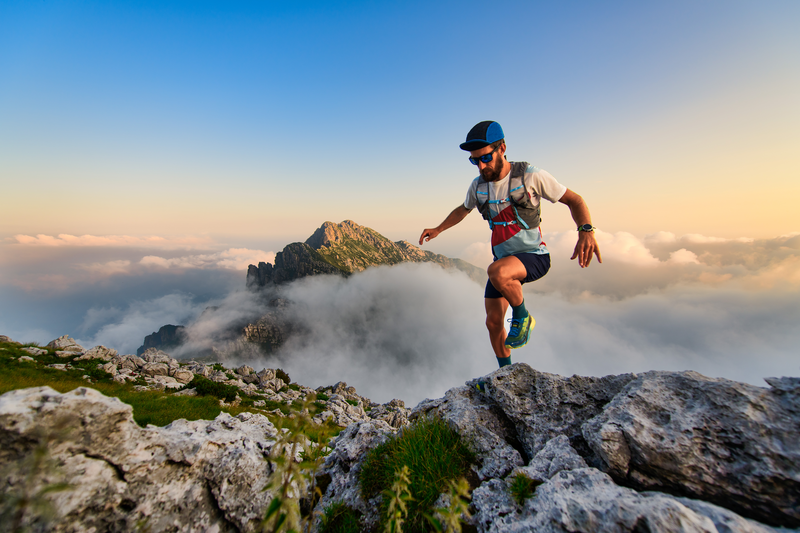
94% of researchers rate our articles as excellent or good
Learn more about the work of our research integrity team to safeguard the quality of each article we publish.
Find out more
ORIGINAL RESEARCH article
Front. Aquac. , 10 October 2023
Sec. Disease and Health Management
Volume 2 - 2023 | https://doi.org/10.3389/faquc.2023.1239402
This article is part of the Research Topic Chrono-Immunonutrition in Aquaculture towards Robust and Resilient fish View all 7 articles
Atlantic salmon (Salmo salar) were fed diets containing black soldier fly larvae (BSFL) meal at two inclusion levels for 13 months in open sea-cages. BSFL meal replaced plant-based ingredients and dietary insect meal inclusion levels were at 5% and 10%. A commercial salmon diet was fed as a control diet. Fish were reared from ~173 g to ~ 4 kg and were randomly distributed into nine open sea-cages with ~6000 salmon/cage (12×12 m2; 1900 m3). Fish from the sea-cages were sampled at two time points (mid and final samplings) to study the dietary effects of BSFL meal on the general health and welfare. Monthly assessments of sea-lice and gill score were conducted to evaluate the overall well-being of the salmon. The findings from the current study revealed that dietary inclusion of BSFL meal up to 10% did not have any significant effects in general growth, welfare or survival. However, significant positive response was observed in the general skin mucosal, hematological, and gene expression profiles of salmon. Notably, the group of salmon fed with 5% BSFL meal showed a significant decrease in plasma aspartate transaminase and alanine transaminase. Also, a significantly higher expression of interleukin1β in both skin and gill along with upregulation of matrix metallopeptidase9 and mucin18 in gill were observed in salmon fed BSFL at 5%, which aided in increased immune responses. Apart from that, this group had significantly higher mucus secretions, decreased cortisol response and increased number of erythrocytes. Furthermore, the delousing stress had a significant effect on the plasma cortisol, and these responses were independent of the dietary effect. Moreover, these immune responses behaved differently at different fish size and time points, acknowledging the influence of various factors in immune modulation. Overall, the findings from this study showed the effects of dietary BSFL meal to modulate the immune status of salmon. This study aims to fill the existing knowledge gaps regarding the impact of incorporating BSFL meal as a functional feed ingredient into the salmon diet on health and immune status replicating real farm conditions.
Graphical Abstract B1, B2, B3-different blocks with each block representing the three dietary group in cages, to eliminate the cage-position effects. BSFL, black soldier fly larvae; S, south; N, North.
Aquaculture has been a rapidly developing industry during the past four decades and is expected to continue in the foreseeable future to feed the growing population (Garlock et al., 2022). Atlantic salmon (Salmo salar) is one of the most farmed species (2.7 MT), accounting for 32.6 % of marine and coastal aquaculture of all finfish species in 2020 (FAO, 2022). Norway is the world’s leading producer of Atlantic salmon, producing around 1.4 MT in 2020. However, the downside of intensification of salmon industry has been the economic losses, due to mortality caused by salmon louse and treatments, infectious diseases, mucosal health, or multiple environmental stressors (Norwegian fish health report 2022). It has been shown that nutritional approach using functional feed ingredients (e.g., β-glucans or mannan-oligosaccharides, nucleotides, or plant extracts) can modulate the immune system of fish species (Ringø et al., 2012) and can protect fish from disease, parasites and various stressors (Hossain et al., 2023), thereby decreasing the mortality rate.
Recently, great attention has been given to the potential of using insect meal as sustainable feed ingredients due to its good nutritional profile (well-balanced content of amino acids, fatty acids, vitamins and minerals), presence of immunostimulant and bioactive compounds (Van Huis and Gasco, 2023). Among the approved insect species for its inclusion in aquafeed, black soldier fly larvae (BSFL) have gained widespread attention at research and commercial scale (Veldkamp et al., 2022b). This popularity of the black soldier fly might be due to its short life cycle, fast larval growth and ability to convert a variety of waste into valuable mass, thereby being an advantage for upscaling the insect production for use by the feed sector (Tran et al., 2022). Dietary BSFL meal can replace both marine and plant-based ingredients (such as fish meal (FM) and soy protein) and an inclusion up to 30% in aquafeed is possible without affecting the growth performance of fish species (Liland et al., 2021). However, dietary inclusion of BSFL meal should not only ensure growth but also modulate the immune responses and thus improve the health of farmed fish species (Mouithys-Mickalad et al., 2020).
The examination of the immunomodulatory effects of BSFL meal as a functional feed ingredient in fish serves to offer insights into the mechanisms and behavior of immune parameters in salmon fed BSFL-based diets. The BSFL meal contains diverse bioactive and immunostimulant compounds such as antimicrobial peptides (AMPs) (e.g., α-helical peptides, cysteine-rich peptides, proline-rich peptides, glycine-rich peptides), fatty acids (lauric acid) and polysaccharides (chitin and chitosan) (Veldkamp et al., 2022a). Insect AMPs play a key part in the innate immune response and have been shown to improve fish resistance to diseases (Hu et al., 2021; Rashidian et al., 2021). Lauric acid is known for its antiviral and antibacterial activities (Lieberman et al., 2006) and is also considered as a good source of energy for salmon (Belghit et al., 2019b). Furthermore, it has been shown that BSFL meal contains chitin, a polysaccharide that can function as a potential prebiotic in animal feed (Song et al., 2014). Studies in juvenile Mozambique tilapia (Oreochromis mossambicus) showed that incorporating small doses (0.5 g/kg feed) of dried prepupae from BSF in their diet had enhanced the hematological parameters (Ushakova et al., 2018). Moreover, dietary inclusion of housefly (Musca domestica) at low levels (between, 0.75% and 7.5%) increased the innate immunity and disease resistance of red sea bream (Pagrus major) (Ido et al., 2015) and black carp (Mylopharyngodon piceus) (Ming et al., 2013). An inclusion of dietary insect meals at low levels might act as a functional feed ingredient with potential health beneficial effects for fish species.
Therefore, based on this knowledge, the current study aimed to investigate the effects of dietary inclusion of BSFL meal as a functional feed ingredient in the Atlantic salmon diet (5% and 10%), reared in open sea-cages over the course of one year, aiming to simulate commercial farming conditions. The study aimed to assess the mucosal health and innate immune responses of salmon when exposed to chronic stress (pathogens and parasites, fluctuating temperature, salinity and water quality) and acute stress (delousing and handling).
Three experimental diets were produced by Skretting Norway (Averøy, Norway) and were formulated to be iso-nitrogenous and iso-lipidic diets, containing the same level of FM (100 g/kg, Table 1). A control diet (Control) was formulated using a standard commercial recipe, containing FM and plant-based protein (guar meal, horse beans, sunflower meal and soy protein concentrate). The main lipid sources in all diets were fish oil and vegetable oils (rapeseed oil and camelina oil). The two experimental diets were formulated by using partially defatted BSFL meal (Protix, 53% crude protein, and 13% crude lipid). Plant-based ingredients were replaced with BSFL meal at 14% (BSFL 5%) and 26% (BSFL 10%) for 4.5 mm pellets. While for the 9.0 mm pellets, 19% (BSFL 5%) and 34% (BSFL 10%) of plant-based ingredients were replaced by the BSFL meal. These two levels of inclusion for BSFL meal were chosen: a low level (5%), which is currently feasible given the available quantities of insect meal, and a high level (10%), which could potentially represent the future standard for insect protein meals in animal diets, expected to be achievable in 10 to 15 years when the insect market can provide larger quantities of insect products (Veldkamp et al., 2022b).
Table 1 Formulation (%) and proximate composition (% of dry matter) of experimental diets (4.5 mm, and 9.0 mm) fed to Atlantic salmon during 13 months in open sea-cages.
The proximate composition of the diets was analyzed according to AOAC (2010) methods. Samples were freeze dried for 48 h (FreeZone 18 Liter Console, Labconco, USA) to obtain the dry matter. The dried feed samples were ground into a fine powder for the analysis of nitrogen (N), fat and ash content. Nitrogen content was analyzed using a CHNS elemental analyzer (Vario Macro Cube, Elementar Analysensysteme GmbH, Langenselbold, Germany) and quantified (AOAC, 2010). The instrument was calibrated with Ethylenediamine tetraacetic acid (Leco Corporation, Saint Joseph, MI, USA). Sulfanilamide (Alfa Aesar GmbH & Co, Karlsruhe, Germany) and a standard meat reference material (SMRD 2000, LGC Standards, Teddington, UK) were used as control sample. Crude protein was calculated as N% × 6.25. Crude lipid was measured gravimetrically after acid hydrolysis. Gross energy was measured by adiabatic bomb calorimetry using manufactures protocol (Parr Instrument Co., Moline, IL, USA). The feed formulation and analyzed nutrient composition of the feeds are provided in Table 1.
The feeding trial was conducted at Austevoll research station, Institute of Marine Research, Norway, from July 2021 to August 2022 (13 months). On the 22nd of July 2021, a total of 56000 Atlantic salmon post smolts (Aquagen strain) were transported from Biofish AS (Tørvikbygd) to the Austevoll station by using a well boat. Approximatively 6000 fish were distributed into each sea-cage using sensors, which detect and record the fish before releasing them into each cage. The fish were randomly distributed into nine open sea-cages (12×12 m2;1922 m3). Diets were assigned to the sea-cages in triplicates using a randomized block design, containing three blocks (B1, B2, and B3) to account for cage position effects. The fish were acclimatized to the control diet one week prior to the start of the experiment. The feed was dispersed using automatic surface feeders with meals given every 3 min during seven hours a day (20 doses/h). The feeding volume were recorded and registered daily using the Mercatus Farmer software (Scale AQ, Norway) to estimate the biomass and feed conversion ratio. The pellet size (4.5- and 9.0-mm pellet) and size of meals were adjusted according to the fish size, biomass and feeding behavior by visual observation from the surface. Lumpfish (Cyclopterus lumpus) and Ballan wrasse (Labrus bergylta) were added to each cage to keep lice levels low (Imsland et al., 2014; Imsland et al., 2018), according to standard routines at the station (Ratio of cleaner fish to salmon was 12% with the same ratio in all cages). The number of dead fish (salmon and cleaner fish) were calculated by counting them daily through visual observation. Towards the end of the trial (week 21-30), the dead fish were macroscopically examined. Microbiological and histological analyses were performed (Pharmaq Analytic, Bergen, Norway) on gill and heart of dead salmon to diagnose for potential bacterial or viral infections.
Temperature, dissolved oxygen and salinity in the Austevoll research station were monitored throughout the year using Mercatus software (Scale AQ, Norway). The average temperature, salinity and oxygen at different depths (0.5, 5, 10, and 20 m) for the entire feeding period were recorded. The monthly average for temperature (°C), salinity (‰) and oxygen (% of saturation) (10 m depth) for an entire year from July 2021- August 2022, ranged between 6-15 °C, 17-30 ‰, and 94-108 (% of saturation), respectively. At 10 m depth, the temperature (°C) range was 14±0.3 (June-August), 13±0.1 (September-November), 8±0.1 (December-February), and 14±0.2 (March-May). Likewise, salinity (‰) was 32±1.2 (June-August), 23±2.0 (September-November), 30±0.6 (December-February), and 32±0.3 (March-May). Oxygen level (% of saturation) was 100±0.6 (June-August), 97±0.5 (September-November), 96±0.5 (December-February), and 101±0.6 (March-May).
Fish were monitored for general growth, health and welfare parameters at multiple time points during the feeding trial:
-Initial sampling (July 2021): 50 fish/cage were removed for recording weight and length.
-Weekly samplings: 20 fish/cage/week were removed to monitor the sea-lice and gill score as a part of welfare assessment for the whole experiment. The gill scoring was qualitative and was evaluated per fish based on a scale of 0 to 5, where 0 represents no signs of infection and 5 represents severe infection (Taylor et al., 2009). The sea-lice (Lepeophtheirus salmonis) were counted manually and the permissible limit in Norwegian salmon farms is 0.2 mature females per salmon during spring and 0.5 for rest of the year (Norwegian Food Safety Authority).
-Mid (April 2022; fed for 9 months) and final samplings (August 2022; fed for 13 months) were conducted to obtain skin mucus (10 fish/cage), blood, plasma, gill and skin tissue (6 fish/cage). Additionally, 6 salmon were sampled from each sea-cage for fillet quality and sensory analysis (results described elsewhere).
-Delousing: During the entire experimental trial, a total of three thermal delousing was performed for different cages before exceeding the recommended limits by the Norwegian Food Safety Authority. In the current study, samples were taken from one of the delousing performed in May 2022 (fed for 10 months). In this sampling, six fish/cage were sacrificed to obtain the mucus and plasma samples. Fish were sampled before delousing (pre-stress) and after delousing (post-stress). Thermal delousing was performed using a thermolicer according to the approved guidelines of Norwegian Food Safety Authority. In short, the fish were pumped from each cage into the thermolicer where it passes through the processing loop for 30 seconds, with a water temperature of 28-32 °C. After the treatment, salmon was pumped back into the same sea-cage.
The initial body weight (IBW; 173 ± 2.7 g), final body weight (FBW), specific growth rate (SGR), weight gain (WG) and weight gain percentage (WG %) (n=50 per cage) and body indices such as hepatosomatic index (HSI), viscero-somatic index (VSI) and condition factor (K) (n=6 per cage) were measured at the termination of the trial. For each sampling, fish were collected from the sea-cage by using a large net hung from one side of the cage for fish to voluntarily jump into a smaller and separate section of the cage. Fish from this smaller and shallower section were taken out of the sea-cage using hand nets and transferred to large tubs. Fish were anesthetized with Finquel vet. (Tricaine Mesylate, 30 mg/L) to check the sea-lice and gill structure and were overdosed with anesthesia (60 mg/L, according to the guidelines of the Norwegian Regulation on Animal Experimentation and European Community Directive 86/609/EEC) and killed by cephalic concussion before collecting tissue samples.
Blood was collected from the caudal vein using 38 mm blood collection needles and Ethylenediamine tetraacetic acid (EDTA) coated vacutainers. Samples were kept at 4°C for up to 24 h before measuring the total red blood cell (RBC) and hemoglobin (Hb) counts. The plasma samples were obtained by centrifuging the blood at 3500 rpm for 10 min in 1.5 mL Eppendorf tube. The plasma samples were aliquoted into small centrifuge tubes for analyzing plasma metabolites. All the samples were frozen immediately and stored at -80 °C until further analysis. For collecting the skin mucus, fish were taken out from the water by holding on the tail until the water dripped off. Later the fish were placed on one side on a clean surface without disturbing the dorsal surface. The skin mucus was collected by absorption according to the method described by Tartor et al. (2020) using sterile medical wipes (2.5 × 7 cm each; Kimberly-Clark, Kent, UK) to measure the total DNA in the skin mucus. The mucus was collected from the dorsal side above the lateral line excluding the head, fins and tail to avoid contamination of samples due to handling. For collecting the skin, and gill tissue for gene expression analysis, a small area of skin tissue from right below the dorsal fin was removed and the upper lamellae of the second gill arch was sampled. These tissues for gene expression studies were flash frozen in liquid nitrogen and later stored at -80 °C until further analysis.
Plasma metabolites such as alkaline phosphatase (ALP) (U/L), alanine transaminase (ALT) (U/L), aspartate transaminase (AST) (U/L), glucose (mmol/L), amylase (U/L), total protein (g/L), total cholesterol (mmol/L) and triglyceride (mmol/L) were analyzed using clinical bioanalyzer (Pentra C400 Horiba Medical, Montpellier, France). Plasma cortisol was analyzed using the cortisol ELISA kit (DEH 3388, Demeditec Diagnostics GmbH, Germany). This enzyme immunoassay was based on the principle of competitive binding and the samples were analyzed using a 96 well microplate based on the manufactures protocol. The absorbance was measured at 450 nm using a plate reader (Tecan Sunrise™,Tecan Trading AG, Switzerland). Plasma cortisol is expressed as ng/mL. The plasma osmolality was measured using Fiske® Micro-Osmometer Model 210 (Fiske® Associates, two Tech ways, Norwood, Massachusetts, USA). The osmolality values are expressed in mOsm/kg. Plasma ions such as K+, Na+, Ca++, Cl-, along with glucose and lactate were measured using ABL 90 Flex Plus (Bergman Diagnostika). The concentration of ions is expressed as mmol/L.
The total RBC and Hb was measured using Diluter771 Swelab and Cell-Dyn 400 (Sequoia-Turner, Santa Clara, CA, USA) according to the manufacturer’s instructions, using Para 12 control blood (Streck, MedMark, 218777) for calibration. To measure the RBC, 40 µL of blood was diluted with 10 mL of phosphate buffered saline (PBS, Merck, 4873) in a tube using the diluter and mixed thoroughly. The samples were diluted again with 10 ml of PBS in a Nunc cup and were placed in the Cell-Dyn 400. The RBC values are expressed as the value obtained ×1012 cells/L. For the Hb, 40 µL of blood was diluted with 10 mL of PBS in a tube using the diluter followed by 6 droplets of Zap-o-globin lytic reagent (Beckman Coulter 7546138) to the solution. The resulting solution was thoroughly mixed by inverting the tubes. After 30 min the tubes were centrifuged for 10 min at room temperature. Later the solution was transferred into a Nunc cup and placed under the capillaries on the Cell-Dyn400 to measure Hb. The Hb measured is expressed as g/100mL.
The mucus DNA concentration was measured using Quant-iT™ PicoGreen™ dsDNA Assay Kit (P7589 Thermo Fisher Scientific, UK) according to manufactures protocol, based on fluorescence (480/520 nm excitation/emission, Ex/Em) using Quant-iT™ PicoGreen™ dye. The skin mucus DNA is expressed in ng/mL. The mucus lysozyme activity was measured using a Lysozyme Activity Assay Kit (ab211113) (Abcam, Cambridge, UK) according to manufactures protocol. The kit is based on the ability of lysozyme to cleave a synthetic substrate, 4-Methylumbelliferone and release a free fluorophore which can be easily quantified using a fluorescence microplate reader at fluorescence 360/445 nm (Ex/Em). Skin mucus lysozyme is expressed as pmol/min/mL. The mucus protein concentration was measured using Pierce 660 assay according to manufactures protocol. The total protein concentration in the mucus sample was determined using bovine serum albumin as a standard using a 96 well plate at 660 nm. Total protein is expressed in ug/uL.
Gene expression analysis was done on mucosal tissues such as skin and gill. The candidate genes selected for monitoring the general health and welfare of salmon were for stress response: heat shock protein 70 (hsp70), heat shock protein 90 (hsp90), immune response: inducible nitric oxide synthase (inos), lipoxygenase5 (lox5), interleukin1β (il1β) and interleukin4/13a (il413a), mucus production: mucin5 (muc5), mucin18 (muc18) and wound repair and healing: matrix metallopeptidase9 (mmp9). The procedure for RNA extraction, reverse transcription and quantitative PCR (qPCR) followed were as described in Hundal et al. (2021). In brief, the total RNA was extracted from skin and gill tissue using EZ1 RNA Universal Tissue Kit (Qiagen) and the BioRobot EZ1 according to the manufacturer’s descriptions. Quality and integrity of RNA were assessed with the NanoDrop ND-1000 UV–Vis Spectrophotometer (NanoDrop Technologies) and the Agilent 2100 Bioanalyzer (Agilent Technologies). A two-step real-time PCR protocol was followed to assess the mRNA transcriptional levels of the selected target genes. The qPCR was run on a LightCycler® 480 Real-Time PCR System with the SYBR Green Mastermix (Roche Applied Sciences, Basel, Switzerland) and using the following temperature program: 5 min denaturisation and activation at 95°C, 45 cycles of 10 s denaturisation at 95°C, 10 s annealing at 60°C and 10 s synthesis at 72°C. A melting point analysis was performed before cooling to 4°C. The stability of the reference genes (geometric mean of both βact and elf1α) and mean normalized expression of the target genes were calculated using CFX Maestro software (Bio-Rad CFX maestro version 1.1, Bio-Rad laboratories). The list of primers and reference genes used are as given in Table 2. The efficiency of all PCR runs was higher than 96% (ranging from 96 to 125%).
Statistical analyses were done using Statistica 13.4 (Statsoft Inc.) and GraphPad Prism version 9.0 (Graphpad Software Inc.). Data were tested for homogeneity of variance and normality using a Kolomogorov–Smirnov test and Shapiro–wilk test, respectively for normal distribution, and non-parametric test were used for non-normal distribution. A randomized block design (RBD) with three blocks (B1, B2, and B3) was used to account for cage-position effects. Data from gene expression analysis were log-transformed before statistical analysis. Data from blood, mucus, plasma ions, osmolality, metabolites, and cortisol and gene expression analysis were subjected to a factorial ANOVA, with diet, block and time points (mid and final sampling) as the main factors and cage as random factor, followed by Tukey multiple comparison tests. Data from mortality, gill score and sea-lice were subjected to Kruskal–Wallis non-parametric analysis, using the median test and multiple pair wise comparisons by ranks. Data from delousing were subjected to a two-way ANOVA, with diet and delousing stress as the two main factors. Only in those cases where a significant effect was observed within a factor, one-way ANOVA followed by tukey’s multiple comparisons were performed for each factor separately. For all statistical tests, P values< 0.05 were considered significant. All results are expressed as mean ± standard error. All the graphs were made using GraphPad Prism version 9.0 (Graphpad Software Inc.).
The growth performance indices such as, IBW and FBW were not significantly affected (P> 0.05) by the experimental diets (Table 3). Salmon were fed for 13 months with one of three experimental diets in triplicate seawater cages, the fish had grown to individual weights of 3394±74 g with an SGR value similar in all dietary groups. The WG and WG % were not significantly different among the dietary groups (Table 3). The body indices such as HSI, VSI and K measured at the termination of the trial were not significantly different among the dietary groups (Table 3). The mortality data of salmon over the 13 months had no significant dietary effects (P>0.05) (Figure 1). The mortality was less until December 2021, but started to increase gradually, peaking in August 2022 (Figure 1). The autopsy result (Pharmaq Analytic, Bergen, Norway) verified the occurrence of Pasteurellosis and Branchiomonas Cysticola and reported heart inflammation and gill epitheliocysts.
Table 3 Growth and body indices of Atlantic salmon fed diets containing BSFL meal during 13 months in open sea-cages.
Figure 1 Total mortality (No.; number of dead salmon collected) during the 13 months experiment. The data are presented as the mean of dead fish/cage (± SE, triplicates cages per diet) collected during each month from July 2021-August 2022 (x-axis).
Figures 2A, B show the gill score and sea-lice count throughout the trial (August 2021-August 2022), respectively. No significant dietary effects on sea-lice counts and gill score were observed, except for in December 2021 where the 10% dietary inclusion of BSFL meal had a higher sea-lice compared with the fish fed control diet (P<0.05).
Figure 2 Gill score (A) and sea-lice count (B) of Atlantic salmon fed different diets during the 13 months experiment [from July 2021-August 2022 (x-axis)]. The data are presented as the mean of 20 fish/cage (± SE, triplicates cages per diet). The statistical difference among the dietary groups were detected with two-way ANOVA with diet and block as two factors and cage as random factor, followed by Tukey multiple comparison tests or multiple pair wise comparisons by ranks. *Denote the statistical difference between fish fed Control and BSFL10%.
There was a significant (P<0.05) difference in the mean cortisol value from the two sampling points (mid and final) (Figure 3). The mean cortisol value from the final sampling (190-358 ng/mL) was almost double the mean value from the mid-sampling (113-144 ng/mL). Moreover, a significant dietary effect was observed among the dietary groups from the final sampling, where the cortisol levels were significantly (P<0.05) lower in the 10% BSFL fed group than the control group.
Figure 3 Plasma cortisol (ng/mL) of Atlantic salmon fed different diets at two time points (Mid and final samplings). The data are represented as mean of 6 fish/cage (± SE, triplicates cages per diet). The letters a and b denote the statistical difference among the dietary groups, and letters A and B denote the statistical difference among the two sampling points. Factorial ANOVA was performed with diet, block and time as three factors and cage as random factor, followed by Tukey multiple comparison tests.
The RBC count and Hb levels at the two sampling points are depicted in Figures 4A, B. No significant dietary effects (P>0.05) were noted during the mid-sampling. However, at the final sampling, the BSFL-fed groups exhibited significantly higher RBC and Hb levels (P<0.05) compared to the control.
Figure 4 Red Blood Cell (RBC; measured number * 10^12 cells/L) (A) and hemoglobin (Hb; g/100 mL) (B) of Atlantic salmon fed different diets at two time points (Mid and final samplings). The data are represented as mean of 6 fish/cage (± SE, triplicates cages per diet). The letters a and b denote the statistical difference among the dietary groups, and letters A and B denote the statistical difference among the two sampling points. Factorial ANOVA was performed with diet, block and time as three factors and cage as random factor, followed by Tukey multiple comparison tests.
At the mid-sampling, the levels of ALT, AST and triglycerides were significantly (P<0.05) lower in salmon fed dietary BSFL meal at 5% compared to salmon fed control diet and BSFL 10% diet (Table 4). Cholesterol level was significantly higher in salmon fed BSFL 10% compared to salmon fed control and BSFL 5%. However, the levels of ALP, amylase, total protein, glucose, ions (K+, Na+, Ca++, Cl-) and Lac were not significantly affected (P>0.05) by diets at this time point. There were no significant differences in the plasma metabolites at the final sampling (Table 4).
Table 4 Plasma metabolites, ions and osmolality of Atlantic salmon fed experimental diets during 8 and 13 months (mid and final samplings, respectively) in open sea-cages.
Figures 5A, B show the mucus DNA concentration and lysozyme activity, at two sampling points (mid and final sampling), respectively. The DNA concentration varied between 10 000-15 000 ng/mL and remained consistent during the mid-sampling, without any dietary effects. However, during the final sampling, the group fed with 5% BSFL had a significantly (P<0.05) higher DNA concentration than the control and BSFL 10% groups (Figure 5A). No significant dietary effect was observed on lysozyme activity during the mid-sampling, but at the final sampling the control group exhibited significantly higher lysozyme activity than the groups fed with BSFL (Figure 5B).
Figure 5 Mucus DNA concentration (ng/mL) (A) and mucus lysozyme (U/mg protein) (B) of Atlantic salmon fed different diets at two time points (Mid and final samplings). The data are represented as mean of 10 fish/cage (± SE, triplicates cages per diet). The letters a and b denote the statistical difference among the dietary groups, and letters A and B denote the statistical difference among the two sampling points. Factorial ANOVA was performed with diet, block and time as three factors and cage as random factor, followed by Tukey multiple comparison tests.
Figures 6A-C display the results of mRNA expression analysis for selected genes such as interleukin1β (il1β), mucin18 (muc18) and matrix metallopeptidase 9 (mmp9) in skin and gill tissues from the two different sampling points. In the skin tissue, il1β was significantly upregulated in salmon fed BSFL 5% at mid-sampling whereas in gill tissue muc18 and mmp9 expression were significantly upregulated in the BSFL 5% fed group compared to the two other dietary groups from the mid-sampling. Meanwhile, il1β was significantly upregulated (P<0.05) in gill of salmon fed BSFL 5% in both sampling points. The other genes heat shock Protein (hsp70, hsp90), inducible nitric oxide synthase (inos), lipoxygenase (lox5) and interleukin413a (il413a), mucin (muc5) that were investigated were not significantly affected due to dietary BSFL meal (data not presented). The average expression stability (M value) for the reference genes were 0.29 and 0.33 M value for gill and skin plates respectively.
Figure 6 Skin and Gill mRNA expression of interleukin1β (il1β) (A), mucin18 (muc18) (B), and matrix metallopeptidase9 (mmp9) (C) of Atlantic salmon fed different diets at two time points (Mid and final samplings). The data are represented as mean of 6 fish/cage (± SE, triplicates cages per diet). The letters a and b denote the statistical difference among the dietary groups, and letters A and B denote the statistical difference among the two sampling points. Factorial ANOVA was performed with diet, block and time as three factors and cage as random factor, followed by Tukey multiple comparison tests (NGE, normalized gene expression).
Figure 7 shows the skin mucus DNA concentration (A) and lysozyme activity (B) in skin mucus, as well as the plasma cortisol (C) and plasma osmolality levels (D) before and after delousing. No effects were detected in the mucus samples for either diet or cortisol-induced stress (P>0.05). However, in the plasma samples, a significant (P<0.05) stress effect was observed in the cortisol level. Additionally, a significant interaction effect was observed between diet and stress in the plasma osmolality, with the BSFL 5% group exhibiting significantly higher levels (P<0.05) than the control group (Figure 7D).
Figure 7 Mucus DNA concentration (A), mucus lysozyme (B), plasma cortisol (C) and plasma osmolality (D) before and after delousing in Atlantic salmon fed different diets. Salmon were sampled before delousing stress (pre-stress) and after one hour (post stress). The data are represented as mean of 6 fish/cage (± SE, triplicates cages per diet). The letters a and b denote the statistical difference among the dietary groups, and letters A and B denote the statistical difference before and after delousing. Two-way ANOVA was performed with diet and block as two factors and cage as random factor, followed by Tukey multiple comparison tests.
The Atlantic salmon fed either 5% or 10% dietary BSFL meal showed a similar growth performance to the fish fed a diet with standard commercial protein sources. Earlier studies performed in laboratory scale in tanks on land, have also shown no effects on growth when using up to 12% full fat BSFL meal in pre smolts (Weththasinghe et al., 2021a) and up to 15% defatted BSFL meal in post smolt salmon (Belghit et al., 2019a) replacing the protein sources in the salmon feed (FM and plant-based protein). The current study thus confirms what has been seen earlier, but now verifying the lack of a negative impact on growth of Atlantic salmon fed BSFL meal in a situation much closer to a commercial aquaculture production.
The general welfare of salmon was monitored weekly (approximately 53 weeks) in terms of gill score and sea-lice count. These parameters were not affected by the experimental diet, except for December 2021, which is likely to be due to a random effect of sampling. Apart from that, the cumulative mortality rate was stable from the start of this trial (July-August 2021) until the mid-sampling (April 2022; 42 weeks post release into sea-cage) and was similar to the mortality pattern observed in Norwegian and Scottish salmon farms studied over the last 5 years (Soares et al., 2011; Bang Jensen et al., 2020), with an overall mortality rate of ~15% in Norwegian farms. However, after this time of the year till the end of the current trial, the mortality rate increased, which peaked in August 2022 (52 weeks post release into sea-cage), making the overall mortality rate of this trial ~34%. In fact, this rate was similar to the mortality rate (~23%) reported for salmon in a similar production zone in 2021 (Norwegian fish health report, 2022). This high mortality in the current study can be linked to various factors including multiple delousing, and diseases faced by salmon during that period. The salmon were exposed to thermal delousing to maintain the adult sea-lice population within the legal limits. Thermal delousing, a commonly used method in salmon farms to control and eliminate sea-lice, can contribute to increased mortality due to its exposure to higher water temperatures (up to 32 °C for ~ 30 sec), and repeated handling that create a highly stressful environment for the salmon (Moltumyr et al., 2021). During one of the delousing procedures, samples were taken before and after the process, indicating a notable increase in stress levels with higher plasma cortisol levels observed after one hour. However, the cortisol response was independent of the dietary BSFL meal in the salmon diet. Previous studies have also attempted different dietary modulation to reduce the delousing stress. For instance, Selvam et al. (2022) modulated the levels of dietary eicosapentaenoic acid and docosahexaenoic acid in Atlantic salmon but did not observe any significant dietary effect when exposed to mechanical delousing stress. Nonetheless, previous studies reported reducing the sea-lice infestations and mortalities in salmon through functional feed ingredients (Jensen et al., 2015) and dietary nucleotides (Burrells et al., 2001a). Apart from the delousing and handling stress, increased mortality might be also due to bacterial infection with Pasteurellosis, and Branchiomonas Cysticola, observed in salmon during the regular farm monitoring (diagnosed in May-June 2022, analyzed by Pharmaq Analytic, Bergen, Norway). These bacteria are known to affect the heart and gills in salmon, if occurred in heavy load, leading to high mortality (Gjessing et al., 2021; Sandlund et al., 2021). However, in the current study, very high gill infection was not observed in the three dietary groups. Hence, a direct correlation between gill score and bacterial occurrences was not observed. Nevertheless, it is plausible that diseases alone might not induce mortalities, but the combined stress from handling, delousing, and pathogenic pressure could contribute to increased mortality among salmon (Segner et al., 2012). There were, however, no effects of diets on growth or survival, demonstrating that diets containing up to 10% BSFL meal can give a long-term performance equal to fish fed a commercial diet in open sea-cages.
Studying hematological indices and mucosal health can be an easy and an important diagnostic tool for observing stress, physical anomalies, diseases symptoms and monitoring health (Assefa and Abunna, 2018; Seibel et al., 2021). The most used indicator for assessing stress levels in fish is plasma cortisol, which was measured at the mid and final sampling of this study to assess potential differences in basal stress level due to diet. At the final sampling, the plasma cortisol levels were higher in the salmon fed the control diet than the fish fed diets containing BSFL meal. The observed higher cortisol in the final sampling is hypothesized to be due to the exposure of salmon to multiple stressors mentioned in this study towards the end of the trial. The series of stressful events eventually resulted in elevated stress in salmon resulting in higher cortisol response in plasma. Furthermore, parallel to the above findings, the control group had a lower RBC and Hb count than the fish fed BSFL 5% at the same time point. This suggests that the elevated stress condition may have reduced the RBC’s oxygen-carrying capacity in control group compared to salmon fed BSFL meal, meaning that this group of salmon faced comparatively less stressful conditions in this experimental trial. A similar pattern of increased erythrocytes and decreased cortisol levels were observed in a study conducted in rainbow trout when fed with roselle (Hibiscus sabdariffa) meal, underlining the reason being the antioxidant capacity of the roselle meal (Hoseini et al., 2021). It has been shown that different insect species, including BSFL contain bioactive compounds with antioxidant properties (Priyadarshana et al., 2022; Veldkamp et al., 2022a) and is also known to modulate the oxidative stress in cellular and animal models (D’Antonio et al., 2021). Stress can affect the general health and welfare of fish. The results obtained in this study showed that salmon fed BSFL diets were able to better cope with the stressful events, probably due to the presence of bioactive compounds (chitin, AMPs) and antioxidant capacity, which might have reduced the oxidative stress in Atlantic salmon. The antioxidant capacity could also be confirmed by analyzing other potential stress biomarkers such as catalase, superoxide dismutase or respiratory burst activity. However, these analyses were not performed in the current study.
Lysozyme is one of the innate immune parameters studied in skin mucus (Pelusio et al., 2022). Differences in the mucus lysozyme activities mostly relate to the mucus composition (e.g., mucins, nucleotides, proteases, AMPs) (Fast et al., 2002) and an increase in lysozyme activity generally is triggered due to high microbial load (Ghafoori et al., 2014). Different studies have shown that plasma lysozyme activity increased in fish species fed diets containing insect meal, particularly after a challenge with pathogens (Su et al., 2017; Ido et al., 2019), triggering the innate immune responses. The evidence so far indicates a dose-dependent relationship between insect meal and plasma lysozyme activity in Atlantic salmon (6.25% vs 12.5% inclusion), as presented by Weththasinghe et al. (2021b). However, in the current study, the level of mucus lysozyme at the end of the feeding trial was lower in salmon fed BSF-based diets compared to the fish fed control diet. Apart from bacterial infection, it has been shown that higher lysozyme activity can also be due to high stress conditions (Dash et al., 2018). As discussed earlier, the control group exhibited high levels of cortisol, an indicator of stress condition, which might have increased the level of mucus lysozyme. A similar proportionality between plasma cortisol and skin mucus lysozymes was observed in Atlantic salmon when exposed to crowding stress (Demers and Bayne, 1997; Djordjevic et al., 2021). Fevolden et al. (1994), discussed that the elevated levels of plasma cortisol and rise in lysozyme could indicate higher susceptibility of organism to pathogens and this proportional relationship might be a compensatory adaptation to enhance innate immunity. Thus, lower levels of lysozyme in BSFL fed diets might indicate that salmon fed BSFL diet were comparatively less stressed due to immunostimulant property of BSFL.
The viscoelasticity of mucus is determined by the presence of various biopolymers such as DNA and mucins, responsible for trapping the pathogens and initiating the inflammatory responses (Kaitlyn et al., 2022). In humans, neutrophils are observed to actively release DNA, and this extracellular DNA plays a significant role in capturing harmful microorganisms (Brinkmann et al., 2004). This implies that DNA might have an immune function in mucus. In fish, the skin mucus contains DNA originating from host cells, as well as from beneficial or harmful bacteria (Brinchmann, 2016). Thus, higher secretions of DNA from neutrophils or other cell types into the skin’s surface can potentially increase the thickness of the mucus layer and thereby protecting the skin by increasing its viscoelasticity (Cameron and Endean, 1973; Al-Hassan et al., 1985; Caballero et al., 2020). In the present study, the DNA concentration in the skin mucus was comparatively lower in the control diet than BSFL fed at 5% (final sampling) but lacked any significant differences at the mid-sampling. Earlier work has shown that gill mucus viscosity was significantly lower in Atlantic salmon and brown trout (Salmo trutta L.) affected with amoebic gill disease compared to healthy fish (Roberts and Powell, 2005). Hender et al. (2021) reported an increase in the number of acidic mucins in Asian seabass (Lates calcarifer), that enhanced the gut and skin mucosal immunity when 30% FM and fish oil were replaced with partially defatted BSFL meal. Previous research findings suggest that supplementing of nucleotides and yeast cell extracts in the diet of Atlantic salmon enhances improved mucosal health and gut microbiota (Burrells et al., 2001b; Wang et al., 2022). Our results are therefore in line with earlier reports. Research has indicated that the low molecular weight antimicrobial peptides found in BSFL meal, specifically defensins, exhibit antibacterial properties. Defensins serve as active agents of the innate immune system, offering a first line of defense against infectious pathogens (Veldkamp et al., 2022a). Furthermore, proline-rich AMPs can interfere with DNA and RNA synthesis by binding to nucleic acids (Kragol et al., 2002) and exhibit antimicrobial properties against various bacteria (Mattiuzzo et al., 2007). Hence, there is a possibility that the presence of these antimicrobial peptides in the diet of salmon might have increased the mucus secretions in the form of DNA and mucins from neutrophils or by modulating the cellular process and producing more viscous mucus. However, further investigation is needed to comprehensively understand the link between diet and mucus defense systems, as well as more knowledge on how DNA concentrations can be used as a measure of mucus viscosity.
In the current study, higher mRNA expression of muc18 and mmp9 in gill (mid-sampling) were observed in salmon fed dietary BSFL at 5% compared to salmon fed control diet. These genes are mainly associated with mucus production and inflammatory processes such as remodeling and wound healing (Swain et al., 2006). An increased transcription of l1β in skin and gill (mid and final sampling) were also observed in salmon fed dietary BSFL meal. This observed transcription of il1β might be due to the bacterium Branchiomonas cisticola which is known to affect inflammatory genes (such as mmp25, mmp13, il1β, il8) (Gjessing et al., 2021). Thus, higher expression of il1β in salmon fed BSFL meal may indicate that they were able to comparatively resist better against pathogens. Furthermore, previous studies reported an upregulation of stress and immune related genes in gut of salmon fed with BSFL meal in vivo (Li et al., 2019; Li et al., 2020) and in vitro using isolated head kidney leucocytes from salmon challenged with bacterial and viral pathogens (Stenberg, 2018). Similar results were obtained in different tissues in Asian seabass, and European seabass when partially defatted BSFL meal and full fat BSFL meal was substituting 22% and 15% of the FM (Abdel-Latif et al., 2021; Hender et al., 2021). Furthermore, studies have also reported that supplementation of prebiotics such as Pediococcus acidilactici to the salmon diet could potentially stimulate the immune system and trigger the release of pro-inflammatory cytokines activation to fight possible pathogen attack (Jaramillo-Torres et al., 2019). In the current study, these observed effects might be linked to the immunostimulant properties of chitin and chitosan (Ghotloo et al., 2015). The chitin or chitosan from the BSFL meal is known to have the potential to stimulate the innate immune response (Veldkamp et al., 2022a). In the current study, however, the content of these components has not been measured. Therefore, the higher expression of these genes involved in the inflammatory process might be due to the content of chitin/chitosan as an immunomodulant in the BSFL meal or due to other compounds in the BSFL meal.
Inflammation triggers the innate immune system, which activates responses causing tissue damage and the release of AST and ALT into the body fluids (Samim and Vaseem, 2021). Compared to the other experimental diets, the diet with BSFL meal fed at 5% showed significantly lower levels of plasma ALT and AST at the mid-sampling. This suggests that incorporating BSFL meal into the diet may have a positive effect on the liver health of salmon. In the current study a reduction in plasma cholesterol and triglycerides was observed in the group fed BSFL which were similar to the studies reported in Japanese seabass (Lateolabrax japonicus) and African catfish (Clarias gariepinus) when FM was replaced by dietary BSFL meal (Wang et al., 2019; Fawole et al., 2020). This effect is likely due to the presence of chitin in BSFL, which has been documented to lower triglyceride and cholesterol levels (Wang et al., 2019). However, it is also possible that dietary cholesterol level or feeding status of salmon may have contributed to the observed effect, as the fish were sampled at a fed state.
Dietary inclusion of BSFL meal up to 10% in Atlantic salmon did not have any significant effects in general growth and survival. The salmon fed BSFL 5% showed an increase in the number of erythrocytes, and a reduced cortisol response when exposed to various stressors during the end of the trial and could indicate the antioxidant capacity of salmon fed BSFL diet. Moreover, this group of salmon exhibited a higher skin mucus DNA concentration which suggested higher mucus secretions enabling better protection against pathogens and stress conditions. Also, the inflammatory gene such as il1β, was upregulated in skin and gill tissue (mid and final samplings), while the plasma ALT and AST levels were lower in salmon fed dietary BSFL meal at 5% (mid sampling). It is worth mentioning that the health indicators observed in salmon behave differently across different fish sizes, time points and environmental conditions. In conclusion, the results of this study showed that dietary BSFL meal can modulate the immune responses in Atlantic salmon reared at large scale under farm-like conditions.
The original contributions presented in the study are included in the article/supplementary material, further inquiries can be directed to the corresponding author.
This trial is exempt from application to the Norwegian Food Safety Authority, according to the regulation “FOR-2015-06-18-761 Regulation concerning the use of animals for scientific purposes, § 6. Godkjenning av forsøk". The approval requirement does not apply to experiments involving only the killing of animals to use organs or tissues from them. The study was conducted in accordance with the local legislation and institutional requirements.
GR: Conceptualization, Methodology, Software, Validation, Formal analysis, Investigation, Resources Data Curation, Writing - Original Draft Writing - Review & Editing, Visualization. AP: Conceptualization, Methodology, Software, Validation, Formal analysis, Investigation, Resources Data Curation, Review & Editing, Visualization, Supervision. MK: Conceptualization, Methodology, Investigation, Review & Editing, Project administration. NL: Conceptualization, Methodology, Software, Validation, Formal analysis, Investigation, Review & Editing, Visualization. E-JL: Conceptualization, Methodology, Validation, Investigation, Review & Editing. IB: Conceptualization, Methodology, Software, Validation, Formal analysis, Investigation, Resources Data Curation, Writing - Original Draft Writing - Review & Editing, Visualization, Supervision, Project administration. All authors contributed to the article and approved the submitted version.
This study was supported by the Institute of Marine Research and the European Union’s Horizon 2020 (SUSINCHAIN, 861976). The authors thank the fellowship provided by the ICAR Netaji Subash International Fellowship.
The authors are thankful to Kjetil Stensland, Bjørn Magne Hufthammer, Ida Berger Waage and Gokstad Svanhild Lohne at Austevoll station and all the people worked under the project for the different samplings (Marta S Silva, David Dominguez, Charles Reffay, Paul Gottar, Chandrasekar Selvam, Angela Etayo, Sahar Yarahmadi, and Nawaraj Gautam). The authors thank the fellowship provided by the Indian Council of Agricultural Research (ICAR) through the ICAR Netaji Subash International Fellowship.
The authors declare that the research was conducted in the absence of any commercial or financial relationships that could be construed as a potential conflict of interest.
Author IB declared that they were an editorial board member of Frontiers, at the time of submission. This had no impact on the peer review process and the final decision.
All claims expressed in this article are solely those of the authors and do not necessarily represent those of their affiliated organizations, or those of the publisher, the editors and the reviewers. Any product that may be evaluated in this article, or claim that may be made by its manufacturer, is not guaranteed or endorsed by the publisher.
Abdel-Latif H. M. R., Abdel-Tawwab M., Khalil R. H., Metwally A. A., Shakweer M. S., Ghetas H. A., et al. (2021). Black soldier fly (Hermetia illucens) larvae meal in diets of European seabass: Effects on antioxidative capacity, non-specific immunity, transcriptomic responses, and resistance to the challenge with Vibrio alginolyticus. Fish Shellfish Immunol. 111, 111–118. doi: 10.1016/j.fsi.2021.01.013
Al-Hassan J. M., Thomson M., Criddle K. R., Summers B., Criddle R. S. (1985). Catfish epidermal secretions in response to threat or injury. Mar. Biol. 88, 117–123. doi: 10.1007/BF00397158
AOAC I. (2010). AOAC official methods of analysis. Association of Official Analytical Chemists Arlington, Virginia.
Assefa A., Abunna F. (2018). Maintenance of fish health in aquaculture: review of epidemiological approaches for prevention and control of infectious disease of fish. Vet. Med. Int. 2018, 10. doi: 10.1155/2018/5432497
Bang Jensen B., Qviller L., Toft N. (2020). Spatio-temporal variations in mortality during the seawater production phase of Atlantic salmon (Salmo salar) in Norway. J. Fish Dis. 43, 445–457. doi: 10.1111/jfd.13142
Belghit I., Liland N. S., Gjesdal P., Biancarosa I., Menchetti E., Li Y., et al. (2019a). Black soldier fly larvae meal can replace fish meal in diets of sea-water phase Atlantic salmon (Salmo salar). Aquaculture 503, 609–619. doi: 10.1016/j.aquaculture.2018.12.032
Belghit I., Waagbø R., Lock E.-J., Liland N. S. (2019b). Insect-based diets high in lauric acid reduce liver lipids in freshwater Atlantic salmon. Aquac. Nutr. 25, 343–357. doi: 10.1111/anu.12860
Brinchmann M. F. (2016). Immune relevant molecules identified in the skin mucus of fish using -omics technologies. Mol. Biosyst. 12, 2056–2063. doi: 10.1039/C5MB00890E
Brinkmann V., Reichard U., Goosmann C., Fauler B., Uhlemann Y., Weiss D. S., et al. (2004). Neutrophil extracellular traps kill bacteria. Science 303, 1532–1535. doi: 10.1126/science.1092385
Burrells C., Williams P. D., Forno P. F. (2001a). Dietary nucleotides: a novel supplement in fish feeds: 1. Effects on resistance to disease in salmonids. Aquaculture 199, 159–169. doi: 10.1016/S0044-8486(01)00577-4
Burrells C., Williams P. D., Southgate P. J., Wadsworth S. L. (2001b). Dietary nucleotides: a novel supplement in fish feeds: 2. Effects on vaccination, salt water transfer, growth rates and physiology of Atlantic salmon (Salmo salar L.). Aquaculture 199, 171–184. doi: 10.1016/S0044-8486(01)00576-2
Caballero S., Galeano A. M., Lozano J. D., Vives M. (2020). Description of the microbiota in epidermal mucus and skin of sharks (Ginglymostoma cirratum and Negaprion brevirostris) and one stingray (Hypanus americanus). PeerJ 8, e10240. doi: 10.7717/peerj.10240
Cameron A. M., Endean R. (1973). Epidermal secretions and the evolution of venom glands in fishes. Toxicon 11, 401–410. doi: 10.1016/0041-0101(73)90115-3
D’Antonio V., Serafini M., Battista N. (2021). Dietary modulation of oxidative stress from edible insects: A mini-review. Front. Nutr. 8. doi: 10.3389/fnut.2021.642551
Dash S., Das S. K., Samal J., Thatoi H. N. (2018). Epidermal mucus, a major determinant in fish health: a review. Iranian J. Vet. Res. 19, 72–81. doi: 10.1016/S1095-6433(02)00109-5.
Demers N. E., Bayne C. J. (1997). The immediate effects of stress on hormones and plasma lysozyme in rainbow trout. Dev. Comp. Immunol. 21, 363–373. doi: 10.1016/S0145-305X(97)00009-8
Djordjevic B., Morales-Lange B., Mclean Press C., Olson J., Lagos L., Mercado L., et al. (2021). Comparison of circulating markers and mucosal immune parameters from skin and distal intestine of Atlantic salmon in two models of acute stress. Int. J. Mol. Sci. 22(3), 1028. doi: 10.3390/ijms22031028
FAO. (2022). The State of World Fisheries and Aquaculture 2022. Towards Blue Transformation. FAO. doi: 10.4060/cc0461en
Fast M. D., Sims D. E., Burka J. F., Mustafa A., Ross N. W. (2002). Skin morphology and humoral non-specific defence parameters of mucus and plasma in rainbow trout, coho and Atlantic salmon. Comp. Biochem. Physiol. Part A: Mol. Integr. Physiol. 132, 645–657. doi: 10.1016/S1095-6433(02)00109-5
Fawole F. J., Adeoye A. A., Tiamiyu L. O., Ajala K. I., Obadara S. O., Ganiyu I. O. (2020). Substituting fishmeal with Hermetia illucens in the diets of African catfish (Clarias gariepinus): Effects on growth, nutrient utilization, haemato-physiological response, and oxidative stress biomarker. Aquaculture 518, 734849. doi: 10.1016/j.aquaculture.2019.734849
Fevolden S. E., Reed K. H., Gjerde B. (1994). Genetic components of post-stress cortisol and lysozyme activity in Atlantic salmon; correlations to disease and resistance. Fish Shellfish Immunol. 4(7), 507–519. doi: 10.1006/fsim.1994.1045
Garlock T., Asche F., Anderson J., Ceballos-Concha A., Love D. C., Osmundsen T. C., et al. (2022). Aquaculture: The missing contributor in the food security agenda. Global Food Secur. 32, 100620. doi: 10.1016/j.gfs.2022.100620
Ghafoori Z., Heidari B., Farzadfar F., Aghamaali M. (2014). Variations of serum and mucus lysozyme activity and total protein content in the male and female Caspian kutum (Rutilus frisii kutum, Kamensky 1901) during reproductive period. Fish Shellfish Immunol. 37, 139–146. doi: 10.1016/j.fsi.2014.01.016
Ghotloo S., Hoseini M. H. M., Alimohammadian M. H., Khaze V., Memarnejadian A., Rostami A. (2015). Immunomodulatory effects of chitin microparticles on Leishmania major-infected BALB/c mice. Parasitol. Int. 64, 219–221. doi: 10.1016/j.parint.2014.12.007
Gjessing M. C., Spilsberg B., Steinum T. M., Amundsen M., Austbø L., Hansen H., et al. (2021). Multi-agent in situ hybridization confirms Ca. Branchiomonas cysticola as a major contributor in complex gill disease in Atlantic salmon. Fish Shellfish Immunol. Rep. 2, 100026. doi: 10.1016/j.fsirep.2021.100026
Hender A., Siddik M. A. B., Howieson J., Fotedar R. (2021). Black Soldier Fly, Hermetia illucens as an Alternative to Fishmeal Protein and Fish Oil: Impact on Growth, Immune Response, Mucosal Barrier Status, and Flesh Quality of Juvenile Barramundi, Lates calcarifer (Bloch 1790). Biology 10(6), 505. doi: 10.3390/biology10060505
Hoseini S. M., Hoseinifar S. H., Van Doan H. (2021). Growth performance and hematological and antioxidant characteristics of rainbow trout, Oncorhynchus mykiss, fed diets supplemented with Roselle, Hibiscus sabdariffa. Aquaculture 530, 735827. doi: 10.1016/j.aquaculture.2020.735827
Hossain M. S., Small B. C., Kumar V., Hardy R. (2023). Utilization of functional feed additives to produce cost-effective, ecofriendly aquafeeds high in plant-based ingredients. Rev. Aquac., 1–33. doi: 10.1111/raq.12824
Hu Q.-Y., Wu P., Feng L., Jiang W.-D., Liu Y., Kuang S.-Y., et al. (2021). Antimicrobial peptide Isalo scorpion cytotoxic peptide (IsCT) enhanced growth performance and improved intestinal immune function associated with janus kinases (JAKs)/signal transducers and activators of transcription (STATs) signalling pathways in on-growing grass carp (Ctenopharyngodon idella). Aquaculture 539, 736585. doi: 10.1016/j.aquaculture.2021.736585
Hundal B. K., Liland N. S., Rosenlund G., Höglund E., Araujo P., Stubhaug I., et al. (2021). Increasing the dietary n-6/n-3 ratio alters the hepatic eicosanoid production after acute stress in Atlantic salmon (Salmo salar). Aquaculture 534, 736272. doi: 10.1016/j.aquaculture.2020.736272
Ido A., Iwai T., Ito K., Ohta T., Mizushige T., Kishida T., et al. (2015). Dietary effects of housefly (Musca domestica) (Diptera: Muscidae) pupae on the growth performance and the resistance against bacterial pathogen in red sea bream (Pagrus major) (Perciformes: Sparidae). Applied Entomology and Zoology 50, 213–221. doi: 10.1007/s13355-015-0325-z
Ido A., Hashizume A., Ohta T., Takahashi T., Miura C., Miura T. (2019). Replacement of Fish Meal by Defatted Yellow Mealworm (Tenebrio molitor) Larvae in Diet Improves Growth Performance and Disease Resistance in Red Seabream (Pargus major). Animals 9 (3), 100. doi: 10.3390/ani9030100
Imsland A. K. D., Hanssen A., Nytrø A. V., Reynolds P., Jonassen T. M., Hangstad T. A., et al. (2018). It works! Lumpfish can significantly lower sea lice infestation in large-scale salmon farming. Biol. Open 7 (9), bio036301. doi: 10.1242/bio.036301
Imsland A. K., Reynolds P., Eliassen G., Hangstad T. A., Foss A., Vikingstad E., et al. (2014). The use of lumpfish (Cyclopterus lumpus L.) to control sea lice (Lepeophtheirus salmonis Krøyer) infestations in intensively farmed Atlantic salmon (Salmo salar L.). Aquaculture 424, 18–23. doi: 10.1016/j.aquaculture.2013.12.033
Jaramillo-Torres A., Rawling M. D., Rodiles A., Mikalsen H. E., Johansen L.-H., Tinsley J., et al. (2019). Influence of Dietary Supplementation of Probiotic Pediococcus acidilactici MA18/5M During the Transition From Freshwater to Seawater on Intestinal Health and Microbiota of Atlantic Salmon (Salmo salar L.). Front. Microbiol. 10. doi: 10.3389/fmicb.2019.02243
Jensen L. B., Provan F., Larssen E., Bron J. E., Obach A. (2015). Reducing sea-lice (Lepeophtheirus salmonis) infestation of farmed Atlantic salmon (Salmo salar L.) through functional feeds. Aquac. Nutr. 21, 983–993. doi: 10.1111/anu.12222
Kaitlyn R. R., William J. K., Matthew R. M., David B. H. (2022). Effects of mucin and DNA concentrations in airway mucus on Pseudomonas aeruginosa biofilm recalcitrance. bioRxiv. 7 (4), e00291-22. doi: 10.1128/msphere
Kragol G., Hoffmann R., Chattergoon M. A., Lovas S., Cudic M., Bulet P., et al. (2002). Identification of crucial residues for the antibacterial activity of the proline-rich peptide, pyrrhocoricin. Eur. J. Biochem. 269, 4226–4237. doi: 10.1046/j.1432-1033.2002.03119.x
Li Y., Kortner T. M., Chikwati E. M., Belghit I., Lock E.-J., Krogdahl Å. (2020). Total replacement of fish meal with black soldier fly (Hermetia illucens) larvae meal does not compromise the gut health of Atlantic salmon (Salmo salar). Aquaculture 520, 734967. doi: 10.1016/j.aquaculture.2020.734967
Li Y., Kortner T. M., Chikwati E. M., Munang'andu H. M., Lock E.-J., Krogdahl Å. (2019). Gut health and vaccination response in pre-smolt Atlantic salmon (Salmo salar) fed black soldier fly (Hermetia illucens) larvae meal. Fish Shellfish Immunol. 86, 1106–1113. doi: 10.1016/j.fsi.2018.12.057
Lieberman S., Enig M. G., Preuss H. G. (2006). A review of monolaurin and lauric acid: natural virucidal and bactericidal agents. Altern. Complement. Therapies 12, 310–314. doi: 10.1089/act.2006.12.310
Liland N. S., Araujo P., Xu X. X., Lock E. J., Radhakrishnan G., Prabhu A. J. P., et al. (2021). A meta-analysis on the nutritional value of insects in aquafeeds. J. Insects as Food Feed 7, 743–759. doi: 10.3920/JIFF2020.0147
Mattiuzzo M., Bandiera A., Gennaro R., Benincasa M., Pacor S., Antcheva N., et al. (2007). Role of the Escherichia coli SbmA in the antimicrobial activity of proline-rich peptides. Mol. Microbiol. 66, 151–163. doi: 10.1111/j.1365-2958.2007.05903.x
Ming J., Ye J., Zhang Y., Yang X., Wu C., Shao X., et al. (2013). The influence of maggot meal and l-carnitine on growth, immunity, antioxidant indices and disease resistance of black carp (Mylopharyngodon piceus). J. Chin. Cereals Oils Assoc. 28, 80–86.
Moltumyr L., Gismervik K., Gu J., Gåsnes S. K., Kristiansen T. S., Rønnestad I., et al. (2021). Does the thermal component of warm water treatment inflict acute lesions on Atlantic salmon (Salmo salar)? Aquaculture 532, 736048. doi: 10.1016/j.aquaculture.2020.736048
Mouithys-Mickalad A., Schmitt E., Dalim M., Franck T., Tome N. M., Van Spankeren M., et al. (2020). Black soldier fly (Hermetia illucens) larvae protein derivatives: potential to promote animal health. Animals 10 (6), 941. doi: 10.3390/ani10060941
Pelusio N. F., Bonaldo A., Gisbert E., Andree K. B., Esteban M. A., Dondi F., et al. (2022). Different fish meal and fish oil dietary levels in European sea bass: welfare implications after acute confinement stress. Front. Mar. Sci. 8. doi: 10.3389/fmars.2021.779053
Priyadarshana M. K. C., Walpita C. N., Ruwandeepika H. A. D., Magamage M. P. S. (2022). Effects of black soldier fly, Hermetia illucens (Linnaeus 1758), larvae incorporated feed on histomorphology, gut microbiota and blood chemistry of cultured. Asian Fisheries Sci. 35, 269–281. doi: 10.33997/j.afs.2022.35.3.005
Rashidian G., Moosazadeh Moghaddam M., Mirnejad R., Mohammadi Azad Z. (2021). Supplementation of zebrafish (Danio rerio) diet using a short antimicrobial peptide: Evaluation of growth performance, immunomodulatory function, antioxidant activity, and disease resistance. Fish Shellfish Immunol. 119, 42–50. doi: 10.1016/j.fsi.2021.09.035
Ringø E., Olsen R. E., Vecino J. G., Wadsworth S., Song S. K. (2012). Use of immunostimulants and nucleotides in aquaculture: a review. J. Mar. Sci.: Res. Dev. 2, 104.
Roberts S. D., Powell M. D. (2005). The viscosity and glycoprotein biochemistry of salmonid mucus varies with species, salinity and the presence of amoebic gill disease. J. Comp. Physiol. B 175, 1–11. doi: 10.1007/s00360-005-0473-5
Samim A. R., Vaseem H. (2021). Assessment of the potential threat of nickel(II) oxide nanoparticles to fish Heteropneustes fossilis associated with the changes in haematological, biochemical and enzymological parameters. Environ. Sci. pollut. Res. 28, 54630–54646. doi: 10.1007/s11356-021-14451-y
Sandlund N., Rønneseth A., Ellul R. M., Nylund S., Sandlund L. (2021). Pasteurella spp. Infections in Atlantic salmon and lumpsucker. J. Fish Dis. 44, 1201–1214. doi: 10.1111/jfd.13381
Segner H., Sundh H., Buchmann K., Douxfils J., Sundell K. S., Mathieu C., et al. (2012). Health of farmed fish: its relation to fish welfare and its utility as welfare indicator. Fish Physiol. Biochem. 38, 85–105. doi: 10.1007/s10695-011-9517-9
Seibel H., Baßmann B., Rebl A. (2021). Blood will tell: what hematological analyses can reveal about fish welfare. Front. Vet. Sci. 8. doi: 10.3389/fvets.2021.616955
Selvam C., Philip A. J. P., Lutfi E., Sigholt T., Norberg B., Bæverfjord G., et al. (2022). Long-term feeding of Atlantic salmon with varying levels of dietary EPA + DHA alters the mineral status but does not affect the stress responses after mechanical delousing stress. Br. J. Nutr. 128, 2291–2307. doi: 10.1017/S0007114522000514
Soares S., Green D. M., Turnbull J. F., Crumlish M., Murray A. G. (2011). A baseline method for benchmarking mortality losses in Atlantic salmon (Salmo salar) production. Aquaculture 314, 7–12. doi: 10.1016/j.aquaculture.2011.01.029
Song S. K., Beck B. R., Kim D., Park J., Kim J., Kim H. D., et al. (2014). Prebiotics as immunostimulants in aquaculture: a review. Fish Shellfish Immunol. 40, 40–48. doi: 10.1016/j.fsi.2014.06.016
Stenberg O. K. (2018). In vitro bacterial and viral response in head kidney leukocytes of Atlantic salmon (Salmo salar) fed dietary insects meal (The University of Bergen). Available at: https://hdl.handle.net/1956/17767.
Su J., Gong Y., Cao S., Lu F., Han D., Liu H., et al. (2017). Effects of dietary Tenebrio molitor meal on the growth performance, immune response and disease resistance of yellow catfish (Pelteobagrus fulvidraco). Fish Shellfish Immunol. 69, 59–66. doi: 10.1016/j.fsi.2017.08.008
Swain P., Sahoo P. K., Ayyappan S. (2006). Fish and shellfish immunology: An introduction (India: Narendra Publishing House).
Tartor H., Luis Monjane A., Grove S. (2020). Quantification of defensive proteins in skin mucus of Atlantic salmon using minimally invasive sampling and high-sensitivity ELISA. Anim. (Basel) 10 (8), 1374. doi: 10.3390/ani10081374
Taylor R. S., Muller W. J., Cook M. T., Kube P. D., Elliott N. G. (2009). Gill observations in Atlantic salmon (Salmo salar, L.) during repeated amoebic gill disease (AGD) field exposure and survival challenge. Aquaculture 290, 1–8. doi: 10.1016/j.aquaculture.2009.01.030
Tran H. Q., Nguyen T. T., Prokešová M., Gebauer T., Doan H. V., Stejskal V. (2022). Systematic review and meta-analysis of production performance of aquaculture species fed dietary insect meals. Rev. Aquac. 14, 1637–1655. doi: 10.1111/raq.12666
Ushakova N. A., Ponomarev S. V., Bakaneva Y. M., Fedorovykh Y. V., Levina O. A., Kotel’nikov A. V., et al. (2018). Biological Efficiency of the Prepupae Hermetia illucens in the Diet of the Young Mozambique Tilapia Oreochromis mossambicus. Biol. Bull. 45, 382–387. doi: 10.1134/S1062359018040143
Van Huis A., Gasco L. (2023). Insects as feed for livestock production. Science 379, 138–139. doi: 10.1126/science.adc9165
Veldkamp T., Dong L., Paul A., Govers C. (2022a). Bioactive properties of insect products for monogastric animals – a review. J. Insects as Food Feed 8, 1027–1040. doi: 10.3920/JIFF2021.0031
Veldkamp T., Meijer N., Alleweldt F., Deruytter D., Van Campenhout L., Gasco L., et al. (2022b). Overcoming technical and market barriers to enable sustainable large-scale production and consumption of insect proteins in Europe: A SUSINCHAIN perspective. Insects 13 (3), 281. doi: 10.3390/insects13030281
Wang J., Jaramillo-Torres A., Li Y., Brevik Ø.J., Jakobsen J. V., Kortner T. M., et al. (2022). Gut health and microbiota in out-of-season Atlantic salmon (Salmo salar L.) smolts before and after seawater transfer under commercial arctic conditions: modulation by functional feed ingredients. Front. Mar. Sci. 9, 860081. doi: 10.3389/fmars.2022.860081
Wang G., Peng K., Hu J., Yi C., Chen X., Wu H., et al. (2019). Evaluation of defatted black soldier fly (Hermetia illucens L.) larvae meal as an alternative protein ingredient for juvenile Japanese seabass (Lateolabrax japonicus) diets. Aquaculture 507, 144–154. doi: 10.1016/j.aquaculture.2019.04.023
Weththasinghe P., Hansen J. Ø., Nøkland D., Lagos L., Rawski M., Øverland M. (2021a). Full-fat black soldier fly larvae (Hermetia illucens) meal and paste in extruded diets for Atlantic salmon (Salmo salar): Effect on physical pellet quality, nutrient digestibility, nutrient utilization and growth performances. Aquaculture 530, 735785. doi: 10.1016/j.aquaculture.2020.735785
Weththasinghe P., Lagos L., Cortés M., Hansen J. Ø., Øverland M. (2021b). Dietary inclusion of black soldier fly (Hermetia illucens) larvae meal and paste improved gut health but had minor effects on skin mucus proteome and immune response in Atlantic salmon (Salmo salar). Front. Immunol. 12. doi: 10.3389/fimmu.2021.599530
Keywords: aquafeed, long term feeding, bioactive compounds, immunostimulants, health, insect meal, salmonids
Citation: Radhakrishnan G, Liland NS, Koch MW, Lock E-J, Philip AJP and Belghit I (2023) Evaluation of black soldier fly larvae meal as a functional feed ingredient in Atlantic salmon (Salmo salar) under farm-like conditions. Front. Aquac. 2:1239402. doi: 10.3389/faquc.2023.1239402
Received: 13 June 2023; Accepted: 21 September 2023;
Published: 10 October 2023.
Edited by:
Byron Morales-Lange, Norwegian University of Life Sciences, NorwayReviewed by:
Leidy Lagos, AKVA Group, NorwayCopyright © 2023 Radhakrishnan, Liland, Koch, Lock, Philip and Belghit. This is an open-access article distributed under the terms of the Creative Commons Attribution License (CC BY). The use, distribution or reproduction in other forums is permitted, provided the original author(s) and the copyright owner(s) are credited and that the original publication in this journal is cited, in accordance with accepted academic practice. No use, distribution or reproduction is permitted which does not comply with these terms.
*Correspondence: Ikram Belghit, aWtyYW0uYmVsZ2hpdEBoaS5ubw==
†Present address: Erik-Jan Lock, Nutrition and feed technology, NOFIMA, Bergen, Norway
Disclaimer: All claims expressed in this article are solely those of the authors and do not necessarily represent those of their affiliated organizations, or those of the publisher, the editors and the reviewers. Any product that may be evaluated in this article or claim that may be made by its manufacturer is not guaranteed or endorsed by the publisher.
Research integrity at Frontiers
Learn more about the work of our research integrity team to safeguard the quality of each article we publish.