177/104063870001200611
Leung T. L., Bates A. E. (2013). More rapid and severe disease outbreaks for aquaculture at the tropics: implications for food security. J. Appl. Ecol., 215–222. doi: 10.1111/1365-2644.12017
Lindstrom N. M., Call D. R., House M. L., Moffitt C. M., Cain K. D. (2009). A quantitative enzyme-linked immunosorbent assay and filtration-based fluorescent antibody test as potential tools to screen broodstock for infection with Flavobacterium psychrophilum. J. Aquat. Anim. Health 21, 43–56. doi: 10.1577/h08-031.1
Liu C., Hao J., Song M., Ye J., Zheng C., Huang Y., et al. (2024). Mycobacterium marinum hand infection: a case report and literature review. Front. Med. 11. doi: 10.3389/fmed.2024.1433153
Lönnström L. G., Hoffrén M. L., Wiklund T. (2008). Flavobacterium psychrophilum associated with mortality of farmed perch, Perca fluviatilis L. J. Fish Dis. 31, 793–797. doi: 10.1111/j.1365-2761.2008.00967.x
Lunder T., Evensen Ø., Holstad G., Håstein T. (2000). 'Winter ulcer' in the atlantic salmon salmo salar. pathological and bacteriological investigations and transmission experiments. Dis. Aquat. Organisms 23 (1), 39–49. doi: 10.3354/dao023039
Mabrok M., Elayaraja S., Chokmangmeepisarn P., Jaroenram W., Arunrut N., Kiatpathomchai W., et al. (2021). Rapid visualization in the specific detection of Flavobacterium columnare, a causative agent of freshwater columnaris using a novel recombinase polymerase amplification (RPA) combined with lateral flow dipstick (LFD) assay. Aquaculture 531, 735780. doi: 10.1016/j.aquaculture.2020.735780
Madetoja J., Dalsgaard I., Wiklund T. (2002). Occurrence of Flavobacterium psychrophilum in fish-farming environments. Dis. Aquat. Organisms 52, 109–118. doi: 10.3354/dao052109
Magariños B., Couso N., Noya M., Merino P., Toranzo A. E., Lamas J. (2001). Effect of temperature on the development of pasteurellosis in carrier gilthead seabream (Sparus aurata). Aquaculture 195, 17–21. doi: 10.1016/S0044-8486(00)00547-0
Magariños B., Toranzo A. E., Romalde J. L. (1996). Phenotypic and pathobiological characteristics of Pasteurella piscicida. Annu. Rev. Fish Dis. 6, 41–64. doi: 10.1016/S0959-8030(96)90005-8
Mainous M. E., Smith S. A. (2005). Efficacy of common disinfectants against Mycobacterium marinum. J. Aquat. Anim. Health 17, 284–288. doi: 10.1577/H04-051.1
Mammeri H., Poirel L., Nazik H., Nordmann P. (2006). Cloning and functional characterization of the ambler class C β-lactamase of Yersinia ruckeri. FEMS Microbiol. Lett. 257, 57–62. doi: 10.1111/j.1574-6968.2006.00148.x
Marzouk M. S. M., Essa M. A., El-Seedy F. R., Kenawy A. M., El-Gawad D. M. A. (2009). Epizootiological and histopathological studies on mycobacteriosis in some ornamental fishes. Global Veterinaria 3, 137–143. Available at: http://www.idosi.org/gv/gv3(2)09/11.pdf (Accessed December 12, 2023).
Matsui T., Nishizawa T. (2009). Modification of KDM-2 with culture-spent medium for isolation of Renibacterium salmoninarum. Fish Pathol. 44, 139–144. doi: 10.3147/jsfp.44.139
Menanteau-Ledouble S., Kumar G., Saleh M., El-Matbouli M. (2016). Aeromonas salmonicida: updates on an old acquaintance. Dis. Aquat. Organisms 120, 49–68. doi: 10.3354/dao03006
Meyburgh C. M., Bragg R. R., Boucher C. E. (2017). Lactococcus garvieae: an emerging bacterial pathogen of fish. Dis. Aquat. Organisms 123, 67–79. doi: 10.3354/dao03083
Michel C., Kerouault B., Martin C. (2003). Chloramphenicol and florfenicol susceptibility of fish-pathogenic bacteria isolated in France: comparison of minimum inhibitory concentration, using recommended provisory standards for fish bacteria. J. Appl. Microbiol. 95, 1008–1015. doi: 10.1046/j.1365-2672.2003.02093.x
Misaka N., Nishizawa T., Yoshimizu M. (2008). Quantitative detection of viable flavobacterium psychrophilum in chum salmon oncorhynchus keta by colony blotting and immunostaining. Fish Pathol. 43 (3), 117–123. doi: 10.3147/jsfp.43.117
Montforts M. H., Keen P. L. (2011). Antimicrobial resistance in the environment (John Wiley & Sons Inc., Hoboken). doi: 10.1002/9781118156247
Morii H., Bharadwaj M. S., Eto N. (2004). Cloning and nucleotide sequence analysis of the ampicillin resistance gene on a conjugative R plasmid from the fish pathogen Photobacterium damselae subsp. piscicida. J. Aquat. Anim. Health 16, 197–207. doi: 10.1577/H03-059.1
Morii H., Hayashi N., Uramoto K. (2003). Cloning and nucleotide sequence analysis of the chloramphenicol resistance gene on conjugative R plasmids from the fish pathogen Photobacterium damselae subsp. piscicida. Dis. Aquat. Organisms 53, 107–113. doi: 10.3354/dao053107
Morii H., Ishikawa Y. (2012). Cloning and nucleotide sequence analysis of the chloramphenicol and erythromycin resistance genes on a transferable R plasmid from the fish pathogen Photobacterium damselae subsp. piscicida. Bull. Faculty Fisheries. 93, 41–50. Available at: http://purl.org/coar/version/c_970fb48d4fbd8a85 (Accessed March 18, 2024).
Morita H., Toh H., Oshima K., Yoshizaki M., Kawanishi M., Nakaya K., et al. (2011). Complete genome sequence and comparative analysis of the fish pathogen lactococcus garvieae. PloS One 6 (8), e23184. doi: 10.1371/journal.pone.0023184
Murray A. G., Munro L. A., Wallace I. S., Allan C. E., Peeler E. J., Thrush M. A. (2012). Epidemiology of Renibacterium salmoninarum in Scotland and the potential for compartmentalised management of salmon and trout farming areas. Aquaculture 324, 1–13. doi: 10.1016/j.aquaculture.2011.09.034
Mutoji K. N. (2011). Investigation into mechanisms of mycobacterial transmission between fish (University of Louisiana at Lafayette).
Nakai T., Sugimoto R., Park K. H., Matsuoka S., Mori K. I., Nishioka T., et al. (1999). Protective effects of bacteriophage on experimental Lactococcus garvieae infection in yellowtail. Dis. Aquat. Organisms 37, 33–41. doi: 10.3354/dao037033
Noble A. C., Summerfelt S. T. (1996). Diseases encountered in rainbow trout cultured in recirculating systems. Annu. Rev. Fish Dis. 6, 65–92. doi: 10.1016/S0959-8030(96)90006-X
Noga E. J. (2010). Fish disease: diagnosis and treatment (John Wiley & Sons, Ames, Lowa). doi: 10.1002/9781118786758
Noga E. J., Levine J. F., Townsend K., Bullis R. A., Carlson C. P., Corbett W. T. (1988). Kidney biopsy: A nonlethal method for diagnosing Yersinia ruckeri infection (enteric red mouth disease) in rainbow trout(Salmo gairdneri). Am. J. Veterinary Res. 49, 363–365. doi: 10.2460/ajvr.1988.49.03.363
Ohtani M., Villumsen K. R., Strøm H. K., Raida M. K. (2014). 3D visualization of the initial Yersinia ruckeri infection route in rainbow trout (Oncorhynchus mykiss) by optical projection tomography. PloS One 9, e89672. doi: 10.1371/journal.pone.0089672
Ordal E. J., Rucker R. R. (1944). Pathogenic myxobacteria. Proc. Soc. Exp. Biol. Med. 56, 15–18. doi: 10.3181/00379727-56-14572
Osorio C. R., Toranzo A. E., Romalde J. L., Barja J. L. (2000). Multiplex PCR assay for ureC and 16S rRNA genes clearly discriminates between both subspecies of Photobacterium damselae. Dis. Aquat. Organisms 40, 177–183. doi: 10.3354/dao040177
Ostevik L., Stormoen M., Hellberg H., Kraugerud M., Manji F., Lie K. I., et al. (2022). A cohort study of gill infections, gill pathology and gill-related mortality in sea-farmed Atlantic salmon (Salmo salar L.): A descriptive analysis. J. Fish Dis. 45, 1301–1321. doi: 10.1111/jfd.13662
Panangala V. S., Shelby R. A., Shoemaker C. A., Klesius P. H., Mitra A., Morrison E. E. (2006). Immunofluorescent test for simultaneous detection of Edwardsiella ictaluri and Flavobacterium columnare. Dis. Aquat. Organisms 68, 197–207. doi: 10.3354/dao068197
Panangala V. S., Shoemaker C. A., Klesius P. H. (2007). TaqMan real-time polymerase chain reaction assay for rapid detection of Flavobacterium columnare. Aquaculture Res. 38, 508–517. doi: 10.1111/j.1365-2109.2007.01695.x
Park J., Roh H., Lee Y., Lee J. Y., Kang H. Y., Seong M. J., et al. (2023). Characterization and pathogenicity of Flavobacterium psychrophilum isolated from rainbow trout (Oncorhynchus mykiss) in Korea. Microorganisms 11, 2546. doi: 10.1046/j.1365-2761.2003.00487.x
Perry G. M., Tarte P., Croisetiere S., Belhumeur P., Bernatchez L. (2004). Genetic variance and covariance for 0+ brook charr (Salvelinus fontinalis) weight and survival time of furunculosis (Aeromonas salmonicida) exposure. Aquaculture 235, 263–271. doi: 10.1016/j.aquaculture.2004.03.002
Plumb J. A. (2018). Health maintenance of cultured fishes: principal microbial diseases (CRC Press, Boca Raton). doi: 10.1201/9781351073141
Prearo M., Zanoni R. G., Dall'Orto B. C., Pavoletti E., Florio D., Penati V., et al. (2004). Mycobacterioses: emerging pathologies in aquarium fish. Veterinary Res. Commun. 28, 315–317. doi: 10.1023/B:VERC.0000045435.19522.af
Pulkkinen K., Suomalainen L. R., Read A. F., Ebert D., Rintamäki P., Valtonen E. T. (2010). Intensive fish farming and the evolution of pathogen virulence. Proc. R. Soc B 277, 593–600. doi: 10.1098/rspb.2009.1659
Purcell M. K., McKibben C. L., Pearman-Gillman S., Elliott D. G., Winton J. R. (2016). Effects of temperature on Renibacterium salmoninarum infection and transmission potential in Chinook salmon, Oncorhynchus tshawytscha (Walbaum). J. Fish Dis. 39, 787–798. doi: 10.1111/jfd.12409
Qin Z., Baker A. T., Raab A., Huang S., Wang T., Yu Y., et al. (2013). The fish pathogen Yersinia ruckeri produces holomycin and uses an RNA methyltransferase for self-resistance. J. Biol. Chem. 288, 14688–14697. doi: 10.1074/jbc.M112.448415
Rhodes M. W., Kator H., Kaattari I., Gauthier D., Vogelbein W., Ottinger C. A. (2004). Isolation and characterization of mycobacteria from striped bass Morone saxatilis from the Chesapeake Bay. Dis. Aquat. Organisms 61, 41–51. doi: 10.3354/dao061041
Rhodes M. W., Kator H., Kotob S., van Berkum P., Kaattari I., Vogelbein W., et al. (2003). Mycobacterium shottsii sp. nov., a slowly growing species isolated from Chesapeake Bay striped bass (Morone saxatilis). Int. J. Systematic Evolutionary Microbiol. 53, 421–424. doi: 10.1099/ijs.0.02299-0
Rhodes M. W., Kator H., McNabb A., Deshayes C., Reyrat J. M., Brown-Elliott B. A., et al. (2005). Mycobacterium pseudoshottsii sp. nov., a slowly growing chromogenic species isolated from Chesapeake Bay striped bass (Morone saxatilis). Int. J. Systematic Evolutionary Microbiol. 55, 1139–1147. doi: 10.1099/ijs.0.63343-0
Rhodes L. D., Nguyen O. T., Deinhard R. K., White T. M., Harrell L. W., Roberts M. C. (2008). Characterization of Renibacterium salmoninarum with reduced susceptibility to macrolide antibiotics by a standardized antibiotic susceptibility test. Dis. Aquat. Organisms 80, 173–180. doi: 10.3354/dao01959
Richards C. A., Murphy C. A., Brenden T. O., Loch T. P., Faisal M. (2017). Detection accuracy of Renibacterium salmoninarum in Chinook salmon, Oncorhynchus tshawytscha (Walbaum) from non-lethally collected samples: Effects of exposure route and disease severity. Prev. Veterinary Med. 145, 110–120. doi: 10.1016/j.prevetmed.2017.06.001
Rodgers C. J. (2001). Resistance of Yersinia ruckeri to antimicrobial agents in vitro. Aquaculture 196, 325–345. doi: 10.1016/S0044-8486(01)00546-4
Rodriíguez-Nava V., Couble A., Devulder G., Flandrois J. P., Boiron P., Laurent F. (2006). Use of PCR-restriction enzyme pattern analysis and sequencing database for hsp65 gene-based identification of Nocardia species. J. Clin. Microbiol. 44, 536–546. doi: 10.1128/jcm.44.2.536-546.2006
Romalde J. L. (2002). Photobacterium damselae subsp. piscicida: an integrated view of a bacterial fish pathogen. Int. Microbiol. 5, 3–9. doi: 10.1007/s10123-002-0051-6
Romero J., Feijoó C. G., Navarrete P. (2012). Antibiotics in aquaculture–use, abuse and alternatives. Health Environ. Aquaculture 159, 159–198. doi: 10.5772/28157
Rucker R. R., Bernier A. F., Whipple W. J., Burrows R. E. (1951). Sulfadiazine for kidney disease. Progressive Fish-Culturist 13, 135–137. doi: 10.1577/1548-8640(1951)13[135:SFKD]2.0.CO;2
Rucker R. R., Earp B. J., Ordal E. J. (1954). Infectious diseases of Pacific salmon. Trans. Am. Fisheries Soc. 83, 297–312. doi: 10.1577/1548-8659(1953)83[297:IDOPS]2.0.CO;2
Saleh M., Soliman H., El-Matbouli M. (2008). Loop-mediated isothermal amplification as an emerging technology for detection of Yersinia ruckeri the causative agent of enteric red mouth disease in fish. BMC Veterinary Res. 4, 1–10. doi: 10.1186/1746-6148-4-31
Saleh M., Soliman H., El-Matbouli M. (2015). “Gold nanoparticles as a potential tool for diagnosis of fish diseases,” in Veterinary infection biology: Molecular Diagnostics and High-Throughput Strategies, 245–252. Springer Nature, Germany. doi: 10.1007/978-1-4939-2004-4_19
Sandeep P., Chamundeswari Devi B., Kumar K. P. (2016). Present status of parasitic and bacterial diseases in fresh water fish seed farms in East Godavari District, Andhra Pradesh. Int. J. Appl. Pure Sci. Agric. 2, 117–121.
Santos P., Peixoto D., Ferreira I., Passos R., Pires P., Simões M., et al. (2022). Short-term immune responses of gilthead seabream (Sparus aurata) juveniles against Photobacterium damselae subsp. piscicida. Int. J. Mol. Sci. 23, 1561. doi: 10.3390/ijms23031561
Sapkota A., Sapkota A. R., Kucharski M., Burke J., McKenzie S., Walker P., et al. (2008). Aquaculture practices and potential human health risks: current knowledge and future priorities. Environ. Int. 34, 1215–1226. doi: 10.1016/j.envint.2008.04.009
Schachte J. H., Carey T. G. (1983). Guide to integrated fish health management in the great lakes basin Great Lakes Fishery Commission, Ann Arbor, Michigan. Spec. Pub. 83-2:272 P.
Schar D., Zhao C., Wang Y., Larsson D. J., Gilbert M., Van Boeckel T. P. (2021). Twenty-year trends in antimicrobial resistance from aquaculture and fisheries in Asia. Nat. Commun. 12, 5384. doi: 10.1038/s41467-021-25655-8
Shinn A. P., Pratoomyot J., Bron J. E., Paladini G., Brooker E. E., Brooker A. J. (2015). Economic costs of protistan and metazoan parasites to global mariculture. Parasitology 142, 196–270. doi: 10.1017/S0031182014001437
Shinn A. P., Pratoomyot J., Griffiths D., Trong T. Q., Vu N. T., Jiravanichpaisal P., et al. (2018). Asian shrimp production and the economic costs of disease. Asian Fisheries Sci. 31, 29–58. doi: 10.33997/j.afs.2018.31.S1.003
Shoemaker C. A., Klesius P. H., Lim C., Yildirim M. E. D. I. H. A. (2003). Feed deprivation of channel catfish, Ictalurus punctatus (Rafinesque), influences organosomatic indices, chemical composition and susceptibility to Flavobacterium columnare. J. Fish Dis. 26, 553–561. doi: 10.1046/j.1365-2761.2003.00489.x
Silva M. T., Dos Santos N. M., Do Vale A. (2010). AIP56: a novel bacterial apoptogenic toxin. Toxins 2, 905–918. doi: 10.3390/toxins2040905
Slany M., Makovcova J., Jezek P., Bodnarova M., Pavlik I. (2014). Relative prevalence of Mycobacterium marinum in fish collected from aquaria and natural freshwaters in central Europe. J. Fish Dis. 37, 527–533. doi: 10.1111/jfd.12135
Snieszko S. F. (1958). Columnaris disease of fishes. US department of the interior, fish and wildlife service.
Snieszko S. F. (1981). Bacterial gill disease of freshwater fishes (US Fish and Wildlife Service, Washington, D.C).
Soltani M., Baldisserotto B., Hosseini Shekarabi S. P., Shafiei S., Bashiri M. (2021a). Lactococcosis a re-emerging disease in aquaculture: Disease significant and phytotherapy. Veterinary Sci. 8, 181. doi: 10.3390%2Fvetsci8090181
Soltani M., Naeiji N., Zagar A., Shohreh P., Taherimirghaed A. (2021b). Biotyping and serotyping of Lactococcus garvieae isolates in affected farmed rainbow trout (Oncorhynchus mykiss) in north Iran. Iranian J. Fisheries Sci. 20, 1542–1559. doi: 10.22092/ijfs.2021.125373
Soltani M., Nikbakht G., Ebrahimzadeh Moussavi H. A., Ahmadzadeh N. (2008). Epizootic outbreak of lactococcosis caused by lactococcus garvieae in farmed rainbow trout (Oncorhynchus mykiss) in iran. Bull. Eur. Assoc. Fish Pathologists 28 (5), 95–106.
Soltani M., Shanker S., Munday B. L. (1995). Chemotherapy of Cytophaga/Flexibacter-like bacteria (CFLB) infections in fish: studies validating clinical efficacies of selected antimicrobials. J. Fish Dis. 18, 555–565. doi: 10.1111/j.1365-2761.1995.tb00360.x
Speare D. J., Ferguson H. W., Beamish F. W. M., Yager J. A., Yamashiro S. (1991). Pathology of bacterial gill disease: sequential development of lesions during natural outbreaks of disease. J. Fish Dis. 14, 21–32. doi: 10.1111/j.1365-2761.1991.tb00573.x
Speare D. J., Markham R. J. F., Despres B., Whitman K., MacNair N. (1995). Examination of gills from salmonids with bacterial gill disease using monoclonal antibody probes for Flavobacterium branchiophilum and Cytophaga columnaris. J. Veterinary Diagn. Invest. 7, 500–505. doi: 10.1177/104063879500700413
Starliper C. E. (2011). Bacterial coldwater disease of fishes caused by Flavobacterium psychrophilum. J. Advanced Res. 2, 97–108. doi: 10.1016/j.jare.2010.04.001
Stephens F. J., Raidal S. R., Buller N., Jones B. (2006). Infection with Photobacterium damselae subspecies damselae and Vibrio harveyi in snapper, Pagrus auratus with bloat. Aust. Veterinary J. 84, 173–177. doi: 10.1111/j.1751-0813.2006.tb12774.x
Stock I., Henrichfreise B., Wiedemann B. (2002). Natural antibiotic susceptibility and biochemical profiles of Yersinia enterocolitica-like strains: Y. bercovieri, Y. mollaretii, Y. aldovae and ‘Y. ruckeri'. J. Med. Microbiol. 51, 56–69. doi: 10.1099/0022-1317-51-1-56
Strøm S. B., Nilsen H. (2021). Pasteurella skyensis in Atlantic salmon (Salmo salar L.) in Western Norway. Bull. Eur. Assoc. Fish Pathologists 41, 160–168. doi: 10.48045/001c.31534
Su F. J., Chen M. M. (2022). Protective efficacy of novel oral biofilm vaccines against Photobacterium damselae subsp. damselae infection giant grouper Epinephelus lanceolatus. Vaccines 10, 207. doi: 10.3390/vaccines10020207
Subasinghe R. P., McGladdery S. E., Hill B. J. (2004). Surveillance and zoning for aquatic animal diseases (No. 451) (Food & Agriculture Organisation of the United Nations). Available at: https://www.fao.org/3/y5325e/y5325e.pdf (Accessed March 5, 2024).
Suzuki K., Misaka N., Mizuno S., Sasaki Y. (2017). Subclinical infection of Renibacterium salmoninarum in fry and juveniles Chum salmon Oncorhynchus keta in Hokkaido, Japan. Fish Pathol. 52, 89–95. doi: 10.3147/jsfp.52.89
Swaim L. E., Connolly L. E., Volkman H. E., Humbert O., Born D. E., Ramakrishnan L. (2006). Mycobacterium marinum infection of adult zebrafish causes caseating granulomatous tuberculosis and is moderated by adaptive immunity. Infection Immun. 74, 6108–6117. doi: 10.1128/IAI.00887-06
Swain P., Mishra S., Dash S., Nayak S. K., Mishra B. K., Pani K. C., et al. (2007). Association of Flavobacterium branchiophilum in bacterial gill disease of Indian major carps. Indian J. Anim. Sci. 77, 646–649.
Talaat A. M., Trucksis M., Kane A. S., Reimschuessel R. (1999). Pathogenicity of Mycobacterium fortuitum and Mycobacterium smegmatis to goldfish, Carassius auratus. Veterinary Microbiol. 66, 151–164. doi: 10.1016/S0378-1135(99)00002-4
Taylor P. W. (2004). Detection of Flavobacterium psychrophilum in eggs and sexual fluids of Pacific salmonids by a polymerase chain reaction assay: implications for vertical transmission of bacterial coldwater disease. J. Aquat. Anim. Health 16, 104–108. doi: 10.1577/H03-053.1
Thune R. L., Fernandez D. H., Hawke J. P., Miller R. (2003). Construction of a safe, stabl, efficacious vaccine against Photobacterium damselae ssp. piscicida. Dis. Aquat. Organisms 57, 51–58. doi: 10.3354/dao057051
Thune R. L., Stanley L. A., Cooper R. K. (1993). Pathogenesis of gram-negative bacterial infections in warmwater fish. Annu. Rev. Fish Dis. 3, 37–68. doi: 10.1016/0959-8030(93)90028-A
Tobback E., Decostere A., Hermans K., Haesebrouck F., Chiers K. (2007). Yersinia ruckeri infections in salmonid fish. J. Fish Dis. 30, 257–268. doi: 10.1111/j.1365-2761.2007.00816.x
Tobback E., Decostere A., Hermans K., Ryckaert J., Duchateau L., Haesebrouck F., et al. (2009). Route of entry and tissue distribution of Yersinia ruckeri in experimentally infected rainbow trout Oncorhynchus mykiss. Dis. Aquat. Organisms 84, 219–228. doi: 10.3354/dao02057
Toranzo A. E., Barja J. L. (1992). First report of furunculosis in turbot reared in floating cages in northwest Spain. Bulletin-European Assoc. Fish Pathologists 12, 147–147.
Toranzo A. E., Casal J. F., Figueras A., Magarin B., Barja J. L. (1991). Pasteurellosis in cultured gilthead seabream (Sparus aurata): first report in Spain. Aquaculture , 99, 1–15. doi: 10.1016/0044-8486(91)90284-E
Toranzo A. E., Magariños B., Romalde J. L. (2005). A review of the main bacterial fish diseases in mariculture systems. Aquaculture 246, 37–61. doi: 10.1016/j.aquaculture.2005.01.002
Trienekens J., Zuurbier P. (2008). Quality and safety standards in the food industry, developments and challenges. Int. J. Production Economics 113, 107–122. Available at: https://EconPapers.repec.org/RePEc:eee:proeco:v:113:y:2008:i:1:p:107-122 (Accessed January 15, 2024).
Van Der Sar A. M., Abdallah A. M., Sparrius M., Reinders E., Vandenbroucke-Grauls C. M., Bitter W. (2004). Mycobacterium marinum strains can be divided into two distinct types based on genetic diversity and virulence. Infection Immun. 72, 6306–6312. doi: 10.1128/IAI.72.11.6306-6312.2004
Van Vliet D., Loch T. P., Smith P., Faisal M. (2017). Antimicrobial susceptibilities of Flavobacterium psychrophilum isolates from the Great Lakes Basin, Michigan. Microbial Drug resistance 23, 791–798. doi: 10.1089/mdr.2016.0103
Vatsos I. N., Thompson K. D., Adams A. (2006). Colonization of rainbow trout, Oncorhynchus mykiss (Walbaum), eggs by Flavobacterium psychrophilum, the causative agent of rainbow trout fry syndrome. J. Fish Dis. 29, 441–444. doi: 10.1111/j.1365-2761.2006.00735.x
Vendrell D., Balcázar J. L., Ruiz-Zarzuela I., De Blas I., Gironés O., Múzquiz J. L. (2006). Lactococcus garvieae in fish: a review. Comp. Immunology Microbiol. Infect. Dis. 29, 177–198. doi: 10.1016/j.cimid.2006.06.003
Vijayan K. K., Sanil N. K. (2012). “Health Management Strategies for profitabl and sustainable Aquaculture, with special reference to diagnostics,” in Lead papers on strategies for Aquaculture Development. Eds. Swain S. K., Swain P., Pillai B. R., Raghunath M. R., Jayasankar P. (ICAR-Central Institute of Freshwater Aquaculture, Bhubaneswar, India).
Villumsen K. R., Neumann L., Ohtani M., Strøm H. K., Raida M. K. (2014). Oral and anal vaccination confers full protection against enteric redmouth disease (ERM) in rainbow trout. PloS One 9, e93845. doi: 10.1371/journal.pone.0093845
Von Graevenitz A. (1990). Revised nomenclature of Campylobacter laridis, Enterobacter intermedium, and “Flavobacterium branchiophila. Int. J. Systematic Evolutionary Microbiol. 40, 211–211. doi: 10.1099/00207713-40-2-211
Waine A., Katsiadaki I., Sebire M., Tidbury H. (2023). Exploring Yersinia ruckeri (O1 Biotype 2) infection in three early life-stages of rainbow trout. Dis. Aquat. Organisms 155, 7–19. doi: 10.3354/dao03737
Wakabayashi H., Huh G. J., Kimura N. (1989). Flavobacterium branchiophila sp. nov., a causative agent of bacterial gill disease of freshwater fishes. Int. J. Systematic Evolutionary Microbiol. 39, 213–216. doi: 10.1099/00207713-39-3-213
Wang R., Feng J., Su Y., Ye L., Wang J. (2013). Studies on the isolation of Photobacterium damselae subsp. piscicida from diseased golden pompano (Trachinotus ovatus Linnaeus) and antibacterial agents sensitivity. Veterinary Microbiol. 162, 957–963. doi: 10.1016/j.vetmic.2012.09.020
Warren J. W. (1991). Diseases of hatchery fish (US Fish and Wildlife Service, Pacific Region, Portland, Oregon).
Wedemeyer G. A. (2001). Fish hatchery management. 2nd ed (Bethesda, MD: American Fisheries Society).
Wiedenmayer A. A., Evans J. J., Klesius P. H. (2006). Experimental edwardsiella tarda infection in nonabraded channel catfish ictalurus punctatus by immersion. Fisheries Sci. 72 (5). doi: 10.1111/j.1444-2906.2006.01266.x
Weir M., Rajić A., Dutil L., Uhland C., Bruneau N. (2012). Zoonotic bacteria and antimicrobial resistance in aquaculture: opportunities for surveillance in Canada. Can. Veterinary J. 53, 619.
Weis J., Andrews C. J., Dyksen P. J. E., Ferrara P. R. A., Gannon J. T., Laumbach R. J., et al. (2017). Report of the NJDEP science advisory board re-use and disposal of hatchery fish diseased by furunculosis. New Jersey.
Welker T. L., Shoemaker C. A., Arias C. R., Klesius P. H. (2005). Transmission and detection of Flavobacterium columnare in channel catfish Ictalurus punctatus. Dis. Aquat. Organisms 63, 129–138. doi: 10.3354/dao063129
Whipps C. M., Butler W. R., Pourahmad F., Watral V. G., Kent M. L. (2007). Molecular systematics support the revival of Mycobacterium salmoniphilum (ex Ross 1960) sp. nov., nom. rev., a species closely related to Mycobacterium chelonae. Int. J. Systematic Evolutionary Microbiol. 57, 2525–2531. doi: 10.1099/ijs.0.64841-0
Wiens G. D. (2011). “Bacterial kidney disease (Renibacterium salmoninarum),” in Fish diseases and disorders. Volume 3: viral, bacterial and fungal infections (CABI, Wallingford UK), . 338–. 374. doi: 10.1079/9781845935542.0338
Wiens G. D., Rockey D. D., Wu Z., Chang J., Levy R., Crane S., et al. (2008). Genome sequence of the fish pathogen Renibacterium salmoninarum suggests reductive evolution away from an environmental Arthrobacter ancestor. J. Bacteriology 190, 6970–6982. doi: 10.1128/JB.00721-08
Wise A. L., LaFrentz B. R., Kelly A. M., Khoo L. H., Xu T., Liles M. R., et al. (2021). A review of bacterial co-infections in farmed catfish: Components, diagnostics, and treatment directions. Animals 11, 3240. doi: 10.3390/ani11113240
Woo P. T., Cipriano R. C. (2017). Fish viruses and bacteria: pathobiology and protection (CABI). doi: 10.1079/9781780647784.0000
Woo P. T., Leong J. A., Buchmann K. (2020). Climate change and infectious fish diseases (CABI, UK). doi: 10.1079/9781789243277.0000
Wood J. W. (1974). Diseases of Pacific salmon: their prevention and treatment (State of Washington, Department of Fisheries, Hatchery Division).
Xie X., Pan Z., Yu Y., Yu L., Wu F., Dong J., et al. (2023). Prevalence, virulence, and antibiotics gene profiles in Lactococcus garvieae isolated from cows with clinical mastitis in China. Microorganisms 11, 379. doi: 10.3390/microorganisms11020379
Yeh H. Y., Shoemaker C. A., Klesius P. H. (2006). Sensitive and rapid detection of Flavobacterium columnare in channel catfish Ictalurus punctatus by a loop-mediated isothermal amplification method. J. Appl. Microbiol. 100, 919–925. doi: 10.1111/j.1365-2672.2006.02853.x
Zamparo S., Orioles M., Brocca G., Marroni F., Castellano C., Radovic S., et al. (2024). Novel insights on microbiome dynamics during a gill disease outbreak in farmed rainbow trout (Oncorhynchus mykiss). Sci. Rep. 14, 17791. doi: 10.1038/s41598-024-68287-w
Zappulli V., Patarnello T., Patarnello P., Frassineti F., Franch R., Manfrin A., et al. (2005). Direct identification of Photobacterium damselae subspecies piscicida by PCR-RFLP analysis. Dis. Aquat. Organisms 65, 53–61. doi: 10.3354/dao065053
Keywords: fish, disease diagnosis, symptoms, epidemiology, treatment
Citation: Ahmed I, Ishtiyaq S and Sayed SF (2025) An overview on understanding the major bacterial fish diseases in freshwater salmonids. Front. Aquac. 4:1515831. doi: 10.3389/faquc.2025.1515831
Received: 29 October 2024; Accepted: 25 February 2025;
Published: 25 March 2025.
Edited by:
Mohamed Faisal, Michigan State University, United StatesReviewed by:
Amel Mohamed El Asely, Benha University, EgyptNevien Abdelkhalek, Mansoura University, Egypt
Copyright © 2025 Ahmed, Ishtiyaq and Sayed. This is an open-access article distributed under the terms of the Creative Commons Attribution License (CC BY). The use, distribution or reproduction in other forums is permitted, provided the original author(s) and the copyright owner(s) are credited and that the original publication in this journal is cited, in accordance with accepted academic practice. No use, distribution or reproduction is permitted which does not comply with these terms.
*Correspondence: Imtiaz Ahmed, aW10aWF6YW11MUB5YWhvby5jb20=