- Aquaculture Program, Lab. de Fisiología Comparada y Genómica Funcional, Centro de Investigaciones Biológicas del Noroeste, S.C., La Paz, Baja California Sur, Mexico
The proportion of fats or oils in natural or artificial feed is generally 6%–30%, since this is essential to cover fish’s energetic and structural requirements. Therefore, studies of the ontogeny or response of lipase activity to food treatments are widespread. A systematic review of articles published over 5 years (2016–2020) on lipase activity in fish in aquaculture was carried out; however, this was taken only as a representative example. Any 5-year period between 201-2022 would have shown similar results in terms of the actual lipase method used. As a result of this review, it was found that the methods used by the authors are very varied and have significant differences in terms of the type of substrate, substrate concentration, bile salt type and concentration, pH, temperature, incubation time, measurement of hydrolysis products, and definition of lipase units. The above does not mean that comparison of these studies is of no value, but that it is significantly limited. The most used methods (with p-nitrophenyl derivates, β-naphthyl derivates, and emulsified natural oils as substrate) can be reviewed to determine the most appropriate standard curves or the corresponding molar extinction coefficient for defining the lipase units. Standardizing current lipase analytical procedures should improve the reliability of comparative studies of aquaculture fish species.
1 The importance of digestive lipase activity
In fish, fertilized eggs contain oil droplets. Lipases hydrolyze the lipid content to obtain an energy reserve for embryo development. Even after hatching, the fish larva may still contain traces of the lipid globule, which is later absorbed during its development (Bik et al., 2020). Feeds used in fish aquaculture generally contain up to 20% lipids. Lipids are also present in natural fish food, including phospholipids, sterols, triglycerides, waxes, and free fatty acids, all of which are characterized by high levels of polyunsaturated fatty acids (Bakke et al., 2011). However, the main functional aspects of fish digestive lipases have yet to be deeply studied (Nolasco et al., 2011).
There are two types of lipases in mammals and fish: pancreatic lipase (pl gene) and lipase activated by bile salts (bsal gene). However, pancreatic lipase is the most important for mammals, and lipase activated by bile salts is the most important for fish (Tang et al., 2022). The fish pancreas synthesizes and secretes lipases into the intestine, which generally function at an alkaline pH, and hydrolyzes lipid food substrates to release the fatty acids absorbed by intestinal enterocytes (Bakke et al., 2011; Rønnestad et al., 2013). The pH in the fish intestine lumen ranges from 6 to 9 (Chakrabarti et al., 1995; Solovyev and Izvekova, 2016). In general, the optimum pH reported for fish lipases is alkaline (i.e., 7 to 9) (Mukundan et al., 1985; Gjellesvik et al., 1992; Iijima et al., 1997; Iijima et al., 1998; Nayak et al., 2004; Kurtovic et al., 2010; Nolasco et al., 2011; Xiong et al., 2011; Görgün and Akpınar, 2012; González-Félix et al., 2018). There are no conclusive data regarding the lipase requirements of Na+ or Ca+2 due to the small number of studies on this topic. Mullet (Mugil cephalus) lipase was not activated by Ca+2 (Aryee et al., 2007); Sparus aurata lipase has an optimum salinity at 50 mM NaCl and was not shown to be activated by Ca+2 or other divalent ions (chloride salts of Mg+2, Mn+2, Fe+2, Co+2, Cu+2, Hg+2, Pb+2, and Zn+2) that were inhibitors (Nolasco et al., 2011). In contrast, a phospholipase A2 from the pyloric ceca of red sea bream, Pagrus major, was activated by 8 mM of Ca+2 (Iijima et al., 1997); salmon (Oncorhynchus tshawytscha) lipase and hoki (Macruronus novaezelandiae) lipase were activated by ions (Kurtovic et al., 2010); and stingray (Dasyatis pastinaca) lipase increased its activity at 0.5 mM Ca+2 (Bouchaâla et al., 2015). The adjustment in the method should also consider the physiological ion concentration of the fish intestine (Nolasco-Soria, 2020).
Micelle formation enormously increases the fraction of lipid molecules accessible to the action of water-soluble lipases in the intestine. Lipase hydrolysis is valid for lipid substrates forming micelles with bile salts, i.e., phospholipids, galactolipids, monoglycerides, and lipolytic enzymes showing a preference for this type of lipid aggregates. Regarding the position of the lipase relative to the emulsified substrate, it is thought that the active site of the lipase is oriented toward the water–oil interface (Brockerhoff, 1973). The gallbladder of vertebrates secretes bile acids or their conjugated forms (bile salts). They collaborate in the action of lipases acting as emulsifiers of their substrates. Taurocholate and taurochenodeoxycholate are the principal bile salts of teleost fish (Une et al., 1991; Alam et al., 2001; Nolasco et al., 2011). In in vitro studies, chenodeoxycholyl-L-cysteinolic acid and taurochenodeoxycholate (12 mM concentration) were better activators for red sea bream pyloric ceca phospholipase (Iijima et al., 1997). Salmon lipase was better activated by sodium taurocholate (10 mM), and hoki lipase was equally activated by sodium cholate and sodium taurocholate (5, 10 mM) (Kurtovic et al., 2010). Red sea bream lipase was better activated by sodium taurocholate and sodium cholate, but was not activated by sodium deoxycholate (Iijima et al., 1998), similar to mullet (Mugil cephalus) lipase (Aryee et al., 2007). Stingray lipase increases its activity at 1 mM sodium taurodeoxycholate, but a concentration higher than 4 mM inhibits lipase activity (Bouchaâla et al., 2015).
Reviews on digestive physiology in larval fish report that lipase activity has been found during all stages of ontogenic development (Rønnestad et al., 2013; Yúfera et al., 2018). For example, in green catfish (Mystus nemurus) larvae, lipase activity showed a basal activity level from day 1 to day 25, and subsequently an increase in lipase activity between 30–40 dph (Srichanun et al., 2012). In leopard groupers (Mycteroperca rosacea) larvae, lipase activity was detected before mouth opening and during feeding, with a significant peak at 30 dph (Martínez-Lagos et al., 2014). In Chinese perch (Siniperca chuatsi) larvae, a low lipase activity was detected before the mouth opened. In contrast to other carnivorous fish, lipase activity did not increase suddenly after the first feeding, but gradually increased until 15 dph, then suddenly decreased at 25 dph, and continued declining until 30 dph (Tang et al., 2021).
Lipids are common nutrient substrates for farmed fish, and lipases are secreted in the intestine for their digestion. Subsequently, fish lipases can be used as enzymatic reagents to determine the in vitro digestibility of food oils (according to the methodology of Espinosa-Chaurand and Nolasco-Soria, 2019) to select the most digestible ones and allow the possible formulation of alternative diets for aquaculture.
According to Yúfera et al. (2018), the most commonly used substrates to measure lipase activity are p-nitrophenyl myristate (p-NPM), β-naphthyl caprylate (β-NC) (colorimetric methods, based on the color generated by releasing p-nitrophenol (p-NP) or β-naphthol (β-N), respectively), and 4-methylumbelliferyl (MU) substrates (MU-butyrate, MU-heptanoate or MU-oleate) (fluorometric methods, based on the fluorescence generated by releasing of 4-methylumbelliferone, MU). Emulsions of water-insoluble short-chain triacylglycerols (e.g., tributyrin) or long-chain triacylglycerols (e.g., triolein) are used as lipase substrates by titration of the released protons with NaOH, or by calculating the moles of NaOH required per unit of time to maintain a constant pH during the lipase hydrolysis of tryglyceride ester bonds.
The methods used by the authors differ in the type of substrate used. Therefore, the hydrolysis products generated (what is measured) are different, making it necessary to use the standard curves or the specific molar extinction coefficients (MEC) to measure the hydrolyzed ester bonds per unit of time. If the measurements are made at different pHs, temperatures, ion concentrations, or types or concentrations of bile salt, this will directly affect the lipase activity. If the definitions of the lipase unit differ, the comparison of results becomes more complex (Yúfera et al., 2018). Considering the above, the objective of this study was to review the authors’ methods to identify their differences and their effects on the quantification of lipase activity, in addition to carrying out experimental analyses to evaluate some of the most critical methodological points for quantifying lipase activity, to propose more ad hoc strategies closer to fish physiology, and lipase unit calculation tools for studies in fish in aquaculture.
2 Lipase determination
2.1 Fish lipase activity
Lipase methods were explored with a Web of Science Core Collection™ (www.webofknowledge.com; Thomson Reuters™) bibliographic search using the keywords “lipase AND fish”, resulting in a total of 936 published articles from 2016 to 2020 (5 years) (Supplementary Material A contains a complete list of reviewed and excluded articles) being found. After removing the studies that did not focus on the lipase of aquaculture fish, a final database of 339 articles was used for their review (Supplementary Material 1), and additional references used by authors were reviewed.
2.1.1 Fish species
Lipase activity has been studied in at least 95 fish genera (127 species) and 10 fish hybrids (Table S1, Supplementary Material 2), with the most studied genera being Oreochromis, Cyprinus, Oncorhynchus, Ctenopharyngodon, and Carassius (29.2%, 102 studies).
2.1.2 Digestive organs
The results indicated (Table S2, Supplementary Material 2) that the studied tissues belonged to either intestine [whole, anterior, medium, posterior (69.0%, 234 studies)], emptied intestine [mechanically or by washing with distilled water (2.7%, 9 studies)], intestinal content [digesta (2.9%, 10 studies)], whole digestive tract (7.4%, 25 studies), whole larvae (8.6%, 279 studies), or dissected larvae (2.7%, 9 studies), for the preparation of lipase extracts. It is essential to know the fish’s anatomy to be studied for the identification of the digestive segments. This system generally comprises the mouth–esophagus, stomach (or pseudostomach), and intestine. The pancreas or hepatopancreas is connected to the first portion of the intestine. Likewise, the pyloric cecum in fish that have it is identified as a cluster of lobes. The number of larvae was from 1 to 600 per sample, and the weights of tissue for lipase crude extract preparation were from 40 mg to 30 g. Unfortunately, 88.2% of the studies did not indicate the amount of tissue used. In general, the authors have used 1 to 66 volumes of extraction solvent: 1–2 (1.8%), 2–4 (9.4%), 5–6 (7.1%), 8–10 (31.0%), 14–25 (2.1%), 30–40 (4.4%), 66 (0.3%), 20,000 (0.3%), and not indicated (41.8%). In the specific case of larvae, the authors used 5 to 66 volumes of extraction solution.
2.1.3 Extraction solution
Regarding the extraction solution, 16.2% of the authors used physiological saline solution (0.65%–0.90%), 12.7% used water (distilled, deionized, Milli-Q, ultrapure), 7.4% used Tris (10 mM–100 mM, pH 7.2–7.5), 6.5% used phosphate-buffered saline (PBS) (pH 7.4–7.5), 6.2% phosphate (20 mM–100 mM, pH 7.0–8.0), and the rest used other solutions or did not specify the solution used for tissue homogenization (Table S3, Supplementary Material 2).
2.1.4 Extract clarification
Enzymatic extracts clarification has been performed at relative centrifugal force (RCF) values that ranged from 125 to 33,000 × g and centrifugation times that varied from 3 min to 60 min (at 4°C). In this regard, the g*min values varied from 1,875 to 900,000. Some authors reported the RCF applied but not the centrifugation time; others reported the rpm applied but did not give the data of the rotor used or their radius (r), which makes it impossible to calculate the RCF applied. Only 29.8% of the studies used a g*min value higher than 200,000 (Table S4, Supplementary Material 2).
For enzyme extract preparation, the method proposed by Nolasco-Soria (2020) is recommended, also considering the time of sacrifice to avoid the effect of the circadian cycle (Yúfera et al., 2018). In the case of larvae, the size (i.e., the weight and length) and thermal units of development should also be considered (Gisbert et al., 2018; Yúfera et al., 2018). In addition, samples should be kept cold while they are being handled, and long freezing times before their enzymatic analysis should be avoided (Solovyev and Gisbert, 2016).
According to differences in the fish intestinal pH (Solovyev and Izvekova, 2016) and the working physiological pH of fish lipases (pH that gives stability to the enzymes), as previously reported, the recommendation is to perform lipase extraction with a buffer at pH 7.0–9.0, or, better, in distilled water if the intestinal pH is unknown or if the optimum pH of fish lipases is expected to be determined. The extract is then doubly clarified by centrifugation of at least 15,000 × g, 15 min (225,000 g*min) at 4°C to reduce turbidity and keep it at −80°C (Nolasco-Soria, 2020).
2.2 Determination of lipase activity
Table 1 shows the methods used by the authors. The reader who knows the rationale of the methods may recognize the difficulty of comparing lipase values reported in fish studies.
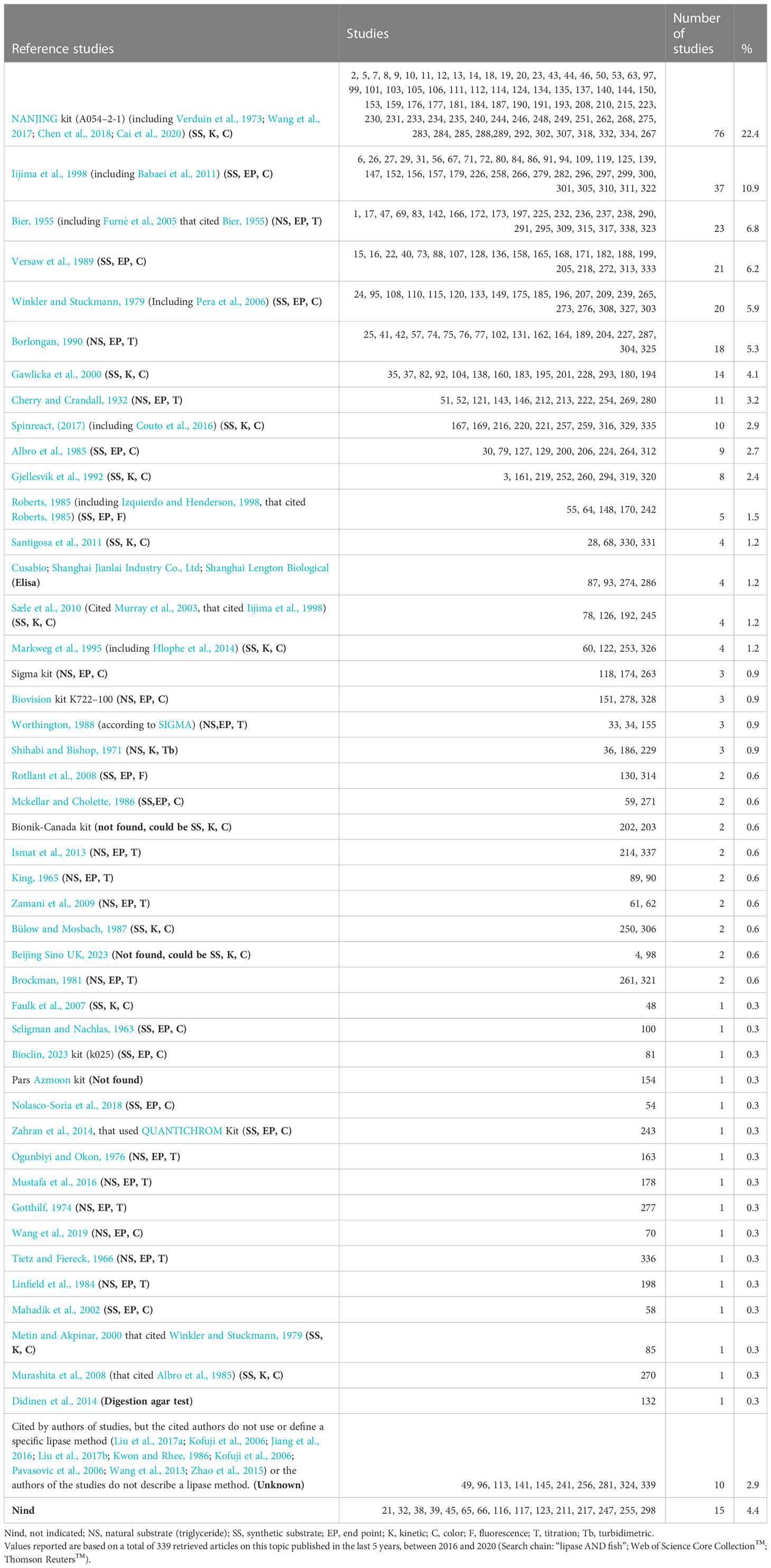
Table 1 Reference protocols (type of method) and their frequency of use for assessing the activity of lipase in larvae and digestive tract of fish.
One of the oldest published procedures for quantifying lipase activity used by some authors (3.2%) was from Cherry and Crandall (1932). The titrimetric protocol described by these authors is as follows:
“In a flask, 1 ml of serum is added to 2 ml of substrate olive oil emulsion (50% olive oil containing 5% of acacia gum as emulsifier and 0.2% of sodium benzoate as preservative), 3 ml of distilled water, and 0.5 ml of phosphate buffer (0.33 M, pH 7.0). After 24 hours of incubation at 40°C, the reaction is stopped by the addition of 3 ml of alcohol (95%). Then, three drops of phenolphthalein (1%), as an indicator, were added, and mixture titrated with NAOH (50 mM), recording the volume in ml required bringing the solution to the faintest permanent pink, as lipase activity.”
These authors also suggested that the term lipase should be reserved for the enzyme capable of hydrolyzing ester bonds on true lipids (fats and oils) and that esterase be used for the enzyme acting upon other esters (Cherry and Crandall, 1932). However, this method, indisputably valuable in determining the presence of true lipases, is tedious because samples need to be individually measured in glass flasks. In addition, this procedure does not permit the miniaturization of volumes to be carried out at a plastic microplate level. Similar methods cited by the authors include Bier (1955) [including Furné et al., 2005, which cited Bier (1955) (6.8%)], King (1965) (0.6%), Tietz and Fiereck (1966) (0.3%), Gotthilf (1974) (0.3%), Ogunbiyi and Okon (1976) (0.3%), Linfield et al. (1984) (0.3%), Borlongan (1990) (5.3%), Zamani et al. (2009) (0.6%), and Ismat et al. (2013) (0.6%). A more straightforward, turbidimetric method using an olive oil emulsion was developed by Shihabi and Bishop (1971). A modified method using olive oil as a substrate and a cupric acetate pyridine reagent for colorimetric free-fatty-acid determination was used by Mustafa et al. (2016) and Wang et al. (2019).
In contrast, at the microplate level, the method most used by the authors is that of a commercial kit. Unfortunately, for obvious reasons, the actual composition and concentration of the reactants are still being determined, which limits their practical utility (for example, if the study requires that the concentrations of substrate or developer be varied). This also applies to all other commercial kits used by the authors (Table 1).
The methods used to quantify fish digestive tract lipase were revised in the 339 studies retrieved from the systematic bibliographic search (Table 1). Without considering commercial kits, the method that was most used by the authors is that of Iijima et al. (Iijima et al., 1998, a modified method of Albro et al., 1985). The original method was explicitly described by Albro et al. (1985) as follows:
“A mixture of 0.4 M Tris-HCI (pH 7.4) at 37°C (200 ml), 116 mM sodium taurocholate (pH 7.4) (70 ml), 14 mM p-nitrophenyl myristate in ethylene glycol monomethyl ether (20 ml), and water (210 ml) was made fresh daily. Each assay tube received 5 ml of this mixture and was equilibrated at 37°C. Enzyme solution (5-100 µl as appropriate) was added and the solution was swirled at 200 rpm for from 5 to 15 min at 37°C. A 0.2 ml aliquot of incubation mixture was added to 2.8 ml of 0.014 M aqueous NaOH in a cuvette and the absorbance at 410 nm was determined relative to a procedure blank.”
In the reaction mixture used by Albro et al. (1985), the final concentrations of the buffer, sodium taurocholate, and p-NPM substrate were 160 mM, 16.2 mM, and 0.56 mM, respectively. In contrast, Iijima et al. (1998) reduced the mixture to one-tenth of its original volume, the bile salt concentration by a factor of 3 (but changed to sodium cholate), and the temperature to 30°C. However, they increased the pH of the reaction and used a stopper-extractor to separate the colored phase of the reaction by centrifugation. The Iijima et al. method was explicitly described as follows:
“Each assay (0.5 ml) contained 0.53 mM p-nitrophenyl myristate, 0.25 mM 2-methoxyethanol, 5 mM sodium cholate, and 0.25 M Tris-HCl (pH 9.0). Typically, 5-10 μl of enzyme solution was added to the substrate solution. Incubation was carried out for 15 min at 30°C, and the reaction was terminated by adding 0.7 ml of acetone/n-heptane (5:2, v/v). The reaction mixture was vigorously mixed and centrifuged at 6,080 × g for 2 min. The absorbance at 405 nm in the resulting lower aqueous layer (0.5 ml) was measured. The extinction coefficient of p-nitrophenol was 16,500 M–1 cm–1 per liter at pH 9.0.”
The p-nitrophenyl substrates with short fatty acids, such as acetate, should be avoided due to their non-specific hydrolysis (De Caro et al., 1988).
As reported by Nolasco-Soria et al. (2018), many authors have modified the original method developed by Albro et al. (1985) for assessing lipase activity, by using different reaction volumes (Gjellesvik et al., 1992), microplates instead of test tubes or kinetic techniques (Sæle et al., 2010, which cited Murray et al., 2003), or including Triton X-100 in the reaction mixture (Gawlicka et al., 2000, which cited German et al., 2004; Murashita et al., 2008, which cited Albro et al., 1985), with all of them using p-nitrophenyl myristate as a substrate (Table 1). Therefore, these authors are part of the most cited group. Winkler and Stuckmann (1979) previously developed a similar method using p-nitrophenyl palmitate as a substrate after it was modified by Markweg et al. (1995) (including Mahadik et al., 2002; Pera et al., 2006; Hlophe et al., 2014). Metin and Akpinar (Metin and Akpinar, 2000, who cited Winkler and Stuckmann, 1979) used p-nitrophenyl acetate as a substrate, as did Bülow and Mosbach (1987). The main disadvantage of some of the abovementioned methods is the requirement for organic solvents (toxic for users) or artificial detergents such as Triton X-100 (lipase inhibitor, in accordance with Aryee et al., 2007 and Nolasco-Soria et al., 2018) to clarify the reaction mixture before absorbance readings can be taken. Faulk et al. (2007) reported a kinetic method at the microplate level, using p-nitrophenyl caproate as a substrate (Table 1). The basis of the above methods is the quantification of the moles of p-nitrophenol released by the lipase hydrolysis of (the ester bond) the synthetic substrate.
The second most used method by the authors is that of Bier (Bier, 1955, including Furné et al., 2005, who cited Bier, 1955), which is very similar to the method previously developed by Cherry and Crandall (1932). According to Bier (1955), the assay protocol is as follows:
“In a 125-ml Erlenmeyer flask are mixed 10 ml of the freshly prepared PVA (polyvinyl alcohol)-emulsified substrate, 5 ml of buffer (McIlvaine), and 5 ml of the enzyme preparation to be tested. The mixture is shaken gently and incubated for 4 hours at 37° with constant shaking. At the end of the incubation time, 30 ml of a 1:1 alcohol-acetone solution is added to stop the reaction and break the emulsion. Phenolphthalein indicator is added, and the solution is titrated with 0.05 N NaOH”.
The basis of the method is the quantification of hydroxide moles, which are required to neutralize the protons released by the hydrolysis of ester bonds on olive oil substrate and alkalize the reaction to the change of the phenolphthalein color.
The third most used method by the authors is that of Versaw et al. (1989) (which is a modified version of the method of Mckellar and Cholette, 1986), using β-naphthyl caprylate (BNC) as a substrate. The original method was explicitly described by Versaw et al. (1989) as follows:
“The incubation mixture of the modified procedure, identical to that of Mckellar and Cholette (1986) in containing 0.2 ml 200 mM Na-T, 1.8 ml 50 mM BES buffer (pH 7.2), and 0.05ml lipase source, was equilibrated to 40°C in a water bath. Then 0.02 ml 200 mM BNC in DMSO was added and the mixture was incubated for 30 min. After incubation, the color reaction was produced by the addition of 0.02 ml Fast Blue BB salt (100 mM in DMSO) followed by an additional 5 min incubation at 40°C. The reaction was stopped with 0.2 ml 0.72N TCA. In McKellar and Cholette’s method, 5 ml ethyl acetate was added, the sample was mixed, centrifuged, and the ethyl acetate layer read for absorbance at 540 nm. At this juncture in our modification, 2.71 ml of a 1:l ethanol (95%)/ethyl acetate (v/v) mixture was added for a final volume of 5 ml. The addition of the mixed solvent produced a clarified sample which was read for absorbance.”
Previously, Seligman and Nachlas (1963) reported an end point method using β-naphthyl laurate. Furthermore, more recently, Nolasco-Soria et al. (2018) published a new method, with a new stopper-clarifier reagent at the microplate level. The basis of this method is quantifying the moles of β-naphthol released by the lipase hydrolysis of the ester bond of the synthetic substrate (β-naphthyl caprylate).
Other techniques for lipase activity, according to the method cited by the authors (Table 1), have a lower use (3% or lower, each), including colorimetric, fluorometric, and enzyme-linked immunosorbent assay (ELISA) methods.
The incomplete definition of the methods and the use of concatenated citations with other references make it difficult to know how lipase activity was measured. In addition, the variations or adjustments made by different authors to a lipase method cited as a reference make comparison difficult, even when the same method and author are cited, which requires a standardization effort, considering the following.
2.2.1 Assay volume
The volumes among the only 55 studies (16.2%) that explicitly reported reaction ranged from 70 µL to 25,000 µL. The remaining studies (n = 284, 83.8%) did not report the trial volume, so it is assumed that they used the one reported in the reference cited by the authors (Table 2). By type of method, the assay volumes were between 100 µl and 7,290 µl for colorimetric, between 100 µl and 3,300 µl for fluorometric, and between 2,100 µl and 31,000 µl for titrimetric procedures.
2.2.2 Type of substrate
The type of substrate used to measure lipase activity is highly relevant, mainly when synthetic substrates are used with a single associated fatty acid and different chain lengths (Nolasco-Soria et al., 2018). Thus, regarding lipase substrate, 175 studies (51.6%) explicitly reported the substrate; most of them used either p-nitrophenyl myristate (16.2%) or olive oil (13.6%). The rest of the studies (n = 164, 48.4%) did not report the substrate in the reaction mixture, so it is assumed that they used the substrate reported in the reference cited by the authors (Table 3). Only 67 studies (19.8%) explicitly reported the substrate concentration, ranging from 0.03 mM to 50 mM (1,666 times difference). By type of substrate, the concentrations were as follows: olive oil, 1% to 40%; other authors reported molarity from 0.1 mM to 100 mM but did not report which of the triglycerides of those found in olive oil (Boskou et al., 2006) was taken as the calculation basis. For p-nitrophenyl substrates (butyrate, caproate, and myristate), 0.04 mM to 50 mM. For 4-methylumbelliferyl substrates (butyrate, heptanoate, octanoate, and oleate), 0.03 mM to 50 mM. For β-naphthyl caprylate, 0.49 mM to 200 mM. For DDGR (1,2-o-dilauryl-rac-glycero-3-glutaric acid-(6′-methylresorufin) ester), 0.12 mM. In addition, an excess of substrate in the reaction mixture must be present during the entire reaction time (according to the Michaelis–Menten law) to determine lipase activity.
2.2.3 Reaction buffer
Regarding the reaction buffer, different solutions and concentrations were reported (Table 4). In particular, most authors who reported this information used a Tris buffer (16.2%) or phosphate buffer (6.2%). Unfortunately, 75.3% of the studies did not explicitly report the buffer employed, so it is assumed that they used the buffer reported in the reference cited by the authors. Most buffers have been used correctly depending on their working pH (Mohan, 2003), and the experiments in most of the reported studies were performed at an alkaline pH of between 7 and 9. If the working pH is between 7 and 9, the use of Tris-HCl buffer at a concentration between 20 mM and 50 mM is recommended. Regardless of the use of ions reported explicitly by authors, only 1.2% of the studies used Na+ (32 nm to 1000 mM), and 2.1% used Ca+2 (0.05 mM). As reported by Nolasco-Soria (2020), the requirement for NaCl and divalent ions, such as CaCl2, has to be previously determined, in this case for lipase activity in fish.
2.2.4 Reaction temperature
As is the case for all enzymes, the reaction temperature directly influences the reaction rate of lipases. Therefore, if there are differences in the incubation temperature (Table 5) in the methods used by the authors, it is complicated to compare the lipase units reported among the studies, even using the same method. In addition, it is advisable to measure lipase activity under the physiological or culture temperature conditions of the fish under study. Notably, 13.6% of the studies reported a reaction temperature higher than 35°C. However, if we consider the temperature used by references cited by the authors, the percentage of studies using temperatures from 35°C to 60°C, which are unusual for fish, rises to 59.7%. Likewise, the optimal temperature determined in the laboratory in vitro tests should not be used to measure lipase activity in fish. It is recommended to use a temperature of 25°C, which is closer to the ecophysiological temperature, at least for temperate-water fish (Gisbert et al., 2018).
2.2.5 Incubation time
Incubation time varies from 1 min to 60 min for synthetic substrates and 2 h to 24 h for natural substrates (oils) (Table 5). It is recommended that the incubation time for lipase determination should be from 5 min to 30 min (Nolasco-Soria et al., 2018) to have a fast and practical method.
2.2.6 Reaction stopper
For end point protocols regarding the method for stopping lipase reaction, the used chemicals are shown in Table 6. The foundation of the stopper is considered adequate. However, those based on acidification (acetic acid) or alkalinization (Na2CO3, NaOH, and Tris) of the medium have their limitations if the enzymes are active at those pH values, particularly if they are alkaline. It is recommended not to use organic solvents to avoid user exposure. One way to avoid stoppers is to use kinetic methods to measure lipase activity.
2.2.7 Wavelength
Only 106 studies (31.3%) reported the wavelength used for absorbance or fluorescence measurements. The wavelengths used are shown in Table 7. In the case of colorimetric methods that use p-Nitrophenyl series substrates, the wavelengths reported (400 nm–415 nm) by the authors to measure p-nitrophenol (p-NP) are close to the maximum absorption peak (Iijima et al., 1998). However, to correctly calculate the p-NP moles released by lipase activity, it is necessary to make a p-NP standard curve at the corresponding wavelength and pH. The same situation occurs in methods using β-naphthyl series substrates, such as β-naphthyl caprylate (Versaw et al., 1989). In the case of the quantification of MU (fluorometric method), the wavelengths reported by the authors are close to those recommended (Roberts, 1985) for excitation and emission. Nevertheless, the fluorometric unit calculation requires the construction of a standard curve for MU under the same experimental conditions. Unfortunately, many studies did not provide specific information on the wavelength for measuring absorbance or fluorescence of the assay mixture, so it is assumed that they used the wavelength reported in the reference cited by the authors (see Table 7).
2.2.8 Molar extinction coefficient
Only 3.0% of the studies reported molar extinction coefficients (MEC; M-1cm-1) for lipase quantification (Table 8). Another 3.8% used a standard curve (p-nitrophenol or β-naphthol standard curve), but without reporting a MEC or curve equation. The remaining 316 studies did not report the MEC used, so it is assumed that they used the MEC or calculation procedure reported in the references cited by the authors. The construction method of the standard curve (including pH) must be reported, in addition to the equation of the curve obtained, or the MEC used, for the calculation of the lipase units.
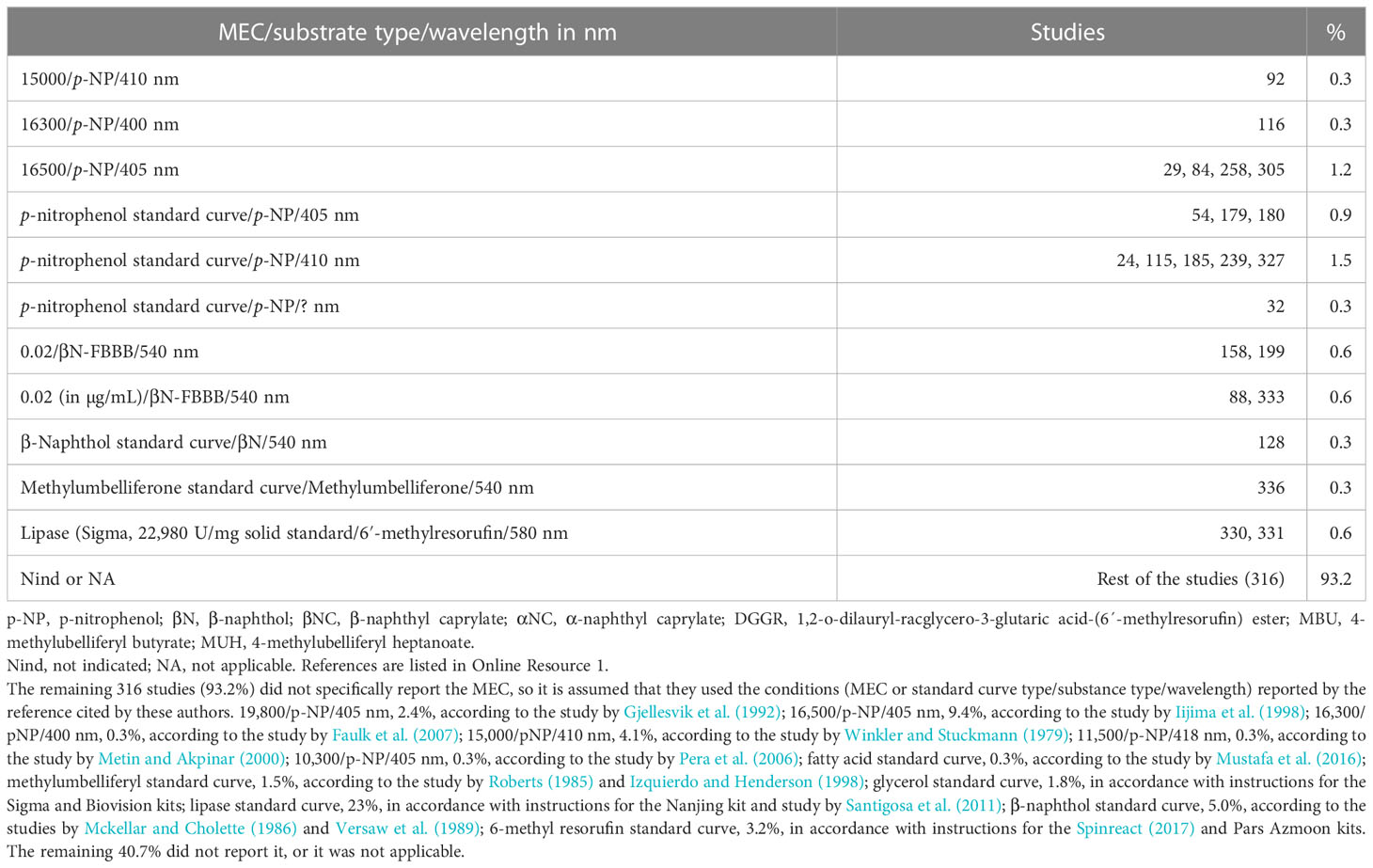
Table 8 Molar (or g/L) extinction coefficient or standard curve type used for lipase activity determination.
2.2.9 Lipase unit definition
Finally, only 118 studies (34.8%) reported the lipase unit definition. Of the studies that used p-nitrophenyl series substrates, only four used absorbance units (at 400 nm or 410 nm). Fortunately, 54 studies used unit expressions relative to moles of hydrolyzed substrate or generated product. Regarding the studies that used β-naphthyl series substrates, eight expressed the units in weight, and only two used unit expressions relative to moles of product generated per unit of time. The 36 studies that used lipid substrates expressed the lipase units in moles of product (fatty acids or glycerol) generated per unit of time (Table 9). The remaining 221 studies (65.2%) did not report the lipase unit explicitly used, so it is assumed that they used the lipase unit definition reported in the reference cited by the authors. The high variability or lack thereof, including the lipase unit definition, makes any comparison difficult. As examples of the variability of the reported results on lipase activity, Table 10 compares lipase units for similar samples and methods.
3 Discussion
According to Brockman (2013), lipases are water-soluble proteins, as ester hydrolases act on non-polar, water-insoluble ester substrates. According to BRENDA (2022), the lipases are classified with EC 3.1.1.3—triacylglycerol lipase. Pirahanchi and Sharma (2022) report that lipases break triglycerides into free fatty acids and glycerol by hydrolyzing the bonds of the latter. Lipases are present in pancreatic secretions for the digestion of dietary fats in the intestine. The natural foods (preys) that fish consume have high lipid contents in oils (rich in triacylglycerols). For example, lipids can account for two-thirds of the total weight of high-latitude zooplankton. In the case of artificial feeds used in aquaculture, fish oil was the most commonly used ingredient (Tocher, 2003; Qiu et al., 2017), but now the most common is vegetable oils (soybean, rapeseed, palm, corn, and sunflower) (Rombenso, 2018), with concentrations of up to 300g/kg dietary DM (Sargent and Tacon, 1999). The digestive capacity of fish will depend on the lipase units that are synthesized in the pancreas and secreted into the intestine to digest dietary lipids. Considering the lipase definition, the crude extract hydrolytic activity on triglycerides (preferably natural ones) must be demonstrated, in agreement with Cherry and Crandall (1932).
The titrimetric methods (Cherry and Crandall, 1932) or pH stat (Kurtovic et al., 2009; Espinosa-Chaurand and Nolasco-Soria, 2019) using oil substrates can be considered tedious, as they require a large reaction volumen and their step-by-step replicate análisis is time-intensive. Because of that, the use of more practical spectrophotometric (colorimetric) methods has been preferred, as these require a smaller reaction volume and can measure the hydrolytic activity on the synthetic substrates (such as p-NPM or β-NC) of several samples simultaneously. However, as evidenced in this review, the variability of the methods makes comparative studies of lipase activity in fish very difficult to conduct. Given that the methods with p-NPM (Iijima et al., 1998) and β-NC (Versaw et al., 1989) have been recognized as sufficient to determine the presence of lipase activity and its quantification, it is considered that they can continue to be used, due to their practicality in being able to evaluate a large number of samples at the same time. However, applying the hydrolysis method of natural oils is also recommended as definitive proof of the presence of lipase activity. In all cases, adjustments must be proposed for a practical standardized method that allows comparative studies for the same or different species.
The result of the review of the 339 articles taken as a sample reports reaction volumes ranging from 70 µL (Moro et al., 2016) to 25,000 µL (Su et al., 2017). The proposal is to use practical volumes of 200 µL (approximately 0.571 cm of the liquid column of a well of a standard 96-well microplate) for spectrophotometric methods with p-NPM and β-NC substrates and only 5 mL for the pH-stat method with natural oils as a substrate (Nolasco-Soria et al., 2018).
Due to the insoluble nature of lipase substrates, lipase measurement methods incorporated a detergent into the reaction mixture (to facilitate substrate solubility or the formation of micelles to multiply the lipid–water surface) or an organic solvent. However, due to the toxic nature of organic solvents, it is recommended not to use them. Triton-X100 must also be avoided due to its potential inhibitory action (Aryee et al., 2007; Nolasco-Soria et al., 2018). Replacing artificial detergents with natural ones, such as bile salts, is recommended. Only 34 of the studies analyzed reported the use of bile salts, including Na cholate (Kenari and Naderi, 2016), Na taurocholate (Frias-Quintana et al., 2016), (Na) taurodeoxycholate (Garcia-Meilan et al., 2016), and (Na) deoxycholate (Weinrauch et al., 2019). Of those bile salts cited, Na cholate was the most used (50%) in the reported cases. The concentration of bile salt used and reported by the authors fluctuated between 1.8 mM and 100 mM: Na cholate (1.8 mM –6 mM, 0.8–2.6 mg/mL), Na taurocholate (5 mM–100 mM, 2.7–53.8 mg/mL), (Na) taurodeoxycholate (3.6 mM, 1.9 mg/mL), and (Na) deoxycholate (5.2 mM, 2.0 mg/mL). It is possible that, in the case of the 100 mM concentration, this refers to the concentration of the reagent used and not to the final concentration in the reaction mixture.
Only four studies reported using 0.8 mM CaCl2 in the lipase reaction mixture. Therefore, the recommendation is to measure the lipase reaction mixture’s calcium requirements (0 mM–10 mM).
The working pH for determining lipase activity varied from pH 7 (Blanco et al., 2016) to pH 9 (Guo et al., 2016). It is proposed to use an intermediate pH between these values (pH 8) to determine lipase activity, which brings the working pH closer to the physiological pH of the fish intestine (average pH 7.5, according to Solovyev and Izvekova, 2016). Tris-HCl buffer is recommended at the final concentration in the reaction mixture, which was about 20 mM–30 mM.
The working temperature for determining lipase activity varied from 4°C (Jayant et al., 2018) to 60°C (Hahor et al., 2016). It is proposed to use a temperature of 25°C, which is closer to the ecophysiological temperature for temperate-water fish (Gisbert et al., 2018).
The working wavelength used to measure reaction mixture absorbance for the lipase determination with p-NP substrates ranged from 400 nm (Rueda-Lopez et al., 2017) to 410 nm (Hahor et al., 2016). Although the absorbance difference within the 400 nm to 410 nm range is slight, the recommendation is to measure the absorbance at the wavelength according to the absorption spectra of the final lipase reaction mixture. In the case of substrates from the β-N group, the wavelengths to measure the absorbance of the reaction mixture were 510 nm (Pujante et al., 2017) or 540 nm (Frias-Quintana et al., 2016). All the authors used the method of Versaw et al. (1989), who proposed using a wavelength of 540 nm. The β-naphthyl caprylate method is recommended for the first analysis of samples with low activity because of its high sensitivity and noticeable purple color. In the case of fluorometric methods, the wavelength used was 450 nm–460 nm (emission) and 365 nm–380 nm (excitation) for the MBU substrate.
The molar extinction coefficient (MEC) of p-NP will vary depending on the wavelength used to measure its absorbance. The few values reported by the authors were 16,300 at 400 nm (Thompson et al., 2019), 16,500 at 405 nm (Ramzanzadeh et al., 2016), and 15,000 at 410 nm (Adeyemi et al., 2020). The recommendation is to build a p-NP standard curve (pH 8.0) and to measure the absorbance at 400 nm to obtain the MEC for converting the absorbance to moles of p-NP to calculate the lipase units. Something similar occurs with the β-N MEC. Only two studies present the value of 0.02 MEC used (Najera-Arzola et al., 2018; Frias-Quintana et al., 2019). Because the MEC value used by authors is a low number, the expression units should differ from M-1cm-1.
The proposal is that lipase units should be expressed in micromoles of the product (p-NP or β-N or fatty acid) released per minute. Each mole would represent the hydrolysis of one mole of ester bonds. The significant differences between the reported lipase units (see Table 10) could be due to methodological differences. The proposal is to standardize the temperature, type of substrate, standard curve or MEC, and calculation formulas.
If lipase activity is measured at a mildly acidic pH, extreme care must be taken, as the color generated by either β-N or p-NP is negatively affected (color decrease). In contrast, at an alkaline pH, the color is not significantly affected. This forces, where appropriate, the building of a specific standard curve for the working pH.
The measurement of lipase activity using emulsified NaT-olive oil as a substrate in pH stat is a practical method (Nolasco, 2008; Nolasco-Soria et al., 2018) recommended as definitive proof of the presence of lipases, according to Cherry and Crandall (1932). If it is supposed that a pH stat (laboratory equipment) is unavailable (because it is relatively expensive), one option would be to use a high-precision potentiometer (with three or four decimal places) or a standard potentiometer (which is available in most laboratories) instead. The pH of the reaction mixture (containing the oil emulsion) is adjusted to the working pH (for example, 8.0), the enzymatic reagent is added (at pH 8.0), and the pH is adjusted to 8.0 every minute of digestion with NaOH at 10 mM or 20 mM (using repeater pipette), depending on the rate of hydrolysis by the experimental lipases. The consumption of NaOH per minute is recorded, and the micromoles per min of NaOH are calculated to have the micromole of ester bonds hydrolyzed per minute. This method can be used to measure the digestibility of edible oils for the species of interest. For this procedure, it is recommended to determine the digestive capacity by quantifying the total lipase units in the digestive tract (pyloric cecum intestine). In addition, the amount of food oil that the fish consumes in one serving should also be calculated. With these values, 10% of the lipase units can be used as enzymatic reagent and 10% of the equivalent oil of an intake can be used as substrate. In this way, the lipase units of a fish would serve to make 10 replicates of in vitro digestibility.
For lipase detection on SDS-PAGE electrophoresis gels, several methods have been used. Agar with emulsified olive oil was placed on the electrophoresis gel to reveal hydrolysis zones using Victoria Blue staining (Villanueva-Gutierrez et al., 2020). The authors also used a 2% agar, 1% oleic acid, and 0.01% phenol red, either with or without 0.01% ox bile salts (in 0.01 M CaCl2), revealing the lipases by the formation of a yellow spot on the agar substrate (González-Félix et al., 2018). Previously, lipase zymograms were obtained using a 4%–30% native gradient PAGE, where β-naphthyl caprylate (200 mM) was copolymerized and revealed in the form of a red spot using Fast Blue BB (100 mM) (Alvarez-González et al., 2008; Nolasco et al., 2011).
Authors should consider using the proposed lipase methods as an alternative to facilitate comparative studies. It is recommended that authors describe their way of calculating lipase activity in detail. Lipase units must be expressed regarding micromoles of hydrolyzed substrate or released product per min. Data on fish, digestive tract, and organ weight must be included. In addition to the specific enzyme activities (U/mg protein), total lipase units are required (lipase units per fish, per gram of fish, per gram of the digestive tract).
A proposal for standardizing lipase methods for fish is required. Because the most used substrate has been p-nitrophenyl substrates (mainly myristate) should be considered. It is necessary to experimentally evaluate some environmental and compositional variants of the lipase reaction mixture to ensure that it is safe, sensitive, and practical at the microplate level: (a) the presence of bile salts (type and concentration) as emulsifiers and lipase activity enhancers; (b) the optimum concentration of ions (Ca+2 and Na+); (c) the proper wavelength for absorbance readings and the adjustment of absorbance values at 1 cm light path; and (d) the use of the MEC according to the corresponding standard curve (built under the same experimental conditions at which the measurement of lipase activity was carried out). In addition, it is proposed that the lipase method using β-naphthyl substrate (mainly caprylate) be considered, because of its high sensitivity and colorful reaction mixture as a medium carbon-length substrate. The measurement of lipase activity using natural oils as substrates in a small volume is proposed as a definitive test to confirm the presence of lipases in the digestive tract of fish.
4 Conclusions
The most widely used methods to measure lipase activity in cultured fish are currently the colorimetric methods (with p-NPM and BNC as substrates) and the titrimetric method with natural oil (with olive oil as a substrate). However, methodological variability, even for studies that use the same type of methodology, makes it difficult to compare the data obtained. Standardizing current lipase analytical procedures should improve the reliability of comparative studies of aquaculture fish species. The above also applies to other taxonomic groups of aquacultural interest, such as crustaceans and mollusks.
Author contributions
HNS: Conceptualization, Methodology, Software, Data curation, Investigation, Validation, Formal analysis, Supervision, Resources, Project administration, Visualization, Funding acquisition, Writing—original draft, Writing—review & editing.
Funding
The research project has been fully sponsored by CONSEJO NACIONAL DE CIENCIA Y TECNOLOGÍA (México)FORDECYT-PRONACES grant number 3266032 “Estudio de frontera sobre la ecofisiología del desarrollo y fisiología digestiva del pulpo pigmeo Paroctopus digueti.
Acknowledgments
The author thanks Patricia Hinojosa Baltazar and María Esther Ojeda Castro from CIBNOR for their technical support. The author thanks CONACYT for financing project 3266032 through the FORDECYT-PRONACES/2020 fund, which includes the proper design of enzymatic methods for the pygmy octopus, and CYTED Network LARVAplus (ref. 117RT0521) for the honor of being invited to collaborate.
Conflict of interest
The author declares that the research was conducted in the absence of any commercial or financial relationships that could be construed as a potential conflict of interest.
The author HNS declared that they were an editorial board member of Frontiers, at the time of submission. This had no impact on the peer review process and the final decision.
Publisher’s note
All claims expressed in this article are solely those of the authors and do not necessarily represent those of their affiliated organizations, or those of the publisher, the editors and the reviewers. Any product that may be evaluated in this article, or claim that may be made by its manufacturer, is not guaranteed or endorsed by the publisher.
Supplementary material
The Supplementary Material for this article can be found online at: https://www.frontiersin.org/articles/10.3389/faquc.2023.1225216/full#supplementary-material
References
Adeyemi J. A., Olise C. C., Bamidele O. S., Akinola B. K. (2020). Effects of ultraviolet photooxidation of cypermethrin on the activities of phosphatases and digestive enzymes, and intestinal histopathology in African catfish, Clarias gariepinus (Burchell 1822). J. Exp. Zool. Part A-Ecol. Integr. Physiol. 333 (8), 543–549. doi: 10.1002/jez.2392
Alam M. S., Teshima S., Ishikawa M., Koshio S. (2001). Effects of ursodeoxycholic acid on growth and digestive enzyme activities of Japanese flounder Paralichthys olivaceus (Temminck & Schlegel). Aquacult. Res. 32, 235–243. doi: 10.1046/j.1355-557x.2001.00020.x
Albro P. W., Hall R. D., Corbett J. T., Schroeder J. (1985). Activation of non-specific lipase (EC 3.1.1.-) by bile salts. Biochim. Biophys. Acta 835, 477–490. doi: 10.1016/0005-2760(85)90117-1
Alvarez-González C. A., Moyano-López F. J., Civera-Cerecedo R., Carrasco-Chávez V., Ortíz-Galindo J. L., Nolasco-Soria H., et al. (2008). Development of digestive enzyme activity in larvae of spotted sand bass Paralabrax maculatofasciatus II: electrophoretic analysis. Fish Physiol. Biochem. 36 (1), 29–37. doi: 10.1007/s10695-008-9276-4
Aryee N. A., Simpson B. K., Villalonga R. (2007). Lipase fraction from the viscera of grey mullet (Mugil cephalus): isolation, partial purification and some biochemical characteristics. Enzyme Microbial. Technol. 40, 394–402. doi: 10.1016/j.enzmictec.2006.07.009
Azmoon P. Lipase kit. Available at: https://www.bioz.com/search?q=pars%20azmoon%20lipase%20kit&other=true&exact_search=true&rating_sort=true.
Babaei S. S., Abedian-Kenari A., Nazari R., Gisbert E. (2011). Developmental changes of digestive enzymes in Persian sturgeon (Acipenser persicus) during larval ontogeny. Aquaculture 318, 138–144. doi: 10.1016/j.aquaculture.2011.04.032
Bakke A. M., Glover C., Krogdahl A. (2011). “Feeding, digestion and absorption of nutrients,” in Fish Physiology: the multifunctional gut of fish. Ed. Brauner M. G. A. F. (London, UK: Elsevier), 444.
Beijing Sino UK. (2023). HY-60115 kit (Beijing, China: Beijing Sino-UK Institute of Biological Technology). Available at: https://www.sinobiological.com/.
Bier M. (1955). “Lipases,” in Methods in enzymology. Eds. Colowick S. P., Kaplan N. O. (New York: Academic Press), 627–642.
Bik E., Ishigaki M., Blat A., Jasztal A., Ozaki Y., Malek K., et al. (2020). Lipid droplet composition varies based on medaka fish eggs development as revealed by NIR-, MIR-, and raman imaging. Molecules 25, 817. doi: 10.3390/molecules25040817
Bioclin. (2023). Lipase K025 (Rua Teles de Menezes, 92 – Santa Brancac, CEP 31565-130 – Belo Horizonte – MG – Brasil: Quinbasa Química Básica Ltda. Available at: https//www-bioclin.com.br/.
Biovision Lipase Activity Colorimetric Assay Kit. Available at: www.biovision.com.
Blanco A., Planas M., Moyano F. J. (2016). Ontogeny of digestive enzymatic capacities in juvenile seahorses Hippocampus guttulatus fed on different live diets. Aquacult. Res. 47 (11), 3558–3569. doi: 10.1111/are.12806
Borlongan I. G. (1990). Studies on the digestive lipases of Milkfish, Chanos chanos. Aquaculture 89, 315–325. doi: 10.1016/0044-8486(90)90135-A
Boskou D., Blekas G., Tsimido M. (2006). “Olive Oil Composition,” in Olive Oil, Chemistry and Technology, 2nd ed. Ed. Boskou D. (New York: AOCS Publishing), 288. doi: 10.4324/9781003040217
Bouchaâla M., BouAli M., Ben Ali Y., Miled N., Gargouri Y., Fendri A. (2015). Biochemical characterization and molecular modeling of pancreatic lipase from a cartilaginous fish, the common stingray (Dasyatis pastinaca). Appl. Biochem. Biotechnol. 176 (1), 151–169. doi: 10.1007/s12010-015-1564-8
BRENDA (2022) Information on EC 3.1.1.3 - triacylglycerol lipase. Available at: https://www.brenda-enzymes.org/enzyme.php?ecno=3.1.1.3.
Brockerhoff H. (1973). A model of pancreatic lipase and the orientation of enzymes at interfaces. Chem. Phys. Lipids 10 (3), 215–222. doi: 10.1016/0009-3084(73)90002-9
Brockman H. L. (1981). Triglyceride lipase from porcine pancreas: EC 3.1. 1.3 Triacylglycerol acylhydrolase. Methods Enzymol. 71, 619–627. doi: 10.1016/0076-6879(81)71074-7
Brockman H. L. (2013). “Lipases,” in Encyclopedia of Biological Chemistry (Second Edition). Eds. Lennarz W. J., Lane M. D. (Waltham: Academic Press), 729–732. doi: 10.1016/B978-0-12-378630-2.00118-3
Bülow L., Mosbach K. (1987). The expression in E. coli of a polymeric gene coding for an esterase mimic catalyzing the hydrolysis of p-nitrophenyl esters. FEBS Lett. 210 (2), 147–152. doi: 10.1016/0014-5793(87)81325–x
Cai L. S., Wang L., Song K., Lu K. L., Zhang C. X., Rahimnejad S. (2020). Evaluation of protein requirement of spotted seabass (Lateolabrax maculatus) under two temperatures, and the liver transcriptome response to thermal stress. Aquaculture 516 (2020), 734615. doi: 10.1016/j.aquaculture.2019.734615
Chakrabarti I., Gani Md. A., Chaki K. K., Sur R., Misra K. K. (1995). Digestive enzymes in 11 freshwater teleost fish species in relation to food habit and niche segregation. Comp. Biochem. Physiol. 112A (1), 167–177. doi: 10.1016/0300-9629(95)00072-F
Chen J., Chen C., Tan Q. (2018). Ontogenic changes in the digestive enzyme activities and the effect of different starvation duration on the digestive enzyme activities of larval red swamp crayfish (Procambarus clarkii). Aquacult. Res. 49, 676–683. doi: 10.1111/are.13497
Cherry I. S., Crandall L. A. (1932). The specificity of pancreatic lipase: its appearance in the blood after pancreatic injury. Am. J. Physiol. 100 (2), 266–273. doi: 10.1152/ajplegacy.1932.100.2.266
Couto A., Barroso C., Guerreiro I., Pousão-Ferreira P., Matos E., Peres H., et al. (2016). Carob seed germ meal in diets for meagre (Argyrosomus regius) juveniles: Growth, digestive enzymes, intermediary metabolism, liver and gut histology. Aquaculture 451, 396–404. doi: 10.1016/j.aquaculture.2015.10.007
Cusabio. Available at: www.cusabio.com.
De Caro J. D., Chautan M. P., Rouimi P., Rovery M. (1988). Acetylation of Lys-373 in porcine pancreatic lipase after reaction of the enzyme or its C-terminal with p-nitrophenyl acetate. Biochimie 70 (12), 1785–1790. doi: 10.1016/0300-9084(88)90039-9
Didinen B., Yardimci B., Onuk E., Metin S., Yildirim P. (2014). Naturally Lactococcus garvieae infection in rainbow trout (Oncorhyncus mykiss Walbaum 1792): new histopathological observations, phenotypic and molecular identification. Rev. Med. Vet. 165 (1–2), 12–19. Available at: http://www.revmedvet.com/2014/RMV165.
Espinosa-Chaurand D., Nolasco-Soria H. (2019). In vitro digestibility of oils used in feed for Macrobrachium tenellum, Macrobrachium americanum and Litopenaeus vannamei adults. Aquaculture 512. doi: 10.1016/j.aquaculture.2019.734303
Faulk C. K., Benninghoff A. D., Holt G. J. (2007). Ontogeny of the gastrointestinal tract and selected digestive enzymes in cobia Rachycentron canadum (L.). J. Fish Biol. 70 (2), 567–583. doi: 10.1111/j.1095-8649.2007.01330.x
Frias-Quintana C. A., Alvarez-Gonzalez C. A., Guerrero-Zarate R., Valverde-Chavarria S., Ulloa-Rojas J. B. (2019). Changes in digestive enzymes activities during the initial ontogeny of wolf cichlid, Parachromis dovii (Perciformes: Cichlidae). Neotropical Ichthyol. 17 (1), e180161. doi: 10.1590/1982-0224-20180161
Frias-Quintana C. A., Dominguez-Lorenzo J., Alvarez-Gonzalez C. A., Tovar-Ramirez D., Martinez-Garcia R. (2016). Using cornstarch in microparticulate diets for larvicultured tropical gar (Atractosteus tropicus). Fish Physiol. Biochem. 42 (2), 517–528. doi: 10.1007/s10695-015-0156-4
Furné M., Hidalgo M. C., Lopez A., Garcia-Gallego M., Morales A. E., Domenzain A., et al. (2005). Digestive enzyme activities in Adriatic sturgeon Acipenser naccarii and rainbow trout Oncorhynchus mykiss, A comparative study. Aquaculture 250, 391–398. doi: 10.1016/j.aquaculture.2005.05.017
Garcia-Meilan I., Ordonez-Grande B., Machahua C., Buenestado S., Fontanillas R., Gallardo M. A. (2016). Effects of dietary protein-to-lipid ratio on digestive and absorptive processes in sea bass fingerlings. Aquaculture 463, 163–173. doi: 10.1016/j.aquaculture.2016.05.039
Gawlicka A., Parent B., Horn M. H., Ross N., Opstad I., Torrissen O. J. (2000). Activity of digestive enzymes in yolk-sac larvae of Atlantic halibut (Hippoglossus hippoglossus): indication of readiness for first feeding. Aquaculture 184 (3-4), 303–314. doi: 10.1016/S0044-8486(99)00322-1
German D. P., Horn M. H., Gawlicka A. (2004). Digestive enzyme activities in herbivorous and carnivorous prickleback fishes (Teleostei: Stichaeidae): Ontogenetic, dietary and phylogenetic effects. Physiol. Biochem. Zool. 77, 789–804. doi: 10.1098/422228
Gisbert E., Nolasco H., Solovyev M. (2018). Towards the standardization of brush border purification and intestinal alkaline phosphatase quantification in fish with notes on other digestive enzymes. Aquaculture 487, 102–108. doi: 10.1016/j.aquaculture.2018.01.004
Gjellesvik D. R., Lombardo D., Walther B. T. (1992). Pancreatic bile salt dependent lipase from cod (Gadus morhua): purification and properties. Biochim. Biophys. Acta 1124, 123–134. doi: 10.1016/0005-2760(92)90088-D
González-Félix M. L., Santana-Bejarano E. B., Perez-Velazquez M., Villalba-Villalba A. G. (2018). Partial characterization, quantification and activity of pancreatic lipase in the gastrointestinal tract of Totoaba macdonaldi. Arch. Biol. Sci. 70 (3), 489–496. doi: 10.2298/ABS180202009G
Görgün S., Akpınar M. A. (2012). Purification and characterization of lipase from the liver of carp, Cyprinus carpio L., (1758), living in lake Tödürge (Sivas, Türkiye). Turkish J. Fish. Aquat. Sci. 12, 207–215. doi: 10.4194/1303-2712-v12_2_03
Gotthilf N. (1974). “Lipase: titrimetric assay,” in Methods of Enzymatic Analysis. Ed. Bergmeyer H. U. (New York: Academic Press Inc.), 814–818.
Guo H. Y., Li J. Z., Ma Z. H., Zhang J. S., Zhen P. L., Cheng D. C. (2016). Ontogenetic development of digestive enzymes in orange-spotted grouper (Epinephelus coioides, Hamilton 1822) Larvae. Israeli J. Aquaculture-Bamidgeh 68 (1302), 1–12. doi: 10.46989/001c.20805
Habte-Tsion H. M., Liu B., Ge X. P., Xie J., Xu P., Ren M. C., et al. (2013). Effects of dietary protein levels on the growth performance, muscle composition, blood composition and digestive enzymes activities of Wuchang bream, Megalobrama amblycephala fry. Isr. J. Aquacult. 65, 1–9. (Bamidgeh 2013 IJA_65. 925). doi: 10.46989/001c.37709
Hahor W., Thongprajukaew K., Yoonram K., Rodjaroen S. (2016). Gastrointestinal functionality of aquatic animal (Oreochromis niloticus) carcass in water allows estimating time of death. J. Forensic Sci. 61 (6), 1647–1655. doi: 10.1111/1556-4029.13227
Hlophe S. N., Moyo N. A. G., Ncube I. (2014). Postprandial changes in pH and enzyme activity from the stomach and intestines of Tilapia rendalli (Boulenger 1897), Oreochromis mossambicus (Peters 1852) and Clarias gariepinus (Burchell 1822). J. App Ichthyol. 30, 35–41. doi: 10.1111/jai.12290
Iijima N., Chosa S., Uematsu1 K., Goto T., Hoshita T., Kayama M. (1997). Purification and characterization of phospholipase A2 from the pyloric caeca of red sea bream, Pagrus major. Fish Physiol. Biochem. 16, 487–498. doi: 10.1023/A:1007745021990
Iijima N., Tanaka S., Ota Y. (1998). Purification and characterization of bile salt-activated lipase from the hepatopancreas of red sea bream, Pagrus major. Fish Physiol. Biochem 18, 59–69. doi: 10.1023/A:1007725513389
Ismat N., Ashraf M., Naeem M., Hafeez-ur-Rehman M. (2013). Effect of different feed ingredients on growth and level of intestinal enzyme secretions in juvenile Labeo rohita, Catla catla, Cirrhinus mrigala and Hypophthalmicthys molitrix. Int. J. Aquac 3, 85–91. doi: 10.5376/ija.2013.03.0016
Izquierdo M. S., Henderson R. J. (1998). The determination of lipase and phospholipase activities in gut contents of turbot (Scophthalmus maximus) by fluorescence-based assays. Fish Physiol. Biochem. 19, 153–162. doi: 10.1023/A:1007734425931
Jayant M., Muralidhar A. P., Sahu N. P., Jain K. K., Pal A. K., Srivastava P. P. (2018). Protein requirement of juvenile striped catfish, Pangasianodon hypophthalmus. Aquacult. Int. 26 (1), 375–389. doi: 10.1007/s10499-017-0216-0
Jiang J., Wu X. Y., Zhou X. Q., Feng L., Liu Y., Jiang W. D., et al. (2016). Effects of dietary curcumin supplementation on growth performance, intestinal digestive enzyme activities and antioxidant capacity of crucian carp Carassius auratus. Aquaculture 463, 174–180. doi: 10.1016/j.aquaculture.2016.05.040
Kenari A. A., Naderi M. (2016). Effects of enriched Artemia by fish and soybean oils supplemented with vitamin E on growth performance, lipid peroxidation, lipase activity and fatty acid composition of Persian sturgeon (Acipenser persicus) larvae. Aquacult. Nutr. 22 (2), 382–391. doi: 10.1111/anu.12260
Kofuji P. Y., Hosokawa H., Masumoto T. (2006). Effects of dietary supplementation with feeding stimulants on yellowtail Seriola quinqueradiata (Temminck & Schlegel; Carangidae) protein digestión at low water temperatures. Aquacult. Res. 37 (4), 366–373. doi: 10.1111/j.1365-2109.2005.01435.x
Kurtovic I., Marshall S. N., Zhao X., Simpson B. K. (2009). Lipases from mammals and fishes. Rev. Fish. Sci. 17 (1), 18–40. doi: 10.1080/10641260802031322
Kurtovic I., Marshall S. N., Zhao X., Simpson B. K. (2010). Purification and properties of digestive lipases from Chinook salmon (Oncorhynchus tshawytscha) and New Zealand hoki (Macruronus novaezelandiae). Fish Physiol. Biochem. 36, 1041–1060. doi: 10.1007/s10695-010-9382-y
Kwon D. Y., Rhee J. S. (1986). A simple and rapid colorimetric method for determination of free fatty acids for lipase assay. J. Am. Oil Chem. Soc 63, 89–92. doi: 10.1007/BF02676129
Linfield W. M., Barauskas R. A., Sivieri L., Serota S., Stevenson R. W. (1984). Enzymatic fat hydrolysis and synthesis. J. Amer Oil Chem. Soc. 61, 191–195. doi: 10.1007/BF02678767
Liu H., Jin J., Zhu X., Han D., Yang Y., Xie S. (2017a). Effect of substitution of dietary fish meal by soybean meal on different sizes of gibel carp (Carassius auratus gibelio): Digestive enzyme gene expressions and activities, and intestinal and hepatic histology. Aquacult. Nutr. 23 (1), 129–147. doi: 10.1111/anu.12375
Liu Z. F., Gao X. Q., Yu J. X., Qian X. M., Xue G. P., Zhang Q. Y., et al (2017b). Effects of different salinities on growth performance, survival, digestive enzyme activity, immune response, and muscle fatty acid composition in juvenile American shad (Alosa sapidissima). Fish Physiol. Biochem. 43, 761–773. doi: 10.1007/s10695-016-0330-3
Mahadik N. D., Puntambekar U. S., Bastawde K. B., Khire J. M., Gokhale D. V. (2002). Production of acidic lipase by Aspergillus Niger in solid state fermentation. Process Biochem. 38, 715–721. doi: 10.1016/S0032-9592(02)00194-2
Markweg H., Lang M. S., Wagner F. (1995). Dodecanoic acid inhibition of lipase from Acinetobacter sp. OPA 55. Enzym Microb. Technol. 17, 512–516. doi: 10.1016/0141-0229(94)00067-2
Martínez-Lagos R., Tovar-Ramírez D., Gracia-López V., Lazo J. P. (2014). Changes in digestive enzyme activities during larval development of leopard grouper (Mycteroperca rosacea). Fish Physiol. Biochem. 40 (3), 773–785. doi: 10.1007/s10695-013-9884-5
Mckellar R. C., Cholette H. (1986). Determination of the extracellular lipases of Pseudomonas fluorescens spp. in skim milk with the b-naphthyl caprylate assay. J. Dairy Res. 53, 301–312. doi: 10.1017/S0022029900024900
Metin K., Akpinar M. A. (2000). Characterizationof lipase in intestine of Oncorhynchus mykiss (Walbaum 1792). Turkish. Turkish J. Biol. 24, 489–502.
Mohan C. (2003). Calbiochem® A guide for the preparation and use of buffers in biological systems. EMD, an affiliate of Merck KGaA, Darmstadt, Germany. 32 p. Available at: http://wolfson.huji.ac.il/purification/PDF/Buffers/CALBIOCHEM_Buffers.pdf.
Moro G. V., Silva T. S. C., Zanon R. B., Cyrino J. E. P. (2016). Starch and lipid in diets for dourado Salminus brasiliensis (Cuvier 1816): growth, nutrient utilization and digestive enzymes. Aquacult. Nutr. 22 (4), 890–898. doi: 10.1111/anu.12305
Mukundan M. K., Gopakumar K., Nair M. R. (1985). Purification of a lipase from the hepatopancreas of oil sardine (Sardinella longiceps Linnaceus) and its characteristics and properties. J. Sci. Food Agric. 36, 191–203. doi: 10.1002/jsfa.2740360310
Murashita K., Fukada H., Rønnestad I., Kurokawa T., Masumoto T. (2008). Nutrient control of release of pancreatic enzymes in yellowtail (Seriola quinqueradiata): involvement of CCK and PY in the regulatory loop. Comp. Biochem. Physiol. A Mol. Integr. Physiol. 150, 438–443. doi: 10.1016/j.cbpa.2008.05.003
Murray H. M., Gallant J. W., Perez-Casanova J. C., Johnson S. C., Douglas S. E. (2003). Ontogeny of lipase expression in winter flounder. J. Fish. Biol. 62, 816–833. doi: 10.1046/j.1095-8649.2003.00067.x
Mustafa A., KarMali A., Abdelmoez W. (2016). A sensitive microplate assay for lipase activity measurement using olive oil emulsion substrate: modification of the copper soap colorimetric method. J. Oleo Sci. 65 (9), 775–784. doi: 10.5650/jos.ess16066
Najera-Arzola I. C., Alvarez-Gonzalez C. A., Frias-Quintana C. A., Peña E., Martinez-Garcia R., Camarillo-Coop S., et al. (2018). Evaluation of Mannan oligosaccharides (MOS) in balanced diets for tropical gar juveniles (Atractosteus tropicus). Hidrobiologica 28 (3), 239–246. doi: 10.24275/uam/izt/dcbs/hidro/2018v28n3/Pena
Nanjing. Available at: www.njjcbio.com.
Nayak J., Nair P. G. V., Mathew S., Ammu K. (2004). A study on the intestinal lipase of Indian major carp Labeo rohita. Asian Fish. Sci. 17, 333–340. doi: 10.33997/j.afs.2004.17.4.006
Nolasco H. (2008). Métodos Utilizados por el Centro de Investigaciones Biológicas del Noroeste (CIBNOR) para la Medición de Digestibilidad in vitro para Camarón. En Manual de metodologías de digestibilidad in vivo e in vitro para ingredientes y dietas para camarón. Eds. Cruz Suárez L.E., Villarreal Colmenares H., Tapia Salazar M., Nieto López M. G., Marie D. A.V. C. (N.L., México: Universidad Autónoma de Nuevo León, Mty.), ISBN: ISBN: 978-607-433-020-5. 215-225.
Nolasco H., Moyano-López F., Vega-Villasante F. (2011). Partial characterization of pyloric-duodenal lipase of gilthead seabream (Sparus aurata). Fish Physiol. Biochem. 37 (1), 43–52. doi: 10.1007/s10695-010-9414-7. FI: 1.607. ISSN: 0920-1742 SSN: 0920-1742.
Nolasco-Soria H. (2020). Improving and standardizing protocols for alkaline protease quantification in fish. Rev. Aquacult. 13, 43–65. doi: 10.1111/raq.12463. ISSN:1753-5131.
Nolasco-Soria H., Moyano-López F., Vega-Villasante F., Del Monte A., Espinoza-Chaurand D., Gisbert E. (2018). “Lipase and Phospholipase Activity Methods for Marine Organisms. Chapter 7,” in Lipases and Phospholipases: Methods and Protocols, Methods in Molecular Biology. Ed. Sandoval G. Springer Science+Business Media, LLC, part of Springer Nature (New York, NY: Humana Press), 1835. doi: 10.1007/978-1-4939-8672-9_7
Ogunbiyi O. A., Okon E. E. (1976). Studies on the digestive enzymes of the African fruit bat Eidolon helvum (Kerr). Comp. Biochem. Physiol. 55 (4), 359–361. doi: 10.1016/0300-9629(76)90061-X
Pavasovic A., Richardson N. A., Mather P. B., Anderson A. J. (2006). Influence of insoluble dietary cellulose on digestive enzyme activity, feed digestibility and survival in the red claw crayfish, Cherax quadricarinatus (von Martens). Aquac. Res. 37, 25e32. doi: 10.1111/j.1365-2109.2005.01389.x
Pera L. M., Romero C. M., Baigori M. D., Castro G. R. (2006). Catalytic properties of lipase extracts from Aspergillus Niger. Food Tech. Biotech. 44, 247–252. Available at: http://hdl.handle.net/11336/45883.
Pirahanchi Y., Sharma S. (2022). Biochemistry, Lipase (Treasure Island (FL: StatPearls Publishing). Available at: https://www.ncbi.nlm.nih.gov/books/NBK537346/.
Pujante I. M., Diaz-Lopez M., Mancera J. M., Moyano F. J. (2017). Characterization of digestive enzymes protease and alpha-amylase activities in the thick-lipped grey mullet (Chelon labrosus, Risso 1827). Aquacult. Res. 48 (2), 367–376. doi: 10.1111/are.13038
Qiu H., Jin M., Li Y., Lu Y., Hou Y., Zhou Q. (2017). Dietary lipid sources influence fatty acid composition in tissue of large yellow croaker (Larmichthys crocea) by regulating triacylglycerol synthesis and catabolism at the transcriptional level. PloS One 12 (1), e0169985. doi: 10.1371/journal.pone.0169985
Quantichrom. Available at: https://www.bioassaysys.com/Lipase-Assay-Kit.html.
Ramzanzadeh F., Yeganeh S., JaniKhalili K., Babaei S. S. (2016). Effects of different photoperiods on digestive enzyme activities in rainbow trout (Oncorhynchus mykiss) alevin and fry. Can. J. Zool. 94 (6), 435–442. doi: 10.1139/cjz-2015-0180
Roberts I. M. (1985). Hydrolysis of 4-methylumbelliferyl butyrate: a convenient and sensitive fluorescent assay for lipase activity. Lipids 20, 243–247. doi: 10.1007/BF02534195
Rombenso A. (2018). Oilseed use in Aquaculture: Current trends and opportunities. Agric. Food–Aquaculture Program.
Rønnestad I., Yúfera M., Ueberschar B., Ribeiro L., Sæle Ø, Boglione C. (2013). Feeding behaviour and digestive physiology in larval fish: current knowledge, and gaps and bottlenecks in research. Rev. Aquac. 5 (Suppl. 1), S59–S98. doi: 10.1111/raq.12010
Rotllant G., Moyano F. J., Andrés M., Díaz M., Estévez A., Gisbert E. (2008). Evaluation of fluorogenic substrates in the assessment of digestive enzymes in a decapod crustacean Maja brachydactyla larvae. Aquaculture 282, 90–96.-. doi: 10.1016/j.aquaculture.2008.06.004
Rueda-Lopez S., Martinez-Montano E., Viana M. T. (2017). Biochemical Characterization and Comparison of Pancreatic Lipases from the Pacific Bluefin Tuna, Thunnus orientalis; Totoaba, Totoaba macdonaldi; and Striped Bass, Morone saxatilis. J. World Aquacult. Soc. 48 (1), 156–165. doi: 10.1111/jwas.12372
Sæle Ø, Nordgreen A., Olsvik P. A., Hamre K. (2010). Characterization and expression of digestive neutral lipases during ontogeny of Atlantic cod (Gadus morhua). Comp. Biochem. Physiol. Part A: Mol. Integr. Physiol. 157, 252–259. doi: 10.1016/j.cbpa.2010.07.003
Santigosa E., García-Meilán I., Valentín J. M., Navarro I., Pérez-Sánchez J., Gallardo M. A. (2011). Plant oils inclusion in high fish meal substituted diets. Effect on digestion and nutrient absorption in sea bream (Sparus aurata). Aquac. Res. 42, 962–974. doi: 10.1111/j.1365-2109.2010.02679.x
Sargent J. R., Tacon A. G. (1999). Development of farmed fish: a nutritionally necessary alternative to meat. Proc. Nutr. Soc. 58, 377–383. doi: 10.1017/s0029665199001366
Seligman A. M., Nachlas M. M. (1963). “Lipase,” in Methods of Enzymatic Analysis. Ed. Bergmeyer H. U. (London: Academic Press), 776–778.
Shanghai Jianlai Industry Co., Ltd. Available at: http://en.jianlianshiye.com/?About.html.
Shanghai Lengton Biological. Available at: https://tracxn.com/d/companies/shanghai-langton-.
Shihabi Z. K., Bishop C. (1971). Simplified turbidimetric assay for lipase activity. Clin. Chem. 17, 1150–1153. doi: 10.1093/clinchem/17.12.1150
Sigma Lipase Activity Assay Kit. Available at: https://www.sigmaaldrich.com/MX/es/product/sigma/mak046?gclid=Cj0KCQjwiqWHBhD2A.
Solovyev M., Gisbert E. (2016). Influence of time, storage temperature and freeze/thaw cycles on the activity of digestive enzymes from gilthead sea bream (Sparus aurata). Fish Physiol. Biochem. 42, 1383–1394. doi: 10.1007/s10695-016-0226-2
Solovyev M. M., Izvekova G. I. (2016). Seasonal changes in pH values in the intestine of fish from Lake Chany (West Siberia). Inland Water Biol. 9, 400–404. doi: 10.1134/S1995082916040131
Spinreact (2017). Lipase-LQ. Spinreact, S. A./ S.A.U. Ctra. Santa Coloma, 7 E-17176 Sant Esteve de Bas (Gi), Spain. MIBEIS19-I.
Srichanun M., Tantikitti M., Vatanakul V., Musikarune P. (2012). Digestive enzyme activity during ontogenetic development and effect of live feed in green catfish larvae (Mystus nemurus Cuv. & Val.). Songklanakarin J. Sci. Technol. 34 (3), 247–254. Available at: http://www.sjst.psu.ac.th.
Su P., Han Y., Jiang C., Ma Y., Pan J., Liu S., et al. (2017). Effects of chitosan-oligosaccharides on growth performance, digestive enzyme and intestinal bacterial flora of tiger puffer (Takifugu rubripes Temminck et Schlegel 1850). J. Appl. Ichthyol. 33 (3), 458–467. doi: 10.1111/jai.13282
Tang S.-L., Liang X.-F., He S., Li L., Alam M. S., Wu J. (2022). Comparative study of the molecular characterization, evolution, and structure modeling of digestive lipase genes reveals the different evolutionary selection between mammals and fishes. Front. Genet. 13. doi: 10.3389/fgene.2022.909091
Tang S., Liang X., He S., Zhang Y., Peng D., Feng H. (2021). Development of digestive enzymatic activity and gene expression during the early ontogeny of Chinese perch (Siniperca chuatsi). (Research Square Company, a CC BY 4.0 License). doi: 10.21203/rs.3.rs-141784/v1
Thompson K. L., Faulk C. K., Fuiman L. A. (2019). ) Applying the ontogeny of digestive enzyme activity to guide early weaning of pigfish, Orthopristis chrysoptera (L.). Aquacult. Res. 50 (5), 1404–1410. doi: 10.1111/are.14015
Tietz N. W., Fiereck E. A. (1966). A specific method for serum lipase determination. Clin. Chim. Acta 13, 352–358. doi: 10.1016/0009-8981(66)90215-4
Tocher D. R. (2003). Metabolism and functions of lipids and fatty acids in teleost fish. Rev. Fish. Sci. 11 (2), 107–184. doi: 10.1080/713610925
Une M., Goto T., Kihira K., Kuramoto T., Hagiwara K., Nakajima T. (1991). Isolation and identification of bile salts conjugated with cysteinolic acid from bile of the red seabream, Pagrosomus major. J. Lipid Res. 32, 1619–1623. doi: 10.1016/S0022-2275(20)41647-5
Verduin P. A., Punt J. M., Kreutzer H. H. (1973). Studies on the determination of lipase activity. Int. J. Clin. Chem. 46, 9–11. doi: 10.1016/0009-981(73)90096-x
Versaw W. K., Cuppett S. L., Winters D. D., Williams L. E. (1989). An improved colorimetric assay for bacterial lipase in nonfat dry milk. J. Food Sci. 54, 1557–1558. doi: 10.1111/j.1365-2621.1989.tb05159.x
Villanueva-Gutierrez E., Maldonado-Othon C. A., Perez-Velazquez M., Gonzalez-Felix M. L. (2020). Activity and partial characterization of trypsin, chymotrypsin, and lipase in the digestive tract of Totoaba macdonaldi. J. Aquat. Food Prod. Technol. 29 (4), 322–334. doi: 10.1080/10498850.2020.1733157
Wang T., Cheng Y. Z., Liu Z. P., Yan S. H., Long X. H. (2013). Effects of light intensity on growth, immune response, plasma cortisol and fatty acid composition of juvenile Epinephelus coioides reared in artificial seawater. Aquaculture 414-415, 135–139. doi: 10.1016/j.aquaculture.2013.08.004
Wang W., Ishikawa M., Dawood M. A. O., Koshio S., Yokoyama S., Moss A. S., et al. (2019). Effects of dietary astaxanthin and vitamin E and their interactions on the growth performance, pigmentation , digestive enzyme activity of kuruma shrimp (Marsupenaeus japonicus). Aquacult. Nutr. 50, 1186–1197. doi: 10.1111/are.13993
Wang P., Zhu J. Q., Feng J., He J. J., Lou Y. D., Zhou Q. C. (2017). Effects of dietary soy protein concentrate meal on growth, immunity, enzyme activity and protein metabolism in relation to gene expression in large yellow croaker Larimichthys crocea. Aquaculture 477, 15–22. doi: 10.1016/j.aquaculture.2017.04.030
Weinrauch A. M., Schaefer C. M., Goss G. G. (2019). Activity and post-prandial regulation of digestive enzyme activity along the Pacific hagfish (Eptatretus stoutii) alimentary canal. PloS One 14 (4), e0215027. doi: 10.1371/journal.pone.0215027
Winkler U. K., Stuckmann M. (1979). Glycogen, hyaluronate, and some other polysaccharides greatly enhance the formation of exolipase by Serratia marcescens. J. Bacteriol. 138, 663–670. doi: 10.1128/jb.138.3.663-670.1979
Worthington C. C. (1988). In Worthington Enzyme Manual. Ed. Worthington C. C. (Freehold, NJ: Worthington Biochemical Corporation), 212–214.
Xiong D. M., Xie C. X., Zhang H. J., Liu H. P. (2011). Digestive enzymes along digestive tract of a carnivorous fish Glyptosternum maculatum (Sisoridae, Siluriformes). J. Anim. Physiol. Anim. Nutr. (Berl) 95 (2011), 56–64. doi: 10.1111/j.1439-0396.2009.00984.x
Yanbo W., Zirong X. (2006). Effect of probiotics for common carp (Cyprinus carpio) based on growth performance and digestive enzyme activities, Animal Feed Sci. Technol 127, 283–292. doi: 10.1016/j.anifeedsci.2005.09.003
Yúfera M., Moyano F. J., Martínez-Rodríguez G. (2018). “The digestive function in developing fish larvae and fry. From Molecular Gene Expression to Enzymatic Activity,” in Emerging Issues in Fish Larvae Research. Ed. Yúfera M. (Cham, Switzerland: Springer International Publishing AG), 296, ISBN: ISBN 978-3-319-73243-5 ISBN 978-3-319-73244-2.
Zahran E., Risha E., Abdelhamid F., Mahgoub H. A., Ibrahim T. (2014). Effects of dietary Astragalus polysaccharides (APS) on growth performance, Immunological parameters, digestive enzymes, and Intestinal morphology of Nile tilapia (Oreochromis niloticus). Fish. Shellfish Immun. 38 (1), 149e157. doi: 10.1016/j.fsi.2014.03.002
Zamani A., Hajimoradloo A., Madani R., Farhangi M. (2009). Assessment of digestive enzymes activity during the fry development of the endangered Caspian brown trout Salmo caspius. J. Fish Biol. 75 (4), 932–937. doi: 10.1111/j.1095-8649.2009.02348.x
Keywords: aquacultural fish, pyloric caeca-intestine, lipase, enzyme unit, standardizing
Citation: Nolasco-Soria H (2023) Fish digestive lipase quantification methods used in aquaculture studies. Front. Aquac. 2:1225216. doi: 10.3389/faquc.2023.1225216
Received: 18 May 2023; Accepted: 18 July 2023;
Published: 11 August 2023.
Edited by:
Youji Wang, Shanghai Ocean University, ChinaReviewed by:
Hüseyin Sevgili, Isparta University of Applied Sciences, TürkiyeCarlos Alfonso Alvarez-González, Universidad Juárez Autónoma de Tabasco, Mexico
Copyright © 2023 Nolasco-Soria. This is an open-access article distributed under the terms of the Creative Commons Attribution License (CC BY). The use, distribution or reproduction in other forums is permitted, provided the original author(s) and the copyright owner(s) are credited and that the original publication in this journal is cited, in accordance with accepted academic practice. No use, distribution or reproduction is permitted which does not comply with these terms.
*Correspondence: Héctor Nolasco-Soria, aG5vbGFzY28wNEBjaWJub3IubXg=
†ORCID: Héctor Nolasco-Soria, orcid.org/0000-0003-1584-4647