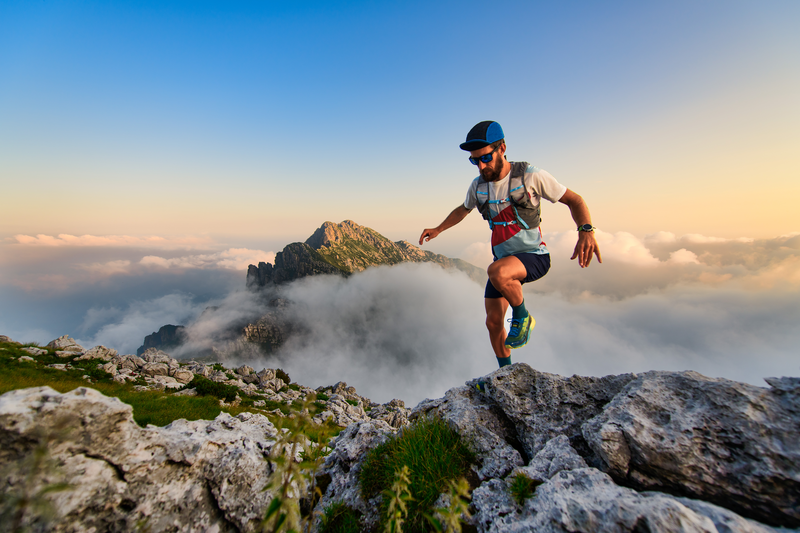
95% of researchers rate our articles as excellent or good
Learn more about the work of our research integrity team to safeguard the quality of each article we publish.
Find out more
ORIGINAL RESEARCH article
Front. Aquac. , 02 May 2023
Sec. Production Biology
Volume 2 - 2023 | https://doi.org/10.3389/faquc.2023.1189325
Understanding nutrition and its effect on growth is essential for developing diets in commercially important species. This study aimed to evaluate the larval development of Callinectes sapidus fed with rotifers (Brachionus plicatilis) enriched with Chlorella pyrenoidosa (Ch) as a main protein source and a lipid emulsion (Spr) rich in highly unsaturated fatty acids. The enriched compositions had seven ratios Ch/Spr (w/w): 100/0, 90/10, 80/20, 70/30, 60/40, 50/50, and 40/60. The enriched rotifers with each ratio were called diets. Lipids increased progressively between 100/0 and 40/60 (7.48 to 11.30 g 100 g-1), as well as energy (21.88 to 23.16 kJ g-1), but the protein level did not show a clear trend (63.93 and 67.50 g 100 g-1). The production response of blue crab larvae showed significant differences within diets (P < 0.05). The highest survival (29 to 34%) was obtained in the 50/50 and 40/60 treatments. In the 40/60, 50/50, and 60/40 diets, the larval cycle was shorter (P < 0.05), and the megalopa stage was reached between 49 and 52 days of culture. Metamorphosis to megalopa occurred between the fifth and eighth molts; its corresponding weight varied from 0.72 to 1.33 mg, the length varied from 2,632 to 2,926 µm, and Le Cren´s condition factor varied from 0.83 to 1.16. The most important factor in the production response was the diet lipid content, which was significantly correlated with the survival, weight, length, and condition factor of C. sapidus larvae. Findings of the present study can be applied in future efforts towards mass culture of blue crab larvae in the Caribbean and can also potentially be used to study other crustacean species with similar characteristics.
The blue crab, Callinectes sapidus, is a brachyuran crustacean of major interest to fisheries in subtropical waters of the Western Atlantic (Daly et al., 2021), but its commercial exploitation is rapidly increasing worldwide, including the Caribbean. Since the 1990s this species began to be captured in Colombia (South America) and soon became an important commercial species; however, recent reports indicate that over 70% of capture was below the maturity size, affecting the reproductive capacity of natural populations (Maestre et al., 2022). One way to overcome the dependence on natural stocks is to develop aquaculture techniques.
After hatching, the blue crab goes through six to eight zoea stages (pelagic phase) until it reaches the last larval metamorphosis to the megalopa (MG) stage (benthic phase), which shares several characteristics with juveniles (Costlow and Bookhout, 1959; Anger, 2001; Anger, 2001; Bacab et al., 2002; Zmora et al., 2005). The time to reach the MG stage takes 26 to 65 days, depending on salinity and temperature conditions (Stuck et al., 2009; Maurer et al., 2017). Initial efforts to culture this species began in 1959, and culture protocols based on live food supplements were established (Costlow and Bookhout, 1959; Sulkin, 1975). Although survival rates above 30% have recently been obtained in waters of the Western Atlantic (Zmora et al., 2005; Maurer et al., 2017), little is known about its larval development from tropical regions specimens (Bacab et al., 2002; Stuck et al., 2009). Also, information about their nutritional requirements during larval development is lacking (Sulkin, 1975; Pletl, 1992; Maurer et al., 2017). The use of known nutritional composition diets has been extensively studied in some crustacean larval and juvenile cultures, with good results in shrimp (Brito et al., 2001; Pedroza-Islas et al., 2004; Calado et al., 2022; Zarantoniello et al., 2023), but information on commercially important brachyuran species is scarce and focused mainly on Scylla sp. and Eriocheir sp. larval cultures (Genodepa et al., 2004; Holme et al., 2007; Sui et al., 2007; Holme et al., 2009; Liu et al., 2021).
The rotifer Brachionus plicatilis has been widely used in the larval culture of marine fish and crustaceans; its morphological characteristics and size make it ideal for species that consume live prey in their early stages (Rodríguez et al., 1996; Li and Olsen, 2015). Zoeae consume live prey, but since the first larval culture of C. sapidus bioassays, it was determined that B. plicatilis use did not allow complete larval development to megalopa due to its nutritional deficiencies (Sulkin, 1975). However, enrichment of rotifers improves their nutritional profile and provides nutrients that, in their original state, are absent or present in low concentrations. Emulsions of oils rich in highly unsaturated fatty acids (HUFAs), which are decisive in the larval phases, have been used (Li et al., 2014; Li and Olsen, 2015; Waqalevu et al., 2019). Increased lipids (especially HUFAs) in rotifers used as live food promote improvements in the survival and morphogenesis of fish and crustacean larvae (Suprayudi et al., 2002; Hu et al., 2017; Jeffs and O´Rorke, 2020; Tian et al., 2020; Singh et al., 2023).
Additionally, protein sources from algae (frozen, powder, or paste), as well as probiotics, vitamins, and minerals, have been used to enrich rotifers (Hamre et al., 2008; Srivastava et al., 2011; Nordgreen et al., 2013; Le et al., 2017; Wang et al., 2019; Fu et al., 2021; Khoa et al., 2021; Louvado et al., 2023). Despite being freshwater microalgae, the genus Chlorella is widely used in marine species culture due to its high protein content and other essential nutrients, which are similar to those in the marine microalgae Nannochloropsis (Maruyama et al., 1997; Ashraf et al., 2011; Raja et al., 2018; Arguelles, 2021). The role of proteins as muscle and shell builders, digestive enzymes, and hormones is crucial (Anger, 2001; Brucet et al., 2005; Lemos and Weissman, 2020). In Callinectes sapidus, Maja brachydactyla, and Portunus trituberculatus, the levels of most amino acids tend to increase during the early stages of zoeae and drop dramatically from the last zoea to megalopa stage (Tucker and Costlow, 1975; Andrés et al., 2010; Shi et al., 2019); consequently, diets with high protein digestibility allow greater survival from the larval to juvenile phase (Anger, 2001; Carter and Mente, 2014; Shi et al., 2019). This study aimed to evaluate the effect of rotifers (B. plicatilis) enriched with Ch. pyrenoidosa powder as a main protein source and a lipid emulsion as a main fatty acid source on the larval development (from the first zoea stage to MG) of C. sapidus from the Caribbean region.
The primary rotifer culture (B. plicatilis) was developed in six 15 L conical tanks at a salinity of 30 g L-1 with vigorous aeration in batch-type cultures, feeding them daily with live Nannochloropsis sp. microalgae (20 to 50 x 103 cells mL-1) and a mixture of dry yeast (Instant Success®), Ch. pyrenoidosa powder (Holistic Herbal Solutions®), and cod liver oil (Scott’s Emulsion®) in proportions of 70, 10, and 20% at a rate of 0.5 g of mixture x 106 rot.
For the experiments, the rotifers were enriched with mixtures of Ch. pyrenoidosa (Ch) powder (Holistic Herbal Solutions®) as a main protein source and a lipid emulsion (S.presso Selco®) abundant in ω3 PUFAs at 150 mg g-1 and 9 DHA/EPA (Spr), in seven different ratios Ch/Spr (w/w): 100/0, 90/10, 80/20, 70/30, 60/40, 50/50 and 40/60. The enrichment was carried out in 24 h periods in seven containers of 1.5 L with ~500 rot. mL-1. Each mixture was added to the respective flask at a rate of 2.0 g mixture/1 x 106 rotifers in four portions (at 0, 6, 12, and 18 h); after 24 h, the enriched batches were harvested and washed with sterile seawater to feed the C. sapidus larvae. The rotifers enriched with each of the mentioned mixtures were called diets.
To determine the proximal composition of the diets (enriched rotifers), ~200 rotifers mL-1 were stocked in 120 L fiberglass tanks. In parallel, according to the previous description, each tank received the respective enrichment portion. This process was repeated until 5 g of the dry weight of rotifers was obtained by diet. Then, the collected batches were dried in a convection oven (Shel Lab) at 105°C for 12 h (A.O.A.C, 2005), and the resulting biomass was stored at –20°C and lyophilized (Labconco Free Zone 4.5) for later analysis.
Adult females of C. sapidus with ovigerous masses in an early stage of development (Hill et al., 1989) were collected at Ciénaga Grande de Santa Marta, Colombia. The organisms were transported to the Jorge Tadeo Lozano University aquaculture laboratory. The females were placed individually in 10 L containers and acclimatized to 30 g L-1 salinity, previously recommended for the larval development of the species (Zmora et al., 2005; Bembe et al., 2017; Maurer et al., 2017). Each container was maintained with sterilized seawater and vigorous aeration. Every other day, 85 to 90% of the water was changed. The temperature was maintained between 25 and 27°C, oxygen was at saturation (> 5 mg L-1), and the photoperiod was 12 L:12 D. During this period, the females were fed once a day with fresh squid and were kept under permanent observation. When hatching was recorded, the zoeae were collected, immediately washed with sterile water at the same salinity, and placed in 3 L containers for acclimatization (described in the next section).
The acclimatization period for the early zoeae lasted four days. The organisms were distributed in 14 containers of 3 L, at a density of ~30 larvae mL-1, with low aeration, a temperature of 27 ± 1°C, and a photoperiod of 12 L:12 D. Beginning at this stage, artificial seawater (Premium Reef Salt Omega One®) previously filtered and autoclaved was used at 30 g L-1 salinity. As a preventive prophylactic treatment, chloramphenicol (10 mg L-1) was added, according to Sulkin and Van Heukelem (1986). Daily, in the morning (7:30 h), each container received between 20,000 and 50,000 cells mL-1 live Nannochloropsis sp. to create a “green water” effect (Zmora et al., 2005; Shan and Lin, 2014; Basford et al., 2021), and in the afternoon (15:00 h), the respective diets were added (~300 rot. mL-1). Every other day, 90% of the water was changed to remove excess microalgae, rotifers, and dead larvae to maintain the water quality.
The experimental design consisted of seven treatments in quadruplicate (four larval batches). Each replicate had a total of 78 larvae by diet. To strictly control the development of the organisms, transparent tissue culture microplates with 24 wells (3 mL each) were used as experimental units; one larva was kept in each well (Maurer et al., 2017). Before stocking, each well received 1 mL of artificial seawater (30 g L-1) that had been previously sterilized.
After acclimatization, the larvae were fed daily with the respective diet (from 15:00 to 17:00 h), according to Table 1. In addition to maintaining water quality, during the first 10 days of the trial, 200 µL day-1 Nannochloropsis sp. microalgae were added to each well (to maintain a density of ~ 50,000 cells mL-1 as green water effect). The photoperiod was 12 L:12 D, and the temperature was 27 ± 1°C. Every other day, the larvae were transferred to clean microplates with sterile seawater. The trials ended when the last larva reached the megalopa (MG) stage or died.
Table 1 Density and size of enriched rotifers supplied as live food during larval development of the blue crab Callinectes sapidus from hatching to the megalopa stage.
Mortality and the presence of molts in the wells were recorded daily; each microplate was reviewed under a stereoscopic microscope (Nikon C-Leds) with 10X magnification. Microphotographs of each living MG were taken to determine the total length as the distance from the base of the rostrum to the lateral tip of the telson (Mantelatto et al., 2014). The images were processed with NIS-Elements Br software (Nikon). The weight was determined with an analytical balance (Precisa 321); previously, excess moisture was removed with absorbent paper. Le Cren´s condition factor of the MG was also determined according to the equation: Kn=W/Wc (Le Cren, 1951), where W= weight of the larva at a given size and Wc= expected weight at the same length (calculated from a regression of weight and height of all the pooled treatments).
The composition of the diets was determined in the chemical and bromatological analysis laboratory of the National University of Colombia, Medellin Campus. Determinations were performed in triplicate. The protein content was determined with the Kjeldahl technique (A.O.A.C, 2000), the lipid content by the Soxhlet method with Velp Scientifica equipment (SER 148) (A.O.A.C, 2000), the ash content by calcination in a muffle furnace (A.O.A.C, 2000), the carbohydrate or total sugar content by the DuBois colorimetric method (DuBois et al., 1956), and the gross caloric value by Ika-Werke bomb calorimetry (C 2000 basic) (A.O.A.C, 2005).
With the collected data, normality (Shapiro−Wilks) and homoscedasticity (Barlett or Levene) tests were performed (Zar, 2010). One-way ANOVAs of the production response of the larvae were performed on final survival, the average time of molting, and the diet content of crude protein, total lipids, and carbohydrates. In cases where the statistical tests were significant (P < 0.05), the Fisher´s LSD post-test was applied. The nonparametric Kruskal−Wallis test was applied to evaluate the ash and energy content of the diets, and Bonferroni´s post-tests were used. A two-way ANOVA was also applied to MG length and Le Cren condition factor (fixed factor: diet; random factor: larval batch), with post-test LSD analysis.
Friedman´s blocks test (diet as a categorical variable and larval batch as the block) was also performed on the duration of the larval cycle, MG weight, and percentage of MGs obtained in each molt, and a Conover´s post-test was performed in cases of statistical significance (P < 0.05). Finally, Spearman’s linear correlation coefficients (r) were determined between the diets’ proximal composition and the larval production response variables. The information was processed with Statgraphics software (version 18) and R Studio 1.3.1093 software. In all tests, the significance level was P < 0.05.
Survival and duration of the larval cycle of C. sapidus were related to the supplied diet. As the content of Spr increased, survival improved, and the duration of the larval cycle was reduced (Table 2). The highest survival (29 to 34%) was obtained with the 50/50 and 40/60 diets. Consistently, the duration of the larval cycle was shorter (P < 0.05) with the 40/60, 50/50 and 60/40 diets, in which the MG stage was reached between 49 and 52 days of culture.
Table 2 Survival and duration of the larval cycle of Callinectes sapidus larvae fed with enriched rotifers as live food (Brachionus plicatilis enriched for 24 h with Chlorella pyrenoidosa, Ch, and S.presso emulsion, Spr) in different proportions (Ch/Spr).
The survival curves show two critical stages. The first is at the beginning of the larval cycle, which comprised the first 13 days of the trial; at this time, the population of zoeae shrank by between 45 and 57%. The second critical stage, although with lower mortality, occurred prior to the MG phase, between days 43 and 46 (Figure 1).
Figure 1 Survival (%) of Callinectes sapidus during larval development (from hatching to megalopa) fed with enriched rotifers (Brachionus plicatilis enriched for 24 h with Chlorella pyrenoidosa, Ch, and S.presso emulsion, Spr) in different proportions (Ch/Spr). The end of the lines indicates the time when all the larvae reached the megalopa stage.
In all diets, the first molt (M1) occurred at 7.90 ± 0.11 days after hatching (the 4-day acclimatization phase is included in this period), and among M2, M3, and M4, the average time of molting was similar. However, in the subsequent molts, the periods began to show variations among diets, which were accentuated toward the end of the experiment (Figure 2). There were no significant differences in the average day on which the larvae molted between diets (P > 0.05).
Figure 2 Periods (days; average ± maximum and minimum day) in which Callinectes sapidus larvae molted from hatching to megalopa (M1-M8), fed with enriched rotifers (Brachionus plicatilis enriched for 24 h with Chlorella pyrenoidosa, Ch, and S.presso emulsion, Spr) in different proportions (Ch/Spr). The drawings indicate the molt at which the megalopa phase appeared.
The zoeae required between 5 and 8 molts to reach the MG stage. The number of molts in which the organisms completed the larval cycle was related to the diet. In the 60/40, 50/50, and 40/60 diets, the first MGs were recorded beginning at the fifth molt (M5), while in the rest, they were recorded beginning at M6 (Figure 3). Extensive metamorphosis occurred at M7 (38.5-73.7%) and M8 (21.1-45.5%) in all diets, with no significant differences between them (P > 0.05) but a significantly higher level occurred than in M5 (P < 0.05; Figure 3).
Figure 3 Relationship of the total percentage of Callinectes sapidus larvae that reached the megalopa stage (in each enriched rotifer diet) with respect to the number of molts necessary to achieve it (M5, M6, M7 and M8). Different letters indicate significant differences in the diet (Friedman’s test and Conover’s post-test; P < 0.05).
The weight of the MG was between 0.72 ± 0.06 and 1.33 ± 0.05 mg ind.-1. The length varied from 2,632 ± 88.8 to 2,926 ± 118.5 µm ind.-1, while Le Cren´s condition factor (Kn) showed an interval from 0.83 ± 0.11 to 1.16 ± 0.12; significant differences (P < 0.05) were detected among diets (Table 3). The lowest values corresponded to the 100/0 diet, and the highest to the 40/60 diet. The difference in the weight of larvae fed the worst (100/0) and the best diets (40/60) was 46%, while that in size was 10% and that in Kn was 28%.
Table 3 Production response of Callinectes sapidus larvae fed whit enriched rotifers as live food (Brachionus plicatilis enriched for 24 h with Chlorella pyrenoidosa, Ch, and S.presso emulsion, Spr) in different proportions (Ch/Spr).
The protein content varied from 63.93 ± 0.67 to 67.50 ± 0.40 g 100 g-1 and was significantly lower (P < 0.05) in the 90/10 diet (Table 4). Lipids were more abundant in diets with the highest proportion of Spr; the interval was between 7.48 ± 0.20 and 11.47 ± 0.31 g 100 g-1, with significant differences (P < 0.05): the poorest diet was 100/0, and the richest was 50/50. Carbohydrates did not show a clear trend within diets (from 0.81 ± 0.11 to 1.38 ± 0.05 g 100 g-1); the lowest content was recorded in the 60/40 diet and the highest in the 50/50 diet (P < 0.05).
Table 4 Nutritional composition of enriched rotifers used as live food (Brachionus plicatilis enriched for 24 h with Chlorella pyrenoidosa, Ch, and S.presso emulsion, Spr) in different proportions (Ch/Spr).
The ash contents were superior in the diets with the highest proportion of Ch; the interval was between 6.90 ± 0.04 and 9.28 ± 0.01 g 100 g-1, with significant differences (P < 0.05). The energy value was also significantly different (P < 0.05), varying between 21.88 ± 0.04 and 23.38 ± 0.09 kJ g-1; the lowest level corresponded to the 100/0 diet and the highest to the 60/40 diet (Table 4).
Total lipids had positive and significant correlation coefficients with survival, weight, and size of MGs, as well as with Kn (r between 0.81 and 0.85; Table 5). Additionally, diet energy was positively correlated with survival and MG size (r between 0.77 and 0.79) and negatively correlated with the duration of the larval cycle (r = - 0.80). The ash content showed a significant negative correlation with survival and MG weight, size, and Kn (r between - 0.84 and -0.91). The correlation coefficient was nonsignificant for the protein and carbohydrate contents with the production response of C. sapidus larvae (r between -0.44 and 0.42 and between -0.04 and 0.34, respectively).
Table 5 Pearson’s linear correlation coefficients (r) between the enriched rotifer composition (diets), and the production response of Callinectes sapidus larvae (significant values P < 0.05, are in bold).
This work showed that the lipid content of enriched live rotifers was the most important factor in the production response of C. sapidus larvae reared in the laboratory. To conduct laboratory feeding studies, it is necessary to adapt the food size (prey) to the larvae. Previous reports from subtropical waters of the Western Atlantic recommended the use of Artemia nauplii for the larval culture of this species, but in this study, preliminary tests (unpublished) showed that C. sapidus larvae from the Colombian Caribbean region could not effectively capture and ingest them (size between 422 to 515 µm); even in later stages, they were only able to capture and consume rotifers (< 120 to > 200 µm). This prevented the use of previously reported feeding protocols (Zmora et al., 2005; Stuck et al., 2009; Maurer et al., 2017). This phenomenon has also been reported for Callinectes similis (Bookhout and Costlow, 1977) and C. danae (Guarizo et al., 2020).
The final survival percentages increased progressively from 6 to 34%, as the diet composition changed between 100/0 and 40/60, in correlation with the lipid content in the diets. In diets with lower lipid contents (100/0 to 70/30), the time to reach the MG phase was longer (> 55 days) compared to that in the rest of the treatments (< 51 days). The Spr product used to enrich rotifers contains fatty acids, including ω3 PUFAs and DHA/EPA; it has been demonstrated that HUFA incorporation into diets improves survival in crab larvae (Suprayudi et al., 2002; Hu et al., 2017; Shi et al., 2019). Previous studies of C. sapidus larval culture show that the time to reach the MG phase is variable. The origin of the larvae and other factors, such as temperature, salinity, photoperiod, culture tanks, and diet supplied, are involved and prevent comparisons (Costlow and Bookhout, 1959; Sulkin, 1975; Sulkin et al., 1976; Bookhout and Costlow, 1977; Sulkin and Van Heukelem, 1986; Bacab et al., 2002; Zmora et al., 2005; Maurer et al., 2017).
In all diets, the highest mortality (43 to 55%) was recorded during the first 13 days after hatching, between the first and second molts (M1 to M2). This phenomenon has been reported in C. sapidus during the first three zoea instars (Z1 to Z3), with ages of 6 to 16 days, and mortalities vary between 30 and 95% (Costlow and Bookhout, 1959; Costlow, 1967; Sulkin et al., 1976; Bacab et al., 2002). These events seem to be associated with the poor ability of the larvae to detect, capture and consume prey. Upon hatching, C. sapidus larvae consumed 45 to 61% of the lipids and 35 to 100% of the initially available proteins (Amsler and George, 1984; Li et al., 2012). A density of ~50 rotifers mL-1 is recommended to feed the first six instars of zoeae; in this way, abundant food is guaranteed, and the additional energy expenditure to search for prey is avoided (Maurer et al., 2017). In this study, the food density supplied was not considered a limiting factor (60 to 100 rot. mL-1); despite this, high mortality was recorded in this period (M1 to M2). This supports the hypothesis that there is no hunting behavior in the early stages. In several decapods, the zoeae move their maxillipeds to swim and generate currents that allow fortuitous encounters with possible prey (Epelbaum and Borisov, 2006; Caracappa and Munroe, 2018; Almeida et al., 2021). From M3 (days 15-19), feed intake may have increased and was associated with survival curve stabilization (Figure 1).
The molting cycle was related to the composition of the diet; the periods were generally longer in low-lipid diets. Considering that the larvae do not feed from the late premolt period to the early postmolt period (Anger, 2001; Lemos and Weissman, 2020), they must accumulate enough nutrients and energy to reach the reserve saturation point during the intermolt period; this guarantees that larvae will be able to complete the molt cycles (Gore, 1995; Anger, 2001). In this study, more than 55% of the zoeae survived the first molting event (M1), which took place between 6 and 9 days after hatching. For this to occur, it was assumed that the necessary energy could come from yolk reserves (Gore, 1995), supplied diets, or a combination of both, as occurred in other decapod larvae (Gore 1985; Anger, 1987; Anger and Spindler, 1987). To achieve subsequent molts (M2 to MG), the larvae will depend at some point only on the availability of nutrients in the diets and their ability to capture food.
Between M1 and M4, the zoeae molted approximately every five days, regardless of diet; subsequently, differences were found: Diets with compositions between 100/0 and 70/30 showed the widest intervals, and these treatments can be considered deficient in supplying the nutritional requirements necessary to molt. In each molting cycle, during temporary starvation, the blister-like cells absorb the nutrients discharged by the resorptive cells in the hepatopancreas to support the organism, which causes a notable decrease in the weight of this organ (Jones et al., 1997; Anger, 2001), and these losses can only be replaced when the larvae feed (Gore, 1995; Anger, 2001). Therefore, if the nutrients supplied are not those required or do not have the appropriate concentrations, the larvae will need more time to replenish the lost biomass and reach the necessary threshold to supply their energy reserves. In Hyas araneus, Carcinus maenas and other decapods, this phenomenon delays the production of the molting hormone and the onset of integumentary apolysis (Anger and Dawirs, 1981; Dawirs, 1984; Anger, 1987; Anger and Spindler, 1987; Suprayudi et al., 2002; Gimenez and Anger, 2005).
Although the ontogenetic development of crustaceans is genetically determined, several decapod species exhibit some degree of plasticity through the addition or omission of stages (Maurer et al., 2017), especially in carideans and brachyurans, which present numerous larval stages (Oliphant and Thatje, 2013; Sugumar et al., 2013). In crustaceans, molts are used to define successive larval stages because changes in size or morphology usually occur; however, in some cases, molting is not associated with significant modifications and sometimes not even with size increments (Anger, 2001; Moller et al., 2020).
In this study, up to eight zoea instars were recorded, and metamorphosis to MG occurred between M5 and M8. In the diets with higher lipid contents (60/40, 50/50, and 40/60), MG were observed beginning in M5, although at very low percentages (< 1.4%). This early presence was also reported by Maurer et al. (2017), although at a higher proportion (10 to 40%). Studies carried out for C. sapidus reported between six and nine stages for zoeae to reach the MG stage, although the highest occurrence was recorded in M7 and M8 (Costlow and Bookhout, 1959; Costlow, 1965; Sulkin, 1975; Sulkin et al., 1976; Bacab et al., 2002; Zmora et al., 2005; Stuck et al., 2009; Maurer et al., 2017), as occurred in the present bioassay.
Each diet produced a specific physiological response, which was reflected in the final length and weight of MGs. Both variables were combined in Le Cren´s condition factor (Kn), which can be used as an indicator of health (Le Cren, 1951). This study showed that the best Kn values coincided with the highest inclusion of Spr (40/60 and 50/50), however i t would have enhanced the study to observe the extended viability of MG under conditions of starvation as an additional indicator of their fitness to complement Kn. This condition factor has been used in a few species of adult brachyurans, but there are no reports for larvae (Amaro and Fiscarelli, 2009; Fassatoui et al., 2021). It has been proposed that this type of morphometric measurement, complemented with lipid content, can be used as a first step to assess the availability of energy reserves in larvae (Lloret et al., 2013).
The positive correlation between the lipid content in the diets and survival and MG weight, length, and Kn reinforces the importance of lipids (particularly HUFAs) in C. sapidus larval development. The negative correlation (r = - 0.80) between the duration of the larval cycle and the energy content of the diets is related to the accumulation of reserves and their conversion to energy, factors involved in the endocrine regulation of the molt cycle (Anger, 1987). Although the content of fatty acids was not determined in this research, it is assumed that the positive effect was associated with the content of lipids indicated in the Spr datasheet (150 mg g-1 ω3 PUFAs and 9 DHA/EPA).
In this experiment, the amount of lipids was between 7.48 ± 0.20 and 11.47 ± 0.31 g 100 g-1, and it was the most important factor in the production response of C. sapidus larvae. Similar concentrations have been tested in Scylla sp. and Portunus sp. larval cultures (6.8 to 16%); in those studies, it was shown that HUFA-unbalanced diets produced low survival rates and a high incidence of “death by molting syndrome” (Wu et al., 2014; Hu et al., 2017; Shi et al., 2019). On the other hand, it was considered that the enrichment period of the rotifers was adequate since Rodríguez et al. (1996) and Li and Olsen (2015) indicated that allowing 24 h to enrich B. plicatilis maximizes the incorporation of lipids into the tissues and allows them to remain stable until one day later.
The protein concentration requirements in decapod larval diets oscillate from 30 to 80%, depending on species, stage, food source, and digestibility (Holme et al., 2007; Shi et al., 2019; Jeffs and O´Rorke, 2020). The microalga Chlorella is often used to cultivate fish and crustacean larvae but is usually complemented with products rich in DHA and EPA to meet the needs of n-3 HUFAs (Taufik et al., 2016; Waqalevu et al., 2019), as was done in this investigation. In previous studies, the proximal composition of Chlorella-fed rotifers showed a wide range of protein (27.6 to 67.3%) and lipid (9.5 to 12%) contents (Dan and Hamasaki, 2011; Hamre, 2016; Waqalevu et al., 2019). The variations may be due to each experiment’s particular conditions and the enrichment period.
The Ch fractions used during the enrichment of the rotifers yielded protein levels between 63.93 ± 0.67 and 67.50 ± 0.40 g 100 g-1 within the required range, but the protein content in the diets did not show significant correlation coefficients with the production response. These results lead us to hypothesize that this variable was not limiting. Nevertheless, other studies could delve into the absorption, metabolism, and function of its amino acids in nutrition since there are few studies of this type in crustacean larval stages (Carter and Mente, 2014; Li et al., 2021).
It may be possible that lipid levels higher than those tested in the present study could further improve survival and growth rates of blue crab larvae. For example, the enrichment of rotifers with another commercial emulsion (EPA28®, Nippon Kagaku Shiryo Co., LTD, Japan) to feed other brachyuran species (Takeuchi et al., 1999; Suprayudi et al., 2002) showed that insertions between 10 to 40 µL L-1 resulted in higher rotifer lipid content (15.4 to 20.3%) than the present study (11.47%). With the results showing that the highest lipid levels 50/50 and 40/60 performed best, and protein levels were not limited, further investigations should focus on higher lipids and less or no proteins in enrichment formulas.
Although culture assays under laboratory conditions in C. sapidus larval have been developed (Zmora et al., 2005; Stuck et al., 2009; Maurer et al., 2017), the proximal composition of live prey and its relation with their larval development had not been covered until this work. The present study is the first to establish the preliminary bases on enriched live food nutritional content with the main variables of the larval development of C. sapidus, setting up the essential foundations of diets of known biochemical composition as a supply for C. sapidus larvae to parameterize their production response.
In conclusion, the evidence presented here indicates that B. plicatilis enrichment for 24 h with different Ch/Spr ratios significantly affects the larval development of C. sapidus in terms of survival, growth, and molting cycle. The diets with the highest lipid content (40/60 and 50/50) showed the best survival, duration of the larval cycle, size and weight of the megalopae, and short molting periods. The correlation coefficients indicated a significant relationship between the diet’s lipid, ash, and energy content and the larvae’s production response variables. Findings of the present study can be applied in future efforts towards mass culture of blue crab larvae in the Caribbean and can also potentially be used to study other crustacean species with similar characteristics.
The raw data supporting the conclusions of this article will be made available by the authors upon request, without undue reservation.
GO-S: Funding acquisition, laboratory assembly and maintenance, fieldwork, experimental design and development, data analysis, original manuscript writing, reviewing and editing. SZ: Funding acquisition, administration, experimental design, advice on statistical analysis, supervision, and reviewing. AM-B: Experimental design, supervision, writing, reviewing and editing. All authors read and approved the final version of the manuscript.
To the Colombian Ministry of Science, Technology, and Innovation —Minciencias, who funded the project: “Diet influence on digestive enzymatic activity and its genic expression during larval development of the blue crab Callinectes sapidus Rathburn, 1895 (Crustacea: Decapoda: Portunidae)” (grant: 110171451096, contract: 149-2016). To Universidad de Bogotá Jorge Tadeo Lozano, Marine Biology Program, Natural Science and Engineering Faculty, who funded the project: “Evaluation of cultivation potential of the blue crab Callinectes sapidus larvae (Decapoda: Brachyura), under laboratory conditions, phase I” (grant: 746-13-16). This study is part of the Doctoral thesis of GHOS, supported by COLCIENCIAS grant (call No. 647–2014), and the Graduate Program in Marine Biology, Universidad Nacional de Colombia - Caribbean campus (CECIMAR contribution No. 559). The rotifers were donated by the Department of Aquatic Sciences of the University of Córdoba, and the microalgae by Ceniacua Company.
The authors declare that the research was conducted in the absence of any commercial or financial relationships that could be construed as a potential conflict of interest.
All claims expressed in this article are solely those of the authors and do not necessarily represent those of their affiliated organizations, or those of the publisher, the editors and the reviewers. Any product that may be evaluated in this article, or claim that may be made by its manufacturer, is not guaranteed or endorsed by the publisher.
Almeida E. V. D., Cardoso C. D. S., Souza M. D. S., Bonecker S. L. C. (2021). Swimming behavior of newly hatched larvae of six decapod species (Crustacea: decapoda). Nauplius 29, 1–12. doi: 10.1590/2358-2936e2021023
Amaro M. A., Fiscarelli A. G. (2009). Length-weight relationship and condition factor of the mangrove crab Ucides cordatus (Linnaeus 1763) (Crustacea, brachyura, ucididae). Braz. Arch. Biol. Techn. 52, 397–406. doi: 10.1590/S1516-89132009000200017
Amsler M. O., George R. Y. (1984). Seasonal variation in the biochemical composition of the embryos of Callinectes sapidus rathbun. J. Crustacean Biol. 4, 546–553. doi: 10.2307/1548068
Andrés M., Estévez A., Hontoria F., Rotllant G. (2010). Differential utilization of biochemical components during larval development of the spider crab Maja brachydactyla (Decapoda: majidae). Mar. Biol. 157, 2329–2340. doi: 10.1007/s00227-010-1499-0
Anger K. (1987). The D0 threshold: a critical point in the larval development of decapod crustaceans. J. Exp. Mar. Biol. Ecol. 108, 15–30. doi: 10.1016/0022-0981(87)90128-6
Anger K. (2001). “The biology of decapod crustacean larvae,” in Crustacean issues, vol. Vol. 14. (Pennsylvania: Balkema Publishers).
Anger K., Dawirs R. R. (1981). Influence of starvation on the larval development of Hyas araneus (Decapoda, majidae). Helgolander Meeresun. 34, 287–311. doi: 10.1007/BF02074124
Anger K., Spindler K. D. (1987). Energetics, moult cycle and ecdysteroid titers in spider crab (Hyas araneus) larvae starved after the D0 threshold. Mar. Biol. 94, 367–375. doi: 10.1007/BF00428242
A.O.A.C (2000). Official methods of analysis (Washington: T he Association of Official Analytical Chemists).
A.O.A.C (2005). Official methods of analysis (Arlington: T he Association of Official Analytical Chemists).
Arguelles E. (2021). Biochemical composition and bioactive properties of Chlorella minutissima (Chm1) as a potential source of chemical compounds for nutritional feed supplement and disease control in aquaculture. Curr. Appl. Sci. Techn. 21, 65–77.
Ashraf M. Y., Javed Iqbal M., Naqvi S. A. (2011). Replacement of expensive pure nutritive media with low cost commercial fertilizers for mass culture of freshwater algae, Chlorella vulgaris. Int. J. Agric. Biol. 13, 484–490.
Bacab F. J., Amador L. E., Valdes R., Cabrera P. (2002). Cultivo de larvas de la jaiba azul Callinectes sapidus en condiciones de laboratorio en la isla del Carmen, campeche, méxico. I Congreso Iberoamericano Virtual Acuicultura - CIVA 2002 122-128.
Basford J. A., Makings N., Mos B., White C. A., Dworjanyn S. (2021). Greenwater, but not live feed enrichment, promotes development, survival, and growth of larval Portunus armatus. Aquaculture 534, 736331. doi: 10.1016/j.aquaculture.2020.736331
Bembe S., Liang D., Chung J. S. (2017). Optimal temperature and photoperiod for the spawning of blue crab, Callinectes sapidus, in captivity. Aquac. Res. 48, 5498–5505. doi: 10.1111/are.13366
Bookhout C., Costlow J. D. (1977). Larval development of Callinectes similis reared in the laboratory. B. Mar. Sci. 27, 704–728.
Brito R., Rosas C., Chimal M. E., Gaxiola G. (2001). Effect of different diets on growth and digestive enzyme activity in Litopenaeus vannamei (Boone 1931) early post-larvae. Aquac. Res. 32, 257–266. doi: 10.1046/j.1365-2109.2001.00548.x
Brucet S., Boix D., López-Flores R., Badosa A., Quintana X. D. (2005). Ontogenic changes of amino acid composition in planktonic crustacean species. Mar. Biol. 148, 131–139. doi: 10.1007/s00227-005-0068-4
Calado R., Carvalho L., Rodrigues A. C. M., Abe F., Silva A. L. P., Soares A. M. V. M., et al. (2022). The physiological consequences of delaying metamorphosis in the marine ornamental shrimp Lysmata seticaudata and its implications for aquaculture. Aquaculture 546, 737391. doi: 10.1016/j.aquaculture.2021.737391
Caracappa J. C., Munroe D. M. (2018). Morphological variability among broods of first-stage blue crab (Callinectes sapidus) zoeae. Biol. Bull. 235, 123–133. doi: 10.1086/699922
Carter C. G., Mente E. (2014). Protein synthesis in crustaceans: a review focused on feeding and nutrition. Cent. Eur. J. Biol. 9, 1–10. doi: 10.2478/s11535-013-0134-0
Costlow J. D. (1965). Variability in larval stages of the blue crab, Callinectes sapidus. Biol. Bull. 128, 58–66. doi: 10.2307/1539389
Costlow J. D. (1967). The effect of salinity and temperature on survival and metamorphosis of megalops of the blue crab Callinectes sapidus. Helgoland. Wiss. Meer . 15, 84–97. doi: 10.1007/BF01618611
Costlow J. D., Bookhout C. G. (1959). The larval development of Callinectes sapidus rathbun reared in the laboratory. Biol. Bull. 116, 373–396.
Daly B. J., Eckert G. L., Long W. C. (2021). Moulding the ideal crab: implications of phenotypic plasticity for crustacean stock enhancement. ICES J. Mar. Sci. 78, 421–434. doi: 10.1093/icesjms/fsaa043
Dan S., Hamasaki K. (2011). Effects of salinity and dietary n-3 highly unsaturated fatty acids on the survival, development, and morphogenesis of the larvae of laboratory-reared mud crab Scylla serrata (Decapoda, portunidae). Aquacult. Int. 19, 323–338. doi: 10.1007/s10499-010-9374-z
Dawirs R. R. (1984). Influence of starvation on larval development of Carcinus maenas l. (Decapoda: portunidae). J. Exp. Mar. Biol. Ecol. 80, 47–66. doi: 10.1016/0022-0981(84)90093-5
DuBois M., Gilles K. A., Hamilton J. K., Rebers P. A., Smith F. (1956). Colorimetric method for determination of sugars and related substances. Anal. Chem. 28, 350–356. doi: 10.1021/ac60111a017
Epelbaum A., Borisov R. (2006). Feeding behaviour and functional morphology of the feeding appendages of red king crab Paralithodes camtschaticus larvae. Mar. Biol. Res. 2, 77–88. doi: 10.1080/17451000600672529
Fassatoui C., Hatira S., Romdhane M. S. (2021). Size-weight relationships and condition factor of the invasive Atlantic blue crab Callinectes sapidus rathbun 1896 (Decapoda: brachyura: portunidae) from northern Tunisia: a preliminary investigation. J. Crustacean Biol. 41, ruab039. doi: 10.1093/jcbiol/ruab039
Fu Z., Yang R., Zhou S., Ma Z., Zhang T. (2021). Effects of rotifers enriched with different enhancement products on larval performance and jaw deformity of golden pompano larvae Trachinotus ovatus (Linnaeus 1758). Front. Mar. Sci. 7. doi: 10.3389/fmars.2020.626071
Genodepa J., Zeng C., Southgate P. C. (2004). Preliminary assessment of a microbound diet as an Artemia replacement for mud crab, Scylla serrata, megalopa. Aquaculture 236, 497–509. doi: 10.1016/j.aquaculture.2004.02.007
Gimenez L., Anger K. (2005). Effects of temporary food limitation on survival and development of brachyuran crab larvae. J. Plankton Res. 27, 485–494. doi: 10.1093/plankt/fbi024
Gore R. H. (1995). “Molting and growth in decapod larvae,” in Crustacean issues 2: larval growth. Ed. Wennwe A. (Rotterdam, Netherlands: Balkema Publishers), 1–66.
Guarizo M., Costa T. M., Marochi M. Z. (2020). Effect of diet during larval development of menippe nodifrons stimpson 1859 and Callinectes danae smith 1869. Aquacult. Int. 28, 1969–1980. doi: 10.1007/s10499-020-00569-2
Hamre K. (2016). Nutrient profiles of rotifers (Brachionus sp.) and rotifer diets from four different marine fish hatcheries. Aquaculture 450, 136–142. doi: 10.1016/j.aquaculture.2015.07.016
Hamre K., Srivastava A., Ronnestad I., Mangor-Jensen A., Stoss J. (2008). Several micronutrients in the rotifer Brachionus sp. may not fulfil the nutritional requirements of marine fish larvae. Aquacult. Nutr. 14, 51–60. doi: 10.1111/j.1365-2095.2007.00504.x
Hill J., Fowler D. L., Van Den Avyle M. J. (1989) Species profiles: life histories and environmental requirements of coastal fishes and invertebrates (Mid-Atlantic) - blue crab. vol. 82. No.11. biological report (Vicksburg, Misisipi: US Fish and Wildlife Services). Available at: https://apps.dtic.mil/sti/pdfs/ADA210181.pdf (Accessed 4 July 2022).
Holme M. H., Southgate P. C., Zeng C. (2007). Survival, development and growth response of mud crab, Scylla serrata, megalopae fed semi-purified diets containing various fish oil: corn oil ratios. Aquaculture 269, 427–435. doi: 10.1016/j.aquaculture.2007.05.024
Holme M. H., Zeng C., Southgate P. (2009). A review of recent progress toward development of a formulated microbound diet for mud crab, Scylla serrata, larvae and their nutritional requirements. Aquaculture 286, 164–175. doi: 10.1016/j.aquaculture.2008.09.021
Hu S., Wang J., Han T., Li X., Jiang Y., Wang C. (2017). Effects of dietary DHA/EPA ratios on growth performance, survival and fatty acid composition of juvenile swimming crab (Portunus trituberculatus). Aquacult. Res. 48, 1291–1301. doi: 10.1111/are.12971
Jeffs A., O´Rorke R. (2020). “Chapter 11: feeding and nutrition of crustacean larvae,” in Developmental biology and larval ecology, vol. Volume 7 . Eds. Anger K., Harzsch S., Mhiel M. (New York: Oxford University Press), 309–331.
Jones D. A., Yule A. B., Holland D. L. (1997). “Larval nutrition,” in Crustacean nutrition. Eds. D’Abramo L. R., Conklin D. E., Akiyama D. M. (Louisiana: World Aquaculture Society), 353–389.
Khoa T. N. D., Waqalevu V., Honda A., Matsui H., Truong N. X., Sakaguchi K., et al. (2021). Enrichment effects of fermented by-product of shochu distillery on brachionus plicatilis sp. rotifer and larviculture performance in Japanese flounder (Paralichthys olivaceus). Aquaculture 535, 736352. doi: 10.1016/j.aquaculture.2021.736352
Le D. V. B., Nguyen P. N., Dierckens K., Nguyen D., De Schryver P., Hagiwara A., et al. (2017). Growth performance of the very small rotifer Proales similis is more dependent on proliferating bacterial community than the bigger rotifer Brachionus rotundiformis. Aquaculture 476, 185–193. doi: 10.1016/j.aquaculture.2017.03.046
Le Cren E. D. (1951). The length-weight relationship and seasonal cycle in gonad weight and condition in the perch (Perca fluviatilis). J. Anim. Ecol. 20, 201–219. doi: 10.2307/1540
Lemos D., Weissman D. (2020). Moulting in the grow-out of farmed shrimp: a review. Rev. Aquacult. 13, 5–17. doi: 10.1111/raq.12461
Li S., Cheng Y., Zhou B., Hines A. H. (2012). Changes in biochemical composition of newly spawned eggs, prehatching embryos and newly hatched larvae of the blue crab Callinectes sapidus. J. Shellfish Res. 31, 941–946. doi: 10.2983/035.031.0405
Li X., Han T., Zheng S., Wu G. (2021). “Nutrition and functions of amino acids in aquatic crustaceans,” in Amino acids in nutrition and health: advances in experimental medicine and biology, vol. vol 1285 . Ed. Wu G. (Switzerland: Springer Nature), 169–198.
Li K., Kjørsvik E., Bergvik M., Olsen Y. (2014). Manipulation of the fatty acid composition of phosphatidylcholine and phosphatidylethanolamine in rotifers Brachionus nevada and Brachionus cayman. Aquacult. Nutr. 21, 85–97. doi: 10.1111/anu.12140
Li K., Olsen Y. (2015). Effect of enrichment time and dietary DHA and non-highly unsaturated fatty acid composition on the efficiency of DHA enrichment in phospholipid of rotifer (Brachionus cayman). Aquaculture 446, 310–317. doi: 10.1016/j.aquaculture.2015.05.005
Liu S., Wang X., Bu X., Zhang C., Qiao F., Qin C., et al. (2021). Influences of dietary vitamin D3 on growth, antioxidant capacity, immunity and molting of Chinese mitten crab (Eriocheir sinensis) larvae. J. Steroid Biochem. 210, 105862. doi: 10.1016/j.jsbmb.2021.105862
Lloret J., Shulman G., Love R. M. (2013). Condition and health indicators of exploited marine fishes (Oxford: John Wiley & Sons Ltd).
Louvado A., Castro C., Silva D. A. M., Oliveira V., Conceição L. E. C., Cleary D. F. R., et al. (2023). Assessing the effects of rotifer feed enrichments on turbot (Scophthalmus maximus) larvae and post-larvae gut-associated bacterial communities. Microorganisms 11, 520. doi: 10.3390/microorganisms11020520
Maestre E. V., Girón A., Alvarez J., Salas S., Escobar-Toledo F. D., Romero J. A., et al. (2022)Capítulo III: causas y tensores del cambio en los ecosistemas marinos y costeros y sus servicios: indicadores de presión. In: Informe del estado de los ambientes y recursos marinos y costeros en Colombia 2021. serie de publicaciones periódicas no. 3 (Santa Marta: Ediprint, S.A.S). Available at: http://www.invemar.org.co/redcostera1/invemar/docs/ier_2015_baja.pdf (Accessed 18 March 2023).
Mantelatto F. L., Reigada A. L. D., Gatti A. C. R., Cuesta J. A. (2014). Morphology of the first zoeal stages of five species of the portunid genus Callinectes (Decapoda, brachyura) hatched at the laboratory. An. Acad. Bras. Ciênc. 86, 755–767. doi: 10.1590/0001-3765201420130030
Maruyama I., Nakao T., Shigeno I., Ando Y., Hirayama K. (1997)Application of unicellular algae chlorella vulgaris for the mass-culture of marine rotifer brachionus. In: Live food in aquaculture. developments in hydrobiology (Dordrecht: Springer-Science) (Accessed 3 July 2022).
Maurer L., Liang D., Chung J. S. (2017). Effects of prey densities and dietary supplementation on the larval development of the blue crab Callinectes sapidus rathbun 1896 (Brachyura: portunidae). J. Crustacean Biol. 37, 674–682. doi: 10.1093/jcbiol/rux079
Moller O. S., Anger K., Guerao G. (2020). “Patterns of larval development, in developmental biology and larval ecology,” in The natural history of the Crustacea, vol. vol. 7 . Eds. Anger K., Harzsch S., Thiel M. (New York: Oxford University Press), 165–194.
Nordgreen A., Penglase S., Hamre K. (2013). Increasing the levels of the essential trace elements Se, zn, Cu and Mn in rotifers (Brachionus plicatilis) used as live feed. Aquaculture 380–383, 120–129. doi: 10.1016/j.aquaculture.2012.11.032
Oliphant A., Thatje S. (2013). Per offspring investment implications for crustacean larval development: evolutionary insights into endotrophy and abbreviated development. Mar. Ecol.- Prog. Ser. 493, 207–217. doi: 10.3354/meps10496
Pedroza-Islas R., Gallardo P., Vernon-Carter E. J., Garcia-Galano T., Rosas C., Pascual C., et al. (2004). Growth, survival, quality and digestive enzyme activities of larval shrimp fed microencapsulated, mixed and live diets. Aquacult. Nutr. 10, 167–173. doi: 10.1111/j.1365-2095.2004.00284.xC
Pletl J. J. (1992) The growth and bioenergetics of callinectes sapidus larvae and the effects of diet quality on larval physiology (Norfolk: Old Dominion University). Available at: https://digitalcommons.odu.edu/oeas_etds/148?utm_source=digitalcommons.odu.edu%2Foeas_etds%2F148&utm_medium=PDF&utm_campaign=PDFCoverPages (Accessed 25 March 2022). Disertation/ PhD thesis.
Raja R., Coelho A., Hemaiswarya S., Kumar P., Carvalho I. S., Alagarsamy A. (2018). Applications of microalgal paste and powder as food and feed: an update using text mining tool. Beni-Suef Univ. J. Basic. Appl. Sci. 7, 740–747. doi: 10.1016/j.bjbas.2018.10.004
Rodríguez C., Pérez J. A., Izquierdo M. S., Cejas J. R., Bolaños A., Lorenzo A. (1996). Improvement of the nutritional value of rotifers by varying the type and concentration of oil and the enrichment period. Aquaculture 147, 93–105. doi: 10.1016/S0044-8486(96)01397-X
Shan X., Lin M. (2014). Effects of algae and live food density on the feeding ability, growth and survival of miiuy croaker during early development. Aquaculture 428–429, 284–289. doi: 10.1016/j.aquaculture.2014.03.021
Shi C., Zeng T., Li R., Wang C., Ye Y., Mu C. (2019). Dynamic metabolite alterations of Portunus trituberculatus during larval development. J. Ocean. Limnol. 37, 361–372. doi: 10.1007/s00343-019-7268-0
Singh P. K., Munilkumar S., Sundaray J. K., Santhanam P., Sharma A., Haque R., et al. (2023). Effect of selenium, vitamin c and highly unsaturated fatty acids- enriched Brachionus calyciflorus on growth, survival, physio-metabolic and anti-oxidative responses in anabas testudineus (Bloch 1792) larvae. Aquaculture 568, 739293. doi: 10.1016/j.aquaculture.2023.739293
Srivastava A., Stoss J., Hamre K. (2011). A study on enrichment of the rotifer brachionus “Cayman” with iodine and selected vitamins. Aquaculture 319, 430–438. doi: 10.1016/j.aquaculture.2011.07.027
Stuck K., Perry H., Graham D., Heard R. W. (2009). Morphological characteristics of early life history stages of the blue crab, Callinectes sapidus rathbun, from the northern gulf of Mexico with a comparison of studies from the Atlantic seaboard. Gulf Caribbean Res. 21, 37–55. doi: 10.18785/gcr.2101.05
Sugumar V., Vijayalakshmi G., Saranya K. (2013). Molt cycle related changes and effect of short-term starvation on the biochemical constituents of the blue swimmer crab. Portunus pelagicus. Saudi J. Biol. Sci. 20, 93–103. doi: 10.1016/j.sjbs.2012.10.003
Sui L., Wille M., Cheng Y., Sorgeloos P. (2007). The effect of dietary n-3 HUFA levels and DHA/EPA ratios on growth, survival and osmotic stress tolerance of Chinese mitten crab Eriocheir sinensis larvae. Aquaculture 273, 139–150. doi: 10.1016/j.aquaculture.2007.09.016
Sulkin S. D. (1975). The significance of diet in the growth and development of larvae of the blue crab, Callinectes sapidus rathbun, under laboratory conditions. J. Exp. Mar. Biol. Ecol. 20, 119–135. doi: 10.1016/0022-0981(75)90019-2
Sulkin S. D., Branscomb E. S., Miller R. E. (1976). Induced winter spawning and culture of larvae of the blue crab, Callinectes sapidus rathbun. Aquaculture 8, 103–113. doi: 10.1016/S0044-8486(76)80067-1
Sulkin S. D., Van Heukelem W. F. (1986). Variability in the length of the megalopal stage and its consequence to dispersal and recruitment in the portunid crab Callinectes sapius rathbun. Bull. Mar. Sci. 39, 269–278.
Suprayudi M. A., Takeuchi T., Hamasaki K., Hirokawa J. (2002). The effect of n-3HUFA content in rotifers on the development and survival of mud crab, Scylla serrata, larvae. Aquacult. Sci. 50, 205–212. doi: 10.11233/aquaculturesci1953.50.205
Takeuchi T., Nakamoto Y., Hamasaki K., Sekiya S., Watanabe T. (1999). Requirement of n-3 highly unsaturated fatty acids for larval swimming crab Portunus trituberculatus. Nippon Suisan Gakk. 65, 797–803. doi: 10.2331/suisan.65.797
Taufik M., Bachok Z., Azra M. N., Ikhwanuddin M. (2016). Effects of various microalgae on fatty acid composition and survival rate of the blue swimming crab Portunus pelagicus larvae. Indian J. Geo.- Mar. Sci. 45, 1512–1521.
Tian H., Yang C., Yu Y., Yang W., Lu N., Wang H., et al. (2020). Dietary cholesterol level affects growth, molting performance and ecdysteroid signal transduction in Procambarus clarkii. Aquaculture 523, 735198. doi: 10.1016/j.aquaculture.2020.735198
Tucker R. K., Costlow J. D. (1975). Free amino acid changes in normal and eyestalkless megalopa larvae of the blue crab, Callinectes sapidus, during the course of the molt cycle. Comp. Biochem. Phys. A 51, 75–78. doi: 10.1016/0300-9629(75)90415-6
Wang J., Shu X., Wang W. X. (2019). Micro-elemental retention in rotifers and their trophic transfer to marine fish larvae: influences of green algae enrichment. Aquaculture 499, 374–380. doi: 10.1016/j.aquaculture.2018.09.066
Waqalevu V., Honda A., Dossou S., Khoa T. N. D., Matsui H., Mzengereza K., et al. (2019). Effect of oil enrichment on Brachionus plicatilis rotifer and first feeding red sea bream (Pagrus major) and Japanese flounder (Paralichthys olivaceus). Aquaculture 510, 73–83. doi: 10.1016/j.aquaculture.2019.05.039
Wu X. G., Zeng C. S., Southgate P. C. (2014). Ontogenetic patterns of growth and lipid composition changes of blue swimmer crab larvae: insights into larval biology and lipid nutrition. Mar. Freshw. Res. 65, 228–243. doi: 10.1071/MF13078
Zarantoniello M., Chemello G., Ratti S., Pulido-Rodríguez L. F., Daniso E., Freddi L., et al. (2023). Growth and welfare status of giant freshwater prawn (Macrobrachium rosenbergii) post-larvae reared in aquaponic systems and fed diets including enriched black soldier fly (Hermetia illucens) prepupae meal. Animals 13, 715. doi: 10.3390/ani13040715
Keywords: Callinectes sapidus, blue crab larval development, rotifer enrichment, live food enrichment, rotifer proximal composition
Citation: Ospina-Salazar GH, Miranda-Baeza A and Zea S (2023) Larval development of the blue crab Callinectes sapidus: effect of enriched rotifers as live food. Front. Aquac. 2:1189325. doi: 10.3389/faquc.2023.1189325
Received: 18 March 2023; Accepted: 21 April 2023;
Published: 02 May 2023.
Edited by:
Charles Weirich, NOAA National Sea Grant Office, United StatesReviewed by:
Robert David Roer, University of North Carolina Wilmington, United StatesCopyright © 2023 Ospina-Salazar, Miranda-Baeza and Zea. This is an open-access article distributed under the terms of the Creative Commons Attribution License (CC BY). The use, distribution or reproduction in other forums is permitted, provided the original author(s) and the copyright owner(s) are credited and that the original publication in this journal is cited, in accordance with accepted academic practice. No use, distribution or reproduction is permitted which does not comply with these terms.
*Correspondence: Gloria Helena Ospina-Salazar, Z2hvc3BpbmFzQHVuYWwuZWR1LmNv
Disclaimer: All claims expressed in this article are solely those of the authors and do not necessarily represent those of their affiliated organizations, or those of the publisher, the editors and the reviewers. Any product that may be evaluated in this article or claim that may be made by its manufacturer is not guaranteed or endorsed by the publisher.
Research integrity at Frontiers
Learn more about the work of our research integrity team to safeguard the quality of each article we publish.