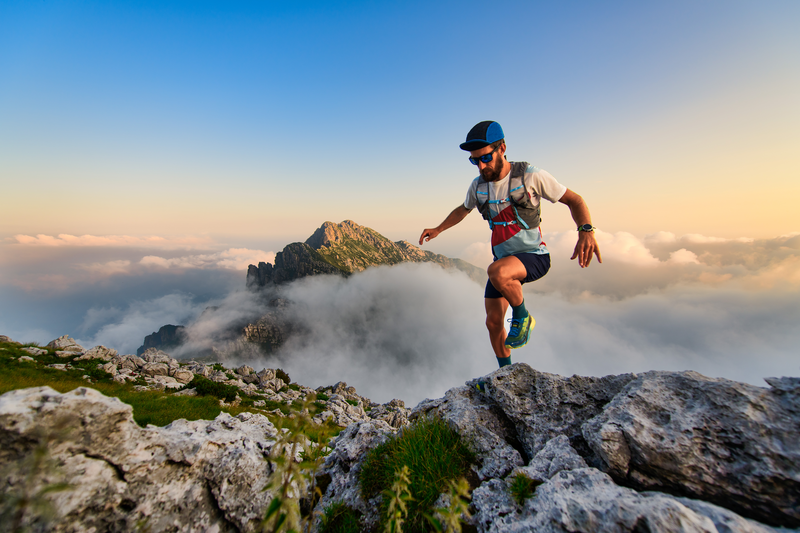
94% of researchers rate our articles as excellent or good
Learn more about the work of our research integrity team to safeguard the quality of each article we publish.
Find out more
REVIEW article
Front. Aquac. , 22 February 2024
Sec. Production Biology
Volume 3 - 2024 | https://doi.org/10.3389/faquc.2024.1281640
Broodstock nutrition is the most essential constituent of sustainable aquaculture production. Its impact on the reproductive performance and profitability of commercial aquaculture has been the subject of considerable research. The present paper reviews the literature available on the subject, examining the implication of Nile tilapia broodstock nutrition on parameters including maturation, fecundity, fertilization, embryo development, larval quality, and survival rate. The provision of a nutritive diet composed of essential macro- and micronutrients including proteins, lipids, carbohydrates, vitamins, minerals, and functional additives, such as prebiotics, enzymes, hormones, and probiotics, in different proportions is discussed. Special emphasis is given to literature dealing with the effects of dietary protein and lipid on vitellogenesis and ovarian maturation, fecundity, egg hatching rate, larval quality, and fry survival number. The impact of the feeding regime on reproductive performance is described.
Nile tilapia (Oreochromis niloticus) is one of the most commercially important fish species native to tropical and subtropical water bodies in the eastern and western parts of Africa, such as the Nile and Niger River basins and Lakes Tanganyika, Albert, Edward, and Kivu (Agnese et al., 1997; Lind et al., 2019; Tibihika et al., 2020). This species is also found outside of its native range in many parts of the world (Tesfaye et al., 2021). Its suitability to different culture techniques; its broad ecological tolerance to various environmental and physicochemical water quality parameters such as fluctuations in pH and salinity, low dissolved oxygen, and high concentrations of chemical nutrients; and its ability to reproduce in captivity make this species one of the most widely cultivated fish around the globe (El-Sayed et al., 2005; Sarmento et al., 2017; Suloma et al., 2017; Tesfaye et al., 2021; Mugwanya et al., 2022). For this reason, the commercial importance of Nile tilapia is undeniable, especially in the top tilapia-producing Asian and African countries where the demand for tilapia larvae and juveniles is increasing exponentially (Ribeiro et al., 2017; Lind et al., 2019). To ensure the sustainable growth and expansion of tilapia farming all over the world, prolific measures such as improved nutrition, feeding, and management, with particular emphasis on broodstock, are essential to providing large and high-quality eggs (Mabroke et al., 2013; Sousa et al., 2013). Providing a balanced diet for broodstock fish is essential not only for the maximum growth and health of juveniles but also to ensure the prime condition of the broodstock fish (El-Sayed et al., 2003; Mabroke et al., 2013).
Proper dietary composition is fundamental to broodstock nourishment (Bhujel et al., 2007; Gebre-tsadik and Bart, 2007), as there is a wealth of evidence on nutrient quality affecting health and both quality and quantity of the supply of offspring (Izquierdo et al., 2001; El-Sayed and Kawanna, 2008; Kumar et al., 2018; Durigon et al., 2019). Nutrition, which is highly determined by diet, has direct and indirect effects on fish maturation, fecundity, semen quality and quantity, fertilization, embryo development, larval quality, and larval survival (Tacon, 2000; Bhujel et al., 2007; Gebre-tsadik and Bart, 2007; Sarmento et al., 2017; Kumar et al., 2018; Hernandez de-Dios et al., 2022). Despite the importance of broodstock fish nutrition, the nutritional requirements of broodstock fish have become poorly understood and less researched due to both the lack of suitable culture facilities that maintain large groups of adult fishes and to the increased costs of extended broodstock feeding investigation (Izquierdo et al., 2001; Mandal et al., 2013; de Oliveira et al., 2014). To date, the nutritional requirements of tilapia broodstock have been summarized in previous reports as key factors in tilapia production strategies (Bhujel, 2000; Ng and Romano, 2013). Since then, research has expanded to consider specific dietary needs associated with the improvement of tilapia reproductive performance. However, these results are not presented in an organized manner. Therefore, this review aimed to gather information about broodstock nutrition in Nile tilapia and its implications on reproductive efficiency.
A recent innovative PRISMA (Preferred Reporting Items for Systematic Review and Meta-Analysis) checklist approach was used to conceptualize findings, synthesize concepts, and include and exclude articles (Salameh et al., 2020). The literature search for this review was performed using a number of scientific databases, including ScienceDirect, ResearchGate, Google Scholar, Web of Science, SciELO, LibGen, PubMed, Science-Hub, Internet archives, Scopus, CrossRef, and EcoPapers, among others. The searched scientific articles had been published over the previous 30 years. The following keywords were used to search the literature: broodstock nutrition in Nile tilapia, reproductive implications of broodstock diet, broodstock diet and reproductive performance, broodstock feed formulation and aquaculture profitability, and Nile tilapia nutrition and production. A total of 293 publications were searched, of which 105 articles were selected; however, 188 papers were excluded due to insufficient scientific standards (see Figure 1). Critical evaluation measures including journal ranking, impact factors, and relevance of the research article to the research theme, timelines, reproducibility, novelty, and reliability were taken into consideration.
Figure 1 A PRISMA flow chart for searching, screening, inclusion and exclusion of literature used for review.
Proper dietary composition is fundamental to broodstock nourishment and to a sustainable aquaculture system (Bhujel et al., 2007; Hernandez de-Dios et al., 2022). There is a wealth of evidence on nutrient quality being able to considerably affect the reproductive performance in fish (Izquierdo et al., 2001; El-Sayed and Kawanna, 2008; Kumar et al., 2018). Therefore, for many cultured species, broodstock nutrition coupled with the availability of optimal diet is the most limiting factor in determining the variability and unpredictability of reproductive performance. Apart from improved egg and sperm quality, good broodstock nutrition results in the mass production of juveniles and the enhanced growth of fish in order to reach a large size (de Oliveira et al., 2014; Siagian and Nugroho, 2018). Despite species-specific variations in fecundity, many large-sized fish species tend to produce more eggs, resulting in larger larvae with enhanced survival benefits (de Oliveira et al., 2014; Ribeiro et al., 2017). Similarly, the quality and the quantity of broodstock nutrition affect the survival of the young. In some fish species, certain essential dietary nutrients, e.g., proteins, lipids, vitamins, and other inorganic compounds, highly determine the fecundity and gonad development aspect of broodstock nutrition (Sousa et al., 2013; Sarmento et al., 2017).
Sustainable commercial production of tilapia is ensured by the continuous year-round supply of high-quality fish eggs. For this reason, broodstock tilapia could produce good quality fertile eggs with maximum growth and survival rates if they are supplied with formulated, nutritive diets composed of essential nutrients (de Oliveira et al., 2014; Xiao et al., 2017). Nile tilapia are also known to reach sexual maturity at a relatively small size. Furthermore, studies have shown that providing broodstock tilapia with a nutritive diet composed of essential nutrients not only reduced age at first maturity, but also improved vitellogenesis, enhancing the egg quality and progeny development (Al-Hafedh et al., 1999; Al-Feky et al., 2014).
Although it is evident that broodstock nutrition has a significant impact on the breeding performance of males, females, and juveniles, there is little information regarding the optimal dietary requirement of many farmed broodstock fish species like the Nile tilapia (de Oliveira et al., 2014; Ribeiro et al., 2017; Ragasa et al., 2022). This might be due to the short history of commercial intensive aquaculture system, the lack of information on the demand of species-specific broodstock diet, technological dependence, and the financial limitations in conducting these studies (Mabroke et al., 2013; Sousa et al., 2013). Recently, broodstock nutrition research in aquaculture has been given great attention due to the formulation of quality feed and the use of genetically selected stocks that would improve the profitability of commercial aquaculture (Saenz, 2021). Therefore, the production of an adequate amount of quality seeds with the formulation of appropriate nutritive broodstock diets can highly enhance the reproductive potential, such as a short generation time, high fecundity, and low mortality of fishes, making the industry profitable (El-Sayed and Kawanna, 2008; Mabroke et al., 2013; Siagian and Nugroho, 2018).
The provision of a formulated and balanced nutritive diet composed of proteins, lipids, carbohydrates, vitamins, and minerals, along with ration size and feed frequency, to broodstock tilapia that is low cost and environment-friendly would result in optimum growth and improved reproduction (Xiao et al., 2017).
Protein is an indispensable dietary ingredient in broodstock tilapia feed that provides essential and nonessential amino acids and is used to offer highly available energy when other energy sources are inadequate and for the synthesis of new tissues, hormones, enzymes, and antibodies (Mandal et al., 2013; de Oliveira et al., 2014). Proteins are used as a source of energy for the reproductive process, including the aggressive behavior of the males, for mating, territory defense, and in oral egg incubation (El-Sayed and Kawanna, 2008). Hence, the inclusion of optimum dietary protein in broodstock nutrition would be fundamental in the formulation of fish feed to avoid poor reproductive performance, stunted growth, and weight loss (Ribeiro et al., 2017). Numerous research findings have shown that the breeding results on larval quality and progeny obtained by protein nourishment are better than those by other purified macronutrients (Abidin et al., 2006; de Oliveira et al., 2014). Similarly, the dietary protein level and the feeding regime of female tilapia highly influence oocyte growth, puberty, spawning performance, and egg quality. Subsequently, the quality and performance of larvae will be exceedingly dependent only on the stored nutrients in the yolk (El-Sayed and Kawanna, 2008; de Oliveira et al., 2014).
The provision of an optimum level of dietary protein to broodstock tilapia would improve the growth, development, health, and maintenance of the species, with a knock-on effect on improved reproduction (Abidin et al., 2006; Xiao et al., 2017). However, there are great variations in the quality and quantity of the protein requirement of broodstock tilapia. For example, the water temperature, salinity, age, sex, amino acid composition, maturation, spawning period, feeding frequency, and dietary energy level are major determinants of the protein requirement (Chong et al., 2004; El-Sayed and Kawanna, 2008; Mandal et al., 2013). Hence, in broodstock tilapia, the optimal amount of dietary protein is the lowest level of protein that results in the best performance under certain circumstances. Thus, quantification of the total dietary protein requirement of broodstock fish is essential for sustainable and profitable aquaculture (El-Sayed et al., 2005; de Oliveira et al., 2014) since the cost of dietary protein is the most expensive component of culture systems, accounting for approximately 50% of the feed costs (Ribeiro et al., 2017). Apart from the dietary protein level, the source of protein (i.e., animal, plant, or both) also vastly determines growth, survival, and other outputs (Mandal et al., 2013).
Numerous studies have revealed that the provision of optimal diet to broodstock fish results in improved body weight, growth, and egg quality (Abidin et al., 2006; Ribeiro et al., 2017). For instance, in broodstock fish fed 40%, 35%, and 30% dietary protein, maximum body size growth, egg quality, and final body weight were observed in those supplied 40% and 35% dietary protein levels. The contribution of broodstock protein toward improved reproduction could be ascribed to the strong association of body size growth with prior maturation of gonads, as many investigations have reported on the earlier development of eggs in large-sized broodstock species (Chong et al., 2004; de Oliveira et al., 2014). Unlike the above, those treated with 30% dietary protein showed the lowest fecundity in addition to poor reproductive potential with an inferior growth rate, indicating the insufficient amount of protein required by females for gonad development. Thus, for egg development, fishes might use protein from their body reserve, as indicated by the highest amount of protein in the egg being observed from fishes supplied with 40% dietary protein, which conferred an increased amount of dietary protein and led to the increased amount of protein in the egg (Abidin et al., 2006).
Many authors have elucidated that a crude protein (CP) content of 30%–40% is optimal for the maximum growth and reproduction of broodstock tilapia (Marty, 2003; El-Sayed and Kawanna, 2008; de Oliveira et al., 2014). For instance, Marty (2003) suggested a dietary CP level of 35% for maximum growth and reproduction of tilapia, while Khattab et al. (2000) reported an optimum dietary CP level of 27%–37% depending on the amount of weight gain and the body length attained. In contrast, El-Sayed and Kawanna (2008) and de Oliveira et al. (2014) elucidated that increasing the dietary CP level of broodstock tilapia between 30% and 40% would significantly improve the growth and reproductive efficiency of the species. This wide range of recommendation for a very expensive nutrient in the fish diet makes it difficult to prepare specific feed that satisfies the needs of brood fish and a viable commercial-scale production. With this issue taken into consideration, Ribeiro et al. (2017) investigated the effect of different levels of CP (32%–50%) and found that the increase in CP levels in the diets of Nile tilapia brood fish did not bring significant improvement in the reproductive performance of males. However, after assessing larval growth and economic viability and the performance of males, it was recommended that using diets containing 44% CP for Nile tilapia brood fish is adequate.
A well-balanced broodstock fish diet composed of essential amino acids is crucial for enhancing reproduction (Table 1). Mazid et al., (1978) identified 10 essential amino acids for the normal growth of tilapia: arginine, histidine, isoleucine, leucine, lysine, methionine, phenylalanine, threonine, tryptophan, and valine. Deficiency in any of these amino acids can limit protein synthesis, growth, and metabolic activity, leading to reduced weight and reproductive potential (Furuya et al., 2023). Santiago and Lovell (1988) determined the quantitative requirements for these essential amino acids as a percentage of dietary protein. Moses et al. (2018) analyzed the amino acid composition of tilapia fish and found that glutamic acid and aspartic acid were the most concentrated amino acids. Ogunji et al. (2005) estimated the essential amino acid requirements for tilapia based on a dietary protein content of 33.32% and provided a specific concentration for each amino acid. Furuya et al. (2023) elaborated on the critical role of amino acids beyond protein synthesis and growth regulation. They were also found to improve reproductive performance, flesh quality, fillet yield, and health status. In addition to the enormous importance of amino acids in metabolic activity, they also have a vital role in biological oxidation, lipogenesis in a cell-specific manner, antioxidant reaction, cell sensing and signaling, improved health, and tissue pigmentation, as depicted by Li et al. (2021).
Table 1 Essential dietary amino acid (percent of dietary protein) requirements of Nile tilapia broodstock and their role.
Furthermore, some amino acids, e.g., arginine and glutamate, are essential in the regulation of hepatic glucose and lipid metabolism. Other amino acids including glutamate, glycine, and tyrosine are also involved in the release of pituitary hormones that regulate food intake and the reproductive behavior of fish (Andersen et al., 2013). Yue et al. (2015) also elaborated on the significant weight gain and increased growth rate of Nile tilapia fed dietary arginine levels up to 15.3 kg−1. The level of arginine has a substantial impact on the enhancement of the immunostimulatory potential of juvenile tilapia to improve their immune response and survival. By including these essential amino acids in the broodstock diet in optimal amounts, tilapia farmers can promote the optimal growth, reproductive performance, and overall health of their fish.
Furuya et al. (2023) elucidated three levels of CP (i.e., 350, 380, and 400 g kg−1 of the diet) that corresponded to different broodstock management systems. The CP level affects the amino acid requirements of fish, as higher protein levels may increase the demand for certain amino acids. An example is when rearing broodstock in earthen ponds, where they have access to natural feed such as plankton, insects, and detritus. This system may reduce the need for supplemental feed and amino acids, but it also poses challenges such as low seed quantity and quality, uncontrolled reproduction, and predation (El-Sayed and Kawanna, 2008). On the other hand, rearing broodstock in tanks with recirculating water and artificial feed may improve the seed quantity and quality, control the reproduction, and prevent predation, but it also requires higher feed and amino acid inputs, as well as more intensive management and maintenance. In contrast, rearing broodstock in brackish or marine water with artificial feed may expand the production area and market potential of Nile tilapia, but it also increases the stress and osmoregulation costs of the fish, as well as their amino acid requirements, especially lysine (de Oliveira et al., 2014).
Dietary lipids are a major source of essential fatty acids and highly digestible energy required by fish to attain optimal growth and development, improved reproductive performance, and health maintenance (Lim et al., 2011; Liang et al., 2022). Broodstock diets supplemented with oils and fatty acids that are rich in n-3 and n-6 improved the feed flavor, texture, and cell membrane permeability. Moreover, fatty acids play a significant role in the absorption of fat-soluble nutrients such as sterols and vitamins A, D, E, and K (Balfry and Higgs, 2001; Liang et al., 2022).
For many freshwater species like the Nile tilapia, the provision of dietary lipids, particularly essential fatty acids, is crucial for numerous physiological functions related to growth and reproduction as they cannot be synthesized in the body (Lim et al., 2011; Mandal et al., 2013). However, their ability to desaturate C18 fatty acids to long-chain polyunsaturated fatty acids (PUFAs), such as 18:2n-6 to arachidonic acid, 20:4n-6 and 18:3n-3 to eicosapentaenoic acid (EPA), and 20:5n-3 to docosahexaenoic acid (DHA), only requires linoleic (18:2n-6) or linolenic (18:3n-3) acid (Balfry and Higgs, 2001; Lim et al., 2011). On the contrary, many marine fishes are unable to desaturate fattyacids and have a dietary requirement of long-chain highly unsaturated fatty acids (HUFAs) (Lim and Webster, 2006). The quality and the quantity of the required essential fatty acids vary by species and the maturity stage of the fish. Hence, various studies revealed that the optimum levels of the essential dietary components of linolenic (n-3) and linoleic (n-6) acids could range between 0.5% and 1% for broodstock tilapia (El-Sayed et al., 2005).
Although there are variations in requirements due to species, sex, age, breeding stage, and other physiological conditions, the levels of dietary lipids significantly influence reproductive performance, such as the induction of secondary vitellogenesis and ovarian maturation, fecundity, the rate of egg hatching, the quality of larva, and the number of fry survival of many species, including tilapia (Lovell, 2009; Mandal et al., 2013; Liang et al., 2022). Numerous studies elucidated that a lipid level of 10%–20% in fish broodstock diet results in optimal growth without any accumulation of excess fatty tissue (Webster and Lim, 2002; Lim et al., 2011). Conversely, the provision of excess dietary lipids to broodstock fish would lead to the excess deposition of fat in the body cavity, which may adversely affect the growth, reproductive performance, value, and storage of processed products and, ultimately, the profitability of the culture system (Lim et al., 2013; Mandal et al., 2013).
The amount of dietary lipid administered to tilapia can be enhanced or diminished due to the sparing effects of proteins or carbohydrates for various activities, such as courtship, migration, and buoyancy (Lim et al., 2013). For example, increasing the level of dietary carbohydrates will replace the energy source from lipids and proteins. According to Jauncey (2000), reducing the level of protein from 33.2% to 25.7% and increasing the levels of dietary lipid and carbohydrate from 5.7% to 9.4% and from 31.9% to 36.9%, respectively, improved the growth and yield of broodstock tilapia. Similarly, decreasing the dietary protein level from 40% to 30% by increasing the dietary lipid level to 12% in the diet of Nile and blue tilapia hybrid resulted in improved growth.
On the other hand, the findings of Santiago and Reye (1993) and El-Sayed et al. (2005) elucidated that considerable amounts of dietary and supplemental lipids in the broodstock diet resulted in the increased number of fry per spawning fish and low number of deformed larvae. Correspondingly, among the different lipid-containing supplemental feeds, a diet with vegetable oils recorded a higher fry per spawner in fish (Table 2). The numbers of viable fry in the broodstock diet without supplemental lipid were negligible. It was shown that all diets with supplemental lipid triggered a significant crude fat content in the ovaries, testes, and female body of Nile tilapia. However, changes in the bodies of male broodstock were insignificant (Santiago and Reye, 1993; El-Sayed and Kawanna, 2008).
Table 2 Spawning frequency and fry production of Nile tilapia supplemented with different lipid-containing diets.
Limiting the fatty acids in the diet of tilapia broodstock can have a significant impact on their reproductive performance (Table 3). Several studies have demonstrated that the incorporation of specific lipid sources into the broodstock diet can enhance reproductive outcomes. For instance, Ng and Wang (2011) found that female Nile tilapia fed diets containing crude palm oil exhibited improved reproductive performance compared to those fed diets with fish oil or linseed oil. Similarly, Santiago and Reye (1993) observed that supplemental lipid sources, such as soybean oil and corn oil, increased the number of females that spawned and the total fry production in Nile tilapia broodstock.
Table 3 Essential fatty acids (percent of dietary fat) and their roles in the broodstock nutrition of Nile tilapia.
The importance of dietary linoleic (n-6) series fatty acids for tilapia has been highlighted by Lim et al. (2011). Takeuchi et al. (1983) suggested that vegetable oils rich in 18:2ω6, such as corn oil and soybean oil, are suitable dietary lipids for tilapia. These findings collectively indicate that limiting the fatty acids, particularly by incorporating specific lipid sources, can positively influence the reproductive performance of tilapia broodstock. Alves et al. (2021) elaborated on the positive effects of incorporating palm oil into the broodstock diet of Nile tilapia. Their study demonstrated that palm oil not only enhances growth and weight gain but also serves as a major source of fatty acids, with high levels of palmitic, oleic, and linoleic acids. These fatty acids were found to promote the growth rate, reproductive performance, larval quality, and survival rate of the juveniles (Torsabo et al., 2022).
Nile tilapia has an essential requirement of 0.5% linoleic acid (C18:2n-6) to improve reproductive performance. Santos et al. (2007) reported that a dietary level of 11.8% C18:2n-6 significantly improved vitellogenesis and fecundity. Similarly, Bahurmiz and Ng (2007) documented greater weight gain and improved fecundity and specific growth rate in Nile tilapia fed palm oil supplement. These studies collectively demonstrate that limiting the fatty acids in the diet of tilapia broodstock, particularly by incorporating specific lipid sources, can have a significant positive impact on their reproductive performance.
Carbohydrates are abundant but nonessential components in the broodstock diet that provide inexpensive energy to spare lipids and proteins for growth and reproduction (Azaza et al., 2020; Callet et al., 2020; Kumkhong et al., 2020). Compared to animal products, plant ingredients contain higher levels of carbohydrates. However, the cellulose and ligneous components in plant-derived carbohydrates make them indigestible to fish (Mandal et al., 2013). Therefore, incorporating excess amounts of carbohydrates in the broodstock diet would lead to physiological disturbance and metabolic stress (Kamalam et al., 2017; Kumkhong et al., 2020). Apart from the economic perspective and health-related issues in glucose-intolerant species, the provision of substantial amounts of carbohydrates in broodstock diet has an ecological benefit through the reduction of nitrogen excretion, which directly contributes to greenhouse gas emissions (Callet et al., 2020; Maas et al., 2020).
Due to its herbivore–omnivore feeding habit, broodstock tilapia has a greater chance of taking more plant materials exposed to higher carbohydrate contents (Mandal et al., 2013; Maas et al., 2020). This species is capable of utilizing dietary carbohydrates as the primary energy source and responds with poor fecundity and spawning efficiency (Kumkhong et al., 2021). This might be due to the low nutritional value of the diet for improving reproduction (Masrizal et al., 2015). Carbohydrates in the broodstock diet are used as a major source of energy for courtship, territorial defense, reproduction, mouth brooding, nest building, and osmoregulation, leaving only a small amount of energy for reproductive performance (El-Sayed and Kawanna, 2008; Lim et al., 2011; Masrizal et al., 2015; Maas et al., 2020). However, in the case of energy deficiency for these activities, the dietary or stored proteins or lipids will be catabolized for energy production in the sparing process (El-Sayed et al., 2003).
Furthermore, many authors reported that numerous broodstock species have the potential to use substantial amounts of carbohydrates during gametogenesis (Kumkhong et al., 2021). However, various factors including the origin of the carbohydrate, the amount of dietary carbohydrate in the diet, the physical state, and molecular complexity seriously affect its efficient utilization by broodstock fish (Suloma et al., 2017; Azaza et al., 2020). For instance, some broodstock species prefer to utilize polysaccharides and oligosaccharides, while other species prefer monosaccharaides for efficient use (Maas et al., 2020). Conversely, when the CP level in the broodstock diet decreases, maintaining an adequate amount of carbohydrate would reduce the adverse effect on reproduction (Callet et al., 2020). Although there is no specified amount for the carbohydrate requirement of broodstock tilapia, many authors have suggested a dietary level of 30%–40% digestible carbohydrate as optimal (El-Sayed and Kawanna, 2008; Mandal et al., 2013; Maas et al., 2020).
Despite the reduced utilization by numerous broodstock fishes, a broodstock diet composed of quality carbohydrates enhances reproductive performance (Table 4). The reduced utilization of carbohydrates by fishes has been ascribed to the lower concentration of the hexokinase enzyme (which is used for digestion) (El-Sayed and Garling, 1988). Shiau and Chen (1993) showed that carbohydrates such as starch or dextrin can spare protein when the dietary protein level in tilapia diets is low. Olvera-Novoa et al. (2002) found that a sunflower seed meal can be used as a protein replacement for fishmeal in tilapia diets, with 10% and 20% substitution levels providing the best growth and feeding efficiency. Finally, Ng and Romano (2013) provided a comprehensive overview of tilapia nutrition and feeding management, discussing the importance of carbohydrates and other nutrients throughout the culture cycle. Excess carbohydrate in the diet of broodstock fish could result in huge fat deposition and overstimulation of the lipogenic enzyme El-Sayed and Garling (1988).
Table 4 Essential carbohydrates (percent of dietary carbohydrate) and their roles in the broodstock nutrition of Nile tilapia.
Vitamins are among the most essential micronutrients required by broodstock tilapia (Ng et al., 2006; Sarmento et al., 2017). Since they cannot be synthesized in the body due to the lack of the gulonolactone oxidase enzyme, the only route wherein fish can access vitamins is through formulated feeds or supplements (Suloma et al., 2017). The most common and basic vitamin supplements used in the broodstock diet are ascorbic acid or vitamins C, D, and E, which play important roles in various metabolic activities such as detoxification reaction, steroid synthesis, collagen formation, and stress tolerance (Ng et al., 2006; Sarmento et al., 2017; Zhang et al., 2021). Furthermore, the inclusion of dietary vitamins in broodstock tilapia diet promotes the release of luteinizing hormone (LH) and follicle-stimulating hormone (FSH), which improve vitellogenesis, spawner number, egg hatching, and fry survival rates with improved immune response in females, as well as the synthesis and maintenance of normal sperm, increased motility, and reduced infertility in males (Sarmento et al., 2017; Zhang et al., 2021).
The optimal dietary vitamin requirements of Nile tilapia ranged between 25 and 119 mg/kg diet (Table 2). However, the exact values vary due to species-specific demands, the presence of antioxidants, and several environmental parameters (Durigon et al., 2019). For example, in brood tilapia, vitamin C degradation will be accelerated under conditions of hyperoxia (Ng et al., 2006), whereas the dietary vitamin E requirements are highly influenced by the dietary lipid levels (Ng et al., 2006).
Nutritionally, minerals and trace elements are those inorganic substances required at the micro or macro level for normal physiological functioning, such as in soft tissue and structural skeletal formation, nerve impulse transmission, osmoregulation, growth, reproduction, and healthy body (Lovell, 2009; Mandal et al., 2013). Moreover, minerals are vital components of many enzymes, hormones, and various metabolic activities (Jauncey, 2000; Durigon et al., 2019). Fishes absorb essential minerals either from their diet or from water. Hence, excess or deficient provision of minerals to fish will affect all of the benefits mentioned above (Saenz, 2021). Conversely, optimal dietary supplements of minerals and trace elements could also be wasted through the interactions and formations of complex products. Thus, their deficiency will inhibit reproductive performance (Lall and Kaushik, 2021).
The dietary provision of minerals and trace elements plays a significant role in improving reproductive performance (Jauncey, 2000). For instance, Ca is essential during the sexual maturity of females and is used as a major binding component to the vitellogenin in egg and Ca binding protein. It has been shown that optimal supplementation of Fe, Cu, Zn, and Mg is essential to the regulation of enzymatic and hormonal activities, an enhanced immune system, vitamin synthesis, and improved growth. In contrast, deficient or excess provisions beyond the required limits will cause toxicity and have adverse effects on the physiology of the fish (Makwinja and Geremew, 2020). Despite the paucity of information and the lack of common agreement on quantifying the level of dietary requirement, Ng and Romano (2013) recommended provision of trace elements within a certain range to enhance hormonal and enzymatic activities (Table 5). For instance, optimal supplementation of Se is an essential constituent of the glutathione peroxidase (GPx) enzyme, which is an antioxidant against reactive oxygen species (ROS) and can detoxify many heavy metals (Durigon et al., 2019).
Table 5 Optimal requirements of vitamins, minerals, and trace elements in the broodstock Nile tilapia diet.
Functional additives such as enzymes, hormones, prebiotics, probiotics, and synbiotics are substances that exert benefits for a specific biological function (Gule and Geremew, 2022; Hernandez de-Dios et al., 2022). Hence, supplementing broodstock diet with these additives in different quantities will improve the progeny quality, egg viability, hatching rate, and larval survival. Furthermore, functional additives improve the efficiency of commercial diets in meeting the required nutritional demands by reducing the anti-nutritional factor in the diet (Sajjadi and Carter, 2004). However, the provision of some additives like hormones beyond the optimum level will bring about malformations in larvae (Hernandez de-Dios et al., 2022).
The reproductive performance of fish can potentially be improved through the application of functional feed additives. Probiotics as a feed additive have been demonstrated to have a positive impact on reproductive performance through improving the egg diameter, fecundity, fertilization, egg hatching rate, and juvenile survival rate in fish. The effect of probiotics in indicating the responsiveness of incompetent follicles to maturation-inducing hormones and changing the oocyte chemical composition during vitellogenic development is well established (Sumon et al., 2022). The positive role of probiotics in testicular cells has also been validated through their role in upregulating the transcription levels of leptin and the bdnf and dmrt1 genes, hence facilitating spermatogenesis. The importance of phytobiotics as an additive in aquaculture feed is increasingly being recognized, i.e., as one agent that improves the reproductive performance of fishes. For example, the supplementation of spirulina in the diet of tilapia was found to have an influence by improving fecundity, the gonadosomatic index (GSI), fry production, and survival (Wahbi and Sangak, 2017). Dias et al. (2020) administered dietary Baccillus subtilis at 1010 CFU g−1 for 90 days, resulting in improved egg production and fry survival rate and in the increased number of O. niloticus spawning females, which led to economic benefits. Similarly, Pronina et al. (2022) incorporated a probiotic “Subtilis-S” at 1 g/kg feed and found increased survival rates of fish, enhanced immunity, and increased fertility of breeding tilapia. Tawfik et al. (2017) reported that tilapia fish fed a diet with various concentrations of zinc oxide nanoparticles showed increased total protein concentrations, improved growth performance, and immunity.
The provision of functional additives to broodstock fish improved the digestibility and availability of minerals, improving reproductive performance. For instance, the enzymes phytase, carbohydrase, protease, and lipase are some of the most common functional additives used to increase the digestibility of the diet, with anti-nutritional factors that reduce the availability of proteins, carbohydrates, lipids, and minerals such as P and Ca (Alemayehu et al., 2018; Gule and Geremew, 2022). Similarly, various functional hormones and steroids, including growth hormone, thyroxin, pituitary hormone, and gonadotropin-releasing hormone (GnRH), are common additives in broodstock species used to improve growth, induce maturity for captive breeding, and reverse sex for monoculture (Celia et al., 2018). Furthermore, administering prebiotic, probiotic, and synbiotic supplements to broodstock species in adequate amounts will provide health benefits and enhance the performance and overall well-being of fish (Mugwanya et al., 2022).
Physical, chemical, and biological factors strongly affect the reproductive performance of broodstock fish. However, the influence of nutrient composition, such as the energy, lipid, and protein levels, in the diet is an inevitable limiting factor for the maturation, fecundity, and survival of larvae (Masrizal et al., 2015; Ribeiro et al., 2017). This includes the availability of essential nutrients that initiate gametogenesis, growth, and reproductive control. Various biomolecules and micronutrients only required in minute amounts play fundamental roles in achieving successful reproductive performance (Kumar et al., 2018). Nevertheless, dietary nutrient deficiency would lead to failure in reproduction, poor hormonal functioning, and delayed puberty, resulting in common reproductive dysfunctions (Varghese et al., 2009; Mylonas et al., 2010; Masrizal et al., 2015).
Fecundity refers to the total number of eggs in the ovaries of female fish prior to the next spawning season, which is beneficial to determining egg quality and quantity (Masrizal et al., 2015). However, an imbalance in the essential nutrients from broodstock diets, coupled with a decreased quantity of feed, reduces fecundity (Izquierdo et al., 2001). For instance, a reduced lipid level in the diet of broodstock fish affects the secretion of various biochemicals from the pituitary gland, which restricts egg formation (Kumar et al., 2019). Similarly, the levels of essential dietary fatty acids have substantial impacts on the fecundity of broodstock species (Palacios et al., 1995; Bhujel, 2000). Furthermore, the amounts of n-3 HUFA and n-6 PUFA in the diet of broodstock tilapia have been shown to improve the spawning frequency and fry production of female fish by influencing the fatty acid composition of the gonads, which are responsible for frequent egg production (Izquierdo et al., 2001; Varghese et al., 2009).
The frequency of spawning, number of fry per spawning, and the total fry production of tilapia were higher when the fish was supplied with soybean oil, which is rich in n-6 fatty acid. Various broodstock fishes utilize the lipid reserve in the muscle and other parts of their body during ovarian maturation. The fatty acid content and composition in the body of female fish vastly affect the quality of eggs in the gonad. Hence, direct correlations between the levels of n-3 HUFA and n-6 PUFA in the diet of many broodstock species including the Nile tilapia have been observed in increased EPA and DHA concentrations, which improved fecundity (Izquierdo et al., 2001). Therefore, decreased amounts of dietary fatty acids in the body of females lead to poor regulation of the production of eicosanoids, particularly the secretion of prostaglandins that are involved in gonad development and the ovulation process (Varghese et al., 2009).
At the opposite extreme, Lim et al. (2011) reported on the insignificant effect of increased dietary fatty acid content on the fecundity of some broodstock species. The decline in fecundity was ascribed to the surplus concentrations of essential n-3 fatty acids in the diet that increased the levels of EPA and DHA. Consequently, this affected various chemical secretions of the pituitary gonad, which lowered the levels of steroidogenic and gonadotropin hormones that increase puberty and fecundity (Palacios et al., 1998; Volkoff and London, 2018).
On the other hand, the provision of dietary protein above the threshold value is essential to inducing a pronounced fecundity and to improving the ovum size, protein content of the egg, number of eggs per spawning, and the spawning intervals in broodstock tilapia (Bhujel, 2000). Supplying broodstock species with higher dietary protein encourages the GnRH that induces the pituitary gland to secrete LH and FSH and to release gonadotropin hormone, which cause ovaries to produce eggs. Furthermore, supplying broodstock tilapia with a high-energy diet leads to the noticeable development of oocytes, higher fecundity with increased egg diameter, and improved fertility and hatching rates of eggs (Masrizal et al., 2015). Conversely, inadequate dietary protein affects the secretion of GnRH and increases the period of maturation, leading to a decline in reproductive performance, i.e., reduced fecundity and egg viability (Volkoff and London, 2018). Hence, a lower dietary protein leads to ovarian recrudescence, prolonged intervals between spawning, and a complete halt in reproduction (Gunasekera et al., 1996; Bhujel, 2000).
The fertilization of eggs in aquatic ecosystems is highly influenced by sperm motility and the chemistry of the water, i.e., salinity and hardness, that gradually make it more difficult for the egg to be penetrated. Moreover, essential fatty acids, e.g., EPA and arachidonic acid, in the sperm are strongly correlated with motility and fertilization. Conversely, a delay in the time of spermiation or ejaculation reduces the rate of fertilization as the egg stays in the water for a while, thus making fertilization more difficult (Izquierdo et al., 2001; Furuita et al., 2002; Kumar et al., 2018). Essential fatty acids in the diet increase the secretion of prostaglandins, which improve the arousal and sexual behavior and the sperm quality of males. For example, the levels of dietary n-3 and n-6 fatty acids played a significant role in membrane integrity, sperm resistance, and egg fertilizability (Varghese et al., 2009).
Gunasekera et al. (1996) elucidated the influence of female nutrition on the fertilizability, hatchability, and larval growth rate of tilapia, indicating significant body size variations due to the amount of CP in the diet. A smaller body size was reached by females fed 10% CP compared to those fed 20% and 35% CP diets. Similarly, female tilapia fed higher amounts of CP showed high percentages of spawning and fertilization. For instance, broodstock tilapia fed 35% and 20% CP spawned and their eggs were fertilized; however, those that were fed 10% CP spawned late and their eggs were not fertilized, as some died after spawning.
It has been shown that broodstock supplied with a decreased protein content exhibited reduced mating behavior and produced fertilized eggs with deformed larvae and lower yolk sac resorption. However, as the amount of supplemental protein in the diet increased, both the fertilizability and hatchability of eggs increased considerably (Abidin et al., 2006). Moreover, broodstock supplied with a higher amount of protein in the diet had higher numbers of normal larvae with reduced number of days taken for hatching and yolk sac resorption. For instance, Gunasekera et al. (1996) explained that fishes supplied diets with 35% and 20% protein showed significantly higher percentages of hatchability, yolk sac resorption, and normal larvae compared to those given 10% protein.
Additional essential nutrients that improve the fertilization of broodstock fish egg and the development of embryo and larvae include vitamins A, C, and E and ascorbic acid. These vitamins function as antioxidants that protect the lipid peroxidation of PUFAs by volatile ROS and improve the steroidogenesis and vitellogenesis activities that protect sperm cells during spermatogenesis. In addition, they protect sperm cells from lipid peroxidation, which is essential for sperm motility (Furuita et al., 2002).
For the normal embryonic development of fertilized eggs, the contributions of essential dietary nutrients such as lipids and vitamins are necessary. The increased level of HUFA such as n-3 in the diet of broodstock reduces the number of morphologically deformed embryos (Palacios et al., 1998; Kumar et al., 2018). Izquierdo et al. (2001) reported the strong association between egg quality, normal embryonic development, and the essential fatty acid content of a diet. Because of its phospholipid component, fatty acids play a remarkable role in the structural makeup of numerous biomembranes, enzymes, and cells. Supplementation of varied fatty acid levels, such as 0.5%, 0.8%, 1.5%, and 2% of n-3 HUFA, to broodstock fish revealed that optimum values (>1%) are essential for the normal early embryonic developmental stages (Furuita et al., 2002).
Furthermore, deficiency in dietary vitamins C and E in broodstock fish results in immature gonads, reduced hatching rates, and lower fry survival rates. In contrast, increased levels of vitamins lead to the increased percentage of buoyant eggs, improved hatching rates, and reduced number of deformed larvae. A nutritive broodstock diet reduces abnormality and improves egg quality (Sarmento et al., 2017; Suloma et al., 2017; Kumar et al., 2018). Farías et al. (2011) also argued that considerable supplementation of dietary protein in broodstock feed improved the fertilization rate of eggs with increased survival rates of larvae. CP is a main component of yolk that is essential for the nourishment of the embryo during development. Thus, quality protein consisting of essential amino acids in the diet exerts a significant influence on the embryonic development of larvae.
All essential maternal reserves are transferred into the oocytes during the early stages of ovarian development. Thereafter, the provision of high-quality nutritive feed has a significant role in the improvement of larval quality through increased survival, growth, and swim bladder inflation (Mazorra et al., 2003). Hence, the quality of broodstock diet and its nutritional composition highly determine larval quality until the beginning of exogenous feeding. Izquierdo et al. (2001) showed that increasing the level of n-3 HUFA from 12% to 18% in many broodstock species enhanced the hatchability and survival of larvae. Subsequently, the larval quality, including larval weight, deformity, and resistance to osmotic shock, improved. However, excess supplementation (>2%) of HUFAs in the diet could also have a negative impact on larval survival due to an increased demand for antioxidant nutrients such as α-tocopherol (Palacios et al., 1998; Furuita et al., 2002).
Essential fatty acids including DHA (22:6n:3) and EPA (20:5n:3) play substantial roles in the quality and survival of larvae. Similarly, arachidonic acid, which contributes to eicosanoid production, also has an inevitable contribution during egg development (Mazorra et al., 2003). Therefore, apart from genetic variability and the non-optimal feeding behavior of female broodstock, the nutritional composition of the diet highly determines the fecundity, embryo development, and egg and larval quality.
Broodstock nutrition can influence the natural breeding behavior of tilapia, such as courtship, spawning, territoriality, parental care, and mouth brooding. Dietary protein levels can affect the vitellogenesis and ovarian maturation of female tilapia, which in turn can affect their readiness to spawn and mate with males (Rana, 1988). Dietary protein deficiency can suppress the ovarian development and vitellogenesis (egg yolk formation) in females, delaying spawning readiness and reducing egg quality (Orlando et al., 2017). Dietary lipids can also provide energy for the reproductive activities of both sexes, such as aggressive behavior, nest building, and oral incubation of eggs and fry (Torsabo et al., 2022). For example, with adequate energy reserves from dietary lipids, males can exhibit stronger courtship displays and territorial aggression. Deficient intake of lipids can hinder nest building, egg incubation, and aggressive behavior in males (Hubbard, 2018). Deficiencies in crucial vitamins and minerals can weaken the immune system, disrupt hormonal balance, and heighten stress susceptibility in tilapia (Makwinja and Geremew, 2020). This can significantly impact the reproductive success and survival in natural environments (Wang et al., 2023).
Broodstock nutrition can also affect the natural breeding biology of tilapia, such as the timing, frequency, duration, and synchrony of spawning; the number, size, and quality of eggs and fry; and the sex ratio and growth of offspring. Dietary protein and lipid levels can affect the GSI, fecundity, and egg size of female tilapia, which can affect the number and quality of the offspring produced (Al-Hafedh et al., 1999; Orlando et al., 2017). The provision of adequate dietary essential fatty acids to tilapia broodstock directly influences the various stages of reproduction, starting from embryonic development to the survival of fry. This influence significantly contributes to the species’ natural breeding biology by improving reproductive success and promoting healthier offspring (Zadeh et al., 2008). Vitamins such as vitamins C and E (Sarmento et al., 2017; Suloma et al., 2017; Kumar et al., 2018) and minerals like zinc (Ng and Romano, 2013; Rohani et al., 2022) can contribute to egg viability, fertilization success, and larval quality. They can also influence the growth, pigmentation, and flesh quality of offspring.
In summary, the nutritional composition of the diet provided to tilapia broodstock significantly impacts their breeding behavior, reproductive biology, and the quality and quantity of their offspring. A well-designed nutritional regime is pivotal to ensuring successful breeding and the health of the tilapia population.
Over the past 20 years, considerable progress has been made in the study of fish nutrition. These include the documentation of nutritional data and the development of effective feeds for many species. However, the broodstock of Nile tilapia faces several challenges in having their nutritional needs met. Hence, future studies should focus on overcoming the limited availability and high cost of quality feeds by giving priority to the nutritional quality and bioavailability of local feedstuffs and to the standardization of methods enhancing the nutritional quality of other feeds and the feeding practice. Furthermore, the interactions between nutrition, disease resistance, and immunological function should be explored. It is crucial to investigate in detail the benefits of functional additives, harmful toxicants, and the associated impacts of anti-nutritional factors. Finally, comprehensive understanding on the nutritional strategy for broodstock Nile tilapia, such as selecting and breeding improved traits, optimizing the feed formulation and feeding frequency, supplementing the feed with essential nutrients and additives, and monitoring and evaluating the nutritional status and performance of broodstock, is necessary.
The lack of balanced, nutritive, and formulated diet that improves rapid body growth and other reproductive conditions is the main constraint in commercial aquaculture. The dietary nutrient composition, including lipids and fatty acids, vitamins, minerals, proteins, carbohydrates, and feed size, has a strong influence on the breeding condition and progeny of many broodstock species such as the Nile tilapia. Provision of these essential nutrients to broodstock tilapia has a significant impact on vitellogenesis, which might directly improve the egg quality, reduced size at first maturity, and progeny development. Broodstock fishes supplied with a diet composed of increased CP; essential amino acids such as arginine, leucine, lysine, methionine, phenylalanine, and tryptophan; and lipids have improved reproductive performance, while those supplied with decreased CP, amino acids, and HUFAs have reduced performance with significant larval deformity. For successful and profitable aquaculture production, further research focusing on the formulation of a nutritive diet and the implications of each nutritional component, e.g., vitamins, minerals, and trace elements, toward the reproductive performance of broodstock species is recommended.
FE: Conceptualization, Data curation, Methodology, Writing – original draft, Writing – review & editing. AG: Conceptualization, Supervision, Validation, Writing – review & editing.
The author(s) declare that no financial support was received for the research, authorship, and/or publication of this article.
The authors declare that the research was conducted in the absence of any commercial or financial relationships that could be construed as a potential conflict of interest.
All claims expressed in this article are solely those of the authors and do not necessarily represent those of their affiliated organizations, or those of the publisher, the editors and the reviewers. Any product that may be evaluated in this article, or claim that may be made by its manufacturer, is not guaranteed or endorsed by the publisher.
Abidin M. Z., Hashim R., Chien A. C. S. (2006). Influence of dietary protein levels on growth and egg quality in broodstock female bagrid catfish (Mystus nemurus Cuv. & Val.). Aquac. Res. 37, 416–418. doi: 10.1111/j.1365-2109.2005.01382.x
Agnese J. F., Adepo-Gourene B., Abban E. K., Fermon Y. (1997). Genetic differentiation among natural populations of the Nile tilapia Oreochromis niloticus (Teleostei, Cichlidae). Heredity 79, 88–96. doi: 10.1038/hdy.1997.126
Alemayehu A. T., Geremew A., Getahun A. (2018). The role of functional feed additives in tilapia nutrition. Fisheries Aquac. J. 9, 249. doi: 10.4172/2150-3508.1000249
Al-Feky S. S. A., El-Sayed A.-F. M., Ezzat A. A. (2014). Dietary taurine improves reproductive performance of Nile tilapia (Oreochromis niloticus) broodstock. Aquac. Nutt 22 (2), 392–399. doi: 10.1111/anu.12256
Al-Hafedh Y. S., Siddiqui A. Q., Al-Saiady M. Y. (1999). Effects of dietary protein levels on gonad maturation, size and age at first maturity, fecundity and growth of Nile tilapia. Aqucul. Int. 7, 319–332. doi: 10.1023/A:1009276911360
Alves L., Rocha J. D. M., Cardoso M., de Fritas J. M. A., Feiden A., Boscolo W. R. (2021). Palm oil in diets for Nile tilapia (Oreochromis niloticus) post-larvae. Res. Soc Devel. 10, 1–10. doi: 10.33448/rsd-v10i14.22099
Andersen S. M., Holen E., Aksnes A., et al. (2013). Dietary arginine affects energy metabolism through polyamine turnover in juvenile Atlantic salmon (Salmon salar). Br. J. Nutr. 110, 1968–1977. doi: 10.1017/S0007114513001402
Azaza M. S., Saidi S. A., Dhraief M. N., EL-feki A. (2020). Growth performance, nutrient digestibility, hematological parameters, and hepatic oxidative stress response in juvenile Nile tilapia, Oreochromis niloticus, fed carbohydrates of different complexities. Animals 10, 1913. doi: 10.3390/ani10101913
Bahurmiz O. M., Ng W. K. (2007). Effects of dietary palm oil source on growth tissue fatty acid composition and nutrient digestibility of red hybrid tilapia, Oreochromis sp. raised from toking to market size. Aquaculture 262, 262. doi: 10.1016/0305-0491(86)90135-5
Balfry S. K., Higgs D. A. (2001). “Influence of dietary lipid composition on the immune system and disease resistance of finfish,” in Nutrition & Fish health (Haworth Press, Binghamton, New York), 213–234.
Bell M. V., Henderson R. J., Sargent J. R. (1986). The role of polyunsaturated fatty acids in fish. Comp. Biochem. Physiol. 83 (4), 711–719. doi: 10.1016/0305-0491(86)90135-5
Bhujel R. C. (2000). A review of strategies for the management of Nile tilapia (Oreochromis niloticus) broodfish in seed production systems, especially hapa-based systems. Aquaculture 181, 37–59. doi: 10.1016/S0044-8486(99)00217-3
Bhujel R. C., Little D. C., Hossain A. (2007). Reproductive performance and the growth of pre-stunted and normal Nile tilapia (Oreochromis niloticus) broodfish at varying feeding rates. Aquaculture 273, 71–79. doi: 10.1016/j.aquaculture.2007.09.022
Callet T., Hu H., Larroquet L., Surget A., Liu J., Plagnes-Juan E., et al. (2020). Exploring the impact of a low-protein high-carbohydrate diet in mature broodstock of a glucose-intolerant teleost, the rainbow trout. Front. Physiol. 11. doi: 10.3389/fphys.2020.00303
Carvalho P. L. P. F., Koch J. F. A., Cintra F. T., Fernandes A. C. Jr., Sartori M. M. P., Barros M. M., et al. (2018). Available phosphorus as a reproductive performance enhancer for female Nile tilapia. Aquaculture 486, 202–209. doi: 10.1016/j.aquaculture.2017.12.023
Celia A. H., Almeida F. L., Reyes F. G. R. (2018). A review on the use of hormones in fish farming: Analytical methods to determine their residues. CyTA. J. @ Food 16, 679–691. doi: 10.1080/19476337.2018.1475423
Chetoui I., Ghribi F., Bejaoui S., Hachana S., El Cafsi M., Azaza M. S. (2022). Incorporation of ω3 fatty acids in the diets of Nile tilapia juvenile (Oreochromis niloticus L.): Effects on growth performance, fatty acid composition, and tolerance to low temperature. Trop. Anim. Health Production 54, 401. doi: 10.1007/s11250-022-03394-2
Chong A. S. C., Ishak S. D., Osman Z., Hashim R. (2004). Effect of dietary protein level on the reproductive performance of female swordtails Xiphophorus helleri (Poeciliidae). Aquaculture 234, 381–392. doi: 10.1016/j.aquaculture.2003.12.003
de Oliveira M. M., Ribeiro T., Orlando T. M., de Oliveira D. G. S., Drumond M. M., de Freitas R. T. F., et al. (2014). Effects crude protein levels on female Nile tilapia (Oreochromis niloticus) reproductive performance parameters. Anim. Rep. Sci. 150, 62–69. doi: 10.1016/j.anireprosci.2014.08.006
Dias D. C., Furlaneto F. P. B., Sussel F. R., Tachibana L., Goncalves G. S., Ishikawa C. M., et al. (2020). Economic feasibility of probiotic use in the diet of Nile tilapia, Oreochromis niloticus, during the reproductive period. Anim. Sci. 42, e47960. doi: 10.4025/actascianimsci.v42i1.47960
Durigon E. G., Kunz D. F., Peixoto N. C., Uczay J., Lazzari R. (2019). Diet selenium improves the antioxidant defense system of juveniles Nile tilapia (Oreochromis niloticus L.). Braz. J. @ Biol. 79, 527–532. doi: 10.1590/1519-6984.187760
El-Sayed A. M., Garling D. M. Jr. (1988). Carbohydrates-to-lipids ratio in diets for Tilapia Zilli fingerlings. Aquaculture 73, 157–163. doi: 10.1016/0044-8486(88)90050-6
El-Sayed A. M., Kawanna M. (2008). Effects of dietary protein and energy levels on spawning performance of Nile tilapia (Oreochromis niloticus) broodstock in a recycling system. Aquaculture 280, 179–184. doi: 10.1016/j.aquaculture.2008.04.030
El-Sayed A. M., Mansour C. R., Altaf A., Ezzat A. A. (2005). Effects of dietary lipid source on spawning performance of Nile tilapia (Oreochromis niloticus) broodstock reared at different water salinity. Aquaculture 248, 187–196. doi: 10.1016/j.aquaculture.2005.04.024
El-Sayed A. M., Mansour C. R., Ezzat A. A. (2003). Effects of dietary protein level on spawning performance of Nile tilapia (Oreochromis niloticus) broodstock reared at different water salinities. Aquaculture 220, 619–632. doi: 10.1016/S0044-8486(02)00221-1
Farías A., Navarro J. C., Cerna V., Pino S., Uriarte I. (2011). Effect of broodstock diet on the fecundity and biochemical composition of eggs of the Patagonian red octopus (Enteroctopus megalocyathus Gould 1852). Cienc. Marinas 37, 11–21. doi: 10.7773/cm.v37i1.1736
Furuita H., Tanaka H., Yamamoto T., Suzuki N., Takeuchi T. (2002). Effects of high levels of n3 HUFA in broodstock diet on egg quality and egg fatty acid composition of Japanese flounder. Paralichthys olivaceus. Aquaculture 210, 323–333. doi: 10.1016/S0044-8486(01)00855-9
Furuya W. M., Cruz T. P. D., Gatlin D. M. III (2023). Amino acid requirements for Nile tilapia. Animals 13, 900. doi: 10.3390/ani13050900
Gebre-tsadik G., Bart A. N. (2007). Effects of feeding, stocking density and water-flow rate on fecundity, spawning frequency and egg quality of Nile tilapia, Oreochromis niloticus (L.). Aquaculture 272, 380–388. doi: 10.1016/j.aquaculture.2007.08.040
Gule T. T., Geremew A. (2022). Dietary strategies for better utilization of aqua feeds in Tilapia farming. Aquac. Nutt. 2022, 1–11. doi: 10.1155/2022/9463307
Gunasekera R. M., Shim K. F., Lam T. J. (1996). Influence of protein content of broodstock diets on larval quality and performance in Nile tilapia, Oreochromis niloticus (L.). Aquaculture 146, 245–259. doi: 10.1016/S0044-8486(96)01380-4
Han H., Wang Z., Wang J., Wang T., Li Y., Guan D., et al. (2021). Impact of high dietary cornstarch level on growth, antioxidant response and immune status in GIFT tilapia, Oreochromis niloticus. Sci. Rep. 11, 6678. doi: 10.1038/s41598-021-86172-8
Hernandez de-Dios M. A., Tovar-Ramírez D., García D. M., Galaviz-Espinoza M. A., Zarco M. S., Maldonado García M. C., et al. (2022). Functional additives as a boost to reproductive performance in marine fish: A review. Fishes 7, 262. doi: 10.3390/fishes7050262
Hoseini S. M., Perez-Jimenez A., Costas B., Azeredo R., Gesto M. (2019). Physiological roles of Tryptophanin Teleosts: current knowledge and perspectives for future studies. Rev. Aquac. 11, 3–24. doi: 10.1111/raq.12223
Hubbard P. C. (2018). “Pheromones, fish,” in Encyclopedia of reproduction (University of Algarve, Faro, Portugal: Elsevier), 458–464. doi: 10.1016/B978-0-12-809633-8.20592-X
Izquierdo M. S., Fernandez-Palacios H., Tacon A. G. J. (2001). Effect of broodstock nutrition on reproductive performance of fish. Aquaculture 197, 25–42. doi: 10.1016/S0044-8486(01)00581-6
Jauncey K. (2000). “Nutritional requirements,” in Tilapias: biology and exploitation, vol. 25 . Eds. Beveridge M. C. M., McAndrew B. J. (Dordrecht), 327–375. doi: 10.1007/978-94-011-4008-9_9
Kamalam B. S., Medale F., Panserat S. (2017). Utilisation of dietary carbohydrates in farmed fishes: New insights on influencing factors, biological limitations and future strategies. Aquaculture 467, 3–27. doi: 10.1016/j.aquaculture.2016.02.007
Khattab Y. A. E., Ahmad M. H., Shalaby A. M. E., Abdel - Tawwab M. (2000). Response of Nile tilapia (Oreochomis niloticus) from different locations to different dietary protein levels. EJABF 4, 295–311.
Kumar M., Gupta G. V., Sharma S. (2018). Role of broodstock nutrition on fish reproductive performance. Aqua Star 3, 24–26.
Kumar S., Sahu N. P., Ranjan A., Gupta S., Sardar P., Kumar M., et al. (2019). Optimization of de-oiled rice bran inclusion level in the diet of labeo rohita: Effect on growth performance, nutrient utilization, insulin-like growth factors i and II gene expression. Aquacul. Nutr. 00, 1–9. doi: 10.1111/anu.12873
Kumkhong S., Marandel L., Plagnes E., Veron V., Panserat S., Boonanuntanasarn S. (2022). Short initial period of high carbohydrate feeding improves nutrient utilisation in juvenile Nile tilapia (Oreochromis niloticus) fed a high carbohydrate die. Aquaculture 561, 738661. doi: 10.3390/ani11092711
Kumkhong S., Marandel L., Plagnes-Juan E., Veron V., Panserat S., Boonanuntanasarn S. (2020). Early feeding with hyperglucidic diet during fry stage exerts long-term positive effects on nutrient metabolism and growth performance in adult tilapia (Oreochromis niloticus). J. Nat. Sci. 9, 1–14. doi: 10.1017/jns.2020.34
Kumkhong S., Marandel L., Plagnes-Juan E., Veron V., Panserat S., Boonanuntanasarn S. (2021). Glucose injection into the yolk influences intermediary metabolism in adult Nile tilapia fed with high levels of carbohydrates. Animal 15, 100347. doi: 10.1016/j.animal.2021.100347
Lall S. P., Kaushik S. J. (2021). Nutrition and metabolism of minerals in fish. Animals 11, 2711. doi: 10.3390/ani11092711
Li X., Zheng S., Wu Z. (2021). Nutrition and functions of amino acids in fish. Adv. Exp. Med. Biol. 1285, 133–168. doi: 10.1007/978-3-030-54462-1_8. Springer.
Li X., Zheng S., Wu Z. (2022). Dietary L-Arginine supplementation of tilapia (Oreochromis niloticus) alters the microbial population and activates intestinal fatty acid oxidation. Amino Acids 54, 339–351. doi: 10.1007/s00726-021-03018-3
Liang X., Luo X., Lin H., Han F., Qin J. G., Chen L., et al. (2022). Effects and mechanism of different phospholipid diets on ovary development in female broodstock Pacific white shrimp, Litopenaeus vannamei. Front. Nutr. 9. doi: 10.3389/fnut.2022.830934
Lim S.-J., Oh D.-H., Khosravi S., Cha J.-H., Park S.-H., Kim K.-W., et al. (2013). Taurine is an essential nutrient for juvenile parrot fish oplegnathus fasciatus. Aquaculture 414-415, 274–279. doi: 10.1016/j.aquaculture.2013.08.013
Lim C., Yildirim-Aksoy M., Klesius P. (2011). Lipid and fatty acid requirements of tilapias. North Amer. J. Aquac. 73, 188–193. doi: 10.1080/15222055.2011.579032
Lim C., Webster C. D. (2006). “Nutrient requirements,” in Tilapia: Biology, culture and nutrition. Eds. Lim C., Webster C. D. (Binghamton, New York: The Haworth Press, Inc), 469–501. doi: 10.1007/s10499-007-9095-0
Lind C. E., Agyakwah S. K., Attipoe F. Y., Nugent C., Crooijmans R. P. M. A, Toguyeni A. (2019). Genetic diversity of Nile tilapia (Oreochromis niloticus) throughout West Africa. Sci. Rep. 9, 16767. doi: 10.1038/s41598-019-53295-y
Lovell T. (2009). Nutrition and feeding of fish (New York: Auburn University, Chapman and Hall), 267.
Maas R. M., Verdegem M. C. J., Wiegertjes G. F., Schrama J. W. (2020). Carbohydrate utilisation by tilapia: a meta-analytical approach. Rev. Aquaculture 12, 1851–1866. doi: 10.1111/raq.12413
Mabroke R. S., Tahoun A. M., Suloma A., El-Haroun E. R. (2013). Evaluation of meat and bone meal and mono-sodium phosphate as supplemental dietary phosphorus sources for broodstock Nile tilapia (Oreochromis niloticus) under the conditions of Hapa-in- pond system. Turkish J. Fisheries Aquat. Sci. 13, 11–18. doi: 10.4194/1303-2712-v13_1_02
Makwinja R., Geremew A. (2020). Roles and requirements of trace elements in tilapia nutrition: Review. Egypt. J. Aqua. Res. 46, 281–287. doi: 10.1016/j.ejar.2020.05.001
Mandal S. C., Armaan U., Muzaddadi A. U., Das P. (2013). Broodstock management of Indian major carps with reference to its nutrition. Adv. Fish Res. 6, 65–87.
Marty R. (2003). Feeding tilapia in intensive recalculating system (East Lansing: United State, Department of Agriculture, Agricultural Research Service, Michigan State University).
Masrizal, Udni Z., Zein M., Bulanin U. (2015). Effect of energy, lipid and protein content in broodstock diet of spawning fecundity and eggs quality of Giant gourami (Ospheronemus gourami Lac). Pakistan J. Nutt. 14, 412–416. doi: 10.3923/pjn.2015.412.416
Mazid M. A., Tanaka Y., Katayama T., Simpson K. L., Chichester C. O. (1978). Metabolism of amino acids in aquatic animals. III. indispensable amino acids for tilapia zillii. Bull. Jpn. Sot. Sci. Fish. 44, 739–742.
Mazorra C., Bruce M., Bell J. G., Davie A., Alorend E., Jordan N., et al. (2003). Dietary lipid enhancement of broodstock reproductive performance and egg and larval quality in Atlantic halibut (Hippoglossus hippoglossus). Aquaculture 227, 2133. doi: 10.1016/S00448486(03)00493-9
Moses S., Agbaji E. B., Ajibola V. O., Gimba C. E. (2018). Amino acid composition and proximate analysis in tilapia (Oreochromis niloticus) fish from dams and Rivers in Zamfara state, Nigeria. J. Appl. Sci. Environ. Manage. 22, 8899–8905. doi: 10.4314/jasem.v22i6.10
Mugwanya M., Dawood M. A. O., Kimera F., Sewilam H. (2022). Updating the role of probiotics, prebiotics, and synbiotics for tilapia aquaculture as leading candidates for food sustainability: a review. Probiotics Antimicrobial Proteins 14, 130–157. doi: 10.1007/s12602-021-09852-x
Mylonas C. C., Fostier A., Zanuy S. (2010). Broodstock management and hormonal manipulations of fish reproduction. Gen. Comp. Endocrinol. 165, 516–534. doi: 10.1016/j.ygcen.2009.03.007
Ng W. K., Wang Y., Yuen K. H. (2006). Vitamin-E levels in tilapia diets affect fillet quality. Global Aquaculture Advocate, 1–6. Available at: https://www.aquaculturealliance.org/advocate/vitamin-e-levels-in-tilapia-diets-affect-fillet-quality/?headlessPrint=AAAAAPIA9c8r7gs82oWZBA.
Ng W., Romano N. (2013). A review of the nutrition and feeding management offarmed tilapia throughout the culture cycle. Rev. Aquaculture 5, 220–254. doi: 10.1111/raq.12014
Ng W.-K., Wang Y. (2011). inclusion of crude palm oil in the broodstock diets of female Nile tilapia, Oreochromis niloticus, resulted in enhanced reproductive performance compared to brood fish fed diets with added fish oil or linseed oil. Aquaculture 314, 122–131. doi: 10.1016/j.aquaculture.2011.01.034
Nguyen L., Davis D. A. (2016). Comparision of crystalline Lysine and intact Lysine used as a supplement in practical diets of Channel catfish (Ictalurus punctatus) and Nile tilapia (Oreochromis niloticus). Aquaculture 464, 331–339. doi: 10.1046/j.aquaculture.22016.07.005
Ogunji J., Wirth M., Rennert B. (2005). Assessing the dietary amino acid requirements of tilapia, Oreochromis niloticus fingerlings 1–10. J. Aquacult. Tropics 20 (4), 241–252.
Olvera-Novoa M. A., Olivera-Castillo L., Martinez-Palacios C. A. (2002). Sunflower seed meal as a protein source in diets for tilapia rendalli (Boulange) fingerlings. Aquacult. Res. 33 (3), 223–229. doi: 10.1046/j.1365-2109.2002.00666.x
Orlando T. M., Oliveira M. M. D., Paulino R. R., Costa A. C., Allaman I. B., Rosa P. V. (2017). Reproductive performance of female Nile tilapia ( Oreochromis niloticus ) fed diets with different digestible energy levels. Rev. Bras. Zootecnia 46, 1–7. doi: 10.1590/s1806-92902017000100001
Palacios H. F., Izquierdo M. S., Gonzalez M., Robaina L., Valencia A. (1998). Combined effect of dietary a-tocopherol and n-3 HUFA on egg quality of gilthead seabream broodstock Sparus aurata. Aquaculture 161, 475–476. doi: 10.1016/S0044-8486(97)00294-9
Prabu E., Felix N., Uma A. (2021). Dietary Arginine requirement in diets of GIFT strain of Nile tilapia, Oreochromis niloticus: effects on growth performance, whole-body composition, growth-related gene expression and Haemato-biochemical response. Aquac. Res. 52, 4816–4828. doi: 10.1111/are.15315
Pronina G. I., Sanaya O. V., Dernakove V. V., Cherkalin A. I., Uvarova E. K. (2022). Application of probiotic “Subtilis-s” in breeding Nile tilapia (Oreochromis niloticus). Fish Farming Fish Economy 3, 201–211. doi: 10.33920/sel-09-2203-04
Ragasa C., Charo-Karisa H., Rurangwa E., Tran N., Shikuku K. M. (2022). Sustainable aquaculture development in sub-Saharan Africa. Nat. Food 3, 92–94. doi: 10.1038/s43016-022-00467-1
Rana K. (1988). “Reproductive biology and the hatchery rearing of tilapia eggs and fry,” in Recent advances in aquaculture. Eds. Muir J. F., Roberts R. J. (Springer, Netherlands), 343–406. doi: 10.1007/978-94-011-9743-4_5
Ribeiro M. J. P., Evangelista M. M., Sanches E. A., Goncalves G. S., Romagosa E. (2017). Crude protein in diets for Nile tilapia brood fish. Bol. Inst. Pesca São Paulo 44, 35–46. doi: 10.20950/1678-2305.2017.35.46
Rohani M. F., Bristy A. A., Hasan J., Hossain M. K., Shahjahan M. (2022). Dietary zinc in association with vitamin E promotes growth performance of nile tilapia. Biol. Trace Element Res. 200, 4150–4159. doi: 10.1007/s12011-021-03001-9
Saenz J. A. C. (2021). Nutritional needs of broodstock tilapia: What should we consider? Available online at: https://www.veterinariadigital.com/en/articulos/nutritional-needs-of-broodstock-tilapia-what-should-we-consider/.
Sajjadi M., Carter C. G. (2004). Effect of phytic acid and phytase on feed intake, growth, digestibility, and trypsin activity in Atlantic salmon (Salmo salar). Aquac. Nutt. 10, 135–142. doi: 10.1111/j.1365-2095.2003.00290.x
Salameh J. P., Bossuyt P. M., McGrath T. A., Thombs B., Hyde C. J., Macaskill P., et al. (2020). Preferred reporting items for systematic review and meta-analysis of diagnostic test accuracy studies (PRISMADAT): Explanation elaboration and checklist. BMJ. 370, m2632. doi: 10.1136/bmj.m2632
Salze G. P., Davis D. A., Stuart K., Mark D. (2019). Effect of dietary taurinein the performance of broodstock and larvae of californiayellowtail Seriola dorsalis. Aquaculture 511, 734262. doi: 10.1016/j.aquaculture.2019.734262
Santiago C. B., Lovell R. T. (1988). Amino acid requirements for growth of Nile tilapia. J. Nutr. 118, 1540–1546. doi: 10.1039/jn/118.12.1540
Santiago C. B., Reye O. S. (1993). Effects of dietary lipid source on reproductive performance and tissue lipid levels of Nile tilapia Oreochromis niloticus (Linnaeus) broodstock. J. App. Ichthy. 9, 33–40. doi: 10.1111/j.1439-0426.1993.tb00385.x
Santos L. D., Furuya W., Matsushita M., Silva L. C. R., Silva T. S. C., Botaro D. (2007). Conjugated linoleic acid (CLA) in diets for Nile tilapia: Reproductive performance and faty acid profile. Rev. Bras. Zootecnia 36, 1481–1488. doi: 10.1590/S1516-35982007000700004
Sarmento N. L. A. F., Martins E. F. F., Costa D. C., Silva W. S., Mattioli C. C., Luz M. R. (2017). Effects of supplemental dietary vitamin C on quality of semen from Nile tilapia (Oreochromis niloticus) breeders. Reprod. Domest. Anim. 52, 144–152. doi: 10.1111/rda.12870
Shiau S. Y., Chen M. J. (1993). Carbohydrate utilization by tilapia (Oreochromis niloticus x O. aureus) as influenced by different chromium sources. J. Nutr. 123 (10), 1747–1753. doi: 10.1093/jn/123.10.1747
Siagian E. R., Nugroho R. A. (2018). Growth performance and carcass composition of African catfish, Clarias gariepinus fed different protein and energy levels. Iran. J. Fish. Sci. 19, 1828–1839. doi: 10.22092/ijfs.2018.117179
Sousa S. M. N., Freccia A., Santos L. D., Meurer F., Tessaro L., Bombardelli R. A. (2013). Growth of Nile tilapia post-larvae from broodstock fed diet with different levels of digestible protein and digestible energy. Rev. Bras. Zootecnia 42, 535–540. doi: 10.1590/S1516-35982013000800001
Suloma A., Tahoun A. M., Mabrok R. S. (2017). Development of broodstock diets for Nile tilapia under hapa-in-pond hatchery system; optimal dietary vitamin C level for the optimum reproductive performance and fry survival. J. Aquac. Res. Dev. S2, 10. doi: 10.4172/2155-9546.S2-010
Sumon M. A. A., Molla M. H. R., Hakeem I. J., Ahammad F., Amran R., Jamal M., et al. (2022). Epigenetics and probiotics application toward the modulation of fish reproductive performance. Fishes 7, 189. doi: 10.3390/fishes7040189
Tacon A. G. J. (2000). Broodstock nutrition: Effects of nutrient levels. Glob. Aquac. Advocate, 1–5.
Takeuchi T., Satoh S., Watanabe T. (1983). Requirement of tilapia nilotica for essential fatty acids. Bull. Jpn. Sot. Sci. Fish. 49, 1127–1134. doi: 10.2331/suisan.49.1127
Tawfik M. M. M., Moustafa M. M., Abumourad I. M. K., El-Meliegy E. M., Refai M. K. (2017). Evaluation of Nano Zinc Oxide feed additive on tilapia Growth and Immunity. 15th International Conference on Environmental Science and Technology. Rhodes Greece. CEST2017_01342. Available at: https://cest2017.gnest.org/sites/default/files/presentation_file_list/cest2017_01342_oral_paper.pdf.
Tesfaye G., Curto M., Meulenbroek P., Englmaier G. K., Tibihika P. D., Alemayehu E., et al. (2021). Genetic diversity of Nile tilapia (Oreochromis niloticus) populations in Ethiopia: insights from nuclear DNA microsatellites and implications for conservation. BMC Ecol. Evol. 21, 113. doi: 10.1186/s12862-021-01829-2
Tibihika P. D., Curto M., Alemayehu E., Waidbacher H., Masembe C., Akoll P., et al. (2020). Molecular genetic diversity and differentiation of Nile tilapia (Oreochromis niloticus, L. 1758) in East African natural and stocked populations. BMC Evol. Biol. 20, 16. doi: 10.1186/s12862-020-1583-0
Torsabo D., Ishak S. D., Noordin N. M., Koh I. C. C., Abduh M. Y., Iber B. T., et al. (2022). Enhancing reproductive performance of freshwater finfish species through dietary lipids. Aquac. Nutt. 2022, 1–13. doi: 10.1155/2022/7138012
Varghese B., Paulraj R., Gopakumar G., Chakraborty K. (2009). Dietary influence on the egg production and larval viability in True Sebae Clown fish Amphiprion sebae Bleeker 1853. Asian Fish. Sci. 22, 7–20. doi: 10.33997/j.afs.2009.22.1.002
Volkoff H., London S. (2018). “Nutrition and reproduction in fish,” in Encyclopedia of Reproduction (Amsterdam: Elsevier), 743–748. doi: 10.1016/B978-0-12-809633-8.20624-9
Wahbi O. M., Sangak Y. (2017). Enhancement of reproductive performance of Nile tilapia Oreochromis niloticus using phytobiotic Spirulina platensis. J. Biol. Sci. 17, 305–311. doi: 10.3923/jbs.2017.305.311
Wang B., Thompson K. D., Wangkahart E., Yamkasem J., Bondad-Reantaso M. G., Tattiyapong P., et al. (2023). Strategies to enhance tilapia immunity to improve their health in aquaculture. Rev. Aquaculture 15, 41–56. doi: 10.1111/raq.12731
Webster C. D., Lim C. (2002). Introduction to fish nutrition, nutrient requirements and feeding of finfish for aquaculture (Wallingford, UK/ New York, USA: CABI Publication), 1–27.
Xiao W., Li D. Y., Zhu J. L., Zou Z. Y., Yue Y. R., Yang H. (2017). Dietary valine requirement of juvenile Nile tilapia, Oreochromis niloticus. Aquac. Nutt. 24 (1), 315–323. doi: 10.1111/anu.12562
Yue Y., Zou Z., Zhu J., Li D., Xiao W., Han J., et al. (2014). Dietary Thereonine requirement of juvenile Nile tilapia. Oreochromis niloticus. Aquac. Int. 22, 1457–1467. doi: 10.1007/s10499-014-9759-5
Yue Y., Zou Z., Zhu J., Li D., Xiao W., Han J., et al. (2015). Effects of dietary arginine on growth performance, feed utilization, haematological parameters and non-specific immune response of juvenile Nile tilapia (Oreochromis niloticus L.). Aquac. Res. 46, 1801–1809. doi: 10.1111/are.12333
Zadeh A. H., Jauncey K., Rana K. (2008). Effects of dietary lipid source on egg and larval quality of Nile tilapia, Oreochromis niloticus (L). 8th International Symposium on Tilapia in Aquaculture 2, 965–977.
Keywords: broodstock, diet, fecundity, nutritive, reproductive performance, Tilapia, dietary components and feeding regimes, egg and larval quality
Citation: Engdaw F and Geremew A (2024) Broodstock nutrition in Nile tilapia and its implications on reproductive efficiency. Front. Aquac. 3:1281640. doi: 10.3389/faquc.2024.1281640
Received: 30 September 2023; Accepted: 30 January 2024;
Published: 22 February 2024.
Edited by:
David Little, University of Stirling, United KingdomReviewed by:
Carlos Alfonso Alvarez-González, Universidad Juárez Autónoma de Tabasco, MexicoCopyright © 2024 Engdaw and Geremew. This is an open-access article distributed under the terms of the Creative Commons Attribution License (CC BY). The use, distribution or reproduction in other forums is permitted, provided the original author(s) and the copyright owner(s) are credited and that the original publication in this journal is cited, in accordance with accepted academic practice. No use, distribution or reproduction is permitted which does not comply with these terms.
*Correspondence: Flipos Engdaw, ZmxpcG9zLmVuZ2Rhd0BhYXUuZWR1LmV0
Disclaimer: All claims expressed in this article are solely those of the authors and do not necessarily represent those of their affiliated organizations, or those of the publisher, the editors and the reviewers. Any product that may be evaluated in this article or claim that may be made by its manufacturer is not guaranteed or endorsed by the publisher.
Research integrity at Frontiers
Learn more about the work of our research integrity team to safeguard the quality of each article we publish.