- 1São Paulo State University (Unesp), Aquaculture Center of Unesp, Jaboticabal, Brazil
- 2Faculdade de Ciências Agrárias, Universidade Zambeze (UniZambeze), Tete, Mozambique
- 3São Paulo State University (Unesp), Faculty of Agricultural Sciences of Vale do Ribeira, Registro, Brazil
Nile tilapia is the main farmed fish in Brazilian aquaculture. Streptococcus agalactiae (GBS) is responsible for high mortality rates in fish farms. Genetic improvement is considered an effective alternative for producing resistant animals in aquaculture. The objective of this study was to estimate genetic parameters for resistance to GBS infection in a breeding population of Nile tilapia from Brazil, based on disease challenge using a circulating serotype (Ib and ST-NT). Furthermore, genetic correlations between resistance to GBS and average daily gain (ADG) were estimated to determine whether these traits can be included in selective breeding programs. Survival (SS) and time until death (TD) were the evaluated traits. The survival of the most resistant families was approximately 30%, indicating high phenotypic variation in resistance to the infection. Low to moderate heritability values for resistance traits in the Nile tilapia population were estimated, ranging from 0.14 to 0.27. Heritability for ADG was moderate to high (0.40) based on a growth trial involving 43 families (735 animals). Phenotypic correlations between the resistance traits (SS and TD) and ADG were positive and low, ranging from 0.09 to 0.18, whereas genetic correlations were close to zero. Phenotypic and genetic correlations between SS and TD were considered high and positive, ranging from 0.38 to 0.72. The results suggest that selection for resistance against GBS does not negatively affect juvenile weight gain in Nile tilapia.
1 Introduction
Nile tilapia (Oreochromis niloticus) is one of the most globally farmed fish (FAO, 2022). Brazil ranks as the fourth-largest producer of Nile tilapia, with an average annual production growth of around 12% since the year 2000 (FAO, 2023), accounting approximately 410 thousand tons (IBGE – Instituto Brasileiro de Geografia e Estatística, 2023).
Streptococcus agalactiae, also known as group B streptococcus (GBS) is the main pathogen responsible for substantial losses in farmed Nile tilapia. GBS is recognized for its morphological and genetic diversity, leading to distinct host–pathogen interactions and immune responses. These factors contribute to disease outbreaks capable of impacting the entire fish population cultured on a farm (Abuseliana et al., 2010). The polysaccharide capsules are considered the major virulence factor of GBS, which are used to identify non-typeable and typeable GBS strains into 10 distinct serotypes (Ia, Ib, II–IX) (Slotved et al., 2007). Five capsular serotypes (Ia, Ib, II, III, and IV) have been associated with disease outbreaks in cultured fish in different countries (Sudpraseart et al., 2021), with capsular serotype Ib predominating in fish infections in the most productive regions of Brazil (Chideroli et al., 2017).
Different Brazilian strains of GBS serotype Ib have been reported, exhibiting heterogeneity in their genome sequences and presenting regional distribution limited according to their genotype. ST-260 and ST-927 are mainly reported in Northeast Brazil, while the non-typeable ST (ST-NT) is widely distributed in Central-Southeast Brazil (Barony et al., 2017). Due to this genetic diversity and the limited cross-protection of commercially available GBS vaccines (Chen et al., 2012), there has been particular concern about the scarcity of commercial vaccines available in Brazil.
Recent genetic studies have suggested that selective breeding for resistance to S. agalactiae is feasible in Nile tilapia (Shoemaker et al., 2017; Suebsong et al., 2019; LaFrentz et al., 2020; Joshi et al., 2021a). However, this approach has not yet been applied to Brazilian populations. For initial studies, additive genetic variation and reliable heritability estimates are prerequisites for any selective breeding program. It is also essential to understand the magnitude of genetic correlations among other commercial traits to optimize selection and control potentially adverse correlated genetic responses.
Genetic parameters have not yet been estimated in Nile tilapia against the main strain and lineage of GBS (serotype Ib and ST-NT) in Brazil. Therefore, our aim was to estimate heritability for resistance against GBS serotype Ib and ST-NT in Nile tilapia within an experimental Brazilian population. Additionally, we investigated the correlated response by estimating the genetic correlation between juvenile average daily gain (ADG) and resistance traits.
2 Methods
2.1 Samples
This study was approved by the Ethics Committee on Animal Use (CEUA number 4935/20) at the University of São Paulo State (UNESP), Jaboticabal Campus, SP, Brazil.
The experimental population comprised 52 Nile tilapia families. However, due to the limited number of animals in some families, only 47 were evaluated for resistance to GBS (serotype Ib and ST-NT) infection (RC), while a juvenile growth trial (GT) was conducted with individuals from 43 families. Specifically, 38 families were included in both experiments (RC and GT), allowing for the assessment of genetic correlations between the traits. Nonetheless there were 9 families exclusively assessed for RC and 5 exclusively for GT. The families originated from the broodstocks of two commercial fish farms in the state of São Paulo, Brazil. These farms produce the GIFT strain (Genetically Improved Farmed Tilapia), the most popular strain in Brazil (Santos et al., 2019), but do not employ genetic selection by pedigree; instead, they use mass selection. Briefly, these two commercial populations share a common origin, derived from genetic material imported from Malaysia (eighth generation of the GIFT) in 2005 by the State University of Maringá – UEM, Brazil (Santos et al., 2011). Subsequently, about 9 generations of selection were conducted by UEM to enhance mainly growth performance in cage farming (Yoshida et al., 2021), but not yet for disease resistance. This genetic material has since been disseminated to various commercial farmers, including the two under investigation in the present study.
The tilapia families were directly sourced separately from each commercial fish farm. In both cases, a similar method was used for grouping broodstocks; specifically, fish breeders were randomly placed in hapas measuring 4 x 2 x 1.5 m3 (approximately 8 hapas per fish farm), with a stocking ratio of 40 females to 20 males. Each experimental family was initially established by collecting eggs from the mouths of females once per hapa. Although the sire of each family is unknown, the procedure of only one collection per hapa provided reliability that the same sire did not fertilize different dams (Fessehaye et al., 2006; Zhao et al., 2016). The collected eggs were transferred to 52 hatcheries for artificial incubation and larval rearing. Subsequently, the swim-up fry were grown with diets containing 45% crude protein for about 30 days. The animals were kept separated by families until they reached the minimal size (approximately 5.0 g and an age of 80 days) for PIT-tagging. After pit-tagging, the fish were released to live communally in 1 m3 fiberglass tanks. All experiments for RC ang GT were conducted at the Center of Aquaculture, UNESP, Jaboticabal, Brazil.
2.2 Records on the average daily gains in the growth trial
The growth trial (GT) was conducted 30 days post-tagging to assess the correlation between ADG and resistance to S. agalactiae. Weight gain was measured in juvenile individuals with an average age of 110 days, housed in a total of 7 fiberglass tanks, each with a water volume of 1 m³. Specifically, approximately six individuals per experimental family were randomly distributed in at least three tanks (triplicate) following a balanced experimental design, totaling around 16 individuals per experimental family and 735 juveniles in total. The density was maintained at approximately 100 fish per m³ in each tank. The 7 tanks were interconnected in a water recirculation system. Throughout the GT, water temperature was kept at 30°C (SD = 0.5°C), dissolved oxygen at 5.0 mg/L (SD = 1.0 mg/L), and pH at 7.2 (SD = 1.0).
Body weight (BW) was recorded at the beginning and end of the 60-day period. The 60-day evaluation period was chosen because most of the animals exhibited a twofold increase in body weight, providing a sufficient timeframe to discern significant differences between families. Throughout the assessment, the fish were provided with 1.2 mm pelleted feed containing 40% crude protein, administered four times daily.
The ADG (g), average daily gain, was calculated according to Ariede et al. (2020) as:
where, Wf represents the weight at the end of the measurement period, Wi represents the weight at the beginning of the measurement period, T represents the total time across the measurement period (60 days).
2.3 Resistance challenge to GBS serotype Ib and ST-NT
The disease resistance challenge (RC) against GBS infection was performed 60 days after the GT. The bacterial isolate (45P, GenBank Accession number KJ561066) was previously recovered from an outbreak of GBS (serotype Ib and ST-NT) in Nile tilapia from the Central-Southeast region of Brazil) (Sebastião et al., 2015). The strain was identified using morphological, biochemical, and molecular tests (16S rRNA gene sequencing) (Assane et al., 2022). Capsular serotyping and sequence typing (Multilocus Sequence Typing) were performed using a multiplex-PCR assay (Poyart et al., 2007), followed by sequencing of seven housekeeping genes (adhP, pheS, atr, glnA, sdhA, glcK, and tkt) (Jones et al., 2003).
For inoculum preparation, bacteria from freshly grown culture plates were transferred to the BHI and incubated for 48 h at 28°C. After growth, the culture medium was washed twice with PBS by centrifugation at 3,000 × g for 10 min at 4°C (de Sousa et al., 2021). The bacterial suspension was adjusted to a lethal dose of 75% (LD75) instead of LD50, considering previous experiences from our research group in disease challenge, in which lower rates of mortality were detected in the final experiments when LD50 was applied (Mastrochirico-Filho et al., 2019). Moreover, the LD75 strategy was implemented to ensure the maximum genetic variance for survival status (a binary trait distinguishing between death or live fish, as explained below in Section 2.4) while maximizing the collection of phenotype data for the time of death.
The LD75 was previously tested by intraperitoneal inoculation in 90 randomly selected individuals from the families (average weight of individuals: 45 g, SD = 23 g), using three dosages of live cells of GBS (serotype Ib and ST-NT). The dosages were adjusted based on the optical density of the solution at 0.400, 0.600 and 0.800 at 625 nm in a spectrophotometer (2100 Unico, Japan), following previous experimental challenges. Subsequently, 100 μl of the inoculum from each dosage was removed to perform serial dilutions and direct plate counts in duplicate. The resulting dosages were: 1.8 x 106 CFU/mL, 3.2 x 106 CFU/mL, and 3.6 x 106 CFU/mL. Before inoculation, the individuals were previously anesthetized with benzocaine during bath exposures at 40 mg/L. Then, each inoculum was intraperitoneally injected at a rate of 0.15 mL for every 10 g of body weight (Khunrang et al., 2023). The chosen dosage of 3.2 x 106 CFU/mL better represented 75% lethality among challenged individuals and was adopted for the RC.
A subsample of the population (n=10) underwent routine microbiological testing using brain and kidney tissue, which confirmed the absence of GBS (serotype Ib and ST-NT) and other pathogens (Laith et al., 2017). Then, for the RC, about 17 individuals from each of the families (a total of 775 individuals) were conditioned in a recirculation system composed of 9 fiberglass tanks (each with a water volume of 1 m3). Each family was distributed in at least three random replicates, similar to the setup for the GT, with about 6 fish per family in different tanks. The experiment was performed with controlled temperature at 30 ± 0.5°C, pH 7.2, and dissolved oxygen (> 5.0 mg/L). A total of 130 individuals was evaluated in each tank. Prior to the inoculation of the bacteria, the individuals were weighted, reaching an average weight of 69.6 g (SD = 49.8 g) with an average age of 268 days (SD = 36.3 days). After the anesthesia of fish in benzocaine (bath exposures at 40 mg/L), the predefined LD75 of live cells of GBS (serotype Ib and ST-NT) was induced by intraperitoneal inoculation (0.15 mL for every 10 g of BW, to avoid any experimental bias caused by variations in BW among individuals). One additional replicate with 130 individuals was used as a control group, where the animals were intraperitonially inoculated with the same volume of PBS (0.15 mL for every 10 g of body weight). The control group was maintained under the same environmental conditions.
The RC experiment was carried out for 21 days to evaluate two resistance traits: survival status (SS) and time of death (TD) in days (Mastrochirico-Filho et al., 2019; Ariede et al., 2020). Clinical and behavioral signs of disease, including exophthalmia, skin darkening, loneliness, loss of appetite, and erratic swimming, were recorded (Suebsong et al., 2019). Dead animals with clinical signs were counted as susceptible. When mortality was detected, the animal was immediately removed from the experiment, and the time of death was recorded.
To confirm infection by GBS (serotype Ib and ST-NT) during the RC, brain and kidney samples were collected from fish nearing death for microbiological analysis (Laith et al., 2017). Pure colonies were utilized for bacterial DNA extraction using the DNeasy Blood and Tissue Extraction Kit (Qiagen). Molecular identification, including 16S rRNA gene sequencing, capsular serotyping, and sequence typing, was performed to confirm the identity of the recovered strain (Assane et al., 2022).
A Kaplan–Meier estimator of survival curves (Kaplan and Meier, 1958) and the log-rank test (p-value< 0.001) were conducted to assess the variation in resistance trends between the three most and the three least resistant families (Mastrochirico-Filho et al., 2019).
2.4 Estimates of the variance components, heritabilities, and genetic correlations
The resistance traits were defined and analyzed using the following definitions:
1. TD (days): Measured along to the RC, from the occurrence of the first mortality event to the last, or defined as the last day of the RC period if the fish survived.
2. SS: Survival status along the RC, with 0 indicating a deceased individual and 1 indicating a surviving individual. This binary trait was evaluated based on two cutoff points:
• SSA: When cumulative mortality reached approximately 50% (up to the 5th day of the RC), maximizing phenotypic variance.
• SSB: At the end of the RC.
The variance components related to TD, SS and ADG were analyzed using two different univariate animal models as described below (following Ariede et al., 2020):
1. Linear models (LIN) were employed to analyze ADG and TD:
where, yij represents the phenotype for the fish j (ADG or TD), in tank i; μ represents the fixed effect of the overall mean; ti represents the fixed effect of the tank i; aj represents the random animal genetic effect of individual j; and eij represents the random residual for the fish j.
2. A binary threshold (probit) model (THR) was utilized to analyze SS:
where, Yij represents the phenotype (SS) for the fish j, in tank i; Φ (·) represents the cumulative standard normal distribution; μ represents the fixed effect of the overall mean; ti represents the fixed effect of the tank i; aj represents the random animal genetic effect of individual j.
THR and LIN models were fitted using ASREML 4.0 software (Gilmour et al., 2009). The significance of the fixed effect fitted in the models was tested by the software using Wald F-statistics. Although the fixed effect was not significant for the evaluated traits (p-value > 0.05), it was not excluded from the models to consider any potential influence on the results. For all the models, the random animal genetic effect was assumed to be , where A is the pedigree-based additive genetic kinship matrix among all the animals included in the population and is the additive genetic variance. Residuals for LIN were assumed to be , where I is an identity matrix and is the residual variance. For the THR model, the residual variance on the underlying scale was set to 1. Regarding the pedigree data, we considered the genetic analysis using half-sib families with missing sire (as described in topic 2.1); thus, the animals from the same family presented a kinship coefficient equal to ¼ in the A matrix, as described by Wright (1922).
The heritability (Falconer and Mackay, 1996) was calculated for both models as:
where, represents the additive genetic variance, and represents the residual variance.
The genetic correlations rg(m, n)between both trait definitions of resistance to S. agalactiae and ADG were calculated using the estimated covariance components in three independent bivariate analyses (TD/SS, TD/ADG, and SS/ADG), as defined by Falconer and Mackay (1996):
where, represents the genetic covariance between the two traits, and represent the additive genetic variance of trait m and trait n, respectively.
The phenotypic correlation rp(m,n) was calculated as:
where, represents the phenotypic covariance between the two traits, and represent the phenotypic variance of trait m and trait n, respectively.
3 Results
3.1 Records on the average daily gains in the growth trial
At the end of the GT, the average BW considering all families was 78.7 g (SD = 51.7 g). Individuals of the family with the highest BW showed an average of 119.6 g (SD = 76.5 g), while the family with the lowest BW registered an average of 25.9 g (SD = 20.9 g). The overall mean of ADG was 0.72 g (SD = 0.45 g), with the worst-performing family demonstrating an average of 0.19 g and the best-performing family an average of 1.13 g (Figure 1).
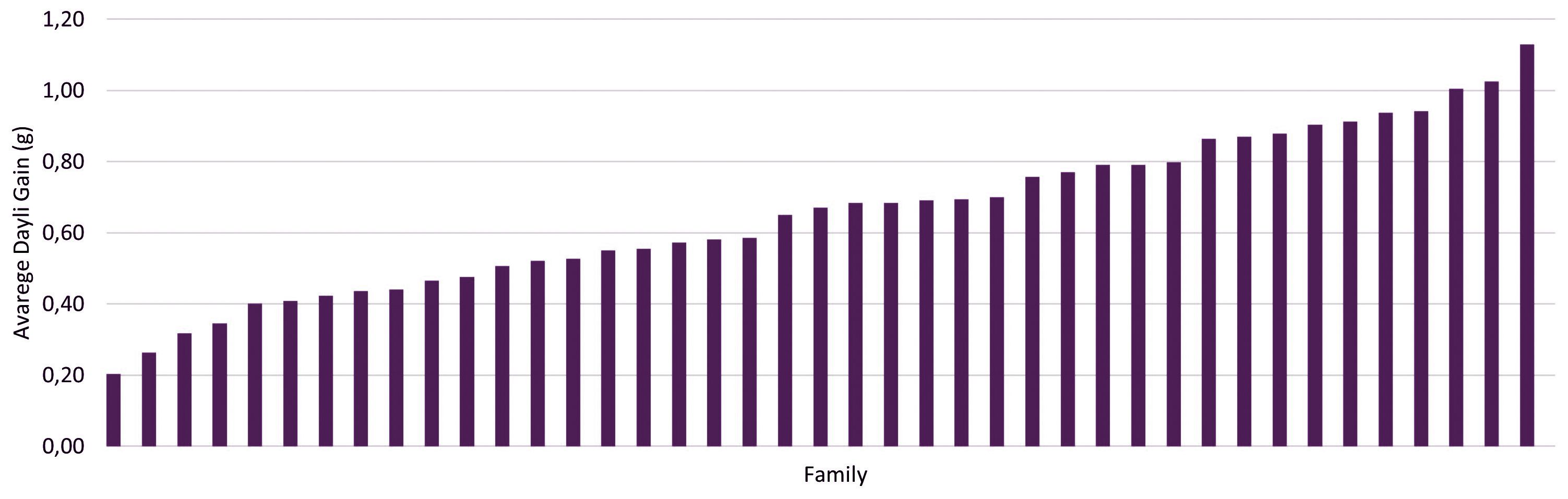
Figure 1 Average daily gain (ADG) in grams of 735 individuals of Nile tilapia (Oreochromis niloticus), representing 43 families of a breeding population. The individuals were evaluated in a growth trial for 60 days.
3.2 Resistance challenge to GBS serotype Ib and ST-NT
After inoculation of the predetermined lethal dose intraperitoneally, infected fish exhibited clinical signs of GBS infection 24 hours post-challenge. Susceptible animals displayed typical clinical signs of the GBS infection, and microbiological analyses confirmed the GBS infection. At the end of the recovery period (21 days), surviving individuals (resistant) were randomly sampled for microbiological analysis, and they were considered infected. No evidence of infection or mortality was found in the animals belonging to the control group.
The first mortality occurred 24 hours post-challenge, while the last mortality was recorded 15 days post-challenge. A mortality plateau was observed 7 days post-challenge. Out of the 775 fish challenged by GBS (Ib and ST-NT), 712 died during the observation period, resulting in a total cumulative mortality of 91.87%. According to Kaplan–Meyer analysis (Figure 2), the three most susceptible families experienced 100% mortality, whereas the three most resistant families showed close to 30% survival at the end of the evaluation. A statistically significant difference (p-value ≤ 0.001) was observed between the survival curves of the least and most resistant families.
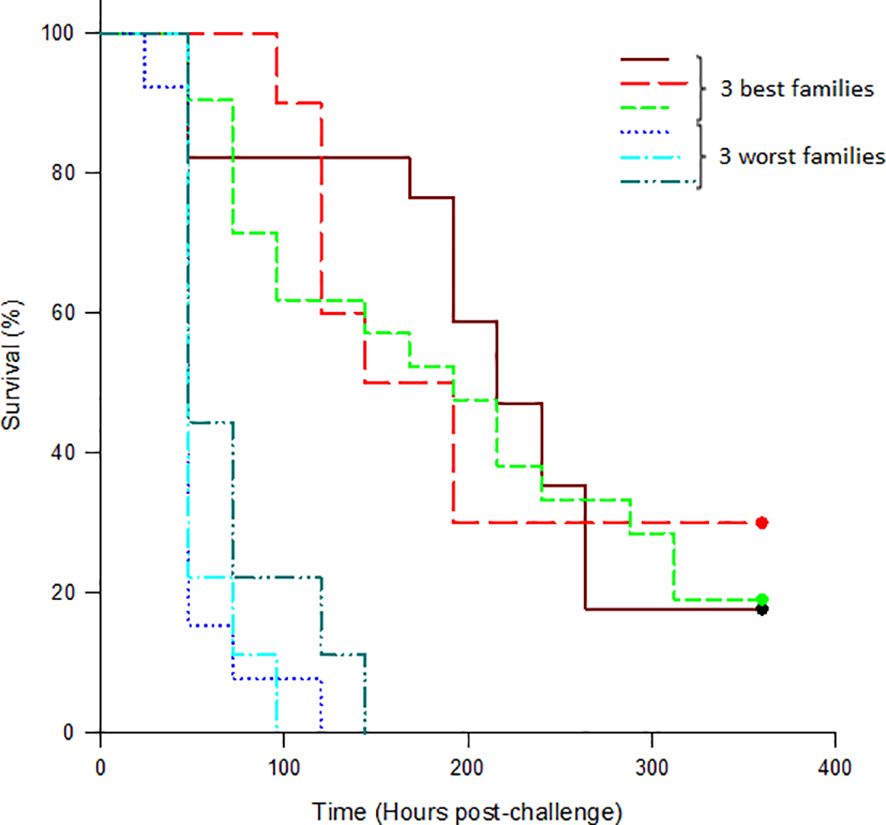
Figure 2 Kaplan–Meier mortality curves of Nile tilapia (Oreochromis niloticus) families infected with Streptococcus agalactiae Ib and ST-NT during a 21-day resistance challenge, without applying the 50% cut-off point in mortality. The data show the cumulative mortality corresponding to more susceptible families (dotted lines) and more resistant families (dashed and full lines) to infection.
When considering only resistant animals surviving past the 5th day, as depicted in Figure 3, i.e., applying a 50% cut-off point to the mortality of the RC, SS varied significantly among families, indicating substantial phenotypic variation concerning resistance against GBS infection. The mean TD due to GBS infection ranged from an average of 2 days (for the least resistant family) to 9 days (for the most resistant family), with an average of 5 days (SD = 1.44 days) (Figure 3).
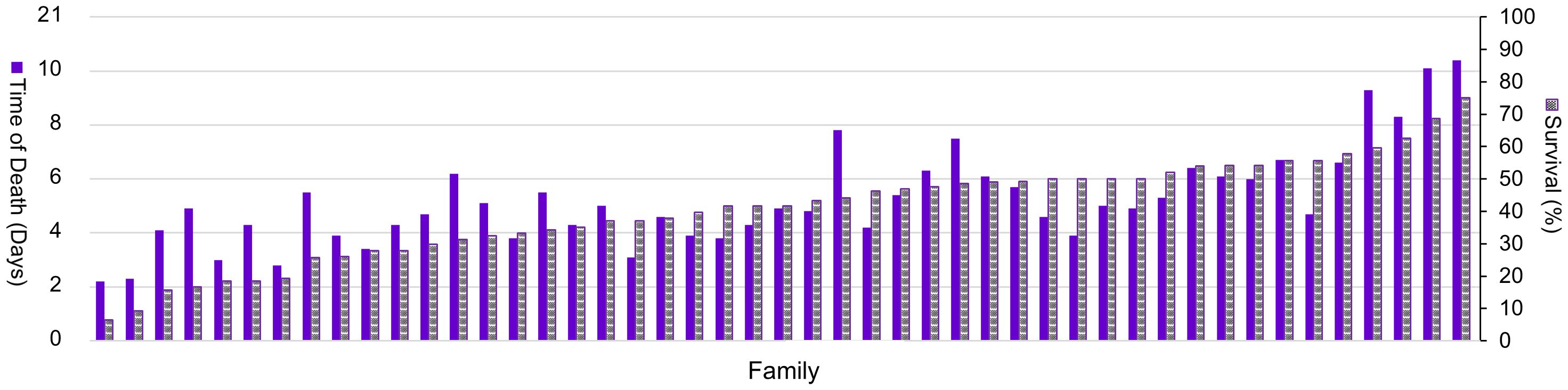
Figure 3 Survival status (%) (bicolored bars) and average time of death (days) (solid bars) of Nile tilapia (Oreochromis niloticus) families infected with Streptococcus agalactiae Ib and ST-NT during a 21-day resistance challenge, applying the 50% cut-off point to the mortality of the experimental challenge. Mesh and full bars represent survival rate and time of death, respectively, for each family of Nile tilapia infected with Streptococcus agalactiae Ib and ST-NT.
3.3 Estimates of the variance components, heritabilities, and genetic correlations
Considerable additive-genetic variance components were observed for both trait definitions of GBS resistance (SSA, SSB, and TD) and ADG. Heritability estimates were low to moderate for SSA (0.27 ± 0.10) and SSB (0.14 ± 0.06). The results indicated moderate heritability for TD (0.24 ± 0.09) and high heritability for ADG (0.40 ± 0.09).
The phenotypic correlations between the two trait definitions of GBS resistance and ADG were positive and low, estimated at 0.16 (SSA/ADG), 0.09 (SSB/ADG) and 0.18 (TD/ADG). The genetic correlations of ADG with the resistance traits were low, close to zero. Phenotypic and genetic correlations between SSA/B and TD were high and positive (Table 1). These findings indicate that genetic improvement can be pursued targeting pathogen resistance without adversely impacting weight gain in Nile tilapia.
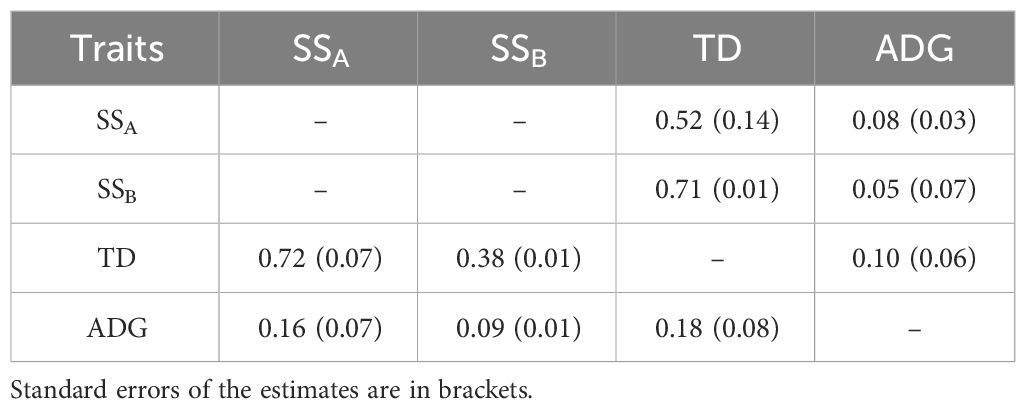
Table 1 Phenotypic and genetic correlations (below and above diagonal, respectively) between survival status (SSA and SSB), time to death (TD) and average daily gain (ADG) in experimental families of the juvenile Nile tilapia.
4 Discussion
Heritability estimates for resistance to GBS in Nile tilapia have recently been reported in populations from the United States (Shoemaker et al., 2017; LaFrentz et al., 2020), Thailand (Suebsong et al., 2019), and the Philippines (Joshi et al., 2021a). However, there have been no investigations addressing commercial populations of Nile tilapia in Brazil. Therefore, one of the main outcomes of this study was the quantification of genetic variation in resistance against GBS serotype Ib (ST-NT variant) in an experimental breeding population of Nile tilapia in Brazil, which has been particularly selected for growth performance (Yoshida et al., 2021).
In Brazil, epidemiological studies of GBS serotype Ib have identified three strains with distinct geographical distribution patterns (Barony et al., 2017): the ST-NT, exclusive to the Central-Southeast region; and the sequence types (ST) ST-260 and ST-927, predominantly found in the Northeast region. The ST-NT strain requires particular attention due to numerous reported outbreaks in the primary region of production in Brazil (Central-Southeast region), contributing to approximately 75% of Nile tilapia production in the country (IBGE – Instituto Brasileiro de Geografia e Estatística, 2023). The ST-NT, having emerged recently (post-1956), also raises concerns regarding vaccine efficacy (Barony et al., 2017), as the effectiveness of commercial vaccines against the ST-NT has not been assessed. Furthermore, none of the previous studies on genetic parameters in Nile tilapia have focused on this GBS serotype Ib strain. Therefore, to maximize host resistance efficacy in field conditions, it is crucial to conduct experimental disease challenges using strains that affect the specific population under investigation, as was performed in this study for the NT-ST strain.
The variation in survival rates among families was within the range of those presented by previous studies in Nile tilapia (Shoemaker et al., 2017; Suebsong et al., 2019; Joshi et al., 2021a, b). The low to moderate heritability values for survival presented herein were also similar to those previously reported for resistance against GBS in different Nile tilapia populations, ranging from 0.21 ± 0.03 (LaFrentz et al., 2020) to 0.38 ± 0.11 (Shoemaker et al., 2017).
In analyzing the heritability values for SS, we posit that SSB (h2 = 0.14 ± 0.06) might be influenced by the low phenotypic variance (constituting 91.87% of cumulative mortality) observed throughout the entire challenge period, whereas SSA (h2 = 0.27 ± 0.10) represents the peak of phenotypic variance for this binary trait (50% cumulative mortality) (Drangsholt et al., 2011). In relation to TD, the moderated heritability (h2 = 0.24 ± 0.09) indicates that genetic selection is viable for this trait, allowing the development of more pathogen-tolerant populations, which provides sufficient time to delineate targeted strategies before mass mortalities occur.
Despite the use of a relatively small sample size compared to previous studies in aquaculture (Barría et al., 2018; Holborn et al., 2018; Hillestad and Moghadam, 2019), the standard error of our heritability estimates was notably low, suggesting statistical reliability for the analyzed population (Storset et al., 2007; Evenhuis et al., 2015; Xiong et al., 2017; Lira et al., 2020). However, we cannot rule out that our heritability values might be overestimated due to the experimental design used for family formation, where the common environment and additive genetic effects could not be distinctly separated, i.e., they could be confounded by the pedigree structure assumed in the present study (1x1). Typically, without the use of hierarchical mating (e.g., each sire mating with several dams) or partial factorial mating to generate half-sib families (e.g., Joshi et al., 2018), there is a limitation in segregating the variance components of the common environment and additive genetic components, and as a result, the heritability can be considered inflated.
The heritability results for ADG during the juvenile stage also revealed a moderate value (h² = 0.40 ± 0.09), similarly to findings from previous studies in Nile tilapia, which reported values of 0.41 and 0.49 for different ages (Barría et al., 2020; Joshi et al., 2021a, b). Further investigations are still needed to assess the magnitude and direction of genetic correlations or potential re-ranking of Estimated Breeding Values (EBVs) for growth-related traits between the juvenile and harvest weight phases in this breeding population. A positive and weak genetic correlation was detected between ADG and resistance traits to GBS in Nile tilapia, similar to the results found for the genetic relationship between growth traits and resistance to bacterial cold-water disease in trout (Silverstein et al., 2009); therefore, our results suggest that selecting more resistant fish will not necessarily result in fish with lower growth performance.
While molecular analyses offer reliability for parentage assignment (Vandeputte and Haffray, 2014), the elevated costs associated with these techniques limit their routine application. Nevertheless, even in the absence of molecular analysis to accurately assign the sire, our experimental design successfully captured the genetic variation between families. Additionally, since we adopted the structure of half-sib families for our genetic analyses (despite the higher probability of them being full-sib families), the estimation of heritability might be underestimated, given that the genetic relation (pedigree) is likely underestimated in such scenario.
This study represents the first characterization of genetic parameters for resistance to GBS (Ib and ST-NT strain) within a breeding nucleus of Nile tilapia originating from Brazil. The heritability estimates indicate the potential for genetic improvement of resistance to this pathogen through selective breeding within this specific Nile tilapia population, without adversely affecting growth performance.
Data availability statement
The original contributions presented in the study are publicly available. This data can be found here: GenBank, accession KJ561066.
Ethics statement
The animal study was approved by Ethics Committee on Animal Use (CEUA number 4935/20). The study was conducted in accordance with the local legislation and institutional requirements.
Author contributions
RO-N: Data curation, Formal analysis, Investigation, Methodology, Project administration, Software, Writing – original draft. VM-F: Formal analysis, Methodology, Writing – review & editing. IA: Data curation, Methodology, Writing – review & editing. RA: Methodology, Writing – review & editing. MF: Methodology, Writing – review & editing. JA: Methodology, Writing – review & editing. CB: Methodology, Writing – review & editing. TG: Writing – review & editing, Methodology. LL: Methodology, Writing – review & editing. RR: Formal analysis, Writing – review & editing. FP: Writing – review & editing. DH: Conceptualization, Funding acquisition, Investigation, Project administration, Resources, Supervision, Validation, Visualization, Writing – review & editing.
Funding
The author(s) declare financial support was received for the research, authorship, and/or publication of this article. This work was supported by São Paulo Research Foundation (FAPESP grant 2019/23029-0), National Council for Scientific and Technological Development (CNPq grant 312250/2021-5) and Coordination of Improvement of Higher Education Personnel – Brazil (CAPES – Finance Code 001).
Conflict of interest
The authors declare that the research was conducted in the absence of any commercial or financial relationships that could be construed as a potential conflict of interest.
Publisher’s note
All claims expressed in this article are solely those of the authors and do not necessarily represent those of their affiliated organizations, or those of the publisher, the editors and the reviewers. Any product that may be evaluated in this article, or claim that may be made by its manufacturer, is not guaranteed or endorsed by the publisher.
References
Abuseliana A., Daud H., Aziz S. A., Bejo S. K., Alsaid M. (2010). Streptococcus agalactiae the etiological agent of mass mortality in farmed red tilapia (Oreochromis sp.). J. Anim. Vet. Adv. 9, 2640–2646. doi: 10.3923/javaa.2010.2640.2646
Ariede R. B., Freitas M. V., Agudelo J. F. G., Borges C. H. S., Lira L. V. G., Yoshida G. M., et al. (2020). Genetic (co)variation between resistance to Aeromonas hydrophila and growth in tambaqui (Colossoma macropomum). Aquaculture 523, 735225. doi: 10.1016/j.aquaculture.2020.735225
Assane I. M., Neto R. R. O., Ferreira D. A. R., Oliveira A. V., Hashimoto D. T., Pilarski F. (2022). Genetic diversity, virulence, antimicrobial resistance genes, and antimicrobial susceptibility of group B Streptococcus (GBS) linked to mass mortalities of cultured Nile tilapia in Brazil. bioRxiv. [Pré-print]. doi: 10.1101/2022.12.25.521911
Barony G. M., Tavares G. C., Pereira F. L., Carvalho A. F., Dorella F. A., Leal C. A. G., et al. (2017). Large-scale genomic analyses reveal the population structure and evolutionary trends of Streptococcus agalactiae strains in Brazilian fish farms. Sci. Rep. 7, 13538. doi: 10.1038/s41598-017-13228-z
Barría A., Christensen K. A., Yoshida G. M., Correa K., Jedlicki A., Lhorente J. P., et al. (2018). Genomic predictions and genome-wide association study of resistance against Piscirickettsia salmonis in coho salmon (Oncorhynchus kisutch) using ddRAD sequencing. G3 - Genes Genom. Genet. 8, 1183–1194. doi: 10.1534/g3.118.200053
Barría A., Trinh T. Q., Mahmuddin M., Benzie J. A. H., Chadag V. M., Houston R. D. (2020). Genetic parameters for resistance to Tilapia Lake Virus (TiLV) in Nile tilapia (Oreochromis niloticus). Aquaculture 522, 735126. doi: 10.1016/j.aquaculture.2020.735126
Chen M., Wang R., Li L., Liang W., Li J., Huang Y., et al. (2012). Screening vaccine candidate strains against Streptococcus agalactiae of tilapia based on PFGE genotype. Vaccine 30, 6088–6092. doi: 10.1016/j.vaccine.2012.07.044
Chideroli R. T., Amoroso N., Mainardi R. M., Suphoronski S. A., de Padua S. B., Alfieri A. F., et al. (2017). Emergence of a new multidrug-resistant and highly virulent serotype of Streptococcus agalactiae in fish farms from Brazil. Aquaculture 479, 45–51. doi: 10.1016/j.aquaculture.2017.05.013
de Sousa E. L., Assane I. M., Santos-Filho N. A., Cilli E. M., de Jesus R. B., Pilarski F. (2021). Haematological, biochemical and immunological biomarkers, antibacterial activity, and survival in Nile tilapia Oreochromis niloticus after treatment using antimicrobial peptide LL-37 against Streptococcus agalactiae. Aquaculture 533, 736181. doi: 10.1016/j.aquaculture.2020.736181
Drangsholt T. M., Gjerde B., Ødegård J., Finne-Fridell F., Evensen Ø., Bentsen H. B. (2011). Quantitative genetics of disease resistance in vaccinated and unvaccinated Atlantic salmon (Salmo salar L.). Heredity 107, 471–477. doi: 10.1038/hdy.2011.34
Evenhuis J. P., Leeds T. D., Marancik D. P., LaPatra S. E., Wiens G. D. (2015). Rainbow trout (Oncorhynchus mykiss) resistance to columnaris disease is heritable and favorably correlated with bacterial cold water disease resistance. J. Anim. Sci. 93, 1546–1554. doi: 10.2527/jas2014-8566
Falconer D. S., Mackay T. F. C. (1996). Introduction to quantitative genetics (Harlow: Longmans Green).
FAO (2022). The State of World Fisheries and Aquaculture 2022. Towards Blue Transformation (Rome: FAO). doi: 10.4060/cc0461en
FAO (2023). FishStatJ: universal software for fishery statistical time series: aquaculture production 1950–2021 (Release date: March 2023) (Rome: FAO).
Fessehaye Y., El-bialy Z., Rezk M. A., Crooijmans R., Bovenhuis H., Komen H. (2006). Mating systems and male reproductive success in Nile tilapia (Oreochromis niloticus) in breeding hapas: A microsatellite analysis. Aquaculture 256, 148–158. doi: 10.1016/j.aquaculture.2006.02.024
Gilmour A., Gogel B., Cullis B., Thompson R., Butler D., Cherry M., et al. (2009). ASReml User Guide Release 3.0. 275 (Hemel Hempstead, UK: VSN International Ltd, UK). Available at: http://www.vsni.co.uk.
Hillestad B., Moghadam H. K. (2019). Genome-wide association study of Piscine Myocarditis virus (PMCV) resistance in Atlantic Salmon (Salmo salar). J. Hered. 110, 720–726. doi: 10.1093/jhered/esz040
Holborn M. K., Ang K. P., Elliott J. A. K., Powell F., Boulding E. G. (2018). Genome wide association analysis for bacterial kidney disease resistance in a commercial North American Atlantic salmon (Salmo salar) population using a 50 K SNP panel. Aquaculture 495, 465–471. doi: 10.1016/j.aquaculture.2018.06.014
IBGE – Instituto Brasileiro de Geografia e Estatística (2023). Produção da pecuária municipal 2022 (Rio de Janeiro: IBGE).
Jones N., Bohnsack J. F., Takahashi S., Oliver K. A., Chan M., Kunst F., et al. (2003). Multilocus sequence typing system for group B streptococcus. J. Clin. Microbiol. 41, 2530–2536. doi: 10.1128/jcm.41.6.2530-2536.2003
Joshi R., Almeida D. B., Costa A. R., Skaarud A., Pereira U. P., Knutsen T. M., et al. (2021b). Genomic selection for resistance to Francisellosis in commercial Nile tilapia population: genetic and genomic parameters, correlation with growth rate and predictive ability. Aquaculture 537, 736515. doi: 10.1016/j.aquaculture.2021.736515
Joshi R., Skaarud A., Alvarez A. T., Moen T., Ødegård J. (2021a). Bayesian genomic models boost prediction accuracy for survival to Streptococcus agalactiae infection in Nile tilapia (Oreochromus nilioticus). Genet. Sel. Evol. 53, 1–10. doi: 10.1186/s12711-021-00629-y
Joshi R., Woolliams J. A., Meuwissen T. H. E., Gjøen H. M. (2018). Maternal, dominance and additive genetic effects in Nile tilapia; influence on growth, fillet yield and body size traits. Heredity 120, 452–462. doi: 10.1038/s41437-017-0046-x
Kaplan E. L., Meier P. (1958). Nonparametric estimation from incomplete observations. JASA 53, 457–481. doi: 10.2307/2281868
Khunrang T., Pooljun C., Wuthisuthimethavee S. (2023). Correlation of Streptococcus agalactiae concentration on immune system and effective dose of inactivated vaccine for Chitralada 3 strain Nile tilapia (Oreochromis niloticus) in Thailand. BMC Vet. Res. 19, 267. doi: 10.1186/s12917-023-03835-6
LaFrentz B., Lozano C., Shoemaker C. A., Garcia J. C., Ospina-Arango J. F., Rye M., et al. (2020). Genetic (co) variation between harvest weight and resistance to both Streptococcus iniae and S. agalactiae capsular type Ib in Nile tilapia (Oreochromis niloticus). Aquaculture 529, 735726. doi: 10.1016/j.aquaculture.2020.735726
Laith A. A., Ambak M. A., Hassan M., Sheriff S. M., Nadirah M., Draman A. S., et al. (2017). Molecular identification and histopathological study of natural Streptococcus agalactiae infection in hybrid tilapia (Oreochromis niloticus). Vet. World 10, 101–111. doi: 10.14202/vetworld.2017.101-111
Lira L. V., Ariede R. B., Freitas M. V., Mastrochirico-Filho V. A., Agudelo J. F., Barría A., et al. (2020). Quantitative genetic variation for resistance to the parasite Ichthyophthirius multifiliis in the Neotropical fish tambaqui (Colossoma macropomum). Aquac. Rep. 17, 100338. doi: 10.1016/j.aquaculture.2012.12.022
Mastrochirico-Filho V. A., Ariede R. B., Freitas M. V., Lira L. V. G., Agudelo J. F. G., Pilarski F., et al. (2019). Genetic parameters for resistance to Aeromonas hydrophila in the Neotropical fish pacu (Piaractus mesopotamicus). Aquaculture 513, 734442. doi: 10.1016/j.aquaculture.2019.734442
Poyart C., Tazi A., Réglier-Poupet H., Billoët A., Tavares N., Raymond J., et al. (2007). Multiplex PCR assay for rapid and accurate capsular typing of group B streptococci. J. Clin. Microbiol. 45, 1985–1988. doi: 10.1128/JCM.00159-07
Santos A. I., Ribeiro R. P., Vargas L., Mora F., Alexandre Filho L., Fornari D. C., et al. (2011). Bayesian genetic parameters for body weight and survival of Nile tilapia farmed in Brazil. Pesqui. Agropecu. Bras. 46, 33–43. doi: 10.1590/S0100-204X2011000100005
Santos V. B., Silva V. V., Almeida M. V., Mareco E. A., Salomão R. A. S. (2019). Performance of Nile tilapia Oreochromis niloticus strains in Brazil: a comparison with Philippine strain. J. Appl. Anim. Res. 47, 72–78. doi: 10.1080/09712119.2019.1571495
Sebastião F. A., Furlan L. R., Hashimoto D. T., Pilarski F. (2015). Identification of bacterial fish pathogens in Brazil by direct colony PCR and 16S rRNA gene sequencing. Adv. Microbiol. 05, 409–424. doi: 10.4236/aim.2015.56042
Shoemaker C. A., Lozano C. A., LaFrentz B. R., García J. C., Soto E., Xu D., et al. (2017). Additive genetic variation in resistance of Nile tilapia (Oreochromis niloticus) to Streptococcus iniae and S. agalactiae capsular type Ib: Is genetic resistance correlated? Aquaculture 468, 193–198. doi: 10.1016/j.aquaculture.2016.10.022
Silverstein J. T., Vallejo R. L., Palti Y., Leeds T. D., Rexroad C. E. III, Welch T. J., et al. (2009). Rainbow trout resistance to bacterial cold-water disease is moderately heritable and is not adversely correlated with growth. J. Anim. Sci. 87, 860–867. doi: 10.2527/jas.2008-1157
Slotved H. C., Kong F., Lambertsen L., Sauer S., Gilbert G. L. (2007). Serotype IX, a proposed new Streptococcus agalactiae serotype. J. Clin. Microbiol. 45, 2929–2936. doi: 10.1128/JCM.00117-07
Storset A., Strand C., Wetten M., Kjøglum S., Ramstad A. (2007). Response to selection for resistance against infectious pancreatic necrosis in Atlantic salmon (Salmon salar L.). Aquaculture 272S1, S62–S68. doi: 10.1016/j.aquaculture.2007.08.011
Sudpraseart C., Wang P. C., Chen S. C. (2021). Phenotype, genotype and pathogenicity of Streptococcus agalactiae isolated from cultured tilapia (Oreochromis spp.) in Taiwan. J. Fish. Dis. 44, 747–756. doi: 10.1111/jfd.13296
Suebsong W., Poompuang S., Srisapoome P., Koonawootrittriron S., Luengnaruemitchai A., Johansen H., et al. (2019). Selection response for Streptococcus agalactiae resistance in Nile tilapia Oreochromis niloticus. J. Fish. Dis. 42, 1553–1562. doi: 10.1111/jfd.13074
Vandeputte M., Haffray P. (2014). Parentage assignment with genomic markers: a major advance for understanding and exploiting genetic variation of quantitative traits in farmed aquatic animals. Front. Genet. 5. doi: 10.3389/fgene.2014.00432
Wright S. (1922). Coefficients of inbreeding and relationship. Am. Nat. 56, 330–338. doi: 10.1086/279872
Xiong X., Chen Y., Liu L., Wang W. (2017). Estimation of genetic parameters to Aeromonas hydrophila in blunt snout bream (Megalobrama amblycephala). Aquaculture 479, 768–773. doi: 10.1016/j.aquaculture.2017.07.011
Yoshida G. M., de Oliveira C. A. L., Campos E. C., Todesco H., Araújo F. C., Karin H. M., et al. (2021). A breeding program for Nile tilapia in Brazil: Results from nine generations of selection to increase the growth rate in cages. J. Anim. Breed. Genet. 139, 127–135. doi: 10.1111/jbg.12650
Keywords: selective breeding, disease resistance, heritability, genetic correlation, aquaculture
Citation: Oliveira-Neto RR, Mastrochirico-Filho VA, Assane IM, Ariede RB, Freitas MV, Agudelo JFG, Borges CHS, Gonçalves TG, Lira LVG, Reis Neto RV, Pilarski F and Hashimoto DT (2024) Resistance of juvenile Nile tilapia Oreochromis niloticus from Brazilian populations to Streptococcus agalactiae (serotype Ib and ST-NT). Front. Aquac. 3:1354029. doi: 10.3389/faquc.2024.1354029
Received: 11 December 2023; Accepted: 11 April 2024;
Published: 25 April 2024.
Edited by:
Mehdi Soltani, Murdoch University, AustraliaReviewed by:
Milad Adel, Iranian Fisheries Research Organization, IranMuhammed Duman, Bursa Uludağ University, Türkiye
Margarita Smirnov, Ministry of Agriculture and Rural Development, Israel
Copyright © 2024 Oliveira-Neto, Mastrochirico-Filho, Assane, Ariede, Freitas, Agudelo, Borges, Gonçalves, Lira, Reis Neto, Pilarski and Hashimoto. This is an open-access article distributed under the terms of the Creative Commons Attribution License (CC BY). The use, distribution or reproduction in other forums is permitted, provided the original author(s) and the copyright owner(s) are credited and that the original publication in this journal is cited, in accordance with accepted academic practice. No use, distribution or reproduction is permitted which does not comply with these terms.
*Correspondence: Diogo Teruo Hashimoto, diogo.hashimoto@unesp.br