- Auburn University Shellfish Laboratory, School of Fisheries, Aquaculture and Aquatic Sciences, Auburn University, Dauphin Island, AL, United States
Demand for oysters in the premium half-shell market drives the need for research to optimize and improve oyster aquaculture production methods. After setting, oysters are typically maintained in nursery upwellers that provide nutrients and oxygen while removing waste. They remain in the nursery until they are large enough to be deployed in aquaculture gear. Land-based upweller systems include silo and bottle upwellers, with bottles requiring less space and maintenance. However, it is unknown if upweller type influences oyster growth, shape, or survival after field deployment. This study reared Eastern oysters, Crassostrea virginica, to 2 mm in silo or bottle upwellers and deployed them to floating bags for a six-week grow-out period. Oysters were sampled initially, then size graded, counted, and redeployed every two weeks. Changes in growth parameters, quality ratios, survival, and hinge backbend deformity prevalence were determined after six weeks. Initially, silo-reared oysters were larger than those reared in bottles, likely due to the need for flow rate optimization and/or the tumbling action that breaks the fragile growing edge of the shell. Despite these initial differences, there were minimal size differences between upweller types after six weeks of growth in the field. There were no significant differences in survival, hinge backbend abnormality prevalence, or size class distribution between upweller types. These results indicate that upweller choice has minimal impact on the grow-out phase of oyster production and both systems are acceptable for Eastern oyster production in the northern Gulf of Mexico.
1 Introduction
Oyster aquaculture production in the United States has increased an average of 1 million pounds per year since 2012. In 2019, over 42.3 million pounds of oysters were produced, valued at over $220 million (National Marine Fisheries Service, 2022). Increased demand for single-set oysters destined for the high-value half-shell market has led to increased interest in Eastern oyster (Crassostrea virginica) farming along the Gulf coast. The first commercial farms in these regions were established in 2009 in Alabama and Louisiana, followed by Florida in 2015, Mississippi in 2019, and Texas in 2021. Despite the expansion of the oyster farming industry, there remains unfulfilled demand in the half-shell oyster market (Botta et al., 2020). To aid in meeting this need, techniques are required that optimize growth and survival of cultured animals.
In oyster aquaculture the post-set animals, termed seed, are typically maintained in upwelling systems until they are large enough for field deployment. In these systems, water is pumped upwards through the seed, providing food and oxygen while removing waste. Upweller systems may be floating in a natural body of water (field-based) or housed in land-based nurseries. Land-based upwellers are typically silo or bottle systems (Figure 1). Silo upwellers consist of a cylindrical frame constructed from fiberglass, plastic, or PVC. A mesh screen is securely fastened across one end of the cylinder, providing a surface to hold the seed. With the silo suspended in a tank, water flows upwards through the mesh and seed and flows out of a drain near the top of the cylinder. Bottle upwellers consist of inverted cylindrical bottles, which can be constructed from a soda bottle with the bottom removed or built using higher quality components. Piping runs the water vertically through the bottle, adding food and removing waste in a similar manner to silo upwellers. Bottles may be advantageous to silos as they require less space and maintenance.
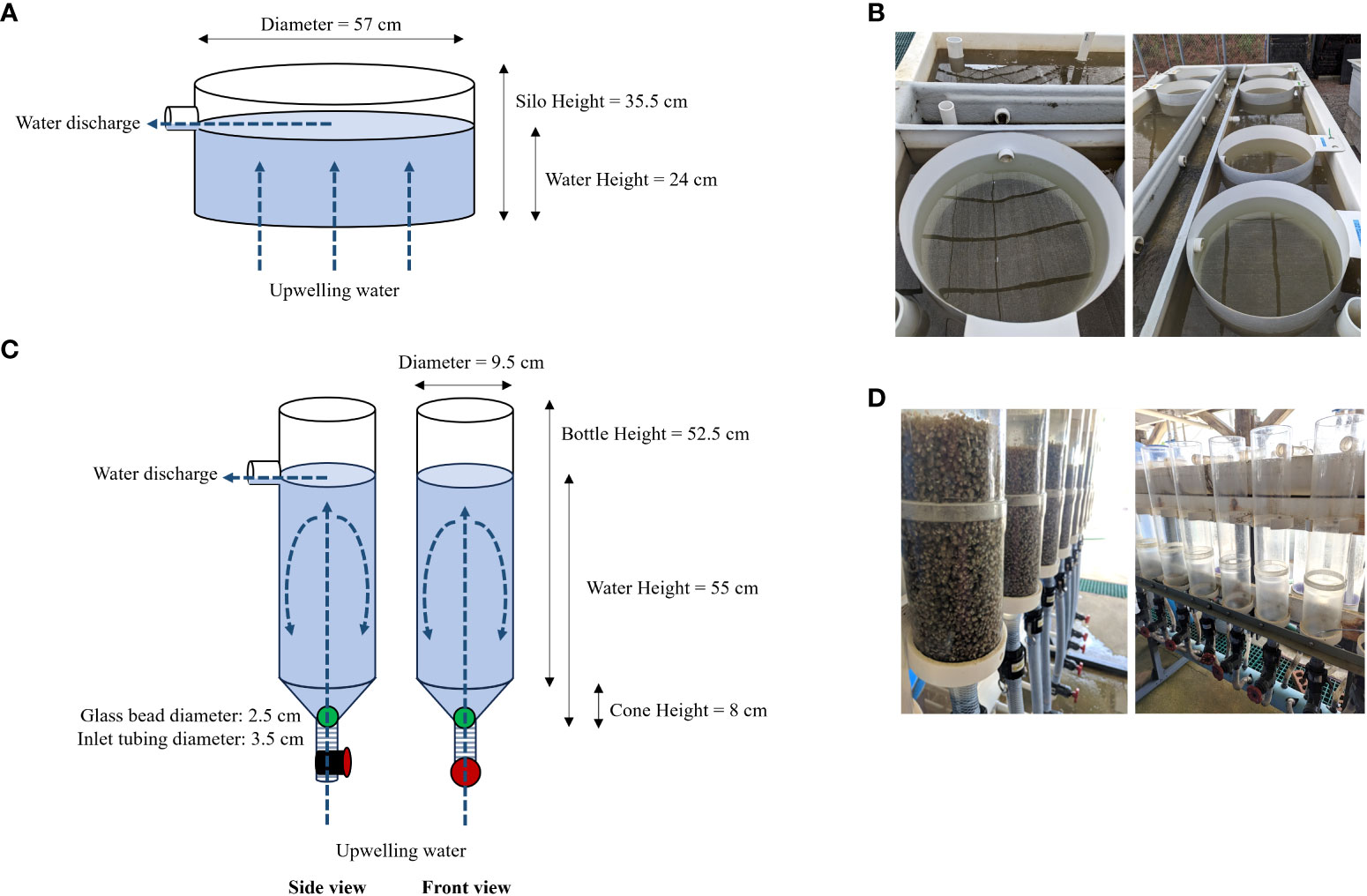
Figure 1 Schematic and images of land-based upweller types used in this study. (A) Silo upweller schematic, (B) Silo upwellers, (C) Bottle upweller schematic, (D) Bottle upwellers.
It is unknown if upweller choice impacts growth, shape, and survival of oysters in later life stages. To that end, the purpose of this study was to investigate the impact of upweller type on subsequent culture of the Eastern oyster.
2 Materials and methods
2.1 Larval rearing
During the summer of 2022, diploid oysters were spawned at the Auburn University Shellfish Laboratory in Dauphin Island, AL using temperature and chemical cues. Larvae were reared in static systems with water changes occurring every other day until larvae with eye spots were observed, at which point water changes occurred daily. Larvae were continuously fed concentrated algae (Shellfish Diet 1800®, Reed Mariculture, Campbell, CA, USA) using a timed pump system. Eyed larvae were graded, moved to downweller silos and allowed to set on 250-micron cultch (finely ground oyster shell). Silo tanks were covered to create a dark setting environment to facilitate the setting process. Post-set oyster seed were graded on a 300-micron screen to separate set oysters from cultch with no set, and set animals were transferred to either silo or bottle upweller systems until they reached R2 (retained on a 2 mm screen during grading). The dimensions for each upweller are shown in Figure 1. Stocking densities were equal across treatments at approximately 75,000 oysters per upweller. Flow rates were not measured in this study; however, known ranges differ between our systems, with bottle upwellers ranging from 3 to 10 liters per minute (L min-1) and silo upwellers ranging from 25 to 35 L min-1. No feed was supplemented to seed during this period.
2.2 Field grow-out
R2 seed was stocked into 1.5 mm mesh bags and deployed in floating cages at the Grand Bay Oyster Park in Grand Bay, Alabama. Seed from each treatment were stocked in triplicate at approximately 18,888 seed per bag except for one bottle replicate that was stocked with 10,000 seed. Oyster quantities were determined using average weight per oyster to calculate weight of oysters to add to the bag. During grow-out, stocking densities were periodically reduced, and bag mesh size increased as described herein. At Week 2, seed was graded through a 6 mm mesh screen and the retained oysters (R6) were restocked at 3,700 seed per replicate in 4 mm mesh bags. At Week 4, seed was graded using a Hoopers Island shaker grader that separates multiple size classes. Oysters retaining on a 16 mm screen (R16) were restocked at 2,500 seed per replicate in 8 mm mesh bags. At each sampling week (Week 2, 4, and 6) following size grading, oysters at each size class were enumerated.
2.3 Sampling
Prior to field deployment, a sample of 50 oysters per treatment was collected for initial measurements (Initial). Final measurements were taken from 25 oysters per replicate bag after six weeks of field deployment. An additional 250 oysters from each replicate were collected at Week 6 to assess hinge backbend abnormalities (a downturn in umbo of the oyster). All animals were frozen at -20°C until analysis. Six-week survival was determined by subtracting the number of remaining live oysters at Week 6 from the Week 4 stocking density (2,500 per bag). Hinge backbend abnormalities were assessed visually from frozen specimens. Shell height, length, and width were measured in mm using digital calipers (see Supplementary Material). Fan, cup, and volume ratios were calculated from these measurements as shown below.
2.4 Data analysis
Differences in Initial measurements between silo- and bottle-reared oysters were assessed using Student’s t-tests. To account for differences in Initial measurements between treatments, change in shell height, length, and width (Final – average Initial for the appropriate upweller) for each individual was calculated at Week 6. For measurements taken after field deployment, technical replicates (individual oysters) were averaged for each replicate bag to generate the numbers used in statistical analyses. Student’s t-tests were used to compare measurements and calculated ratios between upwellers. Distribution of size classes after each grading, six-week survival, and backbend prevalence were compared between upweller types using T-tests. For all analyses, residuals were checked for normality (Shapiro-Wilks) and homoscedasticity (Levene’s test). For Initial fan and cup ratios and Final cup ratios, assumption violations were not improved with transformations, so parameters were analyzed using a non-parametric Wilcoxon rank sum test. All other analyses met the statistical assumptions.
3 Results
3.1 Growth
Prior to field deployment, oysters reared in silos had greater shell height, length, and width than those from bottles, resulting in larger fan and volume ratios (Table 1). To account for initial differences in shell measurements when assessing growth in the field, change in size between Week 6 and initial samples were calculated and analyzed. There were no differences in change of shell height, length, or width between upweller types (Figure 2).
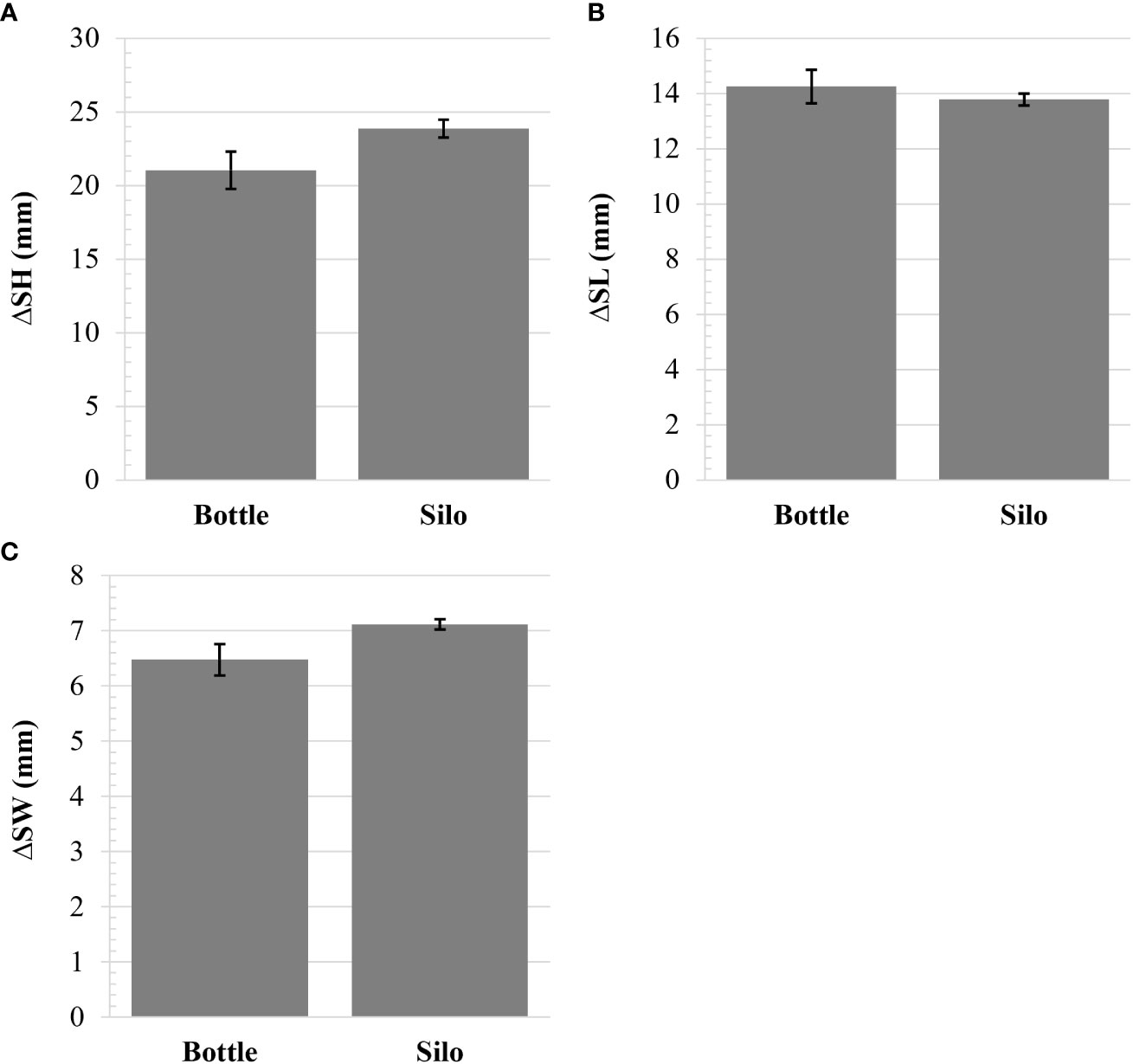
Figure 2 Change in (A) shell height (SH), (B) shell length (SL), (C) and shell width (SW) of oysters reared in each upweller type after 6 weeks of field deployment. There were no significant differences in any measurement (Student’s t-tests).
After 6 weeks in the field, shell width remained significantly higher in oysters from silos, but oysters from bottles had greater fan ratios. Average final shell measurements across both upweller systems were 22.5 mm (SD, 2.2 mm) for height, 14.0 mm (SD, 0.8 mm) for length, and 6.8 mm (SD, 0.5 mm) for width.
3.2 Size classes
The size class distributions were not significantly different between upweller types at any grading (p > 0.05). On average, over 91% of oysters had reached R6 by Week 2. At Week 4, over 91% of oysters were greater than R16, with approximately 14% of these greater than R22. The proportion of oysters at R22 had increased to over 47% at Week 6 (Table 2).
3.3 Survival
There was no difference in survival between upweller types (p = 0.441). Final survival in silo-reared oysters was 88.4% (SD, 1.19%) versus bottle-reared oysters whose final survival was 86.7% (SD, 3.04%).
3.4 Backbend prevalence
There were no significant differences in hinge backbend abnormality prevalence between upweller types (p = 0.669). Backbend prevalence in oysters reared in bottles was 41.0% (SD, 7.22%) and prevalence in oysters reared in silos was 37.3% (SD, 12.2%).
4 Discussion
Results from this study indicate that there are limited effects of upweller type on oyster growth following field deployment. Initially, oysters from silo upwellers were larger than those from bottle upwellers. There are two potential explanations for this discrepancy. Firstly, these differences may be due to varying flow rates in the two upweller systems, as previous research indicates that growth is directly correlated with water flow (Bishop and Hooper, 2005; James, 2022). The increase in flow promotes access to food and improves waste removal. The flow was not recorded in this study but the maximum flow rates for our systems are 10 L min-1 for bottle upwellers and 35 L min-1 for the silos. Therefore, it is likely that the animals in silos had access to more food than those in bottles during the nursery phase, explaining their larger size upon field deployment. Feed supplementation or mechanisms to increase flow may be able to circumvent these differences. The second possibility is that the tumbling action that occurs in bottle upwellers negatively impacts the oysters. This action may be a stressor which results in reduced growth rates and/or the breakage of the fragile growing edges giving the illusion of slower growth (Bodenstein et al., 2021). In this case, the initial size difference may not be avoidable. Regardless, the size differences at the onset of the experiment were minimal (< 1.5 mm in all measurements) and were diminished following field deployment.
Despite silo-reared oysters having higher initial fan ratios, this ratio was higher in bottle-reared oysters at the conclusion of the study. These differences were miniscule (< 0.08) and final fan ratios in both treatments were greater than 0.67 which is considered a good ratio for the shellfish market (Walton et al., 2013). Average cup ratio in both treatments was greater than 0.28 which is also considered acceptable (Walton et al., 2013). Despite having a higher initial volume ratio, silo-reared oysters ended the study with similar volume ratios to bottle-reared oysters. The final volume ratio average between treatments was 166 which is appropriate for the shellfish market and similar to those reported in other upweller studies (Poirier et al., 2020).
Hinge backbend abnormalities make oysters more difficult to shuck. The prevalence of hinge backbend was similar between upwellers. Approximately 40% of individuals had backbends which is lower than the percentage observed in our facility’s previous datasets (~60-70%, unpublished data). It should be noted that the line used in this study has been selectively bred to reduce backbend abnormalities. Total survival and size distributions were also similar between upweller types. Overall, these data indicate that the choice of upweller does not impact the market potential of the product.
Overall, there was minimal effect of upweller type on oyster production through six weeks of field deployment. The results from this study indicate that both bottles and silos are appropriate for Eastern oyster production in the Gulf of Mexico. The relatively small footprint of bottle upwellers may provide a benefit for nurseries with limited space.
Data availability statement
The raw data supporting the conclusions of this article will be made available by the authors, without undue reservation.
Ethics statement
Ethical review and approval was not required for the study on animals in accordance with the local legislation and institutional requirements.
Author contributions
AT and SR designed the experiment. AT led data collection and analysis and writing of the manuscript. KL maintained the oysters while in the field. All authors aided in sampling. All authors contributed to the article and approved the submitted version.
Funding
This research was partially funded through the Dauphin Island Sea Lab NSF REU Program (REU 2150347).
Acknowledgments
Staff and students at the Auburn University Shellfish Laboratory aided in oyster maintenance and sampling, including Emily Johnson, Heather King, Mason Williams, Kayla Boyd, and William Kleist. NSF REU Alexes Cleveland participated in sampling and measurement efforts.
Conflict of interest
The authors declare that the research was conducted in the absence of any commercial or financial relationships that could be construed as a potential conflict of interest.
The author AT declared that they were an editorial board member of Frontiers, at the time of submission. This had no impact on the peer review process and the final decision.
Publisher’s note
All claims expressed in this article are solely those of the authors and do not necessarily represent those of their affiliated organizations, or those of the publisher, the editors and the reviewers. Any product that may be evaluated in this article, or claim that may be made by its manufacturer, is not guaranteed or endorsed by the publisher.
Supplementary material
The Supplementary Material for this article can be found online at: https://www.frontiersin.org/articles/10.3389/faquc.2023.1236346/full#supplementary-material
References
Bishop M. J., Hooper P. J. (2005). Flow, stocking density and treatment against Polydora spp.: Influences on nursery growth and mortality of the oysters Crassostrea virginica and C. ariakensis. Aquaculture 246 (1-4), 251–261. doi: 10.1016/j.aquaculture.2005.01.021
Bodenstein S., Walton W. C., Steury T. D. (2021). Effect of farming practices on growth and mortality rates in triploid and diploid eastern oysters Crassostrea virginica. Aquacult. Environ. Interact. 13, 33–40. doi: 10.3354/aei00387
Botta R., Asche F., Borsum J. S., Camp E. V. (2020). A review of global oyster aquaculture production and consumption. Mar. Policy 117, 1–7. doi: 10.1016/j.marpol.2020.103952
James C. (2022). Determining growth potential of the Eastern oyster by volumetric comparison utilizing soda bottle upwellers (Massachusetts: Aquaculturual Research Corporation).
National Marine Fisheries Service (2022). Fisheries of the United States 2020. Eds. Cody R., Liddel M., Yencho M. (Silver Spring, MD: U.S. Department of Commerce).
Poirier L. A., Clements J. C., Sonier R., Lanteigne L., Nadeau A., Comeau L. A. (2020). Testing the efficacy of bouncing-bucket nursery systems for enhancing shell strength and thickness in on-bottom cultured Eastern oysters (Crassostrea virginica). Aquacult. Eng. 90, 1–7. doi: 10.1016/j.aquaeng.2020.102101
Walton W. C., Rikard F. S., Chaplin G. I., Davis J. E., Arias C. R., Supan J. E. (2013). Effects of ploidy and gear on the performance of cultured oysters, Crassostrea virginica: survival, growth, shape, condition index and Vibrio abundances. Aquaculture 414-415, 260–266. doi: 10.1016/j.aquaculture.2013.07.032
Keywords: eastern oyster, Crassostrea virginica, aquaculture, nursery, upweller
Citation: Tarnecki AM, Landry K and Rikard S (2023) Nursery upweller type has minimal impact on subsequent grow-out of Eastern oysters (Crassostrea virginica). Front. Aquac. 2:1236346. doi: 10.3389/faquc.2023.1236346
Received: 07 June 2023; Accepted: 25 September 2023;
Published: 11 October 2023.
Edited by:
Charles Weirich, NOAA National Sea Grant Office, United StatesReviewed by:
Kevin Marquez Johnson, California Polytechnic State University, United StatesSarah Pedigo, South Carolina Sea Grant Consortium, United States
Copyright © 2023 Tarnecki, Landry and Rikard. This is an open-access article distributed under the terms of the Creative Commons Attribution License (CC BY). The use, distribution or reproduction in other forums is permitted, provided the original author(s) and the copyright owner(s) are credited and that the original publication in this journal is cited, in accordance with accepted academic practice. No use, distribution or reproduction is permitted which does not comply with these terms.
*Correspondence: Andrea M. Tarnecki, YXRhcm5lY2tpQGF1YnVybi5lZHU=