- 1Department of Aquaculture and Sea-Ranching, Faculty of Agricultural and Environmental Sciences, University of Rostock, Rostock, Germany
- 2Working Group Fish Genetics, Research Institute for Farm Animal Biology (FBN), Dummerstorf, Germany
As nutrient-rich water in aquaponic systems cannot supply growing plants with all the required trace elements, supplementation with specific fertilizers is performed to make up for this deficit. While chelated fertilizers such as ethylenediaminetetraacetic acid–zinc disodium complex (Zn-EDTA) are becoming more popular in this context for improving plant growth in aquaponic systems, little is known about their effects on fish. During two experiments, a total of 576 individuals of catfish fry (0.19 g) and fingerlings (220.01 g) of the African catfish (Clarias gariepinus; Burchell, 1822) were kept separately for 32 days under experimental aquarium conditions. The fry was exposed to 0.125 and 0.5 mg/L, while the fingerlings were exposed to 0.5 and 2.0 mg/L Zn-EDTA in a plantless aquaponic system. The third treatment group consisted of a control group without Zn-EDTA. The growth, mortality, and ethological indicators were assessed for all growth stages, while the leukocyte distribution and histopathological changes were additionally determined for the fingerlings. As the feed intake in the experiment was limited, the investigations were focused on the effects of Zn-EDTA and not on the growth process of a respective fish growth stage. While the growth, mortality, and behavior were not significantly different in both growing stages, the number of mature neutrophils changed significantly in all treatments in fingerlings. Zn was not detected in the histologically investigated organs at the tested concentrations using the staining method. However, morphological alterations of the gill epithelium were found on the secondary lamellae. Quantitative multiplex PCR was used to simultaneously evaluate the expression of 17 genes related to Zn metabolism and stress physiology in head kidney samples. The transcripts of several selected genes changed by up to 70-fold. Due to high individual variances, only the copy numbers of the KMT2A (lysine-specific methyltransferase 2a) gene were significantly different across treatment groups and sampling points. However, the present results indicate that the addition of Zn-EDTA at the tested concentrations can be considered relatively benign for the health and welfare of C. gariepinus, as no toxic effects of Zn-EDTA were observed in moderately hard to hard water.
Highlights
● First study investigating the multiple effects of Zn-EDTA exposure on the African catfish, Clarias gariepinus, to aquaponic-related concentrations.
● Zn-EDTA exposure up to 2.0 mg/L showed small effects in the catfish liver.
● Zn-EDTA exposure up to 0.5 mg/L increased the occurrence of edema, fusions, and hyperplasia in catfish gills.
● Zn-EDTA exposure affects the gene expression of KMT2A and MTF1 in catfish fingerlings.
1 Introduction
Presently, fish are increasingly exposed to various fertilizer components originating from fertilizer discharges from agriculture and chemical industry facilities or as emissions resulting from mining activities. In agriculture, the combined production of fish and plant, known as aquaponics, uses circulating nutrient-rich water and the addition of specific fertilizers to provide cultivated plants with all the essential nutrients, which, together, lead to better plant development and productivity of the system. Since the water used for this plant cultivation system usually originates from a closed aquaculture system and is therefore reused for plant growth, aquaponics is generally considered a highly sustainable method for crop production (Stathopoulou et al., 2021). However, as the single nutrient composition of the circulating water of an aquaculture system, as well as through supply by fish feed, is mostly insufficient (Sonneveld et al., 2009; Krishnasamy et al., 2012; Bittsanszky et al., 2016), the supplementation of fertilizers seems inevitable (Da Silva Cerozi, 2020; Stathopoulou et al., 2021). Similar to other plants, aquaponically grown plants have species-specific requirements of macro- and micronutrients. Since nitrogen (N) and phosphorus (P) are supplied by the fish in an aquaponic system, only the trace nutrients such as iron (Fe), copper (Cu), boron (B), molybdenum (Mo), manganese (Mn), zinc (Zn), and potassium (K) might be deficient to meet the plant’s requirements (Strauch et al., 2018). To balance these deficits in an aquaponic system, nutrients can either be added to the water as compounds of salts such as sulfates (SO4−2), chlorides (Cl−), or nitrates (NO3−); as part of chelated compounds such as ethylenediaminetetraacetic acid (EDTA), ethylenediamine-N,N′-bis(2-hydroxyphenylacetic acid) (EDDHA), or diethylenetriaminepentaacetic acid (DTPA); or as components of organic acids including fulvic acid or amino acids such as arginine, glycine, or histidine (Linnik and Ignatenko, 2015; Shuhaimi-Othman et al., 2015; Kasozi et al., 2019; Da Silva Cerozi, 2020; Neocleous et al., 2020; Yep and Zheng, 2020).
Not only for aquaponic plants, Zn is one of several elements necessary for development and growth by protein synthesis, carbohydrate metabolism, pollen formation, heat stress, protection from photo-oxidative damage, biological membrane preservation, or infection resistance (Alloway, 2008). Similar to other plants, aquaponic plants can react to a Zn deficiency situation with many symptoms, including browning of the leaves due to a specific crossing structure, stagnation of the growth process, and the formation of infertile buds (Goddek et al., 2019; Yang and Kim, 2019). In order to avoid the aforementioned deficits, Zn, as a component of different chemical compounds, is already supplemented at concentrations up to 0.45 mg/L in hydroponic systems (Krishnasamy et al., 2012; Neocleous et al., 2020). At present, in aquaponic cultivation systems, the targeted supplementation of Zn is processed in combination with an EDTA chelator, which provides resistance against oxidation or dissolution and enables a constant pH-dependent availability of Zn in the water to a plant (Rakocy, 2012).
As an essential nutrient for both plants and fish, Zn plays a fundamental role in cell metabolism. In finfish, the uptake, efflux, and compartmentalization of Zn ensure homeostasis and prevent its toxic overaccumulation (Zheng et al., 2010). Its main vectors into a fish body are the uptake through the gills or via the gastrointestinal tract (Watanabe et al., 1997; Coetzee et al., 2002; Mahboub et al., 2020). Zn2+ transport via a chelate complex (i.e., EDTA) has also not been excluded for aquatic species (Hockett and Mount, 1996; Karayakar et al. (2021). As an essential element for life, Zn is also present in several amino acids and enzymes and plays a central role in protein folding (Ezeonyejiaku et al., 2010, 2012). In addition, recent studies have underlined its importance in enhancing the reproduction, antioxidant response, and growth performance of fish (Onuegbu et al., 2018; Kaliky et al., 2019).
As a variety of fish species can live in the reused water of an aquaponic system, an imbalance in the essential plant nutrients such as Zn, which are metabolically active to the fish, can negatively affect the growth, health, and welfare of specific fish species (Karayakar et al., 2021). Thus, controlled supplementation is important. In particular, the concentration thresholds of these micronutrients should be well known and not exceeded in cultivated species. An overdose of micronutrients, which is usually species-specific, can ultimately reduce the growth, damage the internal organs, induce different types of diseases, increase mortality, and even change the behavior of fish (Karayakar et al., 2021). Various negative reactions of fish to Zn have recently been reported, including the increased mortality of Clarias gariepinus in LD50 toxicity studies to zinc sulfate (ZnSO4) at 71.0 and 75.3 mg/L supplementation (Ezeonyejiaku et al., 2010; Chidiebere, 2019); the inhibited growth of Oncorhynchus mykiss and Oreochromis niloticus (Eisler, 1993; Abdel-Tawwab et al., 2012); hypocalcemia in O. mykiss (Niyogi and Wood, 2006); the increased glucose, cortisol, and creatinine as a stress response in O. niloticus (Firat and Kargin, 2010; Abdel-Tawwab, 2016); behavioral alterations in C. gariepinus and O. niloticus (Ololade and Ogini, 2009; Duran and Erdem, 2014; Tunçsoy and Erdem, 2014; Chidiebere, 2019); changes in the physiological and biochemical properties of the blood or immune cells in Ictalurus punctatus and C. gariepinus (Lewis and Lewis, 1971; Gatlin et al., 1989; Coetzee et al., 2002; Ololade and Ogini, 2009); and the pathological alterations in the organs of Pimephales promelas, Pseudupeneus prayensis, and C. gariepinus (Brungs, 1969; Heath, 1995; Roméo et al., 1999; Coetzee et al., 2002; Shuhaimi-Othman et al., 2015), such as hemorrhage in the gills or fins and extensive body and gill mucus in Labeo rohita and O. mykiss (Bengari and Patil, 1986; Hogstrand et al., 2002; Wood, 2017). Furthermore, several accumulation ratios of Zn and Zn-EDTA in the gill and liver tissues of Cyprinus carpio had been described by Karayakar et al. (2021), which will be discussed in this context.
Over the last few years, tolerance tests for the compatibility of nitrate–phosphorus–potassium (NPK) fertilizers, trace potassium nutrients, and Fe-DTPA have been carried out for the African catfish (C. gariepinus; Burchell, 1822), confirming their beneficial potential in aquaponics (Ajima et al., 2015; Shuhaimi-Othman et al., 2015; Wenzel et al., 2021; Hildebrand et al., 2023). Aligning with this approach, the present study aimed to quantify the effects of Zn-EDTA on the health and welfare of African catfish fry and fingerlings in two separate experiments using concentrations relevant for aquaculture and aquaponics (approximately 0.5 mg/L). Therefore, the fry and juveniles of African catfish were treated with various concentrations of Zn-EDTA in two separate experiments, focusing on the growth performance, ethological indicators, blood and histopathological analyses, and genetic profiling of critical genes to assess the potential hazards of Zn fertilization to the selected fish species (Seibel et al., 2021). From this assessment, we expected Zn-EDTA to have no adverse effects on the health and welfare of this species at water concentrations equivalent to those recommended for plant growth in aquaponic systems (0.45 mg/L).
2 Material and methods
2.1 Experimental design and system maintenance
In order to investigate the responses to the target concentration of 0.5 mg/L Zn-EDTA of two different growth stages of the African catfish, two separate experiments using concentrations close to 0.5 mg/L were carried out for 32 days each at the aquaculture research facility “Fischglashaus” of the University of Rostock, Germany. In the first experiment (Exp. A), African catfish fry were exposed to concentrations lower than 0.5 mg/L Zn-EDTA (control, 0.125, and 0.5). In the second experiment (Exp. B), African catfish fingerlings were exposed to concentrations higher than 0.5 mg Zn-EDTA (control, 0.5, and 2.0) as they are supposedly less sensitive to nutrient solutions. We applied the definition of the three Zn-EDTA treatments (0.125, 0.5, and 2.0 mg/L) based on the calculated effect size in each experimental design. This included an additional safety threshold of 10% to the required minimum hydroponic concentration of 0.45 mg/L.
To create a respective Zn milieu, we used Zn-EDTA containing 14.5% Zn2+, supplied by Phygenera Germany (EC no. 237–865-0), and labeled the respective treatments in Exp. A as A-ZnEDTA-0.125 and A-ZnEDTA-0.5, while those in Exp. B were accordingly labeled as B-ZnEDTA-0.5 and B-ZnEDTA-2.0. The control treatments in both experiments were labeled ZnEDTA-0 and had a total background Zn concentration of 0.051 mg/L. To maintain stable concentrations of Zn-EDTA, we followed the dilution method and dosing protocol of Hildebrand et al. (2023) for our fertilizer and water management. In each experiment, the fish were stocked as triplicates in three separate recirculation systems, with three tanks (L × W × H: 100 cm × 50 cm × 32 cm for Exp. A and 100 cm × 50 cm × 35 cm for Exp. B) and one filter unit each (approximately 450 L). This resulted in a total system capacity of 950 L in Exp. A and 1,000 L in Exp. B. The rearing volume per tank in the catfish fry experiment was reduced to approximately 50 L habitat by placing perpendicularly oriented filter mats. In the fingerling experiment, no restrictions were imposed on the tank volume (120 L).
Common water quality parameters including temperature, dissolved oxygen (DO), electrical conductivity (EC), redox potential, and pH were determined daily using a portable multimeter (HQ40D; Hach Lange, Berlin, Germany). Samples were taken from the sumps in 3-day intervals to analyze nitrate (NO3−), nitrite (NO2−), ammonium (NH4+), and ortho-phosphate (PO42−) using an Autoanalyzer (Gallery™, Automated Photometric Analyzer, Thermo Fisher Scientific, Waltham, MA, USA). The total Zn concentrations were determined using Hach-Lange cell tests (LCK360 and LCS360), with the acid digestion (LCW902) conducted via a separate photometer (DR3900 RFID Spectrophotometer; Hach Lange). When the Zn concentrations deviated from the target concentrations, an additional Zn-EDTA solution was added.
2.2 Fish stocking and feeding
For each experiment, a total of 288 immature male and female African catfish (mixed sex) were received from a local fish hatchery (PAL Aquaculture GmbH, Kiel, Germany). The fry group (Exp. A) had a mean weight of 0.19 ± 0.02 g and a mean length of 2.8 ± 0.2 cm. Fish were stocked with 14 individuals per tank (3.6 fish/L). The fingerling group (Exp. B) had a mean weight of 220.01 ± 25.09 g and a mean length of 30.0 ± 1.7 cm. They were identified by sex through their abdomen and then stocked equally with 17 fish per tank (7.0 fish/L). Untreated water was used for 9 days to acclimatize the fish before exposure to the different concentrations of Zn-EDTA.
Reared fish were fed a commercial pelleted catfish diet (Exp. A: Coppens Start Premium, 0.3–1.5 mm; Exp. B: Coppens Special Premium, 5.0 mm) containing protein (45% A and 43% B), fat (15% A and 14% B), crude fiber (0.3% A and 1.6% B), ash (11.4% A and 6.7% B), total phosphorus (1.77% A and 1.01% B), and vitamin A (12,000 IU/kg A and 10,000 IU/kg B). In order to determine the performance of the fish in the current study, performance indicators (FCR, SGR, CF) were calculated by following Equations 1–3 respectively. To avoid high nutrient accumulation, the calculated feed amounts for fish fry were set to 3% and for fingerlings to 0.7% of their respective mean body weight. The corresponding daily feeding quantity was distributed by the number of feedings per day, as the catfish fry were fed four times (at 000, 600, 1200, and 1800 hours) and the fingerlings fed only once (at 1200 h). Over the 32-day experimental period, 14.0 g per tank was fed in Exp. A, while 52.3 g was fed in Exp. B.
The feed conversion ratio (FCR) was calculated as:
where TFI is the total feed intake (in grams), W0 is the initial fish weight (in grams), and W1 is the final fish weight (in grams).
The specific growth rate (SGR) was calculated as:
where W0 is the initial fish weight (in grams), W1 is the final fish weight (in grams), and T is the number of days.
2.3 General sampling procedures
The African catfish fry in Exp. A were captured in total and their weight and length measured on days 11, 12, 21, and 32 to monitor their weekly growth performance. Subsequently, the fish were placed back into their respective rearing tanks. In Exp. B, three fingerlings were taken from each group on days 11, 12, 21, and 32, stunned manually, and then killed. Afterward, the sex, length, and weight were determined. The fish collected on days 11, 12, 21, and 32 were further examined for their potential genetic reaction to Zn-EDTA (see Sections 2.5 and 2.6) and for changes in their blood on days 11, 21, and 32 (see Section 2.4). Blood samples of approximately 5 mL were taken from the caudal vessels of the fish, transferred into a cooled EDTA tube (BD Vacutainer, K2E 5.4 mg), and further processed for smear analysis shortly thereafter.
By the end of the experiments, all individuals were counted, measured, and then killed.
Fulton’s condition factor (CF) was estimated according to Ricker (1973).
where CF is Fulton’s condition factor, W is the weight (in grams), and L is the length (in centimeters).
All treatments were carried out in accordance with the EU guidelines for animal experiments and have been approved by the responsible ethics committee.
2.4 Blood smear analysis and skin lesion documentation
In Exp. B, blood samples from days 11, 21, and 32 were transferred into EDTA tubes (BD Vacutainer, K2E 5.4 mg) and cooled subsequently. Blood smear slides were then obtained from 50 µL of the cooled blood samples and stained according to Pappenheim (Mulisch, 2015). Subsequently, the samples were fixed in Canada balsam to assess the immune status by cell type distribution using a leukogram. Collectively, these preparations were analyzed under a light microscope (Olympus BX 53; cellSens Application Software, v. 1.5) at ×500 magnification. In order to create a differential blood count of a specific treatment group, at least 100 cells per blood carrier were counted and the leukocyte types determined. The counting procedure was repeated five times to increase the statistical accuracy according to Rümke and Klein (1987). Finally, the asexual mean values of the respective treatments were calculated, taking into account the cell counts of three samples from a particular group. Possible sex-related differences in the mean values of the cell populations were terminated using a standard counting procedure. Further cell type determination was conducted according to the methodology of Hildebrand et al. (2023).
For the fingerlings in Exp. B, the size and the quantity of skin lesions were registered for each sampled fish. For the determination of the lesion area, a transparent template with a 0.25-cm2 grid was placed on each lesion so that the smallest possible lesion area could be noted. Lesion areas per fish were then summed in total. This procedure was not performed in Exp. A as the fish in this group were too small.
2.5 Organ retrieval and histological analysis
For the fry that were sampled in Exp. B, special attention was paid to the exposure of relevant organs for toxicity testing. Hepatic tissue was collected by cutting a 5-mm3 fragment from the mid-liver area. A tissue sample (1 cm) was collected from the outer left astial arch of a gill. Both of the organs were preserved in 15% formalin/5% methanol and stored at 8°C. On days 11, 12, 21, and 32, the upper part of the head kidney was removed, stored at −80°C, and used for gene expression analyses. Histological specimens were prepared according to the protocol of Hildebrand et al. (2023) and subsequently stained with a Midorikawa solution (Midorikawa and Eder, 1962) to identify Zn2+ inclusions. The created histopathological samples were analyzed using a scanning microscope (Leica DM4 B; LAS X Application Software, v. 5.02). Images were evaluated and the severity grade of each organ sample was determined according to the classification of Johnson et al. (2009). The severity grades were defined as follows (Johnson et al., 2009):
G0: unremarkable (no findings associated with a specific diagnostic criterion);
G1: minimal (unremarkable to barely noticeable, fewer than two occurrences per microscopic field);
G2: mild (conspicuous feature of tissue, three to five occurrences per microscopic field);
G3: moderate (dominant feature of tissue, six to eight occurrences per microscopic field); and
G4: severe (overwhelming feature of tissue, more than nine occurrences per microscopic field)
2.6 RNA isolation, primer design, and multiplex quantitative PCR
A panel of oligonucleotide primers of genes related to Zn homeostasis and general stress responses had been designed in a previous study (Hildebrand et al., 2023). From this set (Table 1), 17 primer pairs were selected to profile the expression of ATP6V1G1 (ATPase H+ transporting v1 subunit g1), CASP3 (caspase 3), CCNB1 (cyclin b1), CS (citrate synthase), HMOX1A (heme oxygenase 1), HSP90AB1 (heat-shock protein 90 alpha family class b member 1), HSPD1 (heat shock protein family d, member 1), IL2 (interleukin-2), KMT2A (lysine-specific methyltransferase 2a), MTF1 (metal regulatory transcription factor 1), NKX2–3 (nk2 homeobox gene 3), SLC30A5 (solute carrier family 30, member 5), SLC39A8 (solute carrier family 39, member 8), SP1 (sp1 transcription factor), STK39 (serine/threonine kinase 39), UCP2 (uncoupling protein 2), and ZEB1 (zinc finger e-box binding homeobox 1). In addition, four reference genes—RNA18S (RNA, 18S ribosomal), RPL (ribosomal protein pseudogene), ACTB (actin beta), and GAPDH (glyceraldehyde-3-phosphate dehydrogenase)—were included to serve as internal normalizers. The preparation of the RNA samples from the head kidneys of the treated and control fish (n = 3 per group) and the multiplex quantitative PCR analyses using the BioMark HD system (Standard BioTools, San Francisco, CA, USA) were carried out according to a previously published protocol (Hildebrand et al., 2023).
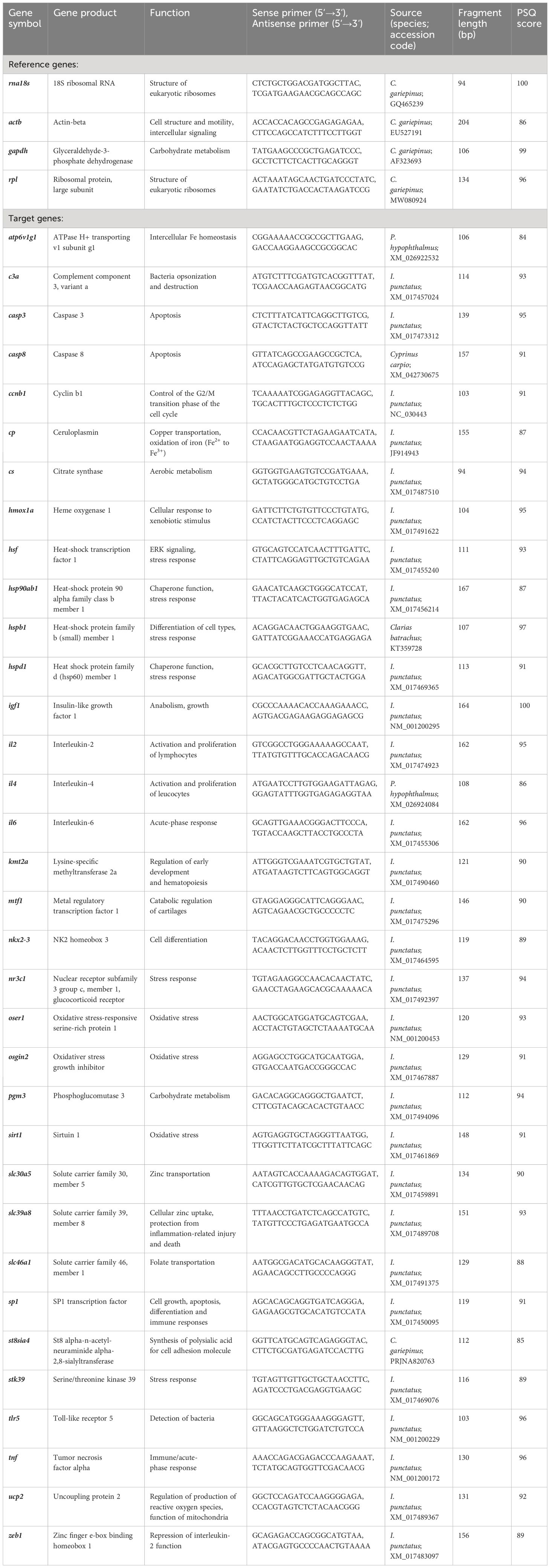
Table 1 Oligonucleotide-primer sequences derived from Clarias gariepinus, Ictalurus punctatus or Pangasianodon hypophthalmus from (Hildebrand et al., 2023).
2.7 Ethology analysis
Video-recorded ethological observations of all reared fish (including the swimming activity, escape attempts, agonistic behavior, air breathing, and stereotypy) were conducted in both experiments in order to assess possible effects of Zn-EDTA on the fish. Three independent recordings of a respective treatment were taken for 20 min on days 8, 18, and 28 following the recording protocol of Hildebrand et al. (2023). No fertilizer was used in the first recording session to provide each group with a reference for the following sessions. This allowed better identification of any variability due to the individual composition of the fish stock. An ethogram based on Van de Nieuwegiessen et al. (2008) was slightly modified following the scheme of Hildebrand et al. (2023) and was used to evaluate behavior. The total fish activity was calculated on the basis of all visible swimming and resting fish within a 5-min interval. All swimming and resting individuals were then counted and the numbers related to the total number of fish.
2.8 Statistics
Statistical analysis was performed using the Statistical Package for the Social Sciences (SPSS), version 27.0 (IBM Corp., 2020, Armonk, NY, USA). Mean values were initially analyzed using the Shapiro and Levene’s tests in order to assess the normal distribution and homogeneity of variances of the data. For normally distributed data, one-way analysis of variance (ANOVA) was performed. Kruskal–Wallis test was performed if the processed data were not normally distributed. If inhomogeneous variances were indicated by the Levene’s test, a one-way Welch ANOVA was performed. In the case of significance (p< 0.05), the post-hoc tests Tukey’s HSD (ANOVA), Dunnett T3 (Kruskal–Wallis), or Games–Howell (Welch’s ANOVA) were conducted. Quantitative PCR (qPCR) data were analyzed using the RealTime PCR Analysis Software v. 4.5.2 (Standard BioTools, San Francisco, CA, USA). The results were then normalized against the geometric mean of three appropriate reference genes (i.e., RNA18S, ACTB, and GAPDH). The normalized copy numbers of the target genes were then analyzed using two-way ANOVA followed by Holm–Šidák’s post-hoc test.
3 Results
3.1 Water quality
The mean water physicochemical parameters are listed in Table 2. The temperature of the water was stable in both experiments (p > 0.05). The measured oxygen saturation was slightly elevated in the control group of Exp. A and differed significantly from that of the ZnEDTA-0.5 group (p< 0.05), whereas the saturation values in Exp. B did not differ significantly between treatments. The EC values fluctuated in the water of both experiments and showed a maximum within the groups exposed to the highest Zn-EDTA concentrations (B-ZnEDTA-0.5 and B-ZnEDTA-2.0), which were significantly different from the control group (ZnEDTA-0). In addition, the measured redox potential decreased with increasing Zn-EDTA (p > 0.05). In both experiments, the pH values were nearly constant, i.e., approximately 8.6 in Exp. A and approximately 6.3 in Exp. B. The concentrations of ortho-phosphate and ammonium were found to be significantly different in Exp. A and Exp. B. A-ZnEDTA-0.125 showed a significantly lower concentration of ortho-phosphate compared with A-ZnEDTA-0.5 and the control, while B-ZnEDTA-0.5 showed a significantly higher concentration of ammonia compared with B-ZnEDTA-2.0 and the control. The CaCO3 concentrations fluctuated slightly between 232.36 and 253.50 mg/L (from 13.03°dH or 2.32 mmol/L to 14.22°dH or 2.54 mmol/L), indicating moderate to hard water. As expected, there was a significant increase in total Zn with rising concentrations in the surrounding water.
3.2 Growth and mortality
The initial lengths and weights of the raised catfish were similar (p > 0.05) in all the treatment groups within the respective experiment. The final lengths, weights, and CF were also similar (p > 0.05). In the course of Exp. A, a few losses occurred (p > 0.05), but not as usual in a tank. In fact, the fish left their tanks by jumping and consequently died. In Exp. B, there was no mortality. The percent mortality and actual numbers are reported in Table 3.
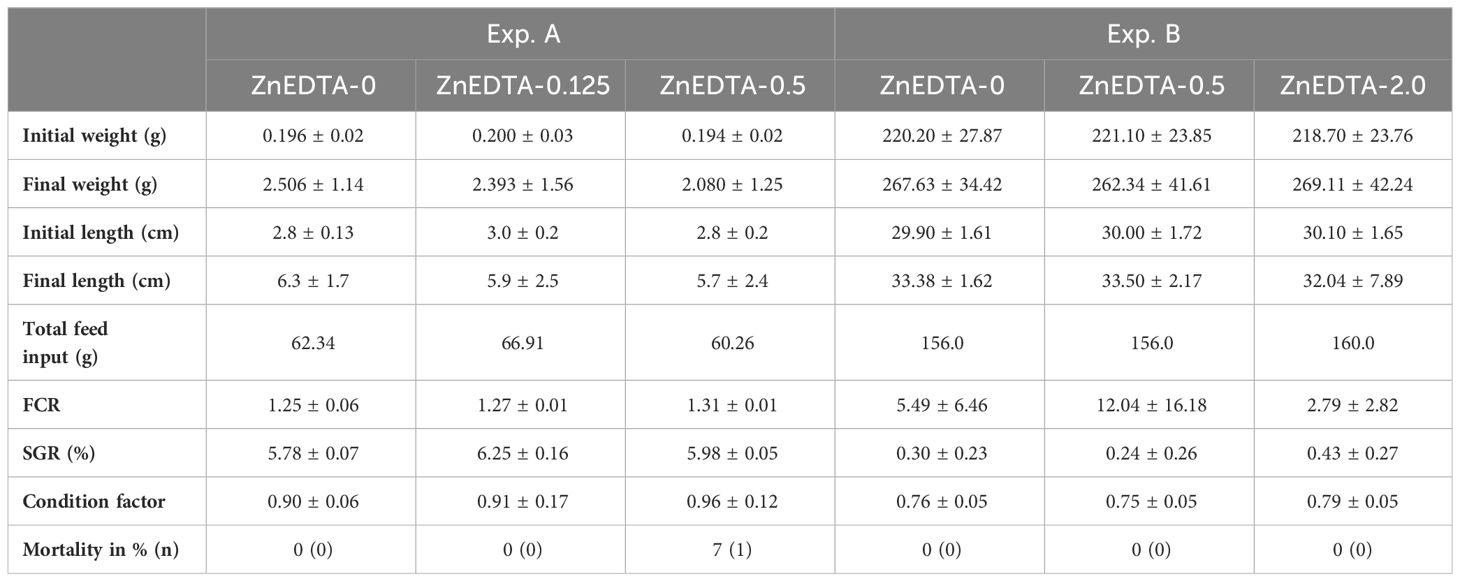
Table 3 Mean growth (± standard deviation (SD)) and mortality of Clarias gariepinus exposed to different Zn-EDTA concentrations.
3.3 Fish behavior
In both experiments, no significant changes in the behavior of fish from the different treatment groups were observed upon the addition of Zn-EDTA. In Exp. A, significant differences were found in the air-breathing and agonistic behavior between days 8 and 28 in A-ZnEDTA-0.5 and A-ZnEDTA-0 (Table 4). Similarly, in Exp. B, the swimming activity and agonistic behavior showed significant differences between days 8 and 28. However, minor effects across all treatment groups were observed in both experiments. For example, an increase in the air-breathing activity was observed in Exp. A and Exp. B over time. The number of skin lesions fluctuated largely during the experiment. Therefore, neither a correlation to the fertilizer addition nor to any other behavioral pattern could be determined.
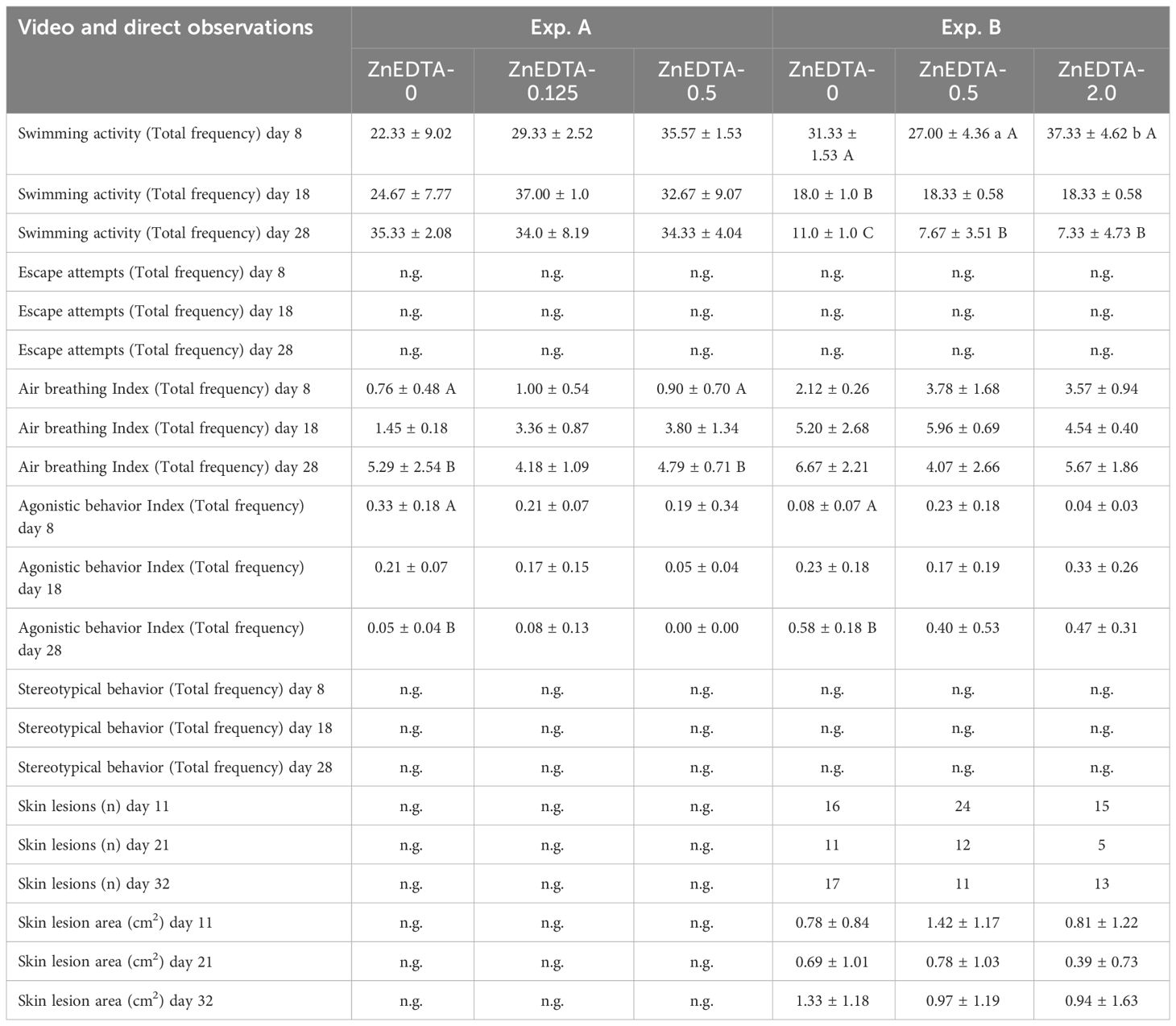
Table 4 Mean behavioral responses (±SD, n = 3) of Clarias gariepinus fry (Exp. A) and fingerlings (Exp. B) exposed to different levels of Zn-EDTA.
3.4 Leukogram
The leukocyte cell type distribution of the fingerlings in Exp. B is shown as percentage in Table 5. As a result, the class distribution of the known African catfish granulocyte types was calculated as a percentage of the total cell count. On day 11, there were no significant differences in the main cell types of leukocytes (e.g., lymphocytes and monocytes) and the types of emerging and mature neutrophils and eosinophils between treatments. Moreover, there was a significant difference in the presence of meta-granulocytes between B-ZnEDTA-0.5 and B-ZnEDTA-0 24 h after exposure. After 32 days of Zn-EDTA exposure, the cell distribution of mature neutrophils was significantly different between B-ZnEDTA-2.0 and B-ZnEDTA-0. Only a significantly lower number of meta-granulocytes were found between the respective control groups among the all-sampling days (p< 0.05). During the entire study (32 days), the cell distribution of mature neutrophils and the cell types of their precursors (neutrophil meta-/meso-granulocytes) changed significantly in all three treatment groups.
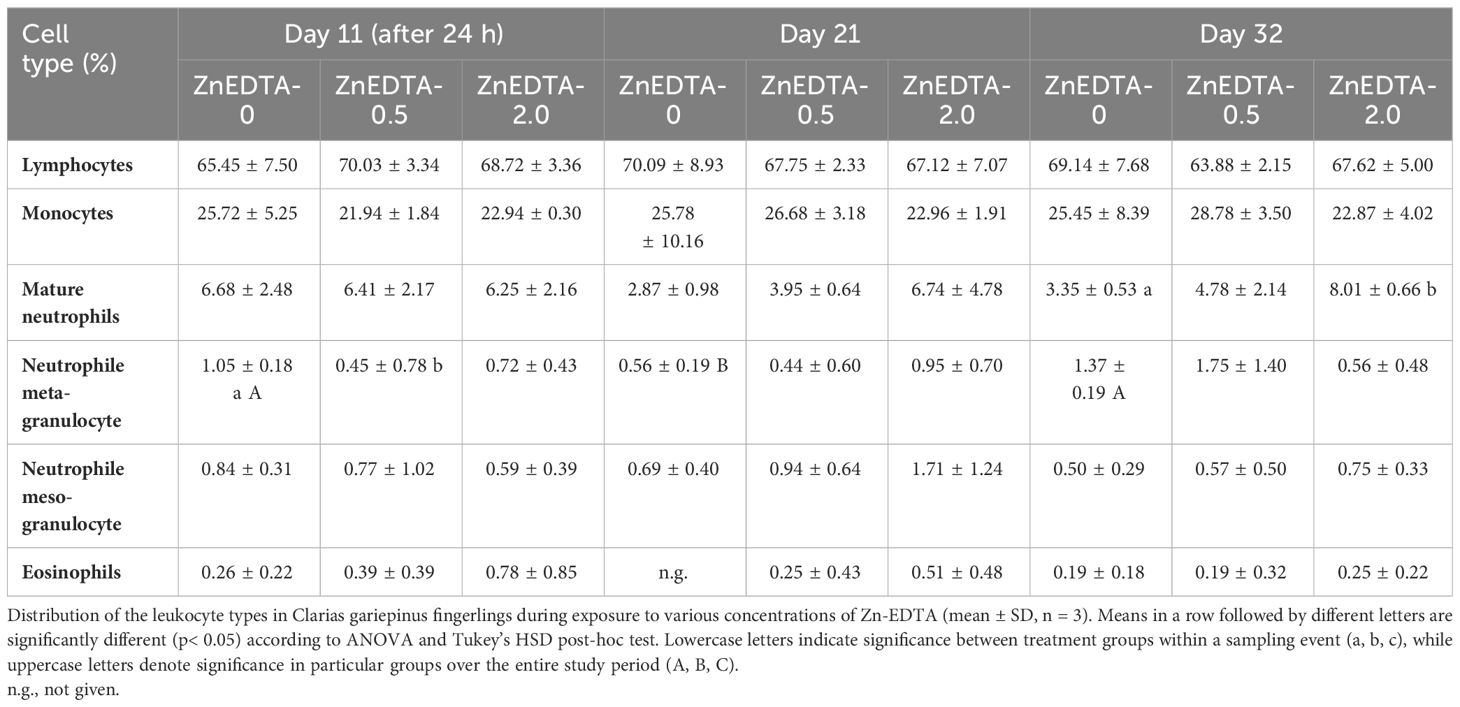
Table 5 Differential blood count in Exp. B. The distribution of leucocyte types of C. gariepinus fingerlings during the exposure to different concentrations of Zn-EDTA (means ± standard deviation, n=3).
3.5 Histological assessment
In Exp. B, the results of the histopathological examination of the organ tissues of the fingerlings are presented in Figures 1A-F. Cells of the primary and secondary gill filaments in each group showed no Zn inclusions. However, mild alterations (G2) such as hyperplasia, fusion of the secondary lamellae, alteration of mucous cells, and edema occurred in the gill filaments, especially in the B-ZnEDTA-0.5 and B-ZnEDTA-2.0 groups. However, this was not noticeable in the control group (G0).
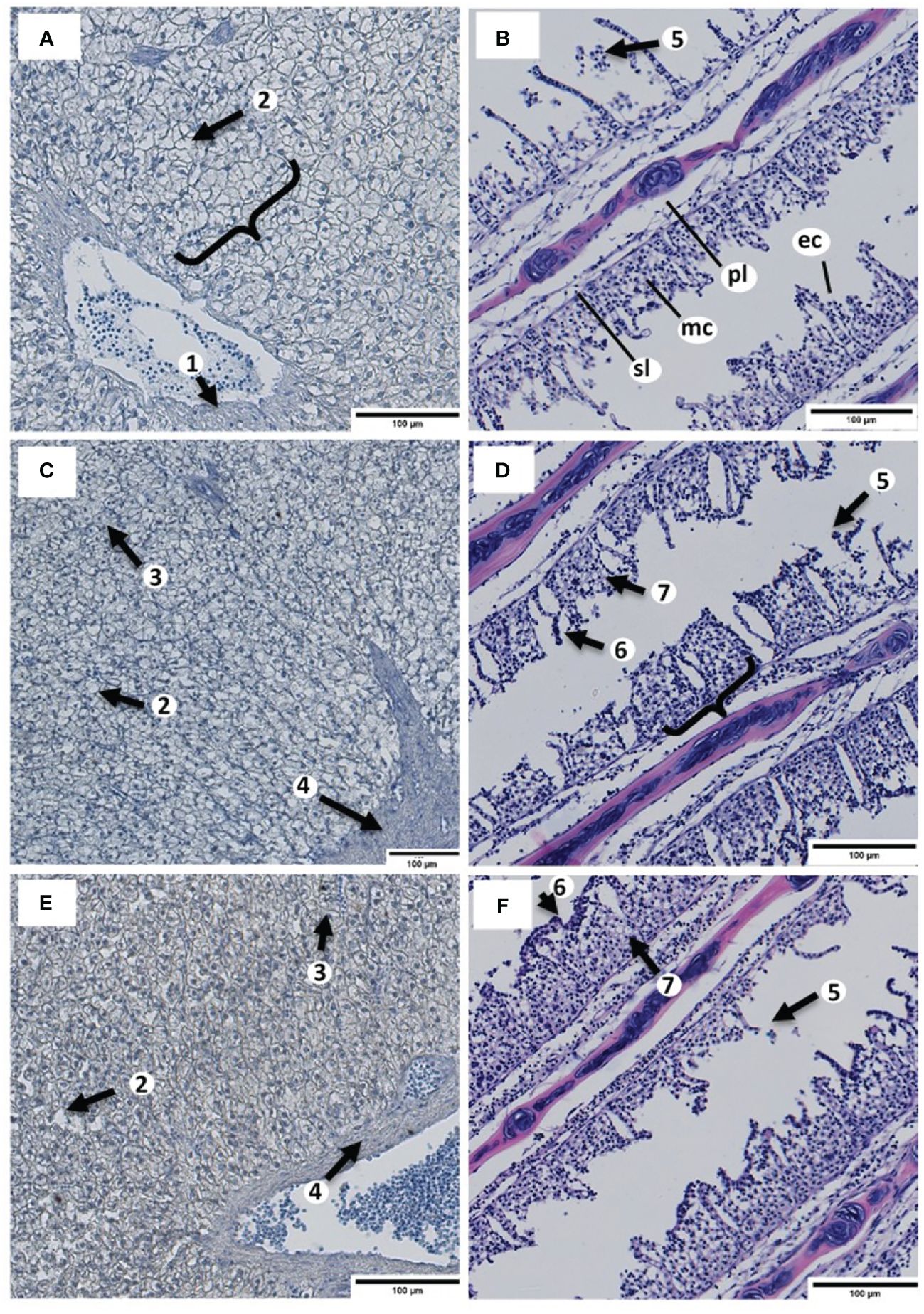
Figure 1 Histopathological evaluation of the liver and gill tissues of Clarias gariepinus fingerlings exposed to different Zn-EDTA concentrations in Exp. B after 32 days to a scale of 100 µm. The liver tissues (A, C, E) were stained to detect Zn (red/brown) according to Midorikawa and Eder (1962). The gill tissues (B, D, F) were stained using a common hematoxylin stain. (A) Liver cells of the B-ZnEDTA-0 control group with hepatic plate (clamp), vein with erythrocytes (arrow 1), white spotted lipid droplets (arrow 2), and without a noticeable coloration by Midorikawa Zn stain. (B) Gill filaments of the B-ZnEDTA-0 control group with primary lamellae (pl), secondary lamellae (sl), mucous cells (mc), and epithelial cells (ec) without remarkable inclusions or necroses. (C) Liver cells of the B-ZnEDTA-0.5 group with lipid droplets occurring next to hepatocytes (arrow 2), sinusoids congested with red blood cells (arrow 3), hepatic artery (arrow 4), and without coloration by Midorikawa stain. (D) Gill filaments of the B-ZnEDTA-0.5 group. Epithelial mucous detachments of the gills occurred in all groups (B, D, F) in Exp. B (arrow 5). Hyperplasia (arrow 6) and fusion of the secondary lamellae (clamp), in addition to an increased number of mucous cells and edema (arrow 7). (E) Liver cells of the B-ZnEDTA-2.0 group with light Midorikawa Zn stain coloration (light red brown hepatocytes) around the vascular arterial tissue (arrow 4), lipid droplets (arrow 2), and sinusoids (arrow 3). (F) Gill filaments of the B-ZnEDTA-2.0 group. Comparable findings to those of B-ZnEDTA-0.5 (D).
3.6 Gene expression profiling
A panel of catfish-specific primers established in a previous study (Hildebrand et al., 2023) was used to profile the expression of 17 genes in the head kidney of African catfish on days 11, 12, 21, and 32 after Zn-EDTA exposure (Table 1). The transcript levels of a number of genes, including SP1, MTF1, ZEB1, HSP90AB1, and STK39, were similar across all treatment groups investigated. A few genes, e.g., HSPD1, UCP2, and NKX2–3, were generally at low levels and were not detectable in distinct treatment groups (indicated by the purple fields in Figure 2A). Although the levels of some transcripts were increased by more than 10-fold (i.e., CASP3 in ZnEDTA-2.0 on days 21 and 32 and IL2 in Zn-EDTA-0 and Zn-EDTA-0.5 on day 32), >20-fold (IL2 in Zn-EDTA-0 on day 21), or even >70-fold (NKX2–3 in Zn-EDTA-0 on day 21) compared with those of Zn-EDTA-0 on day 11 (Figure 2B), these differential expressions were not statistically significant. Only KMT2A revealed threefold significantly elevated transcript levels in the African catfish from the B-Zn-EDTA-2.0 group on day 21 (p< 0.01) and the B-Zn-EDTA-0.5 group on day 32 (p< 0.05) versus B-Zn-EDTA-0 on day 11 and B-Zn-EDTA-0.5 on day 12 (Figures 2A, B). Altogether, the qPCR data revealed poor responses of the 17 selected genes in C. gariepinus when exposed to Zn-EDTA.
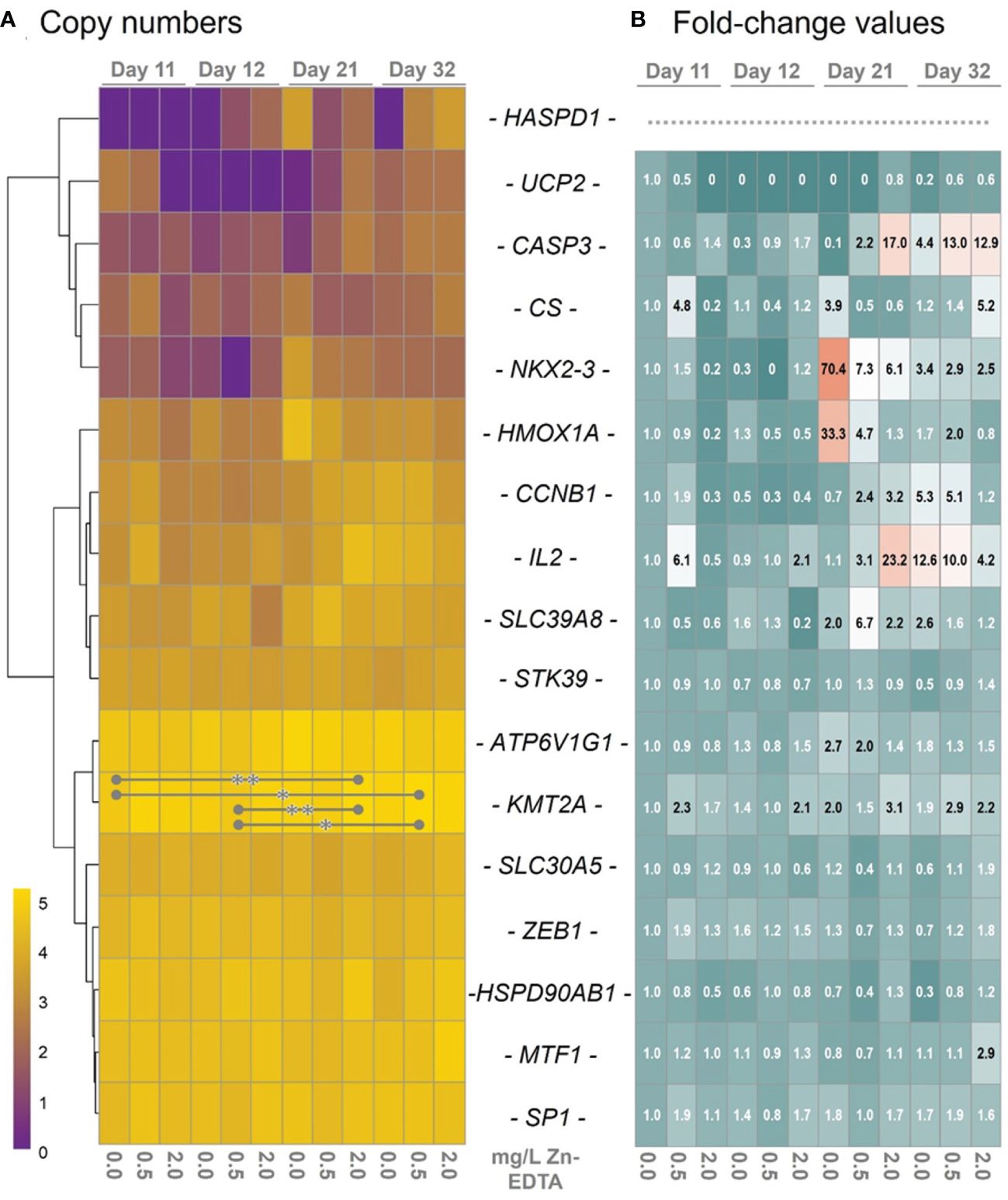
Figure 2 (A) The hierarchical cluster graph on the left depicts the log10-transformed transcript numbers averaged across the individual transcript concentrations in 10 ng pre-amplified reverse-transcribed head–kidney RNA of African catfish exposed to different Zn-EDTA concentrations (as indicated below the heatmap). The sampling time points are indicated above the diagram. The transcripts quantified are listed as gene symbols between the two heatmaps. The transcript levels are colored according to the scale. Asterisks (* p<0.05; ** p<0.01) indicate statistically different expressions between groups. (B) The heatmap on the right illustrates the linear fold change values relative to day 11 at a concentration of 0 mg Zn-EDTA (n = 3) for the genes listed between the two heatmaps. Fold change values above 2.0 were plotted in black. Note that fold change values were not calculated for the HSPD1 gene, as its transcripts were equal to 0 in the reference sample (day 11, 0 mg Zn-EDTA).
4 Discussion
Trace elements such as Zn are necessary to fertilize aquaponic systems and to make up for the lack of supply for an improved and healthy plant growth. To achieve a better understanding of their effect on fish, we examined the responses of a common aquaponic-related living fish species (C. gariepinus) to Zn-EDTA fertilization in two single life stage experiments. In terms of its water quality requirements, C. gariepinus can tolerate various parameters of water quality, including temperatures between 18°C and 32°C, with optimal temperatures for development and growth ranging from 27°C to 29°C (Păpuc et al., 2019; Xu et al., 2022; Baßmann et al., 2023). While a pH value between 5.0 and 9.0 corresponds to the profile requirements of this species (Păpuc et al., 2019; Xu et al., 2022; Baßmann et al., 2023), in aquaponics, the pH value is generally lower (approximately 6.5–7.0) to allow a more efficient bacterial activity and nutrient uptake by plants (Tyson et al., 2004). The recommended DO values range from 3.0 to 6.0 mg/L (Păpuc et al., 2019); however, higher values up to approximately 9.0 mg/L are also considered suitable (Baßmann et al., 2020; Knaus et al., 2020; Baßmann et al., 2023). The reported EC tolerance ranges from 100 to around 2,000 µS/cm (Hanika and Kramer, 2000; Tyson et al., 2004). According to Knaus and Palm (2017) and Homoki et al. (2020), an oxidative regime with a positive redox potential between +83.4 and +151.7 mV is recommended for bacterial activity in aquaponic systems and is well tolerated by the African catfish. Concentrations of NO3, NO2, and NH4(NH3) ranging up to 697.5, 0.6, and 20.46 mg/L, respectively (>0.34 mg/L) and of CO3 from >10 to<500 mg/L have been shown to still be tolerable (Molokwu and Okpokwasili, 2002; Schram et al., 2010, 2014; Roques et al., 2015; Baßmann et al., 2017; Knaus et al., 2020). In this study, the measured water temperatures ranged between 26°C and 28°C, the pH values were between 6.0 and 8.0, and the DO values varied from 6 to 8 mg/L. The EC values were measured between approximately 515 and 648 µS/cm, while the redox potentials ranged from +69 to +148 mV. Subsequently, all relevant parameters fitted the optimal or at least the adequate range for African catfish. Across both experiments, as the concentration of the fertilizer increased, the redox potential decreased, but remained in a positive (oxidative) state. Since the decrease in the redox potential in Exp. B was not linear, it can be assumed that the deactivation of the redox-active metal ions (Zn2+) through the use of an EDTA chelator had a buffering effect on the redox potential (Reddy and Patrick, 1977; Tuncay et al., 2011). The Zn concentrations were measured continuously in short intervals through a monitoring system and were maintained at predefined levels between 0.125 and 0.5 mg/L in Exp. A and between 0.5 and 2.0 mg/L in Exp. B. Although, in some cases, the treatment groups exceeded the general Zn requirement of plants in an aquaponic system (0.45 mg/L), this was not found to have any serious effects on the fish. Further monitored water chemical parameters (i.e., O2, pH, NH4, NO2, NO3, and PO4) remained unaffected by the different Zn-EDTA concentrations or appeared to be less influenced by the treatment. Although it is known that the toxic effect of heavy metals is influenced by the pH and the water hardness (Viljoen et al., 2003; Kiyani et al., 2013; Chidiebere, 2019), in the present study, values between 232 and 265 mg/L (CaCO3) were detected, corresponding to medium-hard to hard water and contributing to a low toxic effect of the metal ions.
As the growth of two African catfish life stages was assessed as additional data in this study, it should be mentioned that both experiments were not aimed at achieving the most efficient growth performance possible for the respective fish. Both experiments were conducted in aquarium systems that allowed only a limited amount of feed (nutrients), ensuring the stability of the system. Consequently, the fish in all treatment groups showed only a few grams of growth. In addition, the mortality of the African catfish life stages was determined in this study. For the African catfish fry (1.15–1.63 g), typical mortality ratios were described, ranging from 4.3% to 20.0% (Adewolu et al., 2008; Marimuthu et al., 2011); for fingerlings (102–288 g), these varied from 2.0% to 4.8% (Van de Nieuwegiessen et al., 2009; Knaus et al., 2020). As the tested lethal concentration of elemental Zn by 50% in 96 h (LC50) to the African catfish has been calculated by Ezeonyejiaku et al. (2011) to the value of 70.135 mg/L and by Chidiebere (2019) to 75.36 mg/L, the exposure concentrations of up to 2.0 mg/L in the current study showed low lethal influence on the investigated fish species. In the present study, there were low mortalities, as only one fish died inside the tank (A-ZnEDTA-0.5). A lethal effect of the various Zn concentrations could therefore not be determined since mostly fish, which died in the experiments, had escaped from their tanks.
In this study, physiological changes from exposure to Zn-EDTA, such as the distribution of leucocytes in fish, were additionally assessed for C. gariepinus fingerlings. The typical distributed leukocytes of unsexed African catfish (weighing approximately 320 g) have been described as 55.0% lymphocytes, 3.2% monocytes, and 43.4% neutrophils (Gabriel et al., 2004). Furthermore, Bello et al. (2014) described lymphocytes at 69.0%, monocytes at 3.0%, neutrophils/heterophils at 25.0%, and eosinophils at 3.0%. This study found the monocyte cell counts to be generally higher in African catfish fingerlings, while the neutrophil counts (even without Zn-EDTA) were lower compared with those in other studies. A significantly higher number of mature neutrophils in the ZnEDTA-2.0 group at the end of the experiment (after 32 days) were found along with a significant decrease in the number of meta-granulocytes between the initiation and the end of the experiment (21 days). Furthermore, the number of monocytes tended to increase over time (p< 0.1). According to Clauss et al. (2008); Havixbeck and Barreda (2015), and Havixbeck et al. (2016), a lower number of mature neutrophils in the peripheral blood together with a slight tendency to monocytosis, as observed in the present study, is suggestive of an incipient inflammatory response (day 21). As the mature neutrophil numbers decrease due to increased phagocytic activity, the proliferation and the formation of large numbers of new neutrophil meso- and metagranulocytes suggest an ongoing immune response. Increased numbers of mature neutrophils indicate the resolution of an earlier immune response as all pathogens have been eliminated (day 32). A potential inflammatory response in the current study was probably the result of severe skin lesions due to increased agonistic interactions rather than a response to the Zn concentration in the water. A distinct effect of the added Zn-EDTA is therefore questionable. Regarding the lesions observed in the African catfish, the average number of skin lesions in individual fish has been reported between 1.4 and 2.4 (Tort, 2011; Baßmann et al., 2017). In the current study, the skin lesion counts ranged from 0.39 to 1.42, which correspond to a normal range and were not influenced by the Zn treatments.
In previous studies, a broad span of different behavioral patterns of teleost fish in response to Zn exposure had been observed. These behavioral patterns include hyperactivity swimming at the surface, lethargy, and uncoordinated movements (C. gariepinus: Chidiebere, 2019); acute lethality (Scyliorhinus canicula: Crespo and Blasch, 1980); and rapid and erratic swimming, stereotypical movements, and loss of balance and general weakness with delayed reactions to light and sound (C. gariepinus: Ololade and Ogini, 2009). In the current study, significance in the behavioral alterations was found for swimming activity and air breathing in Exp. A and Exp. B (Table 4). Previous studies have described the swimming activity of aquacultured African catfish to be 74.6%–99.0% (Van de Nieuwegiessen et al., 2008, 2009) and 71.9%–91.9% (Wenzel et al., 2022); therefore, the results of the current study are in the range between lower and normal activity. The swimming activity of the B-ZnEDTA-2.0 group on day 28 was significantly lower than that on day 8, but did not differ significantly from the control fish, which were more active. Therefore, a behavioral response to Zn-EDTA exposure cannot be excluded; however, the sample size was quite small, and other factors such as the group composition may have also played a role. Studies on the influence of Zn on the respiratory system of fish have shown that Zn disrupts the Ca2+ uptake in the gills of fish and causes hypoxemia, hypercapnia, acidosis, and hypocalcemia, depending on the carbonate concentration in the water (Skidmore, 1970; Spry and Wood, 1984; Sorensen, 1991), as cited in Crafford and Avenant-Oldewage (2011) and Chidiebere (2019). Van de Nieuwegiessen et al. (2008) observed air-breathing activities (i.e., event per fish per hour) between 12.0 and 104.0 with different fish stocking densities from 10 g to around 100 g, as well as activities between 80.0 and 83.0 with fish stocking densities from 102 to around 288 g (Van de Nieuwegiessen et al., 2009). In this study, a significant increase in air-breathing activity was found in the A-ZnEDTA-0.5 group between the start and the end of the experiment. As the effect also appeared in the control group in Exp. A and no similar effects were observed in Exp. B, a possible influence of the Zn concentration on this behavior pattern is questionable.
The dissected organs for histological microscopy analysis of C. gariepinus fingerlings revealed small alterations upon exposure to Zn-EDTA. Studies that examined the toxic effects of various concentrations of elemental Zn in different salts (ZnSO4 or ZnCl2) on O. mykiss, Tilapia sparmanii, or C. gariepinus revealed alterations in the epithelium (Lloyd, 1960; Skidmore, 1970; Hogstrand et al., 2002) and in the functionality of the gills (Burton et al., 1972; Mallatt, 1985; Van Rensbrug, 1989; Wood, 2017), as well as accumulations in different organs such as the gill, liver, muscle, and skin (Awoyemi et al., 2014; Duran et al., 2015). Later, using ICP-MS analysis, the combined effects and influence of Zn and Zn-EDTA (in concentrations from 4.0 to 8.0 mg/L) on C. carpio were investigated simultaneously by Karayakar et al. (2021) for more than 7 days of exposure. Their results revealed accumulations of Zn and Zn-EDTA in the tissues of the liver and gill at different uptake ratios. Moreover, they found a decreased ratio of Zn accumulation in tissue when the EDTA chelating agent was present in the treatment. In carp, the highest concentrations of Zn and Zn-EDTA were accordingly detected in the gills, as this organ is the most exposed to the environment of a fish (Chavan and Muley, 2014). Other studies investigated the toxic effects of Zn-EDTA on Palaemon elegans (Nugegoda and Rainbow, 1988). The authors described low Zn uptake in the presence of EDTA, as the chelator had a 1:1 molar binding ratio to Zn2+ and therefore reduced the amount of bioavailable Zn2+ ions (Nugegoda and Rainbow, 1988). Accordingly, a higher regulation ability of the Zn household was concluded. As metal components are taken up primarily by the fish liver, invading metal ions are therefore transformed into metallothionein proteins (Cherian and Nordberg, 1983; Shukla et al., 2007; Rose et al., 2014). These protein–metal complexes may be water-insoluble and become sequestrated in lipid or fatty tissues, where they accumulate (Montaser et al., 2010; Awoyemi et al., 2014). The polar property of the chelator and its ligands, in order to shift through a membrane and become a metal–protein complex, appears to play a key role in the accumulation process. Hydrophilic metal complexes (Zn-EDTA) do not appear to be able to cross the hydrophobic cell membrane, as it is negatively charged and lipophobic (Nugegoda and Rainbow, 1988). Unlike lipophobic complexes, lipophilic complexes (such as Fe-DTPA) are able to cross these cell membranes and start to accumulate (Asik et al., 2020; Hildebrand et al., 2023). Our data documented low effects of the Zn-EDTA treatment in the liver and moderate effects in the gill tissues based on the staining and microscopy results. As only a light coloration was observed in the liver tissue at a concentration of 2.0 mg/L Zn-EDTA after 32 days of exposure, the accumulation process of Zn-EDTA in the liver tissue of the investigated species could not be verified at a quantitative level using the staining method. Therefore, further investigations on the liver and gill tissues of C. gariepinus are recommend using advanced methods such as atomic absorption spectrophotometry (AAS) or inductively coupled plasma (ICP) analysis in order to verify and assess the accumulation process in this context more precisely. However, the present results are consistent with the findings and expectations of Nugegoda and Rainbow (1988) and Karayakar et al. (2021), who assumed and found a lower accumulation potential of Zn alone and in combination with EDTA chelating agents. In addition, this study emphasized the low toxicity of Zn-EDTA to fish internal organs, which would be expected to be much higher when using elemental Zn chloride or sulfate compounds for exposure (Awoyemi et al., 2014; Duran et al., 2015; Karayakar et al., 2021). As the major effects on the investigated organs in this study were primarily observed in the gill tissues, the present results of the grading system used (G0–G2, unremarkable to mild severity) were therefore based on the morphological alterations of the gill epithelium, which had been previously described by Lloyd (1960), Skidmore (1970), Zheng et al. (2011), and Srivatava and Prakash (2019), but did not have a significant effect on the respiratory system or the behavior.
Profiling of the genetic primers used for the African catfish, which have been established in a previous experiment (Hildebrand et al., 2023) through the catfish head kidney (Table 1), showed the significantly different expression of KMT2A dependent on the exposure time and the Zn-EDTA concentration. KMT2A encodes lysine methyltransferase 2A, which is involved in the early phase of hematopoiesis and in wound healing (Huang et al., 2015; Zhang et al., 2017; Davis et al., 2019), as well as in the development of granulocytes (Fujiki et al., 2011; Ichikawa et al., 2021). Based on the above-mentioned observations, we assumed that the increased granulocyte counts that occurred at later time points in our experiments correlate with the observed elevated KMT2A transcript levels. Furthermore, inconspicuous genes such as MTF1 were similarly expressed through the treatments. As MTF1 is involved in the production of metal-binding metallothionein (Wimmer et al., 2005; Günther et al., 2012), its homogeneous expression across the various Zn-EDTA concentration groups indicates matching Zn families in the examined individuals and a protective effect of Zn-EDTA on the fish organs.
In the aquaculture of African catfish, the process water can reach Zn concentrations between 0.334 and 0.413 mg/L (Strauch et al., 2018). These concentrations are close to those used in hydroponic plant production (0.45 mg/L) (Bittsanszky et al., 2016). In addition to the fact that the Zn in the process water is derived from compounds (such as ZnO, ZnCl, and ZnSO4) in the fish diet, it is not clear whether the Zn compounds in the recirculating water could cause problems for the fish at higher concentrations (Duran et al., 2015) or are harmless to their health. Moreover, it is not known whether additional Zn-EDTA in the process water positively affects the Zn family in the fish or the dissociation equilibrium of the Zn compounds in the systems when the percentage feed intake is higher than that of industry standards. In the present study, the tested Zn-EDTA concentrations of up to 0.5 mg/L did not have any severe effects either to the fry or to the fingerlings at feed intakes between 0.7 and 3.0% bodyweight per day. Furthermore, the tested Zn-EDTA concentrations were most likely not intense enough to trigger the induction of the various relevant genes selected for the current study, although the possibility that the expression of several other genes not included as target genes in the present analysis may well respond to Zn-EDTA exposure could not be ruled out. Only the 2.0 mg/L concentration revealed negative effects, including the Zn accumulation in the liver tissue in the fingerling group, demonstrating a potential overdose. Consequently, the fertilizer ratio for younger fish should be adjusted according to the supplementation process in order to not exceed the maximum Zn concentration in the system. Since the tested concentration of 0.5 mg/L Zn-EDTA in our study did not harm the fish, we support the supplementation of Zn-EDTA to African catfish up to this concentration level. This also allows the supplementation in coupled aquaponics.
5 Conclusions
The growth and mortality of African catfish were not affected by Zn-EDTA at the tested concentrations up to 2.0 mg/L in C. gariepinus fry and fingerlings. The ethological analysis also showed no behavioral dependence on Zn-EDTA treatment. Since only minor histomorphological changes in the gill tissue occurred, especially in treatments with 0.5 and 2.0 mg/L Zn-EDTA, and the fertilization recommendation for Zn in hydroponics is 0.45 mg/L, we do not recommend an addition to aquaponics with African catfish above 0.5 mg/L. Furthermore, long-term studies should clarify whether there can also be a stronger influence on or damage to the animals in the case of a prolonged exposure. Our study revealed new opportunities for the careful use of fertilizers such as Zn-EDTA for a more effective, sustainable water management for entrepreneurs with aquaponic systems. Accordingly, we can now confirm, thus far, the relatively harmless potential of Zn-EDTA as a necessary trace element for plants and its toxic potential to the African catfish (C. gariepinus) to ensure better plant growth without harming the cultivated fish species at the intended concentration of 0.45 mg/L.
Data availability statement
The original contributions presented in the study are included in the article/supplementary material. Further inquiries can be directed to the corresponding author.
Ethics statement
The animal study was approved by Landesamt für Landwirtschaft, Lebensmittelsicherheitund Fischerei Mecklenburg-Vorpommern - Veterinärdienste und Landwirtschaft. The study was conducted in accordance with the local legislation and institutional requirements.
Author contributions
M-CH: Conceptualization, Data curation, Formal analysis, Investigation, Methodology, Resources, Validation, Visualization, Writing – original draft, Writing – review & editing. AR: Investigation, Methodology, Resources, Validation, Visualization, Writing – original draft, Writing – review & editing. TG: Investigation, Writing – original draft. HP: Funding acquisition, Supervision, Writing – review & editing. BB: Conceptualization, Investigation, Methodology, Project administration, Supervision, Validation, Writing – original draft, Writing – review & editing.
Funding
The author(s) declare financial support was received for the research, authorship, and/or publication of this article. This study was funded by the project “Performance and process water management in commercial (integrated) aquaculture systems with African catfish (Clarias gariepinus) in Mecklenburg-Western Pomerania” (MV-II.1-LM-007: 139030000103, EMFF: 7302).
Acknowledgments
We thank Markus Kipp and Frauke Winzer (Institute for Anatomy, University of Rostock) for histological sample preparation and assistance as well as Julian Krinitskij (FBN) for his assistance with the gene-expression profiling. We thank Leopold Hummel, Lisa Carolina Wenzel, Lu Xu, and Laura Ballesteros Redondo (Department of Aquaculture and Sea-Ranching, University of Rostock) for their assistance during the samplings.
Conflict of interest
The authors declare that the research was conducted in the absence of any commercial or financial relationships that could be construed as a potential conflict of interest.
Publisher’s note
All claims expressed in this article are solely those of the authors and do not necessarily represent those of their affiliated organizations, or those of the publisher, the editors and the reviewers. Any product that may be evaluated in this article, or claim that may be made by its manufacturer, is not guaranteed or endorsed by the publisher.
References
Abdel-Tawwab M. (2016). Effect of feed availability on susceptibility of Nile tilapia, Oreochromis niloticus (L.) to environmental zinc toxicity: Growth performance, biochemical response, and zinc bioaccumulation. Aquaculture 464, 309–315. doi: 10.1016/j.aquaculture.2016.07.009
Abdel-Tawwab M., El-Sayed G. O., Shady S. H. (2012). Effects of dietary protein levels and environmental zinc exposure on the growth, feed utilization, and biochemical variables of Nile tilapia, Oreochromis niloticus (L.). Toxicological Environ. Chem. 94, 1368–1382. doi: 10.1080/02772248.2012.703202
Adewolu M. A., Adeniji C. A., Adejobi A. B. (2008). Feed utilization, growth and survival of Clarias gariepinus (Burchell 1822) fingerlings cultured under different photoperiods. Aquaculture 283, 64–67. doi: 10.1016/j.aquaculture.2008.07.020
Ajima M. N. O., Ogo O. A., Akpa L. E., Ajaero I. (2015). Biochemical and haematological responses in African catfish Clarias gariepinus following chronic exposure to NPK (15: 15: 15) fertiliser. Afr. J. Aquat. Sci. 40, 73–79. doi: 10.2989/16085914.2015.1020046
Alloway B. J. (2008). Zinc in soils and crop nutrition Vol. 139 (Brussels, Belgium and Paris, France: IZA and IFA), 9–10.
Asik D., Smolinski R., Abozeid S. M., Mitchell T. B., Turowski S. G., Spernyak J. A., et al. (2020). Modulating the properties of Fe (III) macrocyclic MRI contrast agents by appending sulfonate or hydroxyl groups. Molecules 25, 2291. doi: 10.3390/molecules25102291
Awoyemi O. M., Bawa-Allah K. A., Otitoloju A. A. (2014). Accumulation and anti-oxidant enzymes as biomarkers of heavy metal exposure in Clarias gariepinus and Oreochromis niloticus. Appl. Ecol. Environ. Sci. 2, 114–122. doi: 10.12691/aees-2–5-2
Baßmann B., Brenner M., Palm H. W. (2017). ). Stress and welfare of African catfish (Clarias gariepinus Burchell 1822) in a coupled aquaponic system. Water 9, 504. doi: 10.3390/w9070504
Baßmann B., Hahn L., Rebl A., Wenzel L. C., Hildebrand M. C., Verleih M., et al. (2023). Effects of stocking density, size, and external stress on growth and welfare of african catfish (Clarias gariepinus Burchell 1822) in a commercial RAS. Fishes 8, 74. doi: 10.3390/fishes8020074
Baßmann B., Harbach H., Weißbach S., Palm H. W. (2020). Effect of plant density in coupled aquaponics on the welfare status of African catfish, Clarias gariepinus. J. World Aquaculture Soc. 51, 183–199. doi: 10.1111/jwas.12574
Bello O. S., Olaifa F. E., Emikpe B. O. (2014). Haematological and blood biochemical changes in African catfish, Clarias gariepinus fed walnut (Tetracarpidium conophorum Mull Arg) leaf and onion (Allium cepa Linn) bulb supplemented diets. Am. J. Exp. Agric. 4, 1593–1603. doi: 10.9734/AJEA
Bengari K. V., Patil H. S. (1986). Respiration, liver glycogen and bioaccumulation in Labeo rohita exposed to zinc. Ind. J. Comp. Anim. Physiol. 4, 79–84.
Bittsanszky A., Uzinger N., Gyulai G., Mathis A., Junge R., Villarroel M., et al. (2016). Nutrient supply of plants in aquaponic systems. Ecocycles 2, 17–20. doi: 10.19040/ecocycles.v2i2.57
Brungs W. A. (1969). Chronic toxicity of zinc to the fathead minnow, Pimephales promelas Rafinesque. Trans. Am. Fish. Soc 2, 272–279. doi: 10.1577/1548-8659(1969)98[272:CTOZTT]2.0.CO;2
Burton D. T., Jones A. H., Cairns J. (1972). Acute zinc toxicity to rainbow trout (Salmo gairdneri): confirmation of the hypothesis that death is related to tissue hypoxia. J. Fish. Res. Board Can. 29, 1463–1466. doi: 10.1139/f72-225
Chavan V. R., Muley D. V. (2014). Effect of heavy metals on liver and gill of fish Cirrhinus mrigala. Int. J. Curr. Microbiol. Appl. Sci. 3, 277–288. doi: 10.28978/nesciences.868065
Cherian M. G., Nordberg M. (1983). Cellular adaptation in metal toxicology and metallothionein. Toxicology 28, 1–15. doi: 10.1016/0300-483X(83)90101-4
Chidiebere E. E. (2019). Acute toxicity of copper and zinc and their lethal concentration on Clarias gariepinus (cat fish). Biomed. J. Sci. Tech. Res. 17, 13160–13166. doi: 10.26717/BJSTR.2019.17.003076
Clauss T. M., Dove A. D., Arnold J. E. (2008). Hematologic disorders of fish. Veterinary Clinics North America: Exotic Anim. Pract. 11, 445–462. doi: 10.1016/j.cvex.2008.03.007
Coetzee L., Du Preez H. H., Van Vuren J. H. J. (2002). Metal concentrations in Clarias gariepinus and Labeo umbratus from the Olifants and Klein Olifants River, Mpumalanga, South Africa: Zinc, copper, manganese, lead, chromium, nickel, aluminium and iron. Water SA 28, 433–448. doi: 10.4314/wsa.v28i4.4917
Crafford D., Avenant-Oldewage A. (2011). Uptake of selected metals in tissues and organs of Clarias gariepinus (sharptooth catfish) from the Vaal River System–Chromium, copper, iron, manganese and zinc. Water SA 37 (2), 187–366. doi: 10.4314/wsa.v37i2.65864
Crespo S., Blasch J. (1980). Mortality, accumulation, and distribution of zinc in the gill system of the dogfish following zinc treatment. Bull. Environ. Contam. Toxicol. (United States) 24, 940–944. doi: 10.1007/BF01608215
Da Silva Cerozi B. (2020). Fulvic acid increases iron bioavailability in aquaponic systems: theoretical designs and practical considerations to prevent iron deficiency in plants. Aquacultural Eng. 90, 102091. doi: 10.1016/j.aquaeng.2020.102091
Davis F. M., Kimball A., den Dekker A., Joshi A. D., Boniakowski A. E., Nysz D., et al. (2019). Histone methylation directs myeloid TLR4 expression and regulates wound healing following cutaneous tissue injury. J. Immunol. 202, 1777–1785. doi: 10.4049/jimmunol.1801258
Duran S., Erdem C. (2014). Effects of sublethal concentration of copper, zinc and cadmium, applied singly and in mixture, on some hematological parameters of Oreochromis niloticus (L. 1758). Fresen Environ. Bull. 22, 2977–2980.
Duran S., Tunçsoy M., Yeşilbudak B., Ay Ö., Cicik B., Erdem C. (2015). Metal accumulation in various tissues of Clarias gariepinus exposed to copper, zinc, cadmium and lead singly and in mixture. Fresenius Environ. Bull. 24, 4738–4742.
Eisler R. (1993). Zinc hazards to fish, wildlife, and invertebrates: a synoptic review. Biological Report Fish and Wildlife Service, Report No. 10 (Washington DC, USA: US Department of Interior), 45.
Ezeonyejiaku C. D., Obiakor M. O., Ezenwelu C. O. (2011). Toxicity of copper sulphate and behavioral locomotor response of tilapia (Oreochromis niloticus) and catfish (Clarias gariepinus) species. Online J. Anim. Feed Res. 1, 130–134. doi: 10.5555/20123128298
Ezeonyejiaku C. D., Obiakor M. O., Ezenwelu C. O., Ugochukwu G. C. (2010). Lethal influence of zinc exposure to Clarias gariepinus (Burchell 1822, pisces, clariidae). World J. Fish Mar. Sci. 2, 455–460.
Ezeonyejiaku C. D., Obiakor M. O., Ezenwelu C. O., Ugochukwu G. C. (2012). Lethal influence of zinc exposure to Clarias gariepinus (Burchell 1822, pisces, clariidae). J. Anim. Sci. Adv. 2, 177–183.
Firat O., Kargin F. (2010). Individual and combined effects of heavy metals on serum biochemistry of Nile tilapia Oreochromis niloticus. Arch. Environ. Contam. Toxicol. 58, 151–157. doi: 10.1007/s00244-009-9344-5
Fujiki R., Chikanishi T., Hashiba W., Ito H., Takada I., Roeder R. G., et al. (2011). GlcNAcylation of a histone methyltransferase in retinoic-acid-induced granulopoiesis. Nature 480, 557. doi: 10.1038/nature07954
Gabriel U. U., Ezeri G. N. O., Opabunmi O. O. (2004). Influence of sex, source, health status and acclimation on the haematology of Clarias gariepinus (Burch 1822). Afr. J. Biotechnol. 3, 463–467. doi: 10.5897/AJB
Gatlin D. M., Phillips H. F., Torrans E. L. (1989). Effects of various levels of dietary copper and zinc on channel catfish. Aquaculture 76, 127–134. doi: 10.1016/0044-8486(89)90257-3
Goddek S., Joyce A., Kotzen B., Burnell G. M. (2019). Aquaponics food production systems: combined aquaculture and hydroponic production technologies for the future (Switzerland: Springer Nature), 619. doi: 10.1007/978–3-030–15943-6
Günther V., Lindert U., Schaffner W. (2012). The taste of heavy metals: gene regulation by MTF-1. Biochim. Biophys. Acta (BBA)-Molecular Cell Res. 1823, 1416–1425. doi: 10.1016/j.bbamcr.2012.01.005
Hanika S., Kramer B. (2000). Electrosensory prey detection in the African sharptooth catfish, Clarias gariepinus (Clariidae), of a weakly electric mormyrid fish, the bulldog (Marcusenius macrolepidotus). Behav. Ecol. Sociobiol. 48, 218–228. doi: 10.1007/s002650000232
Havixbeck J. J., Barreda D. R. (2015). Neutrophil development, migration, and function in teleost fish. Biology 4, 715–734. doi: 10.3390/biology4040715
Havixbeck J. J., Rieger A. M., Wong M. E., Hodgkinson J. W., Barreda D. R. (2016). Neutrophil contributions to the induction and regulation of the acute inflammatory response in teleost fish. J. Leucocyte Biol. 99, 241–252. doi: 10.1189/jlb.3HI0215-064R
Heath A. G. (1995). Osmotic and ionic regulation. Water pollut. Fish Physiol. 19, 107–131. doi: 10.1201/9780203718896-7
Hildebrand M. C., Rebl A., Nguinkal J. A., Palm H. W., Baßmann B. (2023). Effects of fe-DTPA on health and welfare of the african catfish clarias gariepinus (Burchell 1822). Water 15, 299. doi: 10.3390/w15020299
Hockett J. R., Mount D. R. (1996). Use of metal chelating agents to differentiate among sources of acute aquatic toxicity. Environ. Toxicol. Chem. 15 (10), 1687–1693. doi: 10.1002/etc.5620151006
Hogstrand C., Balesaria S., Glover C. N. (2002). Application of genomics and proteomics for study of the integrated response to zinc exposure in a non-model fish species, the rainbow trout. Comp. Biochem. Physiol. Part B: Biochem. Mol. Biol. 133, 523–535. doi: 10.1016/S1096-4959(02)00125-2
Homoki D., Minya D., Kovács L., Molnár Á., Balogh K., Bársony P., et al. (2020). Comparison of the technological background of aquaponic systems. Acta Agraria Debreceniensis 1), 47–52. doi: 10.34101/actaagrar/1/4511
Huang Y. C., Shih H. Y., Lin S. J., Chiu C. C., Ma T. L., Yeh T. H., et al. (2015). The epigenetic factor Kmt2a/Mll1 regulates neural progenitor proliferation and neuronal and glial differentiation. Dev. Neurobiol. 75, 452–462. doi: 10.1002/dneu.22235
Ichikawa S., Fujiwara T., Saito K., Fukuhara N., Yokoyama H., Hatta S., et al. (2021). A novel case of γδ T cell leukemia with recurrent genetic abnormalities accompanied by agranulocytosis. Ann. Hematol. 100, 2665–2668. doi: 10.1007/s00277-020-04241-w
Johnson R., Wolf J., Braunbeck T. (2009). OECD guidance document for the diagnosis of endocrine-related histopathology of fish gonads Vol. 96 (Paris: Organization for Economic Co-operation and Development).
Kaliky N. A. P. S. B., Setiawati M., Carman O., Utomo N. B. P. (2019). Effect of zinc (Zn) supplementation on quality and quantity of striped catfish Pangasianodon hypophthalmus sperm. Jurnal Akuakultur Indonesia 18, 46–53. doi: 10.19027/jai.18.1.46-53
Karayakar F., Seçen F., Cicik B. (2021). The effects of EDTA on zinc accumulation in tissues of Cyprinus carpio (Linnaeus 1758). Natural Eng. Sci. 6, 30–38. doi: 10.28978/nesciences.868065
Kasozi N., Tandlich R., Fick M., Kaiser H., Wilhelmi B. (2019). Iron supplementation and management in aquaponic systems: a review. Aquaculture Rep. 15, 100221. doi: 10.1016/j.aqrep.2019.100221
Kiyani V., Hosynzadeh M., Ebrahimpour M. (2013). Investigation acute toxicity some of heavy metals at different water hardness. Int. J. Advanced Biol. Biomed. Res. 1, 134–142.
Knaus U., Palm H. W. (2017). Effects of the fish species choice on vegetables in aquaponics under spring-summer conditions in northern Germany (Mecklenburg Western Pomerania). Aquaculture 473, 62–73. doi: 10.1016/j.aquaculture.2017.01.020
Knaus U., Wenzel L. C., Appelbaum S., Palm H. W. (2020). Aquaponics (sl) Production of spearmint (Mentha spicata) with African catfish (Clarias gariepinus) in Northern Germany. Sustainability 12, 8717. doi: 10.3390/su12208717
Krishnasamy K., Nair J., Bäuml B. (2012). Hydroponic system for the treatment of anaerobic liquid. Water Sci. Technol. 65, 1164–1171. doi: 10.2166/wst.2012.031
Lewis S. D., Lewis W. M. (1971). The effect of zinc and copper on the osmolality of blood serum of the channel catfish, Ictalurus punctatus Rafinesque, and golden shiner, Notemigonus crysoleucas Mitchill. Trans. Am. Fish. Soc 4, 639–643. doi: 10.1577/1548–8659(1971)100<639:TEOZAC>2.0.CO;2
Linnik P. N., Ignatenko I. I. (2015). Molybdenum in natural surface waters: content and forms of occurrence (a review). Hydrobiological J. 51, 13. doi: 10.1615/HydrobJ.v51.i4.100
Lloyd R. (1960). The toxicity of zinc sulphate to rainbow trout. Ann. Appl. Biol. 48, 84–94. doi: 10.1111/j.1744-7348.1960.tb03507.x
Mahboub H. H., Shahin K., Zaglool A. W., Roushdy E. M., Ahmed S. A. (2020). Efficacy of nano zinc oxide dietary supplements on growth performance, immunomodulation and disease resistance of African catfish Clarias gariepinus. Dis. Aquat. Organisms 142, 147–160. doi: 10.3354/dao03531
Mallatt J. (1985). Fish gill structural changes induced by toxicants and other irritants: a statistical review. Can. J. Fisheries Aquat. Sci. 42, 630–648. doi: 10.1139/f85-083
Marimuthu K., Umah R., Muralikrishnan S., Xavier R., Kathiresan S. (2011). Effect of different feed application rate on growth, survival and cannibalism of African catfish, Clarias gariepinus fingerlings. Emirates J. Food Agric. 23, 330–337.
Midorikawa O., Eder M. (1962). Vergleichende histochemische Untersuchungen über Zink im Darm. Z. für Zellforschung und mikroskopische Anatomie Abt. Histochemie 2, 444–472. doi: 10.1007/BF00736501
Molokwu C. N., Okpokwasili G. C. (2002). Effect of water hardness on egg hatchability and larval viability of Clarias gariepinus. Aquaculture Int. 10, 57–64. doi: 10.1023/A:1021395122919
Montaser M., Mahfouz M. E., El-Shazly S. A., Abdel-Rahman G. H., Bakry S. (2010). Toxicity of heavy metals on fish at Jeddah coast KSA: Metallothionein expression as a biomarker and histopathological study on liver and gills. World J. Fish Mar. Sci. 2, 174–185.
Mulisch M. (2015). “Präparation für die konventionelle Rasterelektronenmikroskopie (REM),” in Romeis-Mikroskopische Technik (Springer Spektrum, Berlin, Heidelberg), 145–152. doi: 10.1007/978–3-642–55190-1_8
Neocleous D., Nikolaou G., Ntatsi G., Savvas D. (2020). Impact of chelated or inorganic manganese and zinc applications in closed hydroponic bean crops on growth, yield, photosynthesis, and nutrient uptake. Agronomy 10, 881. doi: 10.3390/agronomy10060881
Niyogi S., Wood C. M. (2006). Interaction between dietary calcium supplementation and chronic waterborne zinc exposure in juvenile rainbow trout, Oncorhynchus mykiss. Comp. Biochem. Physiol. Part C: Toxicol. Pharmacol. 143, 94–102. doi: 10.1016/j.cbpc.2005.12.007
Nugegoda D., Rainbow P. S. (1988). Effect of a chelating agent (EDTA) on zinc uptake and regulation by Palaemon elegans (Crustacea: Decapoda). J. Mar. Biol. Assoc. United Kingdom 68, 25–40. doi: 10.1017/S0025315400050074
Ololade I. A., Ogini O. (2009). Behavioural and hematological effects of zinc on African catfish, Clarias gariepinus. Int. J. Fisheries Aquaculture 1, 22–27.
Onuegbu C. U., Aggarwal A., Singh N. B. (2018). ZnO nano - particles as feed supplement on growth performance of cultured African catfish fingerlings. J. Sci. Ind. Res. 77, 213–218.
Păpuc T., Petrescu-Mag I. V., Gavriloaie C., Botha M., Kovacs E., Coroian C. O. (2019). Swimming in the mud-a short review of environmental parameter ranges tolerated by Clarias gariepinus. Extreme Life Biospeol. Astrobiol. 11, 9–17.
Rakocy J. E. (2012). Aquaponics—integrating fish and plant culture. Aquaculture production Syst., 344–386.
Reddy C. N., Patrick W. H. Jr. (1977). Effect of redox potential on the stability of zinc and copper chelates in flooded soils. Soil Sci. Soc. America J. 41, 729–732. doi: 10.2136/sssaj1977.03615995004100040023x
Ricker W. E. (1973). Linear regressions in fishery research. J. fisheries board Canada 30, 409–434. doi: 10.1139/f73-072
Roméo M., Siau Y., Sidoumou Z., Gnassia-Barelli M. (1999). Heavy metal distribution in different fish species from the Mauritania coast. Sci. total Environ. 232, 169–175. doi: 10.1016/S0048-9697(99)00099-6
Roques J. A., Schram E., Spanings T., van Schaik T., Abbink W., Boerrigter J., et al. (2015). The impact of elevated water nitrite con-centration on physiology, growth and feed intake of African catfish Clarias gariepinus (Burchell 1822). Aquaculture Res. 46, 1384–1395. doi: 10.1111/are.2015.46.issue-6
Rose S., Vincent S., Meena B., Suresh A., Mani R. (2014). Metallothionein induction in fresh water catfish Clarias gariepinus on exposure to cadmium. Int. J. Pharm. Pharm. Sci. 6, 377–383.
Rümke C. L., Klein Z. (1987). Statistische Betrachtungen über die Genauigkeit der Differenzierung und der Zählung von Leukozyten. Z Klin Med. 42, 173.
Schram E., Roques J. A., Abbink W., Spanings T., De Vries P., Bierman S., et al. (2010). The impact of elevated water ammonia concentration on physiology, growth and feed intake of African catfish (Clarias gariepinus). Aquaculture 306, 108–115. doi: 10.1016/j.aquaculture.2010.06.005
Schram E., Roques J. A., Abbink W., Yokohama Y., Spanings T., de Vries P., et al. (2014). The impact of elevated water nitrate concentration on physiology, growth and feed intake of African catfish Clarias gariepinus (Burchell 1822). Aquaculture Res. 45, 1499–1511. doi: 10.1111/are.12098
Seibel H., Baßmann B., Rebl A. (2021). Blood will tell: what hematological analyses can reveal about fish welfare. Front. Veterinary Sci. 8. doi: 10.3389/fvets.2021.616955
Shuhaimi-Othman M., Yakub N., Ramle N. A., Abas A. (2015). Comparative toxicity of eight metals on freshwater fish. Toxicol. Ind. Health 31, 773–782. doi: 10.1177/0748233712472519
Shukla V., Dhankhar M., Prakash J., Sastry K. V. (2007). Bioaccumulation of Zn, Cu and cd in Channa punctatus. J. Environ. Biol. 28, 395.
Skidmore J. (1970). Respiration and osmoregulation in rainbow trout with gills damaged by zinc sulphate. J. Exp. Biol. 52, 481–494. doi: 10.1242/jeb.52.2.481
Sonneveld C., Voogt W., Sonneveld C., Voogt W. (2009). Plant nutrition in future greenhouse production (Dordrecht: Springer Netherlands), 393–403. doi: 10.1007/978–90-481–2532-6_17
Spry D. J., Wood C. M. (1984). Acid-base, plasma ion and blood gas changes in rainbow trout during short term toxic zinc exposure. J. Comp. Physiol. B 154, 149–158. doi: 10.1007/BF00684139
Srivatava N. K., Prakash S. (2019). Effect of zinc on the histopathology of gill, liver and kidney of fresh water catfish, clarias batrachus (Linn.). Int. J. Biol. Innov. 1, 8–13. doi: 10.46505/IJBI.2019.1102
Stathopoulou P., Tsoumalakou E., Levizou E., Vanikiotis T., Zaoutsos S., Berillis P. (2021). Iron and potassium fertilization improve rocket growth without affecting tilapia growth and histomorphology characteristics in aquaponics. Appl. Sci. 11, 5681. doi: 10.3390/app11125681
Strauch S. M., Wenzel L. C., Bischoff A., Dellwig O., Klein J., Schüch A., et al. (2018). Commercial African catfish (Clarias gariepinus) recirculating aquaculture systems: Assessment of element and energy pathways with special focus on the phosphorus cycle. Sustainability 10, 1805. doi: 10.3390/su10061805
Tort L. (2011). Stress and immune modulation in fish. Dev. Comp. Immunol. 35, 1366–1375. doi: 10.1016/j.dci.2011.07.002
Tuncay E., Bilginoglu A., Sozmen N. N., Zeydanli E. N., Ugur M., Vassort G., et al. (2011). Intracellular free zinc during cardiac excitation–contraction cycle: calcium and redox dependencies. Cardiovasc. Res. 89, 634–642. doi: 10.1093/cvr/cvq352
Tunçsoy M., Erdem C. (2014). Accumulation of copper, zinc and cadmium in liver, gill and muscle tissues of Oreochromis niloticus exposed to these metals separately and in mixture. Fresenius Environ. Bull. 23, 1143–1149.
Tyson R. V., Simonne E. H., White J. M., Lamb E. M. (2004). Reconciling water quality parameters impacting nitrification in aquaponics: the pH levels. Proc. Florida State Hortic. Soc. 117, 79–83.
Van de Nieuwegiessen P. G., Boerlage A. S., Verreth J. A., Schrama J. W. (2008). Assessing the effects of a chronic stressor, stocking density, on welfare indicators of juvenile African catfish, Clarias gariepinus Burchell. Appl. Anim. Behav. Sci. 115, 233–243. doi: 10.1016/j.applanim.2008.05.008
Van de Nieuwegiessen P. G., Olwo J., Khong S., Verreth J. A., Schrama J. W. (2009). Effects of age and stocking density on the welfare of African catfish, Clarias gariepinus Burchell. Aquaculture 288, 69–75. doi: 10.1016/j.aquaculture.2008.11.009
Van Rensbrug E. L. (1989). Die Biokonsentrering van Artrasien, Sink en Yster in Tilapia sparmanii (Cichlidae). Ongepubliseerde M.Sc. Verhandeling (Suid Afrika: Rand. Afr. Univ.), 74–105.
Viljoen A., Steyn G. J., Van Vuren J. H. J., Wade P. W. (2003). Zinc effects on the embryos and larvae of the sharptooth catfish, Claias gariepinus (Burchell, 1822). Bull. Environ. Contam Toxicol. 70, 1022–1027. doi: 10.1007/s00128–003-0085–2
Watanabe T., Kiron V., Satoh S. (1997). Trace minerals in fish nutrition. Aquaculture 151, 185–207. doi: 10.1016/S0044-8486(96)01503-7
Wenzel L. C., Berchtold E., Palm H. W. (2022). Effects of stocking density and grading on behaviour, cannibalism and performance of African catfish (Clarias gariepinus) fry. Aquaculture Rep. 27, 101400. doi: 10.1016/j.aqrep.2022.101400
Wenzel L. C., Strauch S. M., Eding E., Presas-Basalo F. X., Wasenitz B., Palm H. W. (2021). Effects of dissolved potassium on growth performance, body composition, and welfare of juvenile african catfish (Clarias gariepinus). Fishes 6, 11. doi: 10.3390/fishes6020011
Wimmer U., Wang Y., Georgiev O., Schaffner W. (2005). Two major branches of anti-cadmium defense in the mouse: MTF-1/metallothioneins and glutathione. Nucleic Acids Res. 33, 5715–5727. doi: 10.1093/nar/gki881
Wood C. M. (2017). “Toxic responses of the gill,” in Target organ toxicity in marine and freshwater teleosts (London: CRC Press), 1–89.
Xu L., Ziethen C. J., Appelbaum S., Palm H. W., Knaus U. (2022). Aquaponics Production of Wheatgrass (Triticum aestivum L.) in Different Horticultural Substrates with African Catfish (Clarias gariepinus) in Northern Germany. AgriEngineering 4, 1076–1094. doi: 10.3390/agriengineering4040067
Yang T., Kim H. J. (2019). Nutrient management regime affects water quality, crop growth, and nitrogen use efficiency of aquaponic systems. Scientia Hortic. 256, 108619. doi: 10.1016/j.scienta.2019.108619
Yep B., Zheng Y. (2020). Potassium and micronutrient fertilizer addition in a mock aquaponic system for drug-type Cannabis sativa L. cultivation. Can. J. Plant Sci. 101, 341–352. doi: 10.1139/cjps-2020-0107
Zhang C., Song C., Liu T., Tang R., Chen M., Gao F., et al. (2017). KMT2A promotes melanoma cell growth by targeting hTERT signaling pathway. Cell Death Dis. 8, e2940–e2940. doi: 10.1038/cddis.2017.285
Zheng D., Kille P., Feeney G. P., Cunningham P., Handy R. D., Hogstrand C. (2010). Dynamic transcriptomic profiles of zebrafish gills in response to zinc supplementation. BMC Genomics 11, 1–19. doi: 10.1186/1471-2164-11-553
Keywords: aquaponics, fertilizer, fish histopathology, trace elements, toxicity
Citation: Hildebrand M-C, Rebl A, Goldammer T, Palm HW and Baßmann B (2024) Effects of Zn-EDTA on the health and welfare of the African catfish, Clarias gariepinus (Burchell, 1822), in a recirculating aquaculture system. Front. Aquac. 3:1383534. doi: 10.3389/faquc.2024.1383534
Received: 07 February 2024; Accepted: 30 April 2024;
Published: 30 May 2024.
Edited by:
Parisa Norouzitallab, Swedish University of Agricultural Sciences, SwedenReviewed by:
Sevdan Yilmaz, Çanakkale Onsekiz Mart University, TürkiyePronob Das, Central Inland Fisheries Research Institute (ICAR), India
Copyright © 2024 Hildebrand, Rebl, Goldammer, Palm and Baßmann. This is an open-access article distributed under the terms of the Creative Commons Attribution License (CC BY). The use, distribution or reproduction in other forums is permitted, provided the original author(s) and the copyright owner(s) are credited and that the original publication in this journal is cited, in accordance with accepted academic practice. No use, distribution or reproduction is permitted which does not comply with these terms.
*Correspondence: Marc-Christopher Hildebrand, bWFyYy1jaHJpc3RvcGhlci5oaWxkZWJyYW5kQHVuaS1yb3N0b2NrLmRl