- 1Department of Ecology, Evolution, and Marine Biology, University of California, Santa Barbara, CA, United States
- 2Baskin School of Engineering, University of California, Santa Cruz, CA, United States
- 3Environmental Studies, University of California, Santa Barbara, CA, United States
Marine co-location, i.e., multiple fixed ocean activities operating in the same place and at the same time, can maximize the space- and resource-use efficiency in crowded seascapes. While interest grows, commercial use is nascent and the collective benefits or limitations of co-locating aquatic food and clean energy remains scattered throughout the literature. In this study, we synthesize multinational findings of co-location scientific publications (N = 102) to better understand the patterns and knowledge gaps at the co-located ocean food-energy nexus. We track and compare food (aquaculture) and energy (tidal, offshore wind, and wave) co-located ocean activities, noting the focus (e.g., ecological), motivation (e.g., impact/risk), and assessment type (e.g., modeling), as well as nine key metrics of interest (depth, distance from shore, aquaculture yield, etc.), mainly for aquaculture co-location. We found the number of annual co-location publications increased over time and space but are largely concentrated in the North Sea (n = 39). We also found about half of publications include aquaculture, one-third of publications report at least one metric – reporting aquaculture yield was particularly rare (n = 1) – and few studies focused on impact/risk (n = 7). However, conducting a targeted post-hoc evaluation of North Sea gray literature (N = 61), due to this region’s importance in the field, showed more coverage of impacts/risk (e.g., liability) and similar attention to aquaculture. Of the scientific papers that did report metrics, the ranges of depth and distance exceeded those reported for standalone sectors, indicating co-location could be facilitating a “push” of ocean activities into farther offshore and/or deeper exposed waters. Ultimately, while aquaculture is commonly cited in the co-location literature, the shortage of metrics, like aquaculture yield, and possible impact/risk evaluations – though gray literature can provide critical insights – emphasizes the need for knowledge sharing and modeling to address and explore the uncertainty, especially for co-located aquaculture production. This study provides a needed snapshot of marine co-location, particularly in emerging regions, highlighting gaps in understanding aquaculture-energy potential in the oceans.
1 Introduction
Globally, there is a growing need for ocean space for the expansion of renewable energy and aquatic food production (McCauley et al., 2015; Lester et al., 2018a; Jouffray et al., 2020; FAO, 2022; GWEC, 2023). However, marine waters are already congested with competing and often opposing sectors, challenging new and/or less prioritized sectors to “fit in” (Tiller et al., 2013; Bull and Love, 2019). The emergence of ocean-based clean energy and seafood sectors could usher in a new era of sustainable and synergistic marine use and management (Lester et al., 2018b; LiVecchi et al., 2019). On the other hand, poor planning of this transition could lead to a breakdown of ocean industries, cascading environmental threats, and the displacement of jobs, both locally and globally (Halpern et al., 2008; Lester et al., 2013; O’Hara et al., 2021). For busy seascapes to sustainably maintain or expand a “blue economy” (Smith-Godfrey, 2016), research must be conducted to shed light on how the various ocean sectors interact, highlighting areas of synergy and discord.
Aquaculture is increasing in significance on the global food stage, now exceeding wild capture fisheries in total volume (Costello et al., 2020; FAO, 2022). However, how to make space in the ocean for marine aquaculture – along with other ocean sectors – is becoming a more widespread question (Sanchez-Jerez et al., 2016; Gentry et al., 2017; Lester et al., 2018a; Couture et al., 2021). Offshore or more exposed aquaculture presents an opportunity to expand aquaculture farther into ocean waters, while also potentially reducing impacts, such as water quality issues and disease (Holmer et al., 2008; Price et al., 2015; Froehlich et al., 2017). Here “offshore” is specifically distance based (i.e., far from shore), which differs from “exposed” or “open ocean”, which can experience high energy conditions (e.g., large waves and/or strong currents) but are still close to shore (see Buck et al., 2024 for definition details). Regardless, aquaculture farther from shore has struggled to establish itself in many regions, such as the United States (Lester et al., 2018b; Fujita et al., 2023) – a large seafood consuming, but low aquaculture producing country (FAO, 2022). As a result, some research has aimed to create solutions to help make space for farming in the oceans (e.g., Sanchez-Jerez et al., 2016; Gentry et al., 2017; Lester et al., 2018a) that allows for aquaculture expansion, while minimizing effects on the marine environment and existing ocean stakeholders.
Co-location of ocean activities has the potential to be a tool that increases the value of a region while reducing trade-offs to stakeholders and the environment by using ocean space more efficiently. Historically, investigations into synergies between ocean sectors have taken different forms. Several studies have been conducted that investigated the relationship and potential opportunities for synergy between fisheries and marine energy (de Groot et al., 2014; Stelzenmüller et al., 2016; Kyvelou and Ierapetritis, 2020). Some relatively recent research on co-location of marine energy and aquaculture systems has been explored (O’Donncha et al., 2017; Di Tullio et al., 2018; Weiss et al., 2018), including potential environmental risks (Benjamins et al., 2020; Demmer et al., 2022). While research into the benefits and tradeoffs associated with co-locating ocean activities is increasing, there is a lack of consistency on how best to define “co-location” (Schupp et al., 2019), which creates uncertainty in management processes, as well as a potential lack of comparative environmental metrics. As aquaculture begins to expand farther from shore and potentially alongside other sectors, a deeper accounting of the current pace and status of the science can help inform knowledge gaps and next steps. Importantly, a more holistic evaluation of existing studies may provide a useful lens to assess the coverage of the standing literature.
Integrating aquaculture around other existing and developing ocean activities requires an accounting of multiple human and ecological dimensions. An ecosystem-based approach to aquaculture (EAA) is an existing planning framework designed to position aquaculture more compatibly within its surrounding ecosystem and reduce competition for space in the ocean, calling for the explicit consideration of ecological, social, and governance aspects of development (Soto et al., 2008; Byron and Costa-Pierce, 2013). Notably, EAA aims to balance competing needs and stakeholders, while also protecting the marine environment that supports them. While there are often obstacles to employing EAA (Brugère et al., 2019), understanding the upper and lower limits of aquaculture in a particular site can be useful in developing long-term and sustainable aquaculture systems. To determine limits, carrying capacities can be evaluated through four dimensions of aquaculture development: physical, production, ecological, and social (McKindsey et al., 2006; Ross et al., 2013). Assessing measures of physical carrying capacity (e.g. depth, distance from shore) are used to inform initial siting potential of a given location. This is typically the first step of any development, aquaculture or otherwise (Ross et al., 2013; Froehlich et al., 2017; Lester et al., 2018a; Morris et al., 2021; Garavelli et al., 2022). Similarly, evaluation of production (e.g., yield), social (e.g., public perception) and ecological (e.g., impact) carrying capacity measures provide guidance for aquaculture development under EAA (McKindsey et al., 2006; Ross et al., 2013). Notably, this approach emphasizes reducing aquaculture impact across all aspects, including underscoring the importance of equity. Ultimately, these dimensions offer an informative structure to assess the breadth and depth of co-location science through an aquaculture lens. Recently, Guyot-Téphany et al. (2024) assessed ocean multi-use – which we argue is a broader definition within which co-location resides (see Methods for our definition) – over the past two decades, comparing the studies to those of multiple-use marine protected areas and marine spatial planning (MSP). Although informative, a quantitative synthesis of co-location from an EAA perspective is missing from the literature – particularly the metrics that inform the four carrying capacities.
This study aims to provide a current snapshot of the standing marine co-location literature, especially as it relates to ocean-based aquaculture. The quantitative review is aimed at providing a comprehensive understanding of the research knowledge and gaps – including motivations, methods, and numeric values (Table 1) – as well as potential benefits and limitations to future co-location development. Importantly, we draw on broad EAA themes, exploring regional comparisons and quantifiable measures, including depth and distance to shore and how they compare to the respective standalone industries (i.e., without co-location). We also provide a more in-depth assessment of the specific marine aquaculture co-location metrics and trends. Finally, we identify key research opportunities needed to advance the field.
2 Methods
Literature on co-location was compiled by conducting a search of the following five terms: “offshore co-location”, “marine co-location”, “marine multi-use”, “marine renewable energy”, “offshore co-management”. The initial literature search was conducted in February 2021 and a secondary search was conducted in February 2023, both using the Web of Science (WOS) search engine. We defined co-location in this scenario as “two or more ocean activities occurring in the same place and at the same time” (Schupp et al., 2019), and more specifically those that are largely stationary with built infrastructure, i.e., excludes shipping, wild capture fisheries, etc. However, many papers included in this corpus were published prior to this definition. Therefore, we refer to many publications as being “co-location”, even though they might use a somewhat different terminology (e.g., multi-use, co-siting). Included publications were limited to those published between the years 2000 and 2022 because we observed no publications that fit our definition of co-location were published prior to 2000. The initial search yielded 2,308 publications, which were filtered to only include those containing key factors of co-location, as defined for this study. Any papers that were limited to discussion of only engineering parameters of specific infrastructure were not included. This search only included papers written in English, which will bias our results towards English-speaking regions and audiences. Further, only papers discussing marine environments were considered for this analysis. A vast majority (98%) of the literature returned in the initial search did not meet these criteria.
The filtering process yielded a smaller corpus of 102 papers. Every paper was read in its entirety to understand the full context. Selected publications were sorted into three categories, by their focus: ecology (n = 47), governance (n = 38), and socio-economics (n = 59), based on the category definitions in Table 1. These categories reflect the broader themes of sustainability and the EAA framework previously described (Ross et al., 2013). Given the interdisciplinary nature of the field, it was common for papers to encompass more than one category and thus the same paper could be placed into multiple categories. Therefore, the summation of each category per year will not equal the total publications in that year. We also documented the motivation (i.e., horizon scanning, industry development, and impact/risk) and assessment type (i.e., review, model-based, or pilot/testing) to discern the stage of development globally. Comparisons were made between categories over time to determine trends in publications of co-location research, particularly any gaps. The data were natural-log transformed and a linear model was fit to examine the relationship between the average publication rate of co-location literature over time.
We also sorted papers into which specific activity was evaluated in each paper (aquaculture = 50, tidal energy = 23, wave energy = 58, wind energy = 89, or other = 39) to identify trends in which activities are most commonly co-located. We define “activity” in this study as being any human-led action in marine waters in a co-location context. The “other” activity designation was assigned to publications that discussed co-location beyond the four primary activities of this study, such as solar energy or desalination. Again, this distinction was crosscutting and publications were often assigned to multiple activity categories. Fisheries co-occurring with energy or aquaculture were not considered as “co-location” in this study. While we see fisheries as an important sector to include in assessments of multi-sector ocean planning, this study was centered on understanding co-location as a tool in sustainable ocean development. Therefore, we did not include fisheries activities in this particular study due to (1) it being a well-established, incumbent industry (Pauly, 2008) and, thus, falling outside of our definition of “development” and (2) the transitory nature of wild capture fishing sets it apart from more permanent, stationary fixtures being developed in ocean spaces, such as aquaculture and energy systems, which are the focus of this study.
For publications that were location or regionally specific, the Large Marine Ecosystem (LME) where the study was focused was recorded. The LME scale was selected because it allowed for grouping of trends across different ocean areas. Regional comparisons helped identify where certain locations were more commonly referenced in co-location literature. We also extracted a series of measures from each publication, depending on which category they were assigned (Table 1). Metrics included in each focus category were identified based on their availability in the literature and relevancy to the ocean activities being addressed in this study. We also compared some of the most commonly reported metrics (distance and depth) in the co-location articles to review-based publications that report on the same metrics, but for the standalone sectors (e.g., aquaculture not co-located). This comparison helps capture how co-location fits into single sector development. In particular, how co-location may be constraining or pushing the respective sectors’ “normal” operational limits.
Finally, an aquaculture-specific analysis was conducted to understand how co-location of activities might help or hinder aquaculture expansion, specifically. The publications that fell into this category did so because they explicitly listed aquaculture as being a sector that either is being co-located or could potentially be co-located with another ocean sector. Where possible, the following additional metrics were recorded from publications, due to their relevance to aquaculture infrastructure and species growth: depth (m), distance to shore (km), current velocity (m/s), significant wave height (m), sea surface temperature (C), chlorophyll-a concentration (mg/m3), species/taxa, production (tons/year), and yield (production/area). Metrics were recorded as representative as possible. In many instances, studies reported a general range, in which case the maximum and minimum values were recorded in addition to the average value of that range. In other cases, only a single value was reported.
The metrics chosen for this study were due to their importance in aquaculture suitability based on the four carrying capacities within the EAA framework (McKindsey et al., 2006; Ross et al., 2013; Buck et al., 2024). Depth and distance can be restricted based on cost or technological limitation. The measures are also pertinent to impacts on the benthos, with greater distance and depth typically reducing impacts (Froehlich et al., 2017). Similarly, wave height and current can prove too strong or weak, balancing infrastructure capacity and environmental interactions, respectively. Sea surface temperature, chlorophyll-a, and taxa are particularly critical from a biological perspective and are basic forms of information necessary to determine aquaculture suitability. Lastly, and relatedly, yield provides a unifying measure of productivity over space, time, and taxa (Fong et al., 2024; Kebede et al., 2024). In addition, we did a qualitative review of these papers, highlighting common themes among them, including mentions of disease, contamination, and regulation, informed by the standing aquaculture literature (Galparsoro et al., 2020). We also highlight studies that report on the most extreme cases and critically assess the robustness of that research.
3 Results
3.1 Publication trends
The publication of co-location literature has increased over time, with only a slight emphasis of the articles being socioeconomic focused more recently (Figure 1A). Co-location research historically had a low publication rate from 2000 to 2013 (2 publications per year), after which publication rate steadily increased until 2017 (14 publications per year) (Figure 1A). In fact, this period of growth (2013–2017) tripled the rate of annual publication before plateauing during the years 2018–2022 (10–14 papers per year) (R2adj = 0.84). Socioeconomic papers accounted for 58% of the studies, while governance (37%) and ecology (46%) were slightly less. Of note, almost half of the socioeconomic papers (41%) evaluated social dimensions, such as social perception (e.g., Wever et al., 2015; Jhan et al., 2022), which can be a dominant factor in determining the capacity for development in some regions (e.g., Byron and Costa-Pierce, 2013). But in general, over time all categories reflected relatively similar patterns, which is a somewhat promising trend from an EAA perspective because it suggests that knowledge is being garnered across the suite of aquaculture carrying capacities (Figure 1B).
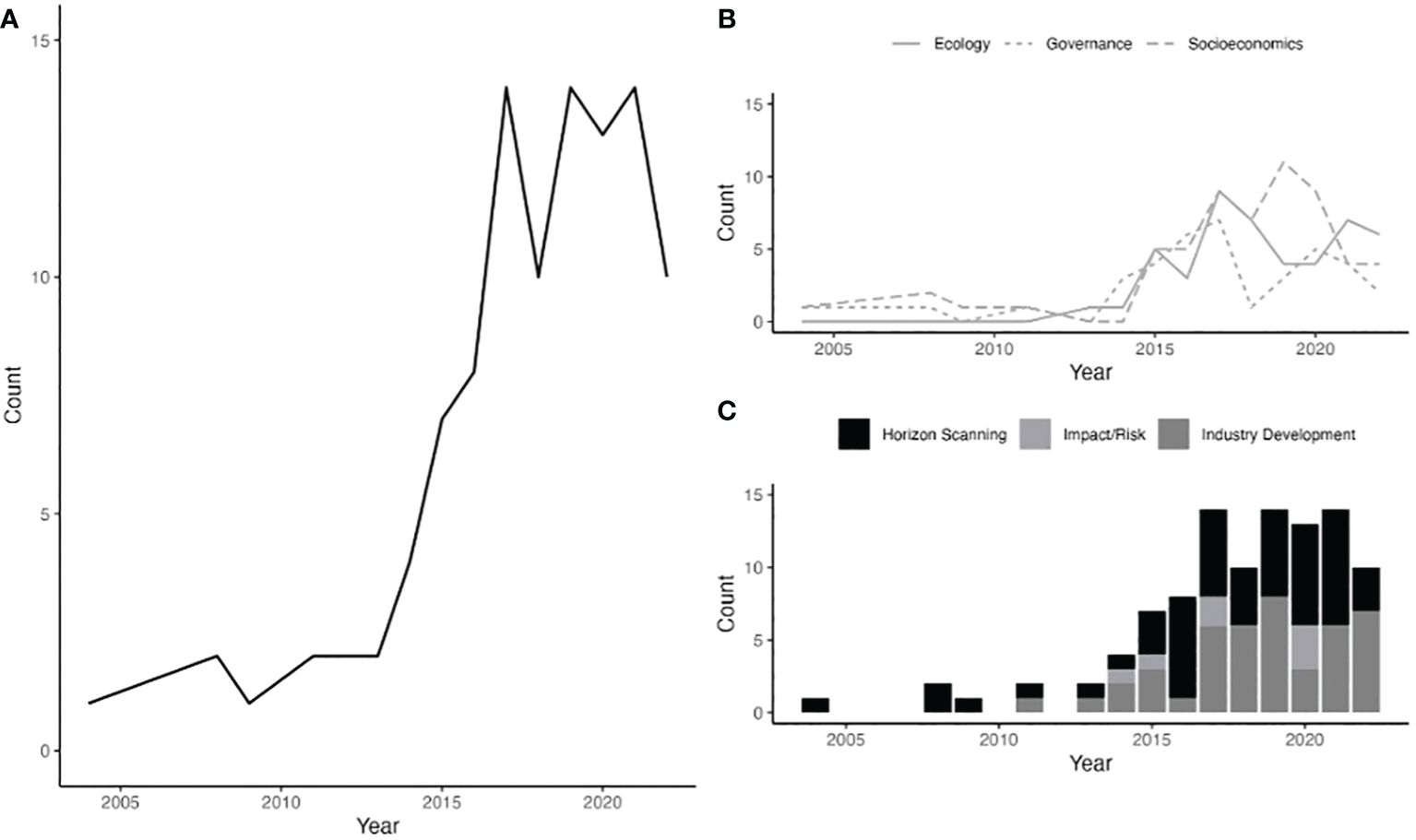
Figure 1. Frequency of publications over time (years 2000–2022). (A) reflects the total frequency of co-location publications published over time (F(1,12) = 69.1, p-value = <0.001, R2adj = 0.84). (B) reflects the frequency of co-location publications, separated by publication focus (i.e., ecology, governance, or socioeconomics), published over time. Publication focuses are cross-cutting, so many publications fall into multiple focus categories. (C) demonstrates the frequency publications, categorized by project motivation (i.e., horizon scanning, impact/risk, or industry development).
Although broader ecosystem themes were relatively equivalent, study motivation differed substantially, with “Horizon Scanning” (50%) and “Industry Development” (43%) dominating the literature, and very little attention given to “Impact/Risk” (7%) (Figure 1C). Of the publications that did contain analyses, most were model-based (n = 58) or reviews (n = 38), but very few were in-water pilot or testing studies (n = 6). Of the impact/risk papers, two suggested a framework or approach for assessing risk in co-located systems (Macadré et al., 2014; Van Hoof et al., 2020), citing risk as a potential obstacle to the expansion of co-located and multi-use systems. Some of the main risks to consider being damage, liability, and lack of safety standards. Others discussed impact and risk to the environment (Onea and Rusu, 2015; Benjamins et al., 2020; Serpetti et al. (2021). Serpetti et al. (2021) in particular presented a model that quantified top-down and bottom-up ecosystem impacts of a hypothetical multi-use platform off the west coast of Scotland. Other themes that emerged included contamination or pollution risks of co-location. Banach et al. (2020) highlighted knowledge gaps surrounding potential human health risks of seaweed cultivation co-located with wind farms, while Elginoz and Bas (2017) conducted a life-cycle assessment of a hypothetical co-located wind and wave system, citing manufacturing as being a large source of pollution. While these studies provide crucial insight into some of the impacts and risks surrounding co-located systems, the scarcity of these analyses suggests that this is a research gap that needs further exploration.
Co-location publications tended to include offshore wind and studies were largely focused on activities in European waters. Wind energy was most often referenced as being co-located (n = 146), followed by wave energy (n = 109), aquaculture (n = 99), and tidal energy (n = 57). Other forms of ocean activities (e.g., marine conservation, tourism) were referenced being co-located 81 times (Figure 2). The spatial focus of co-location publications was widely distributed, appearing near almost every continental coast but South America (Figure 3). However, the articles were largely focused on European waters, with the North Sea having the highest number of publication references (30%). In fact, the very first publication captured in our literature search was based in the North Sea, centered upon the legal constraints and opportunities of co-locating wind farms and aquaculture (Buck et al., 2004).
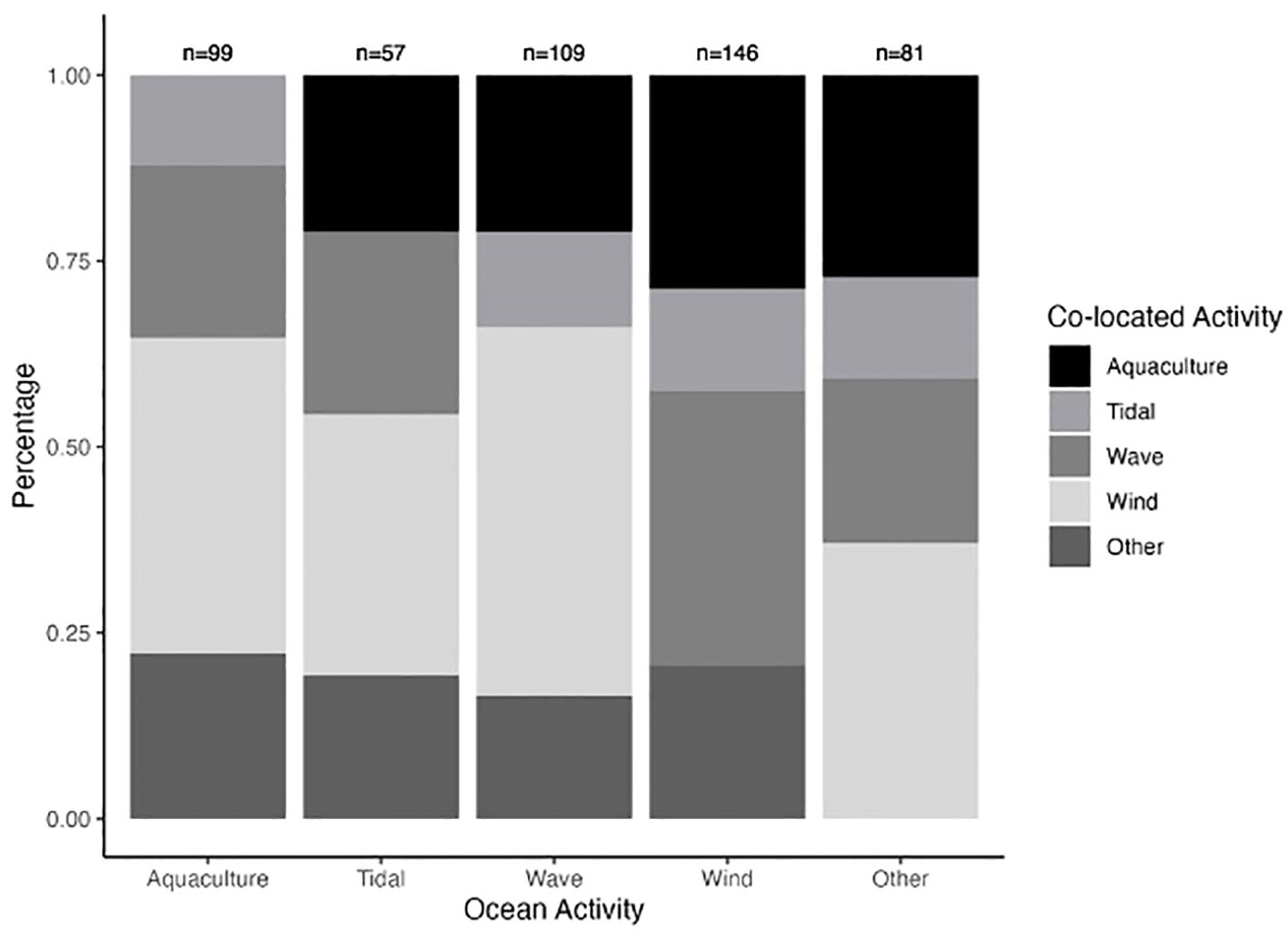
Figure 2. Each ocean activity and its frequency of co-location with another ocean activity. Many publications referenced multiple interacting co-locating activities.
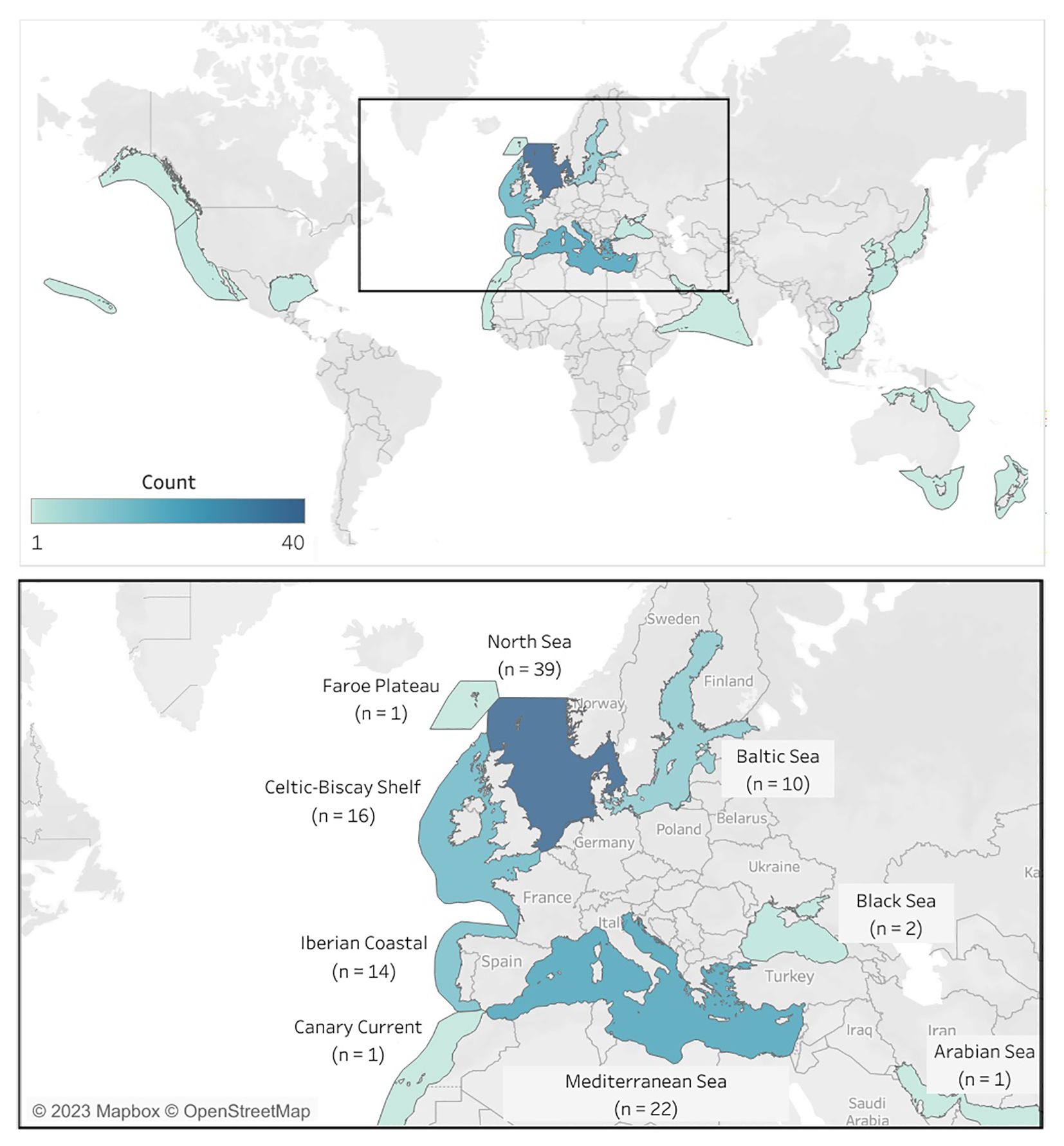
Figure 3. Regional distribution of co-location publications across Large Marine Ecosystems (LMEs) between the years 2000 and 2022. Multinational distribution (top panel) reflects publications that reference location, with a closer look at European waters (bottom panel). The North Sea (n = 39) was the most common LME referenced in co-location literature.
Recognizing peer-reviewed scientific literature may not reflect all actions occurring in a given region (North Sea expert communications, personal communication, 2024), we conducted a post hoc evaluation of available gray literature from co-location projects in the North Sea due to its dominance in the primary literature (N = 57). The Multi-uses in European Seas (MUSES) Project produced 29 reports, newsletters, and infographics that examined multi-use across seven case study locations (MUSES, 2024). MUSES was a two-year (2016 – 2018) project undertaken to explore the potential of multi-use in European waters. While we excluded the co-occurrence of fisheries with energy or aquaculture as a form of “co-location” in our synthesis of scientific WOS publications, the MUSES project included this scenario as a form of co-location. Therefore, we included those publications in our post hoc evaluation of the MUSES gray literature to accurately capture the MUSES findings. Notably, each MUSES case study incorporated a Drivers, Added value, Barriers, and Impacts (DABI) catalog and scoring assessment to categorize key opportunities and barriers across different scenarios (Bocci et al., 2017). More recently, the “multi-Use offshore platforms demoNstrators for boostIng cost-effecTive and Eco-friendly proDuction in sustainable marine activities” (UNITED) project operated from 2020 until 2023 and made a series of briefs available (n = 7), in addition to a series (n = 17) of pilot development deliverables (UNITED, 2024). Also, while some literature concerning the “Innovative multi-purpose offshore platforms: planning, design & operation” (MERMAID) project was captured in our synthesis (Simal et al., 2017; Xepapadeas et al., 2017; Bocci et al., 2019), the final report of the MERMAID project was reviewed in our post hoc evaluation (MERMAID, 2024). Lastly, as a follow-up to UNITED, a project integrating Low-Trophic Aquaculture within Offshore Wind Farms (ULTFARMS Project) launched in 2023 and will run until 2026. Thus far, there are some (n = 3) reports available that describe the monitoring efforts, communication of results, and stakeholder engagement deliverables (ULTFARMS, 2024). We conducted a search of materials for the OLAMUR and “North Sea Farm#1” projects, but given the early stages of these projects, no gray literature was publicly available (NSF, 2024; OLAMUR, 2024). All of the reports reviewed in our post-hoc evaluation made investigations into impact/risk (environmental, social, and/or technical). Given this additional evaluation, it is clear that many of the North Sea projects have produced gray literature that was not captured in scientific literature but is valuable in understanding the state of co-location research and highlights a disconnect in the field. Ultimately, these projects outline a site-specific methodological framework for evaluating trade-offs of co-location projects.
3.2 Metric trends
Of the publications that provided metrics (36%), there was no significant difference (Kruskal-Wallis, p = 0.387) in depth ranges across aquaculture (median ± interquartile range = 66.25 m ± 95.88), wave energy (104.00 m ± 95), and offshore wind studies (100.00 m ± 120) (Figure 4A). Notably, some of the depth metrics provided in some publications far exceeded normal depth ranges (>100 meters) for both reported aquaculture (Froehlich et al., 2017) and wind energy projects (DOE, 2022). Though, later studies have used deeper limits for suitability mapping based on qualitative input from industry, such as 150 m (Morris et al., 2021) or 200 m (Gentry et al., 2017), which is more consistent with the co-location trends. Perhaps these outliers call into question some of the feasibility of co-location and/or suggest that it will push sectors at or past their current limits. While sample sizes were too small and uneven to conduct formal statistics, observationally the depth ranges of just the aquaculture projects seem similar across the different energy-ocean activities (Figure 4B).
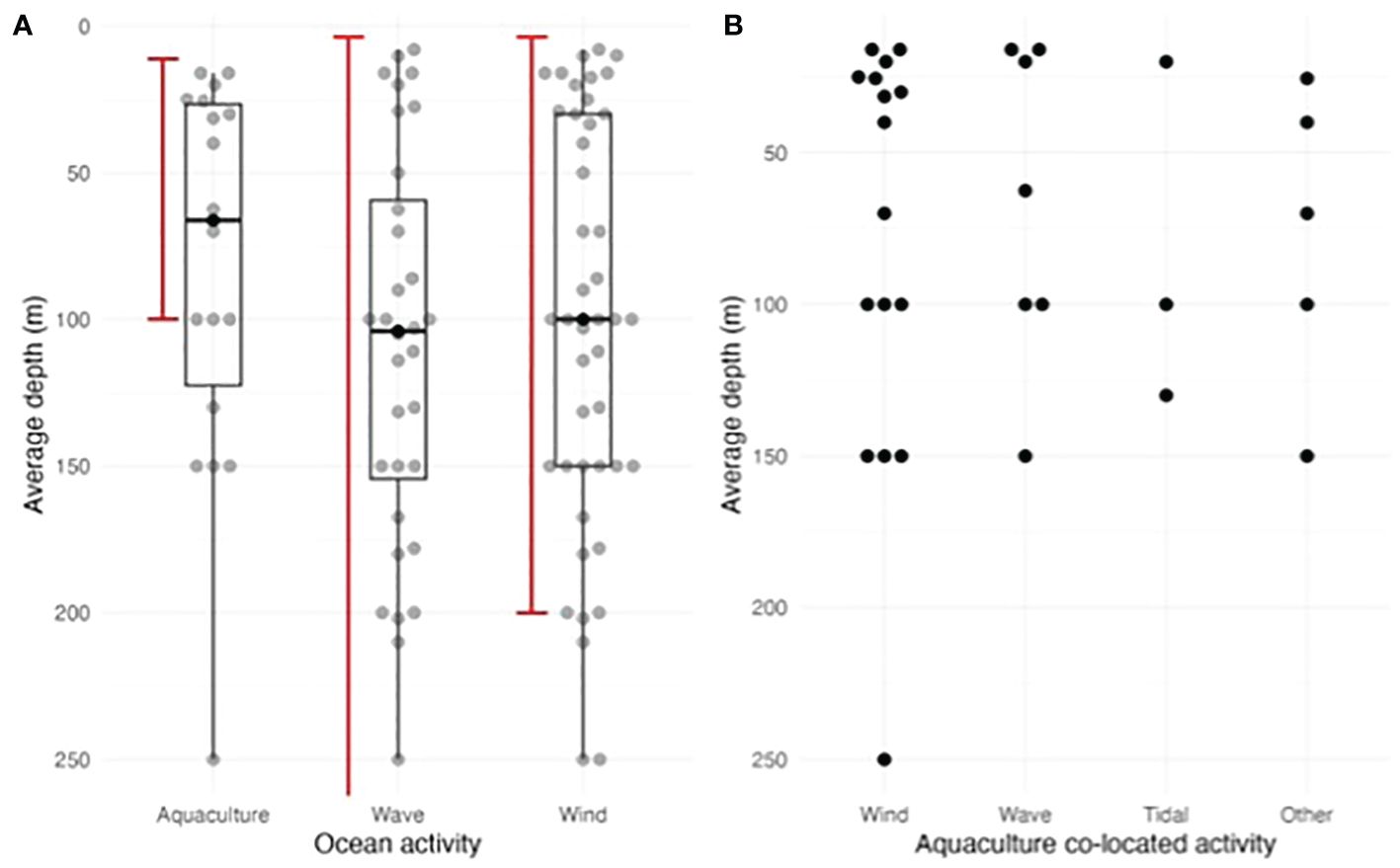
Figure 4. (A) Average depth (meters) across the three most common ocean activities in co-location literature: aquaculture (n = 18), wave energy (n = 32), and wind energy (n = 44) sectors. These ocean activities all reflect similar average reported depths (Kruskal-Wallis H = 1.9, df = 2, p = 0.39). Red lines represent current in situ depth ranges for respective technologies (Froehlich et al., 2017; DOE, 2022; Tethys, 2023). (B) In-depth examination of depth metrics when aquaculture is co-located with wind energy (n = 16, min = 0, median = 55, max = 500), wave energy (n = 7, min = 0, median = 62.5, max = 200), tidal energy (n = 3, min = 0, median = 100, max = 200), and other (n = 5, min = 0, median = 70, max = 300) sectors.
Similar to co-location depth trends, there was no significant difference (Kruskal-Wallis, p = 0.59) in the distance from shore of aquaculture (16 km ± 21), wave energy (20 km ± 35), and offshore wind (17 km ± 33.5) publications in this corpus (Figure 5A). Observing the aquaculture distance metrics, the distances from shore are very similar (1.2–60 km), regardless of which ocean activity aquaculture is being co-located with (Figure 5B). While the distances from shore of co-located aquaculture studies fit within the ranges available in aquaculture literature (Froehlich et al., 2017), there is one outlier in the wind category that greatly exceeded the range of distances from shore currently represented in offshore wind projects (< 120 km; DOE, 2022) (Figure 5A). Also, many of the distances from shore for co-located wave energy studies fell outside the current range from wave energy projects (Tethys, 2023). As with the depth range outliers, the general disparity between distances from shore of current wave energy projects and those of studies on co-located wave energy studies could imply that these data lack feasibility. However, it may suggest that developing co-located systems could enable smaller ocean projects to operate farther from shore.
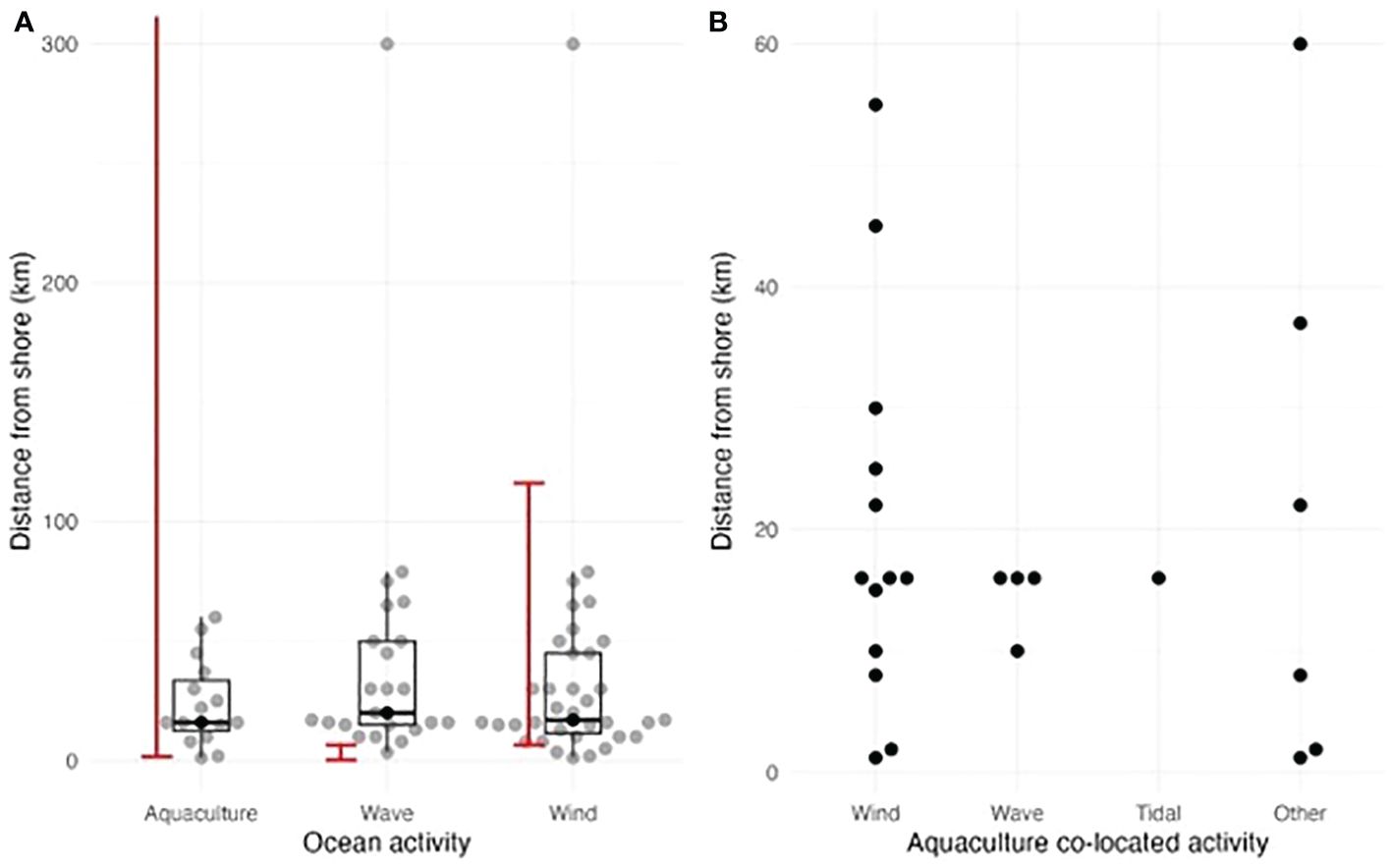
Figure 5. (A) Distance from shore (kilometers) across aquaculture (n = 15), wave energy (n = 23), and wind energy (n = 35). These sectors all reflect similar distances from shore (Kruskal-Wallis H = 1.1, df = 2, p = 0.59). Red lines represent current in situ depth ranges for respective technologies (Froehlich et al., 2017; DOE, 2022; Tethys, 2023). (B) Evaluation of these metrics when aquaculture is co-located with wind energy (n = 13, min = 1.2, median = 16, max = 55), wave energy (n = 4, min = 10, median = 16, max = 16), tidal energy (n = 1, min = 16, median = 16, max = 16), and other (n = 6, min = 1.2, median = 15, max = 60) sectors.
3.3 Aquaculture implications
Out of the original 102 scientific publications in the corpus, 50 (49%) were identified as being aquaculture specific and discussed a variety of different aquaculture types. Topics spanned technical evaluations, physical and biological siting studies, and social acceptance research. Co-located aquaculture publications were most often focused on mollusk culturing (n = 10), followed by fish (n = 8), macroalgae (n = 7), and crustacea (n = 1) (Table 2). The trend towards studies that incorporate unfed aquaculture is likely due to the lower cost and effort required to cultivate those species (Fujita et al., 2023). However, as fed marine species generally have higher market value, offsetting costs of those systems by co-locating with another ocean activity could be useful to the sector, particularly when it comes to aquaculture in waters that are exposed or farther from shore. Of the 61 gray documents, many had logistical or technical focuses that didn’t necessarily evaluate co-location potential at the case study or pilot project level (n = 43). Of the remaining documents that did directly address co-location case studies or pilot projects (n = 18), roughly half included aquaculture (55%), including finfish in the beginning (2012–2018; MERMAID, 2024; MUSES, 2024) and later focusing primarily on low trophic aquaculture (i.e., bivalves and seaweeds) (2020–2024; UNITED, 2024; ULTFARMS, 2024).
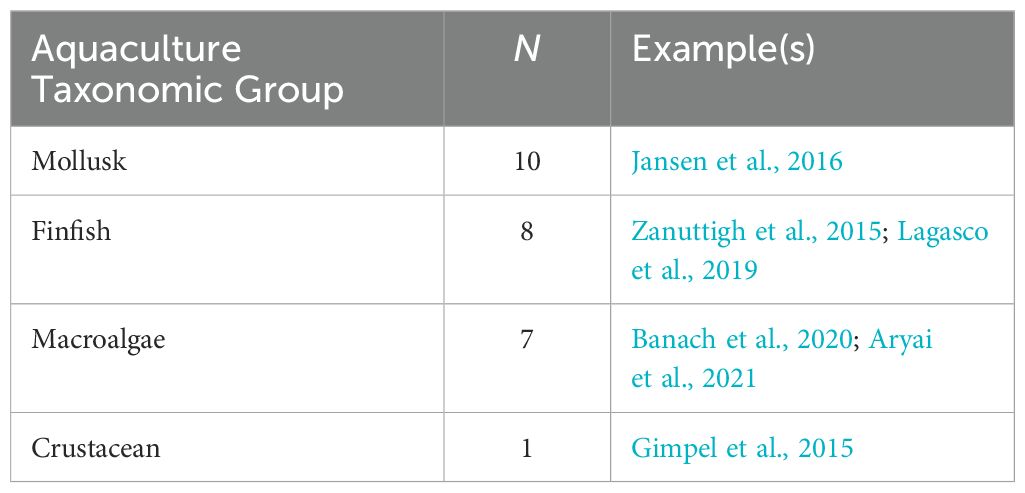
Table 2. Taxonomic groups of aquaculture species listed in any of the 50 aquaculture co-location scientific (WOS) publications.
Few of the co-located aquaculture science publications were focused on impacts or risk (8%) and only one was in the pilot study and testing stage (2%). Similarly to the broader corpus trends, the motivation for aquaculture co-location publication was more frequently horizon scanning (n = 29; e.g., Lacroix and Pioch, 2011; Green et al., 2019) or industry development (n = 17; e.g., Gimpel et al., 2015; Steins et al., 2021), rather than impact and risk (e.g., Banach et al., 2020; Benjamins et al., 2020). Also, the assessment type for co-located aquaculture publications was largely a model (n = 20) or review (n = 29). The only pilot/testing assessment was provided by Flikkema and Waals (2019) and consisted of technical testing of a floating multiple-use platform, both conceptually and in lab conditions. The relative scarcity of papers addressing the pilot stage and impact studies aligns well with the nascency of the aquaculture co-location field.
Similar to the broader WOS corpus trends, only 25 (50%) of aquaculture related scientific publications provided specific metrics. The metrics included depth (n = 18), distance to shore (n = 15), current velocity (n = 8), wave height (n = 8), sea surface temperature (SST; n = 6), and chlorophyll-a (chl-a; n = 3) (Table 3). An additional three publications referenced metrics but did not provide them and were thus not included in the final tally. On the whole, the aquaculture scientific publications that listed a yield estimate were scarce (n = 1). Aryai et al. (2021) reported an estimate of yield (80 tons/year/ha) for seaweed, but this was an estimate based on the industry standard from this system, rather than a site-specific dynamic model or in situ measurement. Other publications (n = 7) listed estimated production values, which could be calculated with farm size to determine yield (e.g., Söderqvist et al., 2017; Lagasco et al., 2019; Benjamins et al., 2020). For example, Benjamins et al. (2020) modeled a multi-purpose platform which includes wind turbines that would supply energy to a 2,500 metric ton salmon farming system off of western Scotland. Again, this study reports the general anticipated production, but did not actually model the aquaculture yield itself. Yield in particular provides a critical and comparative measure; without reference, the ability to truly assess the potential of co-location of the overlapping industries is limited.
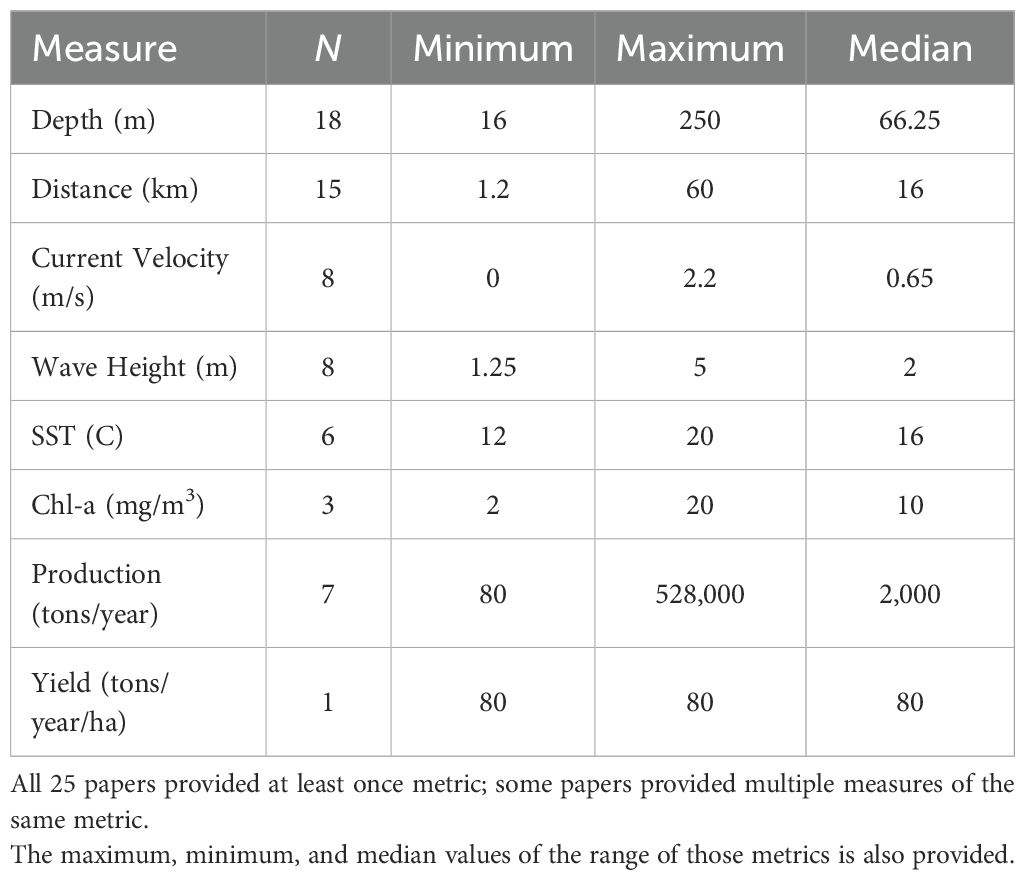
Table 3. List of metrics available in co-located aquaculture scientific (WOS) publications and the number of publications (n) that provide those metrics.
4 Discussion
Co-location research is increasing over time, with the most consideration being given to offshore wind and half of all publications including reference to aquaculture. The focus of publications in these studies were fairly evenly split between ecology, governance, and socioeconomics, which is in line with an EAA management framework that centers around interdisciplinary and holistic assessments (Soto et al., 2008; Lester et al., 2013; Ross et al., 2013). However, there was a general lack of studies assessing impact/risk, when compared to horizon scanning or industry development, though the post-hoc gray literature review of the most data rich and advanced North Sea region showed significant investment and coverage of impact/risk evaluations. That said, metrics in the scientific literature were rarely reported, particularly for aquaculture. Of the data that were provided, ranges fell beyond the currently reported individual, standalone industries (Froehlich et al., 2017; DOE, 2022; Tethys, 2023). These outliers could raise doubts about the feasibility of these proposed systems, or they may indicate that co-location is beginning to push industries beyond their current limits and into new extreme conditions. This “push” underscores the need for more risk and impact assessments given the challenges associated with moving ocean activities farther and deeper in the ocean, particularly when it comes to aquaculture.
While aquaculture accounted for half of co-location publications, focusing heavily on unfed species (mollusks and seaweeds), it lacked important comparative measures (e.g. yield) that allow researchers to truly understand co-location potential. The trend towards unfed species may be due to the typically lower cost but relatively high value for mollusks (Fujita et al., 2023), environmental sustainability of both mollusks and seaweeds (Gephart et al., 2021), and/or potential higher social license to operate in some regions (Fong et al., 2022). However, by this same reasoning, co-location in federal waters as a tool in culturing fed species of aquaculture, specifically finfish, could present an opportunity to reduce conflict around this somewhat more contentious taxonomic group (e.g., Carballeira Braña et al., 2021; Fujita et al., 2023). Notably, standalone offshore aquaculture research has largely focused on finfish production (Froehlich et al., 2017), an interesting divergence of trends. In fact, the gray reports in the North Sea focused on salmon aquaculture in the beginning – likely due to the proximity to Norway, the largest salmon producing nation in the world, and involvement of Scotland, a smaller but notable salmon producing country (FAO, 2022) – but later geared attention towards extractive species. Regardless, one problem with less offshore metric reporting of unfed species in the literature is it is not being compensated for in co-location publications. In addition, in the scientific literature the size and value associated with co-located marine aquaculture systems was unclear due to the general lack of data, including yield. While yield data is frequently underreported and highly variable in the aquaculture literature in general, it is a crucial metric in understanding the comparative scale and efficiency of production of aquaculture systems across taxa, geographies, and over time (Fong et al., 2024; Kebede et al., 2024). Similarly, the aquaculture co-located studies contained few impact/risk evaluations, which could affect the growth, survival, and safety of the farm, directly and indirectly.
Studies that evaluate the impact and/or risk of co-located aquaculture projects will be especially necessary, though currently underrepresented in the peer-reviewed literature but more common in the North Sea gray literature. As co-location trends could be showing the potential to “push” industries beyond their normal physical range, co-located aquaculture projects may trend towards waters that are exposed or farther from shore. It is possible that co-locating aquaculture with other ocean activities could enable “exposed” and “open ocean” aquaculture by lowering costs and reducing risks within these operations (Buck et al., 2008; Stuiver et al., 2016; Fujita et al., 2023; Maar et al., 2023). However, it also could present new obstacles to aquaculture. For instance, co-located systems often have more structures in the water, which can affect the biophysical processes in that region (O’Donncha et al., 2017; Raghukumar et al., 2023). Also, there is some concern regarding the contamination risk of co-locating aquaculture with certain types of energy (Banach et al., 2020), as well as risk to physical operational safety to workers (Van Hoof et al., 2020). Most notably, there are a number of studies that highlight the need for a clear legal framework for co-located aquaculture (Michler-Cieluch and Krause, 2008; Stuiver et al., 2016; Calado et al., 2019). This is important because assigning liability across multiple user groups can be challenging and become an obstacle for co-located systems (Steins et al., 2021), particularly as sectors might be moving towards more exposed waters, where the environment is harsher and some risk factors increase (Fujita et al., 2023). Many of the publications that call for legal clarity of co-located systems originate from the North Sea (Stuiver et al., 2016; Depellegrin et al., 2019), in fact the gray literature categorized these systems as having the “unsolvable problem of liability” (Bocci et al., 2017). However, the MUSES case studies provide some legal clarity with the ultimate goal of implementation. The projects in this region offer a framework that includes liability and impact considerations, such as fishermen compensation, noise impacts, and social acceptance (MUSES, 2024).
Currently, co-location is being studied as a tool to strengthen the energy and food nexus around the world, but there is particular focus in European waters, largely in the North Sea. Due to this focus, we conducted a post-hoc evaluation of co-location in the North Sea region. The North Sea represents a unique set of attributes that make it favorable to co-location. It sits between many European countries (e.g., Denmark, Norway, Germany, the United Kingdom), which results in high multinational competition for space and resources though sectors like fishing, oil and gas, marine protection, and maritime transportation (Stuiver et al., 2016; Bocci et al., 2019). In the last decade, standalone industries entered the seascape, including offshore wind, providing additional demand for North Sea space (Buck et al., 2008; Michler-Cieluch et al., 2009; Benassai et al., 2014; Gimpel et al., 2015). Multiple sectors stemming from multiple countries requiring resources in the North Sea make it a fitting target for co-location. A series of co-location projects have laid a foundation of gray literature with regionally specific contexts and findings to the North Sea (MUSES, 2024; MERMAID, 2024; UNITED, 2024; ULTFARMS, 2024). Unlike the corpus of scientific publications, these gray reports have been largely supported with government funding and are implementation focused. Stemming from the gray literature, the first commercial scale, “in-the-water” co-location activities are taking place in the North Sea (NSF, 2024; OLAMUR, 2024). The North Sea Farmers are currently building the first commercial scale seaweed farm in between existing wind turbines in the Dutch North Sea (NSF, 2024). Similarly, OLAMUR is an EU project that supports commercially viable and sustainable low-trophic aquaculture, with one case study being placed within industrial-scale offshore wind farms at a North Sea pilot site (OLAMUR, 2024). As a result, the North Sea may be a “North Star” for the many other regions considering co-location of aquaculture and wind, moving beyond horizon scanning and modeling to actual application.
Our assessment revealed that only a fraction of co-location WOS papers (36%) included site-specific data. Ultimately, the shortage of quantifiable data within co-location studies hindered our ability to make statistical comparisons. A larger standardized dataset would be ideal and will hopefully be a goal for the future of co-location, especially for the purpose of knowledge sharing. However, in the absence of comprehensive empirical observations, scenario-based modeling could help provide insights into co-location opportunities and impacts at a particular location (Couture et al., 2021). Through modeling, decision makers can embrace uncertainty to help determine more explicit levels of risk and informed needs for co-location development and management, something more common in other maritime resource-use sectors (e.g., Privitera-Johnson and Punt, 2020). Several model-based studies included O’Donncha et al. (2017) on the East Coast of the United States, which assessed the effects of shellfish aquaculture on the water flow to a tidal stream-energy generator, and Clark et al. (2019) in the North Sea, which evaluated the lifecycle cost of wind and wave co-located systems. These studies and others like them could help inform future modeling efforts. For instance, though not captured in our synthesis of scientific publications due to its recent publication, Maar et al. (2023) presents a valuable spatial modeling study of co-located low-trophic aquaculture and offshore wind farm systems, including estimates of aquaculture biomass yield. In addition, gray literature clearly provides more potential details, although may not be peer-reviewed, archival, and/or as focused on reproducibility. While we included the extensive efforts of the North Sea given its significance in the multi-use arena, further aggregation and assessment of other regions could be done to bolster missingness in scientific publication – recognizing the potential difference in information collection and dissemination, and likely challenges to track down the many forms that may exist (Paez, 2017). As previously noted, this study was restricted to publications written in English on the WOS platform, likely excluding important published work, particularly in China where the respective sectors of aquaculture and wind development are expanding quickly (GWEC, 2023; Long et al., 2024). In fact, only 6% of papers in this study focused on Chinese waters. A logical next step of this paper would be to focus synthesis efforts on that particular region, which could provide a nice comparative to the efforts in the North Sea. Despite these caveats, this analysis does capture some important trends in co-location research and examines whether it might affect future ocean development, especially in terms of ocean-based aquaculture.
Our study sets the stage for future investigations into co-location of marine sectors, in particular aquaculture and clean energy in regions that have yet to adopt such spatial approaches. By developing a multinational understanding of co-location trends, researchers and decision makers can leverage the numerous existing models and approaches around co-location. However, there will be a lot of context dependencies to consider. To begin to understand more realized potential of co-location in a specific region, we encourage future research to include more detailed, standardized reporting and modeling of co-location measures – as exemplified in the North Sea gray literature. Incorporating uncertainty and other factors that may pose challenges (or opportunities) would provide useful context to model outputs and inform conclusions about the co-location potential of a site. Indeed, there is a deficit of measures in scientific publications to inform implementation, especially in relation to aquaculture production and associated impacts or risks therein. Seemingly, most of the modeling and data efforts have focused on the energy side of the co-location equation, while the arguably more challenging production of culturing living organisms via aquaculture remains under-assessed. While standalone reporting in the respective fields – like those featured within the reviews highlighted in this study (Froehlich et al., 2017; DOE, 2022; Tethys, 2023) – can provide some valuable insights for co-location, the interactions between the co-sited structures likely create unique challenges. Ultimately, greater attention to what type of aquaculture can grow and how it interacts with the energy it is sited with needs to be investigated more rigorously to understand the true potential of the integrative food-energy ocean future.
Data availability statement
The raw data supporting the conclusions of this article will be made available by the authors, without undue reservation.
Author contributions
CG: Conceptualization, Data curation, Formal analysis, Funding acquisition, Investigation, Visualization, Writing – original draft, Writing – review & editing. SC: Data curation, Investigation, Writing – review & editing. HF: Conceptualization, Funding acquisition, Supervision, Visualization, Writing – review & editing.
Funding
The author(s) declare financial support was received for the research, authorship, and/or publication of this article. This research was conducted with funding from the Schmidt Family Foundation and the Coastal Fund at the University of California, Santa Barbara.
Acknowledgments
Special thanks to Tyler Sclodnick and Dr. Bela Buck for your constructive and critical feedback that improved this manuscript.
Conflict of interest
The authors declare that the research was conducted in the absence of any commercial or financial relationships that could be construed as a potential conflict of interest.
Publisher’s note
All claims expressed in this article are solely those of the authors and do not necessarily represent those of their affiliated organizations, or those of the publisher, the editors and the reviewers. Any product that may be evaluated in this article, or claim that may be made by its manufacturer, is not guaranteed or endorsed by the publisher.
References
Aryai V., Abbassi R., Abdussamie N., Salehi F., Garaniya V., Asadnia M., et al. (2021). Reliability of multi-purpose offshore-facilities: Present status and future direction in Australia. Process Saf. Environ. Prot. 148, 437–461.
Banach J. L., van den Burg S. W. K., van der Fels-Klerx H. J. (2020). Food safety during seaweed cultivation at offshore wind farms: An exploratory study in the North Sea. Mar. Policy 120, 104082.
Benassai G., Mariani P., Stenberg C., Christoffersen M. (2014). A Sustainability Index of potential co-location of offshore wind farms and open water aquaculture. Ocean Coast. Manage. 95, 213–218.
Benjamins S., Masden E., Collu M. (2020). Integrating wind turbines and fish farms: an evaluation of potential risks to marine and coastal bird species. J. Mar. Sci. Eng. 8, p.414.
Bocci M., Ramieri E., Castellani C., De Pellegrin D., Buchanan B., Buck B. H., et al. (2017). Case study methodology, MUSES project (Edinburgh, UK: Edinburgh). Available at: https://sites.dundee.ac.uk/muses/wp-content/uploads/sites/70/2017/07/D3.1-WP3-Case-study-methodology-web.pdf.
Bocci M., Sangiuliano S. J., Sarretta A., Ansong J. O., Buchanan B., Kafas A., et al. (2019). Multi-use of the sea: A wide array of opportunities from site-specific cases across Europe. PloS One 14, e0215010.
Brugère C., Aguilar-Manjarrez J., Beveridge M. C., Soto D. (2019). The ecosystem approach to aquaculture 10 years on–a critical review and consideration of its future role in blue growth. Rev. Aquaculture 11, 493–514.
Buck B. H., Bjelland H. V., Bockus A., Chambers M., Costa-Pierce B. A., Dewhurst T., et al. (2024). Resolving the term “offshore aquaculture” by decoupling “exposed” and “distance from the coast. Front. Aquaculture 3, 1428056.
Buck B. H., Krause G., Michler-Cieluch T., Brenner M., Buchholz C. M., Busch J. A., et al. (2008). Meeting the quest for spatial efficiency: progress and prospects of extensive aquaculture within offshore wind farms. Helgoland Mar. Res. 62, 269–281.
Buck B. H., Krause G., Rosenthal H. (2004). Extensive open ocean aquaculture development within wind farms in Germany: the prospect of offshore co-management and legal constraints. Ocean Coast. Manage. 47, 95–122.
Buhagiar D., Sant T., Farrugia R. N., Aquilina L., Farrugia D., Strati F. M. (2019). Small-scale experimental testing of a novel marine floating platform with integrated hydro-pneumatic energy storage. J. Energy storage 24, 100774.
Bull A. S., Love M. S. (2019). Worldwide oil and gas platform decommissioning: a review of practices and reefing options. Ocean Coast. Manage. 168, 274–306.
Byron C. J., Costa-Pierce B. A. (2013). Carrying capacity tools for use in the implementation of an ecosystems approach to aquaculture. Site selection carrying capacities inland Coast. aquaculture 21, 87–101.
Calado H., Papaioannou E. A., Caña-Varona M., Onyango V., Zaucha J., Przedrzymirska J., et al. (2019). Multi-uses in the Eastern Atlantic: Building bridges in maritime space. Ocean Coast. Manage. 174, 131–143.
Carballeira Braña C. B., Cerbule K., Senff P., Stolz I. K. (2021). Towards environmental sustainability in marine finfish aquaculture. Front. Mar. Sci. 8, 666662.
Clark C. E., Miller A., DuPont B. (2019). An analytical cost model for co-located floating wind-wave energy arrays. Renewable Energy 132, 885–897.
Costello C., Cao L., Gelcich S., Cisneros-Mata M.Á., Free C. M., Froehlich H. E., et al. (2020). The future of food from the sea. Nature 588, 95–100.
Couture J. L., Froehlich H. E., Buck B. H., Jeffery K. R., Krause G., Morris J. A. Jr., et al. (2021). Scenario analysis can guide aquaculture planning to meet sustainable future production goals. ICES J. Mar. Sci. 78, 821–831.
de Groot J., Campbell M., Ashley M., Rodwell L. (2014). Investigating the co-existence of fisheries and offshore renewable energy in the UK: Identification of a mitigation agenda for fishing effort displacement. Ocean Coast. Manage. 102, pp.7–pp18.
Demmer J., Lewis M., Neill S. (2022). “Multi-use platforms at sea: A sustainable solution for aquaculture and biodiversity”. Trends in Renewable Energies Offshore. (Lisbon, Portugal: CRC Press), 797–805.
Department of Energy (DOE) (2022). Offshore Wind Market Report: 2022 Edition (Wind Energy Technologies Office). Available online at: https://www.energy.gov/sites/default/files/2022-09/offshore-wind-market-report-2022-v2.pdf (Accessed October, 28 2023).
Depellegrin D., Venier C., Kyriazi Z., Vassilopoulou V., Castellani C., Ramieri E., et al. (2019). Exploring Multi-Use potentials in the Euro-Mediterranean sea space. Sci. Total Environ. 653, 612–629.
Di Tullio G. R., Mariani P., Benassai G., Di Luccio D., Grieco L. (2018). Sustainable use of marine resources through offshore wind and mussel farm co-location. Ecol. Model. 367, pp.34–pp.41.
Elginoz N., Bas B. (2017). Life Cycle Assessment of a multi-use offshore platform: Combining wind and wave energy production. Ocean Eng. 145, pp.430–pp.443.
FAO (2022). “The State of World Fisheries and Aquaculture,” in Towards Blue Transformation (FAO, Rome). doi: 10.4060/cc0461en
Fong C. R., Gonzales C. M., Rennick M., Gardner L. D., Halpern B. S., Froehlich H. E. (2024). Global yield from aquaculture systems. Rev. Aquaculture. 16(3), pp.1021–1029
Fong C. R., Gonzales C. M., Rennick M., Lahr H. J., Gardner L. D., Halpern B. S., et al. (2022). California aquaculture in the changing food seascape. Aquaculture 553, 738009.
Froehlich H. E., Smith A., Gentry R. R., Halpern B. S. (2017). Offshore aquaculture: I know it when I see it. Front. Mar. Sci. 4, 154.
Fujita R., Brittingham P., Cao L., Froehlich H., Thompson M., Voorhees T. (2023). Toward an environmentally responsible offshore aquaculture industry in the United States: ecological risks, remedies, and knowledge gaps. Mar. Policy 147, 105351.
Galparsoro I., Murillas A., Pinarbasi K., Sequeira A. M., Stelzenmüller V., Borja A., et al. (2020). Global stakeholder vision for ecosystem-based marine aquaculture expansion from coastal to offshore areas. Rev. Aquaculture 12, 2061–2079.
Garavelli L., Freeman M. C., Tugade L. G., Greene D., McNally J. (2022). A feasibility assessment for co-locating and powering offshore aquaculture with wave energy in the United States. Ocean Coast. Manage. 225, 106242.
Gentry R. R., Lester S. E., Kappel C. V., White C., Bell T. W., Stevens J., et al. (2017). Offshore aquaculture: spatial planning principles for sustainable development. Ecol. Evol. 7, 733–743.
Gephart J. A., Henriksson P. J., Parker R. W., Shepon A., Gorospe K. D., Bergman K., et al. (2021). Environmental performance of blue foods. Nature 597, 360–365.
Gimpel A., Stelzenmüller V., Grote B., Buck B. H., Floeter J., Núñez-Riboni I., et al. (2015). A GIS modelling framework to evaluate marine spatial planning scenarios: Co-location of offshore wind farms and aquaculture in the German EEZ. Mar. Policy 55, pp.102–pp.115.
Global Wind Energy Council (GWEC) (2023). Global Wind Report 2023. Available online at: https://gwec.net/wp-content/uploads/2023/04/GWEC-2023_interactive.pdf.
Green R., Copping A., Cavagnaro R. J., Rose D., Overhus D., Jenne D. (2019). Enabling power at sea: Opportunities for expanded ocean observations through marine renewable energy integration (IEEE), OCEANS 2019 MTS/IEEE SEATTLE, Seattle, WA, USA. 1–7. doi: 10.23919/OCEANS40490.2019.8962706
Guyot-Téphany J., Trouillet B., Diederichsen S., Juell-Skielse E., Thomas J. B. E., McCann J., et al. (2024). Two decades of research on ocean multi-use: achievements, challenges and the need for transdisciplinarity. NPJ Ocean Sustainability 3, 8.
Halpern B. S., Walbridge S., Selkoe K. A., Kappel C. V., Micheli F., d’Agrosa C., et al. (2008). A global map of human impact on marine ecosystems. Science 319, 948–952.
Holmer M., Hansen P. K., Karakassis I., Borg J. A., Schembri P. J. (2008). “Monitoring of environmental impacts of marine aquaculture,” in Aquaculture in the Ecosystem (Springer, Dordrecht), 47–85.
Jansen H. M., Van Den Burg S., Bolman B., Jak R. G., Kamermans P., Poelman M., et al. (2016). The feasibility of offshore aquaculture and its potential for multi-use in the North Sea. Aquaculture Int. 24, 735–756.
Jhan H. T., Lee H. T., Ting K. H. (2022). The potential compatibility of designating offshore wind farms within wider marine protected areas—conservation of the Chinese white dolphin regarding fishers’ perception. Fishes 7, p.195.
Jouffray J.-B., Blasiak R., Norström A. V., Österblom H., Nyström M. (2020). The blue acceleration: the trajectory of human expansion into the ocean. One Earth 2 1, 43–54.
Kebede E. A., Ali H. A., Clavelle T., Halley E. F., Gephart J. A., Hartman S., et al (2024). Assessing and addressing the global state of food production data scarcity. Nat. Rev. Earth Environ 5, 295–311.
Konispoliatis D. N., Katsaounis G. M., Manolas D. I., Soukissian T. H., Polyzos S., Mazarakos T. P., et al. (2021). REFOS: a renewable energy multi-purpose floating offshore system. Energies 14, 3126.
Kyvelou S. S. I., Ierapetritis D. G. (2020). Fisheries sustainability through soft multi-use maritime spatial planning and local development co-management: Potentials and challenges in Greece. Sustainability 12, 2026.
Lacroix D., Pioch S. (2011). The multi-use in wind farm projects: more conflicts or a win-win opportunity? Aquat. Living Resour. 24, 129–135.
Lagasco F., Collu M., Mariotti A., Safier E., Arena F., Atack T., et al. (2019). New engineering approach for the development and demonstration of a multi-purpose platform for the blue growth economy. Proceedings of the ASME 2019 38th International Conference on Ocean, Offshore and Arctic Engineering. Volume 6: Ocean Space Utilization. Glasgow, Scotland, UK. 1-9. doi: 10.1115/OMAE2019-96104
Lester S. E., Costello C., Halpern B. S., Gaines S. D., White C., Barth J. A. (2013). Evaluating tradeoffs among ecosystem services to inform marine spatial planning. Mar. Policy 38, 80–89.
Lester S. E., Gentry R. R., Kappel C. V., White C., Gaines S. D. (2018b). Offshore aquaculture in the United States: Untapped potential in need of smart policy. Proc. Natl. Acad. Sci. 115, 7162–7165.
Lester S. E., Stevens J. M., Gentry R. R., Kappel C. V., Bell T. W., Costello C. J., et al. (2018a). Marine spatial planning makes room for offshore aquaculture in crowded coastal waters. Nat. Commun. 9, 1–13.
LiVecchi A., Copping A., Jenne D., Gorton A., Preus R., Gill G., et al. (2019). Powering the Blue Economy; Exploring Opportunities for Marine Renewable Energy in Maritime Markets (Washington, D.C: U.S. Department of Energy, Office of Energy Efficiency and Renewable Energy).
Long L., Liu H., Cui M., Zhang C., Liu C. (2024). Offshore aquaculture in China. Rev. Aquaculture 16, 254–270.
Maar M., Holbach A., Boderskov T., Thomsen M., Buck B. H., Kotta J., et al. (2023). Multi-use of offshore wind farms with low-trophic aquaculture can help achieve global sustainability goals. Commun. Earth Environ. 4, 447.
Macadré L. M., O’Sullivan K., Breuillard A., le Diraison S. (2014). “Risk-based approach for the development of guidelines and standards on combined marine renewable energy platforms,” in Proceedings of the ASME 2014 33rd International Conference on Ocean, Offshore and Arctic Engineering, San Fransisco, California, USA: American Society of Mechanical Engineers (ASME) Vol. 45530. V09AT09A046.
McCauley D. J., Pinsky M. L., Palumbi S. R., Estes J. A., Joyce F. H., Warner R. R. (2015). Marine defaunation: animal loss in the global ocean. Science 347, 1255641.
McKindsey C. W., Thetmeyer H., Landry T., Silvert W. (2006). Review of recent carrying capacity models for bivalve culture and recommendations for research and management. Aquaculture 261, 451–462.
MERMAID. (2024). Innovative multi-purpose offshore platforms: planning, design & operation. CORDIS: EU research results: Reporting. Available online at: https://cordis.europa.eu/project/id/288710/reporting (Accessed May 1, 2024).
Michler-Cieluch T., Krause G. (2008). Perceived concerns and possible management strategies for governing “wind farm–mariculture integration”. Mar. Policy 32, 1013–1022.
Michler-Cieluch T., Krause G., Buck B. H. (2009). Reflections on integrating operation and maintenance activities of offshore wind farms and mariculture. Ocean & Coastal Management 52(1), 57–68.
Morris J. A. Jr, MacKay J. K., Jossart J. A., Wickliffe L. C., Randall A. L., Bath G. E., et al. (2021). An Aquaculture Opportunity Area Atlas for the Southern California Bight Vol. 298 (Beaufort, NC: NOAA Technical Memorandum NOS NCCOS), 485. doi: 10.25923/tmx9-ex26
Multi-uses in European Seas (MUSES) Project (2024). Available online at: https://muses-project.com/?page_id=468 (Accessed May 1, 2024).
North Sea Farmers (NSF) (2024). North Sea Farm1. Available online at: https://www.northseafarmers.org/about-nsf1 (Accessed April, 13 2024).
O’Donncha F., James S. C., Ragnoli E. (2017). Modelling study of the effects of suspended aquaculture installations on tidal stream generation in Cobscook Bay. Renewable Energy 102, 65–76.
O’Hara C. C., Frazier M., Halpern B. S. (2021). At-risk marine biodiversity faces extensive, expanding, and intensifying human impacts. Science 372, 84–87.
OLAMUR (2024). OLAMUR Project Overview. Available online at: https://olamur.eu/project-overview/ (Accessed April, 13 2024).
Onea F., Rusu L. (2015). “Coastal impact of a hybrid marine farm operating close to the Sardinia Island,” in OCEANS 2015 (IEEE, Genova), 1–7.
Paez A. (2017). Gray literature: An important resource in systematic reviews. J. Evidence-Based Med. 10, 233–240.
Price C., Black K. D., Hargrave B. T., Morris J. A. Jr. (2015). Marine cage culture and the environment: effects on water quality and primary production. Aquaculture Environ. Interact. 6, 151–174.
Privitera-Johnson K. M., Punt A. E. (2020). Leveraging scientific uncertainty in fisheries management for estimating among-assessment variation in overfishing limits. ICES. J. Mar. Sci. 77, pp.515–pp.526.
Raghukumar K., Nelson T., Jacox M., Chartrand C., Fiechter J., Chang G., et al. (2023). Projected cross-shore changes in upwelling induced by offshore wind farm development along the California coast. Commun. Earth Environ. 4, 116.
Ross L. G., Telfer T. C., Falconer L., Soto D., Aguilar-Manjarrez J., Asmah R., et al. (2013). Carrying capacities and site selection within the ecosystem approach to aquaculture (Site selection and carrying capacities for inland and coastal aquaculture). Stirling, the United Kingdom of Great Britain and Northern Ireland. FAO Fisheries and Aquaculture Proceedings No. 21. Rome, FAO, 19.
Sanchez-Jerez P., Karakassis I., Massa F., Fezzardi D., Aguilar-Manjarrez J., Soto D., et al. (2016). Aquaculture’s struggle for space: the need for coastal spatial planning and the potential benefits of Allocated Zones for Aquaculture (AZAs) to avoid conflict and promote sustainability. Aquaculture Environ. Interact. 8, 41–54.
Schupp M. F., Bocci M., Depellegrin D., Kafas A., Kyriazi Z., Lukic I., et al. (2019). Toward a common understanding of ocean multi-use. Front. Mar. Sci. 6, 165.
Serpetti N., Benjamins S., Brain S., Collu M., Harvey B. J., Heymans J. J., et al. (2021). Modeling small scale impacts of multi-purpose platforms: an ecosystem approach. Front. Mar. Sci. 8, 694013.
Shawon M. H., Ghosh S. K., Rahman A. (2013). An integrated topology of hybrid marine farm & Wind farm. Int. J. Renewable Energy Res. 3, 246–254.
Sie Y. T., Château P. A., Chang Y. C., Lu S. Y. (2018). Stakeholders opinions on multi-use deep water offshore platform in Hsiao-Liu-Chiu, Taiwan. Int. J. Environ. Res. Public Health 15, 281.
Simal P. D., Ortega S. T., Bas B., Elginoz N., Garcia R. G., del Jesus F., et al. (2017). “Socio-economic assessment of a selected multi-use offshore site in the atlantic,” in The Ocean of Tomorrow: Investment Assessment of Multi-Use Offshore Platforms: Methodology and Applications, Cham, Switzerland: Springer. vol. 1. , 69–84.
Smith-Godfrey S. (2016). Defining the blue economy. Maritime affairs: J. Natl. maritime foundation India 12, 58–64.
Söderqvist T., Bas B., de Bel M., Boon A., Elginoz N., Garção R., et al. (2017). “Socio-economic analysis of a selected multi-use offshore site in the north sea,” in The Ocean of Tomorrow: Investment Assessment of Multi-Use Offshore Platforms: Methodology and Applications, Cham, Switzerland: Springer. vol. 1. , 43–67.
Soto D., Aguilar-Manjarrez J., Brugère C., Angel D., Bailey C., Black K., et al. (2008). “Applying an ecosystem-based approach to aquaculture: principles, scales and some management measures,” in Building an ecosystem approach to aquaculture, vol. 7. (: Palma de Mallorca, Spain: FAO/Universitat de les Illes Balears Expert Workshop). 15–35.
Steins N. A., Veraart J. A., Klostermann J. E., Poelman M. (2021). Combining offshore wind farms, nature conservation and seafood: Lessons from a Dutch community of practice. Mar. Policy 126, 104371.
Stelzenmüller V., Diekmann R., Bastardie F., Schulze T., Berkenhagen J., Kloppmann M., et al. (2016). Co-location of passive gear fisheries in offshore wind farms in the German EEZ of the North Sea: A first socio-economic scoping. J. Environ. Manage. 183, 794–805.
Stuiver M., Soma K., Koundouri P., Van den Burg S., Gerritsen A., Harkamp T., et al. (2016). The governance of multi-use platforms at sea for energy production and aquaculture: challenges for policy makers in European seas. Sustainability 8, 333.
Tethys (2023). OES-Environmental Metadata. Available online at: https://tethys.pnnl.gov/oes-environmental-metadata (Accessed November, 8 2023).
Tiller R., Gentry R., Richards R. (2013). Stakeholder driven future scenarios as an element of interdisciplinary management tools; the case of future offshore aquaculture development and the potential effects on fishermen in Santa Barbara, California. Ocean Coast. Manage. 73, 127–135.
ULTFARMS. (2024). Publications. Available online at: https://www.h2020united.eu/publications (Accessed May 1, 2024).
UNITED. (2024). Multi-Use offshore platforms demonstrators for boosting cost-effective and eco-friendly production in sustainable marine activities. Publications. Available online at: https://www.h2020united.eu/publications (Accessed May 1, 2024).
Van Hoof L., Van den Burg S. W. K., Banach J. L., Röckmann C., Goossen M. (2020). Can multi-use of the sea be safe? A framework for risk assessment of multi-use at sea. Ocean Coast. Manage. 184, 105030.
Weiss C. V., Ondiviela B., Guinda X., del Jesus F., González J., Guanche R., et al. (2018). Co-location opportunities for renewable energies and aquaculture facilities in the Canary Archipelago. Ocean Coast. Manage. 166, 62–71.
Wever L., Krause G., Buck B. H. (2015). Lessons from stakeholder dialogues on marine aquaculture in offshore wind farms: Perceived potentials, constraints and research gaps. Mar. Policy 51, 251–259.
Xepapadeas P., Giannouli A., Koundouri P., Moussoulides A., Tsani S., Xepapadeas A. (2017). “Risk analysis for the selected MERMAID final designs,” in The Ocean of Tomorrow: Investment Assessment of Multi-Use Offshore Platforms: Methodology and Applications, Cham, Switzerland: Springer. vol. 1. , 105–137.
Keywords: co-location, offshore aquaculture, marine spatial planning, renewable energy, multi-use
Citation: Gonzales CM, Chen S and Froehlich HE (2024) Synthesis of multinational marine aquaculture and clean energy co-location. Front. Aquac. 3:1427839. doi: 10.3389/faquc.2024.1427839
Received: 04 May 2024; Accepted: 22 July 2024;
Published: 12 August 2024.
Edited by:
Bela H. Buck, Alfred Wegener Institute Helmholtz Centre for Polar and Marine Research (AWI), GermanyReviewed by:
Sander Van Den Burg, Wageningen University and Research, NetherlandsWolf Isbert, Alfred Wegener Institute Helmholtz Centre for Polar and Marine Research (AWI), Germany
Copyright © 2024 Gonzales, Chen and Froehlich. This is an open-access article distributed under the terms of the Creative Commons Attribution License (CC BY). The use, distribution or reproduction in other forums is permitted, provided the original author(s) and the copyright owner(s) are credited and that the original publication in this journal is cited, in accordance with accepted academic practice. No use, distribution or reproduction is permitted which does not comply with these terms.
*Correspondence: Claire M. Gonzales, Y2xhaXJlZ29uemFsZXNAdWNzYi5lZHU=