- 1The Medical School, University of Jordan, Amman, Jordan
- 2Pfizer Inc., Medical Affairs, Beirut, Lebanon
Objectives: To evaluate the in vitro antimicrobial susceptibilities of Gram-positive and Gram-negative isolates from patients in Jordan between 2010 and 2021, through the Antimicrobial Testing Leadership and Surveillance (ATLAS) programme.
Methods: Medical centres in Jordan collected bacterial isolates from hospitalised patients with defined infection sources between 2010 and 2021 (no isolates collected in 2014). Antimicrobial susceptibility was interpreted using CLSI standards. FDA-approved breakpoints were applied for tigecycline. The identification of β-lactamase genes was performed for a proportion of isolates using multiplex PCR assays.
Results: More than 92% of Acinetobacter baumannii collected were multidrug-resistant (MDR) and/or carbapenem-resistant (CR), and > 50% susceptibility was reported only to minocycline (62.2% among both MDR and CR isolates). Rates of MDR and CR Pseudomonas aeruginosa were 14.3% and 20.5%, respectively, and among all P. aeruginosa collected from adults, susceptibility to ceftazidime/avibactam was 95.3% and to ceftolozane/tazobactam was 88.4%. For Escherichia coli from adults and MDR E. coli, susceptibility to ceftazidime/avibactam, ceftolozane/tazobactam, imipenem, meropenem and meropenem/vaborbactam was 92.1%–98.7%. Susceptibility to tigecycline was > 94% among Klebsiella pneumoniae from adult, paediatric, and ICU patients (all ages). CTX-M-15 was the most frequently identified β-lactamase gene among E. coli and K. pneumoniae. Susceptibility to most antimicrobial agents was < 50% among K. pneumoniae carrying CTX-M-15, CTX-M-9-type, NDM-5, and/or OXA-48 β-lactamase genes. All S. aureus collected were susceptible to teicoplanin, vancomycin, daptomycin, linezolid and tigecycline, with 96.1% of S. aureus from adults were susceptible to ceftaroline. Overall, 58.8% of Staphylococcus aureus were MRSA.
Conclusion: This study provides valuable information regarding antimicrobial susceptibility in Jordan between 2010 and 2021. Continued monitoring of in vitro antimicrobial susceptibility is critical in the fight against antimicrobial resistance.
1 Introduction
The burden of antimicrobial resistance is well-documented, with the World Health Organization naming it among the top 10 global public health threats (WHO, 2021). Antimicrobial resistance was estimated to be associated with nearly 5 million deaths worldwide in 2019, among which Escherichia coli, Staphylococcus aureus, Klebsiella pneumoniae, Streptococcus pneumoniae, Acinetobacter baumannii and Pseudomonas aeruginosa were responsible for 72.1% (Antimicrobial Resistance Collaborators, 2022). Antimicrobial activity can be limited, or even eradicated, by the resistance mechanisms displayed by these clinical pathogens, with carbapenem-resistant (CR) A. baumannii and P. aeruginosa, third-generation cephalosporin-resistant E. coli and methicillin-resistant S. aureus (MRSA), listed as critical or high-priority targets for new antimicrobial agents (Tacconelli et al., 2018).
The surveillance of antimicrobial activity plays a crucial role in efforts to tackle antimicrobial resistance. One such surveillance programme is the Antimicrobial Testing Leadership and Surveillance (ATLAS) database (Antimicrobial Testing Leadership and Surveillance (ATLAS) database, 2023). The ATLAS database was initiated in 2018 and integrates historical data from earlier surveillance programmes, Tigecycline Evaluation and Surveillance Trial (TEST) and International Network for Optimal Resistance Monitoring (INFORM), with regularly updated antimicrobial surveillance data from countries worldwide. The database reports laboratory information collected from clinical Gram-negative and Gram-positive isolates and tracks the antimicrobial activity of a panel of agents, including aztreonam/avibactam, ceftaroline, ceftazidime/avibactam, ceftolozane/tazobactam and meropenem/vaborbactam.
Ceftolozane/tazobactam, ceftazidime/avibactam and meropenem/vaborbactam are all approved for clinical use against antimicrobial-resistant pathogens, and a meta-analysis determined a pooled clinical success rate of 73.3% for all three agents against multidrug-resistant (MDR) Gram-negative organism infections (Wilson et al., 2021). In addition, the combination of aztreonam with avibactam is currently being tested in Phase 3 clinical trials for the treatment of serious bacterial infections caused by Gram-negative pathogens, including MDR metallo-β-lactamase (MBL)-producers (Pfizer Inc, 2023).
Although antimicrobial surveillance data has been frequently published for some geographical regions, there is a paucity of data for others. Therefore, we set out to report on antimicrobial activity and susceptibility among bacterial isolates submitted to ATLAS from centres in Jordan. Previous ATLAS publications have included data from Jordan, presented as part of the Middle East region (Piérard and Stone, 2021; Karlowsky et al., 2021a, 2022; Estabrook et al., 2023; Wise et al., 2023), however, this is the first country-specific ATLAS study on isolates of Gram-negative and Gram-positive pathogens from patients in Jordan. Isolates were collected between 2010 and 2021.
2 Materials and methods
2.1 Participating centres and bacterial isolates
Two medical centres in Jordan collected bacterial isolates from hospitalised patients between 2010 and 2021 (no isolates were collected in 2014). The ATLAS protocol defined the number of bacterial isolates per species that each centre could submit each year, and also defined the acceptable infection sources (intra-abdominal, urinary tract, skin and skin structure, lower respiratory tract or bloodstream infections). Isolates were consecutive, nonduplicate clinical isolates and limited to one per patient per year. Selected demographic data on patient age, sex and ward location, were also collected and are presented in Supplementary Table 1.
2.2 Antimicrobial activity testing
Following identification at the local participating centre, all isolates were shipped to a central reference laboratory (International Health Management Associates, Inc. Schaumburg, IL, USA) for species confirmation using MALDI-TOF (Bruker Biotyper MALDI-TOF, Bruker Daltonics, Billerica, MA, USA).
Antimicrobial MIC values were determined using CLSI broth microdilution methodology (CLSI, 2018). MICs for aztreonam/avibactam and ceftazidime/avibactam were determined at a fixed concentration of 4 mg/L for avibactam (CLSI, 2023). Antimicrobial susceptibility was interpreted according to CLSI guidelines, except for tigecycline, where the FDA-approved breakpoints were applied for S. aureus (including MRSA), S. pneumoniae, E. faecalis (vancomycin-susceptible isolates), and the Enterobacterales (CLSI, 2023; FDA, 2023).
2.3 Antimicrobial resistance phenotypes
The definitions of the resistance phenotypes presented, determined using CLSI breakpoints, are listed in full on the ATLAS website (Antimicrobial Testing Leadership and Surveillance (ATLAS) Definitions, 2023). CR A. baumannii or P. aeruginosa were defined as resistant to meropenem. MDR A. baumannii, P. aeruginosa, E. coli or K. pneumoniae were defined as resistant to ≥ 3 antimicrobial agents from the ATLAS testing panel (excluding agents to which an organism has intrinsic resistance): aminoglycosides (amikacin or gentamicin), carbapenems (doripenem, ertapenem, imipenem or meropenem), cephalosporins (cefepime, ceftriaxone or ceftazidime), quinolones (ciprofloxacin or levofloxacin), penicillin plus β-lactamase inhibitor combinations (ampicillin/sulbactam, amoxicillin/clavulanate or piperacillin/tazobactam), or polymyxins (colistin). Additional agents to be considered for each organism are listed on the ATLAS website (Antimicrobial Testing Leadership and Surveillance (ATLAS) database, 2023).
2.4 Screening for β-lactamase genes
As described previously (Kazmierczak et al., 2018; Piérard and Stone, 2021; Wise et al., 2023), isolates of Enterobacterales with MIC values of ≥ 2 mg/L to meropenem (meropenem-nonsusceptible) (CLSI, 2023), were screened for genes encoding clinically relevant β-lactamases (SHV, TEM, CTX-M, VEB, PER and GES ESBLs; ACC, ACT, CMY, DHA, FOX, MIR and MOX plasmid-mediated AmpC β-lactamases; GES, KPC and OXA-48-like serine carbapenemases; and NDM, IMP, VIM, SPM and GIM MBLs), using published multiplex PCR assays (Lob et al., 2015). Full details of the screening criteria for isolates of Enterobacterales during the study period have been previously published (Kazmierczak et al., 2018; Piérard and Stone, 2021; Wise et al., 2023). From 2020 onwards, not all isolates that qualified for screening were tested for genes encoding relevant β-lactamases. In 2020, 13 of 28 (46.4%) E. coli and K. pneumoniae isolates from Jordan that qualified for screening were tested for β-lactamase genes and in 2021, 29 of 46 (63.0%) qualifying E. coli and K. pneumoniae isolates were tested.
All detected carbapenemase genes were amplified using flanking primers and sequenced, and sequences were compared against publicly available databases maintained by the National Center for Biotechnology Information (www.ncbi.nlm.nih.gov) (Estabrook et al., 2023; FDA, 2023). In the present study, MIC summary and susceptibility data for organisms carrying one of the aforementioned resistance genes are presented where ≥ 20 isolates were reported.
3 Results
3.1 Demographic information
A total of 1,856 isolates (Gram-negative, 67.2%; and Gram-positive, 32.8%) were collected between 2010 and 2021, except for 2014 when no isolates were collected (Supplementary Table 1). Most of the total number of isolates were from adults (age ≥ 18 years, 77.9%) and approximately half were from male patients (51.2%). The most common source of isolates was genitourinary (25.8%), followed by circulatory (19.6%) sources. The percentage distribution of the total number of isolates among each source type was comparable (1.1–1.6-fold difference) between the Gram-negative and Gram-positive species, with the most notable difference seen for respiratory sources (Gram-negative, 19.6% and Gram-positive, 8.2%; 2.4-fold difference). Most isolates were from general medicine wards (24.7%), followed by general surgery wards (18.0%). Susceptibility data are presented for A. baumannii, P. aeruginosa, E. coli, K. pneumoniae, Enterococcus faecalis, S. aureus and S. pneumoniae.
3.2 Antimicrobial susceptibility of non-fermenting Gram-negative organisms
3.2.1 Acinetobacter baumannii (N = 160)
Among A. baumannii isolates from adult patients and ICU patients (all ages), the highest rates of susceptibility were to minocycline (62.6% and 53.3%, respectively) (Table 1). For the paediatric subset there were no data for minocycline and the highest rate of susceptibility was to levofloxacin (28.6%).
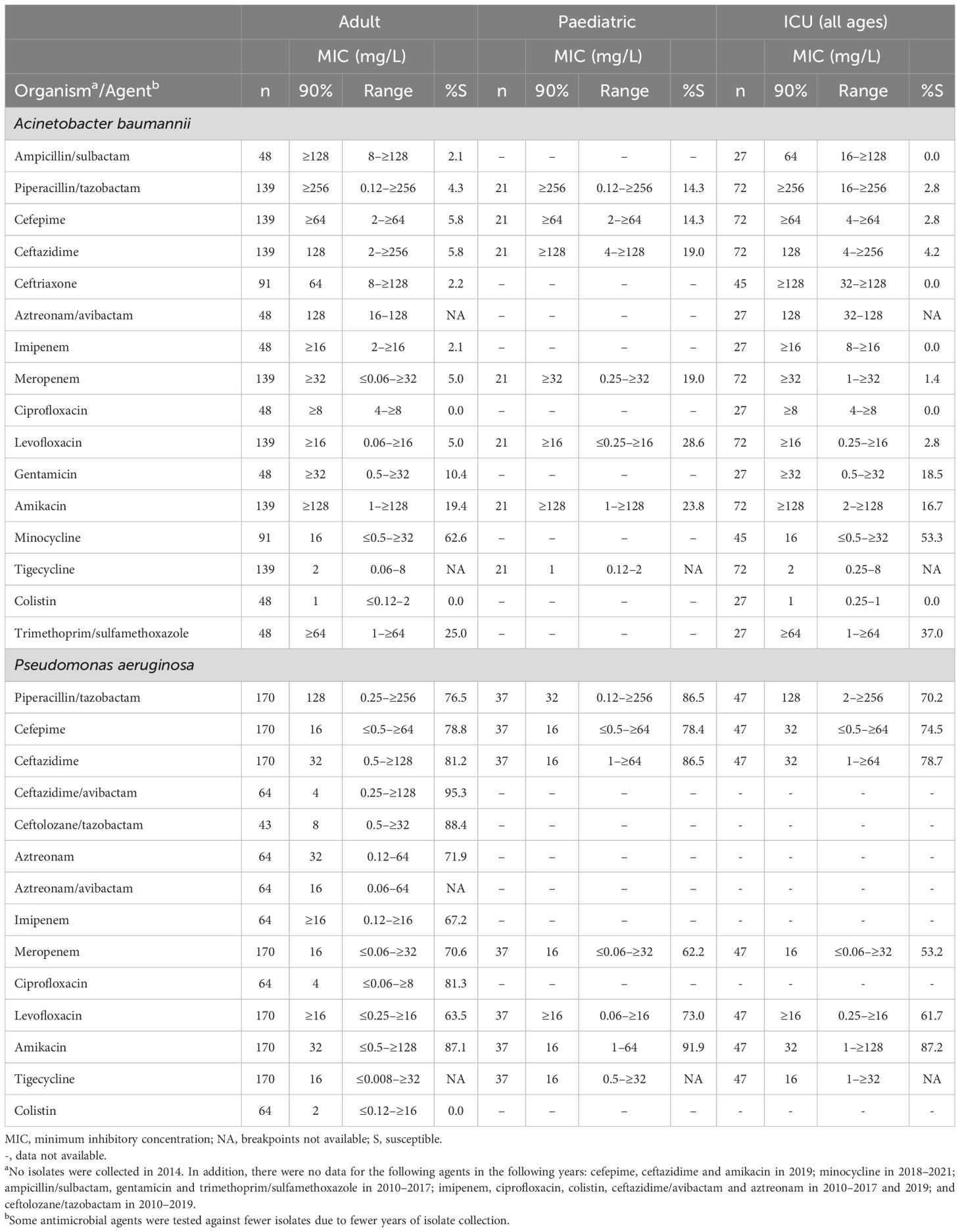
Table 1. In vitro activity and antimicrobial susceptibility of the ATLAS antimicrobial panel against clinical isolates of Acinetobacter baumannii and Pseudomonas aeruginosa collected in Jordan between 2010 and 2021.
A total of 149 (93.1%) A. baumannii isolates were MDR and 148 (92.5%) were CR (Table 2). Susceptibility to minocycline was 62.2% among the MDR and CR subsets, followed by trimethoprim/sulfamethoxazole (29.4% and 30.0%, respectively). The MIC90 of tigecycline was 2 mg/L against the adult, ICU, MDR and CR subsets of A. baumannii (Tables 1, 2), and for isolates of A. baumannii from paediatric patients, the MIC90 for tigecycline was 1 mg/L (Table 1).
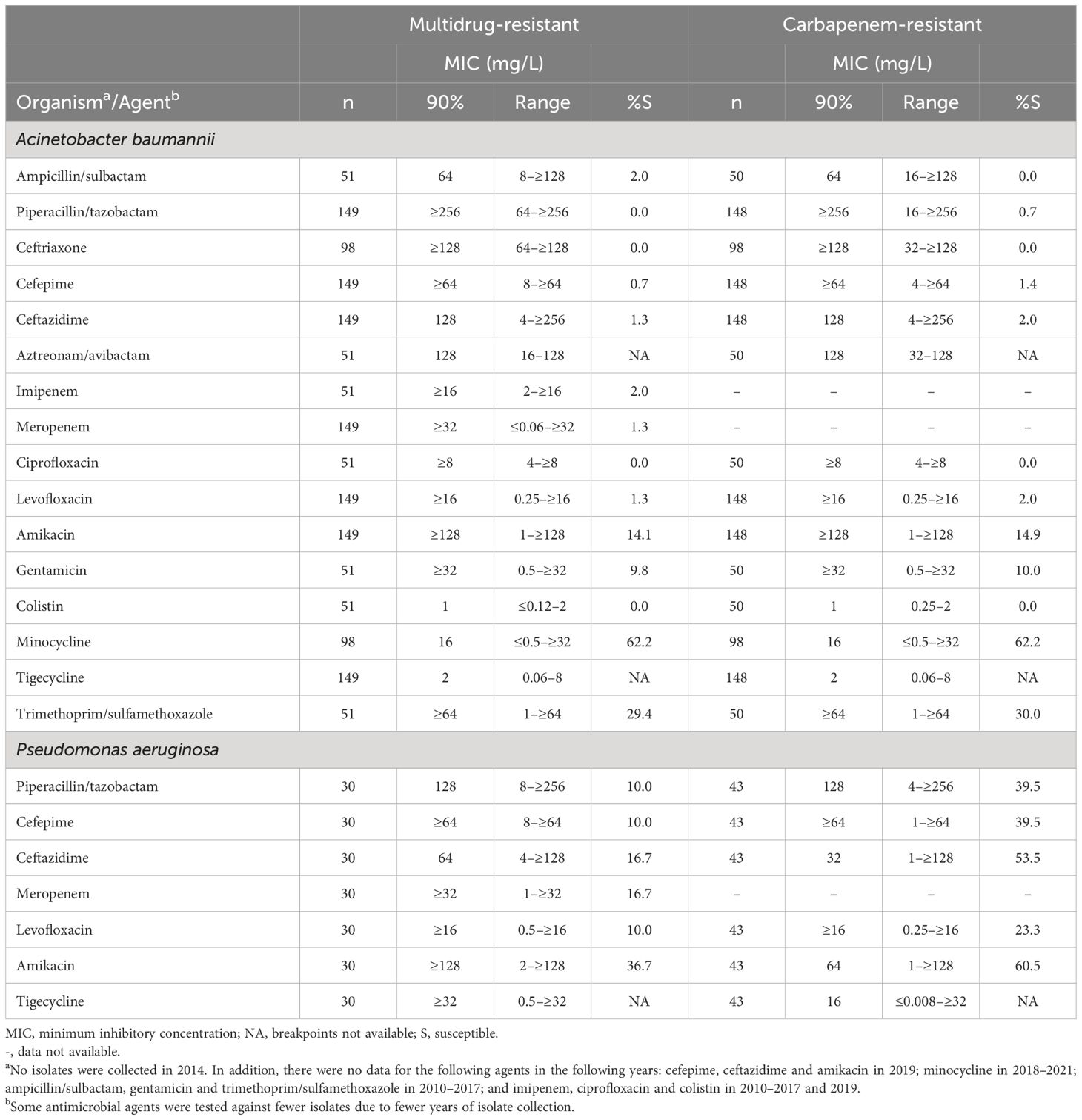
Table 2. In vitro activity and antimicrobial susceptibility of the ATLAS antimicrobial panel against clinical isolates of Acinetobacter baumannii and Pseudomonas aeruginosa with resistance phenotypes collected in Jordan between 2010 and 2021. .
3.2.2 Pseudomonas aeruginosa (N = 210)
For P. aeruginosa isolates from adults, the highest rates of susceptibility were to ceftazidime/avibactam (95.3%), ceftolozane/tazobactam (88.4%) and amikacin (87.1%) (Table 1). Data for ceftazidime/avibactam and ceftolozane/tazobactam were not available for the paediatric or ICU subsets, among which susceptibility was highest to amikacin (91.9% and 87.2%, respectively) (Table 1). Among isolates from paediatric patients, susceptibility to ceftazidime and piperacillin/tazobactam was 86.5% each, compared with 81.2% and 76.5%, respectively, among isolates from adults. Overall, 30 (14.3%) of P. aeruginosa were MDR and 43 (20.5%) were CR. Susceptibility among the MDR isolates was between 10.0% and 36.7% for the available antimicrobials (Table 2). Susceptibility was higher among the CR than MDR isolates, with 60.5% of isolate susceptible to amikacin.
3.3 Antimicrobial susceptibility of Enterobacterales organisms
3.3.1 Escherichia coli (N = 254)
Susceptibility was > 99% to meropenem and tigecycline among the adult and paediatric subsets of E. coli (Table 3). In addition, susceptibility among isolates from adults was high to ceftazidime/avibactam, ceftolozane/tazobactam, imipenem and meropenem/vaborbactam (94.5%–98.7% [not tested against the paediatric subset]). For E. coli isolates from patients in the ICU, all isolates were susceptible to ceftazidime/avibactam, imipenem, meropenem and tigecycline, 85.7% were susceptible to amikacin and 85.0% to gentamicin (Table 3).
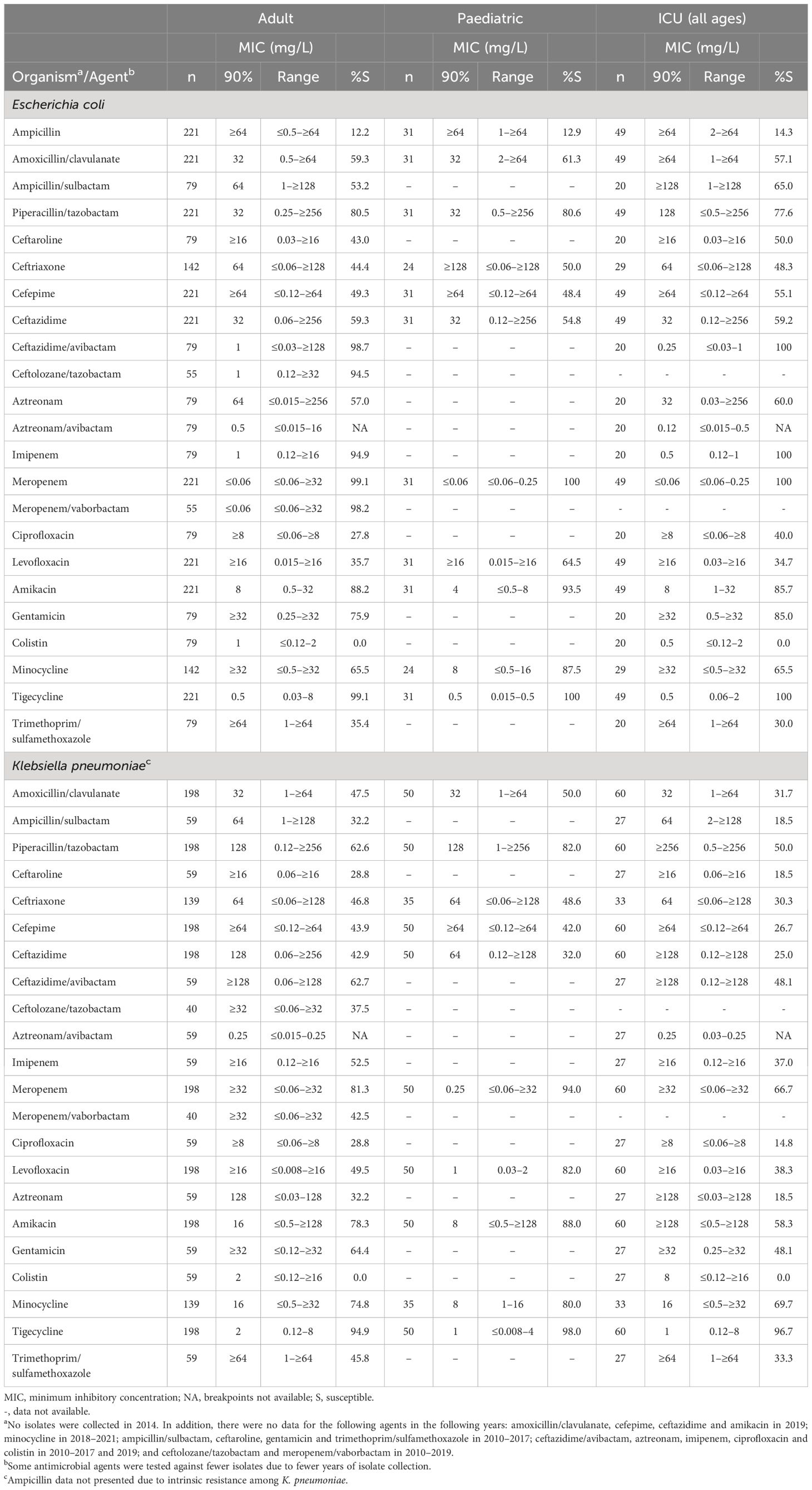
Table 3. In vitro activity and antimicrobial susceptibility of the ATLAS antimicrobial panel against clinical isolates of Escherichia coli and Klebsiella pneumoniae collected in Jordan between 2010 and 2021.
Compared with the adult subset, a higher percentage of E. coli isolates from paediatric patients were susceptible to minocycline (65.5% and 87.5%, respectively) (Table 3). Aztreonam/avibactam was not tested against the paediatric subset but the MIC90 was 0.5 mg/L against isolates from adults.
More than 90% of MDR E. coli were susceptible to ceftazidime/avibactam, ceftolozane/tazobactam, imipenem, meropenem, meropenem/vaborbactam and tigecycline (Table 4). Susceptibility was ≥ 80% to meropenem, amikacin and tigecycline among CTX-M-type-positive E. coli.
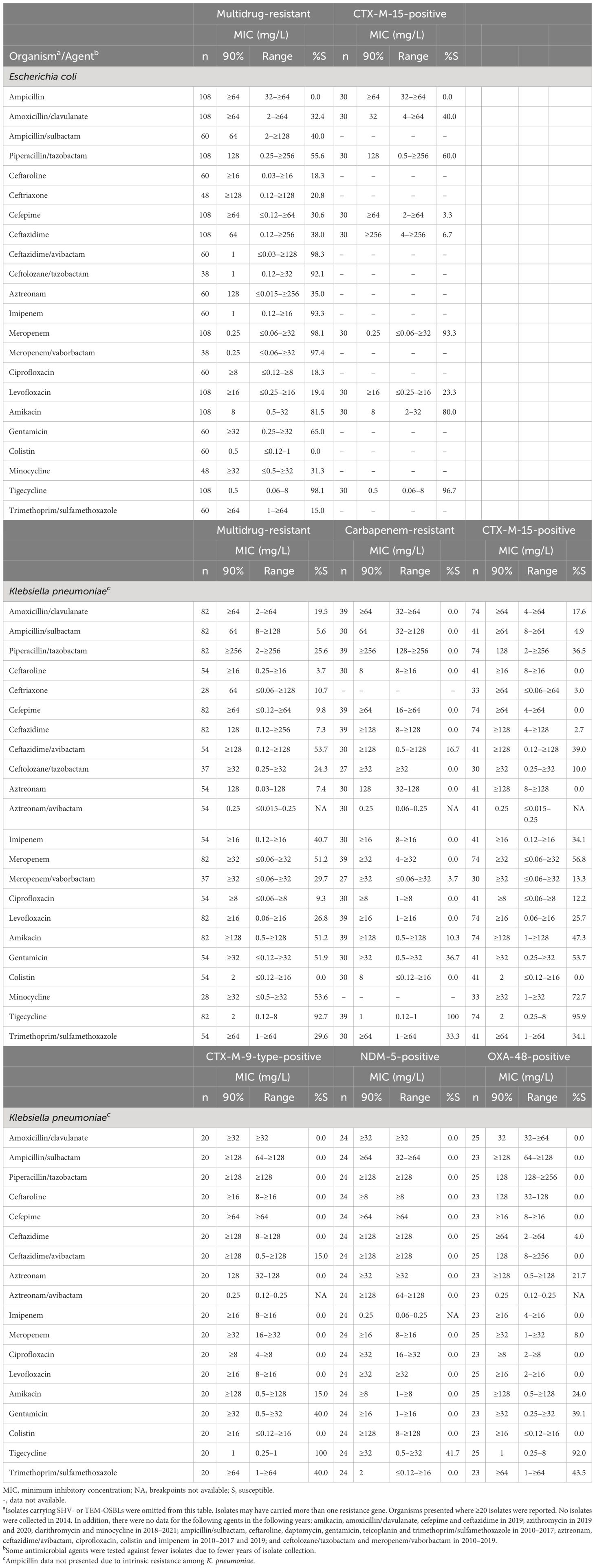
Table 4. In vitro activity and antimicrobial susceptibility of the ATLAS antimicrobial panel against clinical isolates of Escherichia coli and Klebsiella pneumoniae with resistance phenotypes and genotypes collected in Jordan between 2010 and 2021.
Overall, 30 (11.8%) E. coli isolates tested positive for CTX-M-15 enzymes, and all were resistant to ampicillin. There were two CR E. coli isolates: one of the two isolate was positive for an MBL and the other had no resistance genes identified.
3.3.2 Klebsiella pneumoniae (N = 249)
Among isolates from adult and paediatric patients, susceptibility was highest to tigecycline (94.9% and 98.0%, respectively), followed by meropenem (81.3% and 94.0%, respectively) (Table 3). Similarly, among isolates from the ICU (all ages), susceptibility was highest to tigecycline (96.7%).
Comparing rates among isolates from paediatrics and adults showed that susceptibility to piperacillin/tazobactam, meropenem, levofloxacin and amikacin was higher among paediatric isolates than those from adults (Table 3). Among K. pneumoniae from adults, the susceptibility of ceftazidime/avibactam, ceftolozane/tazobactam and meropenem/vaborbactam was 62.7%, 37.5% and 42.5%, respectively, and no isolates were susceptible to colistin.
There were 82 (32.9%) MDR K. pneumoniae isolates and 39 (0.2%) CR K. pneumoniae. Among MDR K. pneumoniae susceptibility was highest to tigecycline (92.7%), followed by ceftazidime/avibactam and minocycline (53.7% and 53.6%, respectively) (Table 4). All 39 CR K. pneumoniae were susceptible to tigecycline; however, susceptibility was low to the majority of other agents with only 16.7% susceptible to ceftazidime-avibactam, 3.7% to meropenem/vaborbactam, and no isolates susceptible to ceftolozane/tazobactam (Table 4). The MIC90 for aztreonam/avibactam was 0.25 mg/L. Antimicrobial activity data for K. pneumoniae where ≥ 20 isolates had a reported β-lactamase resistance gene are presented in Table 4.
Overall, 74 (29.7%) K. pneumoniae isolates were positive for CTX-M-15, 20 (8.0%) for CTX-M-9-type, 24 (9.6%) for NDM-5 and 25 (10.0%) for OXA-48-type enzymes. All K. pneumoniae with NDM-5 genes were susceptible to tigecycline, as were 92.0% of K. pneumoniae carrying OXA-48 genes and ≥ 95.9% carrying CTX-M-type genes. Minocycline susceptibility was 72.7% among K. pneumoniae with CTX-M-15 genes (Table 4). Among the newer combination agents tested, the MIC90 of aztreonam/avibactam was 0.25 mg/L against K. pneumoniae with CTX-M-type, NDM-5 and OXA-48 genes. Among the 39 CR K. pneumoniae 34 (87.2%) tested positive for a carbapenemase.
3.4 Antimicrobial susceptibility of Gram-positive organisms
3.4.1 Enterococcus faecalis (N = 122)
E. faecalis data were limited to isolates from adults (Table 5). All isolates were susceptible to ampicillin, penicillin, teicoplanin, vancomycin, daptomycin and tigecycline, with 98.1% of isolates susceptible to linezolid.
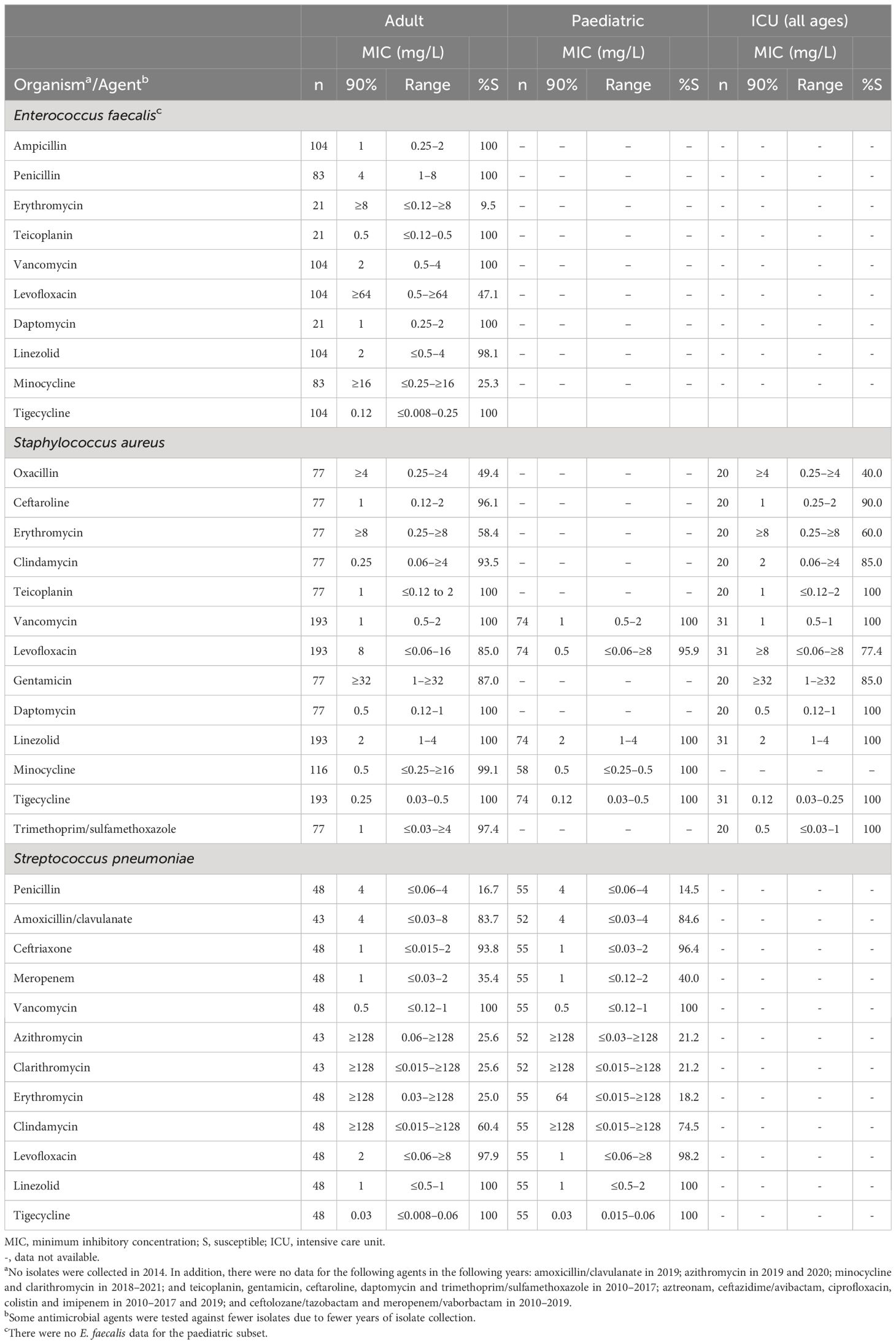
Table 5. In vitro activity and antimicrobial susceptibility of the ATLAS antimicrobial panel against Gram-positive clinical isolates collected in Jordan between 2010 and 2021.
3.4.2 Staphylococcus aureus (N = 267)
Overall, 157 (58.8%) of S. aureus isolates in this study were MRSA. No vancomycin-intermediate or vancomycin-resistant isolates of S. aureus from adult or paediatric patients were identified (Table 5). In addition, > 99% of S. aureus from adult and paediatric patients were susceptible to linezolid, minocycline and tigecycline and. At least 90% of isolates from adults were also susceptible to ceftaroline, clindamycin, teicoplanin, daptomycin, and trimethoprim/sulfamethoxazole (paediatric data not available). Approximately 96% of isolates from paediatric patients were susceptible to levofloxacin, compared with 85.0% of isolates from adults.
All S. aureus isolates from the ICU were susceptible to teicoplanin, vancomycin, daptomycin, linezolid, tigecycline and trimethoprim/sulfamethoxazole, and 90.0% were susceptible to ceftaroline (Table 5). Similar rates of resistance were seen for MRSA (Table 6). In addition, 99.1% of MRSA were susceptible to minocycline.
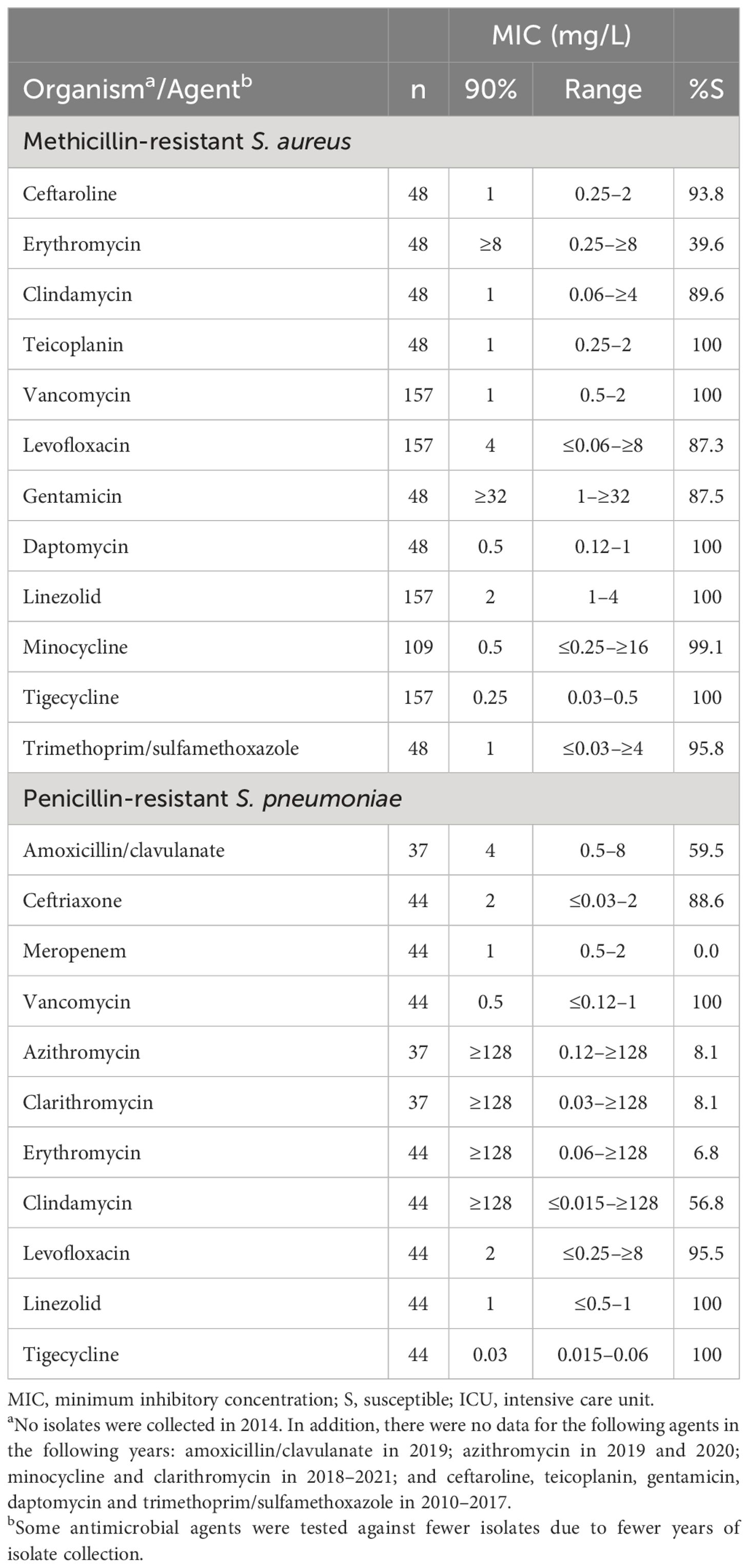
Table 6. In vitro activity and antimicrobial susceptibility of the ATLAS antimicrobial panel against clinical isolates of Staphylococcus aureus and Streptococcus pneumoniae with resistance phenotypes collected in Jordan between 2010 and 2021.
3.4.3 Streptococcus pneumoniae (N = 105)
All isolates from adult and paediatric patients were susceptible to vancomycin, linezolid and tigecycline (Table 5). There were also high rates of susceptibility to ceftriaxone and levofloxacin among both adult and paediatric groups (93.8%–98.2%). No S. pneumoniae isolates were collected from ICUs. All penicillin-resistant S. pneumoniae were susceptible to vancomycin, linezolid, tigecycline with 95.5% and 88.6% susceptible to levofloxacin and ceftaroline, respectively (Table 6). All penicillin-resistant S. pneumoniae isolates were resistant to meropenem.
4 Discussion
This study of bacterial isolates collected from Jordan between 2010 and 2021 found that susceptibility to ceftazidime/avibactam and ceftolozane/tazobactam was high among E. coli, and P. aeruginosa collected from adult patients (> 88%), and was also high to meropenem and meropenem/vaborbactam among E. coli from adults (> 97%). The highest susceptibility among K. pneumoniae isolates from adult patients was to tigecycline (94.9%). Where data were available, similar results to those reported for adults were seen for isolates from paediatric patients and for patients from the ICU, although there was more variation in susceptibility between the different subsets for isolates of A. baumannii and K. pneumoniae. The analysis of MDR and CR Gram-negative isolates demonstrated the lack of activity of many agents against these difficult to treat organisms. In addition, antimicrobial susceptibility among isolates of E. coli and K. pneumoniae carrying β-lactamase genes to most agents, was ≤ 60.0%. Ceftaroline susceptibility was ≥ 90.0% against S. aureus, including MRSA isolates and all Gram-positive isolates collected as part of this study in Jordan were susceptible to tigecycline.
There are limited published antimicrobial surveillance data from Jordan on novel agents, such as ceftazidime/avibactam, ceftolozane/tazobactam, meropenem/vaborbactam and ceftaroline. A multi-hospital study of MDR Gram-negative isolates from Jordan reported susceptibility rates of 73% for ceftazidime/avibactam and 62% for ceftolozane/tazobactam among CR P. aeruginosa collected between 2019 and 2020 (Al Ramahi et al., 2021). Higher susceptibility rates for ceftazidime/avibactam and ceftolozane/tazobactam were reported in the current study against P. aeruginosa from adults (95.3% and 88.4%, respectively); however, this was for P. aeruginosa irrespective of carbapenem activity. Only two isolates of P. aeruginosa among the 210 collected in the present study were found to carry β-lactamase genes [1 isolate carrying VIM (type not recorded) and 1 isolate carrying VIM-4 (Supplemental Data, Supplementary Table 2)], indicating that P. aeruginosa from these patients had not acquired a high proportion of resistance genes during the study period. A 2017–2020 SMART study found that 81.1% of all P. aeruginosa isolates from Jordan were susceptible to ceftolozane/tazobactam, in agreement with the current study (Lob et al., 2022). Similar to the present study data, a 2015–2018 study of Gram-positive isolates from skin and soft tissue infections or respiratory tract infections reported a ceftaroline MIC of < 2 mg/L for all S. aureus, (including MRSA) (Karlowsky et al., 2021b).
The suggested emerging pathogen threats to paediatric patients are MRSA, vancomycin-resistant S. aureus, and ESBL-producing and CR Enterobacterales (Romandini et al., 2021). In the current study, ≥ 94.0% of E. coli and K. pneumoniae from paediatric patients were susceptible to meropenem, and all S. aureus were susceptible to vancomycin. Among the newer agents tested, paediatric data were not available for ceftazidime/avibactam or ceftaroline, which are active against the aforementioned emerging pathogens and approved for paediatric infections. A 2012–2019 surveillance study of isolates from paediatric patients in Kuwait showed that most Enterobacterales (including resistance phenotypes) and P. aeruginosa were susceptible to ceftazidime/avibactam, and most Gram-positive isolates, including resistance phenotypes, were susceptible to ceftaroline (Al-Sweih et al., 2021). Thus, ceftazidime/avibactam and ceftaroline represent promising options for paediatric infections, including those caused by antimicrobial-resistant organisms.
In the present study, the most frequent β-lactamase gene identified among E. coli and K. pneumoniae isolates from Jordan was CTX-M-15. CTX-M-15 was also the most frequent ESBL reported in previous studies from Kuwait, Jordan and Lebanon (Al-Sweih et al., 2021; Hayajneh et al., 2015; Moubareck et al., 2005). Meropenem. amikacin and tigecycline were active against CTX-M-15-positive E. coli (80.0%–96.7% susceptible) in the current study, while tigecycline was also active against K. pneumoniae isolates harbouring CTX-M-15 (95.9% susceptible). In contrast, the susceptibility of CTX-M-15-positive K. pneumoniae to ceftazidime/avibactam in the present study was ≤ 39.0%. Research has shown that the CTX-M-15 enzyme purified from E. coli demonstrated an increased catalytic activity against ceftazidime than other types of CTX-M ESBLs (Poirel et al., 2002), which could explain the low susceptibility to ceftazidime/avibactam.
Overall, 15.7% of K. pneumoniae and 0.8% of E. coli (39 and 2 isolates, respectively) were CR. Carbapenem-resistance would appear to be becoming an increasing problem in Jordan with the CR K. pneumoniae in this study emerging since 2015 (data not shown) and the E. coli collected in 2020 and 2021. The majority of these isolates were carbapenemase-positive (35/41, 85.4%); however, 6 isolates (5 K. pneumoniae, 1 E. coli) were not carbapenemase-positive, suggesting that carbapenem-resistance was mediated through other mechanisms in this small number of isolates. Alternative mechanisms of resistance can be either porin-mediated or through efflux pumps (Elshamy and Aboshanab, 2020). The ATLAS programme does not have methodology in place to detect such mechanisms and this word be a beneficial addition to the programme as CR isolates continue to develop and pose a challenge to effective antimicrobial treatment.
Of concern is the marked lack of antimicrobial activity against K. pneumoniae that were NDM-5-positive (100% resistance or nonsusceptibility to 84% of tested agents with breakpoints), CTX-M-9-type-positive (100% resistance or nonsusceptibility to 71% of agents with breakpoints), and OXA-48-positive (100% resistance or nonsusceptibility to 59% of tested agents with breakpoints). K. pneumoniae carrying CTX-M-9-type genes tended to exhibit lower rates of antimicrobial susceptibility to the majority of agents, except for tigecycline and trimethoprim/sulfamethoxazole, than isolates that were CTX-M-15-positive. A 2012–2013 study of K. pneumoniae in Jordan found that, among carbapenemase-producers CTX-M-9 was harboured by both NDM- and OXA-producers (Aqel et al., 2017). Although CTX-M-9 enzymes have demonstrated higher catalytic activity against cefotaxime than ceftazidime, compared with other tested CTX-M-type enzymes (Bonnet et al., 2001), the co-carriage of CTX-M-9 with OXA-48-like or NDM-5 genes would appear to result in a loss of antimicrobial activity for most agents, with only tigecycline active against all of these subsets. The MIC90 of aztreonam/avibactam was 0.25 mg/L against each subset of β-lactamase-positive K. pneumoniae (versus aztreonam MIC90 values of 128–≥ 128 mg/L), similar to previous global surveillance studies (Biedenbach et al., 2015; Esposito et al., 2021). To date, clinical data have shown that aztreonam/avibactam has been effective and well-tolerated, with a similar safety profile to aztreonam alone (Pfizer Inc, 2023). This agent could therefore represent a valuable future treatment option. In addition, as MBL-producing pathogens are often also MDR through the co-production of other β-lactamases, such as ESBLs, ceftazidime/avibactam may be used in combination with aztreonam in clinical practice to target a greater variety of β-lactamases than each agent alone (Falcone et al., 2021).
We recognise a number of limitations with the ATLAS programme and our analysis. Although the ATLAS surveillance programme is a valuable tool for the monitoring and tracking of antimicrobial activity, the study protocol defines the number of isolates from each species that can be submitted by each centre, each year; therefore, the study cannot be considered to assess the prevalence of antimicrobial-resistant bacteria. The yearly participation of each medical centre in Jordan varied (with no centres participating in 2014) and there was some variation in the number of isolates tested against each antimicrobial agent by year, because of changes in the antimicrobial testing panel, which prevented a year-by-year analysis. In addition, as not all isolates that met the criteria for molecular testing in 2020 and 2021 were tested, there are a small number of isolates which may have carried β-lactamase genes that were not identified. As with all surveillance studies, caution should be exercised in extrapolating surveillance data; nevertheless, the data we report present a picture of the antimicrobial susceptibilities among clinical isolates from adult and paediatric patients in Jordan.
In summary, A. baumannii presents considerable challenges to treatment because of the high percentages of MDR and CR (> 90% of isolates). In contrast, most of the tested P. aeruginosa isolates collected from patients in Jordan were susceptible to ceftazidime/avibactam and ceftolozane/tazobactam. Aztreonam/avibactam was active against isolates of E. coli and K. pneumoniae, including β-lactamase-positive K. pneumoniae, although no breakpoints are currently available to determine susceptibility. Tigecycline was active against most Gram-negative and all Gram-positive isolates from adult and paediatric patients, and the majority of S. aureus isolates were susceptible to ceftaroline. In additional to tigecycline, all S. aureus were susceptible to teicoplanin, vancomycin, daptomycin and linezolid and all S. pneumoniae were susceptible to vancomycin, linezolid and tigecycline. CR and MDR Gram-negative isolates exhibited susceptibility rates of < 60% to most antimicrobial agents. CTX-M-15 was the most frequently identified clinically significant β-lactamase gene among E. coli and K. pneumoniae, which exhibited reduced susceptibility to most agents. In conclusion, the data presented here offer vital information to clinicians and infectious disease specialists to allow them to make informed decisions on appropriate antimicrobial prescribing, based on antimicrobial activity.
Data availability statement
The original contributions presented in the study are publicly available. This data can be found here: NCBI RefSeq - resistance gene CTX-M-15, accession NG_048935.1; resistance gene CTX-M-9, accession NG_049043.1; resistance gene OXA-48, accession NG_049762.1; resistance gene NDM-5, accession NG_049337.1.
Ethics statement
Ethical approval was not required for the study involving humans in accordance with the local legislation and institutional requirements. Written informed consent to participate in this study was not required from the participants or the participants’ legal guardians/next of kin in accordance with the national legislation and the institutional requirements.
Author contributions
DA: Writing – original draft, Writing – review & editing. JB: Writing – original draft, Writing – review & editing. JM: Writing – original draft, Writing – review & editing. JA: Writing – original draft, Writing – review & editing.
Funding
The author(s) declare financial support was received for the research, authorship, and/or publication of this article. This study received funding from Pfizer, Inc. The funder was involved in the study design, the writing of this article, and the decision to submit it for publication. Medical writing and editorial support (provided by Neera Hobson, PhD, at Micron Research Ltd, Ely, UK), and data management services (provided by Micron Research Ltd, Ely, UK), were funded by Pfizer, Inc.
Acknowledgments
The authors wish to thank all participating ATLAS global surveillance programme investigators and laboratories, as well as the central reference laboratory, International Health Management Associates, Inc., for their coordination of the study.
Conflict of interest
JB and JM are employed by Pfizer Inc., Lebanon. The remaining authors declare that the research was conducted in the absence of any commercial or financial relationships that could be construed as a potential conflict of interest.
This study received funding from Pfizer, Inc. The funder was involved in the study design, the writing of this article, and the decision to submit it for publication. Medical writing and editorial support (provided by Neera Hobson, PhD, at Micron Research Ltd, Ely, UK), and data management services (provided by Micron Research Ltd, Ely, UK), were funded by Pfizer, Inc.
Publisher’s note
All claims expressed in this article are solely those of the authors and do not necessarily represent those of their affiliated organizations, or those of the publisher, the editors and the reviewers. Any product that may be evaluated in this article, or claim that may be made by its manufacturer, is not guaranteed or endorsed by the publisher.
Supplementary material
The Supplementary Material for this article can be found online at: https://www.frontiersin.org/articles/10.3389/frabi.2024.1375980/full#supplementary-material
References
Al Ramahi J. W., Said M., Abu Kwaik R., Jamal W., Al Jammal D., Al Radaidah N., et al. (2021). Susceptibility of the multidrug-resistant nosocomial pathogens isolates in Jordan for the new antimicrobial agents. Int. Arabic. J. Antimicrobial. Agents 11, 1–8. doi: 10.3823/852
Al-Sweih N., Jamal W., Mokaddas E., Habashy N., Kurdi A., Mohamed N. (2021). Evaluation of the in vitro activity of ceftaroline, ceftazidime/avibactam and comparator antimicrobial agents against clinical isolates from paediatric patients in Kuwait: ATLAS data 2012–19. JAC. Antimicrob. Resist. 3, dlab159. doi: 10.1093/jacamr/dlab159
Antimicrobial Resistance Collaborators (2022). Global burden of bacterial antimicrobial resistance in 2019: a systematic analysis. Lancet 399, 629–655. doi: 10.1016/S0140-6736(21)02724-0
Antimicrobial Testing Leadership and Surveillance (ATLAS) database. (2023). Available at: https://atlas-surveillance.com.
Antimicrobial Testing Leadership and Surveillance (ATLAS) Definitions. (2023). Available at: https://atlas-surveillance.com/#/resources/definitions.
Aqel A. A., Giakkoupi P., Alzoubi H., Masalha I., Ellington M. J., Vatopoulos A. (2017). Detection of OXA-48-like and NDM carbapenemases producing Klebsiella pneumoniae in Jordan: A pilot study. J. Infect. Public Health 10, 150–155. doi: 10.1016/j.jiph.2016.02.002
Biedenbach D. J., Kazmierczak K., Bouchillon S. K., Sahm D. F., Bradford P. A. (2015). In vitro activity of aztreonam-avibactam against a global collection of Gram-negative pathogens from 2012 and 2013. Antimicrob. Agents Chemother. 59, 4239–4248. doi: 10.1128/AAC.00206-15
Bonnet R., Dutour C., Sampaio J. L., Chanal C., Sirot D., Labia R., et al. (2001). Novel cefotaximase (CTX-M-16) with increased catalytic efficiency due to substitution Asp-240→Gly. Antimicrob. Agents Chemother. 45, 2269–2275. doi: 10.1128/AAC.45.8.2269-2275.2001
CLSI (2018). Methods for Dilution Antimicrobial Susceptibility Tests for Bacteria That Grow Aerobically: Eleventh Informational Supplement M07-S11. (Wayne, P. aeruginosa, USA: CLSI).
CLSI (2023). Performance Standards for Antimicrobial Susceptibility Testing: Thirty-Third Informational Supplement M100-S33 (Wayne, PA, USA: CLSI).
Elshamy A. A., Aboshanab K. M. (2020). A review on bacterial resistance to carbapenems: epidemiology, detection and treatment options. Future Sci. OA 6, FSO438. doi: 10.2144/fsoa-2019-0098
Esposito S., Stone G. G., Papaparaskevas J. (2021). In vitro activity of aztreonam/avibactam against a global collection of Klebsiella pneumoniae collected from defined culture sources in 2016 and 2017. J. Glob. Antimicrob. Resist. 24, 14–22. doi: 10.1016/j.jgar.2020.08.004
Estabrook M., Muyldermans A., Sahm D., Piérard D., Stone G., Utt E. (2023). Epidemiology of resistance determinants identified in meropenem-nonsusceptible Enterobacterales collected as part of a global surveillance study 2018 to 2019. Antimicrob. Agents Chemother. 67, e0140622. doi: 10.1128/aac.01406-22
Falcone M., Daikos G. L., Tiseo G., Bassoulis D., Giordano C., Galfo V., et al. (2021). Efficacy of ceftazidime-avibactam plus aztreonam in patients with bloodstream infections caused by metallo-β-lactamase-producing Enterobacterales. Clin. Infect. Dis. 72, 1871–1878. doi: 10.1093/cid/ciaa586
FDA. (2023). Tigecycline – Injection products. Available online at: https://www.fda.gov/drugs/development-resources/tigecycline-injection-products.
Hayajneh W. A., Hajj A., Hulliel F., Sarkis D. K., Irani-Hakimeh N., Kazan L., et al. (2015). Susceptibility trends and molecular characterization of Gram-negative bacilli associated with urinary tract and intra-abdominal infections in Jordan and Lebanon: SMART 2011-2013. Int. J. Infect. Dis. 35, 56–61. doi: 10.1016/j.ijid.2015.04.011
Karlowsky J. A., Bouchillon S. K., El Mahdy Kotb R., Mohamed N., Stone G. G., Sahm D. F. (2021a). In vitro activity of ceftazidime/avibactam against clinical isolates of Enterobacterales and Pseudomonas aeruginosa from Middle Eastern and African countries: ATLAS global surveillance programme 2015-18. JAC. Antimicrob. Resist. 3, dlab067. doi: 10.1093/jacamr/dlab067
Karlowsky J. A., Bouchillon S. K., El Mahdy Kotb R., Mohamed N., Stone G. G., Sahm D. F. (2022). Carbapenem-resistant Enterobacterales and Pseudomonas aeruginosa causing infection in Africa and the Middle East: a surveillance study from the ATLAS programme, (2018-20). JAC. Antimicrob. Resist. 4, dlac060. doi: 10.1093/jacamr/dlac060
Karlowsky J. A., Hackel M. A., Bouchillon S. L. K., Lowman W., Kotb R. E. M., Mohamed N., et al. (2021b). In vitro activity of ceftaroline against bacterial pathogens isolated from patients with skin and soft tissue and respiratory tract infections in the Middle East and Africa: AWARE global surveillance programme 2015–2018. J. Glob. Antimicrob. Resist. 24, 249–256. doi: 10.1016/j.jgar.2020.12.013
Kazmierczak K. M., Bradford P. A., Stone G. G., de Jonge B. L. M., Sahm D. F. (2018). In vitro activity of ceftazidime-avibactam and aztreonam-avibactam against OXA-48-carrying Enterobacteriaceae isolated as part of the International Network for Optimal Resistance Monitoring (INFORM) global surveillance program from 2012 to 2015. Antimicrob. Agents Chemother. 62, e00592–e00518. doi: 10.1128/AAC.00592-18
Lob S. H., Estabrook M. A., DeRyke C. A., Alekseeva I., Siddiqui F., Young K., et al. (2022). Activity of ceftolozane/tazobactam against clinical isolates of Pseudomonas aeruginosa from patients in the Middle East and Africa –Study for Monitoring Antimicrobial Resistance Trends (SMART) 2017-2020. Int. J. Infect. Dis. 125, 250–257. doi: 10.1016/j.ijid.2022.10.014
Lob S. H., Kazmierczak K. M., Badal R. E., Hackel M. A., Bouchillon S. K., Biedenbach D. J., et al. (2015). Trends in susceptibility of Escherichia coli from intra-abdominal infections to ertapenem and comparators in the United States according to data from the SMART program 2009 to 2013. Antimicrob. Agents Chemother. 59, 3606–3610. doi: 10.1128/AAC.05186-14
Moubareck C., Daoud Z., Hakime N. I., Hamze M., Mangeney N., Matta H., et al. (2005). Countrywide spread of community- and hospital-acquired extended-spectrum beta-lactamase (CTX-M-15)-producing Enterobacteriaceae in Lebanon. J. Clin. Microbiol. 43, 3309–3313. doi: 10.1128/JCM.43.7.3309-3313.2005
Pfizer Inc. (2023). Phase 3 studies of Pfizer’s novel antibiotic combination offer new treatment hope for patients with multidrug-resistant infections and limited treatment options. Available online at: https://www.pfizer.com/news/press-release/press-release-detail/phase-3-studies-pfizers-novel-antibiotic-combination-offer.
Piérard D., Stone G. G. (2021). In vitro antimicrobial susceptibility of clinical respiratory isolates to ceftazidime-avibactam and comparators, (2016-2018). BMC Infect. Dis. 21, 600. doi: 10.1186/s12879-021-06153-0
Poirel L., Gniadkowski M., Nordmann P. (2002). Biochemical analysis of the ceftazidime-hydrolysing extended-spectrum beta-lactamase CTX-M-15 and of its structurally related beta-lactamase CTX-M-3. J. Antimicrob. Chemother. 50, 1031–1034. doi: 10.1093/jac/dkf240
Romandini A., Pani A., Schenardi P. A., Pattarino G. A. C., De Giacomo C., Scaglione F. (2021). Antibiotic resistance in pediatric infections: global emerging threats, predicting the near future. Antibiot. (Basel). 10, 393. doi: 10.3390/antibiotics10040393
Tacconelli E., Carrara E., Savoldi A., Harbarth S., Mendelson M., Monnet D. L., et al. (2018). Discovery, research, and development of new antibiotics: the WHO priority list of antibiotic-resistant bacteria and tuberculosis. Lancet Infect. Dis. 18, 318–327. doi: 10.1016/S1473-3099(17)30753-3
WHO. (2021). Fact sheets: Antimicrobial resistance. Available online at: https://www.who.int/news-room/fact-sheets/detail/antimicrobial-resistance.
Wilson G. M., Fitzpatrick M., Walding K., Gonzalez B., Schweizer M. L., Suda K. J., et al. (2021). Meta-analysis of clinical outcomes using ceftazidime/avibactam, ceftolozane/tazobactam, and meropenem/vaborbactam for the treatment of multidrug-resistant Gram-negative infections. Open Forum Infect. Dis. 8, ofaa651. doi: 10.1093/ofid/ofaa651
Wise M. G., Karlowsky J. A., Mohamed N., Kamat S., Sahm D. F. (2023). In vitro activity of aztreonam-avibactam against Enterobacterales isolates collected in Latin America, Africa/Middle East, Asia, and Eurasia for the ATLAS Global Surveillance Program in 2019-2021. Eur. J. Clin. Microbiol. Infect. Dis. 42, 1135–1143. doi: 10.1007/s10096-023-04645-2
Keywords: antimicrobial resistance, antimicrobial surveillance, clinical isolates, beta-lactamases, phenotypes, Jordan
Citation: Al Jammal D, Bachir J, Moussa JA and Al Ramahi JW (2024) In vitro antimicrobial susceptibility of clinical isolates from adult and paediatric patients in Jordan: Antimicrobial Testing Leadership and Surveillance (ATLAS) 2010–2021. Front. Antibiot. 3:1375980. doi: 10.3389/frabi.2024.1375980
Received: 24 January 2024; Accepted: 18 June 2024;
Published: 08 August 2024.
Edited by:
Md Latiful Bari, University of Dhaka, BangladeshReviewed by:
Moiz Ashraf Ansari, Texas A and M University, United StatesLuthfunnesa Bari, Mawlana Bhashani Science and Technology University, Bangladesh
Copyright This work is authored by Al Jammal, Bachir, Moussa, and Al Ramahi. © 2024 Al Jammal, Al Ramahi, and © 2024 Pfizer Inc. This is an open-access article distributed under the terms of the Creative Commons Attribution License (CC BY). The use, distribution or reproduction in other forums is permitted, provided the original authors and the copyright owner are credited and the original publication in this journal is cited, in accordance with accepted academic practice. No use, distribution or reproduction is permitted which does not comply with these terms.
*Correspondence: Jamal Wadi Al Ramahi, jamalwadimd@yahoo.com
†ORCID: Jamal Wadi Al Ramahi, orcid.org/0000-0003-2242-6232