- 1Department of Food Technology and Nutritional Science, Mawlana Bhashani Science and Technology University, Tangail, Bangladesh
- 2Department of Chemistry, Bangladesh University of Health Science, Dhaka, Bangladesh
- 3Department of Biochemistry and Molecular Biology, Mawlana Bhashani Science and Technology University, Tangail, Bangladesh
Introduction: The misuse of antibiotics in poultry farming is a global issue.
Objective: The focus of this study was the health risk assessment of consumers from the determination of ciprofloxacin (CIP), tetracycline (TC), and oxytetracycline (OTC) in broiler chicken in the raw, frozen, and boiled stages using solid-phase extraction, high-performance liquid chromatography, and ultraviolet detection (SPE-HPLC-UV).
Materials and methods: Chromatographic separation was achieved using 0.3% metaphosphoric acid and acetonitrile (1:10, v/v) for CIP at 280 nm and oxalic acid (0.01 M) and acetonitrile (1:1, v/v) for TC and OTC at 355 nm with different retention times. The method had an acceptable precision with good linearity, specificity, limit of detection, limit of quantification, accuracy, and stability.
Results: Among a total of 252 raw samples, approximately 68.25%, 25.4%, and 7.54% contained CIP, TC, and OTC, respectively. Out of the positive raw samples, CIP exceeded the maximum residual limit (MRL) in 3.6% muscle, 14.3% liver and 17.9% skin samples, whereas TC and OTC were below the MRLs. The residual concentrations of these antibiotics were almost unchanged in frozen samples. After boiling the chicken samples, the TC and OTC residues were reduced significantly compared to CIP. Although the concentrations of CIP in boiled samples were above the MRL set by the European Union, these did not exceed the hazard index 1.
Conclusion: Based on these results, the exposure levels to antibiotics in broiler chicken meats may be considered to have a low risk for human health.
Introduction
Since their discovery and development, antibiotics have been frequently used as veterinary and human medicines to treat various infectious diseases (Samanidou et al., 2007). They can either have activity against a broad spectrum of microorganisms or can be active against a small extent of specific organisms (Samanidou et al., 2006; Samanidou and Evaggelopoulou, 2015). As veterinary drugs, antibiotics play a vital role in the maximal production of industrial farm animals and in reducing the morbidity and mortality rates among livestock (Page and Gautier, 2012). Ciprofloxacin (CIP), tetracycline (TC), and oxytetracycline (OTC) are the most frequently applied veterinary drugs in Bangladesh. The main causes of antibiotic residues include the unauthorized use and unrestricted administration of veterinary medications to healthy animals, as well as failing to consider the withdrawal period, not seeking professional advice, using medications beyond the recommended dosage, administering medications frequently, and increasing dosing (Baran et al., 2011; Croubels and Daeseleire, 2012; Bitas et al., 2018). These residues have the potential to be hazardous to human health and can trigger allergic reactions. In addition, the prolonged accumulation of CIP, TC, and OTC residues can lead to the growth of microorganisms that cause antibiotic resistance (Garcia et al., 2004; Fan et al., 2020). To ensure the reduction of the quantity of veterinary drugs from the edible parts of farm animals, a minimum withdrawal time between the administration of antibiotics and the slaughter has been established (Croubels and Daeseleire, 2012). The European Union (EU) has established maximum residual limits (MRLs) for residues of veterinary pharmaceuticals, including CIP, TC, and OTC, in animal tissues and derivative foods entering the human food chain in Council Regulation EEC 37/2010 and its later modification [Commission Regulation (EU) No. 37/2010 of 22 December 2009, L.15/1-72]. Monitoring is therefore required to ensure that antibacterial agents are not present in quantities that could endanger the health of the general public. Recently, multi-residue techniques have been created for a variety of residue classes observed in animal tissues, including fluoroquinolones (Schneider et al., 2005; Verdon et al., 2005) and TCs (Anderson et al., 2003; Cinquina et al., 2003a). HPLC has a reputation for being a reliable and highly selective separative tool (Almalki et al., 2021) for detecting antibiotic residues. Although a lot of initiatives have been taken from government regulatory bodies, the misuse of antibiotics is still occurring. The presence of antibiotic residues in poultry food has become a matter of concern as its resistance is considered as a health risk. Therefore, annual monitoring is mandatory. The aims of the present study were to determine CIP, TC, and OTC residues from poultry samples collected from Tangail Sadar Upazila using HPLC and to assess their risks to consumer health.
Experimental
Sampling
For this study, a total of 252 breast muscle, liver, and skin samples at raw, cooked, and frozen stages were prepared from 56 fresh broiler chickens. These chickens, weighing 1.0–2.0 kg, were purchased from seven traditional local bazaars in Tangail Sadar Upazila, Bangladesh. Upon collection, all samples were first defeathered and placed in clean, sterile polyethylene bags, which were then transported to the laboratory as quickly as possible. Frozen samples were then immediately stored in a refrigerator (WFA-2A3-ELXX-XX, Walton, Bangladesh) at −2°C for 3 days to determine the quantity of antibiotic residues in each sample.
Chemicals and reagents
Ciprofloxacin hydrochloride (C17H21CLFN3O4, 385.81 g/mol), a fine crystalline powder, and 98% oxytetracycline hydrochloride (C22H24N2O9·HCL, 496.90 g/mol), a yellow crystalline powder, were purchased from the International Laboratory (South San Francisco, CA, USA). Tetracycline hydrochloride (C22H24N2O8·HCL, 480.91 g/mol) was obtained from Alfa Aesar (Thermo Fisher Scientific, Lancashire, UK). HPLC grade methanol was purchased from Thermo Scientific™ (Princeton, NJ, USA), and acetonitrile was manufactured by J.T.Baker®, Avantor® Performance Materials LLC (Center Valley, PA, USA). Analytical grade oxalic acid (HO2CCO2H·2H2O, 126.07 g/mol) was from BDH Laboratory Supplies (Poole, UK). HPLC grade acetic acid (CH3COOH, 100%) was purchased from Sigma-Aldrich (Merck, Darmstadt, Germany). Meta-phosphoric acid (HPO3, 39%–43%) was obtained from Alfa Aesar (Thermo Fisher Scientific, Lancashire, UK). Distilled and deionized water, with resistivity >18 MΩ cm−1 at 25°C and total organic carbon (TOC) <5 ppb before extraction in the laboratory, was obtained from Labconco 9000620 WaterPro PS-series (Kansas City, MO, USA).
Instrumentation
For the chromatographic determination of CIP, TC, and OTC, an LC-20AT pump equipped with a Shimadzu CBM-20A system controller (Kyoto, Japan) permitting an automated operation that delivers the solvent system to the analytical column was employed. A Rok 5 µm C18, 100 Å, 250 × 4.6-mm analytical column (Restek, PA, USA) was used. Injection of the sample was manually accomplished through a Rheodyne™ 7125i injection valve equipped with a 20-µl loop. Detection was performed using a UV detector at a wavelength of 280 nm for CIP and at 355 nm for TC and OTC, which complied with the LabSolutions software by Shimadzu for data acquisition. Mobile phases were degassed with a glass vacuum filter (IDEX Corporation, Benton Harbor, MI, USA) using Whatman filter paper (PALL Corporation, Mumbai, India). A TRXA-C vortex (TRIU, China) and a UBT-580 ultrasonic bath (Unilab, USA) were used for proper homogenization. The samples were extracted with a 50-ml Sorvall-ST-8R centrifuge (Thermo Fisher Scientific, Osterode, Germany) and a rotary vacuum evaporator (2610000; Witeg, Wertheim, Germany). A 3-ml Resprep C18 cartridge (26031; Restek, PA, USA) was used for the cleanup procedure. Table 1 shows the experimental conditions for HPLC for the determination of CIP, TC, and OTC.
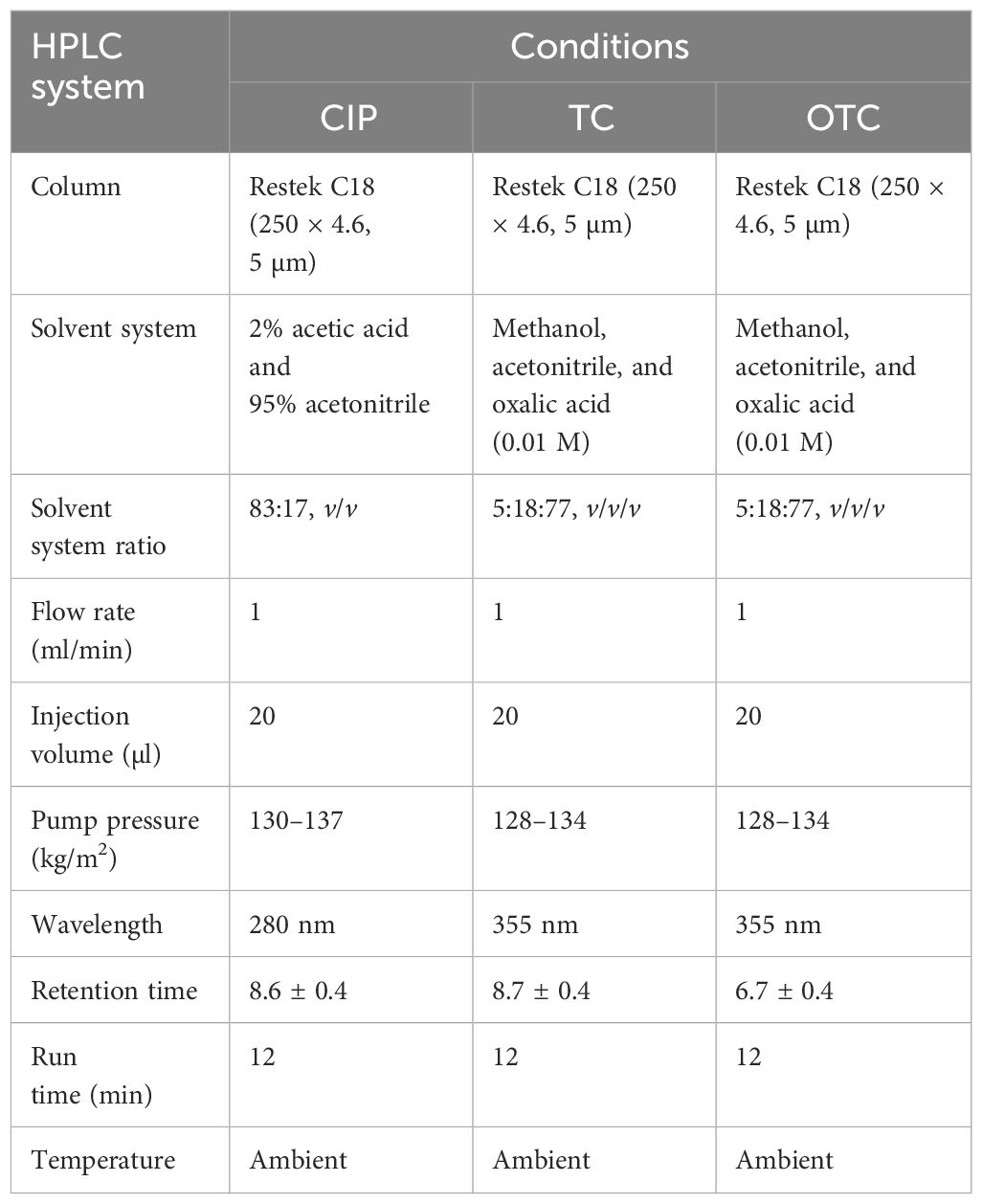
Table 1 Chromatographic conditions for HPLC for the determination of ciprofloxacin (CIP), tetracycline (TC), and oxytetracycline (OTC).
Preparation of standard and sample extraction
Aqueous stock solutions of 100 µg/ml were prepared by taking 5 mg of each CIP, TC, and OTC standard in a 50-ml volumetric flux and made up to the mark by dissolving them (2% acetic acid and 100% acetonitrile in a ratio 82:18, v/v, for CIP and pure methanol for TC and OTC). The stock solutions were found to be stable for at least 1 month when wrapped with paraffin wax and stored at refrigerated temperature (4°C). Working standard concentrations of 0.0195, 0.039, 0.078, 0.156, 0.195, 0.3125, 0.39, 0.625, 0.78, 1.25, 1.56, 2.5, 3.125, and 5 µg/ml were prepared from stock solution using the same dilution solvents.
A precise weight of 5 ± 0.02 g by analytical balance (PH224; OHAUS Corporation, Parsippany, NJ, USA) from each muscle, liver, and skin sample at three stages (i.e., raw, boiled, and frozen) was blended separately using a blender (MX-AC300; Panasonic Corporation, Gurugram, India) or smashed with a mortar and pestle and then transferred into a 50-ml centrifuge tube. Fortified samples were prepared by adding the working solutions of CIP, TC, and OTC at various concentrations with the smashed samples. Subsequently, 15 ml of the extraction solvent [0.3% meta-phosphoric acid and acetonitrile (1:10, v/v) for CIP and 0.01 M oxalic acid and acetonitrile (1:1, v/v) for TC and OTC] was added into the centrifuge tube. The mixture was homogenized using a vortex for approximately 10 min, followed by sonication at 30°C for 10 min. The mixture was then centrifuged at 9,000 rpm at 21°C for approximately 10 min. The residual liquid was centrifuged again under the same conditions. For CIP determination, the supernatant was collected with a 1,000-µl micropipette from the rotary evaporator flask, which was partially evaporated at 40°C for 3–4 min prior to centrifugation. Solid phase extraction (SPE) was carried out with the help of C18 cartridges for the cleanup procedure, which was preconditioned by passing 3 ml methanol and 3 ml HPLC grade deionized water. The sample was then filtered using a Sterlitech (Kent, WA, USA), 25 mm, 0.22 µm, hydrophilic (PTFE) syringe filter, and an aliquot of 20 µl was injected into the HPLC system. The frozen (−2°C) and boiled (100°C) samples were prepared using the same process after thawing and cooling at room temperature.
Method validation
The proposed HPLC analytical method was appropriately evaluated in terms of specificity, linearity, sensitivity, accuracy, precision, and stability using both working standard solutions and spiked samples of muscle, liver, and skin in accordance with the European Community’s defined validation protocol for antibiotic residues in food items of animal origin (Communities, 2002).
Linearity evaluation was performed by injecting seven working standards (range, 0.0195–5 µg/ml) for CIP and five different concentrations each for TC and OTC (0.195, 0.39, 0.78, 1.56, and 3.125 µg/ml) with two replicate injections. The working concentrations were prepared by diluting the stock solutions with dilution solvents. The calibration curves with respective correlation coefficients (R2) were obtained by linear regression analysis. In terms of recovery, accuracy analysis was performed in spiked samples with working standards at four concentration levels (i.e., 250, 500, 1,250, and 2,000 µg/kg) for CIP and at three concentration levels (i.e., 500, 1,000, and 1,250 µg/kg) each for TC and OTC. Repeatability for precision study was performed by diluting the stock standard solution at different concentrations. The intra-assay repeatability at two concentration levels was analyzed three different times within a day. Inter-assay repeatability was performed comparing three consecutive days by assessing the same concentrations. The results were expressed using relative standard deviation. The limit of detection (LOD) and limit of quantification (LOQ) were used to assess the sensitivity of the method. The LOD and LOQ were established at concentrations where, under the circumstances of our HPLC, the signal-to-noise (S/N) ratios were equal to 3.3 and 10, respectively, for each antibiotic residue in broiler chicken samples (i.e., muscle, liver, and skin). The LOD and LOQ were calculated based on S/N ratios occupying the following equations, where σ is the SD of the response and s is the slope of the calibration curve. Specificity of the analytical approach can distinguish between the analytes of interest and other compounds that are likely to be present in the sample matrix. The interference of other compounds present in the matrix was verified by injecting 15 different samples for each antibiotic, including a blank, standard, and a spiked sample (five for each). In the case of the HPLC procedure, full separation of the analyte peaks from other interfering signals originated in the sample matrix was ascertained. Since the stability of stock and matrix constituents during analysis at storage may cause significant deviations in the results, monitoring the stability is of paramount importance. For this, both the standard and the sample matrix were verified at room temperature at 0 h and after 24 h at 25°C and 4°C. Three replicate injections were performed each time.
Health risk assessments
Daily exposure to CIP, TC, and OTC of the human body from edible parts of poultry chicken was assessed by calculating the estimated daily intake (EDI), as described by Moudgil et al. (2019a): EDI (µg/kg body weight per day) = C × DIF/BW, where C is the mean concentration of the antibiotic in poultry (in micrograms per gram), DIF is the daily intake of food (in grams per day), and BW is the mean body weight (bw) of adult (60 kg). To calculate EDI, the maximum mean concentration of the antibiotic was considered. According to FAO/WHO, the average daily intake (ADI) for CIP is 2 µg/kg bw per day and that for both TC and OTC is 30 µg/kg bw per day. The Report of the Household Income and Expenditure Survey (2016) published that the poultry meat consumption rate for a 60-kg Bangladeshi adult is 17.33 g/day (Ahmed et al., 2022). In Bangladesh, the consumption rate of liver tissue was estimated at 3 g/person per day (Zeinali et al., 2019). To predict the long-term effects on health, a hazard index (HI) was calculated using the equation HI = EDI/ADI. HI less than 1 denotes that the poultry meat is safe for human consumption. However, 1 ≤ HI ≤ 10 warns a risk, but not emergency, while HI > 10 indicates an unacceptable risk for consumption (Oyedeji et al., 2019).
Statistical analysis
For this research, a descriptive analysis encompassing the mean, standard deviation (SD), and relative standard deviation (RSD) was performed and analyzed using Microsoft Excel 2010. A t-test was conducted using SPSS version 25.
Results
Validation and method performance
The separation and specificity of the SPE-HPLC-UV method for the analysis of CIP, TC, and OTC residues are illustrated in Figure 1 by comparing the chromatograms of the blank, standard, and the sample spiked with antibiotics. No endogenous compound present in the sample matrix appeared to interfere at the retention times of the CIP (8.5 ± 0.4, 280 nm), TC (8.9 ± 0.3, 355 nm), and OTC (6.9 ± 0.3, 355 nm) peaks, showing that the method was extremely specific for this analysis. The chicken samples containing antibiotic residues were spiked with each standard. The obtained peak area was increased to a significant level, which indicated the specificity of the method. The calibration curves in the concentration range from 0.0195 to 5 µg/ml for CIP and from 0.195 to 3.125 µg/ml for both TC and OTC standards showed good linearity (Figure 2).
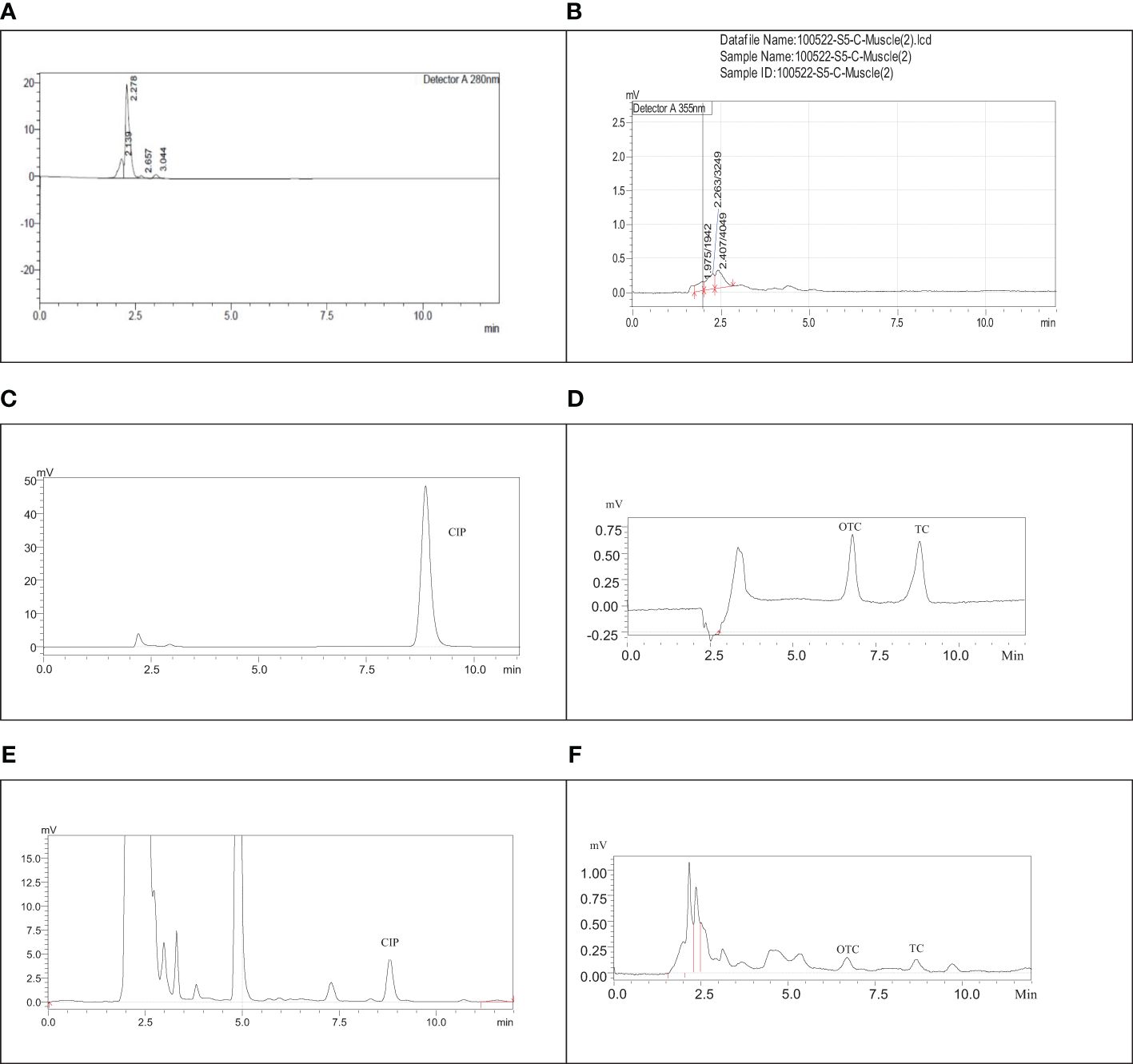
Figure 1 Chromatograms of the blank ciprofloxacin (CIP) (A), blank tetracycline (TC) and oxytetracycline (OTC) (B), CIP standard (C), TC and OTC standard (D), muscle sample spiked with CIP (E), and the skin sample spiked with TC and OTC (F).
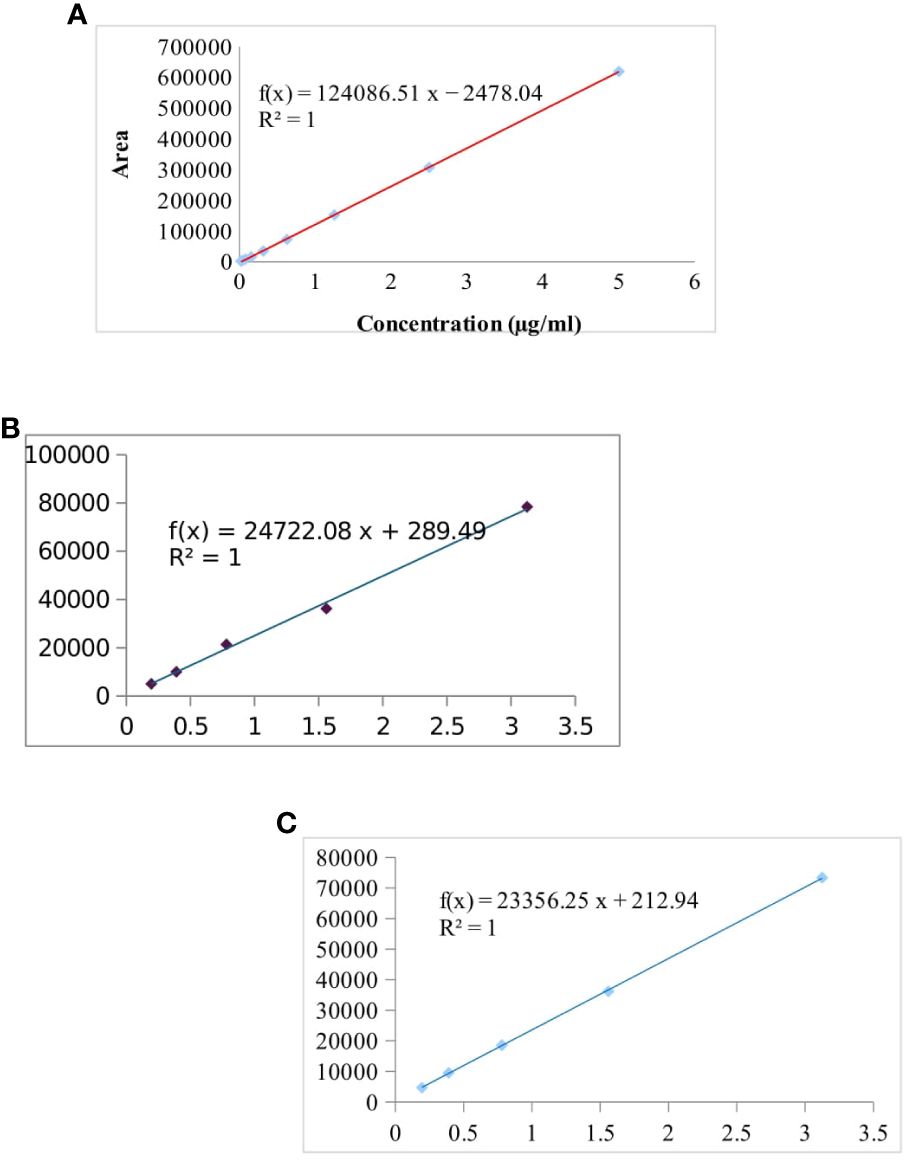
Figure 2 Calibration curve of the ciprofloxacin (CIP) standard (from 0.0195 to 5 µg/ml) (A), tetracycline (TC) standard (B), and oxytetracycline (OTC) standard (0.195 to 3.125 µg/ml for both TC and OTC) (C).
Table 2 shows the highly significant correlation coefficient (R2) values ranging from 0.9964 to 0.9999 from the linear regression for each antibiotic. The accuracy of the CIP-spiked samples was between 60.53% and 77.37%, while that for the TC- and OTC-spiked samples was 85.26%–99.87%. The RSD value of each spiked sample was less than 2, which showed good accuracy of the methods, in accordance with the acceptance criteria set out in the European Commission Decision 2002/657 (Communities, 2002). The LOD and LOQ were found to be 3.8–20.8 and 12.2–69.5 µg/kg, respectively, for the three selected antibiotics (Table 2).
The RSDs of the intra- and inter-day precision of the spiked chicken samples were 1.10%–4.57% and 1.18%–4.64% for CIP, 1%–4.09% and 1.31%–5.74% for TC, and 0.30%–1.28% and 1.29%–3.28% for OTC, respectively, as presented in Table 3. The recovery of CIP observed 62.50%–70.41% in intra-day and 64.58%–70.58% in inter-day precision (Table 3). For TC and OTC, the recoveries (92.52%–101.22%) from the skin were higher than those from the muscle and liver (79.27%–96.70%). These results on precision met the criteria set out in the European Commission Decision 2002/657 (Communities, 2002). The stability studies of the methods for the CIP-, TC-, and OTC-spiked samples are summarized in Table 4. At different times and temperatures, the mean recovery and the %RSD of CIP, TC, and OTC in the muscle were 65.27%–92.12% and 0.52%–2.83%, respectively. In the liver, the mean recovery was 60.92%–93.90%, whereas this was higher in the skin, which ranged 67.17%–106.2%; the RSD was below 2% in both samples of CIP, TC, and OTC.
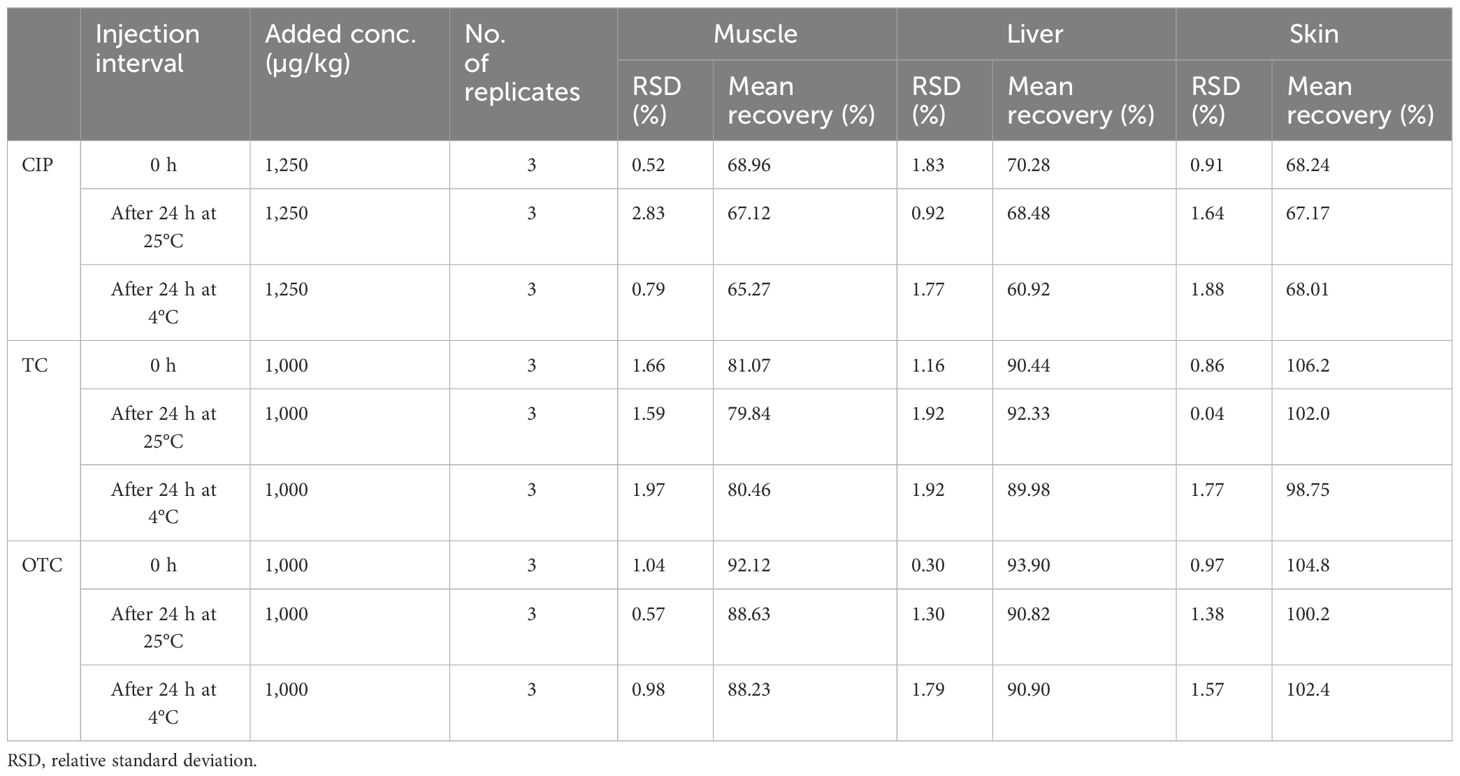
Table 4 Stability of the examined ciprofloxacin (CIP), tetracycline (TC), and oxytetracycline (OTC) in poultry muscle, liver, and skin after 24 h at room temperature and at refrigerated temperature.
CIP, TC, and OTC residues
A total of 504 samples of muscle, liver, and skin in three different stages from 56 broiler chickens (28 for CIP and 28 for TC and OTC) were examined using the validated HPLC method for the determination of CIP, TC, and OTC. Figure 1 shows the chromatograms of CIP, TC, and OTC of the standard and chicken samples. Figure 3 presents the frequency of CIP, TC, and OTC residues in chicken meat, liver, and skin samples at various stages. The analytical results of the CIP, TC, and OTC residues in the muscle, liver, and skin of chicken at three different stages are shown in Table 5 according to the permissible MRLs set by EU Council Regulation no. 2377/90. CIP residues were found in 22.79–341.8 µg/kg in the muscle, 22.83–2,087.296 µg/kg in the liver, and 15.33–484.2 µg/kg in the skin. The assessment of CIP in chicken samples showed that 96.43% of raw liver contained the highest CIP residues, followed by raw skin (85.71%) and muscle (32.14%). Among the positive CIP samples in the raw stage, 14.3% liver, 17.9% skin, and 3.6% muscle exceeded the MRLs. In the frozen stage, no significant change of the CIP residues was found in the muscle and the liver, except for the skin where the CIP residues were reduced moderately after boiling in the liver (7.1%) and the skin (3.6%). Although the CIP residue in muscle was decreased significantly after boiling, it remained above the MRL. Figure 4 shows the comparison between below and above MRL of the number of positive CIP, TC, and OTC residues.
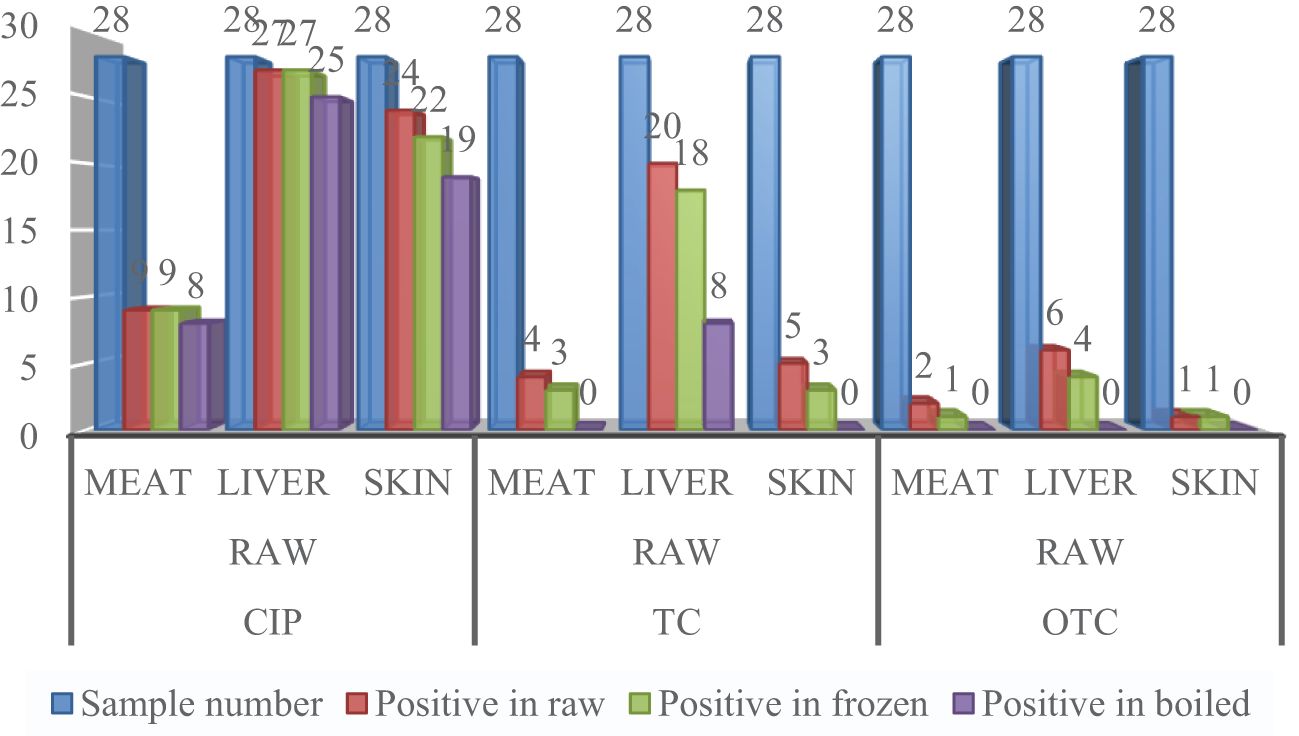
Figure 3 Frequency of ciprofloxacin (CIP), tetracycline (TC), and oxytetracycline (OTC) residues in a number of chicken meat, liver, and skin samples.
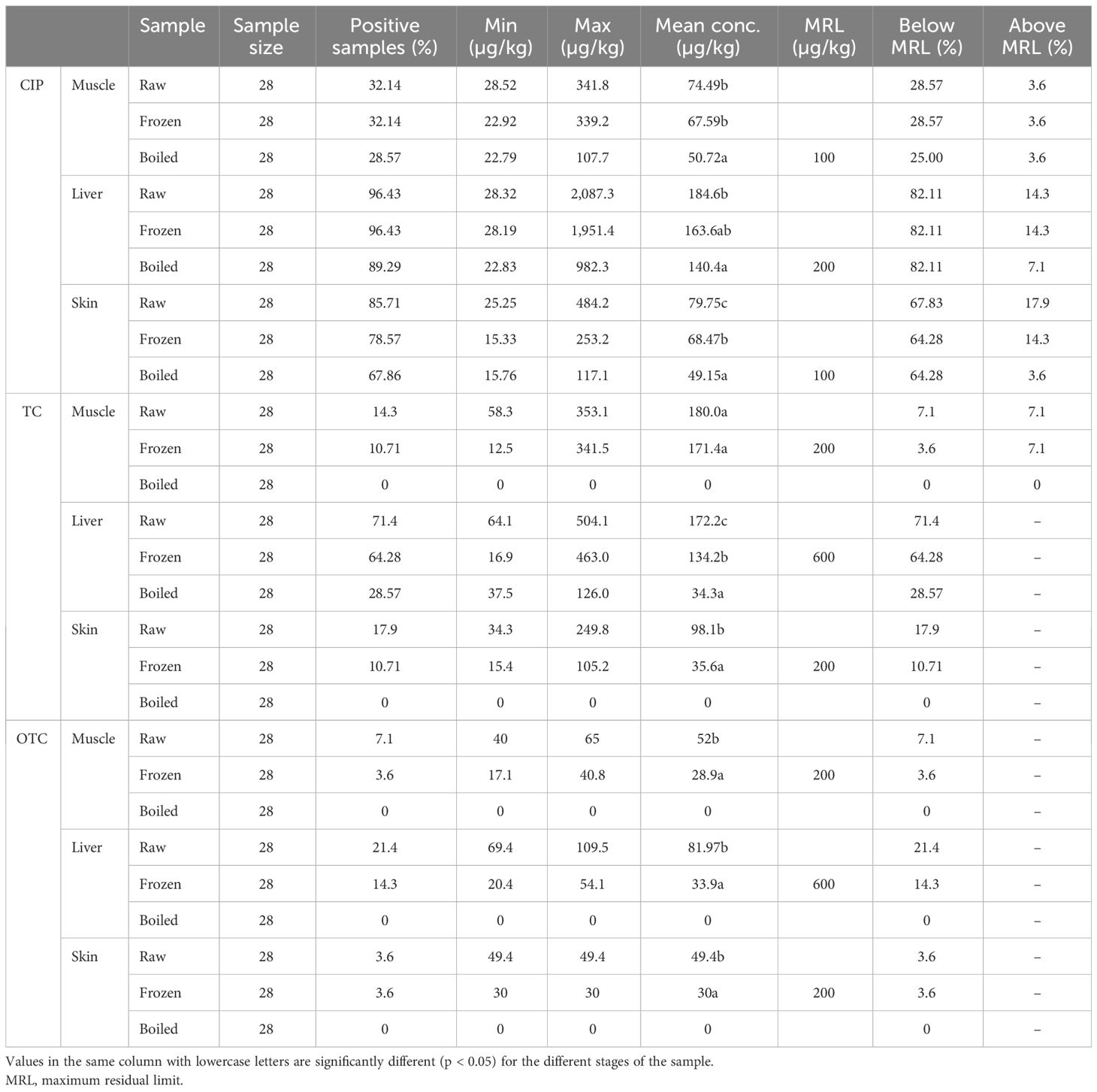
Table 5 Analytical results of the ciprofloxacin (CIP), tetracycline (TC), and oxytetracycline (OTC) residues in raw, frozen, and boiled chicken samples.
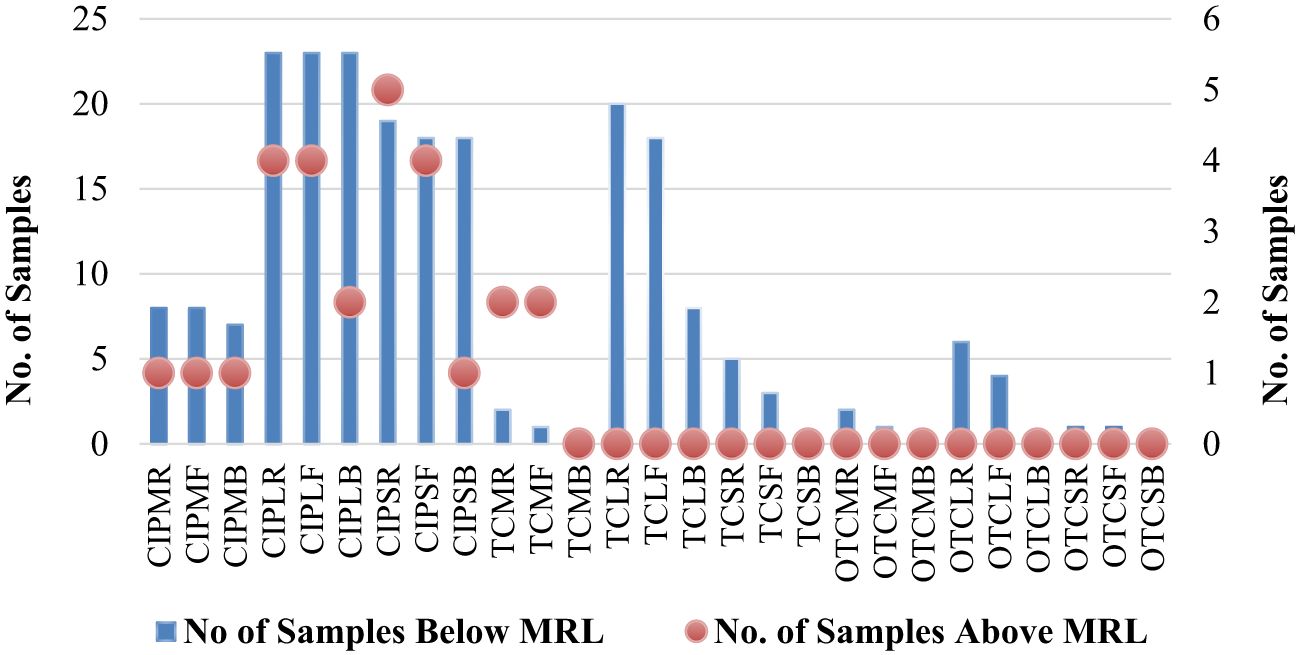
Figure 4 Comparison between below and above MRL of the number of positive antibiotic residues. CIPMR, ciprofloxacin-muscle-raw; CIPMF, ciprofloxacin-muscle-frozen; CIPMB, ciprofloxacin-muscle-boiled; CIPLR, ciprofloxacin-liver-raw; CIPLF, ciprofloxacin-liver-frozen; CIPLB, ciprofloxacin-liver-boiled; CIPSR, ciprofloxacin-skin-raw; CIPSF, ciprofloxacin-skin-frozen; CIPSB, ciprofloxacin-skin-boiled; TCMR, tetracycline-muscle-raw; TCMF, tetracycline-muscle-frozen; TCMB, tetracycline-muscle-boiled; TCLR, tetracycline-liver-raw; TCLF, tetracycline-liver-frozen; TCLB, tetracycline-liver-boiled; TCSR, tetracycline-skin-raw; TCSF, tetracycline-skin-frozen; TCSB, tetracycline-skin-boiled; OTCMR, oxytetracycline-muscle-raw; OTCMF, oxytetracycline-muscle-frozen; OTCMB, oxytetracycline-muscle-boiled; OTCLR, oxytetracycline-liver-raw; OTCLF, oxytetracycline-liver-frozen; OTCLB, oxytetracycline-liver-boiled; OTCSR, oxytetracycline-skin-raw; OTCSF, oxytetracycline-skin-frozen; OTCSB, oxytetracycline-skin-boiled.
Table 5 presents the range of TC residues in the muscle, liver, and skin, which were 12.5–371.5, 16.9–504.9, and 15.4–249.8 µg/kg, respectively. The highest percentage of TC was observed in the liver (71.4%), followed by the skin (17.9%) and muscle (14.3%), in the raw condition. Among these, 7.1% of raw muscle contained TC residues above the MRL. In the frozen stage, the TC residues were reduced significantly in the liver and skin, except for the muscle sample (7.1%) showing above the MRL. After boiling, TC was remarkably reduced in the liver sample, without any residues detected in muscle and skin samples. Assessment of the OTC residues found 17.1–65 µg/kg in the muscle, 20.4–109.5 µg/kg in the liver, and 30–49.4 µg/kg in the skin. The highest percentage of OTC residues was found in raw liver, with a range of positive samples from 3.6% to 21.4%. It was noted that the values of all the OTC-positive samples were below the MRL. A significant reduction was found in OTC residues in the muscle, liver, and skin samples in the frozen stage, but no OTC residues were present in the samples after boiling.
Health risk assessment
Health risk assessment of these three antibiotics was calculated from the ratio of the EDI of the CIP, TC, and OTC residues obtained from chicken (meat and liver) to ADI, which is, as well as the hazard index (HI), is presented in Table 6. The daily intake of chicken skin for a Bangladeshi individual and the ADI for antibiotics in the skin were unavailable. The HI investigated three antibiotic residues in broiler chicken at various stages, which was calculated by considering the maximum mean concentration of the CIP, TC, and OTC residues. Table 6 shows that the EDI range of CIP in chicken meat and liver was 0.0311–0.1044 µg/kg bw per day, while the corresponding HI values ranged from 0.0156 to 0.0522. For TC, the EDI range was 0.0063–0.1020 µg/kg bw per day, while the HI ranged from 0.0 to 0.0034. The EDI and HI values for OTC ranged 0.0–0.0188 µg/kg bw per day and 0.0–0.0006, respectively. The highest EDI of CIP was found in the raw liver chicken sample, whereas the EDI value was found to be 0.00 µg/kg bw per day in boiled muscle for TC and in both boiled muscle and liver for OTC. These findings indicated that the EDI value calculated based on a dietary consumption containing the maximum mean concentrations of CIP, TC, and OTC was considerably lower than the ADI values of the antibiotic residues. The present experimental results showed that all HI values were below 0.0522.
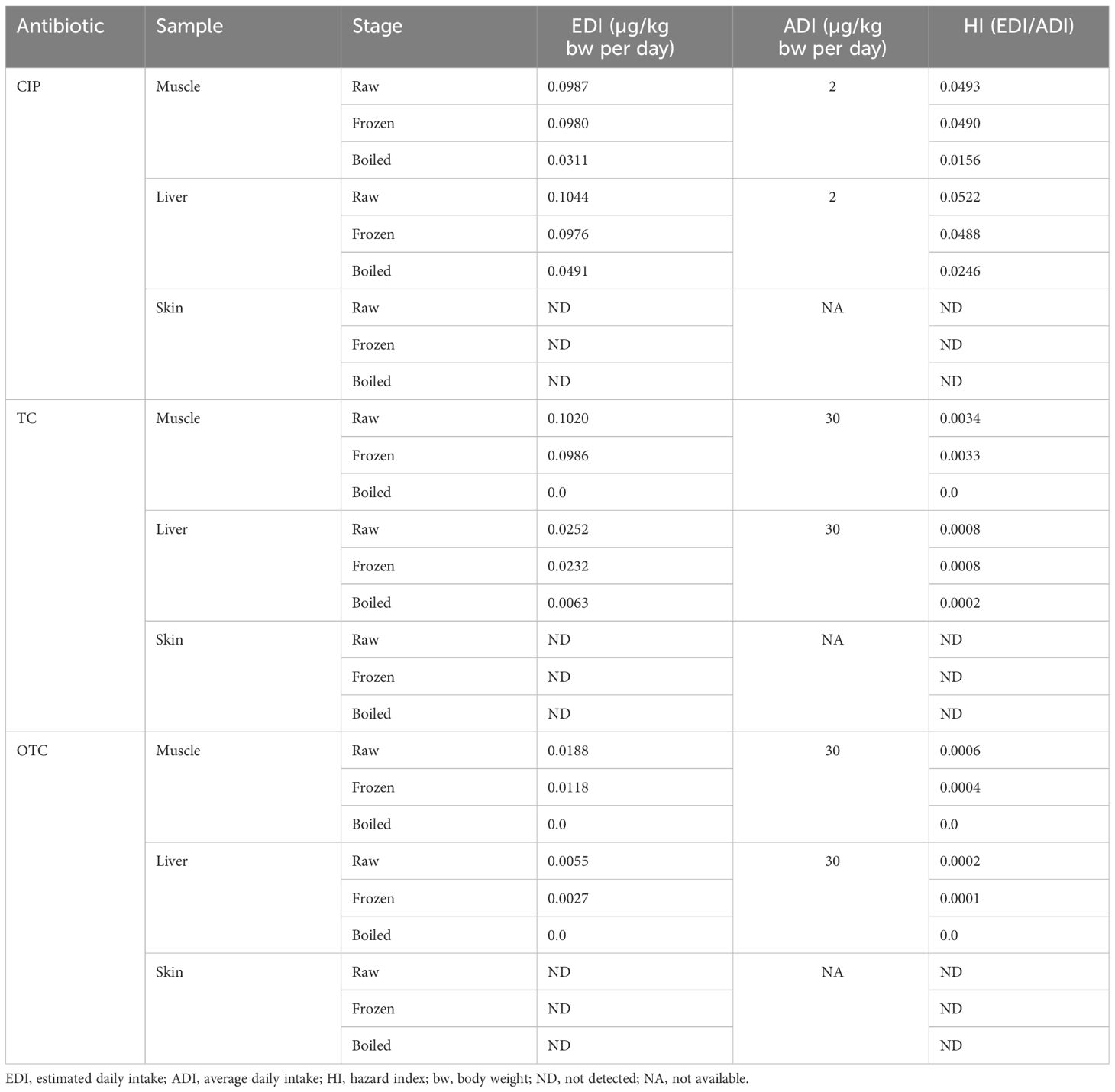
Table 6 Health risks associated with ciprofloxacin (CIP), tetracycline (TC), and oxytetracycline (OTC) residues in edible parts of chicken at three different stages.
Discussion
The present study used SPE in sample preparation, and this required conditioning in order to moisten the sorbent and permit the liquid to penetrate the pores before the sample is introduced (Lu et al., 2019). In our approach, the wavelength used for CIP was consistent with Yorke and Froc (2000). For TC and OTC, a silica-based C18 analytical column, UV detection at 355 nm, flow rate of 1.0 ml/min, SPE C18 cartridges, and a 0.22-μm syringe filter were used following De Ruyck et al. (1999), Miranda et al. (2009); Shalaby et al. (2011), and Zhao et al. (2010). Christodoulou et al., (2007) found 53.5%–83.7% CIP recovery in egg yolk using different extraction solvents. A higher recovery of CIP was obtained in chicken muscle and liver by changing the matrix, extraction solvent, and their ratio (Posyniak et al., 2001). The recovery of OTC reported by Olatoye and Kayode (2012) was 80.5%–87.8%, which was somewhat lower, while Guo et al. (2012) reported 80.9%–119.5% recovery for both TC and OTC, which was higher than our findings. Strong acids and precipitating agents are recommended for deproteinization to improve the extraction of antibiotics from complex matrices as TCs readily bind to proteins (Pérez-Rodríguez et al., 2018). Our recovery values for the edible parts of chicken were comparable to those obtained by Blasco et al. (2009); Yu et al. (2011), and Masawat and Slater (2007). The LOD and LOQ values for TC and OTC were comparable to those obtained by Cornejo et al. (2017), Ozdemir Olgun and Demirata (2020), and Shalaby et al. (2011), but were higher than that in the study by Guo et al. (2012). According to Schneider et al. (2005), the LOD and LOQ of CIP were 1 and 5 µg/kg, respectively, which were smaller than those in our study. The findings for the precision values had similarities to others. Shalaby (1996) revealed a higher recovery in chicken muscle (98.4%–100.3%) and egg yolk (99.3%–101.6%) and lower RSD values (1.9%–3.1%) in chicken muscle and egg yolk (0.4%–2.5%) for intra-day precision. Schneider et al. (2007) reported below 4% RSD and 75%–90% recovery from muscle tissue in his findings. Another study stated that the recovery of intra-day precision for CIP in milk sample was 85.4%–89.1% and that the RSD was below 6% (Christodoulou et al., 2007). Yorke and Froc (2000) disclosed a 67% recovery and an 8.4% RSD as inter-day precision in chicken muscle, whereas Cinquina et al. (2003b) obtained 4.1% RSD. Yu et al. (2011) reported within- and between-day precision values for chicken samples of 6.2%–8.2%, 5.9%–8.1%, and 8.1%–9.8%, and 8.7%–8.8% for both TC and OTC, respectively. Blasco et al. (2009) reported higher RSD values for intra-day (i.e., 14.3% and 11.2%) and inter-day precision (16.5% and 15.6%) for TC and OTC in chicken samples. Moudgil et al. (2019b) carried out a study on accuracy and precision for TC and OTC. He reported that the recovery of accuracy was in the range 90.2%–108.1% for TC and was 99.2%–105.6% for OTC. The RSDs of the intra- and inter-assay precision for TC were 4.7% and 6.7–9.2%, while those for OTC were 6.2% and 9.6–16.2%, respectively.
Numerous investigations have been conducted to identify the presence of antibiotic residues in animal products in Asia, such as in Vietnam and China (Baghani et al., 2019), and the prevalence of antibiotic residues in chicken meat has been reported (Vandenberge et al., 2012; Sarker et al., 2018; Gompo et al., 2020; Maharjan et al., 2020; Rahman et al., 2021). In this study, an alarming number of samples were found to be positive for CIP (quinolone group), with 14.3% liver, 17.9% skin, and 3.6% muscle being above the MRLs in the raw stage, hence a potential health risk for consumption. The presence of CIP residues in the samples indicated that farmers in poultry production used this antibiotic as a therapeutic agent, which might have been excessively used or misused (Widiastuti et al., 2022). In developing countries, antibiotics are available as livestock medication without a prescription, and imported illegal products sometimes lack information about their use in the local language (Yamaguchi et al., 2015). In another study, Pereira et al. (2018) also mentioned that the detection of high frequencies of fluoroquinolone (including CIP) residues indicates the random use of these antibiotics due to the lack of proper monitoring by an authorized body. In the study of Panzenhagen et al. (2016), enrofloxacin (fluoroquinolone group) was found positive by HPLC in the muscles (23%), livers (17%), and kidneys (17%) from chickens. The mean concentrations of CIP found by Hasanen et al. (2016) in chicken thigh, breast, liver, and kidney were 92.11, 131.7, 211.1, and 316 µg/kg, while those in turkey thigh, breast, liver, and kidney were 83.2, 119.2, 205.7, and 291.9 µg/kg, respectively, which were higher than our findings. The results showed that approximately 10.71% muscle, 12.66% liver, and 15.38% skin samples exceeded the MRL (EU) among the positive samples of CIP, which were markedly high. In Malaysia, Marni et al. (2011) found that 10 out of 37 samples were contained ciprofloxacin (3.42–238.11 ng/g) and enrofloxacin in 33 samples (3.51–1,734.61 ng/g). Moreover, 45.7% of samples in a study in Turkey (Kaynak Onurdă Er. et al., 2013) and 59.2% of chicken meat samples in another study in Iran (Mashak et al., 2017) were found to be quinolone-positive. In Pakistan, Aslam et al. (2016) found that 58.3% (21) of meat samples had enrofloxacin residues above the MRL (100 ng/g). The findings of chicken samples containing CIP residues above the MRL of 100 ng/g in developing countries revealed the indiscriminate use of antibiotics, which is different from the picture in developed countries such as Spain, the UK, Portugal (Pereira et al., 2018), and Korea (Lee et al., 2018), where no samples exceeded the MRL of antibiotic residues. To reiterate, the differences in the results between the present study and other studies may be due to the multiple methods of administration of antibiotics in chicken, such as with feed and drinking water, intramuscularly, or intravenously (Abdullah et al., 2012; Ramatla et al., 2017). Furthermore, the detection rate of antibiotics may depend on the length of their use in chickens, slaughter, and sampling (Yamaguchi et al., 2015). Little change was found in the concentration of CIP in the positive samples after freezing, whereas a significant difference was observed in the study of O Aboul El-Nile (2006), who stated that temperatures below −18°C can have a positive effect on the reduction of CIP residues. In this study, in the boiling stage, the mean concentration of CIP was reduced from 74.49 to 50.72 µg/kg in the muscle, from 184.6 to 140.4 µg/kg in the liver, and from 79.75 to 49.15 µg/kg in the skin. In contrast, Hasanen et al. (2016) found that the CIP concentrations in both chicken and turkey did not change after boiling, grilling, and roasting, whereas these were notably reduced after microwave treatment and freezing for 1 month at −20°C. In support of this, Botsoglou and Fletouris (2001) also found that CIP has a high heat stability and is not easily destroyed at cooking temperature. In this study, the TC-positive samples in the raw stage were from 7.1% to 71.4%, with 7.1% of these found to be above the MRL; the OTC-positive samples were 3.6%–21.4%, but no sample was above the MRL. Bahmani et al. (2020) reported that TC residues were present in 54.3–389.1 µg/kg of the chicken samples, which was comparable to our data (40–353.1 µg/kg). According to Miranda et al. (2009), the TC and OTC residues in poultry meat ranged from 197 to 2,564 µg/kg and from 651 to 1,003 µg/kg, respectively, which were higher than our findings. In a study in Nepal, TCs and other antibiotics exceeded the MRLs in 6 out of 66 samples (Raut et al., 2017). This could be explained by marketing representatives promoting the use of these medications with easy affordability to poultry farmers, who then administered them without the assistance of trained veterinary officers and with lack of compliance to the specified withdrawal periods (Odundo et al., 2023). TCs can induce the overproduction of Pseudomonas and Clostridium, which change the normal intestinal flora (Hassan et al., 2020) and cause diarrhea, nausea, and even death. TCs consumed during pregnancy could remain in the structure of newly formed teeth (Muhammad et al., 2019). In Southern Italy, low-frequency OTC levels were reported in the muscle (3%) and liver (7%), showing a similar trend to that of the present study (Cammilleri et al., 2019). In poultry farming, OTC is used for the prevention of mouth infections and pneumonia, which can vary with the change of season depending on humidity (Aalipour et al., 2013). In addition, weather and seasonal variations are important factors that change the rate of a drug’s metabolism in chicken (Cerveny et al., 2021). Al-Ghamdi et al. (2000) found that the TC and OTC levels in beef and liver were lowered after boiling, and according to the observation of Gratacós-Cubarsí et al. (2007), boiling and microwave cooking lower the initial quantities of TC and OTC residues by 56% to 82%, respectively. Similarly, Abou-Raya et al. (2013) observed that the residual concentrations of TC and OTC were reduced by 25.8%–28.0% and 45.35%–67.05%, respectively, after boiling. All these have similarities to our findings.
The percent EDI-to-ADI ratio in 60-kg adults is used to measure the human health risk, whereas ADI is a standard criterion for assessing the safety of chemical contaminants in edible animal tissues. The present data for health risk assessment showed that the HI values for CIP, TC, and OTC from all chicken samples in the three stages were below 1, indicating low-risk exposure for human health. Oyedeji et al. (2019) analyzed the antibiotic residues from imported turkey-chicken products in Nigeria and found that the concentrations of 19 antibiotic residues were within the permissible limits. The HI for CIP in all the samples analyzed was less than 1 (HI < 1), indicating no risk for an adult population in Indonesia (Widiastuti et al., 2022). Pena et al. (2010) in Portugal and Pereira et al. (2018) revealed that the EDIs for enrofloxacin in chickens were 0.029 and 0.46 ng/kg body weight per day, respectively. The estimated daily exposure dose was found below 1 µg/kg bw per day with the presence of TCs, quinolones (QNLs), and sulfonamide antibiotics (SAs) in poultry meat in Shanghai (Wang et al., 2017). From 2012 to 2017, Kyriakides et al. (2020) studied antibiotic exposure from the consumption of pork meat in children and adolescents and found a low HI (below 0.056). These trends were also observed in our results. However, Vragovi´c et al. (2011) obtained significantly higher EDIs for streptomycin than for TCs in meat samples. Antibiotic exposure, even in low doses, develops antibiotic-resistant microorganisms, which could have long-term consequences on human health (Karbiwnyk et al., 2007; Gomes and Kuyucu, 2017; Kyuchukova, 2020). Dietary exposure to antibiotic residues could cause therapeutic failure, particularly in children (Jahantigh and Dizaji, 2015). Individuals who inadvertently consume antibiotic residues through edible chicken parts could suffer from allergies, from mutagenic, teratogenic, and anaphylactic shocks, and digestive disorders (Mund et al., 2016; Jammoul and El Darra, 2019). Arsène et al. (2022) also reported adverse health effects such as reproductive disorders, mutagenicity, alteration of the gut flora, and carcinogenicity, which can be caused by exposure to antibiotic-containing food. Cross-resistance could be produced in a new group of microorganisms from the antibiotic-resistant group due to structural alterations in the enzyme from the mutated gene (Sköld, 2001; Baran et al., 2011; Dimitrios et al., 2018).
Conclusion
This study demonstrated two methods for the determination and validation of CIP, TC, and OTC. These methods were efficient in determining the CIP, TC, and OTC from different parts of poultry samples, which were confirmed by recovery studies. A great number of samples were found to be positive with antibiotics. The overall concentration of each antibiotic detected in broiler chicken was higher in raw samples than those in frozen and boiled samples. A few samples contained antibiotics in concentrations higher than their MRLs even after heat treatment, indicating a hazardous situation for human health. The results from the health risk assessment showed that low exposure to antibiotic residues may be a possible human health concern. In countries such as Bangladesh, where most of the poor and middle-class people are somehow dependent on broiler chicken as a cheaper source of protein and which has become a part of their consumption, it is important for authorities to pay attention to the use of veterinary drugs and to take necessary steps to avoid antibiotic resistance.
Data availability statement
The original contributions presented in the study are included in the article/supplementary material. Further inquiries can be directed to the corresponding author.
Ethics statement
The animal studies were approved by Ethical Review Committee (ERC), Mawlana Bhashani Science and Technology University, Tangail, Bangladesh. The studies were conducted in accordance with the local legislation and institutional requirements. Written informed consent was obtained from the owners for the participation of their animals in this study.
Author contributions
SH: Data curation, Formal analysis, Investigation, Methodology, Software, Writing – original draft, Writing – review & editing. MJ: Data curation, Formal analysis, Investigation, Methodology, Software, Writing – original draft, Writing – review & editing. MK: Formal analysis, Investigation, Methodology, Validation, Visualization, Writing – original draft, Writing – review & editing. MR: Data curation, Formal analysis, Investigation, Methodology, Resources, Software, Visualization, Writing – original draft, Writing – review & editing. ME: Data curation, Software, Writing – review & editing. MA: Data curation, Resources, Software, Writing – review & editing. MZ: Project administration, Validation, Visualization, Writing – original draft, Writing – review & editing. MS: Data curation, Resources, Software, Writing – review & editing. LB: Conceptualization, Funding acquisition, Methodology, Project administration, Resources, Supervision, Validation, Visualization, Writing – original draft, Writing – review & editing.
Funding
The author(s) declare financial support was received for the research, authorship, and/or publication of this article. This study was financially supported the Ministry of Science and Technology, Peoples’ Republic of Bangladesh through grant number 39.00.0000.009.06.009.20-1331-282 BS.
Acknowledgments
The authors are thankful to the laboratory assistant and chicken suppliers.
Conflict of interest
The authors declare that the research was conducted in the absence of any commercial or financial relationships that could be construed as a potential conflict of interest.
Publisher’s note
All claims expressed in this article are solely those of the authors and do not necessarily represent those of their affiliated organizations, or those of the publisher, the editors and the reviewers. Any product that may be evaluated in this article, or claim that may be made by its manufacturer, is not guaranteed or endorsed by the publisher.
Abbreviations
EDI, Estimated daily intake; ADI, Average daily intake; HI, Hazard index; LOD, Limit of detection; LOQ, Limit of quantification; MRL, Maximum residue limit; CIP, Ciprofloxacin; TC, Tetracycline; OTC, Oxytetracycline; bw, Body weight; SPE, Solid phase extraction.
References
Aalipour F., Mirlohi M., Jalali M. (2013). Prevalence of antibiotic residues in com- mercial milk and its variation by season and thermal processing methods. Int. J. Environ. Health Eng. 2, 41. doi: 10.4103/2277-9183.122429
Abdullah O. A., Shareef A. M., Sheet O. H. (2012). Detection of streptomycin residues in local meat of bovine and ovine. Iraqi J. Vet. Sci. 26, 43–46. doi: 10.33899/ijvs.2012.46958
Abou-Raya S. H., Shalaby A. R., Salama N. A., Emam W. H., Mehaya F. M. (2013). Effect of ordinary cooking procedures on tetracycline residues in chicken meat. J. Food Drug Anal. 21, 80–86. doi: 10.6227/jfda.2013210110
Ahmed S., Ahmed M. W., Hasan M. Z., Mehdi G. G., Islam Z., Rehnberg C., et al. (2022). Assessing the incidence of catastrophic health expenditure and impoverishment from out-of-pocket payments and their determinants in Bangladesh: evidence from the nationwide Household Income and Expenditure Survey 2016. Int. Health, 14 (1), 84–96.
Al-Ghamdi M. S., Al-Mustafa Z. H., El-Morsy F., Al-Faky A., Haider I., Essa H. (2000). Residues of tetracycline compounds in poultry products in the eastern province of Saudi Arabia. Public Health 114, 300–304. doi: 10.1016/S0033-3506(00)00350-4
Almalki A. H., Hussein E. A., Naguib I. A., Abdelaleem E. A., Zaazaa H. E., Abdallah F. F. (2021). Development and validation of ecofriendly HPLC-MS method for quantitative assay of amoxicillin, dicloxacillin, and their official impurity in pure and dosage forms. J. analytical Methods Chem. 2021, 5570938. doi: 10.1155/2021/5570938
Anderson A. D., Nelson J. M., Rossiter S., Angulo F. J. (2003). Public health consequences of use of antimicrobial agents in food animals in the United States. Microb Drug Resist. 9, 373–379.
Arsène M. M. J., Davares A. K. L., Viktorovna P. I., Andreevna S. L., Sarra S., Khelifi I., et al. (2022). The public health issue of antibiotic residues in food and feed: Causes, consequences, and potential solutions. Veterinary World 15, 662–671. doi: 10.14202/vetworld.2022.662-671
Aslam B., Kousar N., Javed I., Raza A., Ali A., Khaliq T., et al. (2016). Determination of enrofloxacin residues in commercial broilers using high-performance liquid chromatography. Int.J. Food Prop 19, 2463–2470. doi: 10.1080/10942912.2015.1027922
Baghani A., Mesdaghinia A., Rafieiyan M., Soltan Dallal M. M., Douraghi M. (2019). Tetracycline and ciprofloxacin multiresidues in beef and chicken meat samples using indirect competitive ELISA. J. immunoassay immunochemistry 40, 328–342. doi: 10.1080/15321819.2019.1597735
Bahmani K., Shahbazi Y., Nikousefat Z. (2020). Monitoring and risk assessment of tetracycline residues in foods of animal origin. Food Sci. Biotechnol. 29, 441–448. doi: 10.1007/s10068-019-00665-x
Baran W., Adamek E., Ziemiańska J., Sobczak A. (2011). Effects of the presence of sulfonamides in the environment and their influence on human health. J. hazardous materials 196, 1–15. doi: 10.1016/j.jhazmat.2011.08.082
Bitas D., Samanidou V., Kabir A., Locatelli M. (2018). Food sample preparation for the determination of sulfonamides by high-performance liquid chromatography: state-of-the-art. Separations. 5 (2), 31. doi: 10.3390/separations5020031
Blasco C., Di Corcia A., Pico Y. (2009). Determination of tetracyclines in multi-specie animal tissues by pressurized liquid extraction and liquid chromatography-tandem mass spectrometry. Food Chem. 116, 1005–1012. doi: 10.1016/j.foodchem.2009.03.055
Botsoglou N. A., Fletouris D. J. (2001). Drug residues in foods : pharmacology food safety and analysis. Marcel Dekker. January 2, 2024. Available at: http://www.dekker.com.
Cammilleri G., Pulvirenti A., Vella A., Macaluso A., Dico G. L., Giaccone V., et al. (2019). Tetracycline residues in bovine muscle and liver samples from sicily (Southern Italy) by LC-MS/MS method: A six-year study. Molecules 24, 695. doi: 10.3390/molecules24040695
Cerveny D., Fick J., Klaminder J., McCallum E. S., Bertram M. G., Castillo N. A., et al. (2021). Water temperature affects the biotransformation and accumulation of a psychoactive pharmaceutical and its metabolite in aquatic organisms. Environ. Int. 155, 106705. doi: 10.1016/j.envint.2021.106705
Christodoulou E. A., Samanidou V. F., Papadoyannis I. N. (2007). Validation of an HPLC-UV method according to the European Union Decision 2002/657/EC for the simultaneous determination of 10 quinolones in chicken muscle and egg yolk. J. chromatography. B Analytical Technol. Biomed. Life Sci. 859, 246–255. doi: 10.1016/j.jchromb.2007.10.009
Cinquina A. L., Longo F., Anastasi G., Giannetti L., Cozzani R. (2003a). Validation of a high-performance liquid chromatography method for the determination of oxytetracycline, tetracycline, chlortetracycline and doxycycline in bovine milk and muscle. J. chromatography. A 987, 227–233. doi: 10.1016/S0021-9673(02)01446-2
Cinquina A. L., Roberti P., Giannetti L., Longo F., Draisci R., Fagiolo A., et al. (2003b). Determination of enrofloxacin and its metabolite ciprofloxacin in goat milk by high-performance liquid chromatography with diode-array detection. Optimization and validation. J. chromatography. A 987, 221–226. doi: 10.1016/S0021-9673(02)01800-9
Cornejo J., Pokrant E., Araya D., Briceño C., Hidalgo H., Maddaleno A., et al. (2017). Residue depletion of oxytetracycline (OTC) and 4-epi-oxytetracycline (4-epi-OTC) in broiler chicken’s claws by liquid chromatography-tandem mass spectrometry (LC-MS/MS). Food additives contaminants. Part A Chemistry analysis control exposure Risk Assess. 34, 494–500. doi: 10.1080/19440049.2016.1263876
Croubels S., Daeseleire E. (2012). Veterinary drug residues in food. 148–182. doi: 10.1533/9780857095794.2.148
De Ruyck H., De Ridder H., Van Renterghem R., Van Wambeke F. (1999). Validation of HPLC method of analysis of tetracycline residues in eggs and broiler meat and its application to a feeding trial. Food additives contaminants 16, 47–56. doi: 10.1080/026520399284190
Dimitrios B., Abuzar K., Marcello L., Victoria S. (2018). Food Sample Preparation for the Determination of Sulfonamides by High-Performance Liquid Chromatography: State-of-the-Art. 31–31. doi: 10.3390/separations5020031
Communities, E (2002). Implementing Council Directive 96/23/EC concerning the performance of analytical methods and the interpretation of results. Off. J. Eur. Communities, 100, 3044.
Fan Y., Zeng G., Ma X. (2020). Multi-templates surface molecularly imprinted polymer for rapid separation and analysis of quinolones in water. Environ. SciPollut Res. 27, 7177–7187. doi: 10.1007/s11356-019-07437-4
Garcia I., Sarabia L., Ortiz C. M. (2004). Robustness of the extraction step when parallel factor analysis (PARAFAC) is used to quantify sulfonamides in kidney by high performance liquid chromatography-diode array detection (HPLC-DAD). Analyst. 129 (8), 766–771. doi: 10.1039/B404320K
Gomes E. R., Kuyucu S. (2017). Epidemiology and risk Factors in drug hypersensitivity reactions, Curr. Treat. Options Allergy 4, 239–257. doi: 10.1007/s40521-017-0128-2
Gompo T. R., Sapkota R., Subedi M., Koirala P., Bhatta D. D. (2020). Monitoring of antibiotic residues in chicken meat, Cow and Buffalo milk samples in Nepal, Int. J. Appl. Sci. Biotechnol. 8, 355–362. doi: 10.3126/ijasbt.v8i3.31314
Gratacós-Cubarsí M., Fernandez-García A., Picouet P., Valero-Pamplona A., García-Regueiro J. A., Castellari M. (2007). Formation of tetracycline degradation products in chicken and pig meat under different thermal processing conditions. J. Agric. Food Chem. 55, 4610–4616. doi: 10.1021/jf070115n
Guo L., Chen Y., Zhang L., Yang W., He P. (2012). Development and validation of a liquid chromatographic/tandem mass spectrometric method for determination of chlortetracycline, oxytetracycline, tetracycline, and doxycycline in animal feeds. J. ofAOAC Int. 95 (4), 1010–1015. doi: 10.5740/jaoacint.11-087
Hasanen F. S., Mohammed M. M., Hassan W. M., Amro F. H. (2016). Ciprofloxacin residues in chicken and Turkey carcasses. Benha Veterinary Med. J. 31, 136–143. doi: 10.21608/bvmj.2016.31282
Hassan M. M., Harrington N. E., Sweeney E., Harrison F. (2020). Predicting antibiotic-associated virulence of pseudomonas aeruginosa using an ex vivo lung biofilm model, Front. Microbiol 11. doi: 10.3389/FMICB.2020.568510/BIBTEX
Jahantigh M., Dizaji R. E. (2015). Antimicrobial drug resistance pattern of escherichia coli isolated from chickens farms with colibacillosis infection. Open J. Med. Microbiol. 05, 159–162. doi: 10.4236/ojmm.2015.54019
Jammoul A., El Darra N. (2019). Evaluation of antibiotics residues in chicken meat samples in Lebanon. Antibiotics 8 (2), 59. doi: 10.3390/antibiotics8020069
Karbiwnyk C. M., Carr L. E., Turnipseed S. B., Andersen W. C., Miller K. E. (2007). Determination of quinolone residues in shrimp using liquid chromatography with fluorescence detection and residue confirmation by mass spectrometry. Analytica chimica Acta 596, 257–263. doi: 10.1016/j.aca.2007.06.018
Kaynak Onurdă Er. B. F., Demirhan B., Özgen Özgacar S., Bayhan Öktem A., Abbasoğlu U. (2013). Screening of quinolone antibiotic residues in chicken meat and beef sold in the markets of Ankara, Turkey. Poult. Sci. 92, 2212–2215. doi: 10.3382/ps.2013-03072
Kyriakides D., Lazaris A. C., Arsenoglou K., Emmanouil M., Kyriakides O., Kavantzas N., et al. (2020). Dietary exposure assessment of veterinary antibiotics in pork meat on children and adolescents in Cyprus. Foods (Basel Switzerland) 9, 1479. doi: 10.3390/foods9101479
Kyuchukova R. (2020). Antibiotic residues and human health hazard-review. Bulg. J. Agric. Sci. 26, 664–668.
Lee H. J., Cho S. H., Shin D., Kang H. S. (2018). Prevalence of antibiotic residues and antibiotic resistance in isolates of chicken meat in Korea. Korean J. Food. Sci. Anim. Resour 38, 1055–1063. doi: 10.5851/kosfa.2018.e39
Lu Z., Deng F., He R., Tan L., Luo X., Pan X., et al. (2019). A pass-through solid-phase extraction clean-up method for the determination of 11 quinolone antibiotics in chicken meat and egg samples using ultra-performance liquid chromatography tandem mass spectrometry. Microchemical J. 151, 104213. doi: 10.1016/j.microc.2019.104213
Maharjan B., Neupane R., Dev Bhatta D. (2020). Antibiotic residue in marketed broiler meat of Kathmandu Metropolitan city, Arch. Vet. Sci. Med. 3 (1), 1–10. doi: 10.26502/AVSM.010
Marni S., Mustafa A. M., Marzura M. R. (2011). Analysis of quinolones in poultry muscles using liquid chromatography-tandem mass spectrometry. Malaysian J. Vet. Res. 2, 1–5.
Masawat P., Slater J. M. (2007). The determination of tetracycline residuesin food using a disposable screen-printed gold electrode (SPGE). Sensorsand Actuators B: Chem. 124, 127–132. doi: 10.1016/j.snb.2006.12.010
Mashak Z., Mojaddarlangroodi A., Mehdizadeh T., Fathabad A. E. (2017). Detection of quinolones residues in beef and chicken meat in hypermarkets of urmia, Iran using ELISA. Iran Agric. Res. 36, 73–77.
Miranda J. M., Rodríguez J. A., Galán-Vidal C. A. (2009). Simultaneous determination of tetracyclines in poultry muscle by capillary zone electrophoresis. J. chromatography. A 1216, 3366–3371. doi: 10.1016/j.chroma.2009.01.105
Moudgil P., Bedi J. S., Aulakh R. S., Gill J. P. S. (2019a). Analysis of antibiotic residues in raw and commercial milk in punjab India vis-à-vis human health risk assessment. J. Food Saf. 39 (4), e12643. doi: 10.1111/jfs.12643
Moudgil P., Bedi J. S., Aulakh R. S., Gill J. P. S., Kumar A. (2019b). Validation of hplc multi-residue method for determination of fluoroquinolones tetracycline sulphonamides and chloramphenicol residues in bovine milk. Food Analytical Methods 12, 338–346. doi: 10.1007/s12161-018-1365-0
Muhammad J., Khan S., Su J. Q., Hesham A. E. L., Ditta A., Nawab J., et al. (2019). Antibiotics in poultry manure and their associated health issues: a systematic review, J. Soils Sed 20, 486–497. doi: 10.1007/s11368-019-02360-0
Mund M. D., Khan U. H., Tahir U., Mustafa B. E., Fayyaz A. (2016). Antimicrobial drug residues in poultry products and implications on public health: a review, Int. J. Food Prop. 20, 1433–1446. doi: 10.1080/10942912.2016.1212874
O Aboul El-Nile M. (2006). Effect of boiling and freezing on ciprofloxacin residues in chicken tissues. Assiut Veterinary Med. J. 52, 111–120. doi: 10.21608/avmj.2006.177388
Odundo F., Ngigi A., Magu M. (2023). Sulfonamides and β-lactam antibiotic residues and human health risk assessment in commercial chicken meat sold in Nairobi City, Kenya. Heliyon 9, e18810. doi: 10.1016/j.heliyon.2023.e18810
Olatoye O., Kayode S. T. (2012). Oxytetracycline residues in retail chickenEggs in ibadan, Nigeria. Food Additives Contaminants: Part B 5, 255–259. doi: 10.1080/19393210.2012.702791
Oyedeji A. O., Msagati T. A. M., Williams A. B., Benson. N. U. (2019). Determination of antibiotic residues in frozen poultry by a solid-phase dispersion method using liquid chromatography-triple quadrupole mass spectrometry. Toxicol. Rep. 6, 951–956. doi: 10.1016/j.toxrep.2019.09.005
Ozdemir Olgun F. A., Demirata B. (2020). Evaluation of tetracycline, oxytetracycline and penicillin G residues in chicken meat samples by high performance liquid chromatography with pda detection. Cumhuriyet Sci. J. 41, 665–670. doi: 10.17776/csj.723599
Page S. W., Gautier P. (2012). Use of antimicrobial agents in livestock. Rev. Sci. Tech. 31, 145–188. doi: 10.20506/rst.issue.31.1.49
Panzenhagen P. H. N., Aguiar W. S., Gouvêa R., Oliveira A. M. G. D., Barreto F., Pereira V. L. A., et al. (2016). Investigation of enrofloxacin residues in broiler tissues using ELISA and LC-MS/MS. Food Addit. Contam. Part A 33, 1–5. doi: 10.1080/19440049.2016.1143566
Pena A., Silva L. J. G., Pereira A., Meisel L., Lino C. M. (2010). Determination of fluoroquinolone residues in poultry muscle in Portugal. Anal. Bioanal. Chem. 397, 2615–2621. doi: 10.1007/s00216-010-3819-0
Pereira A., Silva M. P. T., Rodrigues J., Lino C., Pena A. (2018). Risk assessment of fluoroquinolones from poultry muscle consumption: Comparing healthy adult and pre-school populations. Food Chem. Toxicol. 118, 340–347. doi: 10.1016/j.fct.2018.05.035
Pérez-Rodríguez M., Pellerano R. G., Pezza L., Pezza H. R. (2018). An overview of the main foodstuff samplepreparation technologies for tetracycline residue determination. Talanta 182, 1–21. doi: 10.1016/j.talanta.2018.01.058
Posyniak A., Zmudzki J., Semeniuk S. (2001). Effects of the matrix and sample preparation on the determination of fluoroquinolone residues in animal tissues. J. Chromatogr. A 914, 89–94. doi: 10.1016/S0021-9673(00)01088-8
Rahman A., Naznin M., Bari1 L. (2021). Simultaneous estimation of multiple antibiotic residues from chicken samples using High Performance Liquid Chromatography (HPLC). J. Agric. Food Econ 2, 20–26. doi: 10.47440/JAFE.2021.2104
Ramatla T., Ngoma L., Adetunji M., Mwanza M. (2017). Evaluation of antibiotic residues in raw meat using different analytical methods. Antibiotics 6, 34. doi: 10.3390/antibiotics6040034
Raut R., Mandal R. K., Kaphle K., Pant D., Nepali S., Shrestha A. (2017). Assessment of antibiotic residues in the marketed meat of Kailali and Kavre of Nepal, Int. J. Appl. Sci. Biotechnol. 5, 386–389. doi: 10.3126/ijasbt.v5i3.18302
Samanidou V., Evaggelopoulou E. N. (2015). HPLC analysis of penicillins in veterinary drugs. Samanidou Pharm. Anal. Acta 6, 4. doi: 10.4172/2153-2435.1000e174
Samanidou V. F., Evaggelopoulou E. N., Papadoyannis I. N. (2006). Chromatographic analysis of penicillins in pharmaceutical formulations and biological fluids. J. Separation Sci. 29, 1879–1908. doi: 10.1002/jssc.200600137
Samanidou V. F., Nisyriou S. A., Papadoyannis I. N. (2007). Residue analysis of penicillins in food products of animal origin by HPLC: A review. J. Liquid Chromatogr. Related Technol. 30 (9-10), 1145–1204. doi: 10.1080/10826070701274320
Sarker Y. A., Hasan M. M., Paul T. K., Rashid S. Z., Alam M. N., Sikder M. H. (2018). Screening of antibiotic residues in chicken meat in Bangladesh by thin layer chromatography, J. Adv. Vet. Anim. Res. 5 (2), 140–145. doi: 10.5455/javar.2018.e257
Schneider M. J., Vazquez-Moreno L., Bermudez-Almada M. D. C., Guardado R. B., Ortega-Nieblas M. (2005). Multiresidue determination of fluoroquinolones in shrimp by liquid chromatography-fluorescence-mass spectrometry. J. AOAC int. 88 (4), 1160–1166. doi: 10.1093/jaoac/88.4.1160
Schneider M. J., Braden S. E., Reyes-Herrera I., Donoghue D. J. (2007). Simultaneous determination of fluoroquinolones and tetracyclines in chicken muscle using HPLC with fluorescence detection. J Chromatography B 84 6 (1–2), 8–13.
Shalaby A. R., Salama N. A., Abou-Raya S. H., Emam W. H., Mehaya F. M. (2011). Validation of HPLC method for determination of tetracycline residues in chicken meat and liver. Food Chem. 124 (4), 1660–1666. doi: 10.1016/j.foodchem.2010.07.048
Shalaby A. R. (1996). TLC in food analysis in: (Eds), Sherma J., Fried B. Practical thin layer chromatography a multidisciplinary Approach. Boca Raton: CRC press. pp. 169–192.
Sköld O. (2001). Resistance to trimethoprim and sulfonamides. Veterinary Res. 32, 261–273. doi: 10.1051/vetres:2001123
Vandenberge V., Delezie E., Huyghebaert G., Delahaut P., Daeseleire E., Croubels S. (2012). Residues of sulfadiazine and doxycycline in broiler liver and muscle tissue due to cross-contamination of feed. Food additives contaminants. Part A Chemistry analysis control exposure Risk Assess. 29, 180–188. doi: 10.1080/19440049.2011.631194
Verdon E., Couedor P., Roudaut B., Sandérs P. (2005). Multiresidue method for simultaneous determination of ten quinolone antibacterial residues in multimatrix/multispecies animal tissues by liquid chromatography with fluorescence detection: single laboratory validation study. J. AOAC int. 88 (4), 1179–1192. doi: 10.1093/jaoac/88.4.1179
Vragovi´c N., Bazuli´c D., Njari B. (2011). Risk assessment of streptomycin and tetracycline residues in meat and milk on Croatian market. Food Chem. Toxicol. 49, 352–355. doi: 10.1016/j.fct.2010.11.006
Wang H., Ren L., Yu X., Hu J., Chen Y., He G., et al. (2017). Antibiotic residues in meat, milk and aquatic products in Shanghai and human exposure assessment. Food Control 80, 217–225. doi: 10.1016/j.foodcont.2017.04.034
Widiastuti R., Martindah E., Anastasia Y. (2022). Detection and dietary exposure assessment of fluoroquinolones residues in chicken meat from the districts of Malang and Blitar, Indonesia. Trop. Anim. Sci. J. 45, 98–103. doi: 10.5398/tasj
Yamaguchi T., Okihashi M., Harada K., Konishi Y., Uchida K., Bui M. H. N., et al. (2015). Antibiotic residue monitoring results for pork, chicken, and beef samples in Vietnam in 2012-2013. J. Agric. Food Chem. 63, 5141–5145. doi: 10.1021/jf505254y
Yorke J. C., Froc P. (2000). Quantitation of nine quinolones in chicken tissues by high-performance liquid chromatography with fluorescence detection. J. Chromatogr. A 882, 63–77. doi: 10.1016/S0021-9673(00)00165-5
Yu H., Tao Y., Chen D., Wang Y., Yuan Z. (2011). Development of an HPLC–UV method for the simultaneousDetermination of tetracyclines in muscle and liver of porcine, chicken andBovine with accelerated solvent extraction. Food Chem. 124 (3), 1131–1138. doi: 10.1016/j.foodchem.2010.07.024
Zeinali T., Salmani F., Naseri K. (2019). Dietary intake of cadmium, chromium, copper, nickel, and lead through the consumption of meat, liver, and kidney and assessment of human health risk in Birjand, Southeast of Iran. Biol. Trace Element Res. 191, 338–347. doi: 10.1007/s12011-019-1637-6
Keywords: health risk, broiler chicken, ciprofloxacin, tetracycline, oxytetracycline, HPLC, hazard index
Citation: Haque S, Jamil MY, Khan MSH, Reza MSA, Esrafil M, Abedin MZ, Zubair MA, Sikder MA and Bari L (2024) Health risk assessment of ciprofloxacin, tetracycline, and oxytetracycline residues in raw, frozen, and boiled broiler chicken available in a local area of Bangladesh. Front. Antibiot. 3:1364946. doi: 10.3389/frabi.2024.1364946
Received: 03 January 2024; Accepted: 29 February 2024;
Published: 03 April 2024.
Edited by:
Jianping Xie, Southwest University, ChinaReviewed by:
Mohd Nasir Mohd Desa, Putra Malaysia University, MalaysiaSandip Patil, Shenzhen Children’s Hospital, China
Copyright © 2024 Haque, Jamil, Khan, Reza, Esrafil, Abedin, Zubair, Sikder and Bari. This is an open-access article distributed under the terms of the Creative Commons Attribution License (CC BY). The use, distribution or reproduction in other forums is permitted, provided the original author(s) and the copyright owner(s) are credited and that the original publication in this journal is cited, in accordance with accepted academic practice. No use, distribution or reproduction is permitted which does not comply with these terms.
*Correspondence: Luthfunnesa Bari, luthfunnesa.ftns@gmail.com