- 1Faculty of Biosciences, Fisheries and Economics, Norwegian College of Fishery Science, UiT-The Arctic University of Norway, Tromsø, Norway
- 2Department of Sea and Marine Resources, Portuguese Institute for the Sea and Atmosphere (IPMA), Lisbon, Portugal
Food agencies recommend increasing the consumption of aquatic food to promote healthy living and sustainability, and, particularly, to prevent Western lifestyle-related diseases and secure sustainable food systems. This requires growth in global seafood production, and the utilization of low trophic species (LTS) is suggested. LTS are already considered nutritious and important in Asian and Pacific diets. As the use of LTS is expanding in Western diets, producers and consumers need information on the food safety practices associated with them and their nutritional content. LTS are mainly immobile or slow-moving extractive species or organisms that feed on such, and their nutrient and contaminant content varies by species and location. Species-specific knowledge on nutritional contents and safe consumption limits is often missing, making the monitoring and analysis of contaminants, nutrients, and consumption data crucial to guiding the utilization and consumption of LTS. Using global and regional standards regarding nutrients, food hazards, and labeling, this review highlights the appropriate guidelines and regulations for guiding the utilization of selected novel species and also identifies knowledge gaps.
1 Introduction
The United Nations (UN) emphasizes the need for sustainable food security and improved nutrition for all (UN, 2015). Food production and consumption (oral exposure) are responsible for significant global environmental and human health challenges (Gordon et al., 2017; Poore and Nemecek, 2018a), and rapid population growth has exacerbated these. As land-based resources are limited, and this production is reaching its carrying capacity, the procurement of food (and feed) from well-managed oceans is essential for nutritional security, economies, livelihoods, and cultures (SAPEA, 2017; Costello et al., 2020; Gephart et al., 2021; Golden et al., 2021; Crona et al., 2023; Zamborain-Mason et al., 2023). Increased production at lower trophic levels by capture and culture may lead to more sustainable and responsible utilization of marine food resources. (SAPEA, 2017; Costello et al., 2020; Krause et al., 2022). Ocean foods may present an opportunity for better performance regarding nutrition, greenhouse gas emissions, freshwater use, nitrogen and phosphorus emissions, or more sustainable diets (Poore and Nemecek, 2018b; Hallström et al., 2019; Gephart et al., 2021). This strategy, namely of shifting human diets from high-greenhouse gas-emitting land-based sources of protein (e.g., red meat) to lower-greenhouse gas-emitting ocean-based protein sources, and of improving both individuals’ health and the global economy through food (and feed) from marine low trophic species (LTS), has been put forward by multiple actors such as the UN, the Ocean Panel (Costello et al., 2020), and SAPEA (SAPEA, 2017).
The term LTS is used for a range of species, including non-fed or extractive species and species that feed on such, and also deposit or suspension feeders. Several LTS may contribute to improved food security (Krause et al., 2022), including seaweed (macroalgae), abalone (snails), bivalve mollusks, and sea urchins (roe), which are used as examples of LTS in this review and may be a source of essential nutrients such as protein, vitamins, minerals, and omega-3-rich lipids. Higher levels of undesirable contaminants due to the biomagnification in the food chain support the view that a shift toward food sources from LTS is advisable. Mariculture, fresh water, and land-based aquaculture production are perceived as options that can further reduce the content of contaminants, as they allow better control of feed ingredients and outgrowing environments than the harvesting of wild populations.
Increased seafood consumption may also benefit human health globally. An unhealthy diet is a significant risk factor for developing chronic diseases such as cardiovascular heart disease (CVD), stroke, obesity, metabolic syndrome, and diabetes (Murray et al., 2020). It is well documented that increased fish and seafood consumption may reduce the incidence of lifestyle diseases (FAO/WHO, 2011a; EFSA, 2015). The latest thorough risk–benefit assessment by the Norwegian Scientific Committee for Food and Environment (VKM) concluded that the benefits of fish consumption far outweighed the food safety risks in the Norwegian context (VKM, 2022). However, food culture varies around the world, and the Western diet has evolved to include new and novel food resources (e.g., algae, sea cucumbers, and sea urchins). When raw materials, processes, and cuisines change, new challenges to food safety and nutrient availability arise. Food hazards, heavy metals, contaminants, and marine biotoxins are associated with marine food and LTS (Venugopal and Gopakumar, 2017; Eilertsen et al., 2022; Elvevoll et al., 2022; FAO/WHO, 2022; FAO, 2022). The European and global legislation and guidance documents intended to ensure food safety associated with producing and utilizing specific LTS, giving important information such as limits for maximum (MLs) daily or weekly intake, are often lacking. Our aim in this review has been to aggregate the available guidance, as similar standards, laws, and regulations apply to food in general, and to provide a guide on how to assess food safety risks when a regulatory framework is not in place. Altogether, this review paper identifies and highlights appropriate guidelines and regulations and identifies gaps and specific needs for knowledge, to guide the utilization of the selected novel marine LTS.
The objective of this review is to provide a clear and concise overview of the most relevant guidelines and codes of practice adopted by the United Nations [World Health Organization (WHO)/Food and Agriculture Organization (FAO)] Codex Alimentarius Commission (CAC), in addition to the regional regulations from the European Commission (EC) and the United States (USA).
2 Seafood and nutritional contribution
Seafood is an important part of balanced diets. It contains several essential nutrients (Jensen et al., 2016; Durazzo et al., 2022; VKM, 2022; Zamborain-Mason et al., 2023), is generally regarded as nutritious, and its benefits to human health are related to many physiological functions. In seafood, lean animal proteins are combined with healthy long-chain polyunsaturated fatty acids of the omega-3 class (marine n-3 LC-PUFA) and highly bioavailable micronutrients. With a few exceptions, seafood protein compares favorably with other protein sources (Jayasekara et al., 2020; Zamborain-Mason et al., 2023).
When assessing the nutritional benefits of seafood, the focus has been on two marine n-3 LC-PUFAs, eicosapentaenoic acid (EPA, 20:5n-3) and docosahexaenoic acid (DHA, 22:6n-3). This is due to strong scientific evidence of the positive health impact of n-3 LC-PUFAs, their contribution to the attenuation of lifestyle diseases, and the lack of availability from other dietary sources (VKM, 2022). Other key nutrients in seafood include minerals and trace elements such as iodine, selenium, and vitamins D and B12 (Golden et al., 2021). Seafood can provide essential vitamins and minerals such as vitamin D, selenium, iodine, zinc, magnesium, and calcium (Zamborain-Mason et al., 2023). These vitamins and minerals are often more abundant in seafood than in the meat of land animals, making the consumption of seafood a great means of increasing one’s intake of them (Wright et al., 2018).
The nutritional contribution from LTS is less systematically studied, but the field is rapidly evolving. A recent paper has assessed the nutritional contribution and food safety aspects of specific seaweed (Jacobsen et al., 2023). Saccharina latissima and Ulva species are good sources of calcium, magnesium, iron, selenium, and the vitamin B12, as they contribute to > 15% of the daily reference intake (DRI) per 5-g portion of dry weight. The contribution to DRI for fiber, sodium, and protein was lower. Food safety was compromised by very high iodine levels for S. latissima. In sea cucumber, Cucumaria frondosa, the content of micronutrients and minerals is generally high compared with that in other seafood, particularly of the vitamins B12 and E, and selenium (as high as 40% of DRI) (Langdal et al., 2023; Muhsin et al., 2023). The content of n-3 fatty acids in sea cucumbers was similar to that in lean seafood, such as cod and shrimp, and food safety was compromised by the content of inorganic arsenic in sea cucumbers from Norwegian waters.
The recent work of the European Food Safety Authority (EFSA) on nutritional profiling states that fish and shellfish, in addition to their by-products, can significantly increase the intake of n-3 LC-PUFAs, iodine, and vitamin D (EFSA, 2022). The contribution of seafood as a source of animal protein, vitamins and minerals, and carbohydrates was assessed as less significant to the European diet.
2.1 Marine n-3 LC-PUFAs
Seafood consumption is known to reduce the risk of developing cardiometabolic diseases (Rimm et al., 2018; Chandra et al., 2020). These results are based on epidemiological evidence and meta-analyses (Afshin et al., 2019; VKM, 2022) and on preclinical and clinical studies (Maehre et al., 2015b; Innes and Calder, 2020). The recommendations for seafood consumption are often based on the contents of EPA and DHA, and seafood consumption entailing a daily intake of 200 mg–500 mg of EPA and DHA is recommended by the UN Food and Agricultural Organization (FAO) and World Health Organization (WHO) (FAO/WHO, 2011a). Other recommendations and possible mechanisms for the dietary prevention of cardiometabolic diseases have been reviewed elsewhere (Maehre et al., 2015a; VKM, 2022).
2.2 Iodine
The trace element iodine (I) is important for the regulation of metabolic rate, embryology, and physical, mental, and cognitive development (WHO, 2007). In Europe, salt iodization has reduced iodine deficiency. It is, however, still a public health concern (WHO, 2007; EFSA, 2022), and a high prevalence of I deficiency is still evident in subpopulations in several European countries (Ittermann et al., 2020). It is essential that individuals who are pregnant or planning to become pregnant have an adequate I intake to avoid any long-term adverse impact on the child (Hay et al., 2019). However, it is also important to note that excessive I can cause thyroid gland dysfunction. It is worth noting that brown seaweed has an exceptionally high content of I. The high I content makes brown seaweed an excellent source of I, but regular intake of only a few grams would cause excessive intake of I (Aakre et al., 2020; Aakre et al., 2021; Jacobsen et al., 2023). The EFSA’s Scientific Committee on Food (SCF) suggests a maximum upper-level (UL) intake of 600 μg I per day for adults, adjusted for different age groups based on differences in body weight (EFSA, 2014a).
2.3 Selenium
An adequate intake of selenium (Se) is crucial for good health. Se helps activate the thyroid hormone, and selenoenzymes have important antioxidant properties (Winther et al., 2020). Unfortunately, many adult Europeans do not meet the daily recommended, or adequate, intake (AI) of Se, which is 70 μg per day (EFSA, 2014b). A low intake of Se has been linked to reduced immune function, cognitive decline, and an increased risk of goiter, autoimmune thyroiditis (Hashimoto’s disease), hyperthyroidism (Graves’ disease), and mortality (EFSA, 2014b). In addition, Se intake is associated with antiviral mechanisms and infection protection and is regarded as essential for reproduction (Winther et al., 2020).
Interestingly, the intake of LTS may protect against mercury (Hg) toxicity due to the high content of Se in LTS and other seafood (Yamashita et al., 2013; Mellingen et al., 2022; Jacobsen et al., 2023; Langdal et al., 2023). The ideal ratio of Se to mercury is 1 to 1, or greater than 1; this reduces the bioavailability of mercury in the body (Jinadasa et al., 2021). However, it is important to note that while Se is essential for good health, consuming too much Se can have adverse health effects. The UL for all adults, including pregnant and lactating women, for Se intake is 300 μg per day in the European Union (EU; EFSA, 2018a) and 400 μg per day in the USA (NAP, 2019).
2.4 Proteins
Proteins are essential for growth and development, and both quantity and quality are important when evaluating proteins in raw materials and products. A protein intake of 0.83 g per kg body weight per day has been recommended (FAO/WHO/UNU, 2007). Protein absorption, digestion, and assimilation capacity are important factors when considering protein quality, hence, protein source quality is evaluated using different means (Adhikari et al., 2022). Marine proteins contain all the essential amino acids (EAAs) (histidine, isoleucine, leucine, lysine, methionine, phenylalanine, threonine, tryptophan, and valine) and are, in general, good sources of these.
The chemical score (CS) is a tool for assessing the quality of dietary proteins. The CS reflects the potential of the protein to supply the necessary amount of essential amino acids, and this is determined by comparing each EAA in the food protein with the corresponding EAA in the reference protein, as set by FAO/WHO (FAO/WHO/UNU, 2007). Animal proteins typically have a CS of 1.0 and contain sufficient levels of all EAAs, while the CSs of cereal or staple proteins often range from 0.4 to 0.6. Legumes and nuts usually fall somewhere in between. Conversely, seafood has a high protein quality, with levels of all EAAs above or equal to the reference levels (FAO/WHO/UNU, 2007; Jensen et al., 2023). This makes seafood superior to terrestrial plants and comparable to the protein sourced from terrestrial animals.
2.5 Dietary fiber
Although seafood is generally low in carbohydrates, seaweeds, the LTS industries of which are booming, may be considered a new source of dietary fiber (Peñalver et al., 2020). The consumption of dietary fiber is associated with many health benefits, such as improved lipid and carbohydrate metabolism and bowel function. Other fiber characteristics that are believed to mediate beneficial health effects include reduced nutrient absorption, ability to bind water and form gels, increased viscosity in the intestinal tract, and the fermentability and production of short-chain fatty acids in the colon (EFSA, 2022). In mixed diets, dietary fiber consumption has been inversely associated with the risk of cardiovascular disease (CVD) and type II diabetes (T2DM). However, the mechanisms facilitating this are unclear and may depend on the fiber type (EFSA, 2022).
In Europe, the dietary fiber intake is generally below the recommendations (25 g per day). Fiber consumption above the recommendations for maintaining normal bowel function will likely reduce the risk of developing chronic diseases. The EFSA panel considers that increasing dietary fiber consumption is a matter of public health importance (EFSA, 2022).
3 General nutrient recommendations, labeling, and claims
3.1 Nutrient profiling
Following the Farm to Fork strategy, the EFSA is also working on a policy revision for nutrient profiling. Nutrient profiling means classifying or ranking foods in accordance with their nutrient content, which in turn is based on the association of the nutrient’s ability to prevent disease or promote health (EFSA, 2022). In line with this, the EC plans to propose a revision of the existing legislation on providing food information to consumers in 2023. The revision aims to establish “nutrient profiles”, that is, thresholds beyond which nutrition and health claims are restricted or not allowed.
Dietary fiber and potassium intake are assessed as inadequate in many European adult populations. Consequently, an increase in consumption is encouraged for the amelioration of adverse health effects.
It is important to note that the intakes of EPA and DHA may be inadequate for primary CVD risk prevention in populations where the consumption of fish, seafood, and fish products is low (EFSA, 2022). In addition, certain subgroups may have inadequate intakes of vitamin D, calcium, iodine, and iron, and diet modification alone may not be enough to ensure adequate intakes.
However, the EFSA scientific panel has pointed out that average protein intakes are mostly above their population reference intake (PRI) in Europe. PRI is defined as “the level of (nutrient) intake that is adequate for almost all individuals (97.5%) in a population group” (EFSA, 2022).
Even though some processed fish products have a sodium content that is well above the recommended sodium intake, regular fish consumption is generally advised, with recommendations ranging from one to four servings per week, and the recommended serving size ranging from 30 g to 200 g, but mainly from 100 g to 150 g. Some countries recommend the consumption of fatty fish, one country recommends low-to-medium fat fish, and many countries emphasize the importance of eating assorted species, both regarding type of fish and the fishing locations from which they are sourced (EFSA, 2022). This demonstrates the variation in recommendations for fish products that is likely to be translated to other seafood, such as products containing LTS, for which data are often still scarce.
A future shift in human diets from less sustainable terrestrial sources to marine resources has to focus on the utilization of LTS and how they may contribute to health (SAPEA, 2017; Costello et al., 2020). Nevertheless, in this transition it will be necessary to ensure that data collection on LTS and its associated products is carried out, and to initiate clinical and epidemiological studies, to provide the best-possible recommendations to the consumers across the world.
3.2 Nutrient reference values
The FAO/WHO Codex Committee on Nutrition and Foods for Special Dietary Uses (CCNFSDU) revised the 1993 nutrient reference value (NRV) for the essential nutrients, protein, vitamins, and minerals over several years. It presented a report titled “General Principles for Establishing Nutrient Reference Values for the General Population” to make labeling easier for processors and inform consumers globally (Lewis, 2019). The CCNFSDU has agreed on the term NRVs-R (nutrient reference value requirement) for the essential nutrients, protein, vitamins, and minerals (Table 1), which are values to be used as references in nutrition labeling. These values are derived from authoritative recommendations (from actors such as the FAO/WHO, EFSA, and IOM) on the reference nutrient intake values (RDIs) for optimal daily nutrient intake and are based on the best-available scientific knowledge of the daily amount of energy or nutrients needed for optimal health.
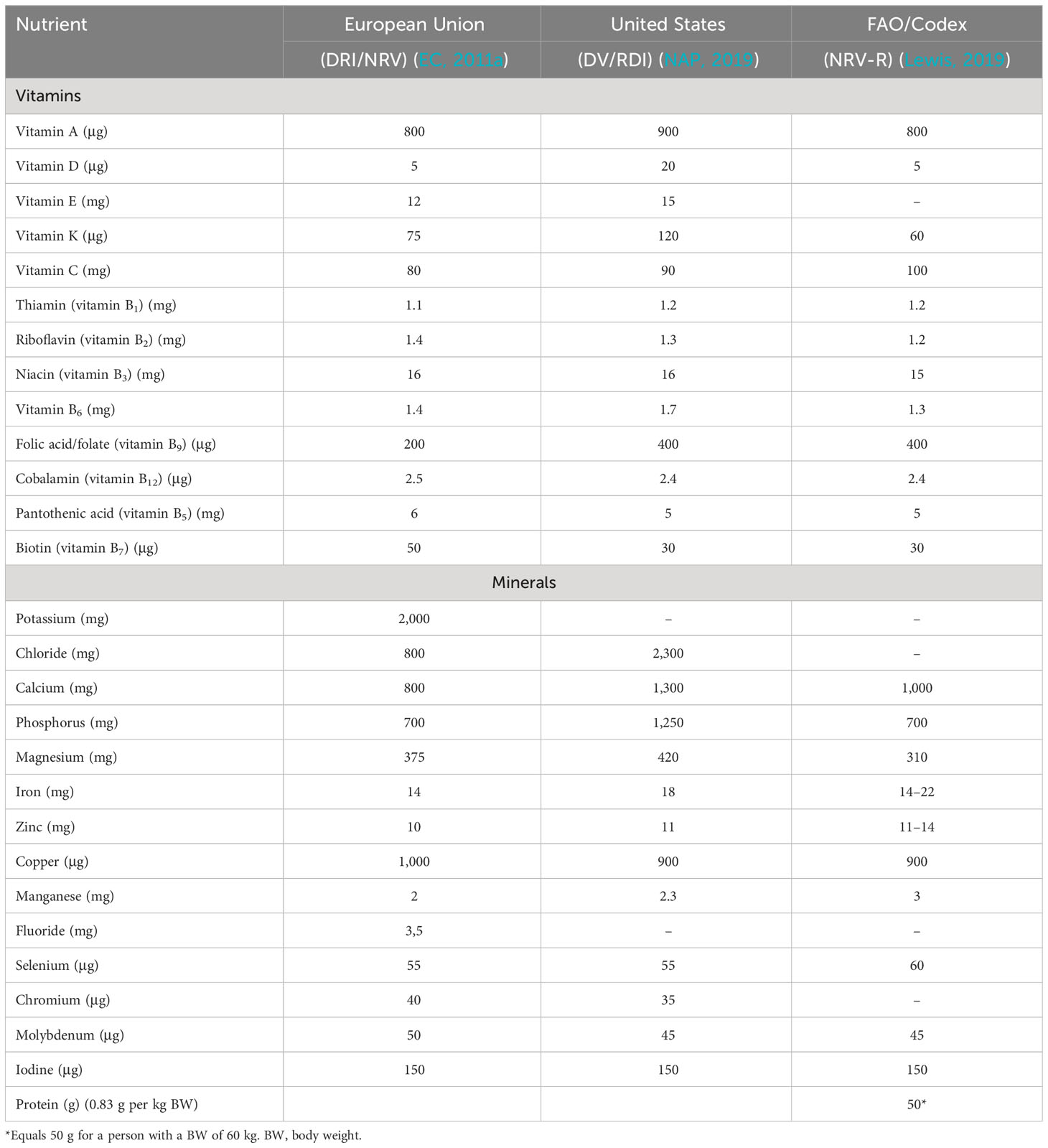
Table 1 Nutrient reference values (NRVs) for the requirement of essential nutrients, that is, protein, vitamins, and minerals, as reviewed by the Codex Committee on Nutrition and Foods for Special Dietary Uses (CCNFSDU) (Lewis, 2019), the European Union (daily reference intakes, DRI) (EC, 2011a), and the USA (daily value and reference daily intake; DV and RDI, respectively) (NAP, 2019).
While the actual NRV is not shown on the nutrition label, labels normally give the percentage of the daily requirement for essential nutrients, to indicate how much each nutrient in a portion of food contributes to healthy nutrient intake. NRVs may be used to guide consumer food choices, compare different food items, and to calculate the intake of the nutrients. The processes used to derive a given NRV for a nutrient, and the actual NRV, are described in detail in the “Codex nutrient reference values” report (Lewis, 2019).
In addition to the CCNFSDU NRVs, the EU (EC, 2011a) and the USA (daily value and reference daily intake; DV and RDI, respectively) (NAP, 2019) have established reference values. These consider region-specific factors such as nutrient absorption or bioaccessibility from regional food products and specific nutrient requirements of their population. In addition to these general reference values, which apply to all foods, the NRV for the essential nutrients (Table 1) and the daily tolerable upper levels of intake (ULs) have been established (Table 2) for the EU and the USA; however, there may be national or other regional recommendations or regulations.
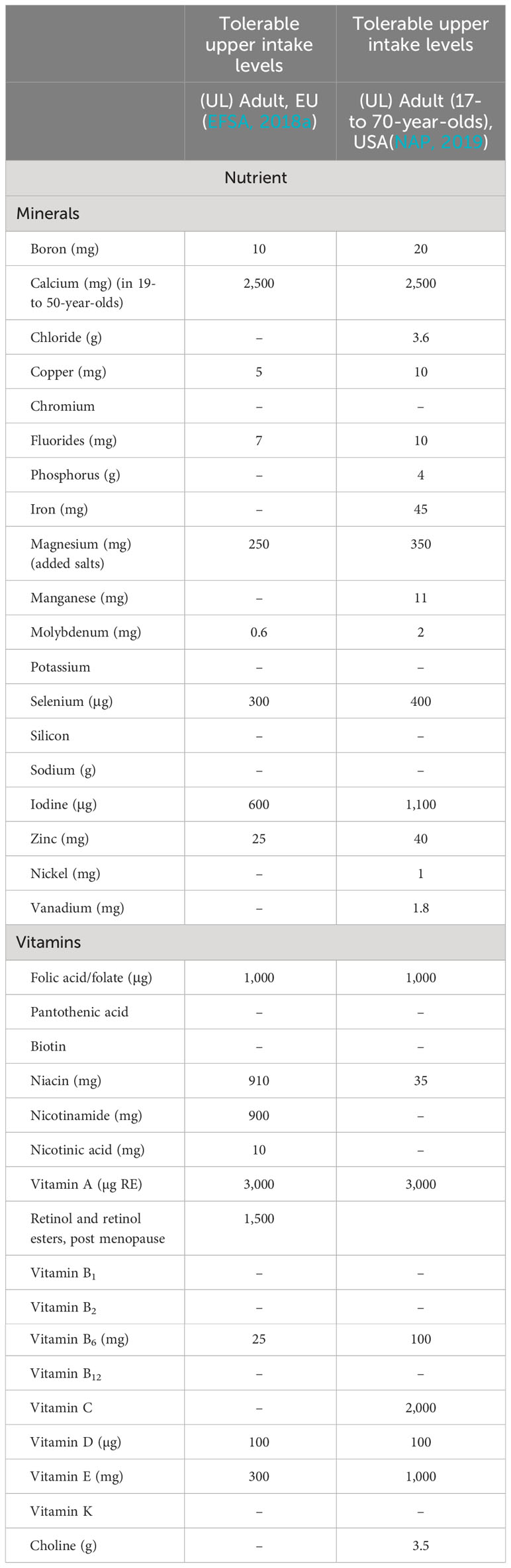
Table 2 Daily tolerable upper intake levels (ULs) of essential nutrients, that is, vitamins and minerals, as reviewed by the European Food Safety Authorities (EFSA) (EFSA, 2018a) and Food and Nutrition Board, National Academies (NAP, US) (NAP, 2019).
3.2.1 Seaweed labeling to prevent excess iodine in the diet
The development of a seaweed industry, increased seaweed production, and the utilization of seaweed in the Western world have recently attracted particular interest. A panel of experts from the FAO/WHO has reviewed all food safety aspects of seaweed globally (FAO/WHO, 2022). One of the aspects of concern was the high content of I.
The intake of I is too low to gain all its health benefits in many European populations (Brantsæter et al., 2018). The content of I in food items is regulated by laws pertaining to ULs both in the USA and the EU (Table 2). Thus, guiding producers and consumers on new food resources is challenging.
It is important to be aware that certain types of seaweed, particularly some brown seaweeds (Laminaria sp. and Saccharina sp.), are high in I (Aakre et al., 2021). Consuming too much I can be harmful, especially for vulnerable groups. The VKM advises that consumers practice moderation when consuming seaweeds to avoid excessive I intake (Aakre et al., 2021; Hogstad et al., 2023).
To assist consumers in making informed choices, Norwegian seaweed producers have created an industrial guideline that includes labeling recommendations for products with a high I content (ALGET2, 2022). When labeling pre-packaged products of pure seaweed, the recommendation is that the overview of nutrient levels must include I content per 100 g. When the I value is high, the label should include the recommended daily intake of I and state that excessive iodine intake over time can affect the thyroid gland. When labeling prepackaged products containing seaweed as an ingredient, only the amount of I in mg per 100 g of the whole product must be on the label.
The content and bioaccessibility of I can be significantly reduced by processing and through common culinary techniques such as blanching, boiling, drying, and frying food (Peñalver et al., 2020; Blikra et al., 2021).
3.3 Nutrition and health claims
Two FAO/WHO Codex nutrition labeling guidelines (FAO/WHO, 2001; FAO/WHO, 2013) give a global standard approach to presenting nutrition information on food labels and describe the conditions for making nutritional and health claims.
Certain foods or ingredients can have specific beneficial nutrients or nutritional properties. These can be marked on food labels as a “nutrition claim” when they satisfy the given regulations. Examples of such nutrition claims are “source of protein”, “source of fiber”, “fat-free”, or “with no added sugars”. In addition, “health claims” suggest a connection between a food item, or one of its constituents, and health. These regulations apply to all foods (EC, 2006c; EC, 2012).
The EC has also instituted regulations related to the labeling and presentation of nutrition and health claims on foods (Gilsenan, 2011). The EC has listed the conditions for using product claims to prohibit false claims, such as that the food is safe or nutritionally adequate and that the claims do not encourage excess consumption (EC, 2012). These nutritional and health claims are regulated and can only be used in the labeling and advertising of foodstuffs if they appear on the lists of authorized claims.
No specific health claim has been developed for any LTS or extracts of these. Thus, to achieve this, well-designed, controlled trials are necessary to confirm any putative nutraceutical benefits of such novel marine raw materials.
On the other side, there are already approved nutritional claims, related to the recommendations for the intake of dietary fiber and also of the marine n-3 LC PUFAs, EPAs, and DHAs. These may be attributed to LTS depending on the nutritional content of the product. The approved health claim for dietary fiber may also be attributed to seaweed products containing enough fiber. Other already approved nutrition and health claims may be attributed to products containing a minimum of 40 mg dietary fiber per 100 g edible weight.
This is an example of the formulation of a nutritional claim:
“a claim that a food is a source of omega-3 fatty acids, and any claim likely to have the same meaning for the consumer, may only be made where the product contains at least 0,3 g alpha-linolenic acid per 100 g and per 100kcal, or at least 40 mg of the sum of EPA and DHA per 100 g and per 100kcal” (EC, 2012).
The associated health claim, “EPA and DHA contribute to the normal function of the heart” may be used only for food that is at least a source of EPA and DHA (EC, 2006c). “To bear the claim, information shall be given to the consumer that the beneficial effect is obtained only with a daily intake of 250 mg of EPA and DHA”. A nutritional.
“claim that a food is high in omega-3 fatty acids, and any claim likely to have the same meaning for the consumer, may only be made where the product contains at least 0.6 g alpha-linolenic acid per 100 g and per 100 kcal, or at least 80 mg of the sum of eicosapentaenoic acid and docosahexaenoic acid per 100 g and per 100 kcal” (EC, 2010).
Other approved health claims for EPA and DHA (EC, 2006c; EC, 2010; EC, 2012) are that:
● “DHA and EPA contribute to the normal function of the heart” (at an intake of 0.25 g per day)
● “DHA and EPA contribute to the maintenance of normal blood pressure” (at an intake of 3 g per day)
● “DHA and EPA contribute to the maintenance of normal blood triglyceride levels” (at an intake of 2 g per day)
● “DHA contributes to maintenance of normal brain function” (at an intake of 0.25 g per day)
● “DHA contributes to the maintenance of normal vision” (at an intake of 0.25 g per day)
● “DHA maternal intake contributes to the normal brain development of the fetus and breastfed infants” [at an intake of 0.2 g DHA plus the daily recommended intake of omega-3 fatty acids (EPA + DHA) for adults, which is 0.25 g per day]
● “DHA maternal intake contributes to the normal development of the eyes of the foetus and breastfed infants” [at an intake of 0.2 g DHA plus the daily recommended intake of omega-3 fatty acids (EPA + DHA) for adults which is 0.25 g per day].
4 Chemical hazards
Specific CAC (FAO/WHO, 1995) and EC (EC, 2006b) maximum limits (MLs) of heavy metals and contaminants remain undetermined for low trophic species such as abalone, sea snails, sea cucumbers, and sea urchins (or any Echinodermata). Thus, the use of MLs for comparable seafood items, such as bivalve mollusks, is extensive when assessing the food or product safety of such species, organisms, or products (EC, 2006b; EC, 2011c; EC, 2015a; Blikra et al., 2021).
In the case of novel resources, even if frequently consumed in Asian cuisines, there is a lack of data on the content in products and the quantities and frequencies of consumption. This lack of data adds to the challenge of assessing food safety. Calculating the tolerable consumption based on the available general limits of consumption may be an alternative strategy to assess these risks. It is important to remember that these general limits are given for the exposure of a specific hazard from all foods in the diet, and thus not only the exposure from the product but that from the whole diet must be assessed. These limits ensure safe consumption without an enhanced lifetime risk of diseases and may be used to guide consumers. Such limits are given as a tolerable weekly intake (TWI), provisional TWI (PTWI), reference dose (RfD), or the BMDL (Table 3). In addition, it is important to consider factors such as body weight and age (in terms of fertility and pregnancy), when assessing these limits to protect vulnerable groups.
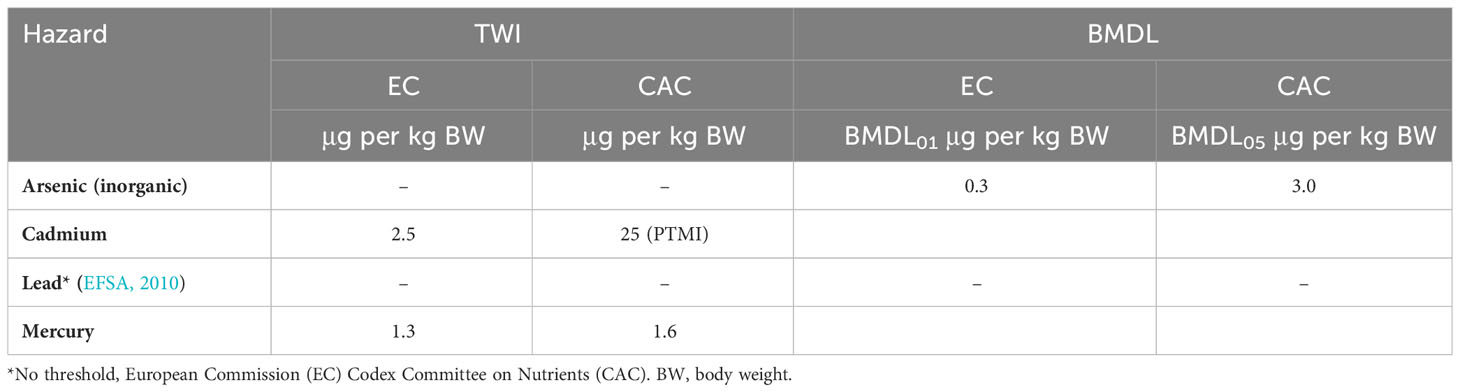
Table 3 General tolerable weekly intakes (TWIs) and lower-end benchmark doses (BMDLs) for dietary consumption issued by the European Commission (EC) (EC, 2006b) and the FAO/WHO Codex Committee on Nutrients (CAC) (FAO/WHO, 1995) for the intake of heavy metals (inorganic arsenic, cadmium, lead, and mercury) from food.
4.1 Heavy metals
4.1.1 Mercury
The inorganic heavy metal mercury (Hg), or more precisely, its organic form methylmercury (MeHg), is known to accumulate in predators and organisms at higher trophic levels. This heavy-metal toxin can cross important barriers in the body, such as the placenta, the blood–brain barrier, and the blood–cerebrospinal fluid barriers (Chiocchetti et al., 2017). MeHg, which may make up more than 90% of the total Hg in seafood, is particularly concerning due to its cytotoxic capacity, which is higher than that of inorganic Hg (Hong et al., 2012; Barone et al., 2021). MeHg is lipid soluble and is easily absorbed by and transported throughout the body (Martinez-Finley and Aschner, 2014). It is important to note that food sources from lower trophic levels generally have a lower Hg content due to the absence of biomagnification (Córdoba-Tovar et al., 2022).
There are TWI for Hg and MeHg to avoid prenatal neurodevelopment toxicity. The TWI for MeHg is 1.6 μg per kg body weight (BW) according to the CAC and 1.3 μg per kg BW according to the EC. The TWI for inorganic Hg is 4 μg per kg BW according to the EC (See Table 3) (FAO/WHO, 1995; EFSA, 2015).
The MLs of Hg for unspecified fish muscle and fishery products, including mollusks and crustaceans, is 0.5 mg per kg wet weight (WW) of edible tissue. Higher limits exist for predatory or long-lived, bottom-dwelling fish (1.0 mg per kg WW) (FAO/WHO, 1995, EC, 2006b). A higher ML may reflect either that their consumption is assessed as low or that some species have an inherently higher content.
4.1.2 Cadmium
Exposure to cadmium (Cd) can have adverse effects on the growth, neurodevelopment, and cognitive function of children (Kippler et al., 2016; Chatzi et al., 2019). In light of this, the Joint FAO/WHO Expert Committee on Food Additives (JECFA) set a provisional tolerable monthly intake (PTMI) of 25 µg per kg BW for Cd in 2011, recognizing the long half-life of Cd in humans (FAO/WHO, 2011b). The EC has established a TWI of 2.5 μg per kg BW. For fish muscle (unspecified), the ML for Cd has been set at 0.05 mg per kg WW, while it is as high as 1.0 mg per kg WW for the edible part of bivalve mollusks (EC, 2006b; EC, 2021).
4.1.3 Arsenic
There are more than 100 arsenic (As) compounds in the environment, and their toxicity varies. Inorganic arsenic (iAs) is classified as a class 1 carcinogen, while organic forms have lower toxicity levels (IARC, 2012). Although there are no EC MLs for total As content in seafood intended for human consumption, some countries have issued regional regulations. For example, China has set a ML of 0.5 mg per kg WW iAs in “aquatic animals and products (excluding fish and fish products)” (CFDA, 2017). France has also a specific regulation or ML of 3.0 mg per kg DW on seaweed food and condiments (ANSES, 2020) (Table 4). It is worth noting that a revision of PTWI of iAs in 2009 established a range from the benchmark dose (BMD). Lower confidence limit BMDL01 values of between 0.3 μg and 8 μg per kg BW per day were associated with a 1% increase in the probability of getting lung, skin, or bladder cancer, and also skin lesions. Thus, the maximum daily dietary intake of iAs should be below 0.3 μg per kg BW. This means that an adult weighing 60 kg should have a maximum of 18 μg iAS per day (EFSA, 2009b). JECFA also performed a risk assessment in 2010 based on lung cancer rates and proposed a BMDL05 value of 3.0 μg per kg BW (FAO/WHO, 2011b; Afonso et al., 2020). Organic As is assumed to be far less toxic (FAO/WHO, 2011b; Coelho, 2019; Afonso et al., 2020), and is the main form present in foods of marine origin. However, some organisms, such as certain seaweed species, may contain high levels of iAs (Duinker et al., 2020). Because analyses or data on levels of total As and the numerous species of As are generally not available, especially for new LTS food resources, this is considered a knowledge gap.
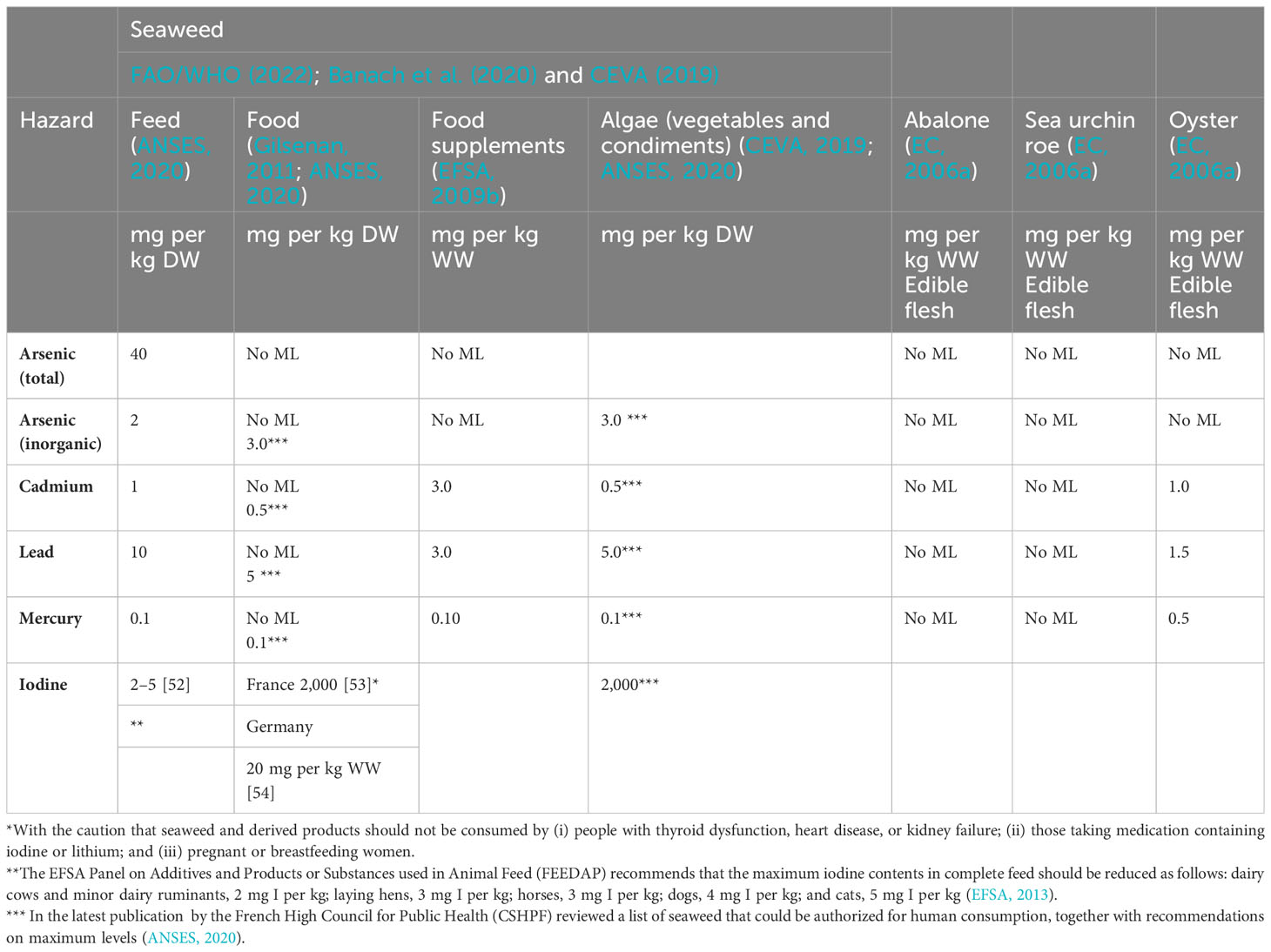
Table 4 Maximum limits (MLs) for the content of heavy metals (arsenic, inorganic arsenic, cadmium, lead, and mercury) in seaweed, seaweed products, abalone, sea urchin roe, and oyster.
4.1.4 Lead
Lead (Pb) is a heavy metal that is particularly harmful to children and the fetus when transferred via the placenta. Its harmful effects may affect many organs and systems, such as the brain, liver, kidney, and bones. The Panel on Contaminants in the Food Chain (CONTAM panel) assessed the harmful effects of Pb in food in 2010 (EFSA, 2010). It could not identify a lower threshold for Pb-induced effects and deemed the prevailing PTWI to be inappropriate. Thus, it is crucial to keep exposure from all Pb sources as low as possible (EFSA, 2010). The European ML of Pb is 0.3 mg per kg WW fish muscle for most fish species but much higher, 1.5 mg per kg WW, for mollusks (EC, 2006b).
4.2 Persistent organic pollutants
It is important to be aware of the presence of persistent organic pollutants (POPs) in seafood, as they can have harmful effects on human health. POPs include halogenated synthetic compounds, which can be chlorinated [such as polychlorinated biphenyls (PCBs), polychlorinated dibenzo-p-dioxins and polychlorinated dibenzo-p-furans (PCDDFs)] or be organochlorine pesticides [such as DDT, toxaphene, and chlordane, in addition to the polybrominated diphenyl ethers (PBDEs)]. Some of these are byproducts of industrial synthesis or combustion (PCDD/Fs), while others are designed for specific industrial uses (e.g., PCBs and PBDEs) or as pesticides (e.g., DDT, lindane, and chlordane). The presence of other POPs such as polycyclic aromatic hydrocarbons (PAHs) has also attracted attention. POPs are toxic compounds that accumulate (during the lifespan of the organism) and magnify successively at higher levels in the food chain, with higher concentrations found in fatty fish/seafood due to their lipid toxicity (EC, 2017). Fortunately, the levels of POPs are generally lower in LTS (Landrigan et al., 2020). Legislative measures have led to a decline in the levels of POPs in the environment (van den Berg et al., 2017; Guo et al., 2019), but they still persist due to their long half-lives.
4.2.1 Dioxins and PCBs
Seventeen of the 210 known dioxins and 12 of the 209 PCBs (the dl-PCBs), are considered food hazards (EFSA, 2018b). These dioxins and dl-PCBs have similar structural and toxicological properties and exert their toxicological effects via binding to the aryl hydrocarbon receptor (AhR). When it comes to fish fillets and fishery products, the European ML for dioxins and dl-PCBs is set at 6.5 pg TE per g WW (EC, 2011c). The TWI for dioxins and dl-PCBs was lowered from 14 pg to 2 pg toxic equivalents (TEQ) per kilogram of body weight due to their documented adverse effects on semen quality and possibly also on male fertility (EFSA, 2018b).
4.2.2 Polycyclic aromatic hydrocarbons
The presence of polycyclic aromatic hydrocarbons (PAHs) in seawater and sediments arises from the incomplete combustion of organic matter, coal, or oil sources. PAH deposits in the marine environment are a result of industrial and transport activity that deposits PAHs directly into the ocean, or inputs from rivers stemming from inland activities. Marine sediments may contain high levels of PAHs and pose a risk through bioaccumulation. The capacity to metabolize PAHs is less developed in some filter feeding organisms than it is in fish and crustaceans, and PAHs may thus accumulate due to low elimination rates (Sampaio et al., 2021). The PAH’s lipophilicity, absorption, persistency, and toxicity increase with its molecular weight. PAH metabolites such as benzo[a]pyrene (BaP), may bind to DNA and cause heritable mutations. There are no MLs for fresh, chilled, or frozen seafood, except for bivalve mollusks, which have a ML of 5 µg per kg WW for BaP and 30 µg per kg WW for PAH4 (the sum of BaP, benzo(a)anthracene, chrysene, and benzo(b)fluoranthene) (EC, 2011b). However, as PAHs arise from the incomplete combustion of organic matter, it is important to note that grilled or smoked fatty seafood may contain high levels of PAHs (EFSA, 2008).
4.2.3 Poly- and perfluorinated compounds
Thousands of poly- and perfluorinated compounds (PFAS) can accumulate in humans and the environment. These compounds are commonly used in non-stick cookware, stain- and water-repellent textiles, carpets, and furniture, and firefighting foams, and have leached into the oceans (Landrigan et al., 2020). Recently, the EC set a TWI of 4.4 ng per kg BW of the sum for selected PFAS compounds, including perfluorooxane sulfonic acid (PFOS), perfluorooctanoic acid (PFOA), perfluorononanoic acid (PFNA), and perfluorohexane sulfonic acid (PFHxS), to protect human health (EFSA, 2020). The EFSA has assessed various associations between serum levels of PFAS and several outcomes. The most critical effect of the sum of PFASs was evaluated to be on the immune system, particularly in children who, after prenatal and postnatal exposure to PFAS, may experience a decreased vaccination response (EFSA, 2020; VKM, 2022).
4.2.4 Pesticide residues
The pesticide contamination of marine organisms is generally the result of leach from pesticide use in terrestrial agriculture. The EU legislation covers more than 1,000 pesticides currently or previously used in agriculture, but for pesticides not covered by the legislation, the EU has set a general maximum residue limit (MRL) of 0.01 mg per kg. It is important to note that farmed seaweeds may contain pesticide residues if pesticides are used in the outgrowth phase, and the EC has established MRLs for some seaweeds (EC, 2005b; FAO/WHO, 2022). However, limited information is available on the content of pesticides in other LTS species, and species-specific MRLs are largely not available.
4.3 Radionucleotides
The allowed content of radionucleotides in food is regulated by very general guidelines. Foods are divided into “infant foods” and “foods other than infant foods”. Most of the marine LTS fall in the second category (FAO/WHO, 1995). The EU has set maximum levels of food after nuclear accidents/disasters (EC, 2016). This includes a broad category, “other food except minor food”, which may apply to seaweed, abalone, sea urchin, and oyster. However, seaweed, which is able to extract minerals and trace elements from its environment, remains somewhat unexplored (Duinker et al., 2020), except when it is used as a bioindicator for 129I and for nuclear leaches in the marine environment (Duinker et al., 2020; FAO/WHO, 2022).
4.4 Biotoxins
Marine biotoxins are chemicals produced by specific types of toxin-producing algae. Seafood, particularly filter feeders, feed on such algae, and exposure may thus occur by eating seafood. The symptoms of poisoning vary with the type and level of toxin consumed (EFSA, 2009a; Jong, 2017).
Ciguatera fish poisoning (CFP) is the most frequent illness associated with the consumption of fish with ciguatoxins (CTXs) from microalgae (Gambierdiscus spp. and Fukuyoa spp.) (Chain, 2010). Neurotoxic shellfish poisoning (NSP) and paralytic shellfish poisoning (PSP), generally caused by shellfish consumption, usually cause gastroenteritis in addition to neurologic symptoms (Watkins et al., 2008; Etheridge, 2010). Amnesic shellfish poisoning (ASP), a result of excessive domoic acid consumption, causes neurologic but not gastrointestinal symptoms (Jeffery et al., 2004). Diarrhetic shellfish poisoning (DSP), caused by excessive okadaic acid and dinophysis toxin consumption, on the other hand, present with gastrointestinal but not with neurologic symptoms (Jong, 2017). Azaspiracid shellfish poisoning (AZP), triggered by azaspiracid toxins, may cause severe gastroenteritis (Jong, 2017).
The EU recognizes that the biotoxin poisoning risk is associated predominantly with bivalve mollusk consumption. Both producers and authorities are subject to regulation to manage food safety in these food chains (Table 5) (EC, 2004). An important component is the continuous monitoring of algal and toxin levels in the water bodies used for the production of, and those in, produced mollusks, to inform and give the ability to close production areas and prohibit harvest when unacceptable biotoxin levels are exceeded. O’Mahony has reviewed regulations and assessed their contribution to the risk management approach (O'Mahony, 2018).
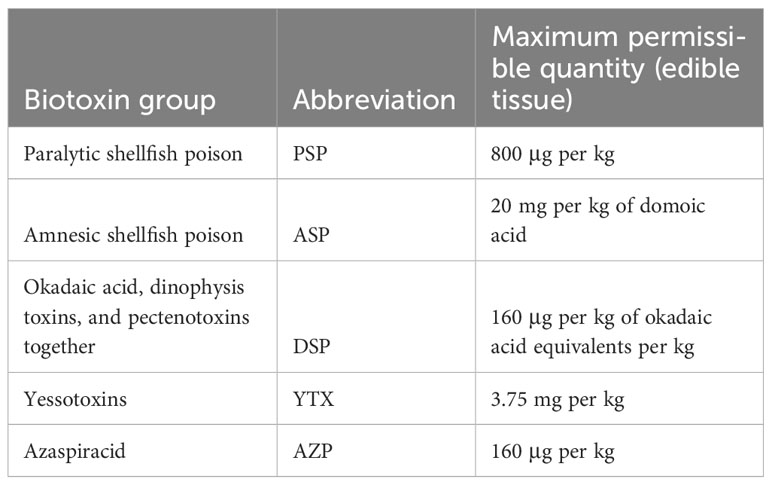
Table 5 Biotoxin health standards in EC regulation 853/2004 (EC, 2004) for live bivalve mollusks, measured in the whole body or any part eaten separately. Table legend: Adapted from (O'Mahony, 2018).
4.5 Microbiological hazards
Pathogenic microorganisms from the growth environment, harvest, processing, or handling may pose a challenge to food safety. The sanitary conditions in the whole food chain and when food is consumed are vital. Naturally occurring viruses (hepatitis A and norovirus) and bacteria (Salmonella) that are associated with fecal contamination and pathogens, such as Vibrio species, may be present at harvest and outgrowing sites (Wright et al., 2018). In EC regulation no. 2073/2005 the only microbiological criterion is a ML for Listeria monocytogenes (EC, 2005a). The FAO and WHO have developed and recently revised technical guidelines on growing area aspects of bivalve mollusk (FAO/WHO, 2021), using the monitoring of E. coli as a proxy for fecal contamination (Table 6), and some aspects of this has been further adapted for sea urchins. There will be a change in EC legislation in terms of the classification of sea urchins as echinoderms, which in turn will change the legislation regarding the harvesting, processing, and retailing of sea urchins. At the time of submitting the final manuscript, this legislation has yet to be ratified.
The producers network in the North Atlantic region (ALGET2) has developed rigorous guidelines that cover the entire value chain on sustainable production or products from macroalga (ALGET2, 2022). These guidelines are derived from Norwegian, Icelandic, and Faroese legislation. France (CEVA) has also put some regulations in place for dried seaweed (CEVA, 2019) (Table 7).
5 Conclusion
Food agencies recommend the consumption of higher levels of aquatic food in the diet for improved health and sustainability. To achieve sustainable production, future gains in the global production of seafood are expected to come from the capture and culture of LTS. As such new raw materials, processes, and cuisines are developed, there will inevitably be new challenges pertaining to food safety and nutritional content. To address this, standards, laws, and regulations to be used when assessing the nutritional value and food safety of LTS have been identified. The global FAO Codex, in addition to the regional EC and US regulations, have been used as main sources, even though some national regulations also exist.
As LTS are mainly extractive species, or feed on such species, the nutritional composition and level of food hazards vary with the species, geographical location, and post-harvest handling. As mentioned, levels of contaminants may originate from a variety of sources, including geochemical processes, leaching soils, sewage, or industrial activities. Due to the variation of species, locations, and the novelty of a proportion of LTS on the markets, more species-specific data on nutritional contents and associated limits of safe consumption are warranted.
A widely used method when assessing food safety when specific MLs are missing for food hazards associated with the species, organism, or product is to use the MLs for comparable seafood items. For example, sea cucumbers, which are widely consumed in Asia, have not been assigned detailed MLs for the content of heavy metals by the CAC (FAO/WHO, 1995), the EC, or the National Standard of China. Risk assessments of such organisms may therefore be performed through comparison with MLs given by the EC (EC, 2006b) for similar organisms, such as “bivalves”, or the ML by the National Standard of China (NSC) for “aquatic animals and their products”(CFDA, 2017; Elvevoll et al., 2022).
In addition, the lack of consumption data (on quantities, frequencies, products, or species) challenges more general food safety and nutritional assessments. When such vital data are missing, a possible approach may be to perform an assessment of daily or weekly consumption specific limits of intake of the nutrients (NRV or UL), and hazards (TWI or BMD) may also be used.
It is important to closely monitor and analyze the nutrients and contaminants present in LTS to make informed decisions about the risks associated with consuming such species. Doing so can ensure that the food supply remains safe and healthy for all. In addition, more rigorous monitoring and analysis of consumption data is needed to enable more valid risk assessments and guide the utilization of LTS.
Author contributions
EE: Conceptualization, Funding acquisition, Investigation, Project administration, Writing – original draft, Writing – review & editing. K-EE: Writing – review & editing. MA: Funding acquisition, Project administration, Writing – review & editing. NB: Writing – review & editing.
Funding
This work was funded by UiT, The Arctic University of Norway, project SECURE, Cristin grant ID 2061344; and by the EU’s H2020 Research and Innovation Programme under grant agreement no. 818173, the AquaVitae project.
Acknowledgments
The author EE thanks the Portuguese Institute for Sea and Atmosphere, I. P. (IPMA, IP), for its hospitality and support during her sabbatical stay at IPMA, Lisbon, in the spring of 2023.
Conflict of interest
The authors declare that the research was conducted in the absence of any commercial or financial relationships that could be construed as a potential conflict of interest.
Publisher’s note
All claims expressed in this article are solely those of the authors and do not necessarily represent those of their affiliated organizations, or those of the publisher, the editors and the reviewers. Any product that may be evaluated in this article, or claim that may be made by its manufacturer, is not guaranteed or endorsed by the publisher.
References
Aakre I., Doblaug Solli D., Wik Markhus M., K. Mæhre H., Dahl L., Henjum S., et al. (2021). Commercially available kelp and seaweed products – valuable iodine source or risk of excess intake? Food & Nutr. Res. 65. doi: 10.29219/fnr.v65.7584
Aakre I., Tveito Evensen L., Kjellevold M., Dahl L., Henjum S., Alexander J., et al. (2020). Iodine status and thyroid function in a group of seaweed consumers in Norway. Nutrients 12 (11), 3483. doi: 10.3390/nu12113483
Adhikari S., Schop M., de Boer I. J. M., Huppertz T. (2022). Protein quality in perspective: A review of protein quality metrics and their applications. Nutrients 14 (5), 947. doi: 10.3390/nu14050947
Afonso C., Matos J., Guarda I., Gomes-Bispo A., Gomes R., Cardoso C., et al. (2020). Bioactive and nutritional potential of Alaria esculenta and Saccharina latissima. J. Appl. Phycology 33, 501–513. doi: 10.1007/s10811-020-02298-8
Afshin A., Sur P. J., Fay K. A., Cornaby L., Ferrara G., Salama J. S., et al. (2019). Health effects of dietary risks in 195 countries 1990-2017: a systematic analysis for the Global Burden of Disease Study 2017. Lancet 393 (10184), 1958–1972. doi: 10.1016/S0140-6736(19)30041-8
ALGET2. (2022). Guidelines for wild growing and cultivated seaweed for use in food in the North Atlantic region. Available at: https://www.norgesvel.no/alget2a/guidelines (Accessed August 2022).
ANSES. (2020). Opinion of the French Agency for Food, Environmental and Occupational Health & Safety on “maximum cadmium levels for seaweed intended for human consumption” Request No 2017-SA-0070 (Maisons-Alfort, France: ANSES). Available at: https://www.anses.fr/en/system/files/ERCA2017SA0070EN.pdf (Accessed 3 October 2022).
Banach J. L., Hoek-van den Hil E. F., van der Fels-Klerx H. J. (2020). Food safety hazards in the European seaweed chain. Comprehens. Rev. Food Sci. Food Safety 19, 332–364. doi: 10.1111/1541-4337.12523
Barone G., Storelli A., Meleleo D., Dambrosio A., Garofalo R., Busco A., et al. (2021). Levels of mercury, methylmercury and selenium in fish: insights into children food safety. Toxics 9 (2), 39. doi: 10.3390/toxics9020039
Blikra M. J., Altintzoglou T., Løvdal T., Rognså G., Skipnes D., Skåra T., et al. (2021). Seaweed products for the future: Using current tools to develop a sustainable food industry. Trends Food Sci. Technol. 118, 765–776. doi: 10.1016/j.tifs.2021.11.002
Brantsæter A. L., Knutsen H. K., Johansen N. C., Nyheim K. A., Erlund I., Meltzer H. M., et al. (2018). Inadequate iodine intake in population groups defined by age, life stage and vegetarian dietary practice in a norwegian convenience sample. Nutrients 10 (2), 230. doi: 10.3390/nu10020230
CEVA. (2019). Edible seaweed and microalgae Regulatory status in France and Europe 2019 update.). Available at: https://www.ceva-algues.com/wp-content/uploads/2020/03/CEVA-Edible-algae-FR-and-EU-regulatory-update-2019.pdf (Accessed 18 September 2020).
CFDA. (2017). National Food Safety Standard for Maximum Levels of Contaminants in Foods (GB 2762-2017). Available at: https://apps.fas.usda.gov/newgainapi/api/report/downloadreportbyfilename?filename=China%20Releases%20the%20Standard%20for%20Levels%20of%20Contaminants%20in%20Foods%20_Beijing_China%20-%20Peoples%20Republic%20of_5-9-2018.pdf (Accessed 20 March 2022).
Chain E. (2010). Scientific Opinion on marine biotoxins in shellfish – Emerging toxins: Ciguatoxin group. Efsa J. 8 (6), 1627. doi: 10.2903/j.efsa.2010.1627
Chandra A., Røsjø H., Eide I. A., Vigen T., Ihle-Hansen H., Orstad E. B., et al. (2020). Plasma marine n-3 polyunsaturated fatty acids and cardiovascular risk factors: data from the ACE 1950 study. Eur. J. Nutr. 59, 1505–1515. doi: 10.1007/s00394-019-02007-3
Chatzi L., Ierodiakonou D., Margetaki K., Vafeiadi M., Chalkiadaki G., Roumeliotaki T., et al. (2019). Associations of prenatal exposure to cadmium with child growth, obesity, and cardiometabolic traits. Am. J. Epidemiol. 188 (1), 141–150. doi: 10.1093/aje/kwy216
Chiocchetti G., Jadán-Piedra C., Vélez D., Devesa V. (2017). Metal(loid) contamination in seafood products. Crit. Rev. Food Sci. Nutr. 57 (17), 3715–3728. doi: 10.1080/10408398.2016.1161596
Coelho J. (2019). Arsenic speciation in algae: case studies in Europe. Compr. Analytical Chem. 85, 179–198. doi: 10.1016/bs.coac.2019.03.003
Córdoba-Tovar L., Marrugo-Negrete J., Barón P. R., Díez S. (2022). Drivers of biomagnification of Hg, As and Se in aquatic food webs: A review. Environ. Res. 204, 112226. doi: 10.1016/j.envres.2021.112226
Costello C., Cao L., Gelcich S., Cisneros-Mata M. A., Free C. M., Froehlich H. E., et al. (2020). The future of food from the sea. Nature 588, 95–100. doi: 10.1038/s41586-020-2616-y
Crona B. I., Wassénius E., Jonell M., Koehn J. Z., Short R., Tigchelaar M., et al. (2023). Four ways blue foods can help achieve food system ambitions across nations. Nature 616, 104–112. doi: 10.1038/s41586-023-05737-x
Duinker A., Kleppe M., Biancarosa I., Heldal H., Dahl L., et al. (2020). Knowledge update on macroalgae food and feed safety-based on data generated in the period 2014–2019 by the Institute of Marine Research, Norway (Bergen, Norway: Havforskningsinstituttet). Available at: Even Fjærehttps://www.hi.no/hi/nettrapporter/rapport-fra-havforskningen-en-2020-44 (Accessed 3 October 2020 2020).
Durazzo A., Di Lena G., Gabrielli P., Santini A., Lombardi-Boccia G., Lucarini M. (2022). Nutrients and bioactive compounds in seafood: quantitative literature research analysis. Fishes 7 (3), 132. doi: 10.3390/fishes7030132
EC. (2004). European Commision, No 853/2004 of the European Parliament and of the Council of 29 April 2004 laying down specific hygiene rules for food of animal origin. Available at: https://eur-lex.europa.eu/legal-content/EN/TXT/PDF/?uri=CELEX:32004R0853&qid=1665228867848&from=EN (Accessed 3 October 2022 2022).
EC. (2005a). Commission Regulation EU. 2073/2005 of 15 November 2005 on microbiological criteria for foodstuffs. Available at: https://eur-lex.europa.eu/legal-content/EN/TXT/PDF/?uri=CELEX:32005R2073&from=EN (Accessed 14 September 2022).
EC. (2005b). European Commission, No 396/2005 on maximum residue levels of pesticides in or on food and feed of plant and animal origin and amending Council Directive 91/414/EEC. EC. Available at: https://eur-lex.europa.eu/legal-content/EN/TXT/PDF/?uri=CELEX:32005R0396&from=EN (Accessed 4 October 2022).
EC. (2006a). Commission Regulation (EC) No 1881/2006 of 19 December 2006 setting maximum levels for certain contaminants in foodstuffs. Off. J. Eur. Union 49 (L 364), 5–24. Avalilable at: https://eur-lex.europa.eu/LexUriServ/LexUriServ.do?uri=OJ:L:2006:364:0005:0024:EN:PDF (Accessed 20 October 2020).
EC. (2006b). Commission Regulation EU. 1881/2006/ setting maximum levels for certain contaminants in foodstuffs. Available at: https://eur-lex.europa.eu/eli/reg/2006/1881/2022-07-01 (Accessed 14 September 2022).
EC. (2006c). European Commission (EC), No 1924/2006 on nutrition and health claims made on foods. Available at: https://eur-lex.europa.eu/LexUriServ/LexUriServ.do?uri=OJ:L:2006:404:0009:0025:En:PDF (Accessed 20 October 2020).
EC. (2010). Commision regulation (EU) No 116/2010 amending Regulation (EC) No 1924/2006 of the European Parliament and of the Council with regard to the list of nutrition claims. Available at: https://eur-lex.europa.eu/LexUriServ/LexUriServ.do?uri=OJ:L:2010:037:0016:0018:EN:PDF (Accessed 10 April 2023).
EC. (2011a). Commision Regulation EU., No 1169/2011 on the provision of food information to consumers. Available at: https://eur-lex.europa.eu/legal-content/EN/TXT/HTML/?uri=CELEX:32011R1169&from=EN#d1e2308-18-1 (Accessed 30 September 2022).
EC. (2011b). Commission Regulation EU., 835/2011 amending Regulation (EC) 1881/2006 as regards maximum levels for polycyclic aromatic hydrocarbons in foodstuffs. Available at: https://eur-lex.europa.eu/LexUriServ/LexUriServ.do?uri=OJ:L:2011:215:0004:0008:En:PDF (Accessed 14 September 2022).
EC. (2011c). Commission Regulation EU. 1259/2011 setting maximum levels of dioxins and PCBs in food. Available at: https://eur-lex.europa.eu/legal-content/en/ALL/?uri=CELEX%3A32011R1259 (Accessed 14 September 2022).
EC. (2012). Commision Regulation (EU), No 432/2012 of 16 May 2012 establishing a list of permitted health claims made on foods, other than those referring to thereduction of disease risk and to children’s development and health. Available at: https://eur-lex.europa.eu/LexUriServ/LexUriServ.do?uri=OJ:L:2012:136:0001:0040:en:PDF (Accessed 10 October 2020 2020).
EC. (2015a). Annex II to Regulation (EC) 854/2004 and Annex I to Regulation (EC) No. 2073/2005 on microbiological criteria for foodstuffs 2285/2015/EU. Available at: https://eur-lex.europa.eu/legal-content/EN/TXT/?uri=CELEX%3A32015R2285.
EC. (2015b). COMMISSION REGULATION (EU) 2015/2285 amending Annex II to Regulation (EC) No 854/2004 of official controls on products of animal origin intended for human consumption as regards certain requirements for live bivalve molluscs, echinoderms, tunicates and marine gastropods and Annex I to Regulation (EC) No 2073/2005 on microbiological criteria for foodstuffs. Available at: https://eur-lex.europa.eu/legal-content/EN/TXT/PDF/?uri=CELEX:32015R2285 (Accessed October 2022 2022).
EC. (2016). European Commission (Euroatom), 2016/52 laying down maximum permitted levels of radioactive contamination of food and feed following a nuclear accident or any other case of radiological emergency, and repealing Regulation (Euratom) No 3954/87 and Commission Regulations (Euratom) No 944/89 and (Euratom) No 770/90 ). Available at: https://eur-lex.europa.eu/legal-content/EN/TXT/PDF/?uri=CELEX:32016R0052&from=en (Accessed 4 October 2022 2022).
EC. (2017). Commission Regulation EU. 2017/644 laying down methods of sampling and analysis for the control of levels of dioxins, dioxin-like PCBs and non-dioxin-like PCBs in certain foodstuffs and repealing Regulation (EU) No 589/2014.). Available at: http://data.europa.eu/eli/reg/2017/644/oj (Accessed 10 September 2020).
EC. (2021). COMMISSION REGULATION (EU) 2021/1323 of 10 August 2021 amending Regulation (EC) No 1881/2006 as regards maximum levels of cadmium in certain foodstuffs. Available at: https://eur-lex.europa.eu/eli/reg/2021/1323/oj (Accessed 3 October 2022).
EFSA. (2008). European food safety authority., polycyclic aromatic hydrocarbons in food - scientific opinion of the panel on contaminants in the food chain. Efsa J. 6 (8), 724. doi: 10.2903/j.efsa.2008.724
EFSA. (2009a). Marine biotoxins in shellfish – Summary on regulated marine biotoxins. Efsa J. 7 (8), 1306. doi: 10.2903/j.efsa.2009.1306
EFSA. (2009b). Panel on contaminants in the food chain., scientific opinion on arsenic in food. Efsa J. 7 (10), 1351. doi: 10.2903/j.efsa.2009.1351
EFSA. (2010). Panel on contaminants in the food chain., scientific opinion on lead in food. Efsa J. 8 (4), 1570. doi: 10.2903/j.efsa.2010.1570
EFSA. (2013). Panel on Additives Products or Substances used in Animal Feed. Scientific Opinion on the safety and efficacy of iodine compounds (E2) as feed additives for all species: calcium iodate anhydrous and potassium iodide, based on a dossier submitted by HELM AG. Efsa J. 11 (2), 3101. doi: 10.2903/j.efsa.2013.3101
EFSA. (2014a). Panel on dietetic products, nutrition allergies., scientific opinion on dietary reference values for iodine. Efsa J. 12 (5), 3660. doi: 10.2903/j.efsa.2014.3660
EFSA. (2014b). Panel on dietetic products, nutrition allergies., scientific opinion on dietary reference values for selenium. Efsa J. 12 (10), 3846. doi: 10.2903/j.efsa.2014.3846
EFSA. (2015). Scientific Committee., Statement on the benefits of fish/seafood consumption compared to the risks of methylmercury in fish/seafood. Efsa J. 13 (1), 3982. doi: 10.2903/j.efsa.2015.3982
EFSA. (2018a). Overview on Tolerable Upper Intake Levels as derived by the Scientific Committee on Food (SCF) and the EFSA Panel on Dietetic Products, Nutrition and Allergies (NDA). Available at: https://www.efsa.europa.eu/sites/default/files/assets/UL_Summary_tables.pdf (Accessed 30 September 2022).
EFSA. (2018b). Risk for animal and human health related to the presence of dioxins and dioxin-like PCBs in feed and food. Efsa J. 16 (11), 5333–5664. doi: 10.2903/j.efsa.2018.5333
EFSA. (2020). Panel on Contaminants in the Food Chain Risk to human health related to the presence of perfluoroalkyl substances in food. Efsa J. 18 (9), e06223. doi: 10.2903/j.efsa.2020.6223
EFSA. (2022). Panel on Nutrition, Novel Foods and Allergens (NDA). Scientific advice related to nutrient profiling for the development of harmonised mandatory front-of-pack nutrition labelling and the setting of nutrient profiles for restricting nutrition and health claims on foods. Efsa J. 20 (4), e07259. doi: 10.2903/j.efsa.2022.7259
Eilertsen H. C., Eriksen G. K., Bergum J.-S., Strømholt J., Elvevoll E., Eilertsen K.-E., et al. (2022). Mass cultivation of microalgae: I. Experiences with vertical column airlift photobioreactors, diatoms and CO2 sequestration. Appl. Sci. 12 (6), 3082. doi: 10.3390/app12063082
Elvevoll E. O., James D., Toppe J., Gamarro E. G., Jensen I.-J. (2022). Food safety risks posed by heavy metals and persistent organic pollutants (POPs) related to consumption of sea cucumbers. Foods 11 (24), 3992. doi: 10.3390/foods11243992
Etheridge S. M. (2010). Paralytic shellfish poisoning: Seafood safety and human health perspectives. Toxicon 56 (2), 108–122. doi: 10.1016/j.toxicon.2009.12.013
FAO. (2022). Thinking about the future of food safety - A foresight report (Rome, Italy). Available at: https://www.fao.org/3/cb8667en/online/cb8667en.html (Accessed 1 June 2022).
FAO/WHO. (1995). General Standard for contaminants and toxins in food and feed, CXS 193-1995, Revised 2019. Codex Alimentarus (CAC) (Rome, Italy: FAO). Available at: https://www.fao.org/fao-who-codexalimentarius/sh-proxy/en/?lnk=1&url=https%253A%252F%252Fworkspace.fao.org%252Fsites%252Fcodex%252FStandards%252FCXS%2B193-1995%252FCXS_193e.pdf (Accessed 10 September 2020 2020).
FAO/WHO. (2001). FOOD LABELLING - COMPLETE TEXTS (Rome, ITaly: FAO/WHO). Available at: https://www.fao.org/uploads/media/Codex_2001_food_labelling.pdf (Accessed 1 April 2022).
FAO/WHO. (2011a). Report of the joint FAO/WHO expert Consultation on the risks and benefits of fish consumption (Rome, Italy: Food and Agriculture Organization of the United Nations). Available at: https://apps.who.int/iris/handle/10665/44666 (Accessed 12 December 2012).
FAO/WHO. (2011b). Safety evaluation of certain contaminants in food, prepared by the seventy-second meeting of the Joint FAO/WHO Expert Committee on food additives. Available at: https://apps.who.int/iris/handle/10665/44520 (Accessed 14 September 2020).
FAO/WHO. (2013). GUIDELINES FOR USE OF NUTRITION AND HEALTH CLAIMS (Rome, Italy: FAO/WHO). Available at: https://www.fao.org/ag/humannutrition/32444-09f5545b8abe9a0c3baf01a4502ac36e4.pdf (Accessed 1 Aprile 2022).
FAO/WHO. (2021). Technical guidance for the development of the growing area aspects of Bivalve Mollusc Sanitation Programmes (Rome, Italy). Available at: https://www.fao.org/documents/card/en/c/cb5072en (Accessed 10 September 2022).
FAO/WHO. (2022). Report of the expert meeting on food safety for seaweed – Current status and future perspectives. Rome, 28–29 0ctober 2021 (Rome, Italy). Available at: https://www.fao.org/3/cc0846en/cc0846en.pdf (Accessed 3 October 2022).
FAO/WHO/UNU. (2007). Protein and amino acid requirements in human nutrition (Geneva, Switzerland: FAO). Available at: https://apps.who.int/iris/handle/10665/43411 (Accessed 10 September 2020).
Gephart J. A., Henriksson P. J. G., Parker R. W. R., Shepon A., Gorospe K. D., Bergman K., et al. (2021). Environmental performance of blue foods. Nature 597 (7876), 360–365. doi: 10.1038/s41586-021-03889-2
Gilsenan M. B. (2011). Nutrition & health claims in the European Union: A regulatory overview. Trends Food Sci. Technol. 22 (10), 536–542. doi: 10.1016/j.tifs.2011.03.004
Golden C. D., Koehn J. Z., Shepon A., Passarelli S., Free C. M., Viana D. F., et al. (2021). Aquatic foods to nourish nations. Nature 598 (7880), 315–320. doi: 10.1038/s41586-021-03917-1
Gordon L. J., Bignet V., Crona B., Henriksson P. J. G., Van Holt T., Jonell M., et al. (2017). Rewiring food systems to enhance human health and biosphere stewardship. Environ. Res. Lett. 12 (10), 100201. doi: 10.1088/1748-9326/aa81dc
Guo W., Pan B., Sakkiah S., Yavas G., Ge W., Zou W., et al. (2019). Persistent organic pollutants in food: contamination sources, health effects and detection methods. Int. J. Environ. Res. Public Health 16 (22), 4361. doi: 10.3390/ijerph16224361
Hallström E., Bergman K., Mifflin K., Parker R., Tyedmers P., Troell M., et al. (2019). Combined climate and nutritional performance of seafoods. J. Cleaner Production 230, 402–411. doi: 10.1016/j.jclepro.2019.04.229
Hay I., Hynes K. L., Burgess J. R. (2019). Mild-to-moderate gestational iodine deficiency processing disorder. Nutrients 11 (9), 1974. doi: 10.3390/nu11091974
Hogstad S., Ceredrberg D., Eriksen H., Kallander B., Olafssson G., Mikkelsen B. (2023). “A Nordic approach to food safety risk management of seaweed for use as food,” in TemaNord (Copenhagen, Denmark: Nordic Council of Ministers). Copyright © 2012 The Korean Society for Preventive Medicine.
Hong Y. S., Kim Y. M., Lee K. E. (2012). Methylmercury exposure and health effects. J Prev Med Public Health, The Korean Society for Preventive Medicine Vol. 45. 353–363. doi: 10.3961/jpmph.2012.45.6.353
IARC. (2012). Arsenic, Metals, Fibres and Dusts. Lyon (FR): International Agency for Research on Cancer. Working Group on the Evaluation of Carcinogenic Risks to Humans. Available at: https://www.ncbi.nlm.nih.gov/books/NBK304375/ (Accessed September 2020 2020).
Innes J. K., Calder P. C. (2020). Marine omega-3 (n-3) fatty acids for cardiovascular health: An update for 2020. Int. J. Mol. Sci. 21, 1362. doi: 10.3390/ijms21041362
Ittermann T., Albrecht D., Arohonka P., Bilek R., de Castro J. J., Dahl L., et al. (2020). Standardized map of iodine status in Europe. Thyroid® 30 (9), 1346–1354. doi: 10.1089/thy.2019.0353
Jacobsen M., Bianchi M., Trigo J. P., Undeland I., Hallström E., Bryngelsson S. (2023). Nutritional and toxicological characteristics of Saccharina latissima, Ulva fenestrata, Ulva intestinalis, and Ulva rigida: a review. Int. J. Food Properties 26 (1), 2349–2378. doi: 10.1080/10942912.2023.2246677
Jayasekara C., Mendis E., Kim S.-K. (2020). “Seafood in the human diet for better nutrition and health,” in Encyclopedia of Marine Biotechnology, John Wiley & Sons Ltd. 2939–2959.
Jeffery B., Barlow T., Moizer K., Paul S., Boyle C. (2004). Amnesic shellfish poison. Food Chem. Toxicol. 42 (4), 545–557. doi: 10.1016/j.fct.2003.11.010
Jensen I. J., Bodin N., Govinden R., Elvevoll E. O. (2023). Marine capture fisheries from western Indian ocean: an excellent source of proteins and essential amino acids. Foods 12 (5), 1015. doi: 10.3390/foods12051015
Jensen I. J., Walquist M., Liaset B., Elvevoll E. O., Eilertsen K. E. (2016). Dietary intake of cod and scallop reduces atherosclerotic burden in female apolipoprotein E-deficient mice fed a Western-type high fat diet for 13 weeks. Nutr. Metab. 13. doi: 10.1186/s12986-016-0068-z
Jinadasa B. K. K. K., Jayasinghe G. D. T. M., Pohl P., Fowler S. W. (2021). Mitigating the impact of mercury contaminants in fish and other seafood—A review. Mar. Pollut. Bull. 171, 112710. doi: 10.1016/j.marpolbul.2021.112710
Jong E. C. (2017). “Chapter 34 - fish and shellfish poisoning: toxic syndromes,” in The Travel and Tropical Medicine Manual, Fifth Edition. Eds. Sanford C. A., Pottinger P. S., Jong E. C. (Elsevier), 451–456. doi: 10.1016/B978-0-323-37506-1.00034-9
Kippler M., Bottai M., Georgiou V., Koutra K., Chalkiadaki G., Kampouri M., et al. (2016). Impact of prenatal exposure to cadmium on cognitive development at preschool age and the importance of selenium and iodine. Eur. J. Epidemiol. 31 (11), 1123–1134. doi: 10.1007/s10654-016-0151-9
Krause G., Le Vay L., Buck B. H., Costa-Pierce B. A., Dewhurst T., Heasman K. G., et al. (2022). Prospects of low trophic marine aquaculture contributing to food security in a net zero-carbon world. Front. Sustain. Food Syst. 6. doi: 10.3389/fsufs.2022.875509
Landrigan P. J., Stegeman J. J., Fleming L. E., Allemand D., Anderson D. M., Backer L. C., et al. (2020). Human health and ocean pollution. Ann. Global Health 86 (1), 151. doi: 10.5334/aogh.2831
Langdal A., Eilertsen K.-E., Kjellevold M., Heimstad E. S., Jensen I.-J., Elvevoll E. O. (2023). Climate performance, environmental toxins and nutrient density of the underutilized norwegian orange-footed sea cucumber (Cucumaria frondosa). Foods 12 (1), 114. doi: 10.3390/foods12010114
Lewis J. (2019). Codex nutrient reference values - especially for vitamins, minerals and protein (Rome). Available at: https://www.fao.org/3/ca6969en/CA6969EN.pdf (Accessed 15 September 2022).
Maehre H. K., Jensen I.-J., Elvevoll E., Eilertsen K.-E. (2015a). ω-3 fatty acids and cardiovascular diseases: effects, mechanisms and dietary relevance. Int. J. Mol. Sci. 16 (9), 22636–22661. doi: 10.3390/ijms160922636
Maehre H. K., Jensen I. J., Elvevoll E. O., Eilertsen K. E. (2015b). Omega-3 fatty acids and cardiovascular diseases: Effects, Mechanisms and dietary relevance. Int. J. Mol. Sci. 16 (9), 22636–22661. doi: 10.3390/ijms160922636
Martinez-Finley E. J., Aschner M. (2014). Recent advances in mercury research. Curr. Environ. Health Rep. 1 (2), 163–171. doi: 10.1007/s40572-014-0014-z
Mellingen R. M., Myrmel L. S., Rasinger J. D., Lie K. K., Bernhard A., Madsen L., et al. (2022). Dietary selenomethionine reduce mercury tissue levels and modulate methylmercury induced proteomic and transcriptomic alterations in hippocampi of adolescent BALB/c mice. Int. J. Mol. Sci. 23 (20), 12242. doi: 10.3390/ijms232012242
Muhsin M. F., Fujaya Y., Hidayani A., Fazhan H., Wan Mahari W. A., Lam S. S., et al. (2023). Bridging the gap between sustainability and profitability: unveiling the untapped potential of sea cucumber viscera. Peer J. 11, e16252. doi: 10.7717/peerj.16252
Murray C. J. L., Aravkin A. Y., Zheng P., Abbafati C., Abbas K. M., Abbasi-Kangevari M., et al. (2020). Global burden of 87 risk factors in 204 countries and territories 1990–2019: a systematic analysis for the Global Burden of Disease Study 2019. Lancet 396 (10258), 1223–1249. doi: 10.1016/S0140-6736(20)30752-2
NAP. (2019). Dietary Reference Intakes for Sodium and Potassium. Appendix J Dietary Reference Intakes Summary Tables (Washington (DC) (US: National Academies Press National Academies of Sciences, Engineering, and Medicine) (Accessed 30 September 2022).
O'Mahony M. (2018). EU regulatory risk management of marine biotoxins in the marine bivalve mollusc food-chain. Toxins (Basel) 10 (3), 118. doi: 10.3390/toxins10030118
Peñalver R., Lorenzo J. M., Ros G., Amarowicz R., Pateiro M., Nieto G. (2020). Seaweeds as a functional ingredient for a healthy diet. Mar. Drugs 18 (6):301. doi: 10.3390/md18060301
Poore J., Nemecek T. (2018a). Reducing food's environmental impacts through producers and consumers. Science 360 (6392), 987–992. doi: 10.1126/science.aaq0216
Poore J., Nemecek T. (2018b). Reducing foods environmental impacts through producers and consumers. Science 360 (6392), 987–992. doi: 10.1126/science.aaq0216
Rimm E. B., Appel L. J., Chiuve S. E., Djousse L., Engler M. B., Kris-Etherton P. M., et al. (2018). Seafood long-chain n-3 polyunsaturated fatty acids and cardiovascular disease: A science advisory from the American Heart Association. Circulation 138 (1), 35–47. doi: 10.1161/Cir.0000000000000574
Sampaio G. R., Guizellini G. M., da Silva S. A., de Almeida A. P., Pinaffi-Langley A. C. C., Rogero M. M., et al. (2021). Polycyclic aromatic hydrocarbons in foods: biological effects, legislation, occurrence, analytical methods, and strategies to reduce their formation. Int. J. Mol. Sci. 22 (11), 6010. doi: 10.3390/ijms22116010
SAPEA. (2017). Food from the oceans: how can more food and biomass be obtained from the oceans in a way that does not deprive future generations of their benefits? No.1 (Berlin: Science Advice for Policy by European Academies).
UN. (2015). Transforming our world : the 2030 Agenda for Sustainable Development (Accessed 7 February 2023).
van den Berg M., Kypke K., Kotz A., Tritscher A., Lee S. Y., Magulova K., et al. (2017). WHO/UNEP global surveys of PCDDs, PCDFs, PCBs and DDTs in human milk and benefit–risk evaluation of breastfeeding. Arch. Toxicol. 91 (1), 83–96. doi: 10.1007/s00204-016-1802-z
Venugopal V., Gopakumar K. (2017). Shellfish: nutritive value, health benefits, and consumer safety. Compr. Rev. Food Sci. Food Saf. 16 (6), 1219–1242. doi: 10.1111/1541-4337.12312
VKM. (2022). Benefit and risk assessment of fish in the Norwegian diet. Report 17 (Norwegian Scientific Committee for Food and Environment (VKM) (Accessed 07 Juni 2022).
Watkins S. M., Reich A., Fleming L. E., Hammond R. (2008). Neurotoxic shellfish poisoning. Mar. Drugs 6 (3), 431–455. doi: 10.3390/md6030431
WHO. (2007). Assessment of iodine deficiency disorders and monitoring their elimination: a guide for programme managers. 3rd ed (Geneva, Switzerland: World Health Organization).
Winther K. H., Rayman M. P., Bonnema S. J., Hegedüs L. (2020). Selenium in thyroid disorders — essential knowledge for clinicians. Nat. Rev. Endocrinol. 16 (3), 165–176. doi: 10.1038/s41574-019-0311-6
Wright A. C., Fan Y., Baker G. L. (2018). Nutritional value and food safety of bivalve molluscan shellfish. J. Shellfish Res. 37 (4), 695–708, 614. doi: 10.2983/035.037.0403
Yamashita Y., Yamashita M., Iida H. (2013). Selenium content in seafood in Japan. Nutrients 5 (2), 388–395. doi: 10.3390/nu5020388
Keywords: seafood, low trophic, aquatic, nutrients, contaminants, heavy metals
Citation: Elvevoll EO, Eilertsen K-E, Aschan M and Bandarra NM (2023) New marine low trophic resources as food: nutritional and food safety aspects. Front. Aquac. 2:1254038. doi: 10.3389/faquc.2023.1254038
Received: 06 July 2023; Accepted: 13 October 2023;
Published: 01 November 2023.
Edited by:
Charles Odilichukwu R. Okpala, University of Georgia, United StatesReviewed by:
Feyisayo Adepoju, Ural Federal University, RussiaMohammad Shamsur Rahman, University of Dhaka, Bangladesh
Copyright © 2023 Elvevoll, Eilertsen, Aschan and Bandarra. This is an open-access article distributed under the terms of the Creative Commons Attribution License (CC BY). The use, distribution or reproduction in other forums is permitted, provided the original author(s) and the copyright owner(s) are credited and that the original publication in this journal is cited, in accordance with accepted academic practice. No use, distribution or reproduction is permitted which does not comply with these terms.
*Correspondence: Edel Oddny Elvevoll, ZWRlbC5lbHZldm9sbEB1aXQubm8=