- 1Department of Nutrition and Feed Technology, Nofima, Tromsø, Norway
- 2Department of Animal and Aquaculture Sciences, Norwegian University of Life Sciences, Ås, Norway
- 3Department of Fish Health, Nofima, Tromsø, Norway
- 4Department of Production Biology, Nofima, Tromsø, Norway
- 5Havsbrún P/F, Fuglafjørður, Faroe Islands
- 6Department of Laboratory Medicine, Division of Diagnostic Services, University Hospital of North Norway, Tromsø, Norway
- 7Department of Medical Biology, Faculty of Health Sciences, UiT-The Arctic University of Norway, Tromsø, Norway
- 8Department of Clinical Medicine, Tromsø Endocrine Research Group, Uit-The Arctic University of Norway, Tromsø, Norway
Introduction: Maturation in seawater-adapted (smoltified) male Atlantic salmon is undesirable yet frequently reported in fish weighing up to 1 kg reared under intensive conditions in land-based facilities. Although the photoperiod regulates both smoltification and maturation, the optimal post-smoltification regimen for the prevention of maturation is unclear.
Methods: In this study, mixed-sex Atlantic salmon weighing 95 g were exposed to three different photoperiod regimes: continuous daylength, gradually decreasing daylength, and short-to-long daylength after the induction of smoltification by the “winter signal” (12 h:12 h light:dark). Fish were maintained under these conditions in triplicate for 18 weeks in a brackish water flow-through system at 12°C and with a salinity of 20 ppt. This initial phase was followed by a grow-out period (1 kg–2.5 kg) in seawater (32-ppt salinity) at 13°C for 11 weeks, with exposure to decreasing daylength. Throughout the 29-week study, body condition, gonad development, and morphological traits were assessed. Additionally, during exposure to brackish water, serum 11-ketotestosterone (11-KT) levels were quantified, and the testes were examined histologically. Upon termination of the study, the maturation rate was determined.
Results: This study exclusively detected maturation among male salmon. Under continuous light exposure, the number of fish initiating maturity steadily increased from week 8 and continued to increase in the subsequent samplings. In the short-to-long daylength group, a subset of male fish showed elevated 11-KT levels at week 14, although their gonadal development and morphological changes were not evident until week 18. Male fish exposed to decreasing daylength showed no substantial increase in 11-KT levels, although a few fish exhibited advanced gonad development by week 18. At the end of the trial, markedly lower percentages of maturing and fully mature male fish were observed among those fish previously exposed to decreasing daylength. The maturation rates at study termination were 44.4% for the short-to-long daylength group, 29.4% for the continuous daylength group, and 4.3% for the decreasing daylength group.
Discussion: These results show that photoperiod can be utilized to regulate maturation in male Atlantic salmon. In addition, decreasing the photoperiod after smoltification seems to limit early sexual maturation without compromising body size development. Thus, decreasing the photoperiod could be utilized to limit maturation in the production of post-smolt salmon or salmon in land-based facilities.
1 Introduction
In commercial aquaculture, Atlantic salmon (Salmo salar) have traditionally been raised in land-based freshwater facilities until the completion of smoltification. The fish are then transferred and kept in open cages in the sea until they reach slaughter size. Sea-based production presents several challenges, however, including variations in ambient temperature, handling events, escape, disease outbreaks, mortality, and salmon lice infestation (Gullestad et al., 2011; Statistics Norway, 2022). The interest in extending the production cycle on land by utilizing land-based closed containment systems (CCSs) has grown in recent years. This approach aims to reduce the time fish spend in the sea by allowing them to reach a larger size in a land-based system (i.e., until they weigh 500 g–1,000 g) (Fossmark et al., 2021; Roalkvam et al., 2021; Ytrestøyl et al., 2022). Currently, several initiatives using CCS approaches to rear salmonids to market size are underway. One of the key requirements of these systems is that they enable fish to maintain a high growth performance. This can be achieved by maintaining high water temperatures and providing continuous light. However, recent studies have revealed that these factors may increase the likelihood of sexual maturation in fish (McClure et al., 2007; Fjelldal et al., 2011; Qiu et al., 2015; Good et al., 2016; Good et al., 2017; Ytrestøyl et al., 2022; Fraser et al., 2023a). Sexual maturation is highly undesirable in commercial aquaculture because mature fish cease feeding, their fillet quality deteriorates (Aksnes et al., 1986; Kadri et al., 1996), and the ability of fish to hypo-osmoregulate is diminished (Fjelldal et al., 2018). This in turn leads to reduced growth and poor welfare, ultimately resulting in economic losses for the producer (McClure et al., 2007).
Early maturation is a significant concern, particularly in male fish. In female fish, by contrast, maturation is not typically initiated until the fish reach a larger body size and achieve an energy surplus, a process that normally requires at least 2 years in nature (Taranger et al., 2010). However, high water temperature may also increase the risk of early maturation in female fish weighing< 1 kg (Fjelldal et al., 2018). The reproductive strategy is quite diverse in both wild and domestic salmon, with high variability in terms of reproductive traits, age, and size at maturation. Post-smolt maturation, commonly referred to as “jacking”, appears to be more prevalent among domesticated male fish raised in commercial facilities (Stefansson et al., 1993; Thrush et al., 1994; Imsland et al., 2014). Multiple factors affect the timing and initiation of puberty in salmon, including the water temperature (Fjelldal et al., 2011), feed intake (Kadri et al., 1996), nutrition (Alne et al., 2009), lipid reserves (Rowe and Thorpe, 1990), growth rate (Duncan et al., 1999), and stock genetics (Wolters, 2010). However, the photoperiod also serves as an important cue, functioning as a zeitgeber (Taranger et al., 2010; Pino Martinez et al., 2023a). Consequently, photoperiod manipulation can either stimulate or suppress sexual maturation in farmed salmonids.
Maturation onset is regulated by activation of the brain–pituitary–gonad axis (Taranger et al., 2010). The activation of this axis triggers an upsurge in the production of follicle-stimulating hormones by the pituitary gland, which subsequently bind to testis receptors and stimulate the increased synthesis of 11-ketotestosterone (11-KT). These hormonal shifts support the progression of spermatogenesis (Maugars and Schmitz, 2008; Schulz et al., 2010). Multiple parameters must be considered when assessing maturity in male salmon, including blood steroid hormones (e.g., 11-KT), relative testis weight (gonadosomatic index), testis cell development (evaluated histologically), and the underlying molecular mechanisms of maturation, such as the transcript abundance of relevant genes expressed in the pituitary gland and gonads (Idler et al., 1971; Hunt et al., 1982; Anderson et al., 1988; Berglund et al., 1995; Melo et al., 2014; Fjelldal et al., 2018; Fraser et al., 2019; Fraser et al., 2023a; Pino Martinez et al., 2023a). However, it is important to note that the cascading events leading to maturation do not occur simultaneously, and puberty can be terminated in salmonids in its initial stages (Fraser et al., 2019). Whether sexual maturation is initiated or terminated depends on various environmental and physiological factors, in addition to genetic background (Fjelldal et al., 2020; Pino Martinez et al., 2023a). The complexity of the process underscores the importance of the approach used to evaluate puberty and sexual maturation.
Rearing salmon under continuous light and elevated water temperature with no exposure to darkness results in a high maturation rate (Imsland et al., 2014). However, exposing fish to the square-wave (long-short-long day) “winter signal” [light hours to dark hours (L:D) 24 h:0 h → 12 h:12 h → 24 h:0 h), which is traditionally employed to induce smoltification, can also trigger maturation. As demonstrated by Fjelldal et al. (2018), smoltification and maturation can occur in parallel, suggesting that the same photoperiodic cue governs these processes. In line with this hypothesis, Pino Martinez et al. (2023a) demonstrated that employing the “winter signal” increases maturation rates compared with continuous light exposure. Good et al. (2016) explored this phenomenon in larger fish in freshwater and reported that salmon subjected to the “winter signal” and subsequently to a scotophase (L:D cycle of 18 h:6 h) exhibited significantly higher maturation rates than salmon reared under continuous light. In contrast, studies by Fjelldal et al. (2011) and Fraser et al. (2023a) found that exposure to scotophase during the latter stages of smoltification induced by the “winter signal” after the short-day period (L:D cycle of 12 h:12 h) reduced pubertal initiation compared with continuous light exposure. However, except for the study of Good et al. (2016), the aforementioned studies were short-term studies; therefore, the final maturation rate achieved using these photoperiods is unknown. In saline environments, Duncan et al. (1999) and Qiu et al. (2015) observed higher maturation rates in salmon exposed to continuous light than in those exposed to a constant scotophase. The abovementioned studies highlight the complexity of assessing photoperiod as a cue and how its effect differs under specific conditions. As illustrated, maturation can occur following exposure to constant scotophase, increasing daylength, or continuous daylength, depending on factors such as temperature, exposure to the “winter signal”, and salinity. However, exposure to a shorter scotophase during the latter stage of the “winter signal” or after the completion of the “winter signal” delays pubertal development in salmon (Duncan et al., 1999; Fjelldal et al., 2011; Qiu et al., 2015; Fraser et al., 2023a).
Few long-term studies have examined the effects of gradual changes in daylength on the growth and sexual maturation of salmon post smolts. Due to the great interest in post-smolt production in CCSs, research aimed at improving productivity and/or suppressing maturity through photoperiodic manipulation should be revisited. The aim of the present study, therefore, was to examine the initiation of maturation during the post-smolt phase and evaluate the incidence of sexually mature fish using an applied approach. We compared the effects of exposure to continuous light, gradually decreasing daylength, and short-to-long daylength on the growth and maturation of fish in brackish water (20-ppt salinity) during the post-smolt phase (100 g–1,000 g), prior to the grow-out phase, during which fish were exposed to decreasing daylength in seawater (32-ppt salinity). Body weight (BW), condition factor (CF), growth rate, gonad scoring, and morphology were assessed throughout the study. During the initial 18 weeks of exposure to brackish water, additional measures were analyzed, including 11-KT levels, gonadosomatic index (GSI), feed utilization, and testis histology. At termination of the study, the maturation rate and GSI of mature fish were determined.
2 Materials and methods
2.1 Fish and experimental design
The fish used in this study were sourced from the commercial freshwater facility Belsvik of Lerøy Midt AS (Hellandsjøen, mid-Norway). The eye eggs used in the study were obtained from the breeding nucleus of AquaGen AS (QTL, IPN/PD/CMS/LICE). Hatching took place on 24 October 2019 under controlled conditions at a water temperature of 6°C. Feeding was initiated on 11 December 2019, under continuous light and a flow-through freshwater system, maintaining a temperature of 12°C. Following the commencement of feeding, all salmon were relocated to tanks connected to a recirculating aquaculture system (RAS) with a temperature of 12°C and subjected to short-day conditions (L:D 12 h:12 h) beginning on 8 April 2020. On 14 May 2020, all fish underwent routine vaccination, which included bath vaccination using AlphaDIP ERM (Pharmaq, Norway), intraperitoneal injection with the oil-adjuvanted vaccine AlphaJECT micro 6 (Pharmaq), and intramuscular injection with the DNA-vaccine Clynav (Elanco, Norway). On 27 May 2020, a total of 8,600 salmon were distributed among four tanks on a truck (Malo Transport AS) and transported to the Nofima Center for Aquaculture Research, located in Sunndalsøra, mid-Norway.
At the Nofima Center for Aquaculture Research, the fish were moved into four tanks and exposed to a continuous light cycle (L:D 24 h:0 h) for a duration of 6 weeks to complete the smoltification process. The progression of smoltification and smolting status were assessed by conducting a seawater challenge test (72 h, 32-ppt salinity) and measuring plasma osmolality, chloride content, and gill Na+ and K+-ATPase activity, both before and after seawater exposure, following the standard procedures reported by Clarke et al., 1996. Following the successful confirmation of smoltification, 2,700 smolts (95.1 g ± 0.3 g, tank mean ± SD, n = 9) were selected from the original group of 8,600 smolts for inclusion in the current experiment. These chosen smolts underwent a careful tagging process utilizing passive integrated transponders (PITs) and were subsequently allocated to nine fiberglass tanks (of 3.2 m3 and 2 m diameter) containing 300 fish each. The distribution and tagging procedures were completed between 29 June 2020 and 3 July 2020. During this period, all smolts underwent a comprehensive examination, which included the visual inspection and determination of their weight and length. During the selection and tagging of smolts, fish exhibiting evident signs of wounds, parr-marks, or poor body condition (runts) were removed and euthanized with a lethal dose of metacaine (MS-222, Alpharma, Animal Health Ltd., Hampshire, UK). The tanks were supplied with running brackish water, maintaining an average salinity of 20.0 ppt ± 0.4 ppt and a mean temperature of 12.1°C ± 0.2°C. The selection of a 20-ppt salinity gradient was deliberate, as several commercial farmers use brackish water (20-ppt salinity) during the production post-smolt phase. This is done to minimize the risk of de-smoltification (Mortensen and Damsgård, 1998) and reduce the osmotic stress/difference of brackish water compared with full-strength seawater.
The smolts underwent a 4-day period of acclimation to their new environment before the first part of the experiment, which lasted from 7 July 2020 to 13 November 2020 (18 weeks). On 7 July 2020, the tanks were randomly allocated to three distinct photoperiodic regimens, each with triplicate setups, as follows: (i) gradually decreasing daylength (DL)—initially, the DL group was subjected to an L:D cycle of 24 h:0 h. This was then gradually modified, with a 45-min reduction in daylight duration initiated once each week. This reduction continued until the end of the first part of the experiment (18 weeks), when the LD cycle reached 10.5 h:13.5 h (Figure 1A). (ii) Continuous daylight (LL). The LL group was maintained under a consistent L:D cycle of 24 h:0 h for the entire 18-week duration of the experiment (Figure 1B). (iii) Short-to-long daylength (SL). The SL group was shifted from an L:D cycle of 24 h:0 h to an L:D cycle of 12 h:12 h at the beginning of the trial. Thereafter, this group was subjected to a weekly increase of 45 min in daylight until week 16, when they reached an LD cycle of 24 h:0 h, which was sustained until week 18 (Figure 1C). Each tank was equipped with a single fluorescent lamp, and the light intensity was consistently monitored at the water surface, maintaining an average of 13.0 ± 0.7 µmols−1 m−2. To mitigate the light pollution among the tanks, each tank was covered with a black tarp.
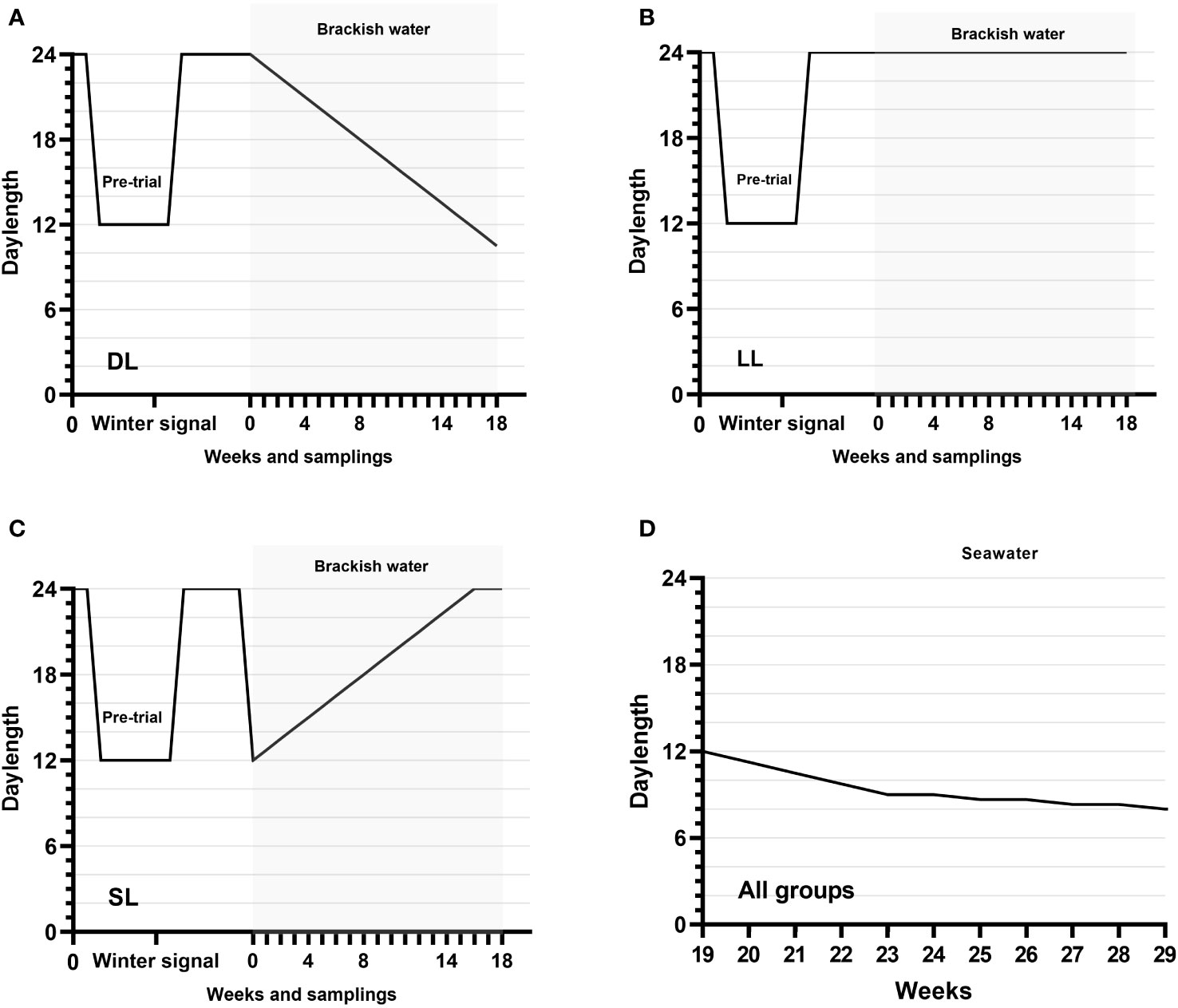
Figure 1 Experimental design. (A) The photoperiod of the DL group exposed to decreasing daylength for 18 weeks in flow-through brackish water, analyzed in triplicate. (B) The photoperiod of the LL group exposed to continuous light for 18 weeks in flow-through brackish water, analyzed in triplicate. (C) The photoperiod of the SL group exposed to increasing daylength for 16 weeks and continuous light for 2 weeks in flow-through brackish water, analyzed in triplicate. (D) All groups were exposed to decreasing daylength for 11 weeks in one seawater RAS tank (weeks 19–29).
At the end of week 18, 100 fish were selected from each tank and relocated to a 100-m3 tank, integrated into a recirculation system with a total volume capacity of 159.8 m3. The tank was supplied with seawater, maintaining a stable salinity of 32.7 ppt ± 0.4 ppt, and the water temperature was maintained at an average of 13.3 ± 0.5°C (mean ± SD). The water flow to the tank was regulated at 34.8 L/min (1,900 L/h), ensuring an effective daily water exchange rate of approximately 31.4% ± 0.1% (mean ± SD). The water quality parameters were closely monitored, with the average pH recorded at 7.38 ± 0.12, alkalinity 88.5 ± 23.33, NO2–N 0.62 ± 0.32, NO3–N 5.52 ± 3.35, and NH4–N 0.21 ± 0.13 (mean ± SD). At the outset of week 19, the tank’s lighting conditions were initiated with an L:D cycle of 12 h:12 h. Subsequently, there was a gradual reduction in daylength by 45 min per week for 4 consecutive weeks (end of week 22). From this point, the daylength for this tank was further reduced by 20 min once every 2 weeks, culminating in an L:D cycle of 8 h:16 h during week 29, marking the end of the experiment (Figure 1D).
2.2 Diets and feed collection
A high-energy extruded feed, in three different pellet sizes, was formulated and manufactured by Havsbrún (Fuglafjørður, Faroe Islands). The pellet sizes were 4 mm, 6 mm, and 9 mm, with protein/fat levels of 47%/25%, 46.5%/29.5%, and 45%/30%, respectively. Fish were fed pellets measuring 4 mm from the start of the experiment until week 14 (i.e., when they weighed 95 g–700 g), pellets measuring 6 mm between weeks 15 and 19 (700 g–1,000 g), and 9 mm from week 20 until termination (1,000 g–2,300 g). Feed was distributed at hourly intervals during daylight hours in each respective group. To monitor feed consumption and feeding efficiency, the tank outlets were equipped with excess feed collectors. These collectors facilitated the retrieval of uneaten feed after each feeding session, which allowed the calculation of daily feed intake and feed conversion ratios. The feed intake calculations adhered to the methodology outlined by (Helland et al., 1996). The feed rations were set to provide fish with 5%–10% more than their estimated daily feed intake, with adjustments made based on the quantity of uneaten feed collected during previous feedings. Following exposure to seawater, no specific feed collection was conducted, and the fish were fed to satiation with a slight overfeeding margin of 2%–5%, as evaluated by feed waste within the RAS.
2.3 Sampling protocol
During the initial phase of the study, spanning from week 0 to week 18, a series of samplings was conducted at the following intervals: weeks 0, 4, 8, 14, and 18. At weeks 0, 8, and 18, all fish underwent individual registration, which encompassed the recording of PIT-tag data, weight, and length. In parallel to these samplings, a specific subset of fish (n = 25 per tank, n = 75 per experimental group) was selectively sampled for further analysis. At weeks 4 and 14, approximately 60% of the fish were randomly selected for the individual registration of PIT-tag data, weight, and length, and the remaining fish were bulk weighed. In both instances, 15 fish per tank (n = 45 per experimental group) were used for further analysis. At week 18, 100 fish from the original 300 per tank (minus the sampled fish) were reallocated and exposed to seawater. The remaining fish (n in the DL group = 55, n in the LL group = 52, n in the SL group = 57) were euthanized through the administration of an overdose of MS-222 before a thorough examination of both the external and internal markers of sexual maturity was conducted, as detailed in section 2.6 below. Prior to the individual registration (weight and length), the fish were gently captured and anesthetized using MS-222 (metacaine 0.1 g L−1; Alpharma, Animal Health Ltd., Hampshire, UK) while they were immersed in oxygenated water. The fish selected for further analysis were euthanized using an overdose of MS-222. The blood samples were collected from the caudal vein at weeks 0, 8, 14, and 18 (from 15 fish per tank, except for week 0, when 10 fish per tank were sampled) using gel vacutainers (BD Vacutainer® silica act clot activator, silicone-coated interior, and red BD Hemogard™ closure). These samples were subsequently centrifuged for 10 min at 5,000 rpm, and the separated serum was then frozen at −80°C until steroid hormone analysis. Sex was determined for all the fish sampled for further analysis, and at the 8-week and 18-week sampling points the gonads were carefully dissected, weighed, and then preserved in containers with 10% neutral formalin (BiopSafe®, Mermaid Medical, Denmark) until further histologic analysis.
In the saltwater phase of the study (weeks 19–29), the PIT-tags of all fish were recorded, and the measurements of weight and length of individual fish were obtained at week 22 and upon the termination of the experiment at week 29. During the final sampling, all experimental fish were euthanized through the administration of an overdose of MS-222 before a thorough examination of both external and internal markers of sexual maturity was conducted, as detailed in section 2.6 below.
2.4 Analysis of 11-KT levels in serum
11-KT was analyzed using liquid chromatography–tandem mass spectrometry (LC-MS/MS) at the Proteomics and Metabolomics Core Facility (PRiME) at the University of Tromsø, Norway. The analyses were carried out using an Acquity UPLC I-Class instrument interfaced to a Xevo TQ-XS tandem mass spectrometer (Waters, Manchester, UK). The samples were analyzed in ESI-positive mode under the following conditions: capillary voltage, 1 kV; desolvation gas temperature, 550°C; source temperature, 150°C; desolvation gas flow, 1,000 L/h; cone gas flow, 150 L/h; nebulizer pressure, 7 bar. The chromatographic separations were achieved on an Acquity Cortecs T3 1.6 µm 100 × 2.1 mm (Waters) column maintained at 50°C at a flow rate of 0.3 mL/min. A linear gradient composed of 0.1% formic acid in water and 0.1% formic acid in methanol was used, starting at 40% (v/v) methanol, and increasing to 70% over 8 min, maintaining at 95% for 0.5 min, then returning to the starting conditions. The autosampler temperature was 6°C, and the sample injection volume was 4 μL. The data were acquired and analyzed using MassLynx software, version 4.2. See Supplementary Materials for used chemicals, sample preparation and retention times etc. of different analytes using this method. To convert from nmol/L (SI unit) to ng/mL (conventional unit), the nmol/L value was multiplied by each hormone’s respective mass (g/mol). LC-MS/MS has been used to quantify hormones such as 11-KT in a variety of biological samples, including those of different fish species (Rege et al., 2013; Nouri et al., 2020; Papadaki et al., 2021; Ikegawa and Hasegawa, 2023). This method has been validated for numerous hormones, including 11-KT, and can detect very low levels of hormones, making it valuable for the quantification of hormone levels in juvenile and/or small fish (Nouri et al., 2020). The LC-MS/MS approach has been proven to reduce analysis time without compromising the accuracy of results when compared with enzyme-linked immunosorbent assays (ELISA) (Bowerbank et al., 2019). However, this method has not been verified for the analysis of salmon plasma.
2.5 Histologic analysis
The testis tissue samples were sectioned and stained at the Norwegian Veterinary Institute in Harstad, Norway. In brief, tissue cross sections were hydrated in distilled water and then stained using 1% Alcian Blue solution (Alfa Aesar) in a 3% acetic acid medium for 15 min. The sections were then transferred to a solution of 1% periodic acid (VWR) for 10 min, and then treated using Schiff’s reagent (Sigma-Aldrich) for 15 min. Subsequently, sections were immersed for 30 s in hematoxylin solution (VWR) to counterstain the nuclei. This was followed by a process of dehydration and ultimate mounting. Tissue sections were digitized using a NanoZoomer S360 scanner (Hamamatsu, Tokyo, Japan). After digitization, each entire tissue section was independently analyzed by two trained histologists.
The testis maturation stage was determined using a scoring system (I–V) adapted from the methodologies outlined by Ciani et al. (2021) and Melo et al. (2014), as presented in Figure 2 and Supplementary Table 1. It is noteworthy that no immunohistochemical techniques were employed to assess the germ and Sertoli cell proliferation, such as the analysis of phosphorylated histone H3 as a marker, as demonstrated in Melo et al. (2014). Consequently, both undifferentiated and differentiated type A spermatogonia were not distinguished, in alignment with the scoring reported by Ciani et al. (2021). Thus, testis scoring involved the assessment of the most advanced germ cells present in the tissue.
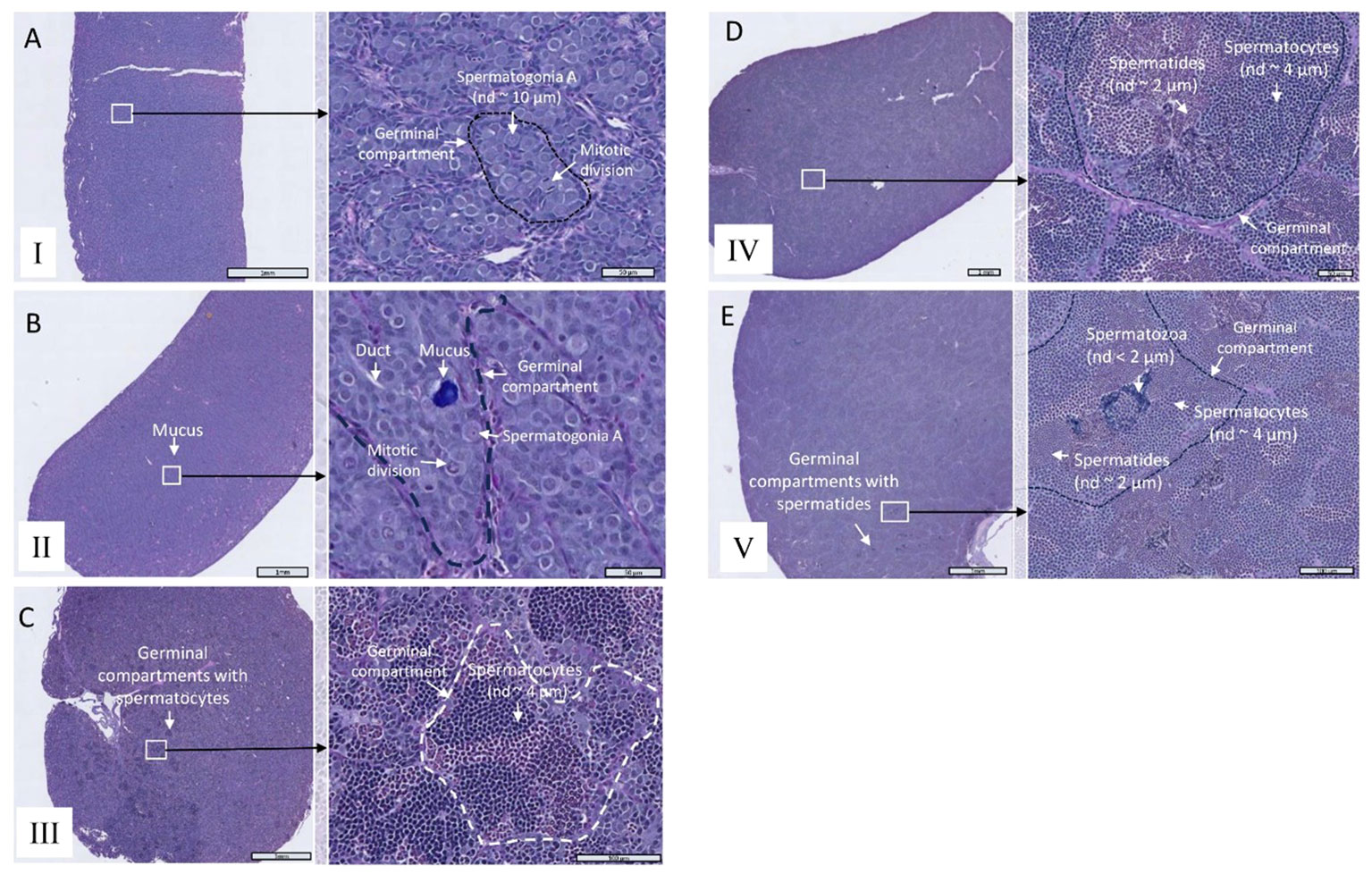
Figure 2 Representative photomicrographs of Alcian blue– and PAS-stained tissue sections and fish testes depicting maturation stages I–V. (A) Stage I, (B) Stage II, (C) Stage III, (D) Stage IV, and (E) Stage V. The images on the left provide an overview of the testicular tissue at a lower magnification, whereas the corresponding images on the right offer detailed views of magnified areas marked by white rectangles. In each section, one germinal compartment is outlined by dotted lines; typically, multiple cell stages are present in one compartment, and the maturation stages are indicated. Nuclear diameter (nd) serves as an indicator of the developmental stage.
2.6 Assessment of sexual maturity and classifying male fish as fully mature
Both external and internal indicators of sexual maturity were assessed. This examination was carried out on all the fish selected for further analysis at week 18 and on all the remaining experimental fish at the conclusion of the study. External attributes such as skin spotting (Figure 3), coloration (specifically the brown-green cover for male fish), and kype development were visually scored on an ordinal scale ranging from 0 to 3. This evaluation was predicated on the amount and intensity of skin spots; the visibility of the green and/or brown skin cover, especially in the head region; and the morphology of the jaw, particularly the presence of a hook-like curvature at the distal end of the lower jaw of male fish. Following the external scoring and determination of sex, each set of gonads was visually assessed and assigned a maturity score ranging from 1 to 5. This scoring system was devised based on consideration of the length and size of the gonads of salmonids, drawing from the categorization initially proposed by Dahl (1917) and later refined by Jonsson and Matzow (1979), as illustrated in Figure 4. The gonads of male fish and female fish with a score of > 1 were dissected and weighed at termination. This simplified scoring method was employed at the final sampling due to practical constraints related to the large number of fish and biomass. Male fish with a gonad score of > 1, at which the lowest GSI was 0.21%, were considered maturing. To identify the fully mature male fish, a bimodal approach was adopted, aligning with observed patterns in the gonad score, skin spots, skin color, jaw development, and CF. Threshold values (≥ 2) were set for the mentioned parameters, along with a CF that was lower than the value recorded in week 22. The individuals meeting or surpassing these thresholds were classified as fully mature.
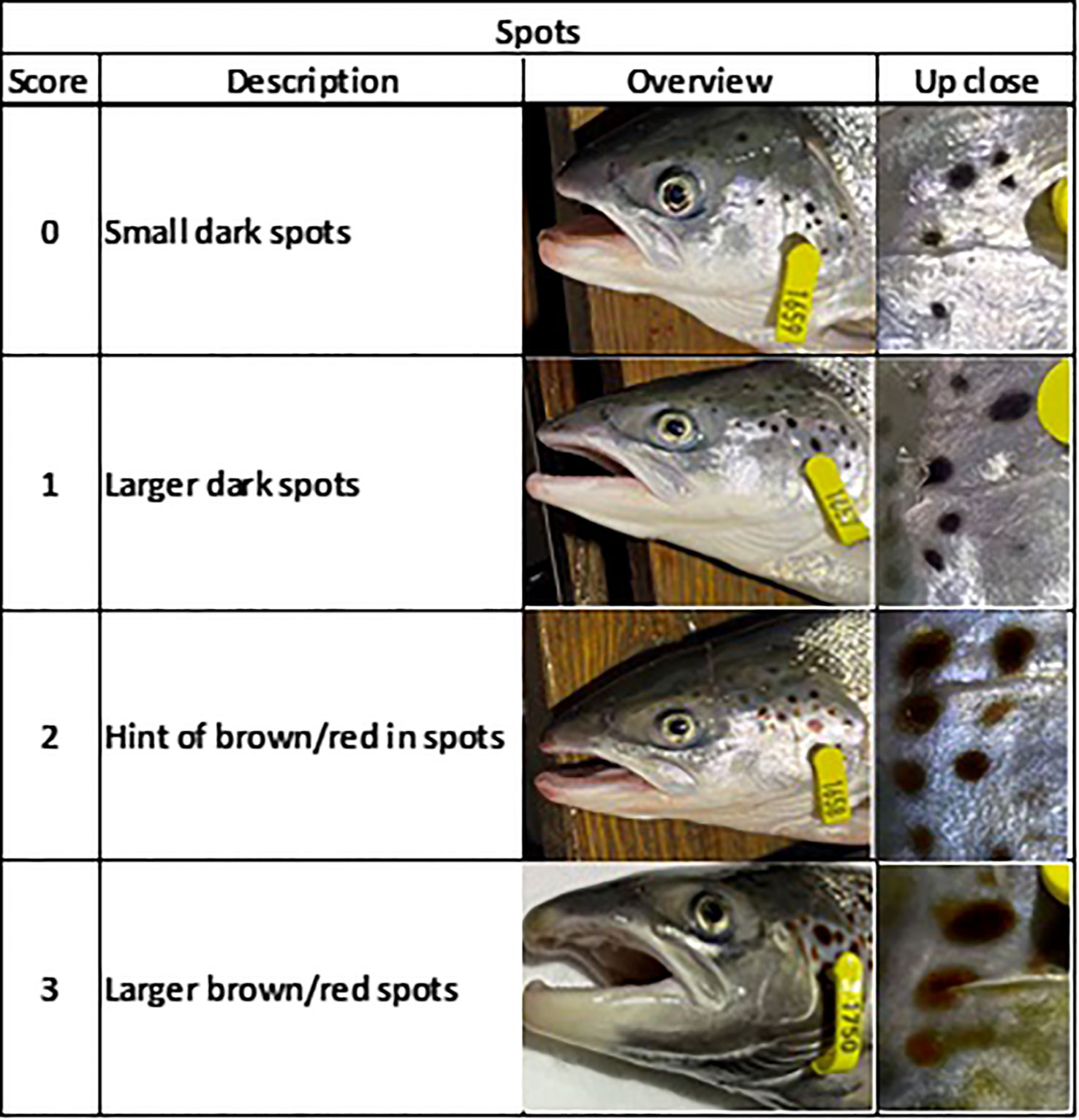
Figure 3 The system for scoring spots on the operculum and skin of Atlantic salmon. The scores were determined by evaluating the size and color of the spots, based on a grade of 0–3.
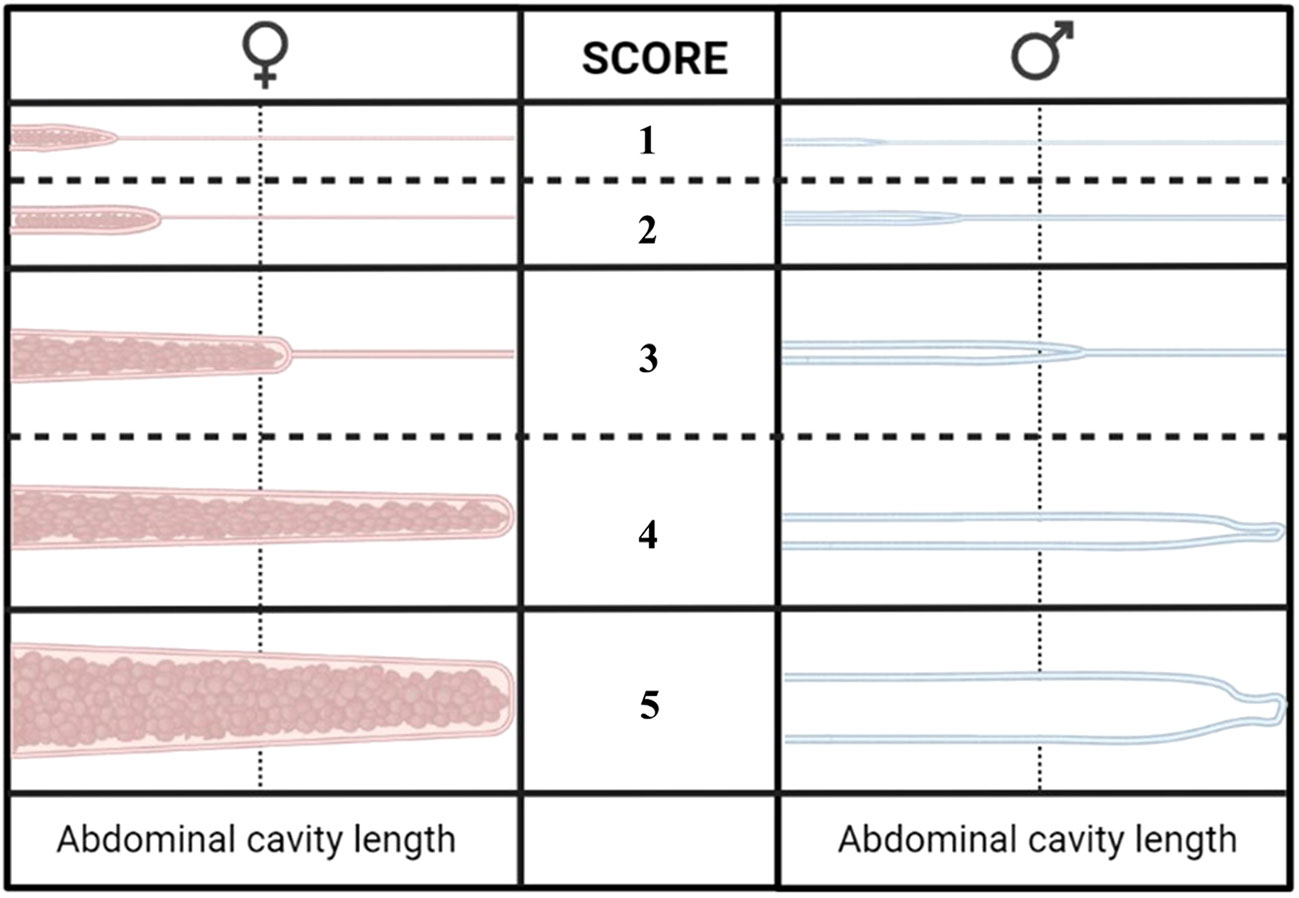
Figure 4 The scoring of gonadal development in Atlantic salmon with a score of 1–5. The scoring system was devised based on considerations of length and size of the gonads of salmonids, drawing from the categorization initially proposed by Dahl (1917) and later refined by Jonsson and Matzow (1979).
Female fish did not exhibit a gonadal score of > 2 and showed no discernible signs of maturation based on external or internal criteria. Therefore, the classification of mature individuals was exclusively carried out for male fish.
2.7 Calculations
The gonadosomatic index (GSI, %) was calculated as:
The condition factor (CF) was calculated as:
where W is fish weight in g, and L is fork length in cm.
2.8 Statistical analyses
The statistical analyses were conducted using SAS 9.4 (SAS Institute Inc., Cary, NC, USA) and GraphPad Prism 9.4.1. software. The Shapiro–Wilk W test, together with distribution and probability plots, was used to assess normality, and Levene’s test was used to assess homogeneity. As the data related to GSI and 11-KT were non-parametric, we used the Kruskal–Wallis test to examine the differences among the photoperiodic treatments (at the three levels, i.e., LL, SL, and DL) across the different sampling points. Due to the small number of fish and large variation in 11-KT levels at weeks and then tested. When the model was significant, Dunn’s post-hoc analysis was performed. To assess the relationship between the photoperiod and the prevalence of male fish with different gonad and skin spot scores, a multi-way contingency table chi-square analysis was carried out based on the count data (the results are presented as frequencies). A non-parametric Spearman correlation analysis was used to describe the association between two variables.
The effect of the pre-photoperiodic treatment (during weeks 0–18) on the incidence of sexual maturing and fully mature male fish at the end of the experiment (week 29) was assessed using the following linear mixed model with the PROC MIXED procedure in SAS:
where y is the recorded binary trait (1=mature, 0=immature), Di is the fixed effect of pre-photoperiodic treatment with three levels (i = 1, 2, and 3), tij is the random effect of the tank within the pre-photoperiodic treatment (j = 1, 2, 3), eijk is the random residual error of fish k within tank ij and , and thus a different residual variance for each of the three pre-photoperiodic treatments. The observed binary trait is binomial distributed, with p = frequency of mature fish, 1 − p = frequency of immature fish, and n = number of recorded fish. The binomial distribution approximated that of the standard normal distribution when the values for both np and n (1− p) were > 5 (Freund and Walpole, 1987).
The biometric data (BW and CF) were analyzed using one-way ANOVA, followed by Duncan’s multiple range test within each sampling point. The level of significance was set at p ≤ 0.05, and p< 0.1 was considered to indicate a trend. The results are presented as mean ± standard error of the mean (SEM) unless stated otherwise.
3 Results
3.1 Serum 11-KT concentration
The serum levels of 11-KT (Figure 5) in the LL group were elevated in certain individual male fish during weeks 8, 14, and 18. A similar trend was observed in the SL group at weeks 14 and 18, with several male fish exhibiting elevated 11-KT levels. In contrast, the same pattern was not observed with DL, as the male fish examined showed either no elevation or only a slight increase in 11-KT levels between the initial measurement and that taken at week 18. When all data were pooled for week, there was a trend toward an effect of the photoperiodic regime on 11-KT levels (Kruskal–Wallis: X2 = 5.4, df = 2, p = 0.066).
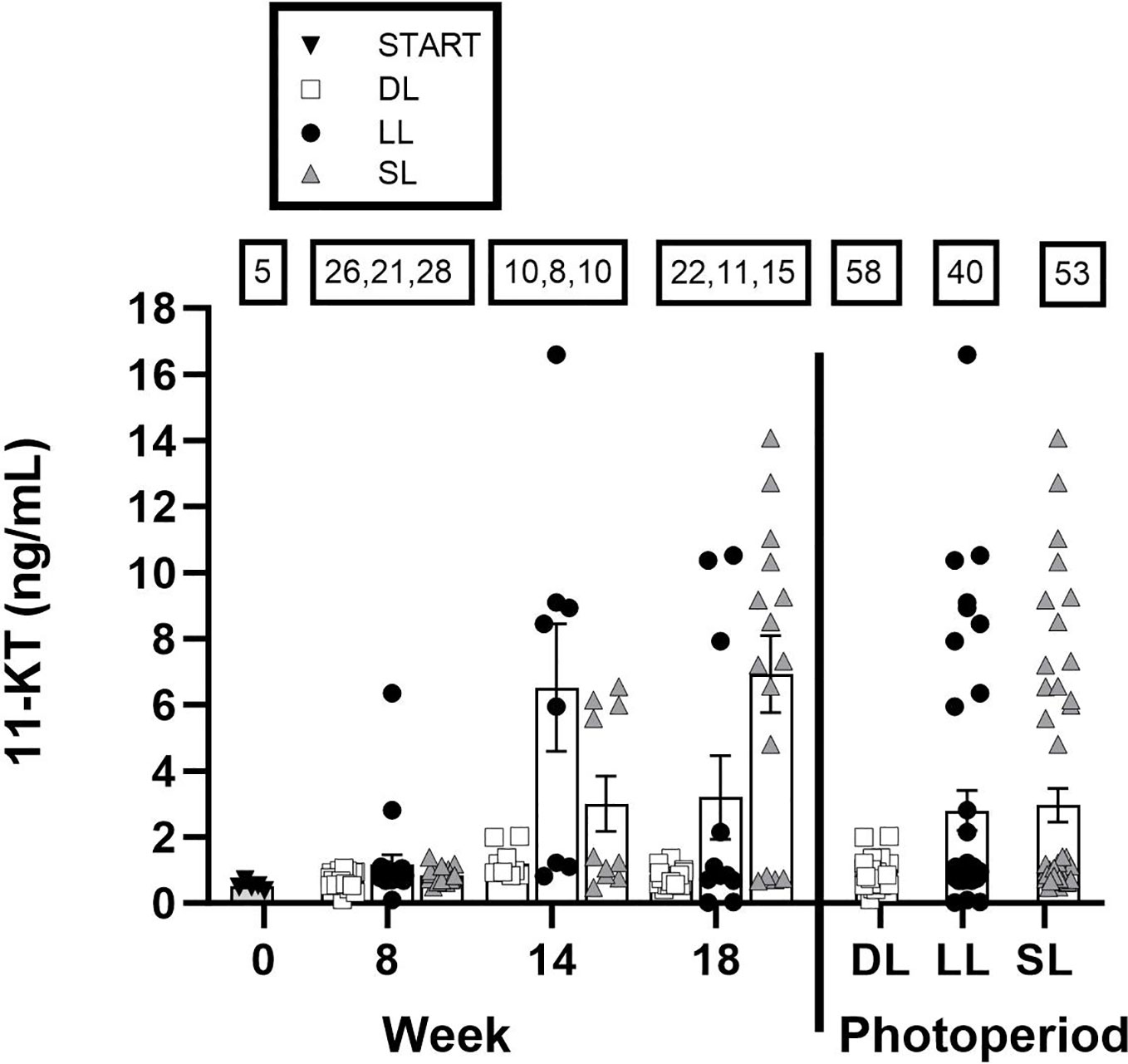
Figure 5 The concentration (ng/mL) of 11-ketotestosterone (11-KT) in the blood of Atlantic salmon male fish exposed to continuous light (LL), short-to-long daylength (SL), or decreasing daylength (DL) for 18 weeks in brackish water (20-ppt salinity). The numbers in squares denote the number of total measured male fish within each group at each timepoint, and the pooled number for all timepoints per treatment. The points represent individual measurements, and the bars show mean ± SEM.
3.2 Testes assessment and morphology between weeks 0 and 18
At week 8, only two fish within the LL group exhibited a high GSI and correspondingly elevated levels of 11-KT (Figure 5). One of these fish had a GSI of 0.24% and exhibited the presence of spermatogonia type B cells (SPB, corresponding developmental stage II), whereas the other fish had a GSI of 0.86% and a more mature testis with some spermatozoa germs cells (SZ, stage V). All other fish (n = 5 in each group) had only spermatogonia type A cells in the tissue, either undifferentiated or differentiated SPA cells, aligning with developmental stage I. The photoperiod regime had a significant effect on GSI at week 14 (Kruskal–Wallis: X2 = 6.8 df = 2, p = 0.03). At this sampling point, fish in the LL group had a significantly (p< 0.05) higher GSI than those in the SL and DL groups (Figure 6A). At week 18, all groups had male fish with an elevated GSI, and no significant effect of the photoperiod was detected (Figure 6B). At this sampling point, fish in the LL and DL groups had the highest GSI, whereas the SL group had the highest incidence of male fish exhibiting a general increase in GSI, especially compared with the DL group. At week 14, elevated 11-KT levels without increased GSI was observed within the SL group. However, the GSI at weeks 14 (Spearman’s correlation analysis results: r = 0.61, n = 28; p = 0.0006) and 18 (Spearman’s correlation analysis results: r = 0.67, n = 48; p< 0.0001) correlated with the 11-KT levels at these respective sampling points.
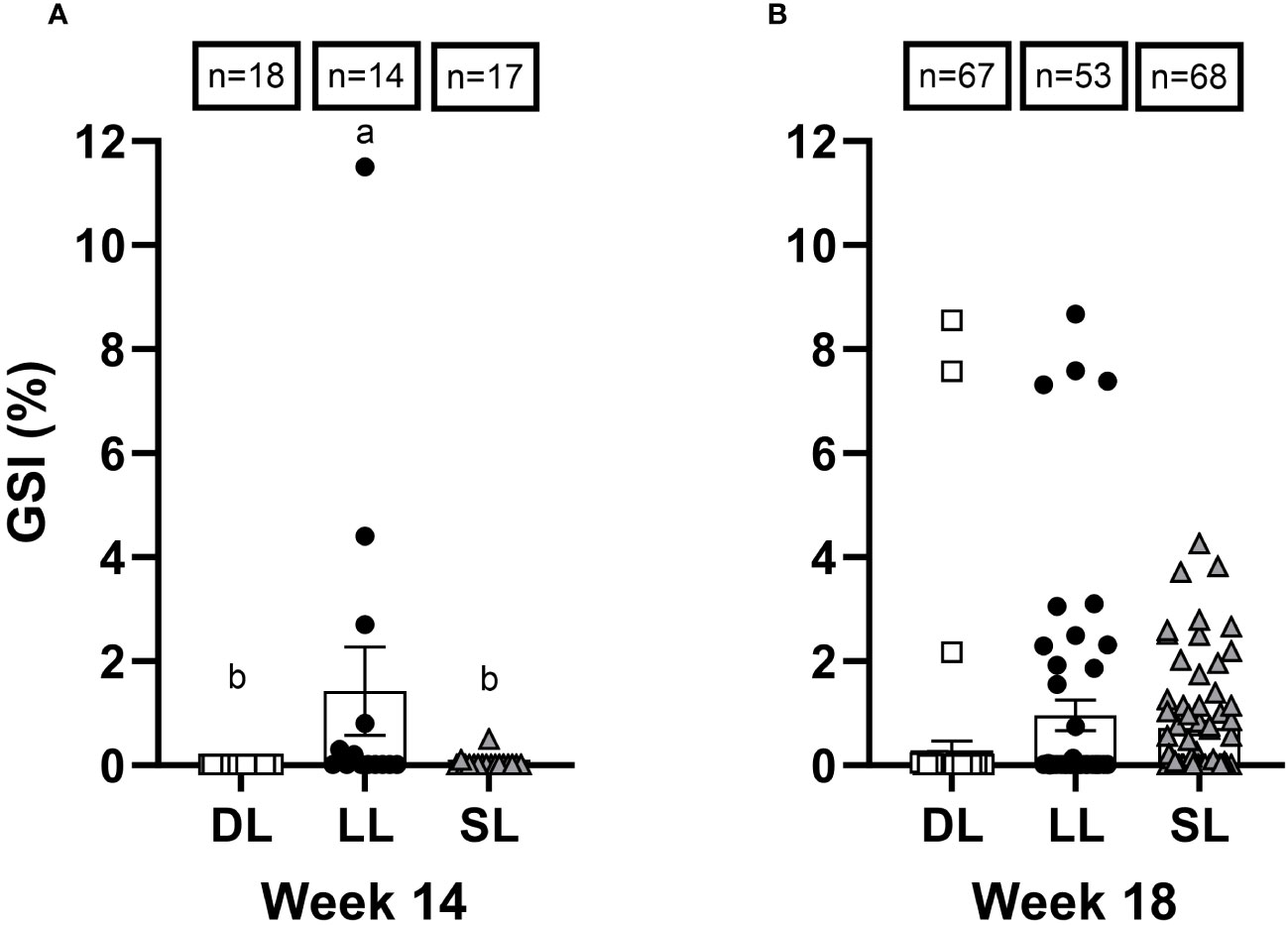
Figure 6 The gonadosomatic index (GSI, %) of Atlantic salmon male fish exposed to continuous light (LL), short-to-long daylength (SL), or decreasing daylength (DL) in brackish water (20-ppt salinity) at week 14 (A) and week 18 (B). The different letters denote the statistically significant differences among the groups. The total number of male fish analyzed in each group at each timepoint is indicated by “n.” The points represent individual measurements, and bars show mean ± SEM.
A contingency chi-square analysis examining gonad and skin spot scores revealed an overall relationship between the photoperiod and the prevalence of male fish with different gonad and skin spot scores at both week 14 (spot score; X2 = 19.8, df = 4, p = 0.001, gonad score; X2 = 18.5, df = 6, p = 0.005) and week 18 (spot score; X2 = 11.5, df = 6, p = 0.07, gonad score; X2 = 24.2, df = 8, p = 0.002). At week 14, the LL group exhibited a higher incidence of male fish with elevated gonad scores than the SL and DL groups (Figure 7A). Conversely, at week 18, the SL group had a higher prevalence of male fish with gonad scores of 2 and 3, surpassing those of the LL and DL groups. However, the SL group did not feature any fish with gonad scores of 4 or 5, whereas both the LL and DL groups had fish with these high scores. In particular, the LL group exhibited the highest frequency of fish with scores of 4 and 5 (Figure 7B). Thus, the distribution of gonad scores was highly correlated with GSI (Spearman’s correlation analysis results: r = 0.85, n = 192; p< 0.0001). The skin spot scores (Figures 7C, D) followed the same trend as gonad scores, and the two variables were also highly correlated (Spearman’s correlation analysis results: r = 0.63, n = 216; p< 0.0001). However, the SL group exhibited both the highest frequency of skin spots and the largest number of fish with high spot scores at week 18, in contrast to the gonad score (Figure 7D). Overall, the DL group had the lowest incidence of male fish with elevated gonad and skin spot scores.
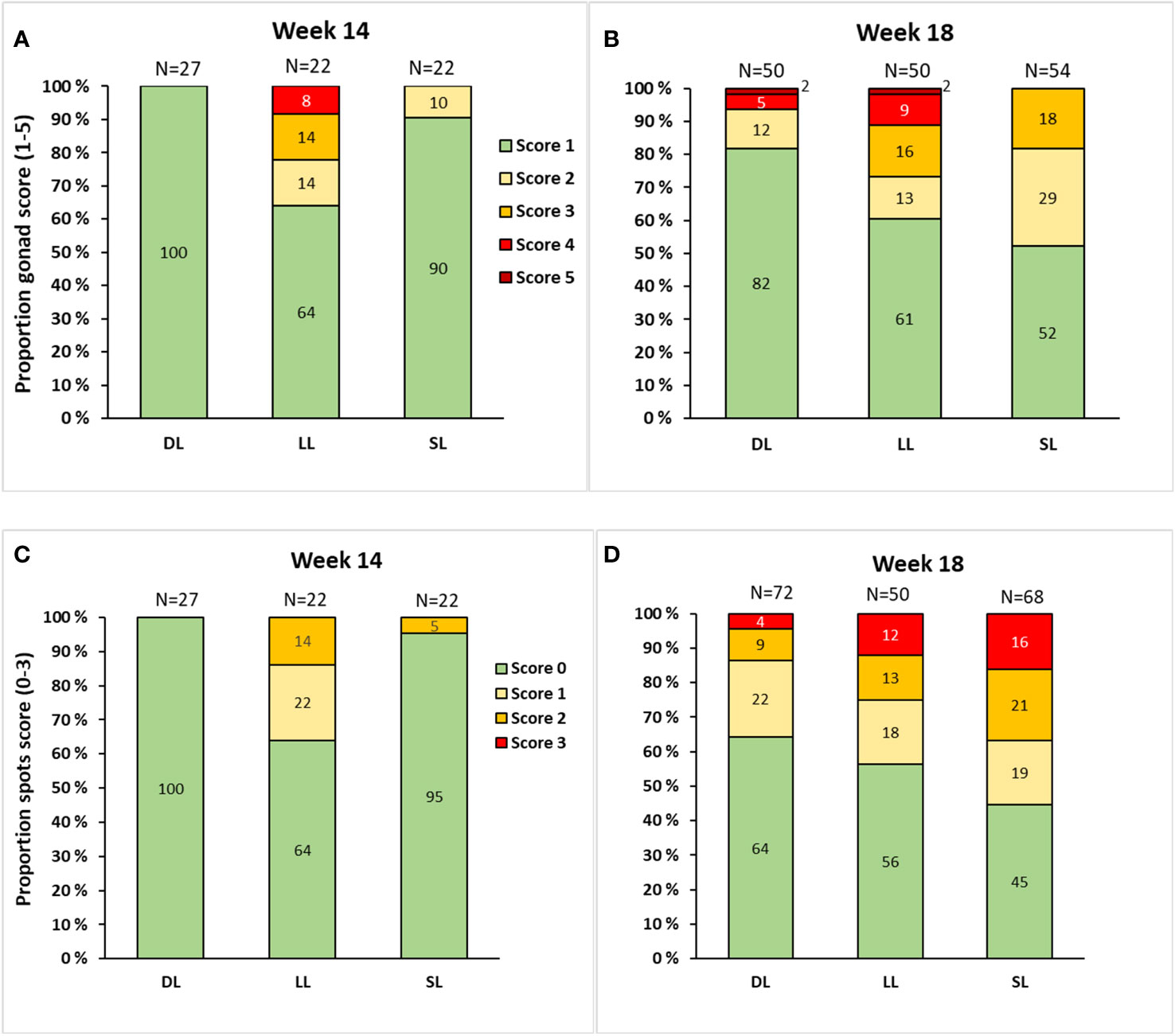
Figure 7 The proportion of male Atlantic salmon with different gonadal scores at week 14 (A) and week 18 (B) and spot scores at week 14 (C) and week 18 (D) after exposure to continuous light (LL), short-to-long daylength (SL), or decreasing daylength (DL) for 18 weeks in brackish water. The data are presented as percentage of the male population. The total number of male fish analyzed in each group is indicated by “n.”.
Table 1 presents the results of histologic assessments of the most advanced testes development stages in male fish at week 18, along with the corresponding GSI and 11-KT means (minimum–maximum). Notably, the two fish that were identified at stage V had a limited tissue coverage of SZ cells (i.e., of approximately 5%, indicating early-stage V). Thus, an overlap in GSI between development stages IV and V was detected. The 11-KT levels overlapped between stages III and IV, with stage III presenting the highest 11-KT levels.
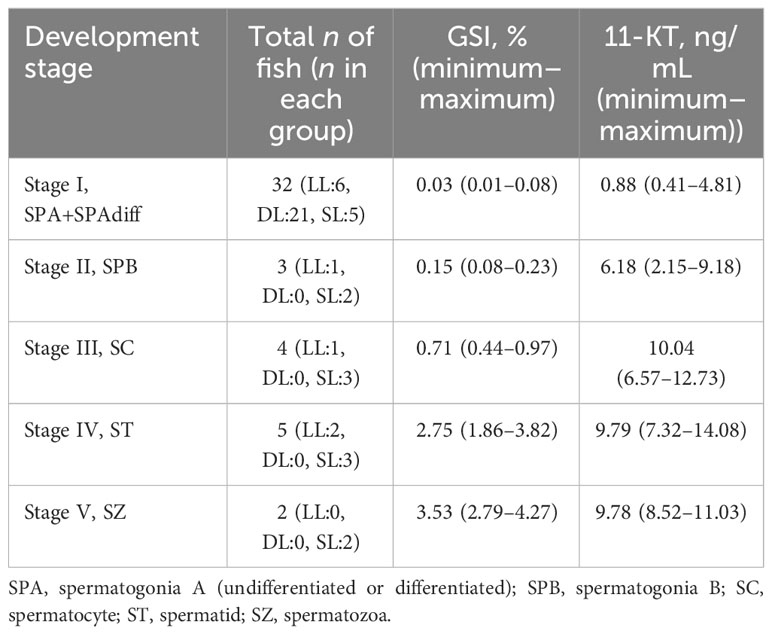
Table 1 A histologic assessment of the most-advanced testes development stages in male fish at week 18, along with the corresponding means (minimum–maximum) for the GSI and 11-KT levels.
3.3 Percentage of maturation and morphology at week 29
At the termination of the experiment at week 29, several male fish were fully mature and had progressed to a gonadal score of 4 and a corresponding skin spot, jaw, and color score of 3 (Figures 8A–D). The lowest GSI among these male fish was 1.64%. The contingency chi-square analysis indicated a significant overall relationship between the photoperiod and the prevalence of male fish with different gonad (X2 = 77.8, df = 8, p< 0.001), skin spot, (X2 = 61.2, df = 4, p< 0.001), jaw (X2 = 59.0, df = 2, p< 0.001), and color (X2 = 62.5, df = 4, p< 0.001) scores. During the final sampling, the SL group showed the largest number of male fish with elevated gonad and skin, jaw, and color scores, whereas those in the LL group had intermediate scores, and those in the DL group had the lowest scores. Notably, the skin, jaw, and color scores tended to align.
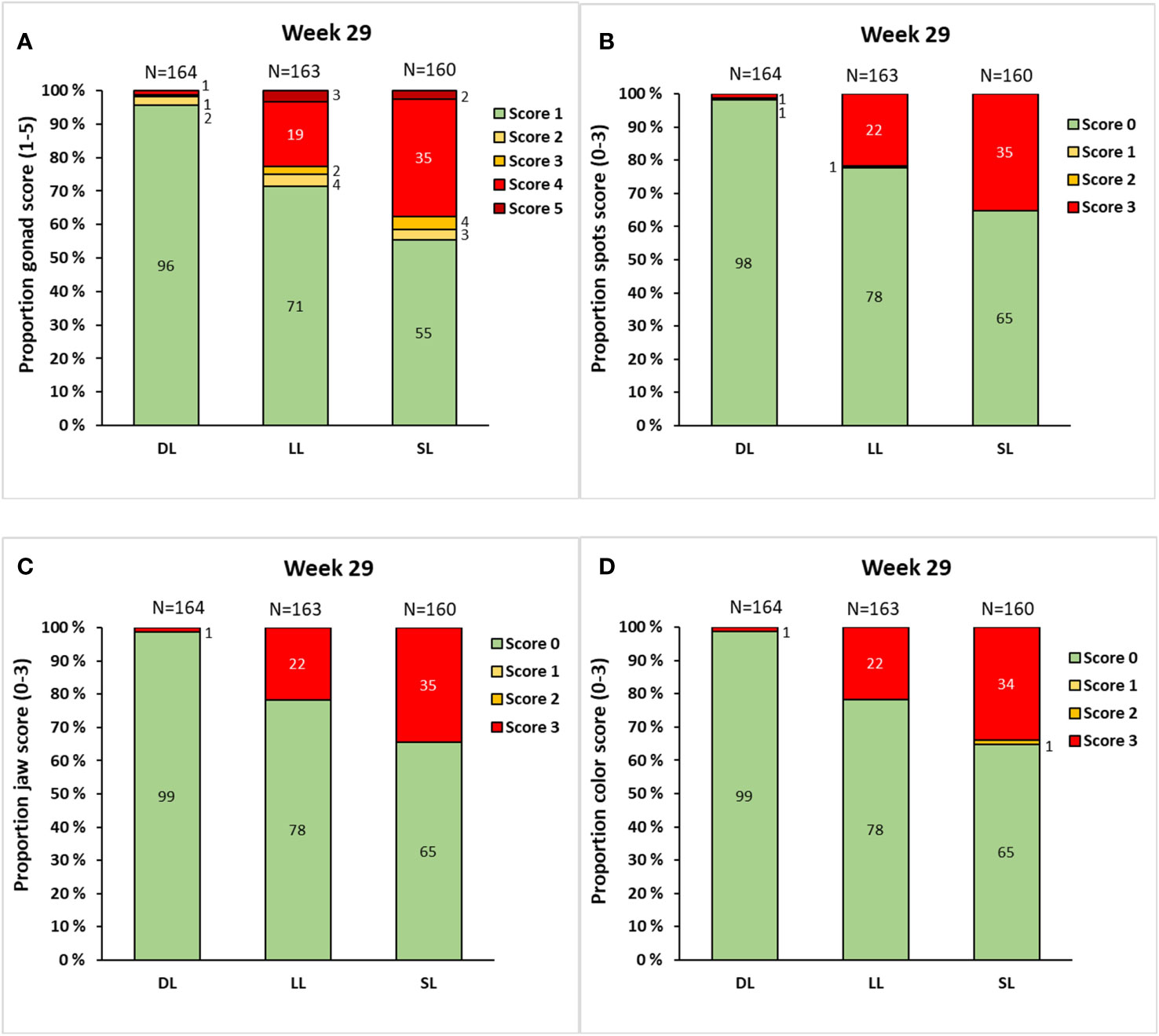
Figure 8 The proportion of male Atlantic salmon with a different gonadal score (A), spot score (B), jaw score (C), and color score (D) at week 29 after exposure to continuous light (LL), short-to-long daylength (SL), or decreasing daylength (DL) for 18 weeks in brackish water, followed by exposure to decreasing daylength for 11 weeks in one seawater RAS tank (weeks 19–29). Data are presented as percentage of the male population. The total number of male fish analyzed in each group is indicated by “n.”.
Significant photoperiod effects on the incidence of sexually maturing male fish (Figure 9A; type III test of fixed effects; F-value = 57.43, df = 2, p = 0.0001) and fully mature male fish (Figure 9C; type III test of fixed effects; F-value = 44.76, df = 2, p = 0.0002) were observed at termination. The SL group had the highest rates of maturing and fully mature male fish, whereas the LL group showed intermediate rates, and the DL group had the lowest rates. The average GSIs of sexually maturing male fish (Figure 9B) were 1.1% ± 1.09%, 3.35% ± 1.58%, and 3.11% ± 1.33% for the DL, LL, and SL groups, respectively (mean ± SD). For fully mature male fish, the average GSIs (Figure 9D) were 2.63% ± 0.29%, 3.96% ± 0.87%, and 3.68% ± 0.76% for the DL, LL, and SL groups, respectively (mean ± SD).
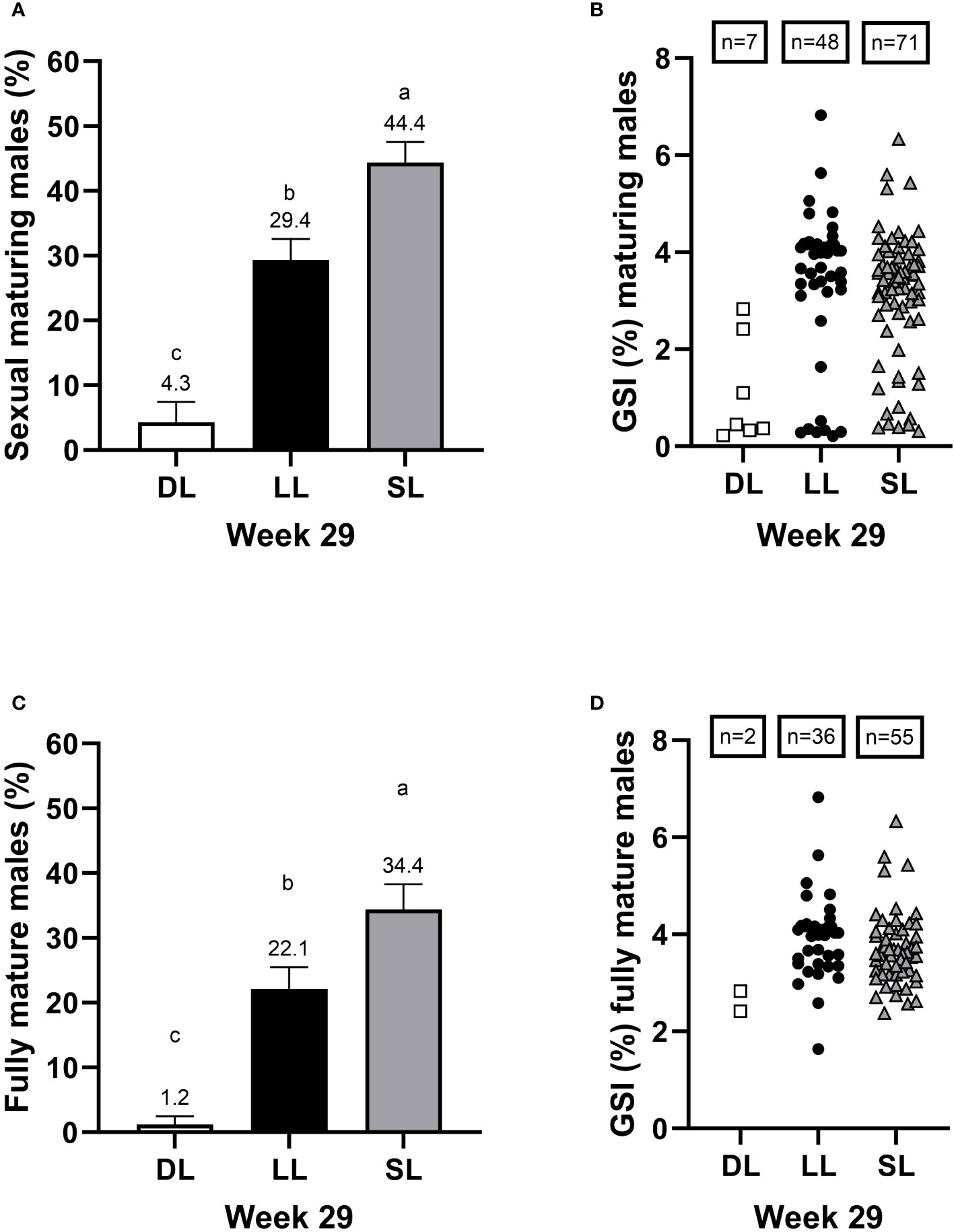
Figure 9 The proportion of male Atlantic salmon found to be maturing (A), with the corresponding GSI (%) of the maturing fish (B), proportion of fully mature males (C), with the corresponding GSI (%) of the fully mature males (D) at week 29 after exposure to continuous light (LL), short-to-long daylength (SL), or decreasing daylength (DL) for 18 weeks in brackish water, followed by exposure to decreasing daylength for 11 weeks in one seawater RAS tank (weeks 19–29). The data for maturing and fully mature fish are presented as percentages of the male population of the pre-photoperiodic treatment. The GSI (%) values represent all individuals within each group. The data regarding maturation are presented as mean ± SEM (n = 3, taking into account the differences in the residual variance within each treatment, see section 2.8). The different letters denote the statistically significant differences among the groups. The number of male fish in which GSI was measured within each group is indicated by “n.”.
3.4 Biometric development of maturing and immature male fish
By week 8, the average BW and CF of immature fish in the DL group were significantly lower than those of maturing SL and maturing LL fish, and the maturing LL fish had a higher CF than those in all other groups (Figures 10A, B). Between weeks 8 and 14, immature SL fish exhibited reduced growth performance, leading to their having a significantly poorer body condition than fish in all other groups between weeks 14 and 22 (Supplementary Figures 1A, B). In general, maturing fish in the SL and LL groups tended to have a numerically (and at some samplings a significantly) higher BW and CF during the initial 14 weeks than fish in the more immature groups. However, from week 14, fish in both the SL and LL groups showed decreasing growth performance and had a significantly lower BW and CF than immature male fish in all groups at the termination of the trial (Figures 10A, B, and Supplementary Figures 1A, B).
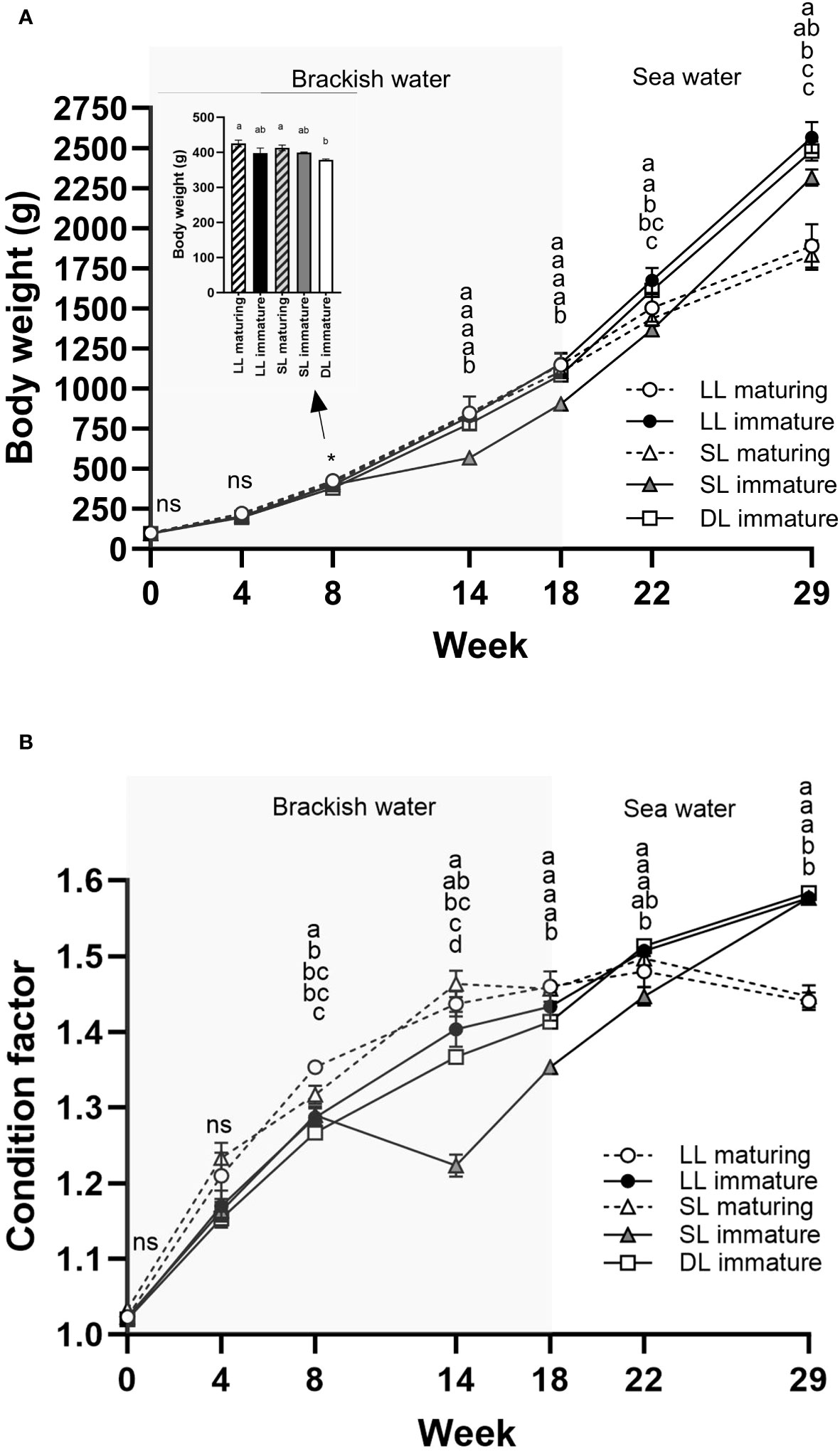
Figure 10 The changes in body weight (BW) (A) and condition factor (CF) (B) of maturing and immature Atlantic salmon male fish exposed to continuous daylength (LL), short-to-long daylength (SL), or decreasing daylength (DL) for 18 weeks in brackish water, followed by exposure to seawater and similar decreasing daylength for 11 weeks (weeks 19–29). The data are presented as mean ± SEM (n = 3). The different letters and * denote the statistically significant differences among the groups of maturing and immature male fish within each treatment group. ns expanded = not significant.
Sampled male fish with an elevated GSI at week 18 followed the same growth pattern from weeks 0–18 as male fish that were found to be mature at termination of the experiment within their respective groups (results not shown). Only a small number of fish, which exhibited a large variation in BW, within the DL group were found to be mature at termination. This was considered insufficient for a good comparison of the growth, BW, and CF of maturing and immature individuals within the DL group.
3.5 Whole-population biometric development
The photoperiod had a significant effect on BW at the group level (including female fish and maturing and immature male fish) throughout this study. The reduced growth of immature male fish and female fish in the SL group between weeks 8 and 14 resulted in it having a significantly lower average BW (in the group as a whole) from week 14 until termination than the LL and DL groups (Figure 11A). During the initial 4 weeks of the study, no differences in CF were observed among the groups (Figure 11B). However, at week 8, both the SL and LL groups exhibited a significantly higher CF than the DL group. Concurrently, during the period of reduced growth of SL fish between weeks 8 and 14, the CF stagnated. This led to a significantly lower CF in the SL group than in the LL and DL groups. This trend continued until week 22, whereas at week 29 no among-group differences in CF were observed (Figure 11B). For further information on feed intake and growth parameters, see Supplementary Table 2.
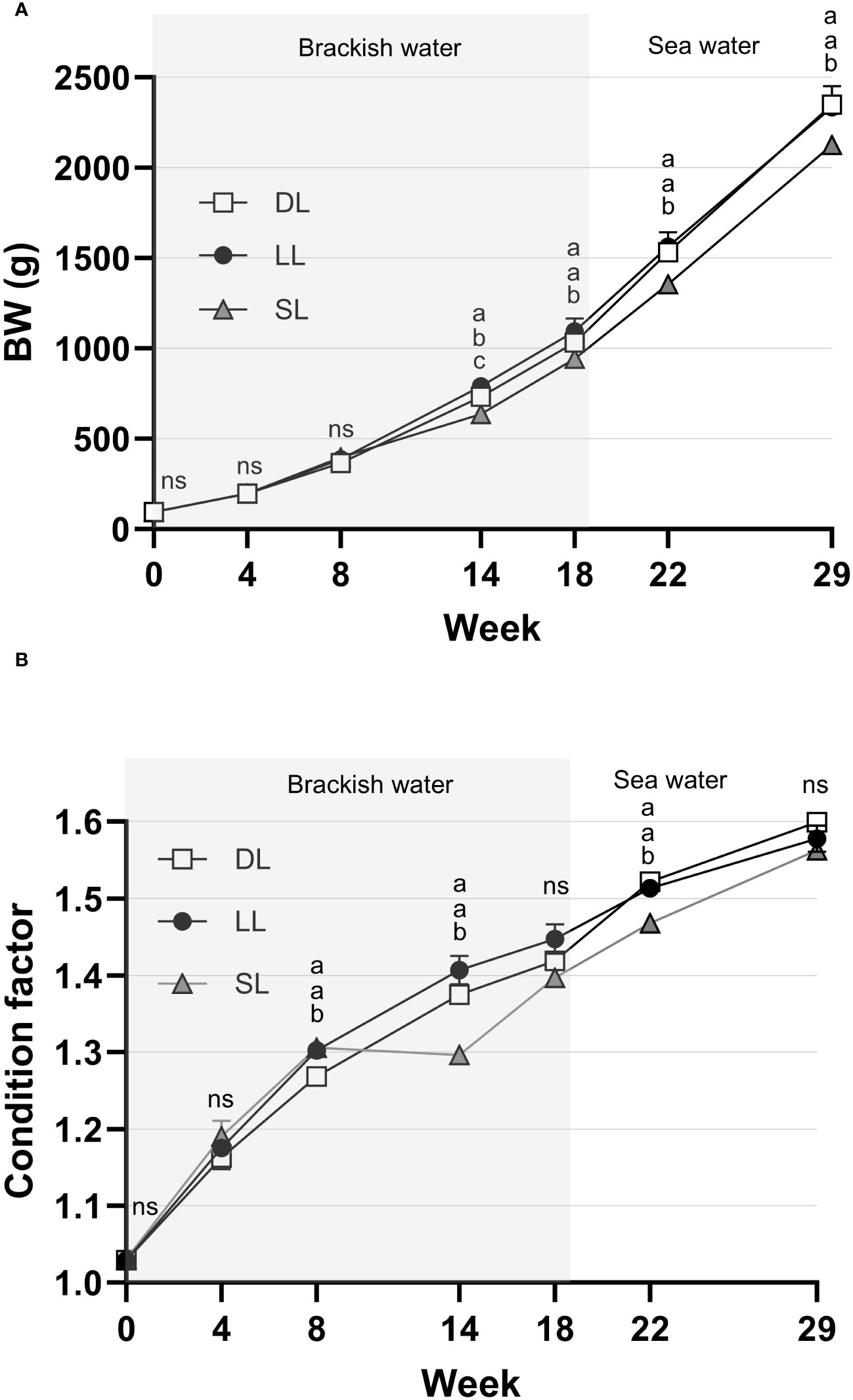
Figure 11 The changes in body weight (BW) (A) and condition factor (CF) (B) of the whole population (including maturing and immature male and female) Atlantic salmon exposed to continuous daylength (LL), short-to-long daylength (SL), or decreasing daylength (DL) for 18 weeks in brackish water, followed by exposure to seawater and similar decreasing daylength for 11 weeks (weeks 19–29). The data are presented as mean ± SEM (n = 3). The different letters denote the statistically significant among the groups. ns expanded = not significant.
4 Discussion
Gradually decreasing daylength or scotophase following smoltification induced by the “winter signal” significantly reduced unwanted maturation in male salmon under saline conditions compared with exposure to continuous light or short-to-long daylength. Importantly, this gradual reduction in daylength did not compromise body growth or adversely affect any of the recorded biometrics throughout the study. These findings provide valuable insights into the risks and consequences of photoperiod manipulation on sexual maturation, highlighting how different light manipulations could impact salmon farming practices.
Male fish exposed to a gradual decrease in daylight showed consistently lower levels of 11-KT and there were fewer individuals with elevated GSI in this group than in the groups exposed to continuous light or short-to-long daylength conditions from weeks 8 to 18. Fjelldal et al. (2011) and Fraser et al. (2023a) evaluated the use of continuous light versus 3- and/or 6-h scotophase during the latter stages of smoltification after exposure to an L:D cycle of 12 h:12 h. In these studies, the scotophases reduced the number of male fish exhibiting elevated 11-KT levels and GSI, along with corresponding lower incidences of puberty and precocious maturation. The low rate of maturing male fish (4.3%) at termination within the decreasing daylength group aligned with previous long-term studies by Duncan et al. (1999) and Qiu et al. (2015). Duncan et al. (1999) reported that approximately 25% and 2.5% of male fish reached advanced maturation stages after 12 months at ambient seawater temperature exposed to an L:D cycle of either 24 h:0 h or 9 h:15 h, respectively. However, an L:D cycle of 9 h:15 h led to lower specific growth rates and final body weights than an L:D cycle of 24 h:0 h. Qiu et al. (2015) noted a decrease in the male fish maturation rate with an L:D cycle of 8 h:16 h or 12 h:12 h compared with an L:D cycle of 24 h:0 h, resulting in male fish maturation rates of approximately 10%, 15%, and 36%, respectively. This was evident in salmon with a large initial size (850 g) reared for 6 months at 16°C in a RAS, which exhibited no adverse effects of the scotophases on individual growth rate or relative weight gain. Thus, the current and previously mentioned studies suggest that reducing the daylength, either during light-induced smoltification or afterward, can limit maturation in male Atlantic salmon. It is also important to note that environmental factors, particularly temperature and photoperiod, significantly affect the maturation rate (Fjelldal et al., 2011; Melo et al., 2014; Fjelldal et al., 2018; Fraser et al., 2023a; Pino Martinez et al., 2023a). Consequently, the prevalence of maturation within the same all-male salmon population can vary across different environments (Fraser et al., 2023b). The differences in maturity among studies are therefore often attributed to the conditions applied, in conjunction with factors such as genetics, age, size, sampling frequency, and study duration.
Although an increasingly consistent body of evidence indicates that scotophase can limit maturation in male fish (Duncan et al., 1999; Fjelldal et al., 2011; Qiu et al., 2015; Fraser et al., 2023a), few studies have examined the potential reasons for this effect. In the present study, the groups containing fish exposed to decreasing daylength had a smaller number of male fish exhibiting increased BW and CF from weeks 8–14 than the group containing fish kept under continuous or short-to-long daylength conditions. This might indicate a lower degree of pubertal initiation, as significantly elevated BW and CF are often observed once the decision to mature has been made (Rowe et al., 1991; Fraser et al., 2019; Fjelldal et al., 2020; Fraser et al., 2023a). The initiation of maturity is typically triggered by and/or associated with short-long day switching and/or continuous light conditions (Fjelldal et al., 2011). The short-to-long switch is thought to serve as a crucial cue, or zeitgeber, to optimize and synchronize the onset of maturation to ensure reproductive success (Bromage et al., 2001; Berrill et al., 2003; Berrill et al., 2006; Fjelldal et al., 2011). The commencement of maturation is believed to become feasible when energy reaches a sufficient level within a specific “critical time window”, and this timing is often linked to the spring, when there is a natural increase in daylength (Thorpe, 1994; Thorpe, 2007; Taranger et al., 2010). Thus, the transition from short-to-long day and/or long-day conditions may be associated with an “open gate” period that facilities the progression of maturation if the physiological threshold is met (Duston and Bromage, 1988; Thorpe, 1989). The transition from long-to-short daylength has been shown to advance spawning in maturing salmon (Taranger et al., 1991; Taranger et al., 1998; Duncan et al., 1999; Taranger et al., 1999), making it an important cue in the latter stages of maturity. Short-day conditions may be associated with a “closing or closed gate” period, during which only maturing fish advance their maturity before spawning. This period is not related to the onset or progression of maturation, potentially causing more fish to abort these processes. Supporting this line of agreement, some studies suggest that the direction of photoperiodic change, rather than the specific daylength, plays a crucial role in orchestrating sexual maturation and the seasonal timing of reproduction (Duston and Bromage, 1986; Bromage et al., 2001). However, we lack data to either support or reject these hypotheses. Nevertheless, decreasing daylength (or alternatively, increasing darkness) seems to limit rather than advance the onset of maturation. Notably, in contrast to studies by Fjelldal et al. (2011) and Fraser et al. (2023a), we induced scotophase after the completion of smoltification induced by the winter signal and not during the latter stages of this process. Thus, the winter signal may have already increased the likelihood of pubertal activation prior to exposure of different photoperiodic regimes. Although the factors and mechanisms involved in suppressing maturation by decreasing daylength remain unclear, future research could explore the brain–pituitary–gonad axis, along with hormonal regulation, to gain deeper insights into why the scotophase seems to limit maturation in male salmon.
Controlling the undesired maturation of male fish by reducing the daylength, without compromising BW, should be of significant interest to the land-based salmon farming industry, especially with regard to the production of post-smolts on land. However, prolonged darkness may affect feeding schedules, the efficiency of RAS biofilters, and operational routines. It is therefore important to explore different strategies, such as varying the decreases in daylength or ensuring consistent periods of darkness, to address these potential challenges. These aspects present compelling avenues for future research seeking to optimize photoperiodic regimes for diverse post-smolt production settings.
In the current study, exposure to continuous daylength led to a larger number of mature and fully mature fish than exposure to decreasing daylength. Several male fish exposed to continuous light conditions had increased 11-KT levels and GSI values across the samplings conducted between weeks 8 and 18, demonstrating variations in the onset of maturity. This was also confirmed by their exhibiting external signs of skin spots and early increases in gonadal scores compared with the other groups. These results suggest that continuous light triggers an unsynchronized “spontaneous” initiation of maturity, which has been referred to in previous studies as “free-running endogenous rhythm” (Duncan et al., 1999). Notably, continuous light exposure in the current study was used following a conventional smoltification process initiated by the “winter signal”, which could have predisposed male fish toward maturation via the short-to-long day switch. Thus, results obtained using the continuous light regime cannot be directly compared with those from studies involving no exposure to darkness. Research has shown that this “spontaneous” initiation of maturity is highly dependent on temperature (Pino Martinez et al., 2023a), and that elevated water temperatures and continuous light promote early maturation (Fjelldal et al., 2011; Imsland et al., 2014; Melo et al., 2014; Fjelldal et al., 2018; Pino Martinez et al., 2023a). A variety of factors can lead to the spontaneous onset of maturity, such as the genetic variations related to maturity within the same salmon population (Ayllon et al., 2015; Fjelldal et al., 2020), the individual variations in the physiological threshold levels for triggering maturation (Rowe and Thorpe, 1990; Jonsson and Jonsson, 2007; McClure et al., 2007), and the potential interactions among these factors under different environmental conditions. A comprehensive discussion and elucidation of these factors and their potential impact on the “spontaneous” initiation of maturity can be found in the work by Pino Martinez et al. (2023a), and we refer to this paper for a concise summary and additional references.
Among the male fish exposed to the short-to-long day photoperiod, some fish showed an increase in 11-KT levels without a simultaneous increase in GSI at week 14. Notably, at this stage, maturing male fish exposed to this photoperiod had a significantly higher CF than immature male fish. By week 18, both the 11-KT levels and GSI were elevated in multiple male fish. This finding was consistent with the observations reported by Fraser et al. (2023a), who noted a similar increase in 11-KT levels preceding an increase in the GSI in pubertal male fish. This may indicate that exposure to the short-to-long daylength condition leads to a more synchronized initiation of maturity than exposure to continuous light. However, hormone levels and GSI were not measured between weeks 0 and 8; therefore, whether the same developmental pattern (related to an increase in 11-KT levels preceding the increase in GSI) occurs in male fish exposed to continuous light from an earlier stage could not be determined. The transition from short to long days is considered a primary driver in the initiation of maturation in salmon with sufficient energy levels (Thorpe, 1994; Bromage et al., 2001; Taranger et al., 2010). Previous studies reported an increased incidence of post-smolt maturation in salmon exposed to a square-wave photoperiod (i.e., > 12 h day, to an LD cycle of 12 h:12 h, and then to an L:D cycle of 24 h:0 h), particularly when using larger fish and elevated water temperatures (Fjelldal et al., 2011; Fjelldal et al., 2018). Maturation and smoltification can occur successively, simultaneously, or mutually exclusively depending on the environmental conditions and life-history strategy (Berrill et al., 2003; Fjelldal et al., 2011; Fjelldal et al., 2018; Pino Martinez et al., 2023a; Pino Martinez et al., 2023b). The SL group was exposed to two successive transitions from long-to-short daylength switches within a brief timeframe. Consequently, separating the potential impacts of each of these sequential cues was not possible. Nevertheless, it is probable that the second shift from long-to-short daylength advanced maturation, given that the parr–smolt transformation had been completed and that the commencement of maturation was likely highly prioritized. Taking this into account, our results appear to confirm the role of photoperiod as a zeitgeber for maturation, with the highest activation of male fish maturation observed at week 18 and at termination (44%) for salmon exposed to a short-to-long daylength (Bromage et al., 2001; Taranger et al., 2010; Fjelldal et al., 2011; Fraser et al., 2023a).
As previously mentioned, the transition from long-to-short daylength is known to advance spawning in maturing farmed Atlantic salmon (Taranger et al., 1991; Taranger et al., 1998; Duncan et al., 1999; Taranger et al., 1999). Following seawater exposure, all fish were subjected to simulated autumn conditions with a decreasing photoperiod and kept in one large RAS tank. Consequently, the prevalence of fully maturing fish at the termination of the study might have been affected by this transition. This change could have prompted maturing male fish to prepare for spawning, yet it cannot be ruled out that it may have also terminated the maturation process in other fish. To explore this possibility, a control tank exposed to continuous light during the latter part of the experiment should have been employed. Limiting the exposure to only decreasing daylength during the later stages also restricted our ability to explore the potential effects of subjecting fish previously exposed to decreasing daylength to continuous or increasing daylength during seawater exposure. If the male fish initially kept under decreasing daylength are exposed to increasing daylight upon transfer to seawater, whether in commercial sea cages or land-based facilities, the likelihood of reaching maturation could be increased. This is particularly probable when a short-to-long daylength switch occurs at a higher fish weight, a condition known to promote maturation. However, if the transfer occurs at low temperatures, typically during early spring conditions, the maturation rate might be limited. The temperature was identified as the primary factor affecting the early maturation of male fish, as highlighted by Fjelldal et al. (2011) and Pino Martinez et al. (2023a); Pino Martinez et al. (2023b).
Various studies have proposed threshold 11-KT levels (1 ng/mL–2 ng/mL) and GSI values (0.05%–0.1%) to evaluate pubertal initiation among male salmon (Peterson and Harmon, 2005; Melo et al., 2014; Good et al., 2017; Ciani et al., 2021; Pino Martinez et al., 2023a). In the current study, the histologic evaluation was unfortunately limited to weeks 8 and 18, and included only a small number of male fish (n = 58). Furthermore, the immunohistochemistry techniques commonly used in other studies were not employed in the present study to assess proliferation of germ and Sertoli cells (Melo et al., 2014; Crespo et al., 2022; Fraser et al., 2023a). Consequently, this study lacks the necessary data and precision to precisely pinpoint the initiation of puberty within the various photoperiod groups. Thus, pubertal determination was not included or assessed in the current study. However, based on the histologic evaluations conducted, a GSI of 0.08% represented the lowest relative testis weight, corresponding to testis development stage II (presence of SPB cells) and indicating active spermatogenesis (Ciani et al., 2021). This GSI aligns with an 11-KT serum level of 2.15 ng/mL, both of which appear to be at the higher end of the suggested threshold values for pubertal activation mentioned in previous studies. The discrepancies in these values can naturally be attributed to differences in the timing of evaluation, BW, genetic background, and environmental conditions across the various studies. The present histologic evaluation was conducted on fish with a relatively high BW (i.e., 400 g–1,000 g) and over a longer sampling interval (i.e., every 4–6 weeks) compared with other studies with a greater focus on puberty (Melo et al., 2014; Ciani et al., 2021; Fraser et al., 2023a; Pino Martinez et al., 2023a). In the study by Fraser et al. (2023a), pubertal activation was meticulously examined (using phosphorylated histone H3 staining) by evaluating fish weighing between 103 g and 333 g through weekly samplings and using bimodality to identify pubertal fish. The pubertal male fish were initially heavier than the immature male fish, and both BW and CF increased significantly once maturation was initiated, in line with previous studies (Rowe et al., 1991; Fraser et al., 2019; Fjelldal et al., 2020). In the current study, we were unable to investigate the effect of initial body size on early maturation due to the experimental setup and the absence of weight differences at the beginning. Due to the chosen sampling interval (4–6 weeks) and the insufficient number of fish sampled for combined analyses of histology, blood serum parameters, and GSI, we were unable to investigate bimodality to identify the initiation of puberty in the same manner as Fraser et al. (2023a). Moreover, during the assessment of maturing male fish at termination, the number of male fish that had initiated maturation could have been underestimated. A gonadal development score of 2 was established as the threshold for maturing male fish, corresponding to a minimum GSI of 0.21%. Consequently, we might have overlooked additional fish that had initiated maturation, exhibiting a GSI of< 0.21%.
In the present study, all groups of fish were fed according to their respective daylength during the initial 18 weeks. The reason for this experimental design was to ensure an applied approach, such that the possible advantages and disadvantages of the different light treatments could be fully exploited and overcome, respectively. A supposed advantage of exposure to continuous light was the ability to feed every hour of the day. Furthermore, the differences in feeding schedules might have introduced a new uncontrolled factor of variability. However, all fish were fed 5%–10% over their estimated daily feed intake, regardless of daylength, to ensure ad libitum feeding conditions. Furthermore, the feed intake of fish exposed to short-to-long daylength during the initial 8 weeks was significantly higher than that of fish in both the continuous and decreasing daylength groups, despite the shorter daylength and feeding time. In addition, although the DL group was subjected to a consistent decrease in daylength and feeding time over the initial 18 weeks, while the LL group was fed every hour of the day, no consistent differences were found between these groups in terms of fish growth or mean BW at week 18, suggesting that photoperiod is the decisive factor. In addition, several studies found that differences in feeding intensity have negligible effects on daily feed intake, growth rate, and size (Jørgensen and Jobling, 1992; Juell et al., 1994).
During the maturation process, Atlantic salmon undergo significant biological, chemical, organoleptic, and morphological changes, especially in the later stages prior to spawning, when feeding ceases and both body condition and energy reserves are substantially reduced (Aksnes et al., 1986; Kadri et al., 1996; Mobley et al., 2021). As a result, an anticipated bimodal pattern of internal and external changes emerges. Upon termination of the present study, this bimodality was evident in the gonadal score, skin spots, skin color, jaw development, and CF. Most maturing male fish were advanced to fully mature, exhibiting marked external changes and reduced body condition, characteristic of the grilsing stage. In 2021 and 2022, sexual maturation indicated by these undesirable external alterations, emerged as a primary reason for downgrading at slaughter. (Sommerset et al., 2022). Gonadal development and skin changes require energy and pigments, which are primarily stored in muscle tissue. In the advanced stages of maturation, these pigments are redirected to the skin, leading to diminished muscle pigmentation and reduced fillet quality (Bjerkeng et al., 1992; Leclercq et al., 2010). During the latter phase of the experiment in the present study, all maturing male fish exhibited a significant reduction in their growth performance and the development of their body condition. This trend was consistent with findings reported by Kadri et al. (1996); Rowe et al. (1991), and Fraser et al. (2019), in which maturing salmon stopped feeding and utilized substantial nutrients and internal fat reserves for gonadal growth and external transformations. These trends pose significant economic risks to the aquaculture industry, as they could lead to financial losses and reduced the utilization of permitted biomass.
Morphological scoring and gonad development were highly correlated. Thus, scoring may be utilized as a practical, non-lethal, and minimally invasive measure for the identification of maturing fish. Interestingly, the skin spot score appeared to be a promising indicator of early maturation in post-smolt fish in the current study. Nevertheless, these traits can vary among genetic families and are affected by the specific environmental rearing conditions. Melanin-based skin spots are linked to both behavioral and physiological stress responses in rainbow trout and Atlantic salmon (Kittilsen et al., 2009). Colored pigments are widely utilized in the animal kingdom to signify quality and attract potential mates. Wedekind et al. (2008) demonstrated that in brown trout, high offspring viability was associated with darker pigmentation in sires, suggesting that darker male fish produced more viable offspring. Additionally, studies have shown that melanophore spot patterns enable long-term individual recognition in Atlantic salmon (Stien et al., 2017). Consequently, various morphological changes present the opportunity to leverage artificial intelligence for potentially predicting the likelihood of maturation or identifying mature fish during the production phase.
5 Conclusion
In the present study, maturation in smoltified male salmon induced by the “winter signal” was increased under continuous and short-to-long daylength conditions. Conversely, gradually decreasing the daylength significantly limited the maturation of male fish. Although the mechanism underlying this phenomenon remains unclear and requires further investigation, this could be relevant for the industry in the context of producing larger post-smolt fish without compromising body size development.
Data availability statement
The original contributions presented in the study are included in the article/Supplementary Material. Further inquiries can be directed to the corresponding author.
Ethics statement
Ethical approval was not required for the study involving animals in accordance with the local legislation and institutional requirements because The experiment was done at Nofima Research Station for sustainable aquaculture in Sunndalsøra, Norway. These research facilities are licensed under Norwegian law to do experimental work on fish according to regulation FOR-2015-06-18-761 “Forskrift om bruk av dyr i forsøk” and is a national adaption of (and in compliance with) the “Directive 2010/63/EU of the European Parliament and of the Council on the protection of animals used for scientific purposes”. In the trial, fish were subject to differentiated treatments regarding light regimes which were comparable to procedures used in commercial production and which were not expected to have adverse effects on their welfare. The experimental plan was pre-evaluated by the person responsible for overseeing compliance with the animal welfare legislation at the site and found to contain no procedures or treatments which required a specific license. All samplings were done on euthanized fish. Thus, the activities were covered by the exemption given in the abovementioned regulation, §2 article f. Here it is stated that a specific license is not required if the experimental treatments are not expected to cause any adverse effects on animals. The experimental diets contained ingredients that are used in commercial salmon feeds and were formulated to meet the NRC nutritional recommendations for salmonid fish (National Research Council, 2011). Tagging of fish in the experiment was done under the site’s general license for tagging, FOTS SL 29627 (mattilsynet.no).
Author contributions
VS, J-ED and K-AR: planning and preparing experiment. Feed production: HJ and RW. VS, J-ED, K-AR, EB, VM, LS, MB and TY: participated in carrying out experiment. VS, J-ED, K-AR, EB, VM, LS, TY, MB, GA and O-MF: analysis. VS, J-ED, K-AR, EB, VM, LS, MB, TY, HJ and RW: writing and editing. All authors contributed to the article and approved the submitted version.
Funding
The author(s) declare financial support was received for the research, authorship, and/or publication of this article. This work was supported by Nofima and carried out as an integrated part of the research conducted under Nofima’s R&D licenses granted by the Norwegian Directorate of Fisheries for largescale industrial research. This work was also partly funded by The Research Council of Norway, Strategic Institute Research Nofima AS, project nr 12878 “Atlantic salmon biology in focus: for a robust fish in a shifting aquaculture industry”.
Acknowledgments
The authors acknowledge the skillful assistance and dedicated fish management of the technicians at Sunndalsøra, with special thanks to Britt Kristin Megård Reiten, Kjellrun Hoås Gannestad, Sissel Nergaard, and Yuriy Marchenko. The authors are grateful to Sietske S. Grijseels and the Proteomics and Metabolomics Core Facility (PRiME, Arctic University of Norway) for assistance with steroid analyses. Gunhild Seljehaug Johansson is acknowledged for input on, and evaluation of, the histologic analyses.
Conflict of interest
The authors declare that the research was conducted in the absence of any commercial or financial relationships that could be construed as a potential conflict of interest.
Publisher’s note
All claims expressed in this article are solely those of the authors and do not necessarily represent those of their affiliated organizations, or those of the publisher, the editors and the reviewers. Any product that may be evaluated in this article, or claim that may be made by its manufacturer, is not guaranteed or endorsed by the publisher.
Supplementary material
The Supplementary Material for this article can be found online at: https://www.frontiersin.org/articles/10.3389/faquc.2023.1235584/full#supplementary-material
References
Aksnes A., Gjerde B., Roald S. O. (1986). Biological, chemical and organoleptic changes during maturation of farmed Atlantic salmon, Salmo salar. Aquaculture 53, 7–20. doi: 10.1016/0044–8486(86)90295–4
Alne H., Thomassen M. S., Sigholt T., Berge R. K., Rørvik K. A. (2009). Reduced sexual maturation in male post-smolt 1+ Atlantic salmon (Salmo salar L.) by dietary tetradecylthioacetic acid. Aquac. Res. 40, 533–541. doi: 10.1111/J.1365–2109.2008.02125.X
Anderson E., Borg B., Lambert J. G. (1988). Aromatase activity in brain and pituitary immature and mature Atlantic salmon (Salvo salar L.) parr. Gen. Comp. Endocrinol. 72, 394–401. doi: 10.1016/0016-6480(88)90161-x
Ayllon F., Kjærner-Semb E., Furmanek T., Wennevik V., Solberg M. F., Dahle G., et al. (2015). The vgll3 locus controls age at maturity in wild and domesticated Atlantic salmon (Salmo salar L.) males. PloS Genet. 11, e1005628. doi: 10.1371/JOURNAL.PGEN.1005628
Berglund I., Åntonopoulou E., Mayer I., Borg B. (1995). Stimulatory and inhibitory effects of testosterone on testes in Atlantic salmon male parr. J. Fish Biol. 47, 586–598. doi: 10.1111/j.1095–8649.1995.tb01925.x
Berrill I. K., Porter M. J. R., Smart A., Mitchell D., Bromage N. R. (2003). Photoperiodic effects on precocious maturation, growth and smoltification in Atlantic salmon, Salmo salar. Aquaculture 222, 239–252. doi: 10.1016/S0044–8486(03)00125-X
Berrill I. K., Smart A., Porter M. J. R., Bromage N. R. (2006). A decrease in photoperiod shortly after first feeding influences the development of Atlantic salmon (Salmo salar). Aquaculture 254, 625–636. doi: 10.1016/J.AQUACULTURE.2005.10.036
Bjerkeng B., Storebakken T., Liaaen-Jensen S. (1992). Pigmentation of rainbow trout from start feeding to sexual maturation. Aquaculture 108, 333–346. doi: 10.1016/0044–8486(92)90117–4
Bowerbank S. L., Carlin M. G., Dean J. R. (2019). A direct comparison of liquid chromatography-mass spectrometry with clinical routine testing immunoassay methods for the detection and quantification of thyroid hormones in blood serum. Anal. Bioanal. Chem. 411, 2839–2853. doi: 10.1007/s00216–019–01724–2
Bromage N., Porter M., Randall C. (2001). The environmental regulation of maturation in farmed finfish with special reference to the role of photoperiod and melatonin. Aquaculture 197, 63–98. doi: 10.1016/S0044–8486(01)00583-X
Ciani E., von Krogh K., Nourizadeh-Lillabadi R., Mayer I., Fontaine R., Weltzien F. A. (2021). Sexual maturation in Atlantic salmon male parr may be triggered both in late summer and early spring under standard farming conditions. Aquaculture 544, 737086. doi: 10.1016/J.AQUACULTURE.2021.737086
Clarke W. C., Saunders R. L., McCormick S. D. (1996). Smolt production. ((red.) b). In: Pennell, W., Barton, B.A. (Eds.), Principles of Salmonid Culture. 29. Elsevier, Amsterdam, The Netherlands, pp. 517–567. doi: 10.1016/S0167-9309(96)80011-1
Crespo D., Skaftnesmo K. O., Kjærner-Semb E., Yilmaz O., Norberg B., Olausson S., et al. (2022). Pituitary gonadotropin gene expression during induced onset of postsmolt maturation in male Atlantic salmon: in vivo and tissue culture studies. Front. Endocrinol. (Lausanne) 13. doi: 10.3389/fendo.2022.826920
Duncan N., Mitchell D., Bromage N. (1999). Post-smolt growth and maturation of out-of-season 0+ Atlantic salmon (Salmo salar) rared under different photoperiods. Aquaculture 177, 61–71. doi: 10.1016/S0044–8486(99)00069–1
Duston J., Bromage N. (1986). Photoperiodic mechanisms and rhythms of reproduction in the female rainbow trout. Fish Physiol. Biochem. 2, 35–51. doi: 10.1007/BF02264072
Duston J., Bromage N. (1988). The entrainment and gating of the endogenous circannual rhythm of reproduction in the female rainbow trout (Salmo gairdneri). J. Comp. Physiol. 164, 259–268. doi: 10.1007/BF00603956
Fjelldal P. G., Hansen T., Huang T. (2011). Continuous light and elevated temperature can trigger maturation both during and immediately after smoltification in male Atlantic salmon (Salmo salar). Aquaculture 321, 93–100. doi: 10.1016/j.aquaculture.2011.08.017
Fjelldal P. G., Hansen T. J., Wargelius A., Ayllon F., Glover K. A., Schulz R. W., et al. (2020). Development of supermale and all-male Atlantic salmon to research the vgll3 allele—puberty link. BMC Genet. 21, 1–13. doi: 10.1186/S12863–020–00927–2/FIGURES/4
Fjelldal P. G., Schulz R., Nilsen T. O., Andersson E., Norberg B., Hansen T. J. (2018). Sexual maturation and smoltification in domesticated Atlantic salmon (Salmo salar L.)—is there a developmental conflict? Physiol. Rep. 6, e13809. doi: 10.14814/phy2.13809
Fossmark R. O., Attramadal K. J. K., Nordøy K., Østerhus S. W., Vadstein O. (2021). A comparison of two seawater adaptation strategies for Atlantic salmon post-smolt (Salmo salar) grown in recirculating aquaculture systems (RAS): nitrification, water and gut microbiota, and performance of fish. Aquaculture 532, 735973. doi: 10.1016/J.AQUACULTURE.2020.735973
Fraser T. W. K., Fjelldal P. G., Schulz R. W., Norberg B., Hansen T. J. (2019). Termination of puberty in out-of-season male Atlantic salmon smolts. Comp. Biochem. Physiol. A Mol. Integr. Physiol. 232, 60–66. doi: 10.1016/j.cbpa.2019.03.011
Fraser T. W. K., Hansen T. J., Fjelldal P. G. (2023b). Environmental and genetic (vgll3) effects on the prevalence of male maturation phenotypes in domesticated Atlantic salmon. Fishes 8, 275. doi: 10.3390/fishes8050275
Fraser T. W. K., Hansen T. J., Norberg B., Nilsen T. O., Schulz R. W., Fjelldal P. G. (2023a). Atlantic salmon male post-smolt maturation can be reduced by using a 3-hour scotophase when inducing smoltification. Aquaculture 562, 738772. doi: 10.1016/J.AQUACULTURE.2022.738772
Freund J. E., Walpole R. E. (1987). Mathematical statistics. 4th ed. (Englewood Cliffs, NJ: Prentice-Hall, Inc).
Good C., Davidson J., Iwanowicz L., Meyer M., Dietze J., Kolpin D. W., et al. (2017). Investigating the influence of nitrate nitrogen on post-smolt Atlantic salmon Salmo salar reproductive physiology in freshwater recirculation aquaculture systems. Aquac Eng. 78, 2–8. doi: 10.1016/j.aquaeng.2016.09.003
Good C., Weber G. M., May T., Davidson J., Summerfelt S. (2016). Reduced photoperiod (18 h light vs. 24 h light) during first-year rearing associated with increased early male maturation in Atlantic salmon Salmo salar cultured in a freshwater recirculation aquaculture system. Aquac. Res. 47, 3023–3027. doi: 10.1111/are.12741
Gullestad P., Bjørgo S., Eithun I., Ervik A., Gudding R., Hansen H., et al. (2011). Effective and sustainable use of areas for aquaculture – land of desire (in Norwegian). Report from an expert panel appointed by Ministry of Fisheries and Coastal Affairs 198 (Oslo, Norway: Ministry of Trade, Industry and Fisheries).
Helland S. J., Grisdale-Helland B., Nerland S. (1996). A simple method for the measurement of daily feed intake of groups of fish in tanks. Aquaculture 139, 157–163. doi: 10.1016/0044–8486(95)01145–5
Hunt S. M. V., Simpson T. H., Wright R. S. (1982). Seasonal changes in the levels of 11-oxotestosterone and testosterone in the serum of male salmon, Salmo salar L., and their relationship to growth and maturation cycle. J. Fish Biol. 20, 105–119. doi: 10.1111/j.1095–8649.1982.tb03899.x
Idler D. R., Horne D. A., Sangalang G. B. (1971). Identification and quantification of the major androgens in testicular and peripheral plasma of Atlantic salmon (Salmo salar) during sexual maturation. Gen. Comp. Endocrinol. 16, 257–267. doi: 10.1016/0016–6480(71)90037–2
Ikegawa K., Hasegawa Y. (2023). Adrenal gland involvement in 11-ketotestosterone production analyzed using LC-MS/MS. Front. Endocrinol. (Lausanne) 14. doi: 10.3389/fendo.2023.1051195
Imsland A. K., Handeland S. O., Stefansson S. O. (2014). Photoperiod and temperature effects on growth and maturation of pre- and post-smolt Atlantic salmon. Aquacult. Int. 22, 1331–1345. doi: 10.1007/s10499–014–9750–1
Jonsson B., Matzow D. (1979). Fisk I Vann og vassdrag: Om økologien Til Aure, Røyr og Laks (Oslo: Aschehoug).
Jonsson N., Jonsson B. (2007). Sea growth, smolt age and age at sexual maturation in Atlantic salmon. J. Fish Biol. 71, 245–252. doi: 10.1111/J.1095–8649.2007.01488.X
Jørgensen E. H., Jobling M. (1992). Feeding behavior and effect of feeding regime on growth of Atlantic salmon, Salmo salar. Aquaculture 101, 135–146. doi: 10.1016/0044–8486(92)90238-G
Juell J. E., Bjordal Å., Fernö A., Huse I. (1994). Effect of feeding intensity on food intake and growth of Atlantic salmon, Salmo salar L., in sea cages. Aquacult. Res. 25, 453–464. doi: 10.1111/J.1365–2109.1994.TB00710.X
Kadri S., Mitchell D. F., Metcalfe N. B., Huntingford F. A., Thorpe J. E. (1996). Differential patterns of feeding and resource accumulation in maturing and immature Atlantic salmon, Salmo salar. Aquaculture 142, 245–257. doi: 10.1016/0044–8486(96)01258–6
Kittilsen S., Schjolden J., Beitnes-Johansen I., Shaw J. C., Pottinger T. G., Sørensen C., et al. (2009). Melanin-based skin spots reflect stress responsiveness in salmonid fish. Horm. Behav. 56, 292–298. doi: 10.1016/J.YHBEH.2009.06.006
Leclercq E., Dick J. R., Taylor J. F., Bell J. G., Hunter D., Migaud H. (2010). Seasonal variations in skin pigmentation and flesh quality of Atlantic salmon (Salmo salar L.): implications for quality management. J. Agric. Food Chem. 58, 7036–7045. doi: 10.1021/JF100723B
Maugars G., Schmitz M. (2008). Expression of gonadotropin and gonadotropin receptor genes during early sexual maturation in male Atlantic salmon parr. Mol. Reprod. Dev. 75, 403–413. doi: 10.1002/MRD.20767
McClure C. A., Hammell K. L., Moore M., Dohoo I. R., Burnley H. (2007). Risk factors for early sexual maturation in Atlantic salmon in seawater farms in New Brunswick and Nova Scotia, Canada. Aquaculture 272, 370–379. doi: 10.1016/J.AQUACULTURE.2007.08.039
Melo M. C., Andersson E., Fjelldal P. G., Bogerd J., França L. R., Taranger G. L., et al. (2014). Salinity and photoperiod modulate pubertal development in Atlantic salmon (Salmo salar). J. Endocrinol. 220, 319–332. doi: 10.1530/JOE-13–0240
Mobley K. B., Aykanat T., Czorlich Y., House A., Kurko J., Miettinen A., et al. (2021). Maturation in Atlantic salmon (Salmo salar, Salmonidae): a synthesis of ecological, genetic, and molecular processes. Rev. Fish Biol. Fish. 31, 523–571. doi: 10.1007/S11160–021–09656-W
Mortensen A., Damsgård B. (1998). The effect of salinity on desmoltification in Atlantic salmon. Aquaculture 168, 407–411. doi: 10.1016/S0044–8486(98)00366–4
Nouri M. Z., Kroll K. J., Webb M., Denslow N. D. (2020). Quantification of steroid hormones in low volume plasma and tissue homogenates of fish using LC-MS/MS. Gen. Comp. Endocrinol. 296, 113543. doi: 10.1016/J.YGCEN.2020.113543
Papadaki M., Mandalakis M., Anastasiou T. I., Pouli M., Asderis M., Katharios P., et al. (2021). Histologic evaluation of sex differentiation and early sex identification in hatchery-produced greater amberjack (Seriola dumerili) reared in sea cages. Fish Physiol. Biochem. 47, 1777–1792. doi: 10.1007/s10695–021–01007–7
Peterson R. H., Harmon P. R. (2005). Changes in condition factor and gonadosomatic index in maturing and non-maturing Atlantic salmon (Salmo salar L.) in Bay of Fundy sea cages, and the effectiveness of photoperiod manipulation in reducing early maturation. Aquac. Res. 36, 882–889. doi: 10.1111/J.1365–2109.2005.01297.X
Pino Martinez E., Balseiro P., Fleming M. S., Stefansson S. O., Norberg B., Imsland A. K. D., et al. (2023a). Interaction of temperature and photoperiod on male postsmolt maturation of Atlantic salmon (Salmo salar L.). Aquaculture 568, 739325. doi: 10.1016/J.AQUACULTURE.2023.739325
Pino Martinez E., Balseiro P., Stefansson S. O., Kaneko N., Norberg B., Fleming M. S., et al. (2023b). Interaction of temperature and feed ration on male postsmolt maturation of Atlantic salmon (Salmo salar L.). Aquaculture 562, 738877. doi: 10.1016/J.AQUACULTURE.2022.738877
Qiu D., Xu S., Song C., Chi L., Li X., Sun G., et al. (2015). Effects of spectral composition, photoperiod and light intensity on the gonadal development of Atlantic salmon Salmo salar in recirculating aquaculture systems (RAS). Chin. J. Oceanol. Limnol. 33, 45–56. doi: 10.1007/s00343–015–4011–3
Rege J., Nakamura Y., Satoh F., Morimoto R., Kennedy M. R., Layman L. C., et al. (2013). Liquid chromatography–tandem mass spectrometry analysis of human adrenal vein 19-carbon steroids before and after ACTH stimulation. J. Clin. Endocrinol. Metab. 98, 1182–1188. doi: 10.1210/JC.2012–2912
Roalkvam I., Drønen K., Dahle H., Wergeland H. I. (2021). A case study of biofilter activation and microbial nitrification in a marine recirculation aquaculture system for rearing Atlantic salmon (Salmo salar L.). Aquac. Res. 52, 94–104. doi: 10.1111/ARE.14872
Rowe D. K., Thorpe J. E. (1990). Suppression of maturation in male Atlantic salmon (Salmo salar L.) parr by reduction in feeding and growth during spring months. Aquaculture 86, 291–313. doi: 10.1016/0044–8486(90)90121–3
Rowe D. K., Thorpe J. E., Shanks A. M. (1991). Role of fat stores in the maturation of male Atlantic salmon (Salmo salar) parr. Can. J. Fish. Aquat. Sci. 48, 405–413. doi: 10.1139/f91–052
Schulz R. W., de França L. R., Lareyre J. J., Le Gac F., Chiarini-Garcia H., Nobrega R. H., et al. (2010). Spermatogenesis in fish. Gen. Comp. Endocrinol. 165, 390–411. doi: 10.1016/J.YGCEN.2009.02.013
Sommerset I., Walde C. S., Bang Jensen B., Wiik-Nielsen J., Bornø G., Oliveira V. H. S., et al. (2022). "Norwegian Fish Health Report 2021", in Norwegian Veterinary Institute Report, series #2a/2022. (Norwegian Veterinary Institute). Available at: www.vetinst.no.
Statistics Norway (2022) Akvakulturstatistikk: matfiskproduksjon av laks, regnbueørret og ørret (Fiskeridirektoratet). Available at: https://www.fiskeridir.no/Akvakultur/Tall-og-analyse/Akvakulturstatistikk-tidsserier/Laks-regnbueoerret-og-oerret/Matfiskproduksjon (Accessed January 31, 2023).
Stefansson S. O., Hansen T. J., Taranger G. L. (1993). Growth and parr-smolt transformation of Atlantic salmon (Salmo salar L.) under different light intensities and subsequent survival and growth in seawater. Aquac. Eng. 12, 231–243. doi: 10.1016/0144–8609(93)90014–3
Stien L. H., Nilsson J., Bui S., Fosseidengen J. E., Kristiansen T. S., Øverli Ø., et al. (2017). Consistent melanophore spot patterns allow long-term individual recognition of Atlantic salmon Salmo salar. J. Fish Biol. 91, 1699–1712. doi: 10.1111/JFB.13491
Taranger G. L., Carrillo M., Schulz R. W., Fontaine P., Zanuy S., Felip A., et al. (2010). Control of puberty in farmed fish. Gen. Comp. Endocrinol. 165, 483–515. doi: 10.1016/J.YGCEN.2009.05.004
Taranger G. L., Haux C., Hansen T., Stefansson S. O., Björnsson B. T., Walther B. T., et al. (1999). Mechanisms underlying photoperiodic effects on age at sexual maturity in Atlantic salmon, Salmo salar. Aquaculture 177, 47–60. doi: 10.1016/S0044–8486(99)00068-X
Taranger G. L., Haux C., Stefansson S. O., Björn Thrandur Björnsson W., Walther B. T., Hansen T. (1998). Abrupt changes in photoperiod affect age at maturity, timing of ovulation and plasma testosterone and oestradiol-17β profiles in Atlantic salmon, Salmo salar. Aquaculture 162, 85–98. doi: 10.1016/S0044–8486(98)00168–9
Taranger G. L., Haux C., Walther B. T., Stefansson S. O., Bjornsson B. T., Hansen T. (1991) Photoperiodic control of growth, incidence of sexual maturation and ovulation in adult Atlantic salmon (Accessed 13.10.2023).
Thorpe J. E. (1989). Developmental variation in salmonid populations. J. Fish Biol. 35, 295–303. doi: 10.1111/J.1095–8649.1989.TB03073.X
Thorpe J. E. (1994). Reproductive strategies in Atlantic salmon, Salmo salar L. Aquac Res. Aquaculture Res. 25, 77–87. doi: 10.1111/J.1365–2109.1994.TB00668.X
Thorpe J. E. (2007). Maturation responses of salmonids to changing developmental opportunities. Mar. Ecol. Prog. Ser. 335, 285–288. doi: 10.3354/meps335285
Thrush M. A., Duncan N. J., Bromage N. R. (1994). The use of photoperiod in the production of out-of-season Atlantic salmon (Salmo salar) smolts. Aquaculture 121, 29–44. doi: 10.1016/0044–8486(94)90005–1
Wedekind C., Jacob A., Evanno G., Nusslé S., Müller R. (2008). Viability of brown trout embryos positively linked to melanin-based but negatively to carotenoid-based colors of their fathers. Proc. Biol. Sci. 275, 1737–1744. doi: 10.1098/RSPB.2008.0072
Wolters W. R. (2010). Sources of phenotypic and genetic variation for seawater growth in five North American Atlantic salmon, Salmo salar, stocks. J. World Aquac Soc 41, 421–429. doi: 10.1111/J.1749–7345.2010.00383.X
Keywords: Salmo salar, sexual maturation, photoperiod, hormone, 11-KT, scotophase, brackish water, growth
Citation: Skjold V, Rørvik K-A, Sveen L, Burgerhout E, Mota VC, Weihe R, Ytrestøyl T, Bou M, Jacobsen HJ, Allaoui G, Fuskevåg O-M and Dessen J-E (2024) Gradually decreasing daylength after smoltification induced by “winter signal“ reduced sexual maturation in male Atlantic salmon. Front. Aquac. 2:1235584. doi: 10.3389/faquc.2023.1235584
Received: 06 June 2023; Accepted: 12 December 2023;
Published: 16 January 2024.
Edited by:
Iciar Martinez, University of the Basque Country, SpainReviewed by:
Thomas Fraser, Norwegian Institute of Marine Research (IMR), NorwayEnrique Pino Martinez, University of Bergen, Norway
Copyright © 2024 Skjold, Rørvik, Sveen, Burgerhout, Mota, Weihe, Ytrestøyl, Bou, Jacobsen, Allaoui, Fuskevåg and Dessen. This is an open-access article distributed under the terms of the Creative Commons Attribution License (CC BY). The use, distribution or reproduction in other forums is permitted, provided the original author(s) and the copyright owner(s) are credited and that the original publication in this journal is cited, in accordance with accepted academic practice. No use, distribution or reproduction is permitted which does not comply with these terms.
*Correspondence: Vetle Skjold, vetle.skjold@nofima.no
†Present address: Vasco C. Mota, Department of Mechanical Engineering and Technology Management, Norwegian University of Life Sciences, Ås, Norway