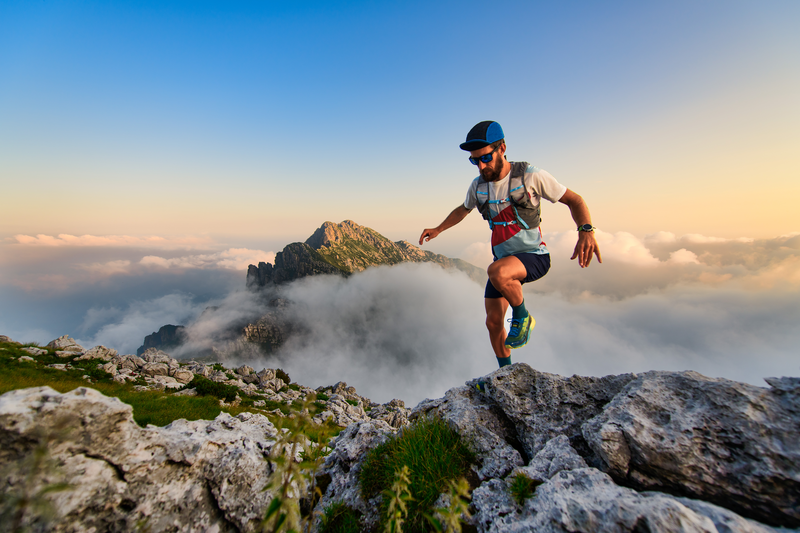
95% of researchers rate our articles as excellent or good
Learn more about the work of our research integrity team to safeguard the quality of each article we publish.
Find out more
MINI REVIEW article
Front. Antibiot. , 03 September 2024
Sec. Antibiotic Resistance
Volume 3 - 2024 | https://doi.org/10.3389/frabi.2024.1448071
This article is part of the Research Topic Acinetobacter spp. – Antibiotic Resistance, Infection Control and Novel Treatments View all 3 articles
Neonatal sepsis causes substantial morbidity and mortality, the burden of which is carried by low-income countries (LICs). The emergence of multidrug-resistant pathogens in vulnerable neonatal populations poses an urgent threat to infant survival. Acinetobacter spp. are increasingly responsible for severe disease in neonates globally. The cause of this escalation remains unclear, but host, pathogen and environmental factors are all likely to contribute. Acinetobacter spp. strains are frequently resistant to the first line empirical treatment for neonatal sepsis as recommended by the World Health Organization (WHO), ampicillin and gentamicin, rendering these antibiotics ineffectual in many critically ill neonates. The resultant escalation to broader spectrum antibiotic regimens in neonatal intensive care units (NICUs) worldwide has led to the emergence of more resistant strains, including carbapenem-resistant Acinetobacter baumanii (CRAB), resulting in infections that are ever more difficult to treat. While some existing antimicrobial agents are under consideration for treatment of Acinetobacter spp. infections, the majority remain a long way from clinical use in neonates. Further research into the clinical phenotype of these infections, transmission dynamics and preventative measures are urgently needed to reduce neonatal deaths. This review aims to summarise the role of Acinetobacter spp. in neonatal sepsis, including host, pathogen and environmental factors, the global epidemiology and clinical features of the disease, the treatment options, and future research priorities.
Neonatal sepsis, defined as a severe systemic infection occurring in newborn infants within the first 28 days of life, contributes substantially to neonatal mortality and morbidity worldwide (Fleischmann et al., 2021). The non-specific clinical features coupled with limited diagnostic test accuracy make clinical diagnosis of the condition challenging (Shane et al., 2017). Clinically the disease is often divided into early-onset sepsis (EOS), characterised by a positive blood or cerebrospinal fluid culture within the first 72 hours of life, and late-onset sepsis (LOS), which manifests after this initial period (Li et al., 2020; Sands et al., 2021). While EOS and LOS are traditionally thought to have different causative pathogens, a recent global observational cohort study found that all commonly identified pathogens were found in both EOS and LOS, with relatively minor differences in proportion (Russell et al., 2023). Risk factors for neonatal sepsis are primarily related to risk of pathogen exposure (e.g. maternal colonisation, prolonged rupture of membranes and NICU admission) and relative immaturity of immune system development (e.g. prematurity) (Shane et al., 2017).
In many cases of neonatal sepsis a causative organism is not definitively found, attributed in part to poor diagnostic test accuracy (Klingenberg et al., 2018). Blood cultures are seen as the ‘gold standard’ diagnostic test, however many factors reduce their diagnostic accuracy including the small volume of blood from neonatal samples, maternal antibiotic administration prior to delivery, and laboratory methodology (Camacho-Gonzalez et al., 2013). Causative pathogen distribution varies based on setting, with a predominance of Gram-negative pathogens worldwide, though with more Gram-positive pathogens (primarily Staphylococcal spp. and Streptococcal spp.) in higher income settings (Fleischmann et al., 2021). Longitudinal data from one South African neonatal unit show increasing Gram-negative prevalence over a 10-year period with static or falling rates of their Gram-positive counterparts (Thomas et al., 2024). Acinetobacter spp., primarily Acinetobacter baumanii, are increasingly implicated in the neonatal population, outpacing comparable Gram-negative pathogens such as P. aeruginosa. This review aims to propose some possible mechanisms for this increase, with consideration of microbial, host and environmental factors, to summarise clinical neonatal data, and to discuss current and novel treatment options, preventative measures and future research priorities.
The transition from an intrauterine to an extrauterine environment results in sudden exposure of neonates to environmental microbes. Microbial colonisation in the postnatal period is key to development of a healthy microbiome, with most ‘good’ bacteria originating from maternal flora and breast milk. The early microbiome contributes to immune system maturation (Sanidad and Zeng, 2020). However, this is a vulnerable time for colonisation with potentially harmful pathogens, given the relative absence of ‘good’ bacteria in the newborn’s gut and skin, particularly in neonates in nosocomial settings (Hartz et al., 2015). The neonatal gut is immature, with low levels of acid secretion, protective mucus and motility, resulting in an increased risk of bacterial translocation through the gut wall (Basu, 2015). Colonisation with Gram-negative bacteria is thought to increase the risk of invasive infection in neonates, though further evidence for this is needed for Acinetobacter spp. infection (Folgori et al., 2018). One study found the presence of Gram-negative bacteria in gastric aspirates and stool samples of hospitalised neonates significantly increased the chance of invasive infection with that same strain. Interestingly, of the neonates with invasive isolates, only 20% of those with A. baumanii isolated in blood cultures had previously been colonised with the same strain. For comparison, of neonates with Klebsiella pneumoniae isolated from their blood culture, 90% had been previously colonised with this same strain. This suggests that while gut colonisation may be linked to invasive Acinetobacter spp. infection, other sources of acquisition, without colonisation, may play a larger role compared to other common Gram-negative pathogens, though it is unclear if culture methods used may have been less appropriate for Acinetobacter spp. (Das et al., 2011). Additional studies with larger sample sizes are required to better characterise this association.
Multiple factors render neonates uniquely susceptible to infections. Neonates lack acquired immunity, resulting in a dependence on innate immune mechanisms, though the lack of a functional complement system means that even these responses are dampened, particularly in premature infants (McGreal et al., 2012; Hensler et al., 2022). Neonates are thought to have impaired neutrophil function, possibly due to a lower proportion of mature neutrophils when compared to older children and adults (Makoni et al., 2016). While the interactions between Acinetobacter spp. and the neonatal immune system are not well described, in adult populations neutropenic patients are particularly at risk, even when compared to other immunocompromised individuals (Karim et al., 1991; Chen, 2020). Immature neutrophil function may therefore contribute to neonatal vulnerability to Acinetobacter spp. infection, though the mechanisms behind this require exploration.
Acinetobacter spp. are Gram-negative coccobacilli with emerging importance in neonatal infections. The Acinetobacter calcoaceticus-baumannii (Acb) complex is a group of phenotypically indistinguishable pathogens including Acinetobacter baumannii, Acinetobacter pitii and Acinetobacter nosocomialis, among others. While there are reports of both Acb and non-Acb Acinetobacter spp. as causative organisms for neonatal sepsis, Acinetobacter baumannii is of particular clinical importance. For the purposes of this review, Acinetobacter spp. is used to encompass both Acb and non-Acb pathogens.
A. baumanii is one of six ESKAPE pathogens, named for their ability to escape the effects of antimicrobial drugs. These pathogens are the leading cause of nosocomial infections worldwide, and have been named high priority by the WHO. A. baumannii was designated in ‘critical’ need for novel antibiotic development (WHO, 2017).
The microbiological characteristics of Acinetobacter spp. partially explain its emergence as a neonatal pathogen. Firstly, Acinetobacter spp. are unique in the diversity of their ecological niche when compared to other pathogenic bacteria. In the natural world they are found in soil, rivers, animals and humans, reflecting the range of environments in which they thrive. One enabler of this is their resistance to external stresses including desiccation, which is rare among Gram-negative bacteria and confers a substantial survival advantage particularly in nosocomial settings (Jawad et al., 1998; Mea et al., 2021). Some isolates have been reported to remain viable for up to 3 months on dry surfaces (Wendt et al., 1997; Jawad et al., 1998). Though the mechanisms behind this tolerance are unclear, biofilm formation, in which capsular polysaccharides envelop the bacterial cells, is likely to contribute to the maintenance of bacterial cell hydration despite adverse external conditions (Espinal et al., 2012). In addition to desiccation resistance, Acinetobacter spp. also demonstrate some resistance to disinfectants, mediated by multidrug efflux pumps. Lanjri et al. (2017) found that from a range of clinical and environmental A.baumanii isolates, while all were susceptible to antiseptics and disinfectants in their purest form, clinical isolates required a higher concentration to achieve sterility than their environmental counterparts. These factors give Acinetobacter spp. an unparalleled ability to colonise nosocomial settings.
Secondly, Acinetobacter spp. have remarkable intrinsic and acquired resistance to antibiotics. Two key intrinsic β-lactamases, ABC and OXA-51, confer some resistance to penicillins, cephalosporins, and carbapenems (Bonomo and Szabo, 2006; Zavascki et al., 2010). However, without additional acquired resistance mechanisms they remain relatively susceptible. For example, strains described in the 1970s were successfully treated with agents such as ampicillin, chloramphenicol or gentamicin (Vázquez-López et al., 2020). The more recent emergence of multidrug resistant strains can be attributed to exceptional genetic plasticity, enabling Acinetobacter spp. to rapidly give and receive antibiotic resistance genes (ARGs) via horizontal gene transfer (Da Silva and Domingues, 2016). Carbepenem-resistant Acinetobacter Baumanii (CRAB) first emerged in the 1990s, primarily in adult intensive care settings, and has since emerged in NICUs worldwide. OXA23 is the most common carbapenem resistance gene, making up 75% of plasmid-acquired OXAs in Acinetobacter spp., though OXA 40, 153 and 235 are also frequently identified (Vázquez-López et al., 2020). One study comparing 20 environmental and clinical isolates of A.baumanii found the clinical isolates to demonstrate significantly higher antibiotic resistance than the environmental isolates, with 12/13 (92%) of clinical isolates characterised as extensively drug resistant (XDR), compared to 1/7 (14%) of environmental isolates (Havenga et al., 2022).
Finally, Acinetobacter spp. have a number of virulence factors that enable pathogen success at every stage of pathogenesis. These include outer membrane proteins (Omps) to facilitate cell membrane attachment and a Type VI secretion system to kill competitor bacterial cells (Shadan et al., 2023). The plastic genome supports transfer of virulence genes from bacteria to bacteria, enabling rapid evolution in response to changing environments and stressors.
The NICU environment contributes significantly to neonatal infection risk. First, it confers a high risk of exposure to multidrug resistant bacteria. Overcrowded units in under-resourced settings are at particular risk, often lacking the space, capacity or funds to institute regular, effective disinfection of surfaces or equipment. Invasive procedures, such as central vascular access and endotracheal intubation, further increase risk of exposure given their penetration of natural immune barriers such as the skin. Some NICUs are forced to care for multiple patients in single incubators or cots, further increasing risk of bacterial transmission between patients. Many neonatal pathogens thrive in one environmental niche within a NICU setting, for example Pseudomonas aeruginosa is associated with water reservoirs, and has therefore been frequently identified in humidified ventilator tubing and taps in neonatal units (Jefferies et al., 2012). The unique adaptability of Acinetobacter spp. enables it to occupy multiple different environmental niches within the neonatal unit, including both moist and dry environments, which may explain its growth in these settings. Reports of Acinetobacter spp. colonisation on neonatal units include detection from wound and device dressings, sinks and dry medical equipment such as syringe drivers (Tsiatsiou et al., 2015; Woon et al., 2023). The lack of a singular environmental niche complicates the design of control interventions aimed at preventing NICU-based transmission of Acinetobacter spp.
A PubMed search was performed to identify systematic reviews and meta-analyses reporting on causative organisms of neonatal sepsis globally. Table 1 lists the identified systematic reviews that reported Acinetobacter spp. as an invasive neonatal isolate. In the six publications identified Acinetobacter spp. infections were reported to comprise an overall proportion 1-6% of all cases of culture-positive neonatal sepsis included in the analysis. The highest overall prevalence was reported in an analysis of 20,828 positive cultures from low and lower-middle income countries globally (Wen et al., 2021). Okomo et al., who collated data from 151 Sub-Saharan African countries, reported that Central African countries were found to have the highest prevalence (11%), while West African countries had the lowest (2%). Regional variation is marked and Wen et al. concluded that the prevalence of invasive Acinetobacter spp. neonatal infections is higher in Asia than in Africa, a finding which is reflected by DENIS (2016), which reported a 22% Acinetobacter spp. prevalence in culture-positive neonatal sepsis among multiple sites in Delhi, India DENIS, 2016. A number of regional studies, for example in Cuba, China and Nigeria, found no Acinetobacter spp. in neonatal isolates, further reflecting the uneven global distribution (Medugu et al., 2018; Oliva et al., 2021). While the underlying causes for this global distribution remain unclear, regional antibiotic prescribing practices may provide a positive selective pressure. Unit to unit variation is noted even between units in similar geographical regions, supporting the hypothesis that unit-level colonisation may drive infections in the neonatal population (Sands et al., 2021; Russell et al., 2023).
Table 1. Systematic review and meta-analyses including Acinetobacter spp. proportion among culture-positive cohorts of neonates with sepsis.
There is limited published evidence on risk factors and clinical features of Acinetobacter spp. infection in a neonatal population, compared to other causative organisms. One global meta-analysis on risk factors for carbapenem and polymixin-resistant infections in neonates found that intubation, prematurity, low birth weight and mechanical ventilation were common risk factors for these infections in almost all countries (Osei Sekyere et al., 2021). Other studies report central venous lines and previous antibiotic use as additional risk factors (Al Jarousha et al., 2009; Zarrilli et al., 2012; Maciel et al., 2018; Ulu-Kilic et al., 2018). No large-scale studies were identified that define a clinical phenotype for neonatal Acinetobacter spp. infections.
There are a number of reports suggesting that Acinetobacter spp. is overrepresented in cases of neonatal meningitis, which is associated with high mortality and adverse neurodevelopmental outcomes. Interestingly, Okomo et al., who analysed a total of 7856 positive cultures from Sub-Saharan Africa, found that Acinetobacter spp. made up 5% of isolates from blood cultures and 10% from CSF cultures. A similar proportion is reported in a multisite South African study where A. baumanii was isolated in 13% and 23% of positive blood and CSF cultures, respectively (Mashau et al., 2022). It remains to be determined whether this reflects a preferential tropism for CSF with Acinetobacter spp., or disease progression secondary to antimicrobial resistance and first-line treatment failure.
Mortality of neonatal sepsis secondary to Acinetobacter spp. infection is very high. NeoObs, a global observational trials of neonatal sepsis reported a mortality of approximately 30% in neonates with culture-positive sepsis secondary to Acinetobacter spp. (Russell et al., 2023). For comparison, mortality secondary to any Gram-negative isolate was reported as 21% in NeoObs. These high estimates are reflected in outbreak mortality, with the case fatality rate in NICU outbreaks caused by A. baumanii estimated to be 34% (Birt et al., 2016). Possible reasons for high Acinetobacter spp. mortality includes higher intrinsic disease severity and AMR-related treatment failure. Individual regions report even higher mortality, for example a multi-site study in India found a 59% mortality from Acinetobacter spp. culture-positive sepsis, compared to mortality of 48% for all other isolates (DENIS, 2016). Regional differences in mortality may be explained by quality of supportive care or virulence factors in local Acinetobacter spp. strains.
Molecular characterisation of invasive neonatal Acinetobacter spp. may bring clues to the host-pathogen dynamics in this vulnerable population. However, there is currently a lack of published data comparing virulence genes in invasive neonatal isolates when compared with environmental and/or invasive isolates in other populations. A number of virulence factors have been reported from neonatal isolates, though no meta-analysis was found for these, with most focusing on antibiotic resistance genes rather than virulence.
Wen et al. reported 70%, 84% and 42% resistance of Acinetobacter spp. isolates to gentamicin, third generation cephalosporin and carbapenems, respectively. These numbers are of clinical relevance in light of the WHO recommendations to use ampicillin and gentamicin first-line and ceftriaxone second line in the empirical treatment of neonatal sepsis worldwide. These high resistance rates are also reflected in recent cohort studies (see Table 2), and demonstrate the growing burden of CRAB worldwide. The most commonly mentioned ARGs are in the OXA group, particularly OXA23 which confers carbapenem resistance.
Table 2. Global cohort studies recruiting neonates with sepsis reporting Acinetobacter spp. proportions.
CRAB now dominates the global burden of Acinetobacter spp. infections, leaving few available treatment options (Osei Sekyere et al., 2021). Though pre-existing and novel antimicrobial options are being explored, resistance mechanisms to these agents often develop quickly. Polymyxin antibiotics, such as polymyxin B and colistin, seem among the most active agents against Acinetobacter infections at present (Kassamali et al., 2015). While these agents are used in some NICUs, their clinical use is hampered by delivery issues, concerns about toxicity and rapidly developing resistance (Cai et al., 2012; Bostanghadiri et al., 2024). Novel tetracyclines, such as tigecycline, have also shown some promise in the treatment of paediatric infections (Sharland et al., 2019). However, these medications have a high toxicity profile, limited clinical data and lack evidence to support a neonatal dose (Falagas et al., 2015). Cefiderocol, a novel cephalosporin antibiotic, has potent in vitro activity against a wide range of Gram-negative pathogens, with some evidence to suggest that this includes Acinetobacter spp. Surveillance data suggest that most clinical isolates are sensitive to cefiderocol, though the mechanisms of resistance in the remainder are not fully understood and clinical efficacy data is limited (Kollef et al., 2023). There is a lack of data to support a neonatal dose, though a pharmacokinetic study is currently ongoing for infants under 3 months of age (NCT06086626). Durlobactam, a novel β-lactamase inhibitor, combined with sulbactam, which has intrinsic activity against Acinetobacter spp., is another possible therapeutic option. A recent Phase 3 trial found this combination non-inferior to colistin in adults, with further trials underway (Kaye et al., 2023). Several landmark clinical trials have compared combination therapies specifically in adults with A. baumanii infection, however there are few paediatric equivalents. Drug development and assessment focused on neonates is urgently required to determine an optimal dose, efficacy and safety of novel regimens in this population, particularly given their unique pharmacokinetic profile which rapidly changes with postnatal age (Williams et al., 2022; Poggi and Dani, 2023; Bamford et al., 2024).
An obvious strategy to reduce Acinetobacter spp. infections in neonates is to target NICU colonisation and transmission. There remains inadequate evidence for the majority of infection prevention and control interventions in this setting. One intervention known to reduce infant colonisation with pathogenic Gram-negative pathogens is intermediate Kangaroo Mother Care (iKMC), which promotes colonisation with non-pathogenic microbiota organisms and reduces neonatal sepsis-related mortality (Arya et al., 2023). A recent Cochrane review assessing the effects of infant isolation or cohorting concluded there was insufficient evidence for these interventions, and that more high quality trials were needed (Hanna et al., 2023). Another meta-analysis concluded that there was insufficient evidence that implementation of surveillance methods reduced NICU-associated infections (Birt et al., 2016). Larger scale studies specifically designed to evaluate infection control interventions including behavioural analysis are required in order to determine optimal Acinetobacter spp. control strategies in this setting, particularly given its lack of clear primary environmental niche.
As conventional antibiotic options dwindle, there is an increasing demand for alternative strategies in the global fight against A. baumanii infections, with recent research focusing on vaccine development. The use of reverse vaccinology has identified numerous targets including outer membrane vesicles, outer membrane protein A, auto-transporter, biofilm-associated protein, K1 capsular polysaccharide, and poly-(beta-1,6)-N-acetyl glucosamide. Active vaccinations have been demonstrated to confer protection in animal models, while a monoclonal antibody targeting K1 capsular polysaccharide has also shown protective effects in vivo (Russo et al., 2013; Chen, 2015). Both active and passive vaccinations could be helpful for neonates, for example via maternal vaccination or targeting high risk populations, though these may be challenging to identify. While these efforts are promising, at present no A. baumannii vaccine candidates have progressed beyond Phase I trials, in part because of the pathogen’s wide antigenic variability (Singh et al., 2022).
Given the burgeoning harm caused by Acinetobacter spp. in neonates, a renewed interest is required and research priorities need to be established. First, further molecular genotyping is needed to compare neonatal strains of Acinetobacter spp. to strains derived from different populations. The use of molecular techniques on invasive isolates (as compared with colonising isolates) could illuminate the pathogen-specific factors that enable neonatal infection. Should an apparent propensity to cause meningitis be consistently shown, a comparison of CSF and blood isolates could help to understand the mechanisms for this. Sample collection would be best performed as a subproject of existing initiatives, such as the African severe neonatal infection platform (SNIP-AFRICA, 2023). As part of this, clinical data could be used to ascertain whether a clinical phenotype for Acinetobacter infection exists in neonates with sepsis that differs from sepsis caused by other pathogens. Pre-existing trials, for example the NeoSep1 study (ISRCTN48721236), could be used as a platform for this data collection.
Second, transmission dynamics using environmental sampling and colonisation data must be used to better characterise the most common ecological niches in Acinetobacter spp. spread in a NICU setting. Acinetobacter spp.-specific infection control interventions should be created and evaluated with robust study designs. Research must prioritise effective and cheap interventions that combat the most frequent ecological niches identified, combined with behavioural change analysis to ensure that these interventions result in sustained results. Finally, there is a need to prioritise development of novel treatments for Acinetobacter spp. infections in the neonatal population, which has been underserved in existing antimicrobial development.
Acinetobacter spp. play an ever growing role in neonatal sepsis and meningitis worldwide, with the burden of disease resting in low income settings. Host, pathogen and environmental factors result in increasing numbers of infections with high mortality. Here, we suggest a number of research priorities going forward; microbiological and clinical characterisation of neonatal sepsis secondary to Acinetobacter spp. as well as evaluations of infection control interventions and prioritisation of neonatal drug development.
KP: Writing – original draft, Writing – review & editing. AR-C: Writing – original draft, Writing – review & editing. SO’B: Writing – review & editing. PH: Writing – review & editing. MS: Conceptualization, Writing – review & editing.
The author(s) declare that no financial support was received for the research, authorship, and/or publication of this article.
The authors declare that the research was conducted in the absence of any commercial or financial relationships that could be construed as a potential conflict of interest.
All claims expressed in this article are solely those of the authors and do not necessarily represent those of their affiliated organizations, or those of the publisher, the editors and the reviewers. Any product that may be evaluated in this article, or claim that may be made by its manufacturer, is not guaranteed or endorsed by the publisher.
Akbarian-Rad Z., Riahi S. M., Abdollahi A., Sabbagh P., Ebrahimpour S., Javanian M., et al. (2020). Neonatal sepsis in Iran: A systematic review and meta-analysis on national prevalence and causative pathogens. PloS One 15, e0227570. doi: 10.1371/journal.pone.0227570
Al Jarousha A. M., El Jadba A. H., Al Afifi A. S., El Qouqa I. A. (2009). Nosocomial multidrug-resistant Acinetobacter baumannii in the neonatal intensive care unit in Gaza City, Palestine. Int. J. Infect. diseases : IJID : Off. Publ. Int. Soc. Infect. Dis. 13, 623–628. doi: 10.1016/j.ijid.2008.08.029
Arya S., et al. (2023). Effect on neonatal sepsis following immediate kangaroo mother care in a newborn intensive care unit: a post-hoc analysis of a multicentre, open-label, randomised controlled trial. EClinicalMedicine 60, 102006. doi: 10.1016/j.eclinm.2023.102006
Bamford A., Masini T., Williams P., Sharland M., Gigante V., Dixit D., et al. (2024). ‘Tackling the threat of antimicrobial resistance in neonates and children: outcomes from the first WHO-convened Paediatric Drug Optimisation exercise for antibiotics. Lancet Child Adolesc. Health 8, 456–466. doi: 10.1016/S2352-4642(24)00048-8
Basu S. (2015). Neonatal sepsis: the gut connection. Eur. J. Clin. Microbiol. Infect. diseases : Off. Publ. Eur. Soc. Clin. Microbiol. 34, 215–222. doi: 10.1007/s10096-014-2232-6
Birt J., Le Doare K., Kortsalioudaki C., Lawn J., Heath P. T., Sharland M. (2016). Lack of evidence for the efficacy of enhanced surveillance compared to other specific interventions to control neonatal healthcare-associated infection outbreaks. Trans. R. Soc. Trop. Med. Hygiene 110, 98–106. doi: 10.1093/trstmh/trv116
Bonomo R. A., Szabo D. (2006). Mechanisms of multidrug resistance in Acinetobacter species and Pseudomonas aeruginosa. Clin. Infect. diseases : an Off. Publ. Infect. Dis. Soc. America 43 Suppl 2, S49–S56. doi: 10.1086/504477
Bostanghadiri N., Narimisa N., Mirshekar M., Dadgar-Zankbar L., Taki E., Navidifar T., et al. (2024). Prevalence of colistin resistance in clinical isolates of Acinetobacter baumannii: a systematic review and meta-analysis. Antimicrobial resistance infection control 13, 24. doi: 10.1186/s13756-024-01376-7
Cai Y., Chai D., Wang R., Liang B., Bai N. (2012). Colistin resistance of Acinetobacter baumannii: clinical reports, mechanisms and antimicrobial strategies. J. antimicrobial chemotherapy 67, 1607–1615. doi: 10.1093/jac/dks084
Camacho-Gonzalez A., Spearman P. W., Stoll B. J. (2013). Neonatal infectious diseases: evaluation of neonatal sepsis. Pediatr. Clinics North America 60, 367–389. doi: 10.1016/j.pcl.2012.12.003
Chen W. (2015). Current advances and challenges in the development of Acinetobacter vaccines. Hum. Vaccines Immunother. 11(10), 2495–2500. doi: 10.1080/21645515.2015.1052354
Chen W. (2020). Host innate immune responses to acinetobacter baumannii infection. Front. Cell. infection Microbiol. 10. doi: 10.3389/fcimb.2020.00486
Da Silva G. J., Domingues S. (2016). Insights on the horizontal gene transfer of carbapenemase determinants in the opportunistic pathogen acinetobacter baumannii. Microorganisms 4, 29. doi: 10.3390/microorganisms4030029
Das P., Singh A. K., Pal T., Dasgupta S., Ramamurthy T., Basu S. (2011). Colonization of the gut with Gram-negative bacilli, its association with neonatal sepsis and its clinical relevance in a developing country. J. Med. Microbiol. 60, 1651–1660. doi: 10.1099/jmm.0.033803-0
DENIS (2016). Characterisation and antimicrobial resistance of sepsis pathogens in neonates born in tertiary care centres in Delhi, India: a cohort study. Lancet Global Health 4, e752–e760. doi: 10.1016/S2214-109X(16)30148-6
Espinal P., Martí S., Vila J. (2012). Effect of biofilm formation on the survival of Acinetobacter baumannii on dry surfaces. J. Hosp. infection 80, 56–60. doi: 10.1016/j.jhin.2011.08.013
Falagas M. E., Vardakas K. Z., Kapaskelis A., Triarides N. A., Roussos N. S. (2015). Tetracyclines for multidrug-resistant Acinetobacter baumannii infections. Int. J. antimicrobial Agents 45, 455–460. doi: 10.1016/j.ijantimicag.2014.12.031
Fleischmann C., Reichert F., Cassini A., Horner R., Harder T., Markwart R., et al. (2021). Global incidence and mortality of neonatal sepsis: a systematic review and meta-analysis. Arch. Dis. childhood 106, 745–752. doi: 10.1136/archdischild-2020-320217
Folgori L., Tersigni C., Hsia Y., Kortsalioudaki C., Heath P., Sharland M., et al. (2018). The relationship between Gram-negative colonization and bloodstream infections in neonates: a systematic review and meta-analysis. Clin. Microbiol. infection : Off. Publ. Eur. Soc. Clin. Microbiol. Infect. Dis. 24, 251–257. doi: 10.1016/j.cmi.2017.08.008
Hanna M., Shah R., Marquez L., Barzegar R., Gordon A., Pammi M. (2023). Infant isolation and cohorting for preventing or reducing transmission of healthcare-associated infections in neonatal units. Cochrane Database systematic Rev. 6, CD012458. doi: 10.1002/14651858.CD012458.pub2
Hartz L. E., Bradshaw W., Brandon D. H. (2015). Potential NICU environmental influences on the neonate’s microbiome: A systematic review. Adv. neonatal care : Off. J. Natl. Assoc. Neonatal Nurses 15, 324–335. doi: 10.1097/ANC.0000000000000220
Havenga B., Reyneke B., Ndlovu T., Khan W. (2022). Genotypic and phenotypic comparison of clinical and environmental Acinetobacter baumannii strains. Microbial pathogenesis 172, 105749. doi: 10.1016/j.micpath.2022.105749
Hensler E., Petros H., Gray C. C., Chung C. S., Ayala A., Fallon E. A. (2022). The neonatal innate immune response to sepsis: checkpoint proteins as novel mediators of this response and as possible therapeutic/diagnostic levers. Front. Immunol. 13. doi: 10.3389/fimmu.2022.940930
Jawad A., Seifert H., Snelling A. M., Heritage J., Hawkey P. M. (1998). Survival of Acinetobacter baumannii on dry surfaces: comparison of outbreak and sporadic isolates. J. Clin. Microbiol. 36, 1938–1941. doi: 10.1128/JCM.36.7.1938-1941.1998
Jefferies J. M. C., Cooper T., Yam T., Clarke S. C. (2012). Pseudomonas aeruginosa outbreaks in the neonatal intensive care unit–a systematic review of risk factors and environmental sources. J. Med. Microbiol. 61, 1052–1061. doi: 10.1099/jmm.0.044818-0
Karim M., Khan W., Farooqi B., Malik I. (1991). ‘Bacterial isolates in neutropenic febrile patients.’, JPMA. J. Pakistan Med. Assoc. 41, 35–37.
Kassamali Z., Jain R., Danziger L. H. (2015). An update on the arsenal for multidrug-resistant Acinetobacter infections: polymyxin antibiotics. Int. J. Infect. diseases : IJID : Off. Publ. Int. Soc. Infect. Dis. 30, 125–132. doi: 10.1016/j.ijid.2014.10.014
Kaye K. S., Shorr A. F., Wunderink R. G., Du B., Poirier G. E., Rana K., et al. (2023). Efficacy and safety of sulbactam-durlobactam versus colistin for the treatment of patients with serious infections caused by Acinetobacter baumannii-calcoaceticus complex: a multicentre, randomised, active-controlled, phase 3, non-inferiority clinical trial (ATTACK). Lancet Infect. Dis. 23, 1072–1084. doi: 10.1016/S1473-3099(23)00184-6
Khalil N., Blunt H. B., Li Z., Hartman T. (2020). Neonatal early onset sepsis in Middle Eastern countries: a systematic review. Arch. Dis. childhood 105, 639–647. doi: 10.1136/archdischild-2019-317110
Klingenberg C., Kornelisse R. F., Buonocore G., Maier R. F., Stocker M. (2018). Culture-negative early-onset neonatal sepsis - at the crossroad between efficient sepsis care and antimicrobial stewardship. Front. Pediatr. 6. doi: 10.3389/fped.2018.00285
Kollef M., Dupont H., Greenberg D. E., Viale P., Echols R., Yamano Y., et al. (2023). Prospective role of cefiderocol in the management of carbapenem-resistant Acinetobacter baumannii infections: Review of the evidence. Int. J. antimicrobial Agents 62, 106882. doi: 10.1016/j.ijantimicag.2023.106882
Lanjri S., Uwingabiye J., Frikh M., Abdellatifi L., Kasouati J., Maleb A., et al. (2017). In vitro evaluation of the susceptibility of Acinetobacter baumannii isolates to antiseptics and disinfectants: comparison between clinical and environmental isolates. Antimicrob. Resist. Infect. Control 6, 36. doi: 10.1186/s13756-017-0195-y
Li J.-Y., Chen S. Q., Yan Y. Y., Hu Y. Y., Wei J., Wu Q. P., et al. (2018). Identification and antimicrobial resistance of pathogens in neonatal septicemia in China-A meta-analysis. Int. J. Infect. diseases : IJID : Off. Publ. Int. Soc. Infect. Dis. 71, 89–93. doi: 10.1016/j.ijid.2018.04.794
Li G., et al. (2020). Towards understanding global patterns of antimicrobial use and resistance in neonatal sepsis: insights from the NeoAMR network. Arch. Dis. childhood 105, 26–31. doi: 10.1136/archdischild-2019-316816
Maciel W. G., da Silva K. E., Croda J., Cayô R., Ramos A. C., de Sales R. O., et al. (2018). Clonal spread of carbapenem-resistant Acinetobacter baumannii in a neonatal intensive care unit. J. Hosp. infection 98, 300–304. doi: 10.1016/j.jhin.2017.10.015
Makoni M., Eckert J., Anne Pereira H., Nizet V., Lawrence S. M. (2016). Alterations in neonatal neutrophil function attributable to increased immature forms. Early Hum. Dev. 103, 1–7. doi: 10.1016/j.earlhumdev.2016.05.016
Mashau R. C., Meiring S. T., Dramowski A., Magobo R. E., Quan V. C., Perovic O., et al. (2022). ‘Culture-confirmed neonatal bloodstream infections and meningitis in South Africa 2014-19: a cross-sectional study. Lancet Global Health 10, e1170–e1178. doi: 10.1016/S2214-109X(22)00246-7
McGreal E. P., Hearne K., Spiller O. B. (2012). Off to a slow start: under-development of the complement system in term newborns is more substantial following premature birth. Immunobiology 217, 176–186. doi: 10.1016/j.imbio.2011.07.027
Mea H. J., Yong P. V. C., Wong E. H. (2021). An overview of Acinetobacter baumannii pathogenesis: Motility, adherence and biofilm formation. Microbiological Res. 247, 126722. doi: 10.1016/j.micres.2021.126722
Medugu N., Iregbu K., Iroh Tam P. Y., Obaro S. (2018). Aetiology of neonatal sepsis in Nigeria, and relevance of Group b streptococcus: A systematic review. PloS One 13. doi: 10.1371/journal.pone.0200350
Okomo U., Akpalu E. N. K., Le Doare K., Roca A., Cousens S., Jarde A., et al. (2019). Aetiology of invasive bacterial infection and antimicrobial resistance in neonates in sub-Saharan Africa: a systematic review and meta-analysis in line with the STROBE-NI reporting guidelines. Lancet Infect. Dis. 19, 1219–1234. doi: 10.1016/S1473-3099(19)30414-1
Oliva A., Carmona Y., de La López C E., Álvarez R., Aung M. S., Kobayashi N., et al. (2021). Characterization of neonatal infections by gram-negative bacilli and associated risk factors, havana, Cuba. Infect. Dis. Rep. 13, 219–229. doi: 10.3390/idr13010025
Osei Sekyere J., Reta M. A., Bernard Fourie P. (2021). Risk factors for, and molecular epidemiology and clinical outcomes of, carbapenem- and polymyxin-resistant Gram-negative bacterial infections in pregnant women, infants, and toddlers: a systematic review and meta-analyses. Ann. New York Acad. Sci. 1502, 54–71. doi: 10.1111/nyas.14650
Poggi C., Dani C. (2023). New antimicrobials for the treatment of neonatal sepsis caused by multi-drug-resistant bacteria: A systematic review. Antibiotics (Basel Switzerland) 12, 956. doi: 10.3390/antibiotics12060956
Russell N. J., Stöhr W., Plakkal N., Cook A., Berkley J. A., Adhisivam B., et al. (2023). Patterns of antibiotic use, pathogens, and prediction of mortality in hospitalized neonates and young infants with sepsis: A global neonatal sepsis observational cohort study (NeoOBS). PloS Med. 20, e1004179. doi: 10.1371/journal.pmed.1004179
Russo T. A., Beanan J. M., Olson R., MacDonald U., Cox A. D., St Michael F., et al. (2013). The K1 capsular polysaccharide from Acinetobacter baumannii is a potential therapeutic target via passive immunization. Infect. Immun. 81(3), 915–922. doi: 10.1128/IAI.01184-12
Sands K., Carvalho M. J., Portal E., Thomson K., Dyer C., Akpulu C., et al. (2021). Characterization of antimicrobial-resistant Gram-negative bacteria that cause neonatal sepsis in seven low- and middle-income countries. Nat. Microbiol. 6, 512–523. doi: 10.1038/s41564-021-00870-7
Sanidad K. Z., Zeng M. Y. (2020). Neonatal gut microbiome and immunity. Curr. Opin. Microbiol. 56, 30–37. doi: 10.1016/j.mib.2020.05.011
Shadan A., Pathak A., Ma Y., Pathania R., Singh R. P. (2023). Deciphering the virulence factors, regulation, and immune response to Acinetobacter baumannii infection. Front. Cell. infection Microbiol. 13. doi: 10.3389/fcimb.2023.1053968
Shane A. L., Sánchez P. J., Stoll B. J. (2017). Neonatal sepsis. Lancet (London England) 390, 1770–1780. doi: 10.1016/S0140-6736(17)31002-4
Sharland M., Rodvold K. A., Tucker H. R., Baillon-Plot N., Tawadrous M., Hickman M. A., et al. (2019). Safety and efficacy of tigecycline to treat multidrug-resistant infections in pediatrics: an evidence synthesis. Pediatr. Infect. Dis. J. 38, 710–715. doi: 10.1097/INF.0000000000002339
Singh R., Capalash N., Sharma P. (2022). Vaccine development to control the rising scourge of antibiotic-resistant Acinetobacter baumannii: a systematic review. 3 Biotech 12(3), 85. doi: 10.1007/s13205-022-03148-9
SNIP-AFRICA (2023). SNIP-AFRICA, SNIP-AFRICA. Available online at: https://www.snip-africa.org/ (Accessed March 03, 2024).
Thomas R., Ondongo-Ezhet C., Motsoaledi N., Sharland M., Clements M., Velaphi S. (2024). Incidence, pathogens and antimicrobial resistance of blood and cerebrospinal fluid isolates from a tertiary neonatal unit in South Africa: A 10 year retrospective review. PloS One 19, e0297371. doi: 10.1371/journal.pone.0297371
Tsiatsiou O., Iosifidis Ε., Katragkou A., Dimou V., Sarafidis K., Karampatakis T., et al. (2015). Successful management of an outbreak due to carbapenem-resistant Acinetobacter baumannii in a neonatal intensive care unit. Eur. J. Pediatr. 174, 65–74. doi: 10.1007/s00431-014-2365-8
Ulu-Kilic A., Gundogdu A., Cevahir F., Kilic H., Gunes T., Alp E. (2018). An outbreak of bloodstream infection due to extensively resistant Acinetobacter baumannii among neonates. Am. J. infection control 46, 154–158. doi: 10.1016/j.ajic.2017.08.007
Vázquez-López R., Solano-Gálvez S. G., Juárez Vignon-Whaley J. J., Abello Vaamonde J. A., Padró Alonzo L. A., Rivera Reséndiz A., et al. (2020). Acinetobacter baumannii resistance: A real challenge for clinicians. Antibiotics (Basel Switzerland) 9, 205. doi: 10.3390/antibiotics9040205
Wen S. C. H., Ezure Y., Rolley L., Spurling G., Lau C. L., Riaz S., et al. (2021). Gram-negative neonatal sepsis in low- and lower-middle-income countries and WHO empirical antibiotic recommendations: A systematic review and meta-analysis. PloS Med. 18, e1003787. doi: 10.1371/journal.pmed.1003787
Wendt C., Dietze B., Dietz E., Rüden H. (1997). Survival of Acinetobacter baumannii on dry surfaces. J. Clin. Microbiol. 35, 1394–1397. doi: 10.1128/jcm.35.6.1394-1397.1997
WHO (2017). Global priority list of antibiotic-resistant bacteria to guide reseatch, discovery and development of new antibiotics (Geneva, Switzerland: WHO).
Williams P. C., Qazi S. A., Agarwal R., Velaphi S., Bielicki J. A., Nambiar S., et al. (2022). Antibiotics needed to treat multidrug-resistant infections in neonates. Bull. World Health Organ. 100, 797–807. doi: 10.2471/BLT.22.288623
Woon J. J., Ahmad Kamar A., Teh C. S. J., Idris N., Zhazali R., Saaibon S., et al. (2023). Molecular epidemiological investigation and management of outbreak caused by carbapenem-resistant acinetobacter baumannii in a neonatal intensive care unit. Microorganisms 11, 1073. doi: 10.3390/microorganisms11041073
Zarrilli R., Di Popolo A., Bagattini M., Giannouli M., Martino D., Barchitta M., et al. (2012). Clonal spread and patient risk factors for acquisition of extensively drug-resistant Acinetobacter baumannii in a neonatal intensive care unit in Italy. J. Hosp. infection 82, 260–265. doi: 10.1016/j.jhin.2012.08.018
Keywords: Acinetobacter spp., Acinetobacter baumanii, neonatal sepsis, biofilm, antibiotic resistance, virulence traits, natural transformation
Citation: Pillay K, Ray-Chaudhuri A, O’Brien S, Heath P and Sharland M (2024) Acinetobacter spp. in neonatal sepsis: an urgent global threat. Front. Antibiot. 3:1448071. doi: 10.3389/frabi.2024.1448071
Received: 12 June 2024; Accepted: 08 August 2024;
Published: 03 September 2024.
Edited by:
Tanusha Ramdin, Department of Health, Republic of South Africa, South AfricaReviewed by:
Rajlakshmi Viswanathan, National Institute of Virology (ICMR), IndiaCopyright © 2024 Pillay, Ray-Chaudhuri, O’Brien, Heath and Sharland. This is an open-access article distributed under the terms of the Creative Commons Attribution License (CC BY). The use, distribution or reproduction in other forums is permitted, provided the original author(s) and the copyright owner(s) are credited and that the original publication in this journal is cited, in accordance with accepted academic practice. No use, distribution or reproduction is permitted which does not comply with these terms.
*Correspondence: Kamla Pillay, a3BpbGxheUBzZ3VsLmFjLnVr
Disclaimer: All claims expressed in this article are solely those of the authors and do not necessarily represent those of their affiliated organizations, or those of the publisher, the editors and the reviewers. Any product that may be evaluated in this article or claim that may be made by its manufacturer is not guaranteed or endorsed by the publisher.
Research integrity at Frontiers
Learn more about the work of our research integrity team to safeguard the quality of each article we publish.