- 1School of Marine Science and Ocean Engineering, University of New Hampshire, Durham, NH, United States
- 2School for Marine Science & Technology, University of Massachusetts, Dartmouth, New Bedford, MA, United States
- 3Department of Marine Technology, NTNU, Trondheim, Norway
- 4Department of Biological Sciences, University of New Hampshire, Durham, NH, United States
To meet growing seafood demands, the US aquaculture industry will need to consider farming the open ocean in a responsible manner. However, offshore environments can be energetic (seas > 8 m) making it difficult to maintain surface cage systems. To minimize potential storm damage, submerged culture technologies can be employed to safeguard the infrastructure and product. Steelhead trout (Oncorhynchus mykiss) have potential as an offshore species, though they have open air bladders (physostomous), and need access to air to inflate their swim bladders. To address this concern, three experiments were developed to explore the ability of O. mykiss to cope with extended periods of submergence. The studies used small (~300 g) and large (~1000 g) trout, in cages that ranged from 3.7 to 68 m3, that were submerged for periods of one to four weeks. Data storage tags (DST), sonar and video were used to quantify their ability to manage with submergence. Results indicated differences in growth, condition, and mortality among the treatments. The study suggests O. mykiss can be submerged for days to weeks with no negative effects, but that submergence times >3 weeks can be detrimental. It is therefore recommended that when culturing O. mykiss in areas exposed to severe storms or hazardous environmental conditions (e.g. harmful algal blooms or icing), submersible cages should be utilized to avoid these events up to 3 weeks.
Introduction
The New England ground fishery has nearly collapsed because of overfishing and habitat loss (Milich, 1999; Hennessey and Healey, 2000; Dadswell et al., 2022). This shortage has stimulated new ideas to produce seafood and to provide economic opportunities for harvesters that have, or will, become displaced from traditional fisheries (Hennessey and Healey, 2000). Among the most logical of the long-term solutions is the further development of marine aquaculture, which has the capacity to produce the needed seafood, provide economic opportunities for displaced harvesters that match their skill sets and existing equipment, and contribute to economic and community development, particularly in New England. Aquaculture remains the fastest growing animal food production sector, largely due to global fish consumption increasing nearly twice as quickly as annual world population growth (FAO, 2022).
It is widely accepted that new marine aquaculture development in the US will occur offshore in less utilized waters. The US government has attempted to facilitate this by developing the National Offshore Aquaculture Act of 2005. In addition, regional centers throughout the US (NH, HI, CA, and FL) were funded to explore hatchery, nursery and grow out technologies for cage culture (Benetti et al., 2010; Fredriksson et al., 2004; Tsukrov et al., 2000; Chambers and Ostrowski, 1999; Tamaru et al., 1998). New marine finfish species, both temperate and tropical were successfully spawned, raised in hatcheries and made available for ocean growout. Submerged cages (e.g. Sea Station™) were preferred to protect juvenile fish from turbulent offshore surface conditions (e.g., wind and waves) that were demonstrated by Rillahan et al. (2011, 2009) and Chambers et al. (2007). Other benefits of submerged culture were less wear on the culture system, more stable temperatures, and less biofouling on the nets due to lower temperatures and less light (Chambers and Howell, 2006).
Rainbow trout (O. mykiss), called steelhead when they transfer from fresh to salt water, are commercially grown in protected waters in Chile, Norway, Faroe Islands, Canada, and to a limited extent in the USA (New Hampshire). Steelhead trout have great potential to succeed as an aquaculture species in New England. First, they have been domesticated for >150 years and are the basis for recreational fisheries and freshwater aquaculture throughout the world. As a result, juveniles are readily available from numerous commercial hatcheries. Second, unlike Atlantic salmon, O. mykiss does not go through true smoltification (internal metabolic processes that allows fish to migrate from fresh to seawater), so juveniles can go directly from a freshwater hatchery to full strength seawater (35 ppt.) (Ward and Slaney, 1988; Bond et al., 2008; Hayes et al., 2011). Third, the species has a relatively fast growth rate in sea cages, reaching marketable size (1-3 kg) in 8 months after stocking at 250g (Akbulut et al., 2002; Chambers, 2013). Finally, they are disease resistant, are more temperature tolerant than Atlantic salmon, and have a high market value (>$13.00/kg) (Hinshaw et al., 2004; D’Agaro et al., 2022).
Submerged cage culture has been successfully demonstrated for at least 11 finfish species, including Atlantic salmon (Oppedal et al., 2011; Dempster et al., 2008, 2009; Korsøen et al., 2009, 2012), Pacific threadfin (Ryan, 2004), cobia (Rapp et al., 2007), red porgy (Papandroulakis et al., 2013), Atlantic cod and haddock (Chambers and Howell, 2006; Chambers et al., 2007; Rillahan et al., 2009, 2011), and halibut (Howell and Chambers, 2005), with commercial production of cobia, amberjack, seabass and seabream. Because suitable inshore aquaculture sites are becoming scarce, winter icing and storms can damage surface cages, and increasingly common harmful algal blooms and other environmental fluctuations can negatively impact fish, there is growing interest in culturing salmonid species in offshore, submerged sea cages. In addition to providing more locations and avoiding ‘visual pollution’, submerged systems could reduce the risk of fish escapees during storms (Naylor and Burke, 2005), and sea-lice infestations (Hevrøy et al., 2003). Despite these advantages, submerged systems for salmonids are only beginning to be considered, and much of the essential knowledge on how submergence will affect the fish is lacking. In the decade since this study took place, interest in submerged salmonid farming is growing worldwide, with farms established or developing in New Zealand, China, Chile and Scotland. To date, however there are no commercial operations growing steelhead trout in submerged cages, indicating a need for further research.
The uncertainty surrounding submergence is related to the assumption that salmonids become negatively buoyant when submerged beneath a cage roof because they cannot access the surface to gulp air to fill their swim bladders (Sievers et al., 2022). Fish can be categorized by whether their swim bladder is connected to their mouth cavity (physostome), separated (physoclist), or if that do not have a swim bladder at all. While physoclist fish (e.g. cod) and those without swim bladders (e.g. cobia) have been successfully cultured for complete growout cycles, physostomes, including salmonids require more careful consideration when culturing in submerged pens. There is an energetic cost when a fish cannot achieve neutral buoyancy that can lead to a reduction in growth and increase in disease and mortality.
Near shore, sea cage studies in Norway demonstrated that Atlantic salmon cope with submergence quite well in cages between 1600–2000 m3 that we submerged for 22 days with the roof held at 3 m depth (Oppedal et al., 2011; Dempster et al., 2008, 2009; Korsøen et al., 2009, 2012; Osland et al., 2001). They feed actively and grow well, albeit at a slower rate than those in surface cages. Dempster et al. (2008) found that 1.7 kg salmon increased their swimming speeds by 1.5 times when submerged, compared to fish in control cages, and suggested that the slower growth was due to the increased energetic expenditure for swimming. They noted, however, that temperature and light differences between fish held in surface cages, verses deeper submerged cages, may also have contributed to the observed growth differences.
To investigate the ability of steelhead trout to incur periods of submergence, three experiments were conducted to test the null hypotheses that the behavior, growth, incidence of fin damage, and mortality in submerged cages does not differ from trout held in surface cages under similar environmental conditions. Submergence durations and fish behavior were closely monitored with data storage tags (DST) and video to quantify their ability to cope with submergence. The external DST’s measured the pitch and roll axes of the tag to determine fish swimming angle. A heads-up angle indicated depletion of air in the swim bladder.
Methods and materials
Timing and locations
The project lasted 24 months, and involved three separate, but related experiments. In the first, which occurred from June through August 2011, we studied juvenile trout in small inshore cages located near the University’s Judd Gregg Marine Research Pier (JGP) in New Castle, NH, USA (Figure 1). The second experiment took place from October through December 2011, in the same location. This time, larger trout and cages were used. The third and final experiment was conducted in the summer of 2012 (June – August), at a study location ~ 0.8 km seaward from the JGP that had more exposure to wind and waves.
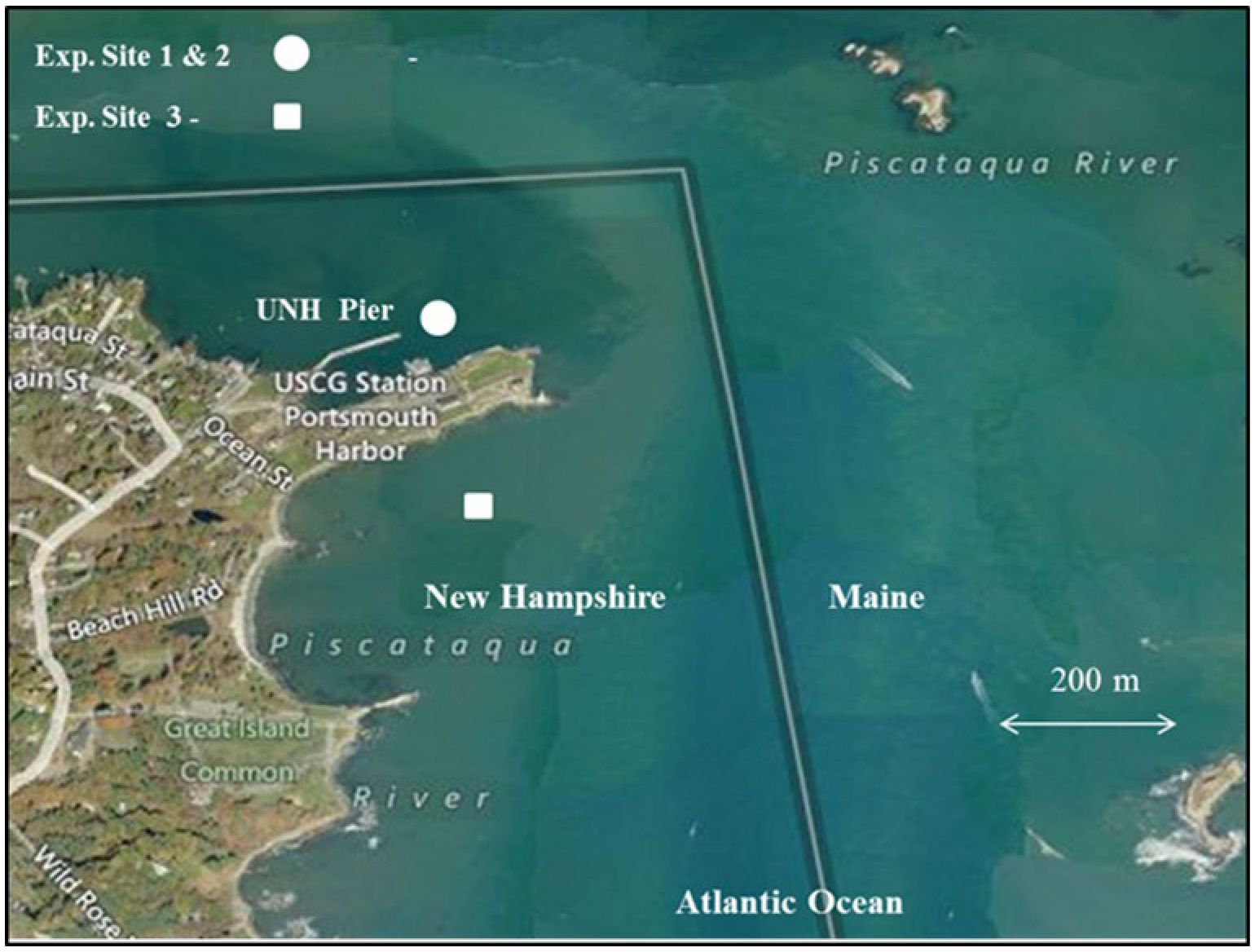
Figure 1. Aerial photo of the Judd Gregg Marine Research Pier in New Castle, NH and proximity of the experimental sites.
Steelhead trout
In all three experiments, juvenile steelhead trout were purchased from Sumner Brook Trout Farm in Ossipee, NH. Sumner Brook acquires eyed eggs from Trout Lodge in Sumner, WA, that are certified disease free. Fingerlings were raised in flow through, freshwater raceways for 8 months to a size of ~150 g. Prior to fish transfer to sea cages, the trout were fed a 3 mm, Skretting Bio-Transfer diet for 7 days. This diet helps transition salmonid smolts from fresh to saltwater environments. It has elevated dietary salts that encourage the development of osmoregulatory mechanisms, while added betaine acts as an osmoprotectant by relieving gastrointestinal stress. Fish were transported to the Jackson Estuarine Marine Lab in Great Bay, NH in insulated 1 m3 containers. Here, they were acclimated from freshwater to brackish bay water (20 ppt.) in flow through, fiberglass tanks for two weeks. They were then moved to a sea cage near the JGP for final acclimation to 30 ppt.
Fish were hand fed to satiation daily with a sinking, Bio Oregon Trout diet (45% protein, 24% lipid). Trout in submerged cages were fed through a flexible PVC hose that extended from the surface down into the center of each cage 1 m. Underwater video cameras were used to help determine feeding satiation in these treatments.
Because environmental variables can influence swimming behavior and depth of salmonids (Johansson et al., 2006, 2007; Oppedal et al., 2001, 2007), a series of environmental measurements were collected. HOBO® temperature loggers were installed 1 m below the surface of each cage and an YSI 6600 V2-2 Water Quality Sonde, located at the end of the pier adjacent to the cage site, collected additional environmental data (temperature, dissolved oxygen, salinity, pH, tidal amplitude, and turbidity). Lastly, current speeds were measured weekly in each cage with a Royal Eijkelkamp current meter, at 1 m depth intervals. Finally, any mortalities were recorded daily.
Experiment 1: two- and four-week submergence
In experiment 1, six, 3.75 m3 cylindrical cages (Figure 2A) were constructed from a 2.5 cm mesh Seawire™ netting. Seawire is a stiff, copper alloy net material which minimizes biofouling that can reduce water flow and quality (Chambers et al., 2012). On 10 June 2011, each cage was stocked with 55 trout with a mean (± SEM) starting weight of 255.6 ± 14.4 g and length of 27.6 ± 0.4 cm. The experimental design had two treatments (A & B) and a control (C), each with two replicates, for a total of six cages. Control cages (C1 and C2) were maintained at the surface throughout the study to allow fish to gulp air and treatment cages were each equipped with a nylon netting cover to keep the fish 1 m below the surface preventing them from accessing the surface to fill their gas bladders (Figure 2B).
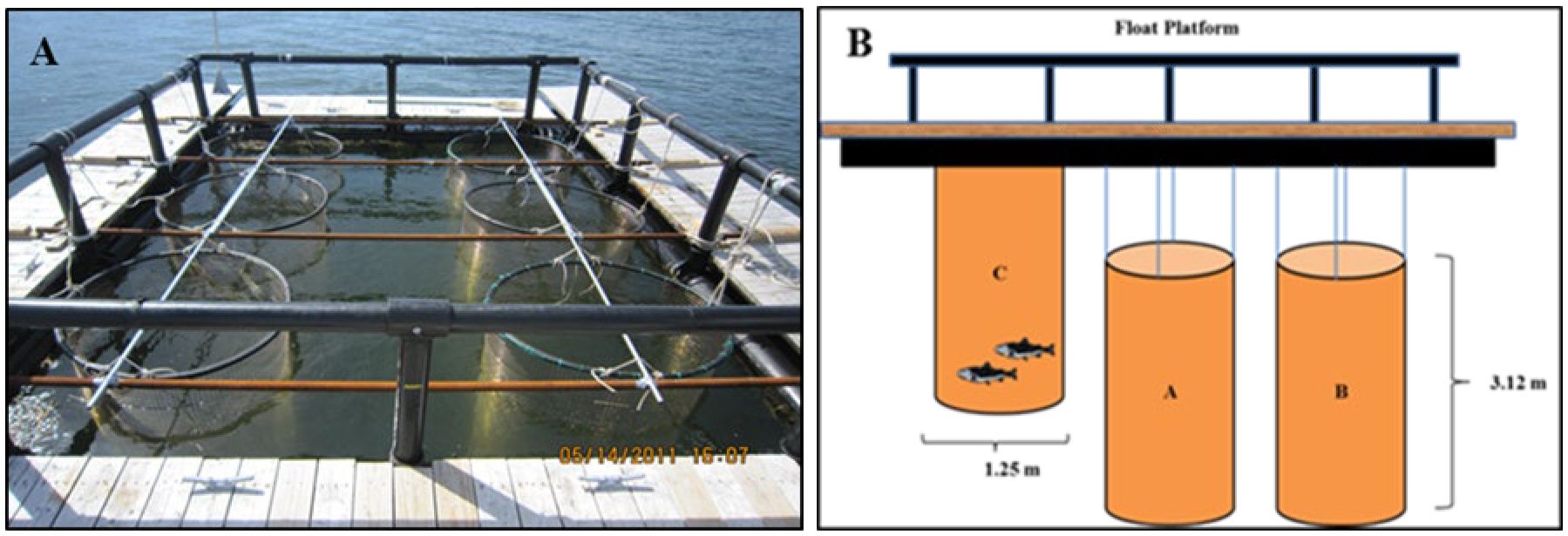
Figure 2. Cage design used in Experiment 1. Six, 3.75 m3 copper-alloy cages were suspended in a 4 x 5 m floating platform made of High-Density Polyurethane (A). Diagram (B) illustrates cage dimensions, the Control cage C (surface) and the submerged treatments cages A and B, 1 m below the surface to keep fish from refilling their swim bladders.
The replicate treatment cages A1 and A2 were submerged for two weeks, brought to the surface for one day, re-submerged for two weeks, brought to the surface for one day, etc. until the experiment was complete after 8 weeks. The replicate treatment cages B1 and B2 were submerged for 4 weeks, brought to the surface for one day, re-submerged for the next 4 weeks, and then brought to the surface at the end of the experiment.
Data from replicate pairs were compared, and because no difference in any of the measured variables was found (Mann-Whitney U tests, p > 0.1), data from the replicate pairs were combined.
Experiment 2: increasing submergence periods and evaluating buoyancy
The second experiment began in October 2011, using fish retained from experiment 1. In the second phase of the submergence project, two 68 m3 floating cages were deployed at the inshore site. Each of the 4.6 x 4.6 x 3.2 m cages were constructed of 2.5 cm knotless nylon twine and were supported at the surface by high-density polyethylene floating frames with wooden walkways (Figure 3). The control cage allowed trout access to the surface to gulp air to fill their swim bladders (Figure 3A) while the treatment cage was submerged 1 m below the surface for sequentially greater periods of time using a series of ropes and weights attached to the to the float platform.
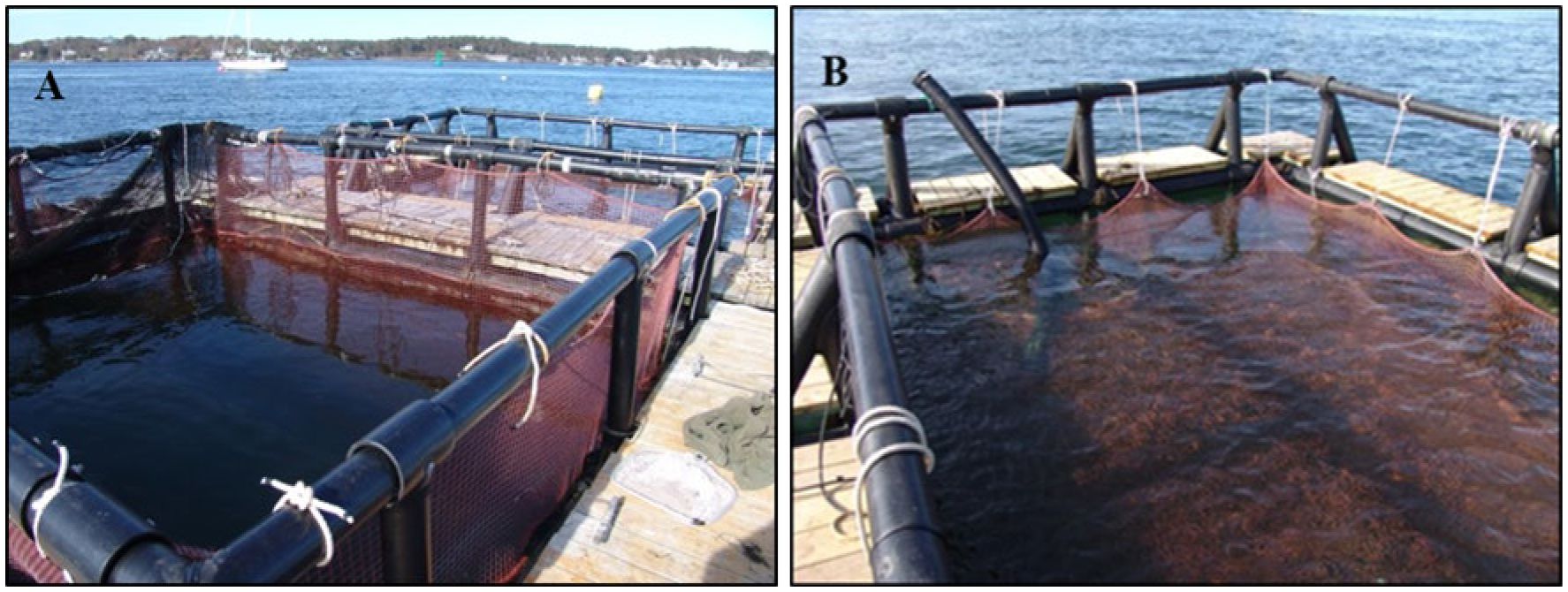
Figure 3. (A) Control cage left where trout were allowed access to the surface to gulp air. (B) Treatment cage with net nylon roof submerged 1 m below surface. Note feeding tube in upper left of image.
Each cage was stocked with 84 trout with a mean (± SEM) weight of 1070.4 ± 53.7 g and a mean length of 41.3 ± 0.7 cm. The experimental design had a single treatment (submerged) and a single surface control. Prior to the start of the experiment, a random sample of 10 fish from the control cage and 10 fish from the treatment cage were captured, anesthetized using 50 mg/L MS-222, and fitted with electronic data storage tags (Star-Oddi™ tilt tags). These tags were attached externally, just anterior-laterally of the dorsal fin because internal (surgical) placement would have made it difficult, if not impossible, to get the pitch and roll axes of the tag aligned with the same axes of the fish. (Figure 4). They recorded temperature (°C), depth (m), pitch angle (degrees head up or head down) and roll angle (degrees side-to-side) at either 45 (pitch and roll) or 90 (temperature and depth) second intervals. To test tag retention and survival, six trout were placed into a 600 L round fiberglass tank with flow through water at the UNH Coastal Marine Laboratory. Three of the trout were surgically implanted with dummy Star-Oddi tags while the remaining three fish were not tagged. After 1 month, all six fish had adapted to the tank, were feeding well and the three tags were still attached firmly to the trout.
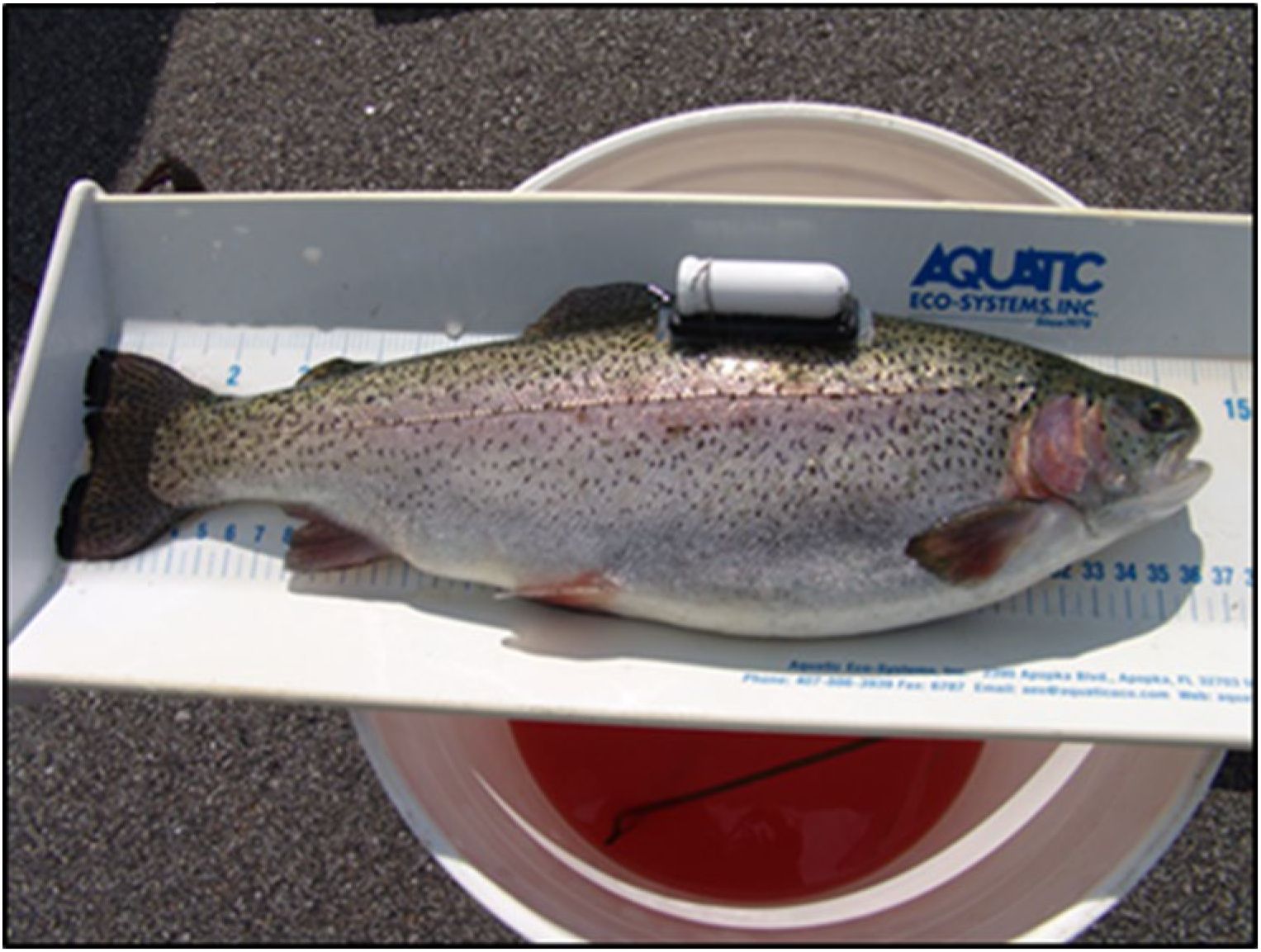
Figure 4. Steelhead trout (~1000 g) attached with a fitted Star-Oddi™ pitch and roll data storage tag.
Delays with the cage manufacturer extended the trial into the fall. With decreasing winter temperatures causing a cessation in feeding (< 5°C) and problems with the DST tags becoming entangled in the net, the submergence schedules were modified. Instead of the 2-, 3- and 4-week schedule, the treatment cage was submerged for 17 days, brought to the surface for 4 days while we reattached the data storage tags, and then re-submerged for 31 days (Nov. 4 through Dec. 5).
Experiment 3: exposed site
In the second year of the research, the two inshore cages used in experiment 2 were towed seaward to an exposed location approximately 0.8 km from the original site. Steelhead trout (~100 g) were purchased in early May 2012 and held at the UNH Jackson Estuarine Lab for 2 weeks to help transition them to brackish bay water (20 ppt). In early June, they were transferred to the UNH JGP (full strength salinity – 30 ppt). They were held in a net pen at the pier until they had grown to a mean weight of 308.1 ± 81.1 g and mean length 28.1 ± 1.8 cm. Fish were transferred to the experimental pens (200/cage) on June 19th, and on June 20th, the treatment cage was submerged 2 m below surface. The experimental pen was raised to the surface on June 27th, one week later and the first set of data was collected from fish (n = 25) in both the submerged and surface cages. Following this 24-hour surface time, the cage was submerged for sequentially longer periods of time (2, 3 and 4 weeks), each separated by 24 hours at the surface.
To quantify the fish swimming angles, a weighted vertical white line was placed in the center of each cage to a depth of 1 m off the net bottom. A Go-Pro™ video camera, mounted on a 2.3 m PVC pole, was lowered into each cage and attached to the side net for 30 minutes on each sampling date. Video was captured as the fish passed by the vertical white line, and later analyzed with Ethovision XT fish tracking software to determine mean tilt angle as the fish moved past the vertical line.
Growth, condition and fin damage
Fish were sampled bi-monthly throughout each experiment. A random sample of 25 fish from each cage was anesthetized with MS-222, weighed, and measured. Standard indices of growth and condition were calculated for each sampling date. Specific growth rate (SGR, % day-1) was calculated as:
where W2 and W1 are the mean live body weights (g) at times t2 and t1, respectively. Fulton’s condition factor (K) was calculated with the formula:
where W is the wet weight (g), and L is the total length (cm). During scheduled resurfacing of the treatment cages, a sample of 25 fish from both the control and treatment cages were visually examined for fin damage and/or snout abrasions that can be caused when the fish encounters the cage ‘roofs’ as they try to surface to obtain air and refill their swim bladders. The degree of snout damage was assigned using a subjective index (Figure 5) from 1 (undamaged) to 5 (extreme damage).
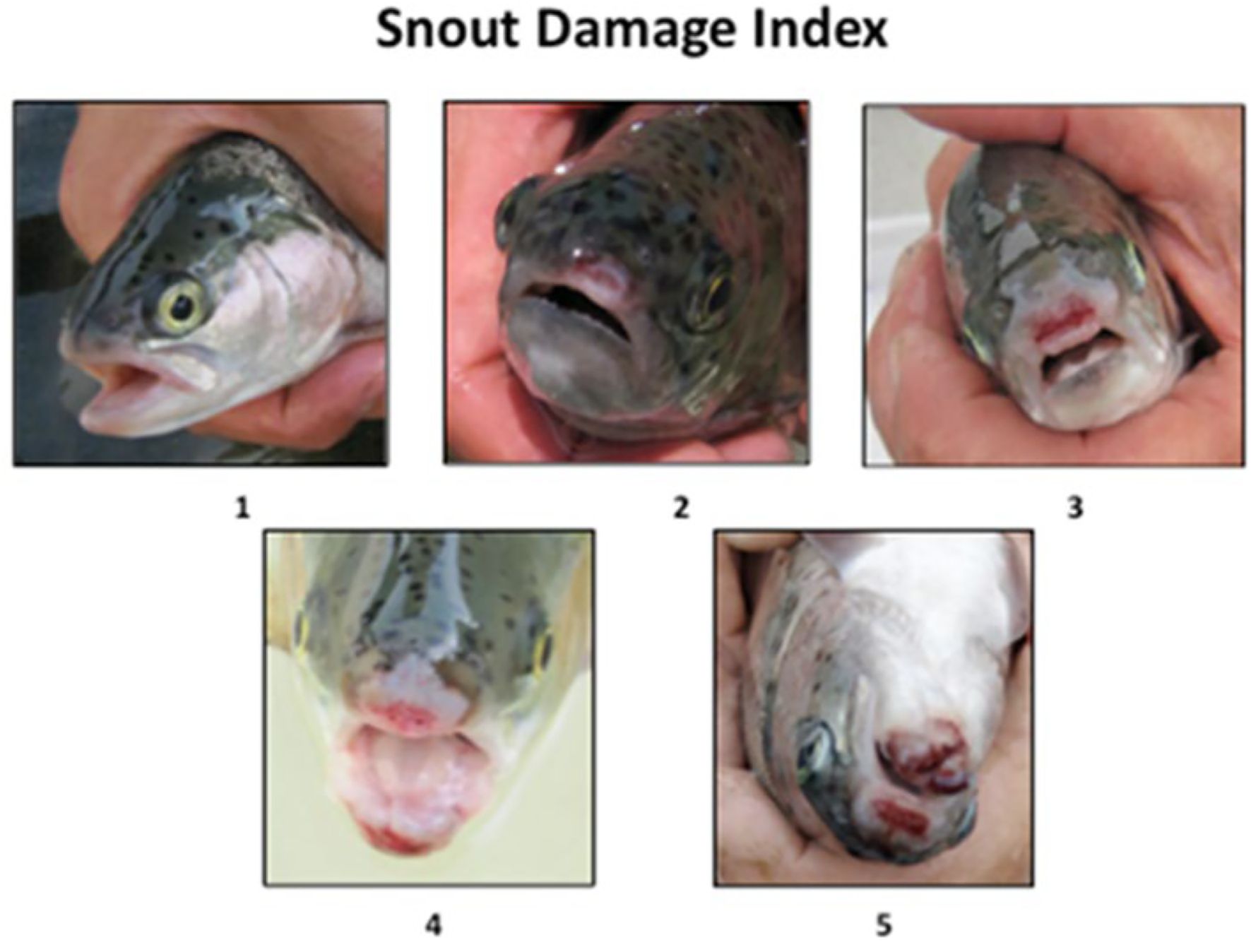
Figure 5. Visual representations of subjective snout index, ranging from no damage (1) to severe damage (5). Nose damage can occur when fish in the submerged treatments try to resurface to gulp air and fill their swim bladders.
Surface rolling and jumping behavior
Jumping and rolling behavior of both the control (surface) and treatment (submerged) cages were observed each time the treatment cages were brought to the surface. When the treatment cages were raised, the total number of jumps and rolls in all cages, during the first 30-min period, were counted. All counts were standardized to rolls or jumps fish-1 hr-1 as described in Furevik et al. (1993).
Statistical analyses
In Experiment 1, statistical analyses varied with experimental design. On those dates when only one of the submerged treatments was brought to the surface, comparisons to the control (surface) fish were made with Mann-Whitney U tests. On those dates when both submerged treatments were resurfaced, either one-way ANOVA, followed by Tukey-Kramer multiple comparison test, or a Kruskal-Wallis test, followed by Dunn’s Multiple Comparison test (if the data were not normally distributed) were used. Response variables compared included fish length and weight, survival percentage (square root arcsine transformed), incidence of fin, snout and body injuries, and condition. In Experiments 2 and 3, where there were not statistical replicates, differences in the same response variables between treatments were compared using Mann-Whitney U tests.
Results
Experiment 1: two- and four-week submergence
After 18 days of submergence, the fish in the surface cages (C) were significantly heavier than those in the submerged cages (A) and were in better condition (p < 0.05; Table 1). There were no differences in mean length or abrasions on the snout between surface and submerged fish (p > 0.05). On the second sampling date, both treatments (2- and 4-weeks submergence, A and B respectively) were sampled, along with the control (surface) cages (C). There were no significant differences in mean lengths or snout condition between treatments, or between treatments and the controls (p > 0.05). Fish in the surface (control) cages were significantly heavier than those submerged for 2 weeks (p < 0.05), but no different than those that had been submerged for 4 weeks (p > 0.05). There was no difference in the condition of the fish submerged for 2 and 4 weeks (p > 0.05), but the condition of the control (surface) fish was significantly higher that both treatments (p < 0.05). On the third sampling date fish in the control (surface) cage were significantly heavier, longer, and in better condition than those in the 2-weeks submergence (A) treatment (p < 0.05). There were no differences, however, in snout abrasions between the treatment and control fish (p > 0.05). At the end of the experiment, on August 11th, fish in the surface (control) cages were significantly heavier and longer than those in both treatment cages (p < 0.01). Fish in the control cages (C) were also in significantly better condition (p < 0.001) than those in the 2-weeks submergence treatment (A), but not better than those in the 4-weeks submergence (B) treatment (p > 0.05). There was no difference in condition between fish in the two treatments, or between nose abrasions in the fish in two treatments and the control (p > 0.05). Overall, trout in the control cages had significantly better weight, length, condition, and less nose abrasions than fish that were submerged for 2 weeks and 4 weeks (p < 0.05).
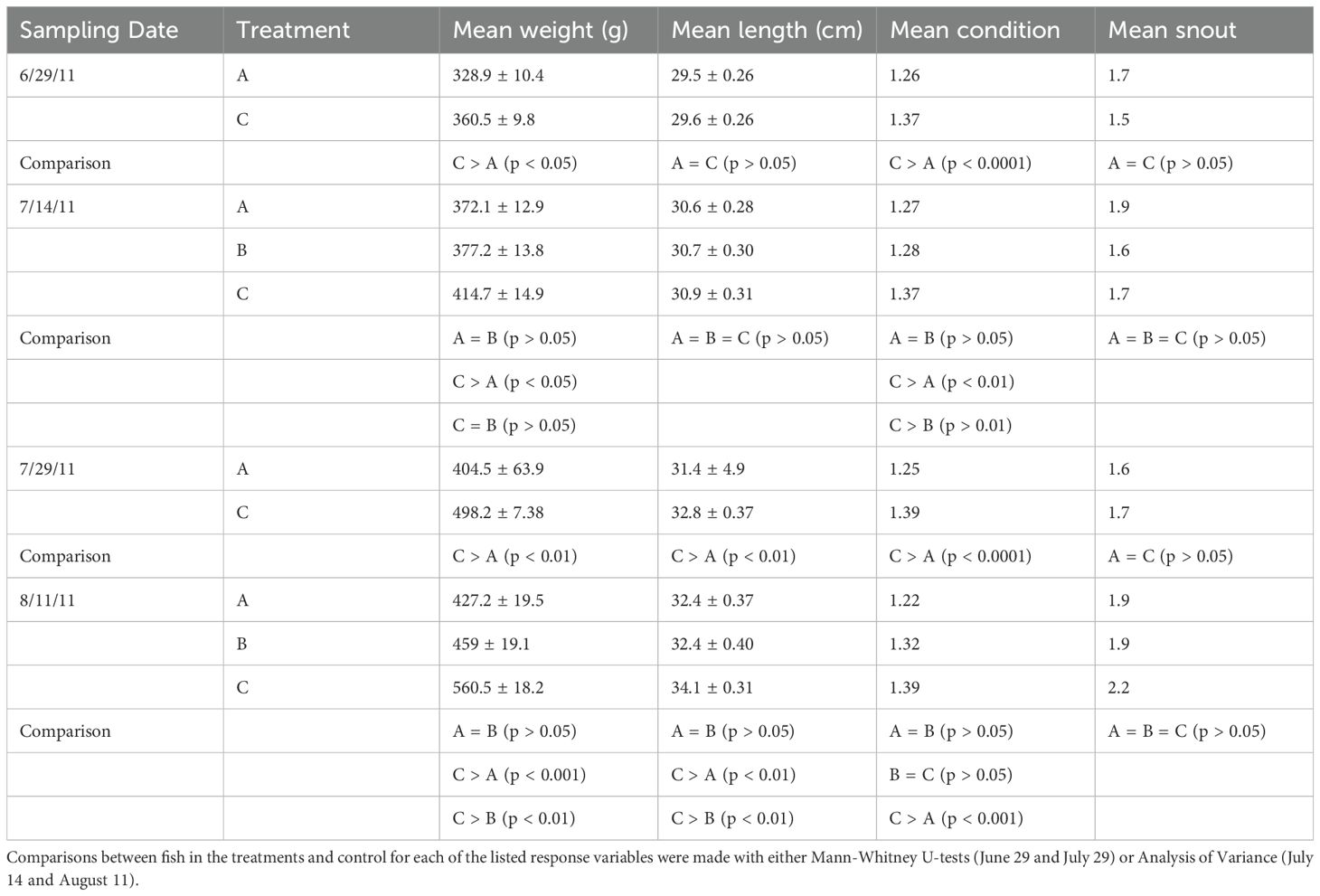
Table 1. Mean weights, lengths, conditions and nose abrasion indices of the fish in the 2-week submergence treatment (A), the 4-week submergence treatment (B) and the surface control (C) on the sampling dates in Experiment 1.
At the end of the 2- and 4-week intervals, trout in the submerged cages were brought to the surface. In all cases, fish that had been submerged, jumped more frequently than control (surface maintained) fish (Figure 6).
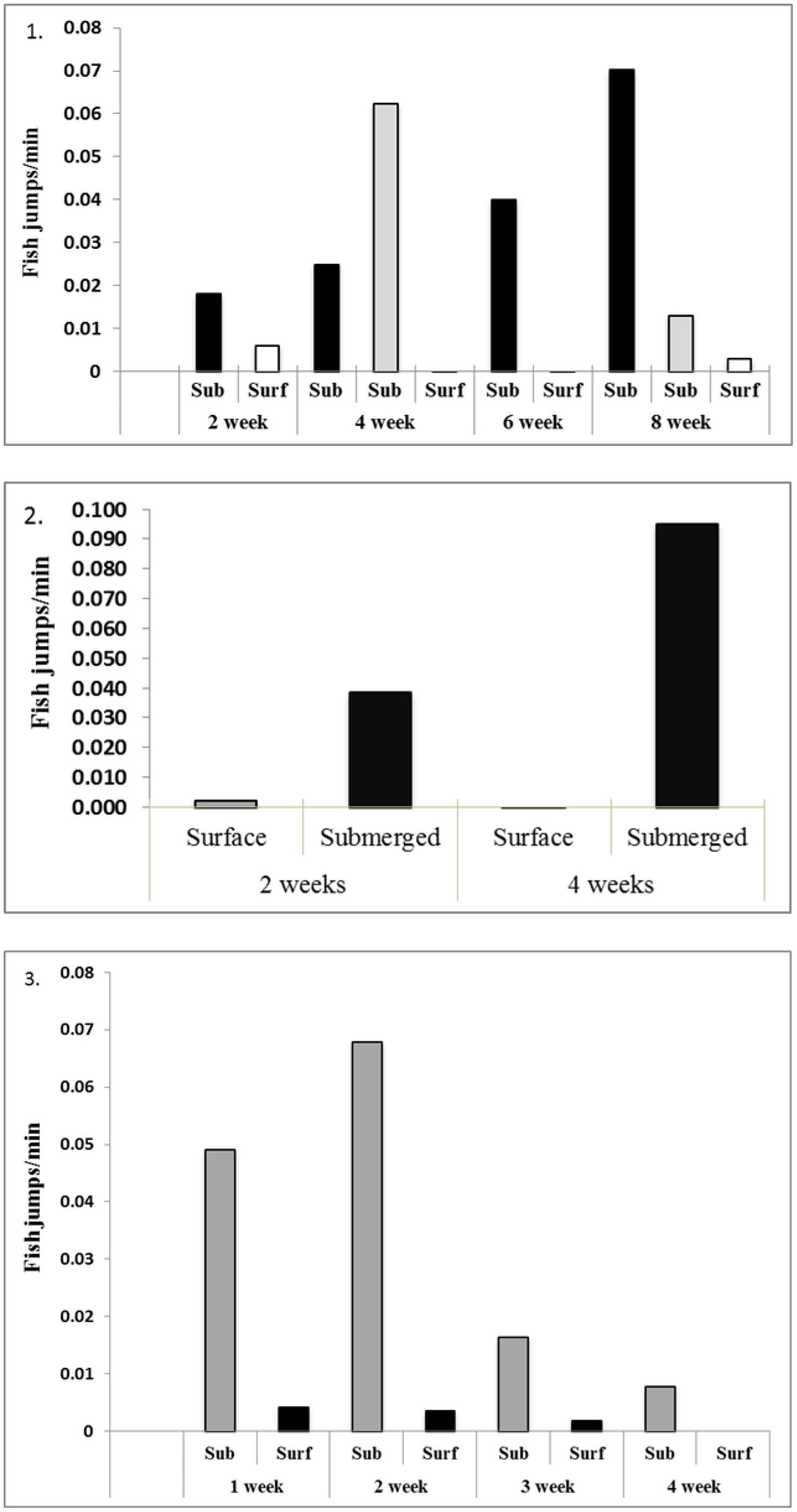
Figure 6. Number of jumps fish-1 minute-1 upon surfacing of submerged cages on the indicated sampling dates during Experiments 1, 2 and 3.
Mean (± SE) survival rates for treatments A and B, and the control (C), in Experiment 1 were 66.4% (± 6.3), 40.9% (± 10.0), and 90.9% (± 5.4), respectively (Figure 7). Analysis of variance (ANOVA) found that the means were significantly different (p < 0.05). The relatively low survival in Treatments A and B were caused by either bacterial or viral infection, characterized by skin lesions, and perhaps exacerbated by the periodic submergence of these two treatments. Trout survival was the lowest during the two-week submergence period.
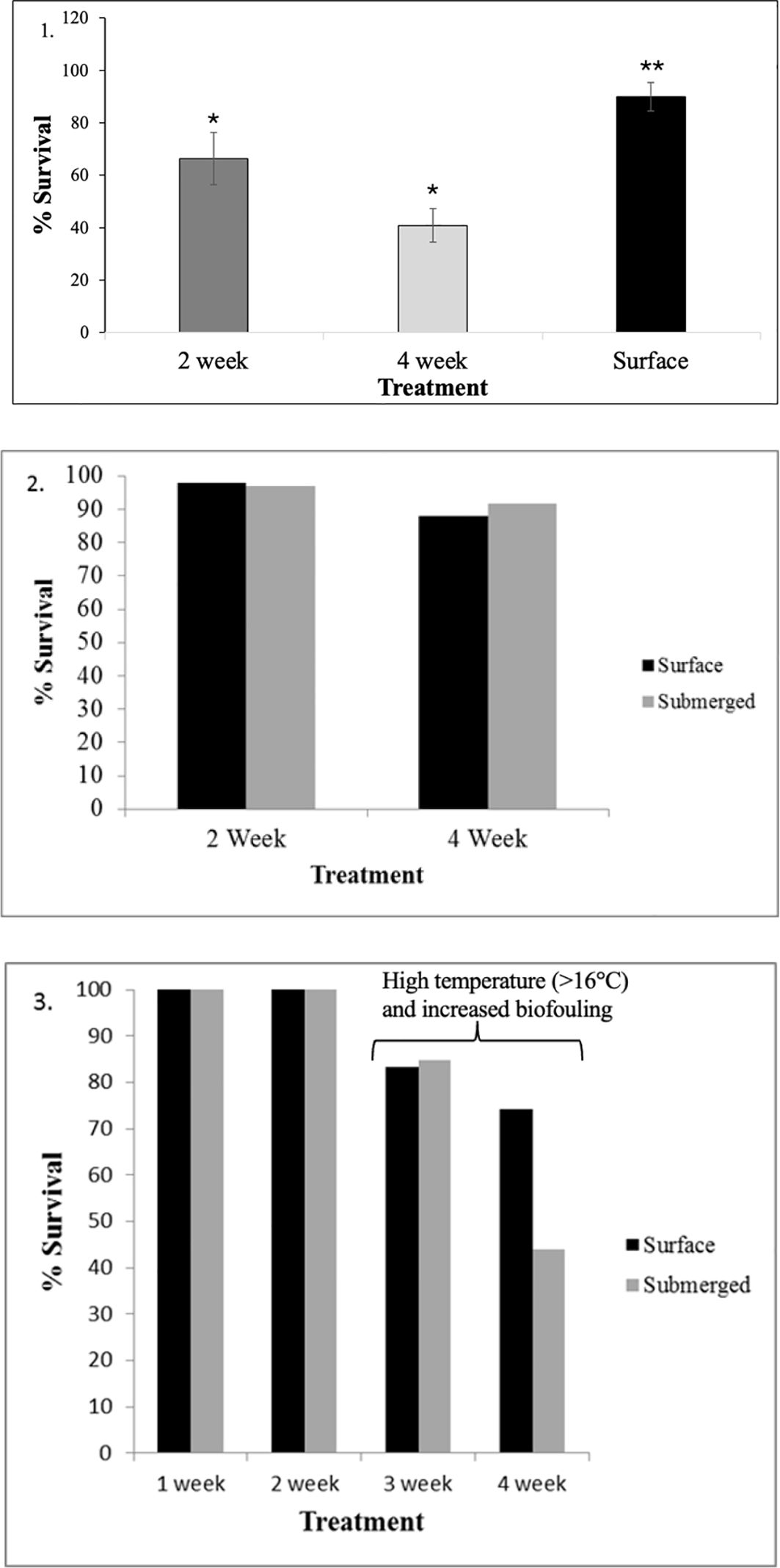
Figure 7. Survival rates of steelhead trout under different submergence conditions (Experiments 1, 2 and 3). Asterisks indicate significant differences between treatments (p > 0.05).
Specific growth rates (SGR) were calculated for fish in each treatment (A, B) and the control (C) in both Experiments 1 and 2 (Table 2). In Experiment 1, growth rates were highest in the surface control fish (C), intermediate in the 4-weeks submergence treatment (B), and lowest in the 2-weeks submergence treatment (A).
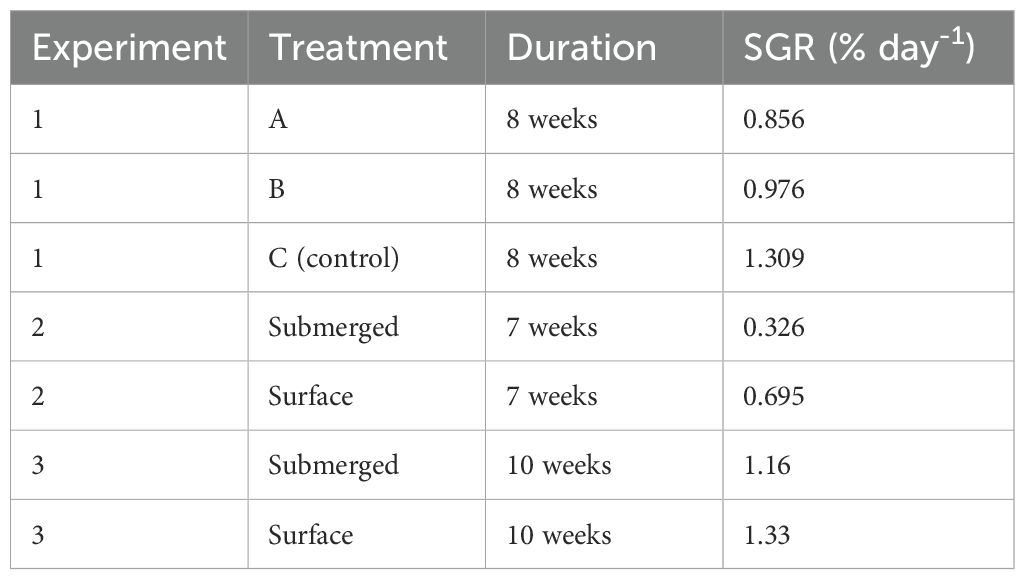
Table 2. Specific growth rates (SGR (%/d)) of each of the indicated treatments at the end of Experiments 1, 2 and 3.
Experiment 2: increasing submergence periods and evaluating buoyancy
In experiment two, 17 days after submergence, there were no differences in mean weight, mean length, mean condition, or mean nose abrasions between surface and submerged fish (p > 0.05; Table 3). However, on the final sampling date, after the treatment fish had been submerged continuously for 31 days, fish held at the surface (control) were significantly heavier than fish that had been submerged (p < 0.05). In fact, surface fish gained weight more than twice as fast as submerged fish. There were no significant differences in mean length, mean condition, or mean nose abrasions between surface and submerged fish (p > 0.05).
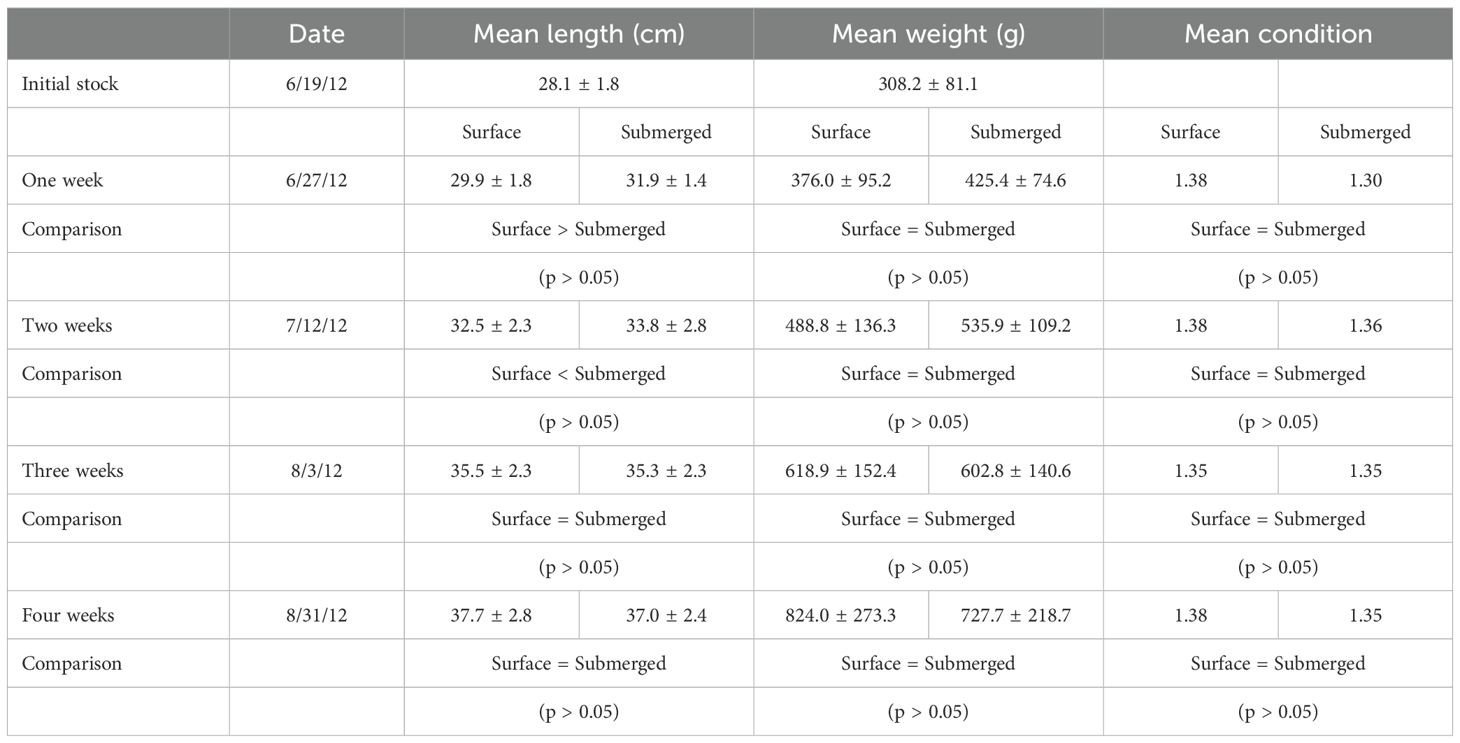
Table 3. Mean weights, lengths, conditions and nose abrasion indices of the fish in the submerged and control cage on each of the sampling dates in Experiment 2.
On both sampling dates, fish that had been submerged jumped more frequently than control (surface maintained) fish (Figure 6). Survival was good in both the surface (88%) and submerged cage (92%) (Figure 7). No statistical comparison was possible due to single replicates, and the cause of death from the few fish that died is unknown.
Because the pitch and roll tags were external, some became snagged in the cage netting, effectively changing their orientation relative to the axes of the fish. For this reason, pitch and roll data from several of the tags was unreliable, and therefore not used.
Experiment 3: exposed site
Results were similar in the 1-, 2-, and 3-week submergence periods (Table 4). There were no significant differences in length, weight, specific growth rates (Table 2) and body abrasions (p > 0.05).
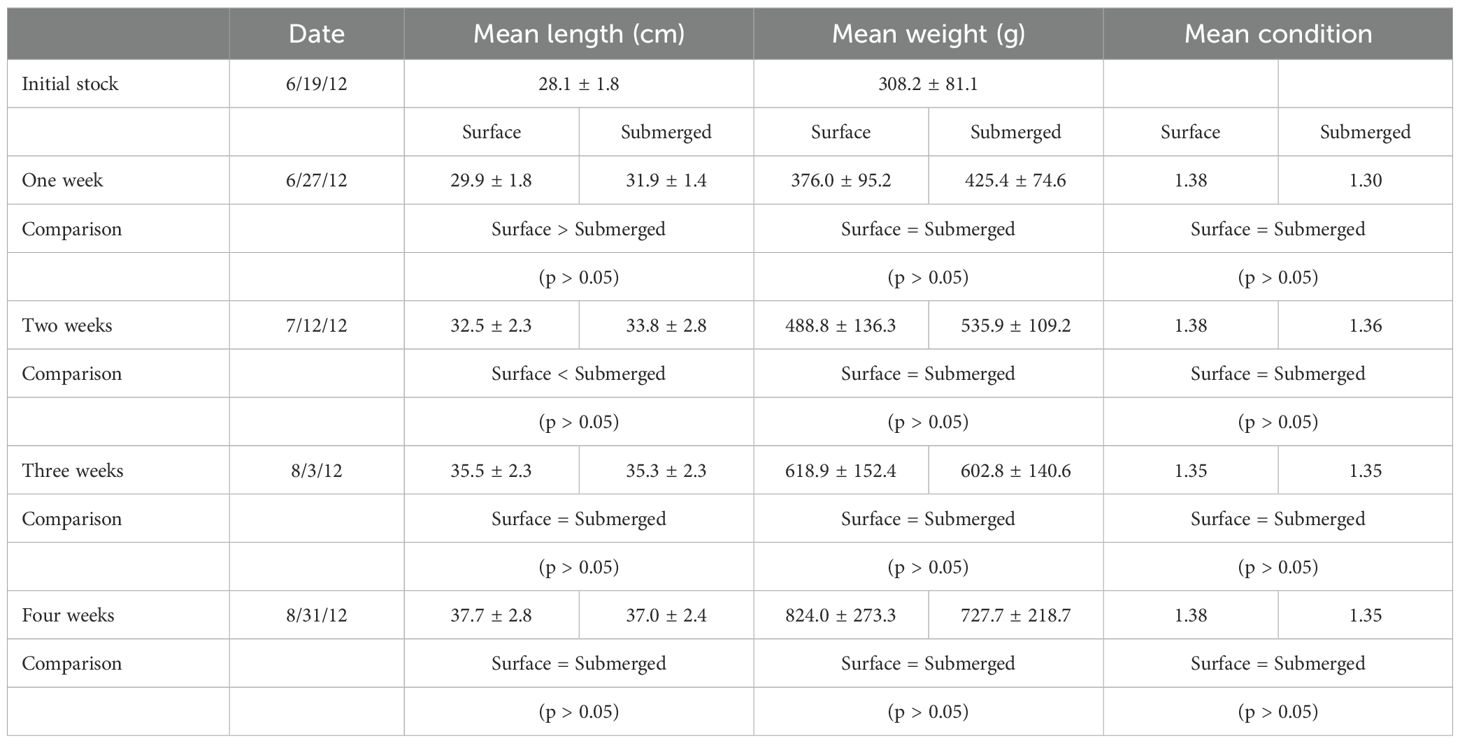
Table 4. Growth and condition index for trout submerged for 1, 2, 3 and 4 weeks during Experiment 3.
Most notable in Experiment 3 was survival and jumping events (upon resurfacing) during the third- and fourth-week submergence trials. At samplings 1 and 2 weeks after submergence, survival was similar at 99% (surface) and 98% (submerged). After 3 weeks submergence, the survival was again similar at 83% and 84% respectfully. A divergence occurred after 4 weeks submergence with a survival of 74% in the surface cage and 43% in the subsurface cage (Figure 7). Also interesting was jumping events decreased when the trout were resurfaced at the third and fourth intervals perhaps indicating that fish in both cages were under stress (Figure 6). During this period, high temperatures (> 16°C) and heavy biofouling of the hydroid Tubularia spp. recruited onto the net and cage frames.
Ethovison XT fish tracking software was used to analyze the underwater video of trout swimming angle past a vertical line suspended within the center of the cages. Results indicated no differences in swimming angle ±3° in both the control and treatment cages.
Discussion
The three experiments conducted to test differences between steelhead trout held in surface verses subsurface cages showed varied results. At the end of Experiments 1 and 2, fish maintained at the surface were significantly heavier than those in submerged cages, but in Experiment 3 there were no differences. These results are similar to those of Korsøen et al. (2009, 2012), who found that salmonids grew slower in cages submerged for longer than one month. Location and cage size may have been responsible for these observed differences. Experiment 1, done with relatively small fish (~300 g), was conducted in small diameter (1.25 m), cylindrical copper-alloy cages at an inshore site exposed to fast tidal currents (0.5 m/s). Snout damage was severe in all treatments, probably because of the small cage size and copper-alloy cage material, and more so in the submerged cages. Submerged fish may have incurred more nose abrasions in trying to reach the surface to access air. Survival was poor in the submerged cages, suggesting that the stresses of snout damage and submergence were additive. The particularly low growth and survival in two-week submergence group was likely due to the additive handling over the eight-week trial and could be mitigated with less sampling in a commercial growout. Furthermore, the increased frequency of jumping in submerged fish can likely be attributed to stress from the prolonged submergence period as well as to fill their depleted swim bladders with air. As a physostomous fish, they need to gulp air to replenish their air in their bladder for buoyancy purposes (Jianfei et al., 2022; Dempster et al., 2009).
In Experiment 2, fish (~1000 g), and cages (68 m3) were larger and made of soft nylon mesh, snout damage was virtually non-existent, and survival was good in both the surface and submerged cage. At the conclusion, surface fish were significantly heavier, indicating that submergence alone can have negative effects on growth performance. It should be noted, however, that fish were submerged for 31 days, which is probably a longer time than would be necessary in an aquaculture application that would avoid a storm, phytoplankton and jellyfish bloom and or warm surface temperatures during the summer (> 16°C). The mechanisms for compromised growth, however, remain elusive. We saw no change in the swimming angle and vertical depth, which would have suggested severe swim bladder depletion as reported by Dempster et al. (2008, 2009) and Korsøen et al. (2009).
In the third experiment, with smaller trout again, 1- and 2-week submergence period indicated significant differences between the surface and subsurface cages. However, during the 3 and 4-week submergence periods, survival and jumping changed significantly in both treatments that may have been brought on by changes in the cage environment. The cause of this was probably warm water temperatures (> 16°C) and heavy biofouling of Tubularia spp. on the nets. Tubularia have stinging nematocysts that can irritate the outer mucous membrane of fish (Figure 8). Anecdotal evidence has shown this to be more of a problem during July and August when surface water temperatures can reach up to 20°C. Secondary bacterial infections caused by stress (temperature and biofouling) may have altered survival rates and jumping behavior. An indication of this stress can be seen in the jumping data in Figure 6. Here, jumping in both cages decreased by the end of the experiment.
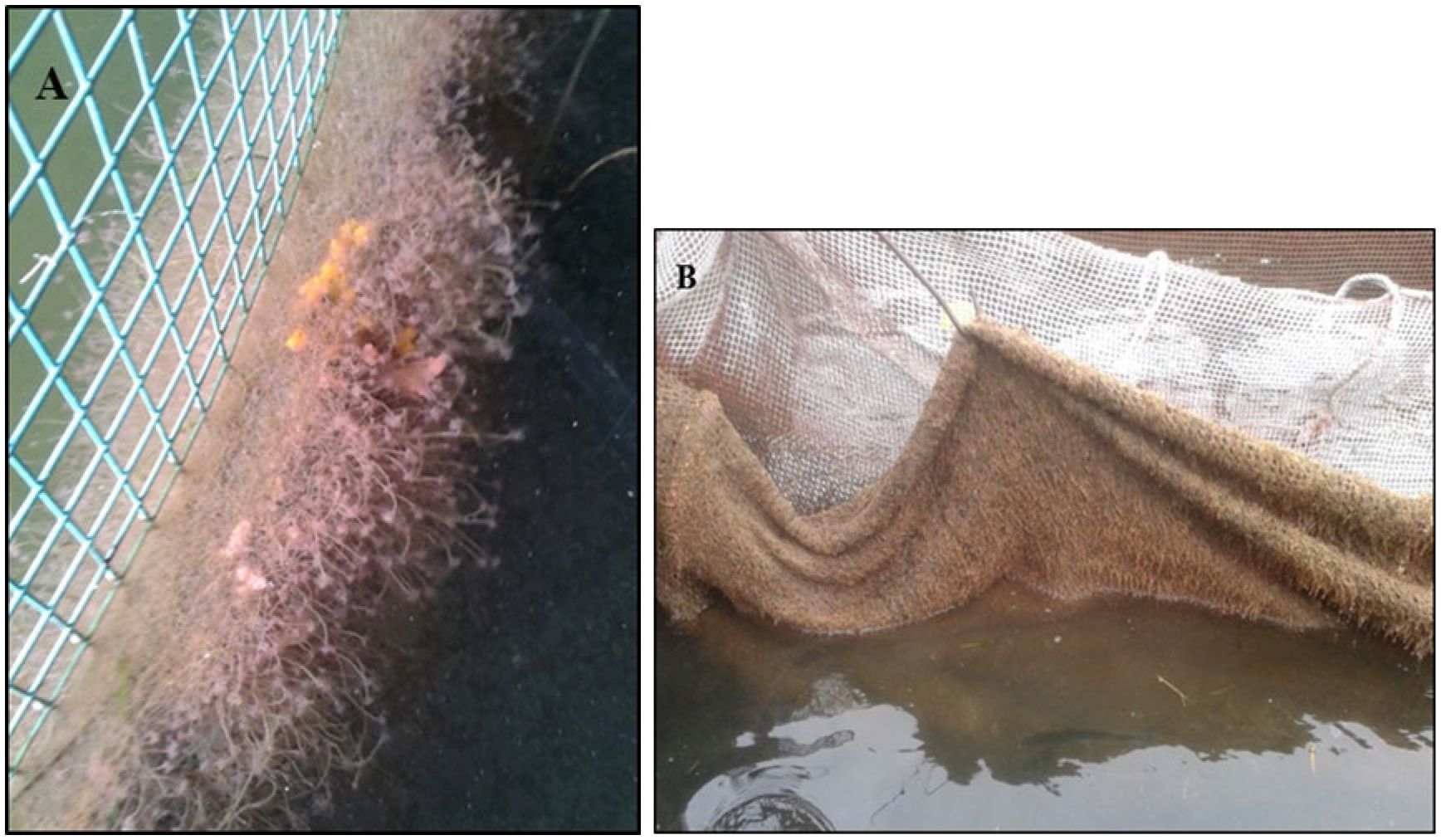
Figure 8. (A) Biofouling of the hydroid Tubularia spp. on a fish net. (B) A heavily fouled net being pulled up for fish sampling.
While we do not have conclusive data, we believe that steelhead trout can be submerged for days to weeks with no negative effects, and this is an important finding for those interested in culturing this species. These conclusions align with those of Dempster et al. (2008, 2009) and Glaropoulos et al. (2019), who found that submergence of Atlantic Salmon for periods less than 21 days had little effect on growth. We were logistically unable to conduct the experiments in deep (> 10 m) water, and hence our submerged cages, while below the surface, were still relatively shallow. Because pressure (depth) may influence the behavior and physiology of forcibly submerged fish, these experiments should be repeated in cages submerged to greater depths (> 10 m) in open ocean conditions. Salmonids spend more than 80 percent of their time in the upper 10 m of the ocean, indicating that there may be physiological implications with increasing depth (Hedger et al., 2017). Test enclosures may have inhibited fish from compensating for negative buoyancy through swimming at sufficient speed to generate hydrodynamic lift through planing (Sfakiotakis et al., 1999). In contrast, sea trials in commercial scale sea-pens (1600–2000 m3) affected salmon behavior, but did not alter growth rates, food conversion ratios, appetite, condition factor or fin condition in comparison with control cages held under similar environmental conditions (Dempster et al., 2009).
Duration of successful submergence will depend on the species, size, temporal season, and depth (Dempster et al., 2008, 2009). However, Oppedal et al. (2020) demonstrated that salmon cultured in a submerged cage with an air dome integrated into the ceiling were able to sustain high growth rates for up to 7 weeks, indicating that regardless of the depth, salmonids need access to air. This study highlights some of the developments in submerged aquaculture technology over the last decade since this study took place. Advancements in materials, mooring systems and buoyancy designs have made net pens more robust and easier to operate while improving animal welfare. As aquaculture moves further offshore, more species are being cultured successfully using submerged pens, demonstrating the advantages of this technology. However, ocean farmed O. mykiss is still exclusively grown in surface cages in the and little research has investigated trout submergence. The results of this study are critical to expanding steelhead trout aquaculture offshore.
Ultimately, cage submergence can be used as an effective management tool to temporarily escape adverse situations at sea. Cage submergence to 10 m below the surface was shown to protect livestock and culture system from extreme weather conditions with 9 m waves (Rillahan et al., 2011; Chambers and Howell, 2006; Chambers et al., 2007). Depth requirements for commercial scale cages will depend on species and exposure to environmental conditions. The additional cost associated with submergence must be taken into consideration by farmers and balanced out with the potential risk of maintaining livestock at the surface. As fish farming expands offshore, it will be important to take advantage of management tools that can measure fish welfare, biology and oceanographic data in real time. With this, farm managers can react appropriately to safeguard their product in varying ocean conditions.
Data availability statement
The raw data supporting the conclusions of this article will be made available by the authors, without undue reservation.
Ethics statement
The animal studies were approved by University of New Hampshire Research Integrity Services, Service Building 51 College Road, Durham, NH 03824-3585. The studies were conducted in accordance with the local legislation and institutional requirements. Written informed consent was obtained from the owners for the participation of their animals in this study.
Author contributions
MCh: Conceptualization, Data curation, Formal analysis, Funding acquisition, Investigation, Methodology, Project administration, Resources, Software, Supervision, Validation, Visualization, Writing – original draft, Writing – review & editing. MCo: Formal analysis, Writing – review & editing. MD: Formal analysis, Writing – review & editing. CR: Conceptualization, Data curation, Formal analysis, Methodology, Software, Visualization, Writing – original draft, Writing – review & editing. PL: Conceptualization, Funding acquisition, Investigation, Project administration, Resources, Validation, Writing – review & editing. WH: Conceptualization, Data curation, Formal analysis, Investigation, Methodology, Project administration, Resources, Supervision, Writing – original draft, Writing – review & editing.
Funding
The author(s) declare financial support was received for the research, authorship, and/or publication of this article. This project was funded by the Research Council of Norway grant no. 193635/I10Norway through SINTEF Fisheries and Aquaculture, Aquaculture Engineering in Trondheim, Norway.
Acknowledgments
I would like to thank the University of New Hampshire staff, and facilities for making this project possible. I would like to thank Gunnar Ek for assistance with marine operations. I would also like to thank the UNH Sea Grant team for sampling equipment and expertise. Finally, I would like to thank Sumner Brook Trout Farm for providing fingerlings for this study.
Conflict of interest
The authors declare that the research was conducted in the absence of any commercial or financial relationships that could be construed as a potential conflict of interest.
Generative AI statement
The author(s) declare that no Generative AI was used in the creation of this manuscript.
Publisher’s note
All claims expressed in this article are solely those of the authors and do not necessarily represent those of their affiliated organizations, or those of the publisher, the editors and the reviewers. Any product that may be evaluated in this article, or claim that may be made by its manufacturer, is not guaranteed or endorsed by the publisher.
References
Akbulut B., Şahin T., Aksungur N., Aksungur M. (2002). Effect of initial size on growth rate of rainbow trout, Oncorhynchus mykiss, reared in cages on the Turkish Black Sea Coast. Turkish J. Fisheries Aquat. Sci. 2 (2), 133–136.
Benetti D., O’Hanlon B., Rivera J., Welch A., Maxey C., Orhun M. (2010). Growth rates of cobia (Rachycentron canadum) cultured in open ocean submerged cages in the Caribbean. Aquaculture 302, 195–201. doi: 10.1016/j.aquaculture.2010.02.021
Bond M. H., Hayes S. A., Hanson C. V., MacFarlane R. B. (2008). Marine survival of steelhead (Oncorhynchus mykiss) enhanced by a seasonally closed estuary. Can. J. Fish. Aquat. Sci. 65, 2242–2252. doi: 10.1139/F08-131
Chambers M. D. (2013). “Integrated multi-trophic aquaculture of steelhead, blue mussels and sugar kelp”, Ph.D. dissertation. Department of Biological Sciences, University of New Hampshire, Durham, NH. Available at: https://www.proquest.com/docview/1508873721
Chambers M. D., Bunker J., Watson W., Howell W. H. (2012). Comparative growth and survival of juvenile Atlantic cod (Gadus morhua) cultured in copper and nylon net pens. J. Aquaculture Res. Dev. 3, 5. doi: 10.4172/2155-9546.1000137
Chambers M. D., Howell W. H. (2006). Preliminary information on cod and haddock production in submerged cages off the coast of New Hampshire, USA. ICES J. Mar. Sci. 63. doi: 10.1016/j.icesjms.2005.10.008
Chambers M. D., Langan R., Howell W., Celikkol B., Watson W., Barnaby R., et al. (2007). Recent developments at the University of New Hampshire open ocean aquaculture site. Bull. Aquacul. Assoc. Canada, 105–103.
Chambers M. D., Ostrowski A. C. (1999). “Development of bluefin trevally (Caranx melampygus) and greater amberjack (Seriola dumerili) for offshore aquaculture,” in Joining Forces with Industry: Proceedings of the Third International Conference on Open Ocean Aquaculture, Corpus Christi, Texas (USA), May 10–15.
D’Agaro E., Gibertoni P., Esposito S. (2022). Recent trends and economic aspects in the rainbow trout (Oncorhynchus mykiss) sector. Appl. Sci. 12, 8773. doi: 10.3390/app12178773
Dadswell M., Spares A., Reader J., McLean M., McDermott T., Samways K., et al. (2022). The decline and impending collapse of the Atlantic Salmon (Salmo salar) population in the North Atlantic Ocean: a review of possible causes. Rev. Fisheries Sci. Aquaculture 30, 215–258. doi: 10.1080/23308249.2021.1937044
Dempster T., Juell J.-E., Fosseidengen J. E., Fredheim A., Lader P. (2008). Behavior and growth of Atlantic salmon (Salmo salar L.) subjected to short-term submergence in commercial scale sea-cages. Aquaculture 276, 103–111. doi: 10.1016/j.aquaculture.2008.01.018
Dempster T., Korsøen Ø., Folkedal O., Juell J. E., Oppedal F. (2009). Submergence of Atlantic salmon (Salmo salar L.) in commercial scale sea-cages: a potential short-term solution to poor surface conditions. Aquaculture 288, 254–263. doi: 10.1016/j.aquaculture.2008.12.003
FAO (2022). The state of world fisheries and aquaculture 2022. Towards Blue Transformation. Rome, FAO. doi: 10.4060/cc0461en
Fredriksson D. W., DeCew J., Swift M. R., Tsukrov I., Chambers M. D., Celikkol B. (2004). The design and analysis of a four-cage grid mooring for open ocean aquaculture. Aquacultural Eng. 32, 77–94. doi: 10.1016/j.aquaeng.2004.05.001
Furevik D. M., Bjordal Å., Huse I., Fernö A. (1993). Surface activity of Atlantic salmon (Salmo salar L.) in net pens. Aquaculture 110, 119–128. doi: 10.1016/0044-8486(93)90266-2
Glaropoulos A., Stien L. H., Folkedal O., Dempster T., Oppedal F. (2019). Welfare, behaviour and feasibility of farming Atlantic salmon in submerged cages with weekly surface access to refill their swim bladders. Aquaculture 502, 332–337. doi: 10.1016/j.aquaculture.2018.12.065
Hayes S. A., Bond M. H., Hanson C. V., Jones A. W., Ammann A. J., Harding J. A., et al. (2011). Down, up, down and “smolting” twice? Seasonal movement patterns by juvenile steelhead (Oncorhynchus mykiss) in a coastal watershed with a bar closing estuary. Can. J. Fisheries Aquat. Sci. 68, 1341–1350. doi: 10.1139/f2011-062
Hedger R. D., Rikardsen A. H., Strøm J. F., Righton D. A., Thorstad E. B., Næsje T. F. (2017). Diving behaviour of Atlantic salmon at sea: effects of light regimes and temperature stratification. Mar. Ecol. Prog. Ser. 574, 127–140. doi: 10.3354/meps12180
Hennessey T., Healey M. (2000). Ludwig’s ratchet and the collapse of New England groundfish stocks. Coast. Manage. 28, 187–213.
Hevrøy E. M., Boxaspen K., Oppedal F., Taranger G. L., Holm J. C. (2003). The effect of artificial light treatment and depth on the infestation of the sea louse Lepeophtheirus salmonis on Atlantic salmon (Salmo salar) culture. Aquaculture 220, 1–14.
Hinshaw J. M., Fornshell G., Kinnunen R. (2004). A profile of the aquaculture of trout in the United States (Mississippi, USA: USDA Risk Management Agency, Mississippi State University).
Howell W. H., Chambers M. D. (2005). Growth performance and survival of Atlantic halibut (Hippoglossus hippoglossus) grown in submerged net pens. Bull. Aqua. Assoc. Canada 9, 35–37.
Jianfei Yu, Dong S., Zhou Y., Guo Y., Gao Q., Dong Y. (2022). Effects of different types of air supplementation on rainbow trout confined underwater. Aquacultural Eng. 96, 102214. doi: 10.1016/j.aquaeng.2021.102214
Johansson D., Juell J. E., Oppedal F., Stiansen J. E., Ruohonen K. (2007). The influence of the pycnocline and cage resistance on current flow, oxygen flux and swimming behaviour of Atlantic salmon (Salmo salar L.) in production cages. Aquaculture 265, 271–287. doi: 10.1016/j.aquaculture.2006.12.047
Johansson D., Ruohonen K., Kiessling A., Oppedal F., Stiansen J. E., Kelly M., et al. (2006). Effect of environmental factors on swimming depth preferences of Atlantic salmon (Salmo salar L.) and temporal and spatial variations in oxygen levels in sea cages at a fjord site. Aquaculture 254, 594–605. doi: 10.1016/j.aquaculture.2005.10.029
Korsøen Ø., Dempster T., Fjelldal P. G., Oppedal F., Kristiansen T. (2009). Long-term culture of Atlantic salmon (Salmo salar L.) in submerged cages during winter affects behavior, growth and condition. Aquaculture 296, 373–381. doi: 10.1016/j.aquaculture.2009.08.036
Korsøen O. J., Dempster T., Oppedal F., Kristiansen T. S. (2012). Individual variation in swimming depth and growth in Atlantic salmon (Salmo salar L.) subjected to submergence in sea-cages. Aquaculture 334, 142–151. doi: 10.1016/j.aquaculture.2011.12.031
Milich L. (1999). Resource mismanagement versus sustainable livelihoods: the collapse of the Newfoundland cod fishery. Soc. Natural Resour. 12, 625–642. doi: 10.1080/089419299279353
Naylor R., Burke M. (2005). Aquaculture and ocean resources: Raising tigers of the sea. Annu. Rev. Environ. Resour. 30, 185–218. doi: 10.1146/annurev.energy.30.081804.121034
Oppedal F., Dempster T., Stien L. (2011). Environmental drivers of Atlantic salmon behavior in sea-cages. Aquaculture 311, 1–18. doi: 10.1016/j.aquaculture.2010.11.020
Oppedal F., Folkedal O., Stien L., Vagseth T., Fosse J. O., Dempster T., et al. (2020). Atlantic salmon cope in submerged cages when given access to an air dome that enables fish to maintain neutral buoyancy. Aquaculture 525, 735286. doi: 10.1016/j.aquaculture.2020.735286
Oppedal F., Juell J. E., Johansson D. (2007). Thermo- and photo-regulatory swimming behavior of caged Atlantic salmon: Implications for photoperiod management and fish welfare. Aquaculture 265, 70–81. doi: 10.1016/j.aquaculture.2007.01.050
Oppedal F., Juell J. E., Taranger G. L., Hansen T. (2001). Artificial light and season affects vertical distribution and swimming behavior of post-smolt Atlantic salmon in sea cages. J. Fish. Biol. 58, 1570–1584. doi: 10.1111/j.1095-8649.2001.tb02313.x
Osland H., Sandvik J. I., Holm J., Heuch P.-A., Bakke S. (2001). Studie av lakseluspåslag og tilvekst hos Atlantisk laks (Salmo salar) i nedsenkede merder. HSF-report, R-NR 4/01 (Norwegian: Hogskulen 1 Sogin Og Fjordane), 22.
Papandroulakis N., Mesa-Rodriguez A., Anastasiadis P., Lisac D., Asderis M., Pavlidis M. (2013). Installation, operation and evaluation of a submerged cage at 45M depth in Crete for the rearing of red porgy Pagrus pagrus. Aquaculture Res. 44, 1196–1205. doi: 10.1111/j.1365-2109.2012.03119.x
Rapp P., Ramírez W. R., Rivera J. A., Carlo M., Luciano R. (2007). Measurement of organic loading under an open ocean aquaculture cage, using sediment traps on the bottom. J. App Ichthyol. 23, 661–667. doi: 10.1111/j.1439-0426.2007.00900.x
Rillahan C., Chambers M. D., Howell W. H., Watson W. H. (2011). The behavior of cod (Gadus Morhua) in an offshore aquaculture net pen. Aquaculture 310, 361–368. doi: 10.1016/j.aquaculture.2010.10.038
Rillahan C., Chambers M. D., Watson W. H., Howell W. H. (2009). A self- contained system for observing and quantifying the behavior of Atlantic cod, Gadus morhua, in an offshore aquaculture cage. Aquaculture 293, 49–56. doi: 10.1016/j.aquaculture.2009.04.003
Ryan J. (2004). Farming the Deep Blue. Report to the Irish Sea Fisheries Board and the Irish Marine Institute. 67.
Sfakiotakis M., Lane D. M., Davies J. B. C. (1999). Review of fish swimming modes for aquatic locomotion. IEEE J. Oceanic Eng. 24. doi: 10.1109/48.757275
Sievers M., Korsøen Ø., Warren-Myers F., Oppedal F., Macaulay G., Folkedal O., et al. (2022). Submerged cage aquaculture of marine fish: A review of the biological challenges and opportunities. Rev. Aquaculture 14, 106–119. doi: 10.1111/raq.12587
Tamaru C. S., Carlstrom-Trick C., Helsley C. E. (1998). “Aquaculture in Hawaii: lessons from the past,” in Open Ocean Aquaculture’97 Charting the Future of Ocean Farming Proceedings of an International Conference, April 23-25, 1997, Maui, Hawaii, University of Hawaii Sea Grant College Program, Honolulu, HI. 257–278.
Tsukrov I., Ozbay M., Fredriksson D. W., Swift M. R., Baldwin K., Celikkol B. (2000). Open ocean aquaculture engineering: numerical modeling. Mar. Technol. Soc. J. 34, 29–40. doi: 10.4031/MTSJ.34.1.4
Keywords: open ocean aquaculture, submergence, Oncorhynchus mykiss, open ocean, submerged aquaculture
Citation: Chambers M, Coogan M, Doherty M, Rillahan C, Lader P and Howell WH (2025) Feasibility and challenges of submerged culture of steelhead trout Oncorhynchus mykiss for open ocean aquaculture in the United States. Front. Aquac. 3:1506210. doi: 10.3389/faquc.2024.1506210
Received: 07 October 2024; Accepted: 31 December 2024;
Published: 06 February 2025.
Edited by:
Gouranga Biswas, Central Institute of Fisheries Education (ICAR), IndiaReviewed by:
Daniel Matulić, University of Zagreb, CroatiaCosmas Nathanailides, University of Ioannina, Greece
Copyright © 2025 Chambers, Coogan, Doherty, Rillahan, Lader and Howell. This is an open-access article distributed under the terms of the Creative Commons Attribution License (CC BY). The use, distribution or reproduction in other forums is permitted, provided the original author(s) and the copyright owner(s) are credited and that the original publication in this journal is cited, in accordance with accepted academic practice. No use, distribution or reproduction is permitted which does not comply with these terms.
*Correspondence: Michael Chambers, TWljaGFlbC5DaGFtYmVyc0B1bmguZWR1