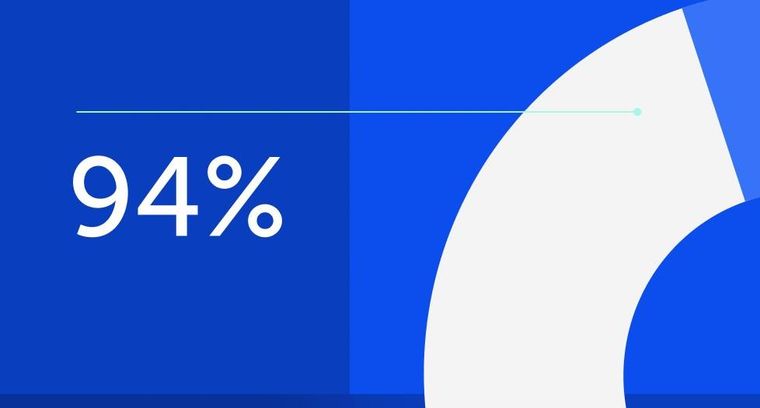
94% of researchers rate our articles as excellent or good
Learn more about the work of our research integrity team to safeguard the quality of each article we publish.
Find out more
REVIEW article
Front. Aquac., 30 August 2024
Sec. Production Biology
Volume 3 - 2024 | https://doi.org/10.3389/faquc.2024.1428206
This article is part of the Research TopicDifferentiating and defining ‘exposed’ and ‘offshore’ aquaculture and implications for aquaculture operation, management, costs, and policyView all 13 articles
In 2017, the Chilean government through the Chilean Economic Development Agency (CORFO) (an agency under the Ministry of Economy) launched a public call for the execution of a Technological Program to adopt, adapt, and/or developing enabling technologies for the development of Ocean Aquaculture in places with high-energy (strong waves, winds and/or currents). The consortium of companies, technology centers, and universities led by Ecosea Farming (Ecosea), focused its efforts on aspects related to structural engineering, mooring systems, sensors, Internet of Things (IoT), and other integral components, as well as essential aspects of regulation and standards. On this last topic, intensive collaborative work was carried out between the technical teams of the Andrés Bello University, the Undersecretariat of Fisheries and Aquaculture (Subpesca), the National Fisheries and Aquaculture Service (Sernapesca), and CORFO, with the aim of gathering relevant information from international experience, and establishing the main differences between aquaculture traditionally developed in the fjords, coast, estuaries, and inland sea of southern Chile and aquaculture in the high seas – a practice not yet clearly defined and still indistinctly known as offshore or open ocean aquaculture. This document summarizes the main findings obtained and can be a useful guide for future experiences in other countries with important aquaculture developments.
In the last decade, aquaculture has been the fastest-growing food industry (Aanesen et al., 2023; Cantillo et al., 2023). One of the factors contributing to this growth is the efficiency of fish farming in terms of feed conversion rate (FCR) and carbon footprint. Unlike other protein-producing industries fish farming maintains a low FCR (Cantillo et al., 2023), indicating that aquaculture can generate a higher amount of animal proteins with less feed (Yi and Kim, 2020a). Another relevant factor is the increase in demand for seafood products driven by population growth (Luna et al., 2023), which has pushed the salmon industry toward rapid growth and significant economic success (Garlock et al., 2020; Olsen et al., 2023). In Norway for example, remarkable changes have taken place over 50 years. The industry has gone from being a small sector guided by small entrepreneurs to becoming an industrialized, science-driven sector with high social and economic impact, currently being Norway’s third-largest exporting industry (Hersoug, 2022). Another example is Chile, which has significantly increased its production to become one of the leading global producers of salmonids (Poblete et al., 2019), with a production of over one million tons in 2023 (www.subpesca.cl/portal/618/articles-120507_documento.pdf), focused mainly on Atlantic salmon (Salmo salar), which represents about 55% of the total national aquaculture harvest and approximately 75% of the country’s salmonid production (Chávez et al., 2019; Lorena et al., 2022).
Although the contribution of aquaculture to human development is evident, negative perceptions of the industry have proven to be a major obstacle to its growth (Mazur and Curtis, 2008; Froehlich et al., 2021; Nathan Young; Whitmarsh and Palmieri, 2009). For example, in 2013 public scrutiny was partly responsible for the provincial moratorium on new salmon farm leases in Canada (CBC, 2015), societal interest in environmental sustainability is in more demand than ever before, and marine ecosystems and their interrelationships are better understood (Garza-Gil et al., 2016). In many cases, mariculture is an important ecosystem modifier in coastal areas, and one that can bring enormous social and economic benefits while potentially generating serious impacts on the health of marine ecosystems and humans (Uglem et al., 2014). Therefore, understanding public perception of the industry is crucial for policymakers and industry actors seeking to improve societal support (Olsen et al., 2023).
One of the most important impacts of intensive aquaculture is the release of large amounts of waste from fish cages in aquaculture areas, which are transported by the water currents and eventually settle on the sea bottom, consequently leading to deoxygenation of the aquatic environment (Yokoyama, 2002; Troell et al., 2009). Opposition groups have successfully slowed or even stopped the development of the industry in protected areas, demonstrating the importance of considering social dimensions when designing development strategies (Noakes et al., 2003; Barton and Fløysand, 2010; Knapp and Rubino, 2016). This criticism of social and environmental issues has hindered the growth of the salmon industry in several countries (Olsen et al., 2023). One example is the present situation in Chile, where the Chilean government is planning a new department for “Biodiversity and protected areas”. The new department is expected to reduce the number of existing aquaculture concessions and impose constraints on the growth of this sector1. Consequently, there is a tense atmosphere between the current government and the aquaculture companies.
As an alternative to reduce competition for space in coastal areas and increase salmon farm production, offshore aquaculture has gained increasing attention in recent years (Watson et al., 2022), with this new way of production the farms will be moved farther off the coast, into the less protected ocean environment (Froehlich et al., 2017). Welch et al. (2019) conducted an environmental assessment of a fish farm operating in an exposed area under stronger currents and greater depths off the coast of Panama and demonstrated that wholesome marine fish for human consumption can be produced with minimal environmental impact. However, moving the farms to the open ocean can still present several challenges, such as fish escapes and the spread of disease (Jacquet et al., 2024), moreover, offshore aquaculture will not be free from ecological risks, which may be very similar to those associated with coastal aquaculture (Fujita et al., 2023).
Despite the increasing interest in offshore aquaculture, there is no clear consensus on the definition of “offshore aquaculture” (Holmer, 2010; Fujita et al., 2023), but it clearly involves activities located in open waters several kilometers from the coast (Morro et al., 2022). Recently, leading aquaculture countries (e.g., China and Norway) have moved toward large-scale salmon farming using offshore platform technologies, which would overcome environmental constraints (Zhao et al., 2019). Thus, China launched the Deep Blue 1 facility, which operates in shallow marine layers (Yi and Kim, 2020b). Another example is Chile, with the development of the offshore aquaculture consortium which is working with a submersible copper net pen (The Fish Site, 2021). Therefore, much of the current interest in aquaculture expansion has been stimulated by the development of infrastructure capable of containing marine organisms in waters with strong currents, bigger waves, and technology capable of supplying feed and monitoring operations at facilities located offshore (Fujita et al., 2023). Thus, the offshore aquaculture operation will require a review of the current regulations and their update, which will include logistics, environmental protection, and other relevant aspects (Watson et al., 2022).
In this research, we address three questions that the Chilean aquaculture and fishery authority has regarding offshore aquaculture. As a major world producer of salmon in ocean cage systems, Chile can simultaneously contribute and benefit from interacting with other major producing countries. The questions are:
1. What limitations does the current legislation have in granting aquaculture farms in offshore zones?
2. What do we understand by offshore aquaculture? Which term to consider in its definition?
3. What parameters should be considered in evaluating the suitability of future offshore aquaculture areas?
For the first question, we analyzed the current regulation of aquaculture activity in Chile, especially those carried out in maritime areas under aquaculture concessions granted by the administrative authority. Although there are extensive regulations, for the purposes of this study, we focused on the norms found in the following regulatory bodies: a) General Law of Fishing and Aquaculture and its main regulations; b) Law of Maritime Concessions and its regulation; c) General Law of Environmental Bases and its Regulations; d) United Nations Convention on the Law of the Sea (analysis of the powers of coastal states in the Exclusive Economic Zone).
For the other two questions, the main methodology employed in this research involved conducting literature searches across two databases: Scopus and Google Scholar. The review process is outlined in Figure 1. The keywords used for the literature search were “Offshore aquaculture” and “Ocean aquaculture”. To refine the search results further, the search was restricted to ‘Title’ for the Google Scholar database and “keywords” for the Scopus database. The search yielded 172 articles for Scopus and 20 for Google Scholar for the keyword “offshore aquaculture”, and 4 articles for Scopus and 10 for Google Scholar for the keyword “ocean aquaculture”. Out of these initial papers, 72 were discarded due to duplication, with only incidental mentions of “offshore aquaculture or ocean aquaculture” without being the main topic of research or being inaccessible. Ultimately, 134 articles were analyzed. We thoroughly examined each article to determine if they provided a definition of “offshore aquaculture” or “ocean aquaculture.” In our research, we did not search the keyword “Open Ocean” as it could excessively broaden the scope of studies to analyze, given its wide use in various research topics such as fisheries (Gordon and Shipley, 2019; Joseph et al., 2019), microplastics (Pham et al., 2023; Sambolino et al., 2023), nutrient cycles (Bonelli et al., 2022; Baumas and Bizic, 2024), and migratory route studies (Hays et al., 2020), among many others. In addition, by searching the term “open ocean aquaculture,” we found the studies we had already considered evaluating with the other keywords.
In parallel with the literature search, we conducted a search for documents from governmental agencies, bill proposals, or laws that defined “offshore aquaculture” or “ocean aquaculture” around the world. Each definition was coded using the software NVivo 14 to explore the main concepts and relevant words. We categorized the definitions into two groups: “literature” for definitions from scientific papers and “government documents” for definitions from government agency files, bills, or other documents required from a legal authority. In conjunction with the above analysis, we identify the main features that are mentioned in each definition.
Our research on Chile’s legal framework is restricted to laws, decrees, norms, and other legal documents in force until April 2024. The literature search encompasses works in Spanish or English. The exploration for definitions of ocean aquaculture or offshore aquaculture in legal documents of countries other than Chile is limited to four countries: Norway, the United States, Australia, and New Zealand. In a previous (unpublished) study conducted by our team for the Chilean authority, additional countries were analyzed. However, the aforementioned countries have research programs in offshore aquaculture that are relevant to Chile’s interests, and a form of offshore aquaculture is expected to be more aligned with Chile’s development. Therefore, we decided to confine the search to these four countries.
The current methodology confines itself to literary research conducted between January 1, 2010, and April 1, 2024. Our scope is restricted to English language materials accessible for download in any format that permits complete readability.
The aquaculture sector in Chile is a highly regulated industry. Through the analysis carried out, various regulatory bodies dedicated to each aspect of the sector’s activity were identified. A summary of the most important ones can be seen in Figure 2. As evident, all regulations stem from the political constitution of the country, which establishes rights leading to the creation of other rules and laws. For instance, Article 19, paragraph 8, establishes “the right to live in an environment free of pollution. It is the duty of the State to ensure that this right is not affected and to protect the preservation of nature.” Additionally, numeral 21 establishes “The right to develop any economic activity…, respecting the legal norms that regulate it.” Law 18,892 regulates this economic activity in its Title VI, “On Aquaculture,” outlining the legal norms to be observed for its development. This includes aspects such as the concession title granted for carrying out this activity on national public property and the operation and exercise of the farming activity.
At the same time, another relevant norm is the Law on General Bases of the Environment, the law 19.300 established a system whereby various economic activities outlined within it are required to undergo an evaluation of their environmental impacts prior to their execution or modification. The National Policy for the Use of the Coastal Border created by the Supreme Decree 475 of the year 1994, applies to fiscal beach lands situated within an eighty-meter-wide strip, measured from the highest tide line of the coastline. This policy encompasses the beach, bays, gulfs, straits, inland channels, and the territorial sea under the control of the Ministry of National Defense and, Undersecretary of the Navy. This area is referred to as the Coastal Border of the Littoral.
Other, more specific laws and decrees stem from the aforementioned regulations, addressing matters such as the procedure for allocating concessions. For instance, Supreme Decree No. 290 of 1993 establishes the regulations for aquaculture concessions. In 2001, the Ministry of Economy, Development, and Reconstruction issued Supreme Decree No. 320, approving the Environmental Regulations for Aquaculture (RAMA). These regulations stipulate that aquaculture concessions must operate within the capacity limits of the water bodies in which they are situated. Simultaneously, the Regulation of Protection, Control, and Eradication Measures of High-Risk Diseases for Hydrobiological Species (RESA) was established through Supreme Decree 319 of 2001. This decree sets forth measures to protect and control against the introduction of high-risk diseases affecting hydrobiological species, whether originating from aquaculture activities for any purpose or in the wild. It mandates the isolation of any such diseases, prevention of their spread, and efforts toward their eradication.
Only twenty-three out of the total articles reviewed included definitions of offshore aquaculture or ocean aquaculture, accounting for 17% of the total. Conversely, we found only seven government documents that define offshore aquaculture or ocean aquaculture. Consistent with Figure 1, we found more definitions in the literature search that used the term “offshore aquaculture” over “ocean aquaculture.” Legal definitions tend to use “marine aquaculture” over “offshore aquaculture”, the main term used in each group of definitions could be observed in the word cloud in Figure 3.
Figure 3. Word cloud for the two groups of definitions: (A) Government document definitions, and (B) Scientific literature definitions.
Similarly, scientific publications’ definitions of offshore aquaculture often mention environmental and/or oceanographic attributes, including “energy” (1.17%), “depth” (1.17%), and “water” (1.87%), with the latter term typically referring to depth. In contrast, legal definitions tend to focus on distances or specific locations, such as “miles” (2.11%), “nautical” (2.11%), and “areas” (1.41%). Regarding the main term in Figure 4, we observe that the distance from the coast is the primary term in both literature and legal definitions. Simultaneously, the term “open ocean” or “open sea” is the second most relevant. Furthermore, definitions derived from literature often encompass a broader array of environmental characteristics, including depth, currents, and waves, while also considering technological attributes. In our examination of government documents, we identified 24 relevant sources, comprising five from the Australian government, seven from Norway, seven from New Zealand, and five from the United States.
Figure 4. Main concepts in the definition of offshore aquaculture for literature review and government document.
Of all the research and scientific papers reviewed from the countries surveyed, 37 describe the need to develop a maritime spatial planning process for offshore areas that consider other interests such as fishing, energy production, tourism, national defense, or conservation. However, only 9 of these documents conduct an empirical study to identify suitable areas for ocean aquaculture, providing criteria, parameters, and methodologies that can be replicated or adapted to other locations. The parameters used in these studies, along with the sub-models, specific weightings assigned to each criterion, and the target species for each study, are detailed in Table 1.
Table 1. Parameters, their specific weights, and sub-models utilized in determining suitable areas for offshore aquaculture as per the literature.
It is necessary to determine if there are any limitations in the current legal framework for moving forward with offshore aquaculture in Chile. The General Fisheries and Aquaculture Law (LGPA) stands as a pivotal legal framework for regulating aquaculture activity in Chile. Under this law, aquaculture is defined as “the production of hydrobiological resources organized by humans” (LGPA, Article 2, paragraph 3) and is subject to specific legal standards for its development. Recognizing various forms of aquaculture, including concessions on national public assets, private waters, and private lands, the LGPA establishes a comprehensive regulatory mechanism.
According to the LGPA, aquaculture concessions, granted by the Ministry of National Defense, have a duration of 25 years and are renewable subject to environmental and location requirements (LGPA, Article 2, paragraph 12). The process for obtaining a concession entails the submission of a technical project and compliance verification by the Undersecretariat of Fisheries and Aquaculture (Subpesca) and the maritime authority.
Moreover, the LGPA integrates with the Law on General Principles of the Environment (Law No. 19.300), which mandates environmental impact assessment for various economic activities, including aquaculture. Specifically, Article 10 of the LGPA, in conjunction with Article 3 of the Environmental Impact Assessment System Regulation, outlines the types and magnitudes of aquaculture projects subject to environmental evaluation.
The United Nations Convention on the Law of the Sea (UNCLOS), provides a comprehensive framework delineating the rights and jurisdictions of states across diverse maritime zones. Within territorial waters, internal waters, the territorial sea, and contiguous zone states exercise full sovereignty, in these areas the coastal state extends its sovereignty to the air space over the territorial sea as well as to its bed and subsoil. This authority is explicitly stated in UNCLOS, which underscores states’ rights in these areas (UNCLOS, Articles 2 and 3).
Moving beyond these areas, the Exclusive Economic Zone (EEZ) emerges as a distinct domain where coastal states possess sovereign rights for various economic activities, including exploration and exploitation of natural resources. However, it is essential for coastal states to acknowledge the rights of other states and ensure their actions align with the provisions of UNCLOS. Specifically, Articles 56 and 58 of UNCLOS outline the scope of these sovereign rights within the EEZ and emphasize the need for compatibility with international norms and agreements.
Moreover, UNCLOS acknowledges the exclusive right of coastal states to regulate activities such as exploration, exploitation, conservation, and management of hydrobiological resources, allowing to coastal state to construct and install structures for diverse economic purposes within the EEZ. This provision, as articulated in Article 60 of UNCLOS, extends to potential activities like aquaculture, providing a legal basis for coastal states to engage in such endeavors within their EEZ. However, it is crucial for any regulations governing aquaculture within the EEZ to adhere to the principles laid out in UNCLOS, particularly regarding the rights freedom of navigation, as stipulated in Article 87, thus preventing any undue interference or infringements on this fundamental right.
Based on the preceding analysis, the primary constraint hindering the progression toward offshore aquaculture in Chile pertains to the jurisdiction of the Ministry of National Defense, specifically delegated to the Undersecretary of the Armed Forces, which is limited to granting aquaculture concessions within the territorial sea. Consequently, Chile’s ability to extend its aquaculture operations into offshore regions is restricted, limited to a distance of only up to 12 nautical miles. Article 47 of the LGPA law designates the first five nautical miles, measured from the coast’s baseline, for artisanal fishing. This allocation does not restrict the granting of offshore aquaculture farms. However, to prevent potential conflicts with this economic activity in the future, it is advisable to explore areas beyond the fifth nautical mile to the west. Based on the current legal framework, offshore aquaculture farms can be granted in Chilean maritime areas within the territorial sea, located west of the 5 nautical miles measured from the normal baselines, spanning from the country’s northern to southern limits. Figure 5 provides an example of the recommended area for advancing offshore aquaculture, we showed potential areas for zones in the south, center, and north of Chile. Aquaculture could be promoted according to the environmental characteristics of each zone. Finally, the Chilean authorities should incorporate additional criteria, to determine the definitive suitable areas.
Figure 5. Potential offshore aquaculture zones within the current limitations of the legal framework in Chile.
Terminology is important for streamlining marine policy, communication, and research. A better understanding of how words or terms are used can help identify key areas of overlap and/or differences and help make terms more tractable to stakeholders. Indeed, communication between the public, managers, and scientists requires better elucidations of terms, particularly at a global scale (Froehlich et al., 2017). Within this context, the definition of offshore aquaculture holds considerable significance for the prospective industry and its regulatory framework. The delineated terms within this definition possess the potential to delineate permissible operational zones for industry and requisite technological infrastructures. In our search, we discovered that literature predominantly discusses terms associated with the physical conditions in the environment, technological characteristics of the equipment and infrastructure used for farming, and political terms related to the spatial planning, in which offshore aquaculture farms operate. For a better understanding of the results, we classified the different terms used in the definitions into three categories: physical-environmental terms (such as current speed, wind, waves, depth, and distance to the coast), technological terms (such as the type of naval structures and vessels used), and legal terms (such as borders and definitions into UNCLO).
This group contains the largest number of terms, including distance to the coast, depth, current speed, wave height, and wind, on the one hand, the last three being particularly influenced by factors such as lunar cycles, seasonal patterns, and temporal variations. Froehlich et al. (2017) observed that the literature indicates offshore conditions typically commence with current speeds ranging from 0.1 m/s to 0.5 m/s. In our investigation, we encountered only three studies incorporating “current” as a component in their definition of offshore aquaculture. These studies describe the currents as “strong currents” (Holmer, 2013), “high-energy currents” (Morro et al., 2022), and “strong ocean current circulation” (Fukae et al., 2021). However, none of these definitions specify a particular threshold to define what constitutes a “strong current”. In the case of waves, Froehlich et al. (2017) identified twelve distinct studies that referenced “waves” as a component within their definitions of offshore aquaculture, with reported wave heights ranging from 0.4 to 12 meters. In our investigation, we encountered five studies incorporating “waves” as a term in their definitions. Similar to the term current, four of these studies referred to “high waves” without specifying a particular threshold. (Silva et al., 2018; Morro et al., 2022; Visch et al., 2023) and “expose to waves” (FAO, 2013), Only one research provided a specific value for high waves in offshore conditions, indicating a threshold of 5 meters (Holmer, 2010). Moreover, there is no mention of the wave period, which is an important factor to consider. Because the increase in wave height and period leads to a non-linear increase in drag forces on the net pent (Martin et al., 2021), this can cause deformations and damage to the structures under extreme oceanographic conditions (López et al., 2024).
Some definitions of offshore aquaculture also mention the term “winds” as a differentiating factor, but to a lesser extent than the previously mentioned terms, in the study by Froehlich et al. (2017), only four research studies were identified that provided values for winds in the context of offshore aquaculture, with reported ranges spanning from 4 m/s to 35 m/s. In our investigation, only two definitions included the term winds. However, both instances merely indicated that winds in offshore areas are described as “strong” (Holmer, 2013) and without specifying numerical values, or farms are “exposed” (FAO, 2013). Including these terms in the definition of offshore aquaculture may introduce more uncertainty than clarity when delineating an offshore aquaculture site, a concern amplified by the potential impact of climate change on these physical phenomena. Furthermore, projections indicate that by the end of the century (2100), sea surface temperature (SST) will have increased by an average of 2.58°C and pH will have decreased by 0.32 units (IPCC, 2022; de Almeida et al., 2023). Consequently, potential future climate change scenarios could alter physical-environmental terms such as ocean currents (Bhanu Deepika et al., 2024) and waves (Liu et al., 2024). As a result, ranges previously defined as “high” or “strong,” which were characterized as sporadic, could become the new “normal.” This shift may necessitate redefining offshore aquaculture according to these new ranges.
On the other hand, physical environmental terms such as depth and distance from the coast do not exhibit significant seasonal or temporal variation. Consequently, these two terms systematically emerge as constants in the literature. Froehlich et al. (2017) and Watson et al. (2022) highlight the prevalence of these terms across various definitions of offshore aquaculture. Additionally, in our research, these terms exhibited consistent mentions, with 19 references to distance from the coast and 10 references to depth (Figure 4). For depth, the ranges presented in the literature mentioned offshore starting from 20 meters deep (Lester et al., 2018; Wu et al., 2024). Furthermore, other studies mention that the offshore condition begins at 30 meters (Froehlich et al., 2017), or even at 50 meters deep (Holmer, 2010; Sanz-Lazaro et al., 2021; Zheng et al., 2024).
In the Chilean context, the depths indicated as starting points for offshore conditions are often found within coastal aquaculture. According to current legislation, aquaculture farms are classified into seven categories according to the depth, production level, and characteristics of the seabed. Salmon farms with a depth greater than 60 meters are classified as category 5 (resolution No. 3612 of 2009 of the Ministry of Economy), these categories apply exclusively to farms located in channels and fjords. In the years 2021 and 2022, at least 168 and 153 category 5 salmon farms operated, respectively. This means that more than 150 farms each year were operating in sites deeper than 60 meters (SUBPESCA, 2023). In our analysis, we found that various definitions suggest distances ranging from two (Holmer, 2010; Nam et al., 2014; Zheng et al., 2024) to three kilometers (Sanz-Lazaro et al., 2021). Froehlich et al. (2017) also noted discrepancies, in his results he reports that different studies determine the distance from the coast that characterizes an offshore farm and that they can start from 0.38 nm to 25 nm, with an average of 3 nm. Although there is no clear consensus on the distance from the coast or depth at which the offshore zone begins, it is possible to state that this term refers to areas without large seasonal fluctuations. The small temporal fluctuation allows for terms such as depth and distance to the coast to be used in the definition of offshore aquaculture without major limitations, while simultaneously differentiating offshore aquaculture from coastal aquaculture.
Another term included in the definition of offshore aquaculture pertains to the technology utilized for operations in offshore aquaculture areas. Morro et al. (2022) state that specialized equipment and practices are necessary for accordance with the environmental conditions of offshore areas, additionally, Fukae et al. (2021) mention that offshore aquaculture has the ability to install larger-scale cages compared to coastal aquaculture, because, farms will have more physical space (will be larger in size) allowing for bigger farms (Fukae et al., 2021). Due to the more dynamic conditions in offshore areas, efforts have been made to design new structures that ensure reliable and safe farming, thereby preventing fish escapes and safeguarding human lives (Morro et al., 2022), The new offshore aquaculture infrastructure and equipment must withstand or be resilient to strong offshore waves, winds, and currents as well as resist corrosion and fouling (Fujita et al., 2023). In order to achieve that, there are 15 initiatives worldwide evaluating technologies to enable offshore aquaculture at the experimental level, and 18 at the commercial or pilot commercial level (Fujita et al., 2023). Consequently, today offshore aquaculture farms vary significantly in scale (Fujita et al., 2023). Among these designs, the famous Ocean Farming 1 by SalMar stands out, comprising a structure of 110 meters in diameter and 69 meters in height (Yi and Kim, 2020b), or the more traditional design used by Open Blue in Panama with submerged net pens measuring 35 meters in diameter and 24 meters in height (Welch et al., 2019). As a result, considering that offshore aquaculture technology is still in development, it would be unwise to include any technological term in the legal definition of offshore aquaculture.
The last group of terms used in the definition of offshore aquaculture is the political terms, as those outlined in the UNCLOS agreement could offer a viable alternative. This is exemplified by the proposed legislation exclusively aimed at regulating offshore aquaculture, as seen in the AQUAA bill 2023, which defines offshore aquaculture as “aquaculture conducted in the exclusive economic zone.” These political divisions would facilitate the differentiation between traditional aquaculture, typically conducted in territorial or inland sea waters and offshore aquaculture. Today the National Oceanic and Atmospheric Administration (NOAA) classifies “Oceanic Aquaculture” as the aquaculture “which takes place in Aquaculture Opportunity Areas, in federal waters (from mile 3 to 200)” (Riley et al., 2021). In the case of New Zealand, offshore aquaculture is defined under the Resource Management (National Environmental Standards for Marine Aquaculture) Regulations 2020 as follows: “Offshore marine farm means any of the 5 existing marine farms initially granted coastal permits before the commencement of these regulations and located according to Schedule 2, and marine farms granted coastal permits after the commencement of these regulations but not located within 500 meters of mean high-water springs or within harbors and other areas described in Schedule 3.” In Schedule 2, the government of New Zealand provides the geographic coordinates of the five offshore aquaculture farms, while Schedule 3 outlines the geographic boundaries in various areas of New Zealand where offshore aquaculture farms can be located.
Similarly, Norwegian authorities utilize a distinct definition when examining potential new offshore aquaculture sites. Although this definition has not yet acquired legal status, it draws a clear distinction between aquaculture operations in traditional farming sites and prospective offshore farms. It defines offshore aquaculture as “aquaculture that takes place further out at sea than is common today. In accordance with the salmon allocation regulations” (Fiskeridirektoratet, 2023). It is important to note that this definition is directly translated from Norwegian and may vary from an official translation provided by the Norwegian state. Following the previous direction and the recommendations outlined earlier, the definition of offshore aquaculture in Chile could be “all aquaculture activities conducted west of the artisanal fishing reserve area, between the Exclusive Economic Zone and the territorial sea”.
The identification of appropriate areas for marine aquaculture development is a critical concern for spatial planning. A site selection study prior to determining the feasibility of offshore aquaculture by Benetti et al., 2010, addresses this issue. This research outlines essential criteria for selecting offshore aquaculture sites, including logistical, environmental, and regulatory factors. Furthermore, the study recommends assessing the economic and social conditions of potential locations, examining the hydrography of the area, and utilizing numerical models to estimate the environment’s carrying capacity based on the food supplied to the fish (Benetti et al., 2010). Another pertinent study in this field is the research conducted by Gentry et al. (2017). They devised a comprehensive methodology that integrates scientific analysis to aid spatial planning for offshore aquaculture development. Their suggestions entail selecting sites characterized by strong currents and deeper water to mitigate impacts on the benthic ecosystem and minimizing connectivity between farms to manage disease outbreaks (Gentry et al., 2017).
The criteria and priorities chosen by decision-makers strongly determine the results of identifying suitable areas. For example, the NOAA study in the Gulf of Mexico and Baja California evaluated general criteria without targeting a specific species, aiming to open marine spaces for all species that can utilize these areas (Riley et al., 2021). In contrast, the study conducted by the Norwegian government focused solely on Atlantic salmon as the target species and could incorporate animal welfare criteria over operational or structural criteria (Fiskeridirektoratet, 2019). All the studies were multi-criteria, considering more than one parameter or constraint to decide which site is the most optimal for offshore aquaculture.
In studies that construct models for offshore aquaculture, current velocity (m/s) is the parameter most commonly chosen to explore the suitability (Gimpel et al., 2015; Weiss et al., 2018; Fiskeridirektoratet, 2019; Garavelli et al., 2022; Cosgrove et al., 2023; Hasankhani et al., 2023). In most of the studies, current velocity is used as a limiting factor for the structures. For example, in the work of Weiss et al. (2018) utilized a 50-year dataset to determine suitable areas for cages, and the current was assessed to evaluate whether the structure could withstand the conditions, they employed a generic cage across three distinct environmental scenarios: high exposure (more than 1.5 m/s), substantial exposure (1.0–1.5 m/s), and moderate exposure (0.5–1.0 m/s). The authors found that current velocities were not a limiting factor, with percentages of suitable areas exceeding 95% across all three conditions for each ocean (Weiss et al., 2018). The previous findings are corroborated by Garavelli et al. (2022), who found that current velocities were not a limiting factor. In their work, they identified suitable areas for offshore aquaculture and wave energy plants. Their research delineated suitable areas within the range of 0 to 1 m/s, employing the HYCOM hydrodynamic model to estimate currents in the study area.
Another point of view with respect to current velocity is in the research of Fiskeridirektoratet (2019) on a study commissioned by the Norwegian Ministry of Trade, Industry, and Fisheries, also involving the Directorate of Fisheries in partnership with the Institute of Marine Research. Their objective was to map and identify areas potentially suitable for offshore aquaculture. However, this mapping was confined to opportunity areas located beyond one nautical mile outside the baseline and within the exclusive economic zone, the baseline for the Norwegian authority is the line that defines the political maritime territory, according to UNCLOS technical requirements (Geirr Harsson and Preiss, 2012). In this study, the authors utilized ocean currents as a constraint on the swimming capacity of salmon. The aim of the Norwegian authority is to maintain optimal conditions for salmon welfare. They studied the Critical Swimming Velocity (CSV) for Atlantic salmon, the CSV is the maximum prolonged swimming speed and is obtained in laboratory trials by using swim tunnel systems (Hvas et al., 2021). Fiskeridirektoratet (2019) referenced the findings of the report by Hvas et al. (2021). In their research, the authors observed that the critical velocity varies with water temperature, oxygen concentration, fish size, and feeding status because after eating the fish reduces swimming capacity. The Norwegian authority’s model aims to identify locations where ocean currents do not exceed 80% of the salmon’s critical swimming velocity. With these criteria and the other parameters of the model, the State of Norway was able to identify 11 probable offshore aquaculture zones.
The second most selected parameters to study the suitability for offshore aquaculture are bathymetry (m), wave height (m), and sea surface temperature (°c) (Dapueto et al., 2015; Gimpel et al., 2015; Weiss et al., 2018; Fiskeridirektoratet, 2019; Garavelli et al., 2022; Yu et al., 2022; Cosgrove et al., 2023; Hasankhani et al., 2023). Bathymetry appears to be one of the primary limiting parameters for offshore farming structures, in the study conducted by NOAA to determine the aquaculture opportunity areas in the Gulf of Mexico, it was determined that the minimum depth to allow proper anchoring of the cages was 36.5 meters (120 feet). Dapueto et al. (2015) considered depths greater than 50 meters and less than 10 meters as limitations for their model, since depths greater than 50 meters increase costs and make anchoring more difficult. Garavelli et al. (2022) defined suitable areas within the range of 25 to 100 meters, depths greater than 100 meters are unfeasible for combining offshore farms and wave energy plants. Hasankhani et al. (2023), utilized a broader range of 0–250 meters, with a constraint over 250 meters. In general, depths over 200 meters are increasingly expensive as the depth increases (Gentry et al., 2017), longer mooring lines will be required in deeper waters, and optimal configurations may vary (Morro et al., 2022), with the development of new offshore technologies, the range in which an offshore farm can be installed will expand to deeper waters.
In addition to bathymetry, areas previously designated for other purposes have also been considered as restrictions. For instance, Dapueto et al. (2015) incorporated areas such as marine protected areas or diving sites as constraints into their model, this could be particularly relevant for countries where there is a conflict between coastal aquaculture and Marine Protected Areas (MPA). This is particularly relevant for Chile, where salmon farms often operate in MPA areas and generate social conflict. However, certain uses like military zones were not deemed as limiting constraints; instead, they were assigned lower percentages in the models. For example, Gimpel et al. (2015) and Riley et al. (2021) assigned weights of 10% and 25%, respectively, to military areas compared to other parameters considered in their models, as shown in Table 1.
Concerning the structure of the models used to estimate suitable areas for offshore aquaculture, a balanced model is built with the same weight for each parameter, an example of this is the model of Riley et al. (2021) which assigns equal weight to each of its sub-models (25%), which means that each of the four sub-models (National Security, Industry & Navigation, Natural & Cultural Resources, and Fishing & Aquaculture) is of equal importance. Conversely, Barillé et al. (2020) assess different scenarios by adjusting the values of their sub-models to determine the best approach for allocating offshore oyster farms, in their study, Barillé et al. (2020) evaluate which submodel most limits offshore areas by considering three submodels: socio-economic submodels, optimal growth, and environmental conditions. Dapueto et al. (2015), meanwhile, employ varied weights in their sub-models, with socioeconomic factors carrying the most weight in the outcome of their model.
Regarding the constraints of the models, we found that there is a wide variety of criteria. For example, Natura 2000, a network of protected areas, emerged as the most limiting factor for offshore aquaculture in Europe (Barillé et al., 2020), as strong environmental studies are required in these areas. In the study by Weiss et al. (2018), the primary limiting factors for offshore aquaculture areas were biological criteria, specifically salinity and sea surface temperature. Similarly, Yu et al. (2022) found sea surface temperature to be the limiting factor in their model of the Yellow Sea. On the other hand, Garavelli et al. (2022), explored suitable areas for aquaculture and energy generation, for their research, the most limiting factor was wave-generated power, which was not an inherent parameter of farm structure or the biology of the target species (Vázquez Pinillos et al., 2023). Similarly, Riley et al. (2021) considered the presence of active oil and gas wells, shipping lanes, coral and hardbottom habitats, environmental sensors, and buoys as constraints for their model.
To structure the model for estimating suitable areas for offshore aquaculture, it is interesting to analyze the two different approaches used by Riley et al., 2021, and Fiskeridirektoratet, 2019. The first study emerged from Executive Order 13921, which aims to promote competition and growth in the seafood production industry (Riley et al., 2021). Therefore, the model developed by the NOAA does not have a specific target species and does not employ specific biological parameters. On the other hand, the model developed by Fiskeridirektoratet, 2019, aims to expand aquaculture in Norway, specifically focusing on Atlantic salmon. Thus, significant factors considered in this model include fish welfare, disease proliferation among these fish, and their interaction with coastal aquaculture farms. It is the responsibility of the legal authorities, who possess the legitimacy to make decisions, to select the appropriate criteria, parameters, and methodology to determine suitable sites.
If a new aquaculture industry is to be created, we should learn from the past and consider the opinions of different stakeholders and local communities. Decision-makers should incorporate these opinions to determine suitable areas in collaboration with these stakeholders from the beginning. As outlined by Riley et al. (2021), this participatory approach involves conducting workshops with stakeholders to document the permitting framework and evaluate opportunities for offshore aquaculture development. These workshops also facilitated the establishment of initial parameters essential for commencing the study of aquaculture opportunity zones. Riley et al. (2021) indicate that over 175 one-on-one sessions were conducted with stakeholders and experts to inform their methodology and analysis. In the case of Norway, a new process was developed with extensive participation from stakeholders to determine the most suitable methodology for identifying offshore areas (Fiskeridirektoratet, 2023), The process establishes at least three instances where public consultations are deemed necessary to define sampling methodologies and analyze the results. These examples aim to foster greater social consensus during the industry’s expansion.
In conclusion, the establishment of offshore aquaculture encounters various domestic constraints and necessitates a comprehensive understanding of legal frameworks, technological factors, and environmental parameters, as well as social issues. Chile’s legal framework, governed by the LGPA and supplemented by international agreements like the UNCLOS, does not pose a constraint for granting offshore farms within Chilean waters. However, limitations arise from jurisdictional constraints within the Undersecretariat of the Armed Forces, which can solely issue maritime concessions in the territorial sea. If Chile intends to expand its aquaculture activities beyond this zone, modifications to the legal framework are imperative to broaden the jurisdiction of the Undersecretariat of the Armed Forces.
The precise definition of offshore aquaculture holds paramount importance for both effective governance and industry advancement. While physical parameters like depth and distance from the shore commonly serve as reference points, consensus regarding the specific criteria marking the transition to open ocean conditions varies considerably depending on the intended location of the offshore farm. Nonetheless, employing criteria that remain constant over time, unaffected by seasonal fluctuations, such as depth and distance from shore, could facilitate the establishment of a legal definition applicable uniformly across territories. However, incorporating technological aspects into the definition might prove counterproductive due to the dynamic nature of aquaculture technology, with diverse projects worldwide proposing various technological solutions. Instead, aligning with established policy frameworks such as the territorial sea divisions delineated in the UNCLOS agreement could offer a more standardized approach to defining offshore aquaculture.
Parameters for determining the feasibility of offshore aquaculture include bathymetry, wave height, sea surface temperature, and current velocity. These parameters vary in importance depending on the specific objectives of the model and the environmental conditions of the target area. Additionally, factors such as stakeholder and local community support, as well as previous uses of the space, play significant roles in determining suitable areas for offshore aquaculture. The structure of models used to estimate suitable areas for offshore aquaculture varies, with some employing a balanced approach while others prioritize specific factors such as socioeconomic considerations. Understanding these different approaches is essential for policymakers and stakeholders involved in offshore aquaculture development.
CC-M: Conceptualization, Methodology, Visualization, Writing – original draft, Writing – review & editing. DF: Conceptualization, Investigation, Writing – original draft, Writing – review & editing. CH: Investigation, Supervision, Writing – original draft, Writing – review & editing. FP: Conceptualization, Data curation, Formal analysis, Investigation, Writing – original draft, Writing – review & editing. DB: Resources, Supervision, Writing – original draft, Writing – review & editing.
The author(s) declare financial support was received for the research, authorship, and/or publication of this article. Project funding was given by the Chilean Economic Development Agency (CORFO), under the program for the technological development of ocean aquaculture in Chile 2023 (17PTECAO-84019).
A heartfelt thank you to the entire team at the Centro de Biotecnologia de Sistemas, comprising members from both Fraunhofer Chile and Universidad Andrés Bello, for their invaluable contributions to the implementation of this program. Special appreciation goes to Fabian Avilés, the general director of the ocean aquaculture program, for his unwavering support and guidance to the team throughout.
The authors declare that the research was conducted in the absence of any commercial or financial relationships that could be construed as a potential conflict of interest.
All claims expressed in this article are solely those of the authors and do not necessarily represent those of their affiliated organizations, or those of the publisher, the editors and the reviewers. Any product that may be evaluated in this article, or claim that may be made by its manufacturer, is not guaranteed or endorsed by the publisher.
Aanesen M., Czajkowski M., Lindhjem H., Navrud S. (2023). Trade-offs in the transition to a blue economy - Mapping social acceptance of aquaculture expansion in Norway. Sci. Total Environ. 859, 160199. doi: 10.1016/j.scitotenv.2022.160199
Barillé L., Le Bris A., Goulletquer P., Thomas Y., Glize P., Kane F., et al. (2020). Biological, socio-economic, and administrative opportunities and challenges to moving aquaculture offshore for small French oyster-farming companies. Aquaculture 521, 735045. doi: 10.1016/j.aquaculture.2020.735045
Barton J. R., Fløysand A. (2010). The political ecology of Chilean salmon aquaculture 1982–2010: A trajectory from economic development to global sustainability. Global Environ. Change 20, 739–752. doi: 10.1016/j.gloenvcha.2010.04.001
Baumas C., Bizic M. (2024). A focus on different types of organic matter particles and their significance in the open ocean carbon cycle. Prog. Oceanogr. 224, 103233. doi: 10.1016/j.pocean.2024.103233
Benetti D. D., Benetti G. I., Rivera J. A., Sardenberg B., O’Hanlon B. (2010). Site selection criteria for open ocean aquaculture. Mar. Technol. Soc. J. 44, 22–35. doi: 10.4031/MTSJ.44.3.11
Bhanu Deepika P., Mohan S., Srinivas G. (2024). Intercomparison of tropical Indian Ocean circulation in ocean reanalysis and evaluation in CMIP6 climate models. Dynam. Atmos. Oceans 106, 101456. doi: 10.1016/j.dynatmoce.2024.101456
Bonelli A. G., Loisel H., Jorge D. S. F., Mangin A., d’Andon O. F., Vantrepotte V. (2022). A new method to estimate the dissolved organic carbon concentration from remote sensing in the global open ocean. Remote Sens. Environ. 281, 113227. doi: 10.1016/j.rse.2022.113227
CBC (2015). New Nova Scotia aquaculture regulations “more accountable.” (CBC News). Available at: https://www.cbc.ca/news/canada/nova-scotia/nova-scotia-aquaculture-fish-farms-moratorium-1.3288661.
Cantillo J., Martín J. C., Román C. (2023). Understanding consumers’ perceptions of aquaculture and its products in Gran Canaria island: Does the influence of positive or negative wording matter? Aquaculture 562, 738754. doi: 10.1016/j.aquaculture.2022.738754
Chávez C., Dresdner J., Figueroa Y., Quiroga M. (2019). Main issues and challenges for sustainable development of salmon farming in Chile: a socio-economic perspective. Rev. Aquacult. 11, 403–421. doi: 10.1111/raq.12338
Cosgrove S., Murphy W., Farrell C., O’Keeffe D., O’Brien N. (2023). “An MCASS tool for enhanced offshore aquaculture site selection and successful licencing acquisition,” OCEANS 2023 - Limerick, Limerick, Ireland, 1–5. doi: 10.1109/OCEANSLimerick52467.2023.10244353
Dapueto G., Massa F., Costa S., Cimoli L., Olivari E., Chiantore M., et al. (2015). A spatial multi-criteria evaluation for site selection of offshore marine fish farm in the Ligurian Sea, Italy. Ocean Coast. Manage. 116, 64–77. doi: 10.1016/j.ocecoaman.2015.06.030
de Almeida M., da Fonseca L. T., Pinheiro B. R., Pereira N. S., de Jesus Flores Montes M. (2023). Daily variability of pH and temperature in seawater from a near-pristine oceanic atoll, Southwest Atlantic. Mar. pollut. Bull. 196, 115670. doi: 10.1016/j.marpolbul.2023.115670
FAO (2013). A global assessment of potential for offshore mariculture development from a spatial perspective. Available online at: https://openknowledge.fao.org/items/63e5c66d-6be9-4e28-8e15-a77361872079 (Accessed 5.4.24).
Fiskeridirektoratet (2019). Kartlegging og identifisering av områder egnet for havbruk til havs (Kudos). Available online at: https://kudos.dfo.no/dokument/31098/kartlegging-og-identifisering-av-omrader-egnet-for-havbruk-til-havs (Accessed 4.26.24).
Fiskeridirektoratet (2023). Forslag til utredningsprogram for havbruk til havs (Fiskeridirektoratet). Available online at: https://www.fiskeridir.no/Akvakultur/Dokumenter/Hoeringer/forslag-til-utredningsprogram-for-havbruk-til-havs (Accessed 5.5.24).
Froehlich H. E., Gentry R. R., Lester S. E., Cottrell R. S., Fay G., Branch T. A., et al. (2021). Securing a sustainable future for US seafood in the wake of a global crisis. Mar. Policy 124, 104328. doi: 10.1016/j.marpol.2020.104328
Froehlich H. E., Smith A., Gentry R. R., Halpern B. S. (2017). Offshore aquaculture: I know it when I see it. Front. Mar. Sci. 4. doi: 10.3389/fmars.2017.00154
Fujita R., Brittingham P., Cao L., Froehlich H., Thompson M., Voorhees T. (2023). Toward an environmentally responsible offshore aquaculture industry in the United States: Ecological risks, remedies, and knowledge gaps. Mar. Policy 147, 105351. doi: 10.1016/j.marpol.2022.105351
Fukae K., Imai T., Yamabe S., Arai K., Kobayashi T. (2021). “Development of observation device with multi sensor platform for underwater aquaculture cages, in: 2021 IEEE 45th annual computers, software, and applications conference (COMPSAC),” in Presented at the 2021 IEEE 45th Annual Computers, Software, and Applications Conference (COMPSAC), Madrid, Spain. 1633–1638. doi: 10.1109/COMPSAC51774.2021.00244
Garavelli L., Freeman M. C., Tugade L. G., Greene D., McNally J. (2022). A feasibility assessment for co-locating and powering offshore aquaculture with wave energy in the United States. Ocean Coast. Manage. 225, 106242. doi: 10.1016/j.ocecoaman.2022.106242
Garlock T., Asche F., Anderson J., Bjørndal T., Kumar G., Lorenzen K., et al. (2020). A global blue revolution: aquaculture growth across regions, species, and countries. Rev. Fish. Sci. Aquacult. 28, 107–116. doi: 10.1080/23308249.2019.1678111
Garza-Gil M. D., Vázquez-Rodríguez M. X., Varela-Lafuente M. M. (2016). Marine aquaculture and environment quality as perceived by Spanish consumers. Case shellf. demand. Mar. Policy 74, 1–5. doi: 10.1016/j.marpol.2016.09.011
Geirr Harsson B., Preiss G. (2012). Norwegian baselines, maritime boundaries and the UN convention on the law of the sea. Arct. Rev. 3, 108–129. doi: 10.23865/arctic.v3.28
Gentry R. R., Lester S. E., Kappel C. V., White C., Bell T. W., Stevens J., et al. (2017). Offshore aquaculture: Spatial planning principles for sustainable development. Ecol. Evol. 7, 733–743. doi: 10.1002/ece3.2637
Gimpel A., Stelzenmüller V., Grote B., Buck B. H., Floeter J., Núñez-Riboni I., et al. (2015). A GIS modelling framework to evaluate marine spatial planning scenarios: Co-location of offshore wind farms and aquaculture in the German EEZ. Mar. Policy 55, 102–115. doi: 10.1016/j.marpol.2015.01.012
Gordon J. D. M., Shipley O. N. (2019). “Open Ocean Fisheries for Deep-Water Species☆,” in Encyclopedia of Ocean Sciences, 3rd ed. Eds. Cochran J. K., Bokuniewicz H. J., Yager P. L. (Academic Press, Oxford), 460–469. doi: 10.1016/B978-0-12-409548-9.11340-5
Hasankhani A., Ewig G., McCabe R., Won E. T., Haji M. (2023). Marine spatial planning of a wave-powered offshore aquaculture farm in the northeast U.S. OCEANS Limerick. 1628–1637. doi: 10.1109/OCEANSLimerick52467.2023.10244332
Hays G. C., Cerritelli G., Esteban N., Rattray A., Luschi P. (2020). Open ocean reorientation and challenges of island finding by sea turtles during long-distance migration. Curr. Biol. 30, 3236–3242.e3. doi: 10.1016/j.cub.2020.05.086
Hersoug B. (2022). One country, ten systems” – The use of different licensing systems in Norwegian aquaculture. Mar. Policy 137, 104902. doi: 10.1016/j.marpol.2021.104902
Holmer M. (2010). Environmental issues of fish farming in offshore waters: perspectives, concerns and research needs. Aquacult. Environ. Interact. 1, 57–70. doi: 10.3354/aei00007
Holmer M. (2013). “Sustainable development of marine aquaculture off-the-coast and offshore – a review of environmental and ecosystem issues and future needs in temperate zones,” in Expanding mariculture farther offshore – Technical, environmental, spatial and governance challenges, FAO Fisheries and Aquaculture Proceedings, Vol. 24. 135–172. Available at: https://www.fao.org/4/i3092e/i3092e.pdf.
Hvas M., Folkedal O., Oppedal F. (2021). Fish welfare in offshore salmon aquaculture. Rev. Aquacult. 13, 836–852. doi: 10.1111/raq.12501
IPCC (2022). The Ocean and Cryosphere in a Changing Climate: Special Report of the Intergovernmental Panel on Climate Change. [Pörtner H.-O., Roberts D.C., Tignor M., Poloczanska E.S., Mintenbeck K, Alegría A, et al (eds.)] 1st ed (Cambridge, UK and New York, NY, USA: Cambridge University Press), 3056 pp. doi: 10.1017/9781009157964
Jacquet J., Franks B., Browning H., Donoso A., Hayek M., Hessler K., et al. (2024). Away from an offshore aquaculture industry in the United States. Mar. Policy 160, 105986. doi: 10.1016/j.marpol.2023.105986
Joseph J., Shipley O. N., Siskey M. R. (2019). “Open Ocean Fisheries for Large Pelagic Species☆,” in Encyclopedia of Ocean Sciences, 3rd ed. Eds. Cochran J. K., Bokuniewicz H. J., Yager P. L. (Academic Press, Oxford), 470–479. doi: 10.1016/B978-0-12-409548-9.11375-2
Knapp G., Rubino M. C. (2016). The political economics of marine aquaculture in the United States. Rev. Fish. Sci. Aquacult. 24, 213–229. doi: 10.1080/23308249.2015.1121202
Lester S. E., Gentry R. R., Kappel C. V., White C., Gaines S. D. (2018). Offshore aquaculture in the United States: Untapped potential in need of smart policy. Proc. Natl. Acad. Sci. United States America 115, 7162–7165. doi: 10.1073/pnas.1808737115
Liu J., Li R., Li S., Meucci A., Young I. R. (2024). Increasing wave power due to global climate change and intensification of Antarctic Oscillation. Appl. Energy 358, 122572. doi: 10.1016/j.apenergy.2023.122572
López J., Hurtado C. F., Toledo J. P., Suazo G., Zamora V., Queirolo D., et al. (2024). Structural integrity of a submersible sea cage exposed to extreme oceanographic conditions. J. World Aquacult. Soc. 55, e13052. doi: 10.1111/jwas.13052
Lorena R., Karina Y., Ekaterina P., Cecilia B., Sebastián Z., Miguel B. J., et al. (2022). Impacts of COVID-19 on the Chilean salmon: A first approach to the effects of the pandemic in the industry. Mar. Policy 144, 105185. doi: 10.1016/j.marpol.2022.105185
Luna M., Llorente I., Luna L. (2023). A conceptual framework for risk management in aquaculture. Mar. Policy 147, 105377. doi: 10.1016/j.marpol.2022.105377
Martin T., Tsarau A., Bihs H. (2021). A numerical framework for modelling the dynamics of open ocean aquaculture structures in viscous fluids. Appl. Ocean Res. 106, 102410. doi: 10.1016/j.apor.2020.102410
Mazur N. A., Curtis A. L. (2008). Understanding community perceptions of aquaculture: lessons from Australia. Aquacult. Int. 16, 601–621. doi: 10.1007/s10499-008-9171-0
Morro B., Davidson K., Adams T. P., Falconer L., Holloway M., Dale A., et al. (2022). Offshore aquaculture of finfish: Big expectations at sea. Rev. Aquacult. 14, 791–815. doi: 10.1111/raq.12625
Nam H., An S., Kim C.-H., Park S.-H., Kim Y.-W., Lim S.-H. (2014). “Remote monitoring system based on ocean sensor networks for offshore aquaculture, in: 2014 Oceans - St. John’s,” in Presented at the 2014 Oceans - St. John’s, St. John’s, NL, Canada. 1–7. doi: 10.1109/OCEANS.2014.7003046
Nathan Young R. M. The Aquaculture Controversy in (UBC Press). Available online at: https://www.ubcpress.ca/the-aquaculture-controversy-in-Canada (Accessed 1.12.24).
Noakes D. J., Fang L., Hipel K. W., Kilgour D. M. (2003). An examination of the salmon aquaculture conflict in British Columbia using the graph model for conflict resolution. Fish. Manage. Ecol. 10, 123–137. doi: 10.1046/j.1365-2400.2003.00336.x
Olsen M. S., Amundsen V. S., Osmundsen T. C. (2023). Exploring public perceptions and expectations of the salmon aquaculture industry in Norway: A social license to operate? Aquaculture 574, 739632. doi: 10.1016/j.aquaculture.2023.739632
Pham C. K., Estevez S. G., Pereira J. M., Herrera L., Rodríguez Y., Domínguez-Hernández C., et al. (2023). Three-dimensional evaluation of beaches of oceanic islands as reservoirs of plastic particles in the open ocean. Sci. Total Environ. 900, 165798. doi: 10.1016/j.scitotenv.2023.165798
Poblete E. G., Drakeford B. M., Ferreira F. H., Barraza M. G., Failler P. (2019). The impact of trade and markets on Chilean Atlantic salmon farming. Aquacult. Int. 27, 1465–1483. doi: 10.1007/s10499-019-00400-7
Riley K. L., Wickliffe L. C., Jossart J. A., Mackay J. K., Randall A. L., Bath G. E., et al. (2021). An Aquaculture Opportunity Area Atlas for the U.S. Gulf of Mexico. NOAA Technical Memorandum NOS NCCOS 299, 545. doi: 10.25923/8CB3-3R66
Sambolino A., Iniguez E., Herrera I., Kaufmann M., Dinis A., Cordeiro N. (2023). Microplastic ingestion and plastic additive detection in pelagic squid and fish: Implications for bioindicators and plastic tracers in open oceanic food webs. Sci. Total Environ. 894, 164952. doi: 10.1016/j.scitotenv.2023.164952
Sanz-Lazaro C., Casado-Coy N., Calderero E. M., Villamar U. A. (2021). The environmental effect on the seabed of an offshore marine fish farm in the tropical Pacific. J. Environ. Manage. 300, 113712. doi: 10.1016/j.jenvman.2021.113712
Silva D., Rusu E., Guedes Soares C. (2018). The effect of a wave energy farm protecting an aquaculture installation. Energies 11, 2109. doi: 10.3390/en11082109
SUBPESCA (2023). INFORME AMBIENTAL de la ACUICULTURA. Undersecretary of Fisheries and Aquaculture of Chile. Available at: https://www.subpesca.cl/portal/616/articles-120345_documento.pdf.
The Fish Site (2021). Encouraging results for submersible copper salmon farm (The Fish Site). Available online at: https://thefishsite.com/articles/encouraging-results-for-submersible-copper-salmon-farm (Accessed 4.12.24).
Troell M., Joyce A., Chopin T., Neori A., Buschmann A. H., Fang J.-G. (2009). Ecological engineering in aquaculture — Potential for integrated multi-trophic aquaculture (IMTA) in marine offshore systems. Aquaculture 297, 1–9. doi: 10.1016/j.aquaculture.2009.09.010
Uglem I., Karlsen Ø., Sanchez-Jerez P., Sæther B.-S. (2014). Impacts of wild fishes attracted to open-cage salmonid farms in Norway. Aquacult. Environ. Interact. 6, 91–103. doi: 10.3354/aei00112
Vázquez Pinillos F. J., Barragán Muñoz J. M., Ther Ríos F., Martínez González G. O. (2023). Diagnosis of the coastal management model in Chile: The island and the sea of Chiloé governance. Region. Stud. Mar. Sci. 68, 103242. doi: 10.1016/j.rsma.2023.103242
Visch W., Layton C., Hurd C. L., Macleod C., Wright J. T. (2023). A strategic review and research roadmap for offshore seaweed aquaculture—A case study from southern Australia. Rev. Aquacult. 15, 1467–1479. doi: 10.1111/raq.12788
Watson L., Falconer L., Dale T., Telfer T. C. (2022). ‘Offshore’ salmon aquaculture and identifying the needs for environmental regulation. Aquaculture 546, 737342. doi: 10.1016/j.aquaculture.2021.737342
Weiss C. V. C., Ondiviela B., Guanche R., Castellanos O. F., Juanes J. A. (2018). A global integrated analysis of open sea fish farming opportunities. Aquaculture 497, 234–245. doi: 10.1016/j.aquaculture.2018.07.054
Welch A. W., Knapp A. N., El Tourky S., Daughtery Z., Hitchcock G., Benetti D. (2019). The nutrient footprint of a submerged-cage offshore aquaculture facility located in the tropical Caribbean. J. World Aquacult. Soc. 50, 299–316. doi: 10.1111/jwas.12593
Whitmarsh D., Palmieri M. G. (2009). Social acceptability of marine aquaculture: The use of survey-based methods for eliciting public and stakeholder preferences. Mar. Policy 33, 452–457. doi: 10.1016/j.marpol.2008.10.003
Wu D., Zhu Z., Yang Z. (2024). Mapping the potential spatial distribution for offshore finfish aquaculture in China under climate change. Aquaculture 586, 740815. doi: 10.1016/j.aquaculture.2024.740815
Yi S., Kim S. (2020a). Economic value of coastal environment improvement programs in aquaculture zones in South Korea. Ocean Coast. Manage. 195, 105277. doi: 10.1016/j.ocecoaman.2020.105277
Yi S., Kim W. (2020b). Potential for offshore aquaculture development in North Korea: Focusing on Atlantic salmon farming. Mar. Policy 119, 104092. doi: 10.1016/j.marpol.2020.104092
Yokoyama H. (2002). Impact of fish and pearl farming on the benthic environments in Gokasho Bay: Evaluation from seasonal fluctuations of the macrobenthos. Fish. Sci. 68, 258–268. doi: 10.1046/j.1444-2906.2002.00420.x
Yu S.-E., Dong S.-L., Zhang Z.-X., Zhang Y.-Y., Sarà G., Wang J., et al. (2022). Mapping the potential for offshore aquaculture of salmonids in the Yellow Sea. Mar. Life Sci. Technol. 4, 329–342. doi: 10.1007/s42995-022-00141-2
Zhao Y., Guan C., Bi C., Liu H., Cui Y. (2019). Experimental investigations on hydrodynamic responses of a semi-submersible offshore fish farm in waves. J. Mar. Sci. Eng. 7, 238. doi: 10.3390/jmse7070238
Keywords: offshore aquaculture, ocean aquaculture, environment, spatial planning, mariculture
Citation: Carroza-Meza C, Fuentes D, Hurtado CF, Palacio F and Benetti D (2024) Recommendations for facilitating offshore aquaculture: lessons from international experience. Front. Aquac. 3:1428206. doi: 10.3389/faquc.2024.1428206
Received: 06 May 2024; Accepted: 06 August 2024;
Published: 30 August 2024.
Edited by:
Kevin Gerald Heasman, Cawthron Institute, New ZealandReviewed by:
Jonathan Van Senten, Virginia Tech, United StatesCopyright © 2024 Carroza-Meza, Fuentes, Hurtado, Palacio and Benetti. This is an open-access article distributed under the terms of the Creative Commons Attribution License (CC BY). The use, distribution or reproduction in other forums is permitted, provided the original author(s) and the copyright owner(s) are credited and that the original publication in this journal is cited, in accordance with accepted academic practice. No use, distribution or reproduction is permitted which does not comply with these terms.
*Correspondence: Carlos Carroza-Meza, Y2hjOTlAbWlhbWkuZWR1
Disclaimer: All claims expressed in this article are solely those of the authors and do not necessarily represent those of their affiliated organizations, or those of the publisher, the editors and the reviewers. Any product that may be evaluated in this article or claim that may be made by its manufacturer is not guaranteed or endorsed by the publisher.
Research integrity at Frontiers
Learn more about the work of our research integrity team to safeguard the quality of each article we publish.