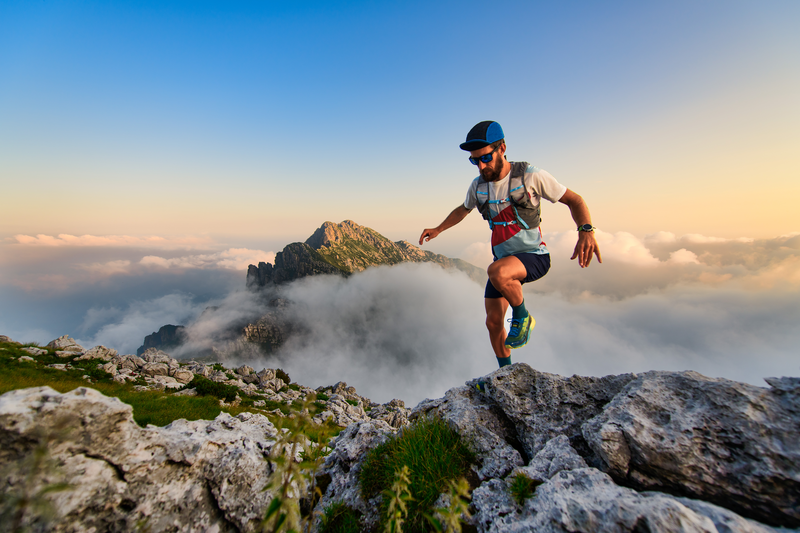
94% of researchers rate our articles as excellent or good
Learn more about the work of our research integrity team to safeguard the quality of each article we publish.
Find out more
ORIGINAL RESEARCH article
Front. Aquac. , 05 December 2024
Sec. Disease and Health Management
Volume 3 - 2024 | https://doi.org/10.3389/faquc.2024.1496943
This article is part of the Research Topic Aquatic Animal Health and Epidemiology: Disease Surveillance, Prevention and Control View all 6 articles
Introduction: Yellow mouth disease, caused by Tenacibaculum maritimum, is an important disease of farmed salmonids. Disease management currently necessitates the use of antimicrobials, raising concerns about antimicrobial resistance (AMR) in aquatic and potentially terrestrial environments. Identifying management, production, environmental, and other factors associated with the development of yellow mouth in salmonids will help to elucidate disease control strategies and decrease the economic and environmental burden of its treatment. The objective of this scoping review was to synthesize the available literature to identify factors associated with disease in farmed and wild salmonids from T. maritimum.
Methods: The scoping review followed the framework outlined in the Joanna Briggs Institute Reviewer’s Manual and PRSIMA-ScR reporting guidelines. The protocol was developed a priori in consultation with a librarian and was used to search Environment Complete®, Earth, Atmospheric, and Aquatic Science®, Scopus®, and Web of Science™ databases on July 21, 2022, and again on April 27, 2023. Articles were included if they focused on T. maritimum infection in salmonids and discussed factors (environmental, management, or other) that impacted the disease and/or organism of interest.
Results: Twenty-five articles were included for review. Over half were published within the last five years (n=14/25). The included articles revealed a complex interplay of salmonid (host)-specific factors (age/size), management practices (vaccination, marine transfer, stocking density, gill/body abrasion), environmental conditions (water temperature, oxygenation, salinity, algal blooms, vectors), and microbial dynamics (load, co-infections, strain, biofilms, microbiome) influencing T. maritimum infections. Only one study conducted multivariable analysis to understand this complex interplay between the diverse factors that impact infection with T. maritimum.
Discussion: The review highlights the complex, multifactorial nature of T. maritimum infections, including the interplay of host biology, environmental factors, and pathogen characteristics. A comprehensive approach incorporating both management and environmental components is essential to mitigate T. maritimum infections in salmonid production.
Food insecurity is growing due to the increasing global population and challenges like the COVID-19 pandemic (Food and Agriculture Organization of the United Nations, 2022). As the world’s population continues to grow, demand for food also rises (Food and Agriculture Organization of the United Nations, 2022). Subsequently, the demand for seafood is rising and finfish is becoming a popular source of protein, accounting for 16.6 percent of global animal protein intake (Food and Agriculture Organization of the United Nations, 2022). Consumption of aquatic foods has expanded at an average of three percent per year between 1961 and 2019 (twice the rate of population growth) (Food and Agriculture Organization of the United Nations, 2022). Due to the environmental limitations associated with wild-capture fisheries, aquaculture farms are increasing production to meet the growing demand, and account for 56 percent of total aquatic animal food supply (Food and Agriculture Organization of the United Nations, 2022). These production systems are uniquely suited to meet the goals of the United Nations 2030 Agenda for Sustainable Development (UN General Assembly, 2015). As salmonid farming operations across the globe increase output to supply the growing demand, subsequent challenges such as bacterial diseases emerge (Asche et al., 2018; Ojasanya et al., 2022).
Bacterial diseases are a major challenge affecting both the sustainability and economic stability of finfish farming (Gourzioti et al., 2016). To combat these bacterial diseases, producers administer antimicrobials through medicated feed (Morrison and Saksida, 2013). Vaccines have been successful in reducing the need for antimicrobials in common bacterial diseases in finfish, but are ineffective against yellow mouth (Morrison and Saksida, 2013). The causative agent of this disease is Tenacibaculum maritimum, an opportunistic bacterium associated with many fish species worldwide (Frisch et al., 2018). Infection with T. maritimum can also result in tenacibaculosis (formerly known as marine flexibacteriosis); a more widespread and clinically unique disease characterized by frayed fins, tail rot, and ulcerative lesions (Toranzo et al., 2005; Fernandez-Alvarez and Santos, 2018; Frisch et al., 2018). In contrast to tenacibaculosis, yellow mouth is specific to the western Pacific coast in British Columbia (BC), Canada, and Washington state (Frisch et al., 2018). Mortality rates in BC farmed salmon attributed to yellow mouth can be as high as 15% (Ostland et al., 1999), with an economic burden that has been estimated to be $1.6 million per year for a single company (Wade and Weber, 2020). Since there is no commercially available vaccine to protect salmonids from yellow mouth, antimicrobials such as florfenicol and potentiated sulfonamides are prescribed to treat and control the disease (Morrison and Saksida, 2013). Antimicrobial use (AMU) in salmonid production in BC is largely attributed to the treatment and control of yellow mouth (Morrison and Saksida, 2013). For example, in 2011, 98% of the antimicrobials prescribed for BC aquaculture operations were written for bacterial stomatitis (Morrison and Saksida, 2013). This suggests that AMU could be dramatically reduced in western Canadian aquaculture if the disease could be prevented by other means, but little is known about management, production, environmental, and other factors contributing to its incidence, and subsequent risk reduction strategies (Wade and Weber, 2020).
Although many factors may be associated with the development of disease caused by T. maritimum, a search of Web of Science, Scopus, Environment Complete, and Earth, Science & Aquatic Collection on June 15th, 2022, and April 27, 2023, did not identify a systematic or scoping review on this topic. The objective of this scoping review study was to synthesize the range of existing research on the factors associated with disease in farmed and wild salmonids caused by T. maritimum infection.
This scoping review follows the framework outlined in the Joanna Briggs Institute Reviewer’s Manual (Aromataris, 2017) and was reported according to the PRISMA-ScR guidelines (Tricco et al., 2018; Aromataris, 2017).
A comprehensive search strategy was developed with the assistance of Janice Kung - a librarian at the University of Alberta - to identify articles that reported factors that contribute to T. maritimum infection in farmed and wild salmonids. This a priori review protocol and all amended protocols were time stamped and are accessible through Open Science Framework (Wassmuth, 2023). Search strings (Table 1) (Wassmuth, 2023) were used to search Environment Complete®, Earth, Atmospheric, and Aquatic Science®, Scopus®, and Web of Science™ databases on July 21, 2022, and again on April 27, 2023.
To be included, studies had to report that they were focused on T. maritimum infection in salmonids and factors (environmental, management, or other) that impact the disease and/or organism of interest. Factors were defined as observations that were hypothesized or measured to have a relationship with infection from T. maritimum. The search strings did not include a factor component; instead, this was assessed during screening to ensure that all relevant articles were captured for screening. No search restrictions were placed on language, publishing date, or geography. Review articles, conference abstracts, preprints, books, book chapters, theses, dissertations, commentaries, opinion pieces, editorials, and newspaper articles were excluded.
Articles were screened for eligibility via a two-stage process by two independent reviewers. Article titles, abstracts, and keywords were screened in the first stage, with articles proceeding to secondary screening if both reviewers determined that they fully met the inclusion criteria or were unclear. Secondary screening utilized a “1 in 2 out” procedure, where all articles were screened by a primary reviewer. If the reviewer included the article, it was automatically included in the review, if the reviewer excluded the article, it was screened by a secondary reviewer to confirm exclusion. This second screening round protocol amendment was reflected in the protocol which was uploaded onto Open Science Framework on September 19, 2022. After the second search was conducted on April 27, 2023, the amended protocol with search results were uploaded on May 3, 2023. Screening conflicts were resolved by discussion between reviewers, with a third independent reviewer available to resolve conflicts if required. Google translate was used to translate any non-English article for screening.
All articles retrieved from the database search were downloaded into EndNote X9 for automatic and manual removal of duplicates. The remaining articles were uploaded to DistillerSR® (Evidence Partners, Ottawa, ON, Canada) and automatically removed/deduplicated at a confidence level of 80%; manual deduplication was also performed during screening. Data extraction was performed by a single primary reviewer after consultation. Citation data, study location, year(s) of data collection, study type, characteristics of host (salmonid) and bacteria (T. maritimum), disease diagnosis technique, clinical presentation of infection, description of factors identified, and how those factors impact the disease and/or bacteria of interest were all extracted. Article information was collected in DistillerSR® and exported to a pre-developed data extraction form within Excel (Microsoft, Redmond, WA) for further interpretation. A narrative approach was used for data synthesis.
The primary search on July 21, 2022, identified 1,974 articles. A secondary search April 27, 2023, identified an additional 79 articles. After deduplication, 1,555 articles went to primary screening, where 1,437 articles were excluded, with the main reasons being that the research did not focus on T. maritimum (n=1,214) or salmonids (n=170). Of the 125 full-text articles identified for secondary screening, 7 were excluded during full text retrieval. Three of these were grey literature or book chapters. Three non-English articles did not meet the screening criteria. One article (a one-page abstract) was inaccessible after an extensive search through our institutional library and interlibrary loan program. After secondary screening, 93 articles were excluded for not pertaining to research on T. maritimum (n=45), salmonids (n=6), factors of interest (n=27), or was not primary research (n=15). Following all stages of screening, 25 studies were eligible for data extraction (Figure 1). All extracted data are provided in Supplementary Table 1.
Figure 1. PRISMA scoping review flow diagram of the study selection process for the scoping review of salmonid infection with Tenacibaculum maritimum.
Most of the 25 included articles were published between 2017 to 2023 (n=14/25), but dated back to 1994 (Table 2, Supplementary Table 1). The study designs ranged from experimental (n=10/25) to longitudinal (n=7), randomized controlled trials (n=3), cross-sectional (n=2), and case reports (n=3). All included articles originated from high-income countries (UN Department of Economic and Social Affairs, 2022) with Australia (n=7) and Canada (n=8) representing the highest proportions. Most studies reported on Atlantic salmon as one of the salmonid species of interest (n=21). Disease caused by T. maritimum was defined as yellow mouth (n=9), tenacibaculosis (n=9), marine flexibacteriosis (n=2), acute gill disease (n=1), or not defined (n=3).
Table 2. Key characteristics of peer-reviewed articles included in the scoping review of factors related to salmonid infection with Tenacibaculum maritimum.
Several thematic categories were reported for factors that related to T. maritimum infection, including salmonid (n=6), management (n=15), environmental (n=16), and microbial factors (n=15) (Table 3, Supplementary Table 1). Most studies reported on more than one factor of interest. Out of the 25 studies included in the review, 14 did not run formal statistical comparisons. Multivariable analysis was conducted in only one study to examine the impact of temperature, salinity, and oxygenation at different depths with florfenicol use (Nowlan et al., 2021a). The remainder of the studies used statistical analysis to characterize a change in one parameter with a significant increase or decrease in another outcome variable.
Table 3. Thematic breakdown of all articles included in the scoping review of factors related to salmonid infection with Tenacibaculum maritimum according to their corresponding factors of interest.
Six articles reported on one or more salmonid factors that contributed to infection with T. maritimum (Table 3, Supplementary Table 1) (Soltani et al., 1996; Handlinger et al., 1997; van Gelderen et al., 2011; Downes et al., 2018; Bateman et al., 2021; Bass et al., 2022). Two studies reported an increased prevalence of infection during the first year of production at sea when the fish are smaller (Downes et al., 2018; Bateman et al., 2021), with a possible factor being the softness of scales at a young age (van Gelderen et al., 2011). In one study, the size of fish was investigated by comparing the mass of wild fish (kg) to their current length (cm) during their first year at sea (Bass et al., 2022). Authors reported that fish with a higher bacterial load of T. maritimum had a lower-than-expected mass for their length, which was identified as a function of decreased feeding rates and feed consumption in fish infected with the bacteria (Bass et al., 2022).
A challenge trial to assess the fish species effect found that a T. maritimum challenge concentration of 1.6 x 106 cells/mL was significantly (p<0.05) associated with higher mortality in Atlantic salmon (74.9%) compared to Rainbow trout (50.0%); there were no differences at other challenge concentrations (1.8 x 103, 2.3 x 104, 2.3 x 105, and 1.6 x 107 cells/mL) (Soltani et al., 1996). When salmonids were compared to non-salmonids such as greenback flounder, there was significantly higher mortality (10% vs 2%) and morbidity with lesions (20% vs 0%) in Atlantic salmon (Soltani et al., 1996). Another study reported species-specific variability in the response to infection with T. maritimum (Handlinger et al., 1997). Greenback flounder showed mild to moderate erosions on the fins and tail with minimal histological lesions compared to the higher susceptibility and more severe lesions observed in rainbow trout and Atlantic salmon (Handlinger et al., 1997).
Most of the 15 articles that mentioned management factors (Table 3, Supplementary Table 1) investigated gill/body abrasion (n=8) (Handlinger et al., 1997; Powell et al., 2004; Powell et al., 2005; Olsen et al., 2011; van Gelderen et al., 2011; Apablaza et al., 2017; Smage et al., 2017; Nowlan et al., 2021a) or pen elements (netting, cleaning systems) (n=3) (van Gelderen et al., 2011; Rud et al., 2017; Nowlan et al., 2021a). Abrasion of the gills was reported to disturb respiration, which ultimately enhanced the progression of disease from T. maritimum (Powell et al., 2004; Apablaza et al., 2017; Wynne et al., 2020). Bodily abrasion, which could be a result of contact with netting or other pen elements, was reported to enhance the rate and severity of infection (Olsen et al., 2011; van Gelderen et al., 2011). Skin lesions were commonly found in areas that were more prone to abrasion or movement, such as dorsal and pectoral fins (van Gelderen et al., 2011). Other studies reported that abrasion allowed bacterial proliferation below the epidermis (van Gelderen et al., 2011) and that the infiltration of T. maritimum was restricted to necrotic tissue (Handlinger et al., 1997). One article reported that infection with T. maritimum appeared to occur only after abrasion of the skin (Olsen et al., 2011). However, another study reported that at high concentrations, the fish became infected with T. maritimum and died after 72 hours with no prior abrasion to the epithelium, and little sign of erosion (van Gelderen et al., 2011).
Contact with pen elements (van Gelderen et al., 2011), other fish (van Gelderen et al., 2011), or jellyfish (Smage et al., 2017), or net pen cleaning (Nowlan et al., 2021a), could have introduced T. maritimum, as it was reported that T. maritimum exists in several reservoirs such as tank walls, net pens, and water samples (van Gelderen et al., 2011; Rud et al., 2017; Nowlan et al., 2021a). Studies also reported on transfer from freshwater to saltwater (Smage et al., 2017; Nowlan et al., 2021a), and time since transfer to saltwater between three to eight weeks (Frelier et al., 1994), and one week and one year, as factors for T. maritimum infection (Downes et al., 2018; Nowlan et al., 2021a). The prevalence of T. maritimum in dead and dying salmon (from all causes) was also found to be the highest in the first year after ocean entry (Bateman et al., 2021). Other management factors such as vaccines were examined (van Gelderen et al., 2009; Frisch et al., 2018). A vaccine developed for yellow mouth using isolates from western Canada was reported to be unsuccessful in protecting fish under experimental challenge conditions (Frisch et al., 2018). In another vaccination study on marine flexibacteriosis, authors reported that naïve Atlantic salmon had significantly better survival rates when injected with vaccine and adjuvant than the control group or vaccine only group (van Gelderen et al., 2009).
Environmental factors were reported by 16 of the included studies (Tables 3–5, Supplementary Table 1). One study reported that infection with T. maritimum did not always result in clinical signs, therefor other environmental factors might be necessary to result in clinical disease (Brosnahan et al., 2019). Water temperature was the most frequently reported environmental factor (n=10) (Tables 3, 4) (Frelier et al., 1994; Handlinger et al., 1997; Barker et al., 2009; Olsen et al., 2011; van Gelderen et al., 2011; Apablaza et al., 2017; Downes et al., 2018; Brosnahan et al., 2019; Nowlan et al., 2021a; Ghosh et al., 2022). An increased water temperature was reported to result in a greater prevalence of T. maritimum in the gill arches of farmed salmon (Downes et al., 2018) or an increased frequency of application of florfenicol treatments (Nowlan et al., 2021a). The remaining eight articles reported a potential connection between water temperature and T. maritimum (Frelier et al., 1994; Handlinger et al., 1997; Barker et al., 2009; Olsen et al., 2011; van Gelderen et al., 2011; Apablaza et al., 2017; Brosnahan et al., 2019; Ghosh et al., 2022). Some studies reported an increased number of disease outbreaks of disease caused by T. maritimum during periods of warmer water temperatures (Barker et al., 2009; Olsen et al., 2011; Downes et al., 2018). One study reported that infection during periods of warmer water temperature was the result of increased stress on fish and increased bacterial growth (van Gelderen et al., 2011). In contrast, one study reported that the prevalence of T. maritimum did not appear to have a strong correlation with warmer water, although no statistical analysis was presented (Brosnahan et al., 2019). Another reported an overgrowth of Tenacibaculum species in the fecal microbiota of salmonids undergoing low-temperature water treatment, however, the species could not be confirmed as T. maritimum (Ghosh et al., 2022). Increases in water temperature were reported to be associated with algal blooms (van Gelderen et al., 2011; Apablaza et al., 2017), which have been hypothesized to be a risk factor for T. maritimum infection.
Table 4. Key findings and significance of temperature as an environmental factor from articles included in the scoping review of salmonid infection with Tenacibaculum maritimum.
Table 5. Key findings and significance of other environmental factors from articles included in the scoping review of salmonid infection with Tenacibaculum maritimum.
Seasonality was reported in numerous studies and relationships to other factors such as water temperature, salinity, and dissolved oxygen were considered (n=7) (Tables 3, 5) (Frelier et al., 1994; Barker et al., 2009; van Gelderen et al., 2011; Downes et al., 2018; Nowlan et al., 2021a; Bass et al., 2022). Ultraviolet irradiation from the sun was reported as a possible cause of skin lesions that then propagate the growth of T. maritimum (Handlinger et al., 1997). One study found that increased numbers of all Tenacibaculum species, higher fish mortality, and increased tenacibaculosis outbreaks were recorded in the spring and summer compared to the fall and winter months (Nowlan et al., 2021a). Mortalities and antimicrobial applications to treat yellow mouth outbreaks during the spring and summer months were also identified to be indirectly correlated with increased temperature and decreased dissolved oxygen (Nowlan et al., 2021a). Another study reported decreased dissolved oxygen to be an environmental stressor (Apablaza et al., 2017) that could result from events such as algal blooms, contributing to an increased prevalence of infection with T. maritimum. The prevalence of T. maritimum found in sea lice surrounding farmed salmonid pens was also highest in the summer at times with the highest water temperature and lowest dissolved oxygen (Nowlan et al., 2021a). Another study stated that decreased levels of T. maritimum in Chinook salmon were associated with decreased mortality in fall and winter (Bass et al., 2022).
An increase in water salinity was reported as another factor associated with the application of antimicrobials (Nowlan et al., 2021a). Outbreaks have been described to occur during periods where salinity levels were between 29-32% (Frelier et al., 1994). One study reported that they found no association between the bacterial load of T. maritimum in salmonid-parasitizing sea lice and changes in water salinity, however, the statistical significance of these results was not reported (Barker et al., 2009).
Vectors of T. maritimum have also been reported as factors of infection. Horizontal transmission of T. maritimum between smolts has been experimentally reported (Frisch et al., 2018). Other proposed vectors include sea lice (Barker et al., 2009; Llewellyn et al., 2017; Nowlan et al., 2021a), lumpsuckers (Frisch et al., 2018), and from farmed onto wild salmonids (Bateman et al., 2022). One study reported that salmon infected with sea lice (Lepeophtheirus salmonis) did not have increased levels of T. maritimum compared to control fish (Llewellyn et al., 2017). Jellyfish have also been proposed as a vector, however, two studies reported that they were not suspected as the original source of T. maritimum infection (Smage et al., 2017; Downes et al., 2018). However, one article reported that jellyfish species do have the ability to carry T. maritimum and deposit it on or into the epidermis of fish upon contact (Ferguson et al., 2010).
Microbial factors were reported by 14 of the included studies (Tables 3, 6, Supplementary Table 1). Several studies reported that T. maritimum is a natural part of the microbial community on the surface of salmonid skin, mucosal layer, and the oral cavity (van Gelderen et al., 2011; Reid et al., 2017; Wynne et al., 2020; Nowlan et al., 2021a; Ghosh et al., 2022). One study also reported that T. maritimum was able to form a biofilm on different surfaces such as tank walls (Frisch et al., 2018). Reports also suggested that endotoxins may be involved in the pathogenesis of disease from T. maritimum, since there was a lack of inflammatory markers found at the site of lesions (Handlinger et al., 1997; van Gelderen et al., 2011).
Table 6. Key findings and significance of microbial factors from articles included in the scoping review of salmonid infection with Tenacibaculum maritimum.
Fish microbiota has been reported to shift according to stressors such as temperature or co-infection, which may predispose fish to growth of T. maritimum (Reid et al., 2017). The amount of T. maritimum exposure, or bacterial load, has been reported as a possible factor for infection (Powell et al., 2004; van Gelderen et al., 2011). In two experimental studies, higher concentrations (2.3 x 105 cells/mL & 1 x 108 cells/mL) of T. maritimum in a bath challenge at constant salinity and temperature were reported to result in 100% mortality from yellow mouth within 3 days, whereas lower concentrations (< 2.3 x 105 cells/mL) only resulted in mortalities after days to weeks (Soltani et al., 1996; van Gelderen et al., 2011).
In skin lesions on the dorsal and pectoral fins, it was observed that T. maritimum was restricted to the necrotic areas of the epithelium and did not infiltrate the musculature of salmon at any concentration (van Gelderen et al., 2011). The size of skin lesions was reported to be smaller in challenges with higher doses, and larger as doses decreased, although in the highest dose (1 x 108 cells/mL) lesions across dorsal, lateral, and pectoral areas were found in similar percentages as the other doses (van Gelderen et al., 2011). This finding was similarly reported in another study, which stated that superficial lesions were common in early mortalities with a higher challenge concentration (2.3 x 105 – 1.6 x 107 cells/mL) and later mortalities had eroded ulcers (Soltani et al., 1996). Another study reported that wild salmon with a higher T. maritimum load had reduced lower-than-expected mass for their measured length (Bass et al., 2022).
Co-infection with other pathogens such as salmonid alphavirus (SAV) has been reported to increase the bacterial load of Tenacibaculum species on the skin of fish in a dose-dependent manner (Reid et al., 2017). This may be a response to a change in the microbial makeup of the skin, which allowed for T. maritimum to act as an opportunistic pathogen (Reid et al., 2017). One study also found higher levels of T. maritimum in dead and dying fish when compared to live fish collected from various salmon farm locations across British Columbia (Bateman et al., 2021). In cases of yellow mouth, it has been reported that T. maritimum is the dominant bacteria found in the oral cavity, although it is also one of the common bacteria in the mouths of unaffected or recovered salmon as well (Wynne et al., 2020). Co-infection with T. maritimum and Vibrio species was significantly associated only in salmon with yellow mouth, where Vibrio spp. load significantly increased in fish with clinical signs of yellow mouth when compared to healthy fish (Wynne et al., 2020). It was also reported that amoebic gill disease in salmon was not found to be a significant risk factor for the development of yellow mouth (Downes et al., 2018).
This study synthesized the range of existing research from 25 studies on salmonid management, environmental, and microbial factors associated with disease in farmed and wild salmonids caused by T. maritimum infection. Most articles were published within the last five years, with study designs ranging from experimental to case reports. Most articles (n=16) reported more than one factor, which speaks to the multifactorial nature of the disease. In general, many articles identified salmonid factors such as age and size, environmental factors such as higher temperatures and salinities, management factors such as stress from transfer, and microbial traits as a risk factor for infection with T. maritimum, along with stress and abrasion, and risk of co-infection due to its role as an opportunistic pathogen. However, most studies did not conduct multivariable analyses to understand the interplay between factors in disease caused by T. maritimum. This speaks to a knowledge gap and area for future research to elucidate the multifactorial of T. maritimum infection and identify areas for future research into management and control options. With continued research regarding the multifactorial etiology of yellow mouth, there is the potential to reduce AMU through well informed management solutions.
The age and size of salmonids appear to be a contributing factor of T. maritimum infection. Younger fish, particularly during their first year at sea when they are smaller and have softer scales, are reported as more susceptible to infection (van Gelderen et al., 2011; Downes et al., 2018; Bateman et al., 2021). Due to its lack of host specificity, disease from T. maritimum has been described in many other species such as dover sole, sea bass, red/black sea bream, and turbot, with a wide geographic range (Bernardet et al., 1994). In red/black sea bream, younger and smaller fish are reported to have more severe clinical signs than older and larger (>60 mm long) fish (Wakabayashi et al., 1984). Infection in these fish only occurred between 1-2 weeks following transfer from freshwater to saltwater (Wakabayashi et al., 1984). In Dover sole, the condition has also been described to be more common in younger fish, specifically during 60-100 days after hatching (McVicar and White, 1979). Decreased size as a function of increased T. maritimum load was also reported by a study, where it was concluded that this was a result of a decreased feeding rate (Bass et al., 2022). This is consistent with previous research indicating that T. maritimum infected fish become anorexic, making treatment with oral antimicrobials difficult (Soltani et al., 1995; Avendano-Herrera et al., 2006b).
The comparison between different salmonid species (Atlantic salmon and Rainbow trout) and non-salmonids (like greenback flounder) highlights variations in susceptibility, with Atlantic salmon showing significantly higher mortality and morbidity rates than greenback flounder, and similar morbidity/mortality to Rainbow trout (Soltani et al., 1996; Handlinger et al., 1997). There were no studies comparing morbidity and mortality in Atlantic and Pacific (including Chinook and Coho) salmon. However, in one study comparing Atlantic salmon and Rainbow trout at different bacterial concentrations, there was only a significant difference in mortalities at a concentration of 1.6 x 106 cells/mL, not at any of the other concentrations, including a higher concentration of and 1.6 x 107 cells/mL (Soltani et al., 1996). This result was not discussed by the authors, and based on the results of the other challenges, Rainbow trout and Atlantic salmon are assumed to show similar patterns of infection; consistent with other studies (Soltani et al., 1996; Handlinger et al., 1997). This information is crucial to understand the vulnerability of fish of different species, sizes, and ages to T. maritimum, which can inform infection risk.
Management practices likely play a pivotal role in T. maritimum infection and subsequent prevention. T. maritimum has been shown to be a part of the microbial community in both healthy and yellow mouth-affected salmonids (Wynne et al., 2020). This emphasizes its role as an opportunistic pathogen that could cause disease in immunocompromised fish (Wynne et al., 2020; Bateman et al., 2021). Management events such as transfer from fresh to saltwater, pen cleaning resulting in abrasion, and aggression from high stocking densities could all be stressful events resulting in infection (Wynne et al., 2020). Although at high enough concentrations, disease from T. maritimum has been demonstrated in the absence of abrasion (van Gelderen et al., 2011), many studies report that abrasion of the gills and body results in an increased severity of infection and mortality (Powell et al., 2004; Olsen et al., 2011; van Gelderen et al., 2011; Apablaza et al., 2017; Wynne et al., 2020). This was reported in another study involving sea bass, when scarified and smeared with Flexibacter maritimus broth culture, total mortality occurred within four days, with no mortality occurring in fish injected with the bacteria (Bernardet et al., 1994). The potential role of pen cleaning events in introducing T. maritimum emphasizes the need for careful maintenance of aquaculture facilities (van Gelderen et al., 2011). Higher stocking densities and improper feeding practices could result in aggressive behavior in Atlantic salmon (van Gelderen et al., 2011) who have been shown to bite and charge, resulting in abrasions (Mork et al., 1999). Reduced mortality due to T. maritimum has been demonstrated in Tasmania, where changed management procedures such as feeding practices and stocking densities were decreased (Handlinger et al., 1997).
Transfer from freshwater to saltwater was also identified as a factor (Smage et al., 2017; Nowlan et al., 2021a), especially during the first year at sea (Frelier et al., 1994; Downes et al., 2018; Nowlan et al., 2021a). This transfer is a stressful event that can pre-dispose fish to infection with T. maritimum (Wakabayashi et al., 1984; Frisch et al., 2018). This change has also been shown to alter the microbial community of the salmonid gut (Dehler et al., 2017), which could lead to dysbiosis and the overgrowth of opportunistic pathogens such as T. maritimum. Future research investigating microbial indicators could identify salmonids at risk for dysbiosis and disease (Dehler et al., 2017).
Vaccine development is a crucial management tool that could decrease T. maritimum outbreaks and thus antimicrobial use. AMR is a problem that exists at the interface of humans, animals, and the environment, therefore, we must consider it from a One Health perspective (Collignon and McEwen, 2019). With AMU in aquaculture, there comes the risk of AMR bacterial strains and genes developing in the aquatic environment and spreading to the terrestrial environment (Collignon and McEwen, 2019). By developing vaccines to prevent disease outbreaks or reduce morbidity/mortality of fish due to yellow mouth, the environmental and economic damages associated with yellow mouth can be modulated. Currently, the only vaccine approved for use against T. maritimum is for turbot in Spain (Nowlan et al., 2021b). In a vaccination study conducted in Tasmania and published in 2009, naïve Atlantic salmon had significantly better survival rates when injected with vaccine and adjuvant (Freund’s incomplete adjuvant), than the control group or vaccine-only group when challenged with T. maritimum (van Gelderen et al., 2009). This suggests the necessity for an adjuvant to demonstrate protection, however, the adjuvant group developed areas of melanin with granulomas and cysts focused in the fundic region (van Gelderen et al., 2009). These side-effects could lead to growth impairment and feed impaction (van Gelderen et al., 2009). A more recent study developed a vaccine for yellow mouth using isolates from western Canada that was able to elicit an antibody response, however, in a challenge scenario protection was not observed (Frisch et al., 2018). A difficulty in the development of a vaccine for salmonids may be due to the lack of repeatable and reliable challenge models (Nowlan et al., 2021b). Further, clinical signs of tenacibaculosis may be attributable to several Tenacibaculum species, making strain and species selection a challenge (Wynne et al., 2020; Nowlan et al., 2021b). This emphasizes the need for a reliable challenge model for T. maritimum in salmonids, and further vaccine development research.
Environmental conditions could significantly impact the amount of T. maritimum in the environment and therefor increase the risk of T. maritimum infection. Water temperature was reported as a factor of infection in many studies, with a consistent theme being that warmer water increases the prevalence of T. maritimum. The optimum growth range for T. maritimum is between 15-30°C, with temperatures above 15°C and higher salinities between 30-35% described as risk factors for tenacibaculosis (Avendano-Herrera et al., 2006b; Downes et al., 2018). Outbreaks in both salmonids and other farmed fish such as wedge sole have been reported to occur at water temperatures between 15-20°C, which may be the result of an increased stress response in fish and increased bacterial growth (van Gelderen et al., 2011; Mabrok et al., 2023). In adult Chinook salmon, warmer water temperature (16-24°C) has been associated with decreased growth and impaired smoltification (Carter, 2005). In salmonids, it is suggested that risk from all diseases is limited at temperatures between 12-13°C, with risk increasing from 14-17°C and high from 18-20°C (Carter, 2005). Exposure to temperatures outside of optimum rearing ranges may result in increased stress, which has immunosuppressive action (Abram et al., 2017). There is an observed suppression of the immune system when fish are exposed to cold stress due to overwintering strategies (Abram et al., 2017). This could explain why outbreaks of tenacibaculosis have also been observed in the winter months, why another study described an overgrowth of Tenacibaculum species in the fecal microbiota of salmonids undergoing low-temperature water treatment (Ghosh et al., 2022), or why decreased levels of T. maritimum in Chinook salmon in the fall and winter months were associated with decreased mortality (Bass et al., 2022). This further highlights the multiplicity of considerations at play even within one environmental factor of interest.
It has been postulated that factors such as UV irradiation, changes in salinity, and dissolved oxygen levels are associated with yellow mouth in salmonids (Wade and Weber, 2020). Decreasing the salinity and/or temperature in a pen has previously been shown to reduce yellow mouth mortality in affected salmonids (Soltani and Burke, 1995), however, subsequent studies found that freshwater treatments had no significant effect on the presence of the bacteria (Downes et al., 2018). Outbreaks of yellow mouth are significantly correlated with seasonality, with increasing prevalence in the summer, followed by a decline in outbreaks over the winter months (Downes et al., 2018). The predisposing source of tissue damage by UV irradiation and subsequent T. maritimum infiltration has been supported by several outbreak cases where spongy changes, as reported by Bullock et al., 1988, were observed to be the likely result of UV damage (Bullock, 1988; Handlinger et al., 1997). In natural infections, eye and dorsal surface lesions were more common in comparison to experimental conditions, which could also confirm the importance of UV irradiation (Handlinger et al., 1997). However, this is not the sole predisposing factor, since disease with similar lesions in different locations has been found in fish in settings with controlled lighting (Handlinger et al., 1997). Algal blooms have been suggested as a factor in T. maritimum infection outbreaks, since algal blooms decrease oxygenation and increase stress on fish (Riisberg and Edvardsen, 2008; Apablaza et al., 2017). Higher water temperatures which are conducive to the growth of T. maritimum also lead to algal blooms, which could result in a multiplicity of stressors leading to infection (Riisberg and Edvardsen, 2008; Apablaza et al., 2017). Recognizing these environmental factors can aid in predicting and mitigating T. maritimum outbreaks, especially in regions where aquaculture is prevalent.
T. maritimum has no host specificity and can transmit horizontally, therefore vectors are a factor of interest. Potential vectors for transmission in salmonids could be sea lice (Barker et al., 2009; Llewellyn et al., 2017; Nowlan et al., 2021a), jellyfish (Smage et al., 2017; Downes et al., 2018), and lumpsuckers (Frisch et al., 2018). However, none of the included studies were able to demonstrate that the vector of interest was the original source of T. maritimum. Instead, vectors such as jellyfish or plankton may play a role in tissue damage leading to abrasion, which could allow T. maritimum to proliferate (Ferguson et al., 2010; Apablaza et al., 2017). T. maritimum has been detected in Pelagia quadtrata and Muggiaea atlantica and Pelagia notiluca jellyfish species which are known to cause gill damage leading to disease in salmon (Delannoy et al., 2011; Fringuelli et al., 2012). The transmission of T. maritimum between wild and farmed salmonids in BC has also been a concern in the recent years, due to the decline of Sockeye salmon in the region (Bateman et al., 2022). In a study screening Sockeye salmon smolts as they migrate past salmon farms in the Discovery Islands region, there was a peak of 12.7 times the background level of T. maritimum prevalence (Bateman et al., 2022). However, this could not be confirmed because of interaction with farmed fish, as the region is described as a hydrographic funnel that forces migrating salmon into a higher density and could magnify all sources of T. maritimum pressure (Bateman et al., 2022).
T. maritimum is part of the natural microbial community on the surface of salmonids (van Gelderen et al., 2011; Reid et al., 2017; Wynne et al., 2020; Nowlan et al., 2021a; Ghosh et al., 2022). Based on previous bacterial culture reports, T. maritimum is difficult to culture in non-sterile seawater, which may suggest that its growth is inhibited in the natural aquatic environment due to inhibition of other bacteria (Avendano-Herrera et al., 2006a). Understanding the microbial makeup of fish skin, mucus, and oral cavity is essential in assessing the risk of T. maritimum infections. Fish microbiota can shift and undergo dysbiosis because of challenges from stress such as temperature, fresh to saltwater transfer, and co-infection (Reid et al., 2017; Ghosh et al., 2022). Under experimental conditions, it has been demonstrated that temperature has a significant effect on the salmonid gut microbiota, skin mucous, and water microbiota (Ghosh et al., 2022). This dysbiosis allows for pathogenic and opportunistic bacteria to proliferate, for example, salmonid alphavirus infection can increase the bacterial load of Tenacibaculum species on the skin of infected fish (Reid et al., 2017). Differences in microbial load challenge studies, such as number of data replicates must also be considered. Studies have also reported strong and significant positive correlations between T. maritimum and many other infectious agents in farmed Atlantic salmon in BC (Bateman et al., 2021). Tenacibaculum dicentrarchi and Tenacibaculum finnmarkense have also been linked to tenacibaculosis outbreaks in Chile and Canada (Avendano-Herrera et al., 2020; Nowlan et al., 2021a). These bacteria are often found together and may result in disease displaying similar clinical signs such as mouth erosions and frayed fins (Wynne et al., 2020; Nowlan et al., 2021a; Mabrok et al., 2023). Therefore, it is becoming increasingly important to understand the natural microbial community of salmonids and the pathogenic source of outbreaks, to further develop treatment and control strategies.
The identification of multivariable models that simultaneously account for several factors simultaneously associated with T. maritimum infections in salmonids is notably absent in the literature, representing a significant gap in our understanding of the disease’s multifactorial etiology. Although the articles identified individual factors—such as environmental conditions, management practices, and host-specific traits—that influence T. maritimum infections, the interactions among these variables remain poorly characterized. To better understand the nature of yellow mouth outbreaks, the antagonistic or synergistic effects of a combination of factors must be examined.
To reduce the risk of not capturing all eligible articles, this review followed a systematic approach (Pham et al., 2014). The search strategy did not include any restrictions regarding language, however, a few articles were excluded because of difficulty with translation. This review was specific to salmonids and infection with T. maritimum and its resulting diseases. Due to the complex interactions of pathogens that may result in infection, and the lack of common naming standards, it is possible that some articles were missed. The lack of statistical analysis and multivariable analysis limited the ability to understand the interplay between factors for infection, as well as clinical disease-specific factors resulting from infection. Also, species other than salmonids were outside of the scope of this review, therefore, some factors that impact other species of fish which could give insight to salmonid disease may not have been included and are a topic for further study. Given the focus of this review on factors associated with salmonid infection with T. maritimum, it is not possible to infer the geographical distribution of the disease from the locations of the studies included in the review. It is possible that disease in other regions may have different factors associated with infection, which were not captured by this review.
These results suggest a complex interplay of factors contributing to T. maritimum infections in salmonids. Effective management and prevention strategies should consider the age and size of fish, minimize gill and body abrasion, environmental conditions, and account for the microbial composition of fish and their surroundings. Future research to conduct experiments and observational studies that allow for assessment of the interplay between factors is crucial to fill this data gap. This will be crucial for developing targeted approaches to reduce the impact of T. maritimum in farmed and wild salmonid.
The original contributions presented in the study are included in the article/Supplementary Material. Further inquiries can be directed to the corresponding author.
Ethical approval was not required for the study involving animals in accordance with the local legislation and institutional requirements because this is a scoping review of published literature.
RW: Conceptualization, Data curation, Formal analysis, Investigation, Methodology, Validation, Visualization, Writing – original draft, Writing – review & editing. EJ: Data curation, Writing – review & editing. FU: Conceptualization, Data curation, Methodology, Writing – review & editing. RR-S: Funding acquisition, Resources, Writing – review & editing. KR: Conceptualization, Data curation, Methodology, Project administration, Writing – review & editing. SO: Conceptualization, Funding acquisition, Investigation, Methodology, Project administration, Resources, Supervision, Writing – original draft, Writing – review & editing.
The author(s) declare financial support was received for the research, authorship, and/or publication of this article. Funding for this project was provided by a NSERC Discovery Grant (NSERC RGPIN-2020-04447) held by SO. Funding for RW and EJ stipends were provided in part by a NSERC Discovery Grant (NSERC RGPIN-2020-04447) and by the Public Health Agency of Canada. KR was supported by funding from the AMR One Health Consortium (Major Innovation Fund, Alberta Ministry of Jobs, Economy, and Innovation RCP-19-003-MIF).
We thank Janice Kung from the University of Alberta Library for her assistance to develop the search strings for the review.
The authors declare that the research was conducted in the absence of any commercial or financial relationships that could be construed as a potential conflict of interest.
All claims expressed in this article are solely those of the authors and do not necessarily represent those of their affiliated organizations, or those of the publisher, the editors and the reviewers. Any product that may be evaluated in this article, or claim that may be made by its manufacturer, is not guaranteed or endorsed by the publisher.
The Supplementary Material for this article can be found online at: https://www.frontiersin.org/articles/10.3389/faquc.2024.1496943/full#supplementary-material
Abram Q. H., Dixon B., Katzenback B. A. (2017). Impacts of low temperature on the teleost immune system. Biology 6, 39. doi: 10.3390/biology6040039
Apablaza P., Frisch K., Brevik O. J., Smage S. B., Vallestad C., Duesund H., et al. (2017). Primary isolation and characterization of Tenacibaculum maritimum from Chilean Atlantic aalmon mortalities associated with a Pseudochattonella spp. algal bloom. J. Aquat. Anim. Health 29, 143–149. doi: 10.1080/08997659.2017.1339643
Aromataris E. M. Z. (2017). Joanna Briggs Institute reviewer's manual. Eds. Lockwood C., Porritt K., Pilla B., Jordan Z. (Adelaide, Australia: The Joanna Briggs Institute).
Asche F., Cojocaru A. L., Roth B. (2018). The development of large scale aquaculture production: A comparison of the supply chains for chicken and salmon. Aquaculture 493, 446–455. doi: 10.1016/j.aquaculture.2016.10.031
Avendano-Herrera R., Collarte C., Saldarriaga-Cordoba M., Irgang R. (2020). New salmonid hosts for Tenacibaculum species: Expansion of tenacibaculosis in Chilean aquaculture. J. Fish Dis. 43, 1077–1085. doi: 10.1111/jfd.13213
Avendano-Herrera R., Irgang R., Magarinos B., Romalde J. L., Toranzo A. E. (2006a). Use of microcosms to determine the survival of the fish pathogen Tenacibaculum maritimum in seawater. Environ. Microbiol. 8, 921–928. doi: 10.1111/j.1462-2920.2005.00981.x
Avendano-Herrera R., Toranzo A. E., Magarinos B. (2006b). Tenacibaculosis infection in marine fish caused by Tenacibaculum maritimum: a review. Dis. Aquat. Organisms 71, 255–266. doi: 10.3354/dao071255
Barker D. E., Braden L. M., Coombs M. P., Boyce B. (2009). Preliminary studies on the isolation of bacteria from sea lice, Lepeophtheirus salmonis, infecting farmed salmon in British Columbia, Canada. Parasitol. Res. 105, 1173–1177. doi: 10.1007/s00436-009-1523-9
Bass A. L., Bateman A. W., Connors B. M., Staton B. A., Rondeau E. B., Mordecai G. J., et al. (2022). Identification of infectious agents in early marine Chinook and Coho salmon associated with cohort survival. Facets 7, 742–773. doi: 10.1139/facets-2021-0102742
Bateman A. W., Schulze A. D., Kaukinen K. H., Tabata A., Mordecai G., Flynn K., et al. (2021). Descriptive multi-agent epidemiology via molecular screening on Atlantic salmon farms in the northeast Pacific Ocean. Sci. Rep. 11, 3466. doi: 10.1038/s41598-020-78978-9
Bateman A. W., Teffer A. K., Bass A., Ming T., Kaukinen K., Hunt B. P. V., et al. (2022). Atlantic salmon farms are a likely source of Tenacibaculum maritimum infection in migratory Fraser River sockeye salmon. Can. J. Fisheries Aquat. Sci. 79, 1225–1240. doi: 10.1139/cjfas-2021-0164
Bernardet J.-F., Kerouault B., Michel C. (1994). Comparative study on Flexibacter maritimus strains isolated from farmed sea bass (Dicentrarchus labrax) in France. Fish Pathol. 29, 105–111. doi: 10.3147/jsfp.29.105
Brosnahan C. L., Munday J. S., Ha H. J., Preece M., Jones J. B. (2019). New Zealand rickettsia-like organism (NZ-RLO) and Tenacibaculum maritimum: Distribution and phylogeny in farmed Chinook salmon (Oncorhynchus tshawytscha). J. Fish Dis. 42, 85–95. doi: 10.1111/jfd.12909
Bullock A. M. (1988). “Solar ultraviolet radiation: a potential environmental hazard in the cultivation of farmed finfish,” in Recent Advances in Aquaculture: Volume 3 (Dordrech: Springer, Netherlands), 139–224.
Carter K. (2005). The effects of temperature on Steelhead trout, Coho salmon, and Chinook salmon biology and function by life stage. California regional Water Qual. control board., 1–26. Available online at: https://www.waterboards.ca.gov/northcoast/water_issues/programs/tmdls/shasta_river/060707/28appendixaetheeffectsoftemperatureonsteelheadtroutcohosalmonandchinooksalmonbiologyandfunction.pdf (Accessed November 5, 2024).
Collignon P. J., McEwen S. A. (2019). One Health-Its importance in helping to better control antimicrobial resistance. Trop. Med. Infectioius Dis. 4 (10), 22. doi: 10.3390/tropicalmed4010022
Dehler C. E., Secombes C. J., Martin S. A. M. (2017). Seawater transfer alters the intestinal microbiota profiles of Atlantic salmon (Salmo salar L.). Sci. Rep. 7, 13877. doi: 10.1038/s41598-017-13249-8
Delannoy C. M., Houghton J. D., Fleming N. E., Ferguson H. W. (2011). Mauve Stingers (Pelagia noctiluca) as carriers of the bacterial fish pathogen Tenacibaculum maritimum. Aquaculture 311, 255–257. doi: 10.1016/j.aquaculture.2010.11.033
Downes J. K., Yatabe T., Marcos-Lopez M., Rodger H. D., MacCarthy E., O'Connor I., et al. (2018). Investigation of co-infections with pathogens associated with gill disease in Atlantic salmon during an amoebic gill disease outbreak. J. Fish Dis. 41, 1217–1227. doi: 10.1111/jfd.12814
Ferguson H. W., Delannoy C. M. J., Hay S., Nicolson J., Sutherland D., Crumlish M. (2010). Jellyfish as vectors of bacterial disease for farmed salmon (Salmo salar). J. Veterinary Diagn. Invest. 22, 376–382. doi: 10.1177/104063871002200305
Fernandez-Alvarez C., Santos Y. (2018). Identification and typing of fish pathogenic species of the genus. Tenacibaculum. Appl. Microbiol. Biotechnol. 102, 9973–9989. doi: 10.1007/s00253-018-9370-1
Food and Agriculture Organization of the United Nations (2022). The state of world fisheries and aquaculture 2022 (Rome, Italy: Food and Agriculture Organization of the United Nations). Available at: https://openknowledge.fao.org/items/11a4abd8-4e09-4bef-9c12-900fb4605a02.
Frelier P. F., Elston R. A., Loy J. K., Mincher C. (1994). Macroscopic and microscopic features of ulcerative stomatitis in farmed Atlantic salmon. Salmo salar. Dis. Aquat. Organisms 18, 227–231. doi: 10.3354/dao018227
Fringuelli E., Gordon A. W., Rodger H., Welsh M. D., Graham D. A. (2012). Detection of Neoparamoeba Perurans by duplex quantitative Taqman real-time PCR in formalin-fixed, paraffin-embedded Atlantic salmonid gill tissues. J. Fish Dis. 35, 711–724. doi: 10.1111/j.1365-2761.2012.01395.x
Frisch K., Smage S. B., Vallestad C., Duesund H., Brevik O. J., Klevan A., et al. (2018). Experimental induction of mouthrot in Atlantic salmon smolts using Tenacibaculum maritimum from Western Canada. J. Fish Dis. 41, 1247–1258. doi: 10.1111/jfd.12818
Ghosh S. K., Wong M. K.-S., Hyodo S., Goto S., Hamasaki K. (2022). Temperature modulation alters the gut and skin microbial profiles of chum salmon (Oncorhynchus keta). Front. Mar. Sci. 9, 1027621. doi: 10.3389/fmars.2022.1027621
Gourzioti E., Kolygas M. N., Athanassopoulou F., Babili V. (2016). Tenacibaculosis in aquaculture farmed marine fish. J. Hellenic Veterinary Med. Soc. 67, 21–32. doi: 10.12681/jhvms.15620
Handlinger J., Soltani M., Percival S. (1997). The pathology of Flexibacter maritimus in aquaculture species in Tasmania, Australia. J. Fish Dis. 20, 159–168. doi: 10.1046/j.1365-2761.1997.00288.x
Jones M. A., Powell M. D., Becker J. A., Carter C. G. (2007). Effect of an acute necrotic bacterial gill infection and feed deprivation on the metabolic rate of Atlantic salmon. Salmo salar. Dis. Aquat. Organisms 78, 29–36. doi: 10.3354/dao01855
Llewellyn M. S., Leadbeater S., Garcia C., Sylvain F. E., Custodio M., Ang K. P., et al. (2017). Parasitism perturbs the mucosal microbiome of Atlantic salmon. Sci. Rep. 7, 43465. doi: 10.1038/srep43465
Mabrok M., Algammal A. M., Sivaramasamy E., Hetta H. F., Atwah B., Alghamdi S., et al. (2023). Tenacibaculosis caused by Tenacibaculum maritimum: Updated knowledge of this marine bacterial fish pathogen. Front. Cell. Infection Microbiol. 12. doi: 10.3389/fcimb.2022.1068000
McVicar A., White P. (1979). Fin and skin necrosis of cultivated Dover sole. Solea solea (L.). J. Fish Dis. 2, 557–562. doi: 10.1111/j.1365-2761.1979.tb00418.x
Mork O., Bjerkeng B., Rye M. (1999). Aggressive interactions in pure and mixed groups of juvenile farmed and hatchery-reared wild Atlantic salmon Salmo salar L. @ in relation to tank substrate. Aquaculture Res. 30, 571–578. doi: 10.1046/j.1365-2109.1999.00356.x
Morrison D. B., Saksida S. (2013). Trends in antimicrobial use in Marine Harvest Canada farmed salmon production in British Columbia (2003–2011). Can. Veterinary J. 54, 1160.
Nowlan J. P., Britney S. R., Lumsden J. S., Russell S. (2021a). Application of Quantitative-PCR to monitor netpen sites in British Columbia (Canada) for Tenacibaculum species. Pathogens 10, 414. doi: 10.3390/pathogens10040414
Nowlan J. P., Britney S. R., Lumsden J. S., Russell S. (2021b). Experimental induction of tenacibaculosis in Atlantic salmon (Salmo salar L.) using Tenacibaculum maritimum, T. dicentrarchi, and T. finnmarkense. Pathogens 10, 1439. doi: 10.3390/pathogens10111439
Ojasanya R. A., Gardner I. A., Groman D. B., Saksida S., Saab M. E., Thakur K. K. (2022). Antimicrobial susceptibility profiles of bacteria commonly isolated from farmed salmonids in Atlantic Canada (2000-2021). Veterinary Sci. 9 (4), 159. doi: 10.3390/vetsci9040159
Olsen A. B., Nilsen H., Sandlund N., Mikkelsen H., Soerum H., Colquhoun D. J. (2011). Tenacibaculum sp. associated with winter ulcers in sea-reared Atlantic salmon Salmo salar. Dis. Aquat. Organisms 94, 189–199. doi: 10.3354/dao02324
Ostland V. E., LaTrace C., Morrison D., Ferguson H. W. (1999). Flexibacter maritimus associated with a bacterial stomatitis in Atlantic salmon smolts reared in net-pens in British Columbia. J. Aquat. Anim. Health 11, 35–44. doi: 10.1577/1548-8667(1999)011<0035:Fmawab>2.0.Co;2
Pham M. T., Rajić A., Greig J. D., Sargeant J. M., Papadopoulos A., McEwen S. A. (2014). A scoping review of scoping reviews: advancing the approach and enhancing the consistency. Res. synthesis Methods 5, 371–385. doi: 10.1002/jrsm.1123
Powell M., Carson J., van Gelderen R. (2004). Experimental induction of gill disease in Atlantic salmon Salmo salar smolts with Tenacibaculum maritimum. Dis. Aquat. Organisms 61, 179–185. doi: 10.3354/dao061179
Powell M. D., Harris J. O., Carson J., Hill J. V. (2005). Effects of gill abrasion and experimental infection with Tenacibaculum maritimum on the respiratory physiology of Atlantic salmon Salmo salar affected by amoebic gill disease. Dis. Aquat. Organisms 63, 169–174. doi: 10.3354/dao063169
Reid K. M., Patel S., Robinson A. J., Bu L., Jarungsriapisit J., Moore L. J., et al. (2017). Salmonid alphavirus infection causes skin dysbiosis in Atlantic salmon (Salmo salar L.) post-smolts. PLoS One 12, e0172856. doi: 10.1371/journal.pone.0172856
Riisberg I., Edvardsen B. (2008). Genetic variation in bloom-forming ichthyotoxic Pseudochattonella species (Dictyochophyceae, Heterokonta) using nuclear, mitochondrial and plastid DNA sequence data. Eur. J. Phycology 43, 413–422. doi: 10.1080/09670260802299602
Rud I., Kolarevic J., Holan A. B., Berget I., Calabrese S., Terjesen B. F. (2017). Deep-sequencing of the bacterial microbiota in commercial-scale recirculating and semi-closed aquaculture systems for Atlantic salmon post-smolt production. Aquacultural Eng. 78, 50–62. doi: 10.1016/j.aquaeng.2016.10.003
Smage S. B., Brevik O. J., Frisch K., Watanabe K., Duesund H., Nylund A. (2017). Concurrent jellyfish blooms and tenacibaculosis outbreaks in Northern Norwegian Atlantic salmon (Salmo salar) farms. PLoS One 12 (11), e0190762. doi: 10.1371/journal.pone.0187476
Soltani M., Burke C. M. (1995). Responses of fish-pathogenic Cytophaga/Flexibacter-like bacteria (CFLB) to environmental conditions. Bull. Eur. Assoc. Fish Pathologists 14, 185–187.
Soltani M., Munday B. L., Burke C. M. (1996). The relative susceptibility of fish to infections by Flexibacter columnaris and Flexibacter maritimus. Aquaculture 140, 259–264. doi: 10.1016/0044-8486(95)01157-9
Soltani M., Shanker S., Munday B. (1995). Chemotherapy of Cytophaga/Flexibacter-like bacteria (CFLB) infections in fish: studies validating clinical efficacies of selected antimicrobials. J. Fish Dis. 18, 555–565. doi: 10.1111/j.1365-2761.1995.tb00360.x
Toranzo A. E., Magariños B., Romalde J. L. (2005). A review of the main bacterial fish diseases in mariculture systems. Aquaculture 246, 37–61. doi: 10.1016/j.aquaculture.2005.01.002
Tricco A., Lillie E., Zarin W., O’Brien K., Colquhoun H., Levac D., et al. (2018). PRISMA extension for scoping reviews (PRISMA-ScR): checklist and explanation. Ann. Internal Med. 169, 467–473. doi: 10.7326/M18-0850
UN Department of Economic and Social Affairs (2022). World Economic Situation and Prospects 2022. Available online at: https://www.un.org/development/desa/dpad/publication/world-economic-situation-and-prospects-2022/. (accessed November 5, 2024)
UN General Assembly (2015). Transforming our World: The 2030 agenda for sustainable development, A/RES/70/1 (New York: UN GAOR, 70th session, Plenary, Item 15 & 16). Available at: https://sustainabledevelopment.un.org/post2015/transformingourworld/publication.
van Gelderen R., Carson J., Nowak B. (2009). Experimental vaccination of Atlantic salmon (Salmo salar L.) against marine flexibacteriosis. Aquaculture 288, 7–13. doi: 10.1016/j.aquaculture.2008.11.012
van Gelderen R., Carson J., Nowak B. (2011). Experimentally induced marine flexibacteriosis in Atlantic salmon smolts Salmo salar. II. pathology. Dis. Aquat. Organisms 95, 125–135. doi: 10.3354/dao02329
Wade J., Weber L. (2020). Characterization of Tenacibaculum maritimum and mouthrot to inform pathogen transfer risk assessments in British Columbia (Ottawa, Canada: DFO Canadian Science Advisory Secretariat).
Wakabayashi H., Hikida M., Masumura K. (1984). Flexibacter infection in cultured marine fish in Japan. Helgol Meeresunters 37, 587–593.
Wassmuth R. (2023). Yellowmouth Scoping Review Protocol Open Science Framework (OSF) (Open Science Framework (OSF). doi: 10.17605/OSF.IO/NCQ56
Keywords: yellow mouth disease, Tenacibaculum maritimum, aquaculture, salmon, management practices, environmental conditions, microbial dynamics
Citation: Wassmuth RM, de Jongh EJ, Uhland FC, Reid-Smith RJ, Robertson K and Otto SJG (2024) Factors associated with disease in farmed and wild salmonids caused by Tenacibaculum maritimum: a scoping review. Front. Aquac. 3:1496943. doi: 10.3389/faquc.2024.1496943
Received: 15 September 2024; Accepted: 22 October 2024;
Published: 05 December 2024.
Edited by:
Krishna Thakur, University of Prince Edward Island, CanadaReviewed by:
Muhammed Duman, Bursa Uludağ University, TürkiyeCopyright © 2024 Wassmuth, de Jongh, Uhland, Reid-Smith, Robertson and Otto. This is an open-access article distributed under the terms of the Creative Commons Attribution License (CC BY). The use, distribution or reproduction in other forums is permitted, provided the original author(s) and the copyright owner(s) are credited and that the original publication in this journal is cited, in accordance with accepted academic practice. No use, distribution or reproduction is permitted which does not comply with these terms.
*Correspondence: Simon J. G. Otto, c2ltb24ub3R0b0B1YWxiZXJ0YS5jYQ==
Disclaimer: All claims expressed in this article are solely those of the authors and do not necessarily represent those of their affiliated organizations, or those of the publisher, the editors and the reviewers. Any product that may be evaluated in this article or claim that may be made by its manufacturer is not guaranteed or endorsed by the publisher.
Research integrity at Frontiers
Learn more about the work of our research integrity team to safeguard the quality of each article we publish.