- 1Riphah Institute of Pharmaceutical Sciences, Riphah International University, Lahore, Punjab, Pakistan
- 2Department of Pharmaceutical Sciences, Faculty of Pharmacy, Superior University, Lahore, Punjab, Pakistan
- 3Department of Pharmacy Practice, Faculty of Pharmacy, The Islamia University of Bahawalpur, Bahawalpur, Punjab, Pakistan
- 4Department of Community Medicine, Quaid-i-Azam Medical College, Bahawalpur, Bahawalpur, Punjab, Pakistan
- 5Department of Pharmacy Practice, Faculty of Pharmacy, Bahauddin Zakariya University, Multan, Punjab, Pakistan
Background: Antimicrobial resistance (AMR) has provoked a global health issue. Antimicrobial stewardship programs should be implemented to overcome this issue. The aim of this study was to determine the sensitivity patterns of the WHO Access, Watch, Reserve (AWaRe) group of antibiotics that assists in the selection of appropriate empiric antibiotic therapies.
Method: A descriptive, cross-sectional study was conducted for 6 months, in which 422 culture sensitivity sample reports from the Ghurki Trust Teaching Hospital’s laboratory were obtained through a convenience sampling technique, and the sensitivity patterns of nine offending bacteria to the WHO AWaRe group antibiotics were determined. Descriptive statistics and differences in frequency distribution among the categorical variables were obtained using the Statistical Package for Social Sciences (SPSS) software, version 21.
Results: Among 422 culture sensitivity sample reports, Escherichia coli (16.1%) was the most common Gram-negative pathogen. Acinetobacter, E. coli, Klebsiella, and Pseudomonas showed 100% sensitivity to polymyxin-b and colistin. Proteus showed the highest sensitivity to meropenem (90%), Staphylococcus aureus showed a 98% sensitivity to linezolid, Staphylococcus epidermidis was 100% sensitive to vancomycin and linezolid, and Streptococcus showed the highest sensitivity to penicillin (100%) and vancomycin (94.7%). Polymyxin b and colistin were found to be the most effective antibiotics against Gram-negative bacteria (100%). Gram-positive bacteria were highly sensitive to linezolid (99.4%), vancomycin (98.2%), chloramphenicol (89.5%), and tigecycline (82.6%).
Conclusion: Culture sensitivity reports help to rationalize the empirical use of antibiotics in clinical practice in addressing the challenge of antimicrobial resistance. This study showed that polymyxin-b and colistin were the most effective antibiotics against Gram-negative isolates and that Gram-positive bacteria were highly susceptible to linezolid. Updated antibiograms should be used by clinicians to evaluate bacterial susceptibility patterns and rationalize antibiotic empiric therapy.
Introduction
Antimicrobial resistance (AMR) poses a global threat due to resistant infections, the rate of which, by 2050, is expected to exceed 10 million per year (Klinker et al., 2021). It is a major global challenge due to its associated high rates of morbidity and mortality. Gram-negative bacteria, including Pseudomonas aeruginosa, Acinetobacter baumannii, and Extended-spectrum beta-lactamase (ESBL)- and carbapenemase-producing organisms are the most common antibiotic-resistant bacteria (CDC, 2019). The Infectious Diseases Society of America has identified six organisms that cause infections that are the most challenging to address. These are known as ESKAPE organisms (namely Enterococcus faecium, S. aureus, Klebsiella pneumoniae, Acinetobacter baumannii, Pseudomonas aeruginosa, and Enterobacter species) (Mulani et al., 2019). Conventional antimicrobials are unable to treat Gram-positive and Gram-negative bacteria with multidrug-resistant patterns, resulting in untreatable infections. In many healthcare settings, early detection of causative microorganisms and their antimicrobial susceptibility patterns in patients with bacterial infection is lacking. As a result, broad-spectrum antibiotics are redundantly used widely (Akova, 2016).
AMR has emerged as a major public health concern in the 21st century and poses a significant threat to the effective treatment and prevention of an ever-growing number of diseases caused by bacteria that are resistant to commonly used antibiotics (Dixit et al., 2019). To overcome the challenge of increasing bacterial resistance, the current shortage of effective drugs, and the lack of successful prevention measures, the development of novel and alternative antimicrobial therapies is required (Mühlen and Dersch, 2015). Developing strategies against rising rates of antibiotic resistance is a major global challenge for public health (Chellat et al., 2016). The WHO has categorized antibiotics into the Access, Watch, Reserve (AWaRe) group, including first-/second-line antibiotics into the empiric therapy, restricted use, and last resort categories. The implementation of antimicrobial stewardship programs (ASPs) that promote effective empiric antibiotic therapies will help to reduce bacterial resistance. A useful tool that aids in the selection of appropriate empiric antibiotic therapies is an antibiogram.
A hospital antibiogram is a periodic summary of antimicrobial susceptibilities of the local bacterial isolates that are submitted to the hospital’s microbiology laboratory. It helps clinicians to identify local bacterial susceptibility rates, which assist in their selection of empiric antibiotic therapies, and to determine resistance patterns over time within an institution (Joshi, 2010). The aim of this study was to determine the sensitivity patterns of different bacterial isolates against WHO AWaRe group antibiotics at a tertiary care hospital, so as to aid clinicians in the selection of the appropriate antibiotic therapy.
Method
Study design
A descriptive, cross-sectional study was conducted for 6 months, from January 2021 to June 2021, for which we collected the available culture reports of blood and wound isolates from the Ghurki Trust Teaching Hospital laboratory to observe the sensitivity patterns of bacterial isolates against WHO AWaRe group antibiotics.
Study center
The present study was conducted at Ghurki Trust Teaching Hospital, which is a charitable organization. It is a 600-bed hospital that was established under the Societies Act XXI of 1860, with the reference number RP/4476/L/91/1018. Ghurki Trust Teaching Hospital is an ISO 9001:2015-accredited facility that is affiliated with Lahore Medical & Dental College, which is recognized by the Pakistan Medical & Dental Council (PMDC) and affiliated with the University of Health Sciences (UHS).
Sample size
Convenience sampling was carried out for the collection of culture reports from the microbiology laboratory of Ghurki Trust Teaching Hospital. A total of 422 culture sensitivity reports of all the patients admitted to hospital with any bacterial infection involving nine offending bacteria, namely Acinetobacter, Citrobacter, E. coli, Klebsiella, Pseudomonas, Proteus, S. aureus, S. epidermidis, and Strep species, against AWaRe group antibiotics were included in the study.
Access group antibiotics included amikacin, ampicillin, chloramphenicol, co-amoxiclav, co-trimoxazole, clindamycin, gentamycin, penicillin, and tetracycline. Watch group antibiotics included cefixime, cefoperazone/salbactum, ceftazidine, ceftriaxone, cefuroxime, ciprofloxacin, doripenem, ertapenem, erythromycin, fusidic acid, imipenem, meropenem, metacycine, minocycline, norfloxacin, levofloxacin, piperacillin/tazobactum, rifampicin, teicoplanin, tobramycin, and vancomycin. Reserve group antibiotics included cefipime, colistin, linezolid, polymyxin b, and tigecycline.
Data collection
Culture sensitivity tests were performed by trained and experienced microbiologists following standard operating procedures (SOPs) in the microbiology laboratory of Ghurki Trust Teaching Hospital to determine the sensitivity pattern of bacteria. Antibiotic susceptibility testing was performed on Mueller–Hinton agar using the Kirby–Bauer disk diffusion method in accordance with the Clinical Laboratory Standard Institute (CLSI) guidelines. The lowest concentration of an antibiotic that will inhibit the growth of a given microorganism (MIC) was used to determine bacterial resistance. Culture sensitivity reports were collected from the hospital laboratory for Global Antimicrobial Resistance Surveillance System (GLASS) priority pathogens. Patient demographics including age, gender, culture specimen (either blood or wound swab samples), and sensitivity and resistance to antibiotics were noted. If several cultures were collected during patient care, the duplicate isolated bacteria from the same patient were excluded, and only the first isolate was reported for each patient per surveyed specimen type and tested pathogen.
Data analysis
Data were analyzed using Statistical Package for Social Sciences (SPSS) software, version 21.0. Descriptive statistics and differences in frequency distribution among the categorical variables were obtained via crosstabulation (Pearson’s chi-square). The general sensitivity patterns of different bacteria against antibiotics were also presented as frequencies and percentages.
Results
Among the 422 culture sensitivity reports, 11 (3%) were for children, 49 (12%) were for adults, 362 were for (86%) elderly patients, 321 (76%) were for male patients, and 101 (24%) were for female patients. Of the 422 samples used for the culture sensitivity test, wound swab samples were taken from 414 (98%) patients, and blood samples were taken from 8 (2%) patients. Out of the 422 culture reports, Acinetobacter was isolated in 36 (8.5%) culture reports, E. coli in 68 (16.1%), Klebsiella in 20 (4.7%), Proteus in 30 (7.1%), Pseudomonas in 41 (9.7%), S. aureus in 177 (41.9%), Citrobacter in 20 (4.7%), S. epidermidis in 11 (2.6%), and Strep in 19 (4.5%), as shown in Table 1.
Microbes like Acinetobacter, Citrobacter, and S. aureus did not show any remarkable difference in prevalence among male and female patients. However, it is evident that E. coli was more commonly the cause of infection in female patients (19%) than in male patients (15%). Similarly, Pseudomonas was a pathogen that was more commonly isolated in male patients (11%) than in female patients (5%). Strep was responsible for 7% of infections in female patients and for 4% of infections in male patients (Figure 1).
Acinetobacter was the most prevalent bacteria in children patients (18%) and the least prevalent in elderly patients (8%), whereas Citrobacter was more predominant in adult patients (12%) than in children (9%) and elderly patients (4%). E. coli was equally common in adults and elderly group (16 %) whereas 18 % in child group. Klebsiella was most prevalent in child patients (9%) and least common in elderly patients (5%). Proteus was the most common cause of infection in elderly patients (47%); S. aureus was the most common cause of infection in adult patients (47%) and the least common cause of infection in child patients (36%). S. epidermis was responsible for infection in 3% of elderly patients. Strep was responsible for causing infection in 6% of adult patients and 4% of elderly patients (Figure 2).
Antibiotics susceptibility pattern of Gram-negative and Gram-positive pathogens of GLASSAMR
Among the nine offending bacteria, Acinetobacter showed 100% sensitivity to polymyxin-b and colistin (in 36 out of 36 cases). Citrobacter was found to be sensitive in 19 out of 19 cases (100%) to polymyxin b and colistin and in 18 out of 20 cases (90%) to tigecycline but was not sensitive (0 out of 18 cases, 0%) to ampicillin. E. coli was found to be most sensitive to polymyxin b (in 67 out of 67 cases, 100%) and colistin (in 66 out of 66 cases, 100%), but showed no sensitivity (0 out of 67 cases, 0%) to ampicillin (Table 2).
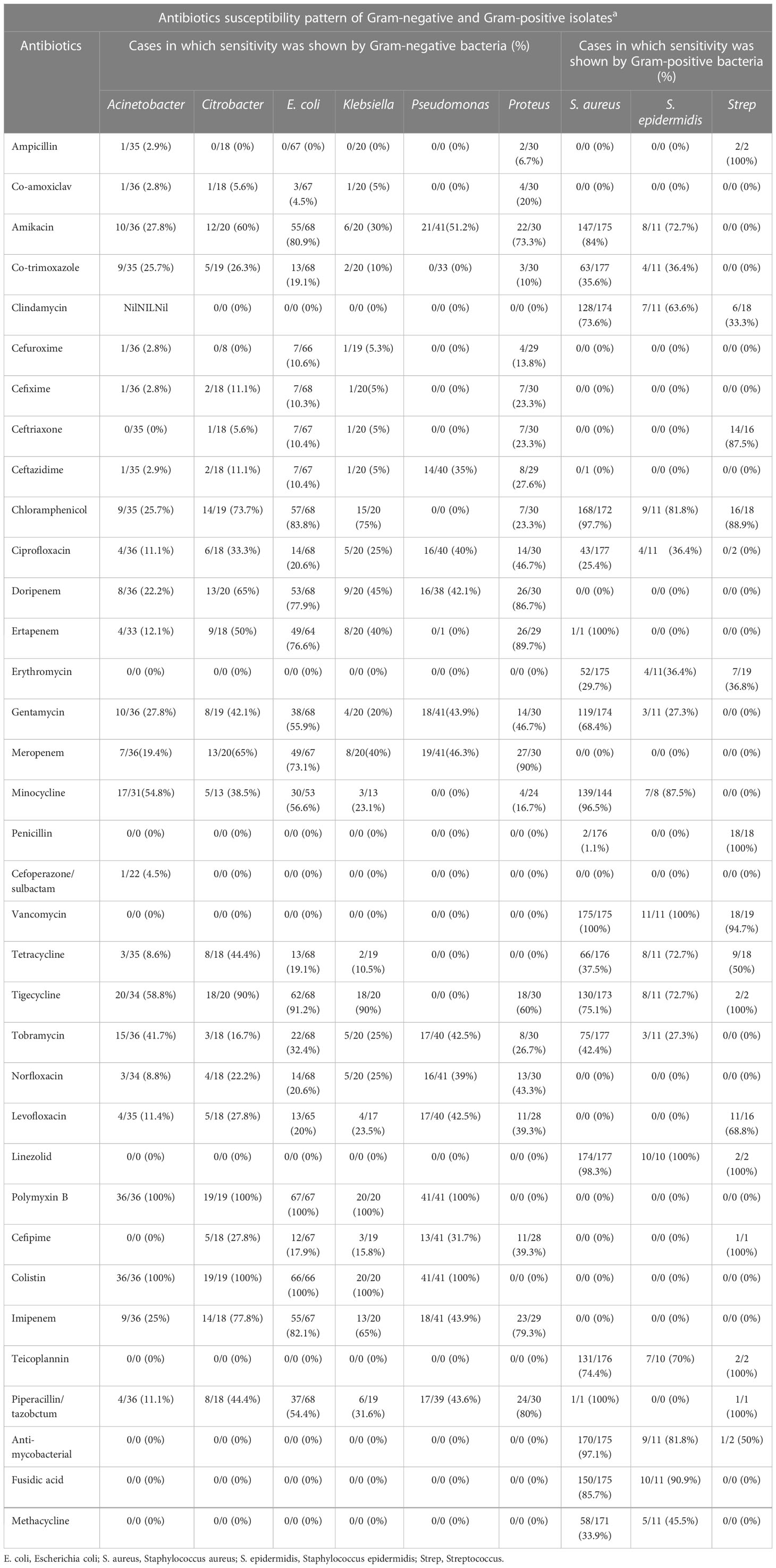
Table 2 Antibiotic susceptibility patterns of Gram-negative and Gram-positive pathogens of GLASS AMR.
Klebsiella showed sensitivity to polymyxin b and colistin in 20/20 cases (100%) and was sensitive to tigecycline in 18 out of 20 cases (90%) but showed no sensitivity to ampicillin (0 out of 20 cases, 0%). Pseudomonas showed sensitivity to polymyxin b and colistin in 41 out of 41 cases (100%) and no sensitivity (0 out of 33 cases, 0%) to co-trimoxazole. Proteus showed the most sensitivity (27 out of 30 cases, 90%) to meropenem, and was least sensitive to co-trimoxazole (3 out of 30 cases, 10%) and ampicillin (2 out of 30 cases, 6.7%) (Table 2).
S. aureus showed sensitivity to linezolid (174 out of 177 cases, 98%), chloramphenicol (168 out of 172 cases, 97.7%), rifampicin (170 out of 175 cases, 97.1%), minocycline (139 out of 144 cases, 96.5%), but showed less sensitivity to penicillin and ceftazidime (in 2 out of 176 cases and 0 out of 1 case, 1.1% and 0%, respectively). S. epidermidis was 100% sensitive to vancomycin (11 out of 11 cases) and linezolid (10 out of 10 cases), but was less sensitive to gentamycin and tobramycin (3 out of 11 cases, 27.3%). Strep was more sensitive to penicillin (18 out of 18 cases, 100%) and vancomycin (18 out of 19 cases, 94.7%) but showed no sensitivity to ciprofloxacin (0 out of 1 case, 0%) (Table 2).
Gram-negative and Gram-positive isolate sensitivity among different classes of antimicrobial agents
Among aminoglycosides, amikacin, gentamicin, and tobramycin were effective against both Gram-positive and Gram-negative bacteria. Rifampicin was effective against Gram-positive bacteria only (96%). Piperacillin/tazobactam was more effective against Gram-positive pathogens (100%) but less so (46%) against Gram-negative bacteria. Among carbapenems, ertapenem was completely effective (100%) against Gram-positive bacteria, and all drugs in the carbapenem class showed almost equal effectiveness against Gram-negative isolates, that is, meropenem (67%) followed by imipenem (63%), ertapenem (62%), and doripenem (59%). Cephalosporins showed more effectiveness against Gram-negative than Gram-positive organisms, with ceftriaxone being the most effective against both Gram-negative (94%) and Gram-positive (88%) bacteria, whereas cefepime was more effective against Gram-positive bacteria (100%) than Gram-negative bacteria (21%). All other antibiotics in this class were only effective against Gram-negative bacteria, that is, cefuroxime (78%), ceftazidime (16%), cefixime (10%), and cefoperazone/sulbactam (5%) (Table 3).
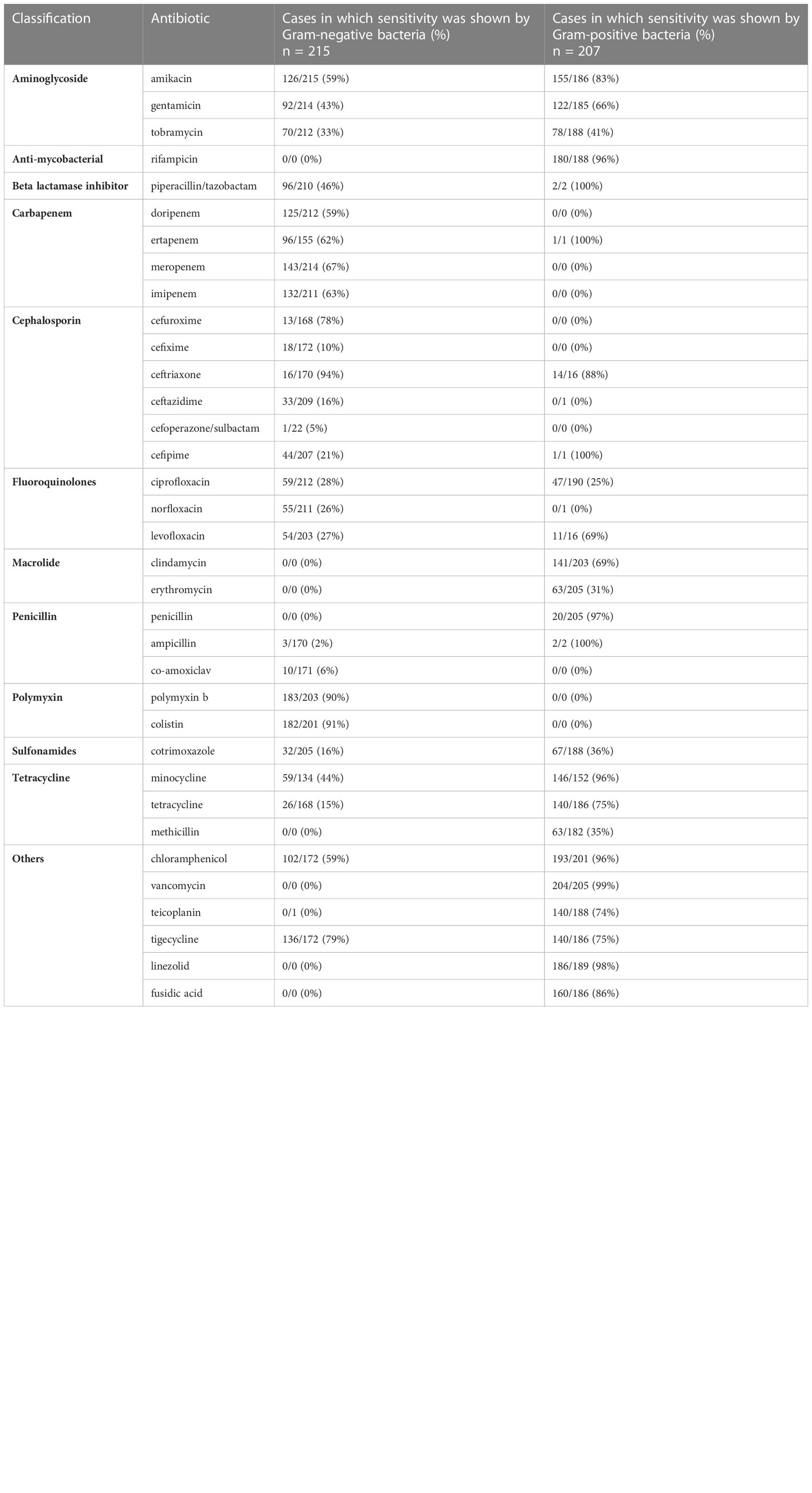
Table 3 Frequency distribution of the sensitivity of Gram-negative and Gram-positive isolates to different classes of antimicrobial agents.
Among fluoroquinolones, ciprofloxacin was more effective against Gram-negative (28%) than Gram-positive (25%) bacteria, levofloxacin was more effective against Gram-positive (69%) than Gram-negative (27%) bacteria, and norfloxacin was effective against Gram-negative (26%) bacteria only. Macrolides were effective against Gram-positive bacteria only, with clindamycin being the most effective (69%), followed by erythromycin (31%). Among the penicillin class, ampicillin was most effective against Gram-positive (100%) than Gram-negative (2%) bacteria, penicillin was effective against Gram-positive (97%) bacteria only, and co-amoxiclave was found to be effective against Gram-negative bacteria only (6%).
Polymyxins were effective against Gram-negative bacteria only, and colistin showed more effectiveness (91%) than polymyxin b (90%). Among sulfonamides, co-trimoxazole was shown to be more effective against Gram-positive (36%) than against Gram-negative (16%) bacteria. Tetracyclines, that is, minocycline, tetracyline, and methacycline, showed more effectiveness against Gram-positive bacteria (96%, 75%, and 35% effectiveness, respectively) than against Gram-negative bacteria minocycline (44%, 15%, and 0%, respectively). Among other antibiotics, chloramphenicol was more effective against Gram-positive (96%) than against Gram-negative (59%) bacteria, and tigecycline was more effective against Gram-negative (79%) than Gram-positive (75%) bacteria. All other antibiotics in this class were effective against Gram-positive bacteria, that is vancomycin (99%), followed by linezolid (98%), fusidic acid (86%), and teicoplanin (74%) (Table 3).
Discussion
Antibiotic resistance is a worldwide problem and its incidence is increasing globally. Resistance patterns to antibiotics vary due to emerging infectious disorders and over-the-counter sales and non-prescription consumption of antibiotics (Saeidynia et al., 2014). Knowing the trends in sensitivity and resistance patterns can help physicians and policymakers to make appropriate decisions to overcome the challenge of antibiotic resistance (Gopalakrishnan and Sureshkumar, 2010). In hospitals and clinical settings, multidrug-resistant infections lead to prolonged hospitalization, increased rates of morbidity/mortality, and overall healthcare sector costs (Revelas, 2012). Antibiotic susceptibility testing of microorganisms (via antibiograms) should be carried out at least once a year and serve as a basis for updating hospital empiric antibiotic policies (Akualing and Sri Rejeki, 2018).
Culture sensitivity reports help to identify specific antibiotics for particular pathogens, thus resulting in low physiologic and economic loss for the patient. Antibiograms rationalize the use of antibiotics in a clinical setting and serve as the main tool to cope with this ever-increasing problem of antimicrobial resistance. It is important to consider many factors before selecting an antibiotic for the patient (Leekha et al., 2011). To promote the rational use of antibiotics, the WHO has classified antibiotics into three groups (AWaRe) for the effective implementation of antimicrobial stewardship (McGettigan et al., 2017).
In this study, access group antibiotics (ampicillin, co-amoxiclave, cephalosporins, minocycline, tetracycline, and macrolides) showed comparable sensitivities to both Gram-positive and Gram-negative bacteria. Antibiotics from the watch group (meropenem and imipenem, gentamicin, piperacillin/tazobactum, third-generation cephalosporins, vancomycin, and quinolones) showed better responses to different Gram-negative bacteria. The reserve group antibiotics (cefipime, tigecycline, teicoplanin, and piperacillin/tazobactum) were found to be most effective among all the studied antibiotics, and polymyxins, linezolid, tigecycline, and cefipime were shown to be far more sensitive to Gram-negative bacteria than Gram-positive bacteria.
According to this study, among Gram-negative bacteria, Acinetobacter was highly sensitive to polymyxin-b (100%) and colistin (100%), followed by tigecycline (58.8%), minocycline (54.8%), tobramycin (41.7%), amikacin (27.8%), gentamycin (27.8%), chloramphenicol (25.7%), cotrimoxazole (25.7%), and imipenem (25%), However, in another study, Acinetobacter showed sensitivity to cefepime (70%), amikacin (66%), piperacillin/tazobactum (66%), meropenem (66%), gentamycin (50%), and cotrimoxazole (22%) (Mushtaq et al., 2013). The decrease in sensitivity of Acinetobacter toward amikacin and gentamycin in particular is due to the irrational and inappropriate use of antibiotics, which results in reduced clinical efficacy. Citrobacter showed high sensitivity to polymyxin-b (100%), colistin (100%), tigecycline (90%), and imipenem (77.8%), followed by chloramphenicol (73.7%), doripenem (65%), meropenem (65%), and amikacin (60%), and was least sensitive to minocycline (38.5%). However, as shown in a previous study, Citrobacter was highly sensitive to imipenem (100%), amikacin (85.2%), and gentamycin (77.4%), followed by cefoperazone/sulbactam (67.1%), nitrofurantoin (66.1%), cefepime (60.4%), ciprofloxacin (56.2%), levofloxacin (54.7%), ceftriaxone (50.9%), tobramycin (50%), cefoperazone/sulbactam (48.1), cefixime (45.8%), and cefotaxime (43.3%) (Sami et al., 2017).
E. coli was most sensitive to polymyxin-b (100%), colistin (100%), tigecycline (91.2%), chloramphenicol (83.8%), amikacin (80.9%), doripenem (77.9%), ertapenem (76.6%), meropenem (73.1%), minocycline (56.6%), gentamicin (55.9%), piperacillin/tazobactum (54.4%), and tobramycin (32.4%), compared with previously reported data that showed it was most sensitive to polymyxin-b (100%), followed by nitrofurantoin (95.5%), amikacin (94%), ampicillin (49.3%), nalidixic acid (44.7%), co-trimoxazole (35.8%), gentamicin (28.4%), cefotaxime (22.4%), and ciprofloxacin (19.4%) (Murmu et al., 2018). The Klebsiella species showed high sensitivity toward polymyxin-b (100%), colistin (100%), tigecycline (90%), chloramphenicol (75%), imipenem (65%), doripenem (45%), ertapenem (40%), meropenem (40%) and piperacillin/tazobactum (31.6%), whereas, in a previous study, it was most sensitive to amikacin (66%), ciprofloxacin (68%), gentamicin (62%), cefepime (60%), imipenem (56.66%), and aztreonam (52.63%) (Shilpa et al., 2016).
In the current study, Pseudomonas was found to more sensitive to polymyxin (100%), followed by amikacin (51.2%), meropenem (46.3%), gentamicin (43.9%), imipenem (43.9%), tobramycin and levofloxacin (42.5%), doripenem (42.1%), ciprofloxacin (40%), norfloxacin (39%), and cefepime (31.7%), but another study showed that it was most sensitive to amoxicillin/cloxacillin (72.7%), followed by amikacin (50%), ampicillin (25%), gentamicin (25%), and imipenem (25%) (Shrestha et al., 2012). Another study reported that Pseudomonas has the highest sensitivity to ciprofloxacin (68%) and amikacin (66%), followed by gentamicin (62%), cefepime (60%), imipenem (56.66%), and aztreonam (52.63%) (Sharifian et al., 2006).
The Proteus species was found to be highly sensitive to meropenem (90%), ertapenem (89.7%), doripenem (86.7%), imipenem (79.3%), amikacin (73.3%), tigecycline (60%), ciprofloxacin (46.7%), gentamicin (46.7%), norfloxacin (43.3%), levofloxacin (39.3%), and cefepime (39.3%). However, in a previous study, Proteus was found to be more sensitive to amikacin (90%), followed by amikacin (61.1%), cefoxitin (48.2%), aztreonam (47.7%), piperacillin/tazobactum (44.1%), ceftazidime (37.8%), gentamicin (36.8%), co-amoxiclav (32.2%), and ciprofloxacin (32.2%) (Bahashwan and El Shafey, 2013).
Among Gram-positive bacteria, S. aureus showed the most sensitivity to ertapenem (100%), piperacillin/tazobactum (100%), vancomycin (100%), linezolid (98.3%), chloramphenicol (97.7%), rifampicin (97.1%), minocycline (96.5%), and, to a lesser extent, to gentamycin (68.4%). Another study showed that it had the highest sensitivity to co-amoxiclav (83%), oxfloxacin (75.9%), nitrofurantoin (63.5%), and amoxicillin (58.9%), and the least to gentamycin (50.2%) (Akortha and Ibadin, 2008). The sensitivity pattern of Streptococcus species was observed to be highest against teicoplanin, piperacillin/tazobactum, cefepime, linezolid, tigecycline, penicillin, and ampicillin (100%), followed by vancomycin (94.7%), chloramphenicol (88.9%), ceftriaxone (87.5%), and levofloxacin (68.8%). Similar results are shown in a previous study, which found that the Strep species showed 100% sensitivity toward azithromycin, ceftriaxone, cefotaxime, cefuroxime, cephalexin, ciprofloxacin, clindamycin, cloxacillin, erythromycin, levofloxacin, imipenem, meropenem, linezolid, piperacillin/tazobactum, teicoplanin, and vancomycin (Trojan et al., 2016).
Gram-positive bacteria showed the most sensitivity to vancomycin and linezolid (94%–100), followed by teicoplanin, chloramphenicol, rifampicin, and fusidic acid (70%–90%). However, polymyxins (polymyxin-b and colistin), imipenem, norfloxacin, meropenem doripenem, cefixime, and cefuroxime showed no sensitivity against Gram-positive bacteria. All Gram-negative bacterial strains showed maximum sensitivity (100%) toward polymyxins (polymyxin-b and colistin) due to the recent use of these antibiotics in the hospital in which this study was carried out. The second most effective antibiotic was tigecycline (80%). Vancomycin, clindamycin, and cefoperazone/sulbactam showed no sensitivity to Gram-negative bacteria. In general, cefuroxime, cefixime, ceftriaxone, ceftazidime, ampicillin, and co-amoxiclav were the least effective antibiotics.
The overuse of antibiotics, issuing of prescriptions without sufficient sensitivity testing, and overdosing have led to bacteria having reduced sensitivities toward antibiotics that they previously had maximum sensitivities to. Multidrug resistance represents an increasing challenge to successful disease management (Nkang, 2009). Because antimicrobial resistance patterns are constantly changing and multidrug-resistant (MDR) organisms develop progressive antimicrobial resistance, it is critical to keep antimicrobial susceptibility profiles up to date so that safe and effective empiric therapy can be provided (Hirsch and Tam, 2010).
Conclusion
Culture sensitivity reports help to improve the rational, empiric use of antibiotics in clinical settings, which play a pivotal role in handling antimicrobial resistance. The findings of this study showed that polymyxin-b and colistin were the most effective antibiotics against Gram-negative isolates, whereas Gram-positive bacteria were highly susceptible to linezolid. Updated antibiograms should be used by clinicians to evaluate susceptibility patterns and rationalize antibiotic empiric therapy, which will help to reduce antibiotic resistance.
Data availability statement
The original contributions presented in the study are included in the article/supplementary material. Further inquiries can be directed to the corresponding author.
Ethics statement
The studies involving human participants were reviewed and approved by the ethical committee of Ghurki Trust and Teaching Hospital (study reference no. #3713/HR/GTTH). Written informed consent for participation was not required for this study in accordance with national legislation and institutional requirements.
Author contributions
ZA and ZS contributed to the concept and design of the research; NK contributed to the acquisition of data; JA and SS contributed to the analysis and interpretation of the data; ZA and NM drafted the manuscript. All authors critically revised the manuscript, agree to be fully accountable for ensuring the integrity and accuracy of the work, and read and approved the final manuscript. All authors contributed to the article and approved the submitted version.
Acknowledgments
The authors would like to thank the Riphah Institute of Pharmaceutical Sciences and Ghurki Trust and Teaching Hospital for their support.
Conflict of interest
The author ZS declare that they were an editorial board member of Frontiers at the time of submission. This had no impact on the peer review process and the final decision.
The remaining authors declare that the research was conducted in the absence of any commercial or financial relationships that could be construed as a potential conflict of interest.
Publisher’s note
All claims expressed in this article are solely those of the authors and do not necessarily represent those of their affiliated organizations, or those of the publisher, the editors and the reviewers. Any product that may be evaluated in this article, or claim that may be made by its manufacturer, is not guaranteed or endorsed by the publisher.
Abbreviations
E. coli, Escherichia coli; S. aureus, Staphylococcus aureus; Strep; Streptococcus; AWaRe, Access, watch and reserve group antibiotic; WHO, World Health Organization; GLASS, Global antimicrobial resistance and use surveillance system.
References
Akortha E. E., Ibadin O. K. (2008). Incidence and antibiotic susceptibility pattern of staphylococcus aureus amongst patients with urinary tract infection (UTI) in UBTH Benin city, Nigeria. Afr. J. Biotechnol. 7 (11), 1637–1640. doi: 10.5897/AJB08.176
Akova M. (2016). Epidemiology of antimicrobial resistance in bloodstream infections. Virulence 7 (3), 252–266. doi: 10.1080/21505594.2016.1159366
Akualing J. S., Sri Rejeki IGAAP. (2018). ANTIBIOGRAM (Antibiogram). Indonesian J. Of Clin. Pathol. And Med. Lab. 23 (1), 90–95. doi: 10.24293/ijcpml.v23i1.1191
Bahashwan S. A., El Shafey H. M. (2013). Antimicrobial resistance patterns of Proteus isolates from clinical specimens. Eur. Sci. J. 9 (27), 188–202. doi: 10.4103/ijcm.IJCM_217_18
Chellat M. F., Raguž L., Riedl R. (2016). Targeting antibiotic resistance. Angewandte Chemie Int. Edition 55 (23), 6600–6626. doi: 10.1002/anie.201506818
CDC. (2019). Antibiotic resistance threats in the United States. Atlanta, GA:U.S. Department of Health and Human Services, CDC; 2019. The full 2019 AR Threats Report. Available at: https://www.cdc.gov/DrugResistance/Biggest-Threats.html.
Dixit A., Kumar N., Kumar S., Trigun V. (2019). Antimicrobial resistance: progress in the decade since emergence of new Delhi metallo-β-lactamase in India. Indian J. Community medicine: Off. Publ. Indian Assoc. Prev. Soc. Med. 44 (1), 4.
Gopalakrishnan R., Sureshkumar D. (2010). Changing trends in antimicrobial susceptibility and hospital acquired infections over an 8 year period in a tertiary care hospital in relation to introduction of an infection control programme. J. Assoc. Physicians India 58 Suppl, 25–31.
Hirsch E. B., Tam V. H. (2010). Detection and treatment options for klebsiella pneumoniae carbapenemases (KPCs): an emerging cause of multidrug-resistant infection. J. antimicrobial chemotherapy 65 (6), 1119–1125. doi: 10.1093/jac/dkq108
Joshi S. (2010). Hospital antibiogram: a necessity. Indian J. Med. Microbiol. 28 (4), 277–280. doi: 10.4103/0255-0857.71802
Klinker K. P., Hidayat L. K., DeRyke C. A., DePestel D. D., Motyl M., Bauer K. A. (2021). Antimicrobial stewardship and antibiograms: importance of moving beyond traditional antibiograms. Ther. Adv. Infect. Dis. 8, 20499361211011373. doi: 10.1177/20499361211011373
Leekha S., Terrell C. L., Edson R. S. (2011). General principles of antimicrobial therapy. Mayo clinic proceedings; 2011 February (Elsevier), 156–167.
McGettigan P., Roderick P., Kadam A., Pollock A. M. (2017). Access, watch, and reserve antibiotics in India: challenges for WHO stewardship. Lancet Global Health 5 (11), e1075–e1076. doi: 10.1016/S2214-109X(17)30365-0
Mühlen S., Dersch P. (2015). “Anti-virulence Strategies to Target Bacterial Infections.” in How to overcome the antibiotic crisis. Eds. Stadler M., Dersch P.. Current Topics in Microbiology and Immunology, vol 398. (Cham: Springer). doi: 10.1007/82_2015_490
Mulani M. S., Kamble E. E., Kumkar S. N., Tawre M. S., Pardesi K. R. (2019). Emerging strategies to combat ESKAPE pathogens in the era of antimicrobial resistance: a review. Front. Microbiol. 10, 539. doi: 10.3389/fmicb.2019.00539
Murmu M., Mahesh K P K., Meher R. K., Pradhan B., Kar A., Naik M. (2018). Spectrum of uropathogens and their antibiotic sensitivity pattern in diabetes mellitus patients at a tertiary care hospital in odisha, India. Int. J. Res. Med. Sci. 6 (5), 1549. doi: 10.18203/2320-6012.ijrms20181432
Mushtaq S., Javeid I., Hassan M. (2013). Antibiotic sensitivity pattern of acinetobacter species isolated from clinical specimens in a tertiary care hospital. Biomedica 29 (1), 23–26.
Nkang A. O. (2009). Assessment of antibiotics susceptibility profiles of some selected clinical isolates from laboratories in Nigeria. J. Microbiol. Antimicrobials 1 (2), 019–026.
Revelas A. (2012). Healthcare–associated infections: a public health problem. Nigerian Med. journal: J. Nigeria Med. Assoc. 53 (2), 59. doi: 10.4103/0300-1652.103543
Saeidynia F., Keihanian F., Saeidynia A. (2014). Antibiotic resistance in blood culture samples from patients referred to razi laboratory of rasht, 2006-2011. Adv. Infect. Dis. 2014. doi: 10.4236/aid.2014.43023
Sami H., Sultan A., Rizvi M., Khan F., Ahmad S., Shukla I. (2017). Citrobacter as a uropathogen, its prevalence and antibiotics susceptibility pattern. CHRISMED J. Health Res. 4 (1), 23–26. doi: 10.4103/2348-3334.196037
Sharifian M., Karimi A., Tabatabaei S. R., Anvaripour N. (2006). Microbial sensitivity pattern in urinary tract infections in children: a single center experience of 1,177 urine cultures. Japanese J. Infect. Dis. 59 (6), 380.
Shilpa K., Thomas R., Ramyashree A. (2016). Isolation and antimicrobial sensitivity pattern of klebsiella pneumoniae from sputum samples in a tertiary care hospital. Int. J. BioMed. Adv. Res. 7 (2), 53–57. doi: 10.7439/ijbar
Shrestha R., Shrestha J. M., Gurung B. (2012). Antibiotic usage and its sensitivity pattern in the NICU. Kathmandu Univ. Med. J. 10 (2), 27–32. doi: 10.3126/kumj.v10i2.7339
Keywords: antibiotic, resistance, antibiogram, empiric therapy, sensitivity analysis
Citation: Khalid N, Akbar Z, Mustafa N, Akbar J, Saeed S and Saleem Z (2023) Trends in antimicrobial susceptibility patterns of bacterial isolates in Lahore, Pakistan. Front. Antibiot. 2:1149408. doi: 10.3389/frabi.2023.1149408
Received: 21 January 2023; Accepted: 30 May 2023;
Published: 20 June 2023.
Edited by:
Santi M. Mandal, Indian Institute of Technology Kharagpur, IndiaReviewed by:
Hasan Ejaz, Al Jouf University, Saudi ArabiaMrinal Bhattacharjee, Long Island University-Brooklyn, United States
Copyright © 2023 Khalid, Akbar, Mustafa, Akbar, Saeed and Saleem. This is an open-access article distributed under the terms of the Creative Commons Attribution License (CC BY). The use, distribution or reproduction in other forums is permitted, provided the original author(s) and the copyright owner(s) are credited and that the original publication in this journal is cited, in accordance with accepted academic practice. No use, distribution or reproduction is permitted which does not comply with these terms.
*Correspondence: Zunaira Akbar, enVuYWlyYS5ha2JhckBob3RtYWlsLmNvbQ==