- 1College of Agriculture, Yanbian University, Yanji, Jilin, China
- 2Animal Disease Prevention and Control Center, Yanbian Korean Autonomous Prefecture, Yanji, Jilin, China
Introduction: Animal hair growth is an important research topic in the field of breeding. Hair growth is affected by circulating exosomes in the plasma, and sheep wool fiber thickness is altered by intravenous injection of miRNA mimics.
Methods: This study investigated the effect of miRNAs in circulating exosomes on an animal’s hair growth. Circulating exosomes were isolated from Xinji fine-wool and small-tailed Han sheep plasma using a two-phase system. A blank control group (NC), groups of model mice injected with exosomes from Xinji fine-wool sheep (XPE), and groups of model mice injected with exosomes from small-tailed Han sheep group (SPE) were set up as three groups of hair regeneration models.
Results: The results showed that the diameter of hair follicles in the XPE group was significantly smaller than that of the NC and SPE groups (P < 0.05), and the diameter of hair follicles in the SPE group was significantly larger than that of the NC and XPE groups (P < 0.05). There was no significant difference in the number of hair follicles among the three groups directly (P > 0.05). The diameter of hairs in the SPE group was highly significantly greater than that in the NC and XPE groups (P < 0.001), and the diameter of hairs in the XPE group was significantly greater than that in the NC and SPE groups (P < 0.01). In addition, the expression of the hair follicle growth markers Lef1, Wnt10b, Dkk1, b-catenin, Ptch1, and Shh indicated that circulating exosomes can accelerate the hair follicle development cycle. The expression of the hair melanin synthesis genes FZD3 and FZD4 indicated that circulating exosomes can affect hair follicle melanin synthesis. Immunohistochemistry results showed that the SPE group had greater levels of a-SMA protein. miR-31-5p, miR-133b, miR-433-3p, and miR-218 were similarly expressed in different samples, and the expression of all these miRNAs was significantly greater in the SPE group compared with the XPE group (P < 0.05). Circulating exosomes in Xinji fine-wool and small-tailed Han sheep altered the hair growth in mice.
1 Introduction
Circulating exosomes are microscopic vesicles secreted by cells that circulate in the body via the bloodstream (Isaac et al., 2021). Circulating exosomes come from various tissues and organs and are stable in the blood, so microRNAs (miRNAs) contained in circulating exosomes in the blood are usually regarded as stable and reproducible biomarkers (Chen et al., 2008).
It has been reported that depression-like symptoms can be alleviated in mice by injecting blood exosomes from depressed patients (Wang et al., 2021). The aging phenotype of aged mice was alleviated by injecting vesicles from the blood of young mice. These studies demonstrate that circulating exosomes in the blood affect biological development.
Synthesis of animal fibers is a complex physiological process that is influenced by macroscopic factors such as breed, environment, nutrition, and metabolic levels (Rogers, 2006). At the cellular level, animal papilla cells are also affected by small nucleic acids such as miRNAs (Kirgiafini et al., 2024). However, how macrofactors interact with miRNAs has not been studied. In recent years, circulating exosomes in the blood have been shown to affect hair follicle development in a wave-like pattern of metabolism (Ma et al., 2022). The expression of circulating exosomes is susceptible to change due to environmental, dietary, and species factors (Alberro et al., 2021), which suggests that when animals are affected by the environment and other factors, the animals deliver miRNAs through their own circulating exosomes, altering their own fiber traits. In-depth research on the relationship between circulating exosomes and hair growth animals will be of great practical significance for livestock breeding and feeding.
The hair follicle is important for wool growth, and the hair follicle is considered to be a miniature organ follicle formed by the interaction of the neural ectoderm and mesoderm (Schmidt-ullrich and Paus, 2005). Development of the hair follicle begins in the early embryo. Its normal development and alternation of the hair follicle cycle are promoted through interactions between Wnt, Hedgehog, Notch, and bone morphogenetic protein signaling pathways (Mou et al., 2006). Hair growth is usually marked by skin pigmentation as an indicator of the hair follicle growth cycle, whereas hair morphology requires the interaction of mesenchymal and epithelial cell signaling molecules during the early stages of hair follicle development to induce cells to undergo cellular differentiation (Schmidt-ullrich and Paus, 2005). Currently, platelet-rich plasma is used clinically to treat androgenetic alopecia (Balasundaram et al., 2023). Some studies have shown that circulating exosomes can induce hair papilla cells (Nilfououshzadeh et al., 2021), suggesting that blood and exosomes may influence hair follicle growth.
The fineness of sheep wool fibers can be changed by intravenous injection of miRNA mimics (Wang et al., 2023); this experiment considers the influence of a sheep’s own subcortical cells and exosomes on exogenous exosomes. Plasma exosomes of Xinji fine-wool and small-tailed Han sheep were injected into the subcutaneous tissue of hair regeneration model mice. miRNAs have the ability to communicate with each other across species (Schuh et al., 2019), and the phylogenetically conserved sequences of miRNAs do not easily mutate (Ambros, 2004). Therefore, this experiment provides a new perspective for the study of hair follicle growth in domestic animals which will be relevant for feeding optimization and biosecurity in modern animal husbandry.
2 Materials and methods
2.1 In-vivo testing, sample collection, and exosomal characterization
2.1.1 Animals and procedures
Sheep and mice used in this experiment were provided by the Laboratory Animal Experiment Center and the College of Agriculture of Yanbian University (Yanji, China). All animal experiments were conducted in accordance with the International Guidelines for the Care and Use of Laboratory Animals and approved by the College of Agriculture, Yanbian University. The experimental period was 30 days. The study was approved by the Experimental Animal Welfare Ethics Committee of Yanbian University (IACUC) (IACUC Approval No. YD202401015002, approval date: 22 January 2024).
2.1.2 Isolation of circulating exosomes in sheep
The Xinji fine-wool and small-tailed Han sheep used in this experiment were supplied by the College of Agriculture of Yanbian University (Yanji, China). Thirty milliliters of anticoagulated blood was collected aseptically before feeding, and sheep plasma was extracted by gradient centrifugation. The supernatant was centrifuged at 300g at 4°C for 10 min, at 3,000g at 4°C for 10 min, and finally at 10,000g at 4°C for 15 min. The supernatant was mixed with the exosome isolation solution (1:1), allowed to stand for 10 h, and then centrifuged at 12,000g at 4°C for 1 h. Plasma exosomes were obtained by blowing the bottom precipitate of the colloid with ddH2O. Circulating exosomes were obtained by blowing the gelatinous precipitate at the bottom.
2.1.3 Exosome identification
Exosome morphology was observed by transmission electron microscopy (TEM). Ten microliters of sample solution was dropped on a copper grid, incubated at room temperature for 10 min, and washed with sterile evaporated water for 30 s. Then, 10 μL of 2% uranyl acetate was dropped on the copper grid, and the grid was negatively stained for 1 min, dried under a blazing light for 2 min, and observed with TEM.
NTA nanoparticle tracking analyzed the particle size distribution of the samples. The sample cell was washed with 1× phosphate-buffered saline (PBS); samples were taken at 12,000-fold dilution in 1× PBS buffer and readings were recorded at 11 locations and cycled twice at each location. Anomalous locations were optimized by the machine software to compute the mean, median, and diameter of the samples.
Western blotting was used to identify 10 µL of exosome sample solution, and 1 µL of super nuclease and 20 µL of protein supersampling buffer were mixed and boiled at 100°C for 10 min. Proteins were separated on 8%–12% sodium dodecyl sulfate polyacrylamide gels with a Trans-Blot Turbo (Bio-Rad, CA, USA). Membranes were blocked in 5% skimmed milk for 1 h at room temperature and incubated overnight at 4°C with the following primary antibodies: anti-Tsg101 (1:1,000, Abcam, Cambridge, MA, USA) and anti-CD9 (1:1,000, Smart-Life Science, CN).
2.1.4 Animal experimental study design
After 1 week of acclimatization, 21 mice were randomly divided into three groups: control group (NC), model mice injected with exosomes from Xinji fine-wool sheep (XPE), and model mice injected with exosomes from the small-tailed Han sheep group (SPE). The dorsal hair of the mice was shaved with a pet shaver and hair removal was achieved with a depilatory cream, which was applied for 5–10 min after being wiped with lukewarm water to expose the pink skin. The exosomes were used to determine the circulating exosome concentration in sheep using the BCA method. Based on the results of the BCA assay, the exosome concentration was diluted to 10 mg/mL using ddH2O, and 0.2 mL of exosomes were injected subcutaneously into the test area (Figure 1) of each mouse once a day for 7 days. Photographs were taken and recorded on days 5, 10, and 15 after injection. Observations were recorded on day 15 and the whole test period was 23 days. At the end of the experimental period, mice were anesthetized with 2% tribromoethanol by intraperitoneal injection at a dose of 0.01 mL/g. The skin on the backs of animals was collected following anesthesia.
2.1.5 Melanin measurement
Mice skin samples were cut and dissolved in 0.1 M of NaOH for 12 h. The absorbance of the solution was determined spectrophotometrically at 500 nm.
2.1.6 Determination of the diameter and number of hair follicles in the skin of model mice
Fifteen days after exosome injection, the skin tissues of the test area on the backs of mice were spread on filter paper, and the filter paper and samples were immersed in 10% neutral buffered formalin (NBF; 4% formaldehyde w/v in phosphate buffer, pH 7.2) for 48 h at room temperature. After fixation, specimens were dehydrated through a graded ethanol series (70%, 80%, 90%, 95%, and 100%) with 1-h immersion at each concentration, prior to paraffin embedding. Tissue sections (5 μm) were prepared and stained with hematoxylin and eosin (H&E) and then mounted with neutral balsam. After section staining, the total number of hair follicles was counted and the diameter of hair follicles was measured by taking cross-sections of the skin in the same horizontal plane, and to ensure unbiased sampling, we first systematically randomly selected three fields of view from a predetermined grid in the sample area. Then, under a ×100 objective lens, we further randomized them by blindly choosing stage coordinates using a random number table.
2.1.7 RT-qPCR
To verify the effect of circulating exosomes on mice skin, qPCR was used to compare the expression levels of Lef1, Wnt10b, Dkk1, and β-catenin, key genes for early hair follicle development; Ptch1 and Shh, key genes for hair follicle flourishing development; and FZD3 and FZD4, key genes for hair fiber diameter. The relative expression trends of miRNAs related to hair follicle development, i.e., miR-31-5p, miR-133b, miR-433-3p, and miR-218, in sheep plasma exosomes and skin versus that of model mice were determined using RT-qPCR. Skin tissues were collected from the dorsal test area, and miRNA and mRNA were extracted according to the instructions of miRcute miRNA Extraction and Isolation Kit (QIAGEN, Beijing, DP501, China); miRNA reverse transcription was performed according to the miRcute Enhanced miRNA cDNA First Strand Synthesis Kit (QIAGEN, Beijing, KR211, China); mRNA reverse transcription was performed according to the instructions of FastKing One-Step Degenomic cDNA First-Strand Synthesis Premix Kit (QIAGEN, Beijing, KR118, China), and qRT-PCR analyses were performed with FastKing One-Step RT-PCR Kit (QIAGEN, Beijing, KR123, China) on the Eco48 Real-Time PCR System using the primer sets listed in Table 1. β-Actin and U6 were used as internal reference genes. To assess changes in miRNA and mRNA expression levels, we used the 2−ΔΔCt method to calculate normalization and fold change data analysis. Hair growth marker genes and miRNA primers were referenced (Table 1).
3 Results
3.1 Identification of sheep serum exosomes
TEM showed a distinct membrane boundary and a large circular concave teacup-shaped structure from 30 nm to approximately 90 nm (Figure 2A); particle size tracking analysis (NTA) showed that the particles were mainly concentrated in the range of 30 nm to 150 nm, with a peak concentration of particles at 90 nm (Figure 2B). Western blotting demonstrated that the samples contained the exosome marker proteins TSG101 and CD9 (Figure 2C).
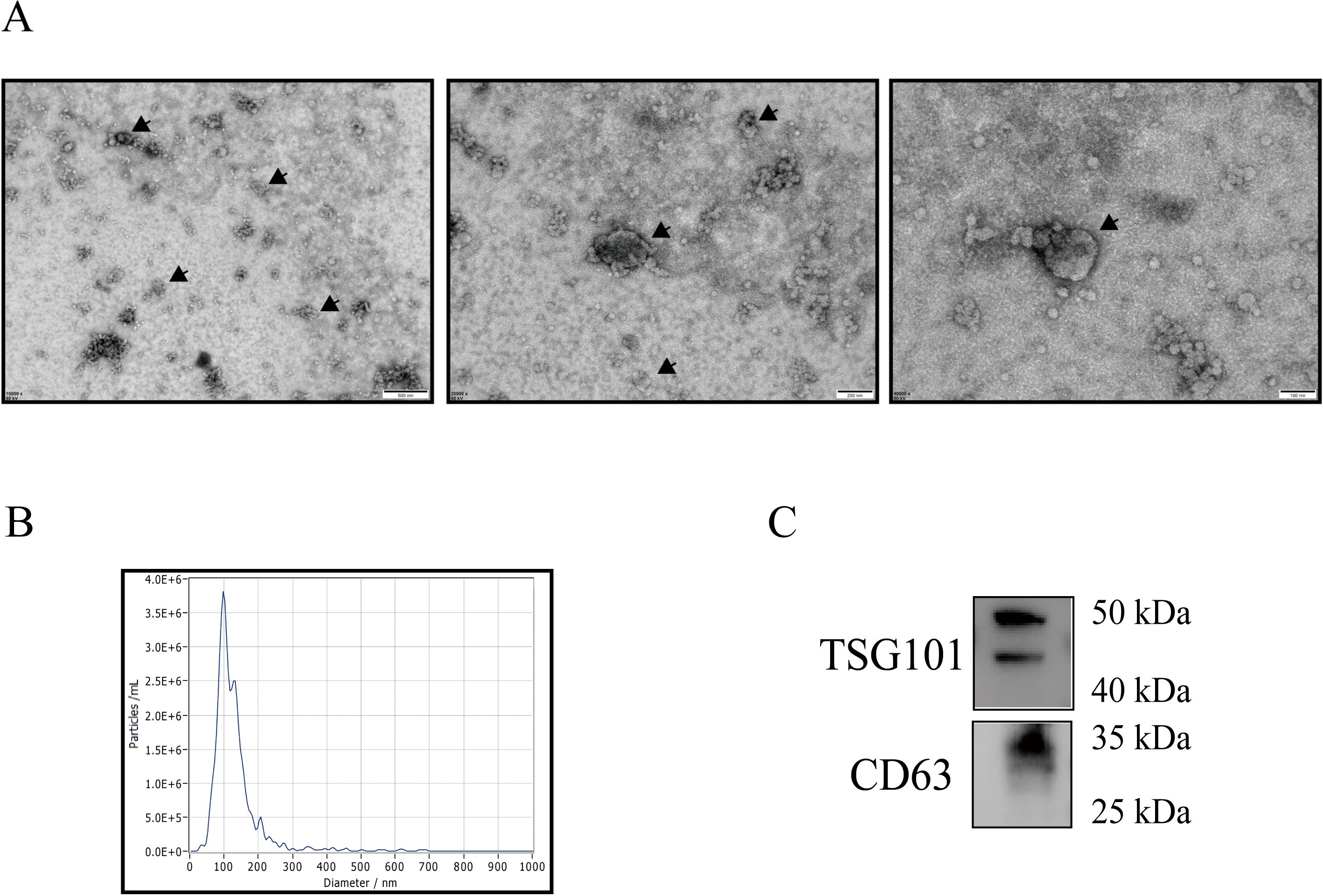
Figure 2. Exosome identification. Transmission electron microscopy (A). Nanoparticle tracking analysis (B). Western blotting of TSG101 and CD9 (C).
3.2 Intravenous injection of circulating exosomes promotes hair growth in mice
3.2.1 Evaluation of hair growth function in model mice
Examination of the skin on the backs of mice at different stages of exosome injection showed that melanin pigmentation in the XPE group was lighter than in the NC group on day 5. In contrast, the SPE group showed deeper pigmentation compared to the NC group (Figure 3). Hair growth on the backs of mice was vigorous on days 10 and 15, and there was no significant difference in the visual observation of the mice.
3.2.2 Diameter and number of hair follicles in mouse skin
Hair follicles in the NC and XPE groups were rounded with resting phase characteristics, while hair follicles in the SPE group grew and extended to the rump with a teardrop shape (Figures 4A, B), which was characteristic of anagen development. Statistically, the diameter of hair follicles in the XPE group was significantly smaller than that in the NC and SPE groups (P < 0.05), and the diameter of hair follicles in the SPE group was significantly larger than that in the NC groups (P < 0.05) (Figure 4C). There was no significant difference in the number of hair follicles between the three groups (P > 0.05) (Figure 4D).
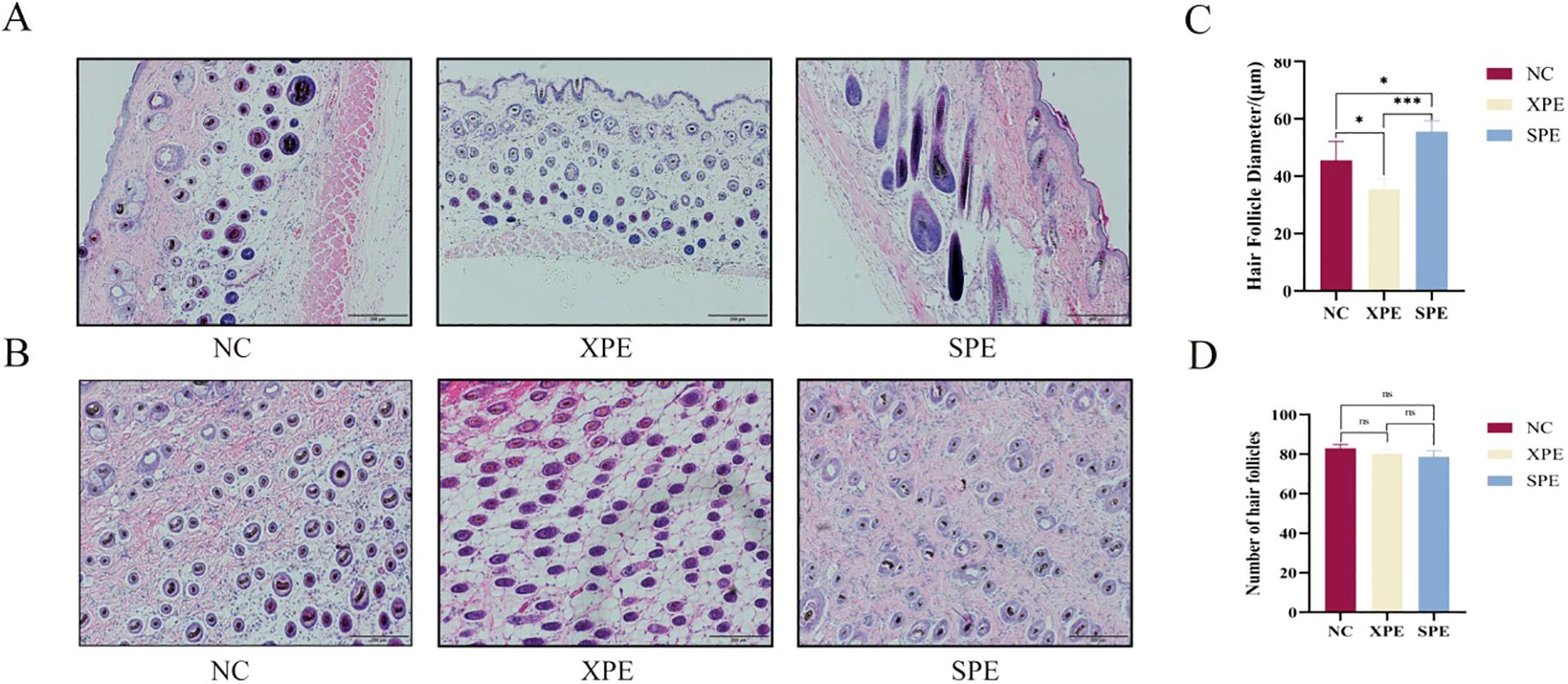
Figure 4. Longitudinal tissue section of the skin (A). Skin transverse tissue section (B). Quantification of hair follicle diameter (C) and number of hair follicles (D). *P<0.05 and ***P<0.001. ns, not significant (P ≥ 0.05).
3.2.3 Hair fiber diameter and melanin measurement in mice
Measurement of hair fiber fineness showed that hair diameter in the SPE group was significantly greater than that in the NC and XPE groups (P < 0.001) (Figures 5A, C) and hair diameter in the XPE group was significantly lower than that in the NC and SPE groups (P < 0.01) (Figures 5A, C). The concentration of melanin in the hairs of the SPE group was statistically significantly higher than that of the NC and XPE groups (P < 0.001) (Figures 5B, D).
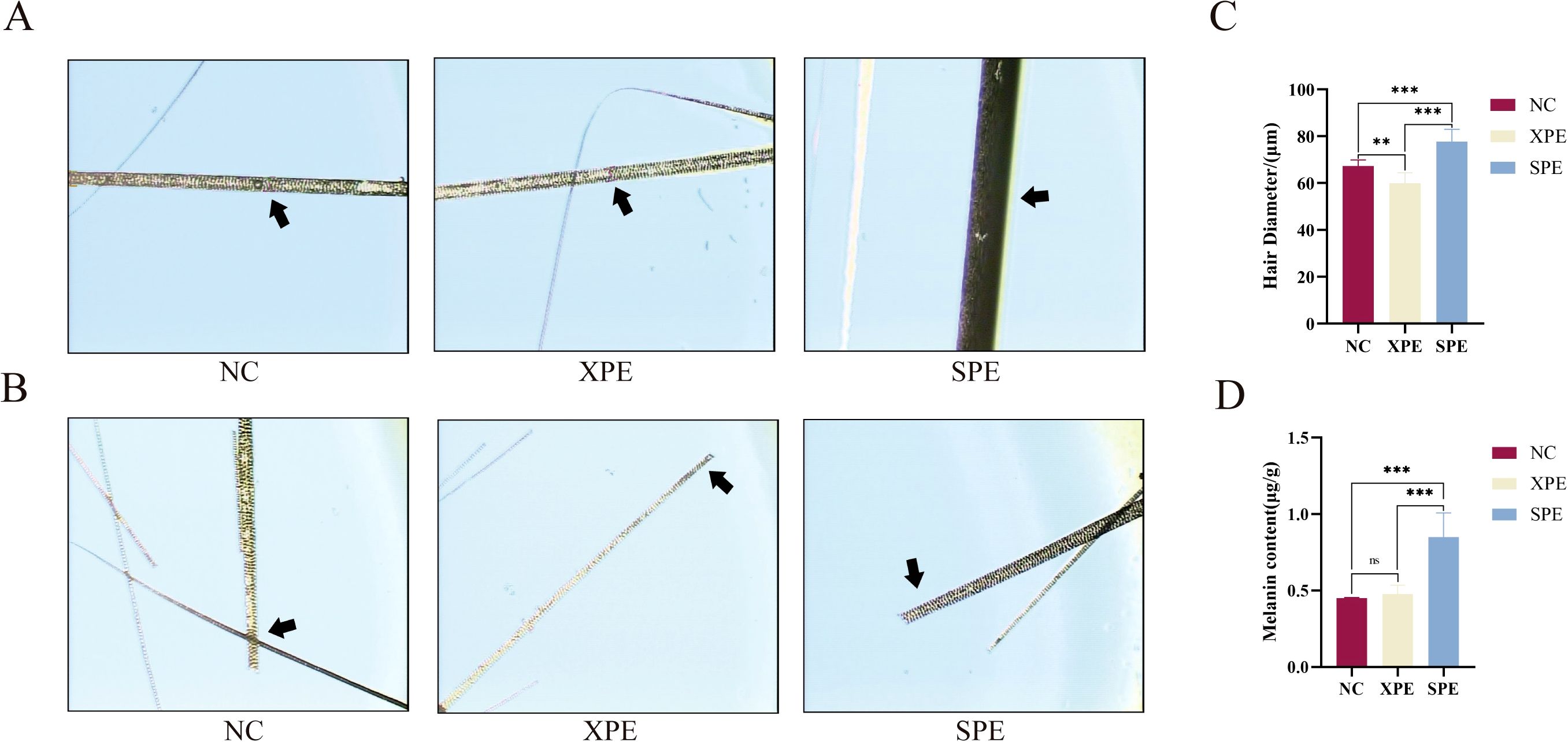
Figure 5. Hair fiber diameter measurement (A). Hair root observation (B). Quantification of hair fiber diameter (C) and hair melanin content (D), ** indicates (P<0.01) and ***P < 0.001. ns, not significant (P ≥ 0.05).
3.2.4 Mouse skin immunohistochemistry
The results of immunohistochemical tests on mouse skin tissues of the negative and positive control groups showed that the hair follicles within the reticular layer all expressed the hair papilla-associated marker α-SMA, and the SPE group bound more α-SMA (Figure 6).
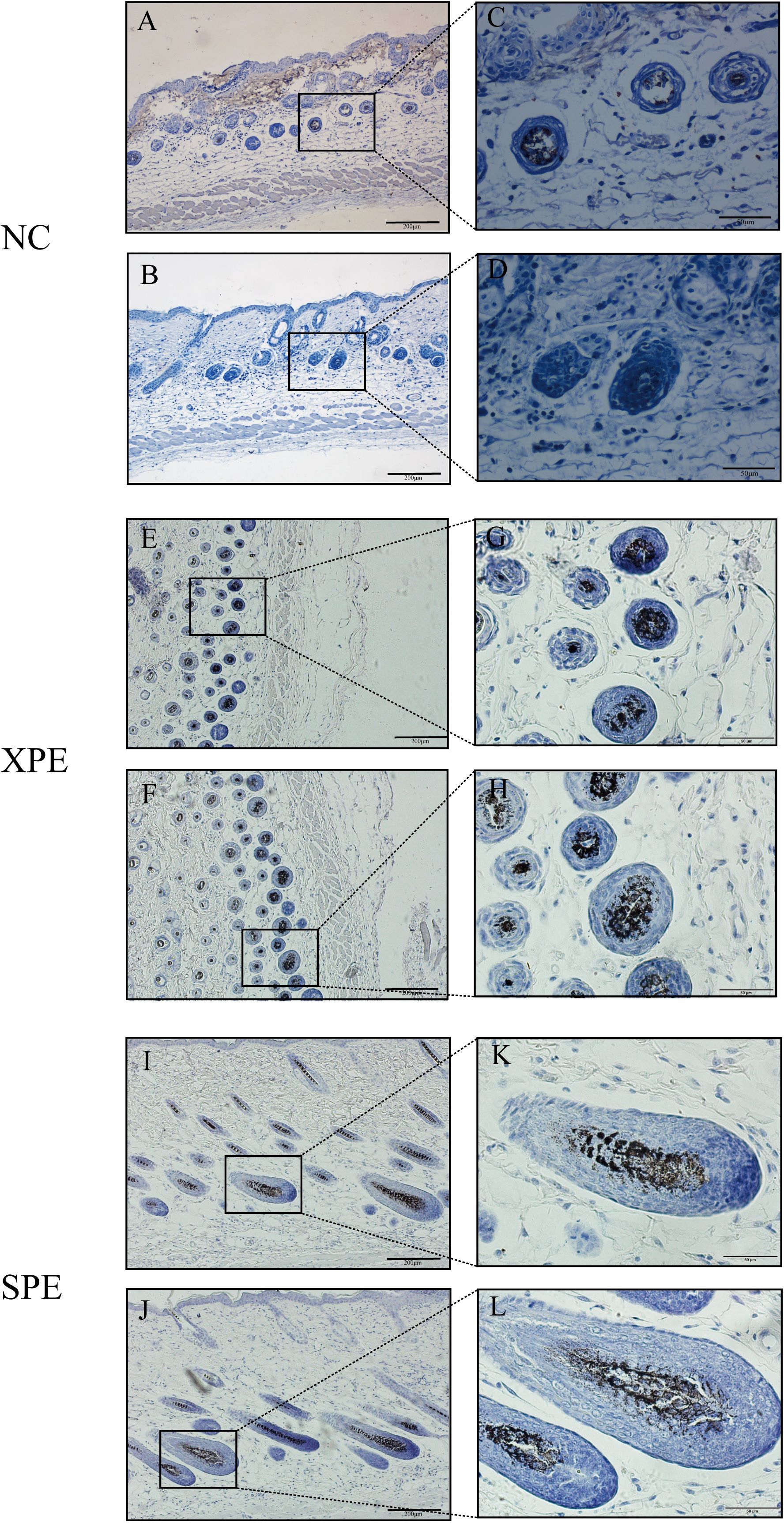
Figure 6. Immunohistochemistry of the skin tissue. NC-positive group (A, C). NC-negative control group (B, D). XPE-positive group (E, G). XPE-negative control group (F, H). SPE-positive group (I, K). SPE-negative control group (J, L).
3.2.5 Assessment of hair follicle development markers in mouse skin
Some marker genes play a crucial role in activating the Wnt and Shh/Hedgehog signaling pathways, which promote the proliferation of hair follicle cells (Figure 7A). Both pathways converge on transcriptional regulation, highlighting their critical roles in modulating cell proliferation mechanisms. To further understand the impact of these markers on hair growth, we analyzed their relative expression levels. RT-qPCR was used to compare the relative expression of genes involved in the early development of hair follicles. The expression of Lef1 in the SPE group was significantly higher than that in the NC and XPE groups (P < 0.001), while the expression of Wnt10b in the XPE group was significantly higher than that in the NC and SPE groups (P < 0.05). The expression of Dkk1 in the NC group was significantly higher than that in the XPE and SPE groups, while the expression of β-catenin in the SPE group was significantly higher than that in the NC and XPE groups (P < 0.01) (Figure 7B). Among the genes involved in hair follicle anagen development markers, the expression of Ptch1 in the XPE group was significantly higher than that in the NC and SPE groups (P < 0.01); at the same time, the expression in the NC group was significantly higher than that in the SPE group (P < 0.01). The expression of Shh in the SPE group was significantly lower than that in the NC and XPE groups (P < 0.001), and the expression of FZD3 in the SPE group was significantly higher than that in the NC and XPE groups (P < 0.001). FZD3 expression was significantly higher than that in the NC and XPE groups (P < 0.001). FZD4 expression in the XPE group was significantly higher than that in the NC and SPE groups (P < 0.01) (Figure 7C).
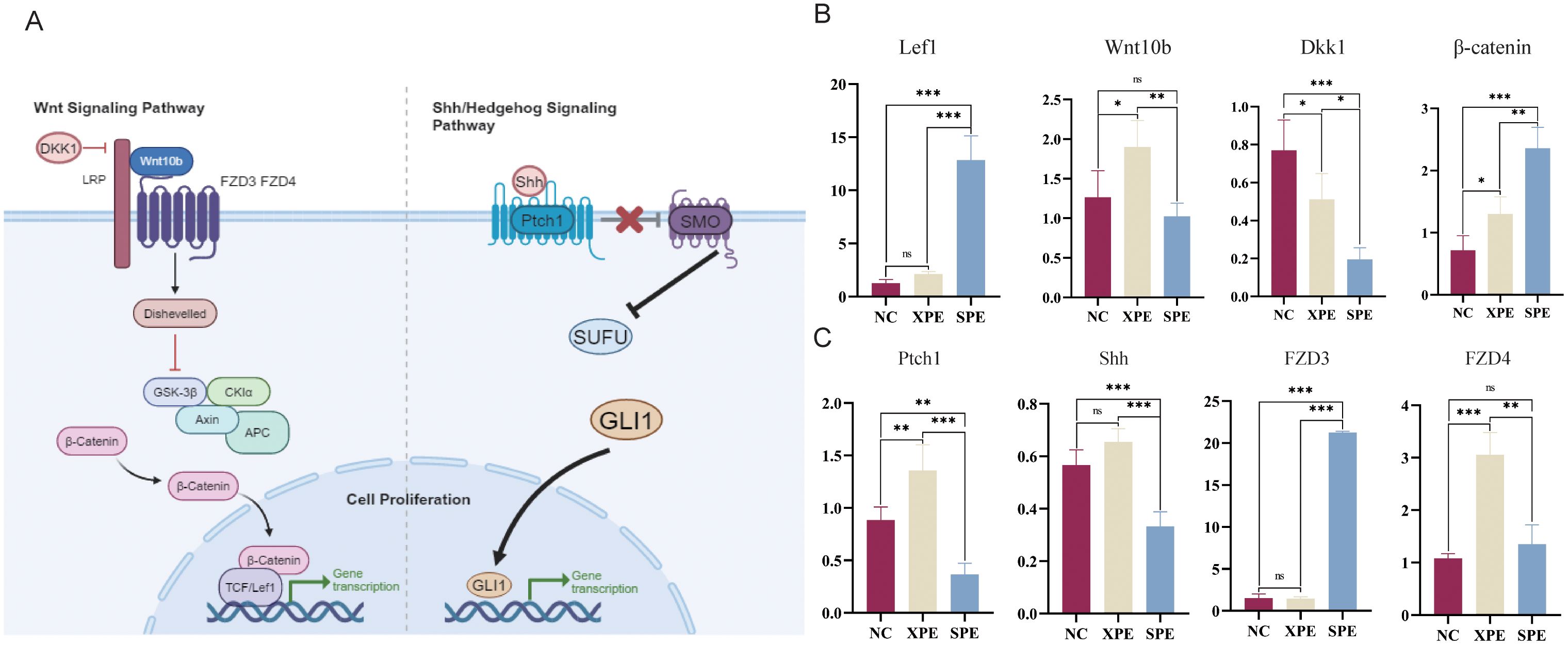
Figure 7. Schematic diagram of the marker pathways (A). Key genes involved in early hair follicle development (B). Key genes involved in hair follicle anagen stage and hair fiber diameter (C). *P < 0.05, ** indicates (P<0.01) and ***P < 0.001. ns, not significant (P ≥ 0.05).
3.3 RT-qPCR quantification of miRNAs affecting animal hair growth
miRNA quantification showed that the relative expression levels of miR-31-5p, miR-133b, miR-433-3p, and miR-218 in the plasma exosomes of sheep were all higher in the SXW group of small-tailed Han sheep than those in the XFWS group of Xinji fine-wool sheep (P < 0.05) (Figure 8A) and that the relative expression of these genes in the skin tissues of sheep was higher in the SXW group of small-tailed Han sheep than that in the XFWS group of Xinji fine-wool sheep (P < 0.001) (Figure 8B). The relative expression of skin tissues of model mice in the SPE group was higher than in the XPE group, and at the same time, the relative expression of both the SPE group and the XPE group was higher than that of the NC group (P < 0.001) (Figure 8C).
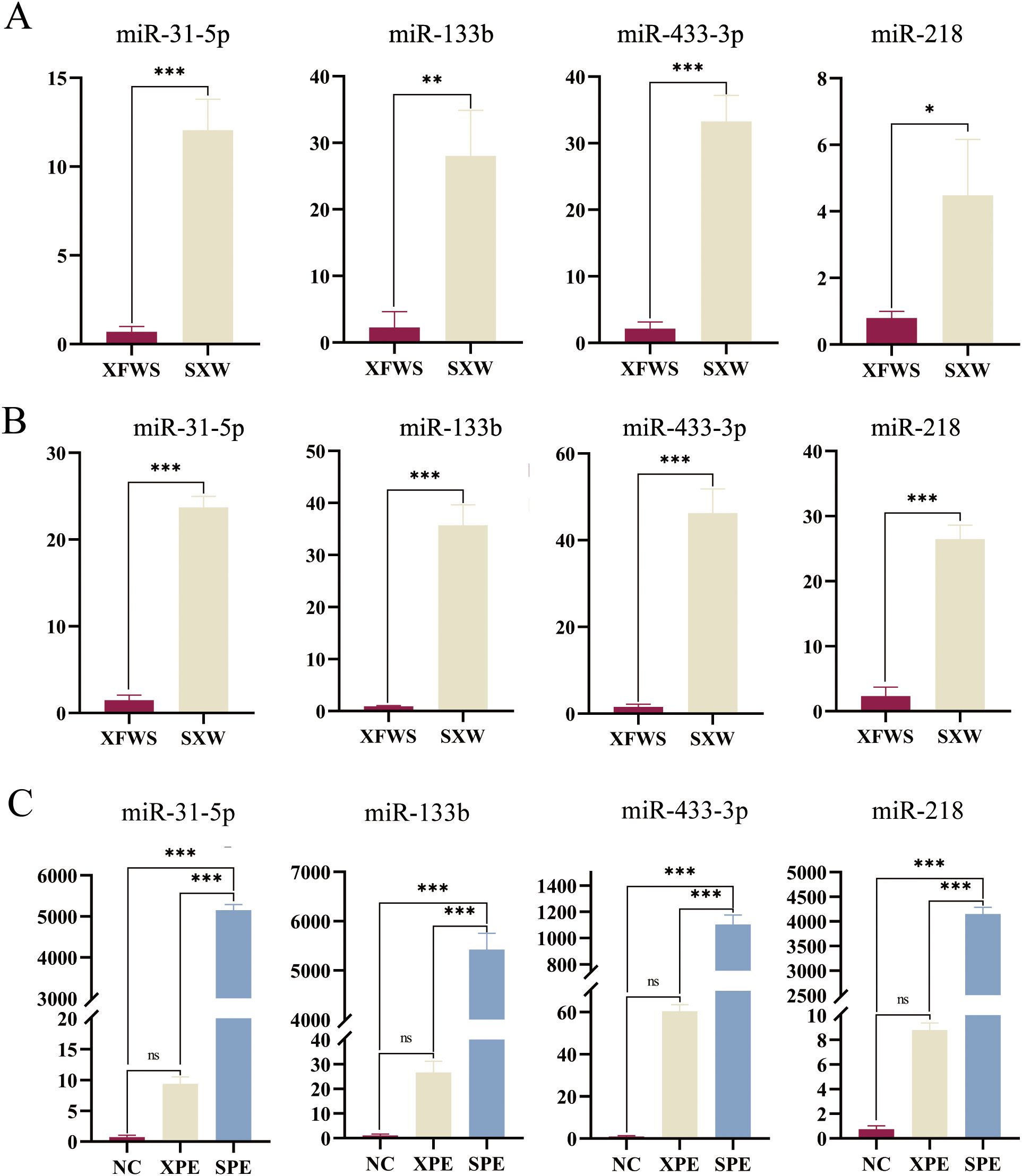
Figure 8. Relative expression of miRNAs in plasma exosome samples of sheep (A). Relative expression of miRNAs in skin tissues of sheep (B). Relative expression of miRNAs in skin tissues of model mice (C). *P < 0.05, ** indicates (P<0.01) and ***P < 0.001. ns, not significant (P ≥ 0.05).
4 Discussion
The regulatory role of circulating exosomes in organisms has become increasingly clear following in-depth studies. In a study of neuropathic pain in mice treated with cross-species and cross-sex serum, circulating exosomes and the miRNAs they contain had a long-distance regulatory role in organisms (Buchheit et al., 2023). For example, research shows that homologous and heterologous sera are used to culture bone marrow mesenchymal stem cells, which showed different differentiation trends (Fani et al., 2016), and miRNAs released from adipose tissue reach the liver via the bloodstream to regulate gene expression and metabolic control in host tissues (Fatima and Nawaz, 2017). Moreover, research also shows that circulating exosomal miRNAs can be used as biomarkers for the systemic skin disease lupus erythematosus (Li et al., 2020). Emerging evidence, including studies by Ma et al. (2022) and Wang et al. (2023), demonstrates that circulating exosomes regulate hair growth by modulating follicular cell signaling pathways. Specifically, Ma et al. found that exogenous exosomes could diffuse into the hair follicle through their own metabolism and activate the Wnt/β-catenin signaling pathway associated with hair regeneration by injecting fluorescently labeled exosomes into veins (Ma et al., 2022). Jiankui Wang et al. altered hair regeneration by injecting vectorless miRNA mimics into the veins of sheep. miRNA mimics were also injected into the veins of sheep to alter sheep wool fiber fineness (Wang et al., 2023). These results provide more confidence for future trials on sheep.
In the present study, we measured the diameter of mouse hair, determined the number and diameter of hair follicles in mice, and compared the expression of genes related to the influence of hair growth. Sheep circulating exosomes promote mouse hair growth via Lef1 and β-catenin upregulation, thereby activating the canonical Wnt/β-catenin signaling pathway in a manner mechanistically identical to human umbilical cord mesenchymal stem cell exosomes (Jiao et al., 2024). A comparative analysis of Wnt10b and Dkk1 expression levels in Rex rabbit skin during seasonal molt cycles showed that sheep circulating exosomes promote mouse hair growth by modulating hair follicle growth cycles (Liu et al., 2023). Moreover, the Shh Hedgehog signaling pathway modulates mouse hair diameter. Studies have demonstrated that secondary hair follicles of cashmere goats circulating exosomes significantly modulate mouse hair fiber diameter by Shh and Ptch1 expression to activate the Shh/Hedgehog signaling pathway (Zhang et al., 2024). Comparative transcriptome analysis of datasets associated with wool fiber diameter variation in Angora rabbits demonstrates that Xinji fine-wool sheep and small-tailed Han sheep modulate mouse hair fiber diameter via differential regulation of FZD3 and FZD4 expression (Huang et al., 2023). Our results indicate that the hair follicle development markers in mouse skin tissues under the influence of exosomes cause a rapid transition to the anagen phase and influence the diameter of mouse hair fiber by regulating the expression of genes such as FZD3 and FZD4. The results of mouse skin immunohistochemistry are consistent with these tests. We can conclude that miRNAs in circulating exosomes in the plasma of small-tailed Han sheep and Xinji fine-wool sheep affect animal hair growth.
However, there are some limitations to our experiments; for example, when measuring the diameter of hair follicles in mice, it is difficult to distinguish the primary hair follicles from the secondary hair follicles (Chi et al., 2015). All the hair diameters measured in the XPE group were relatively large, but this did not affect the conclusions of the experiment that plasma exosomes from Xinji fine-wool sheep reduce hair in mice. The same issue exists with skin tissue sections, although SPE and XPE did not show different effects. Both the SPE and XPE groups promoted the development of hair follicles compared to the NC group, but upon observation and analysis, it was found that there was a reduction in the diameter of the long protected hair follicles in the dermis in the XPE group compared to the other two groups; this will require further corroboration. We suspected that the erratic therapeutic efficacy in the treatment of pemphigus vulgaris using platelet-rich plasma is largely influenced by the quality of native plasma (Nilfououshzadeh et al., 2021; Laufer britva et al., 2021; Gupta et al., 2021).
As the trial proceeded, we were also able to conclude that miRNAs can be regulated across species by circulating exosomes. At the beginning of the study, we thought that the heterologous model could circumvent the resistance of our own subcortical cells and exosomes to exogenous exosomes. As the experiment progressed, regulation of miRNAs in exosomes for animal hair growth was observed. For example, an assay used by Bilbao-Arribas et al. showed the presence of specific miRNAs in sheep (Bilbao-Arribas et al., 2023). This suggests that species can allow miRNAs to be stabilized in vivo. Friedman et al. demonstrated that miRNAs are not only conserved among species but most mammalian mRNAs are their conserved targets (Friedman et al., 2009). This led us to the possibility of cross-species epigenetic modification of miRNAs. Since it is difficult to screen miRNAs from plasma exosomes that affect fiber diameter in sheep, we found that the hair papilla exosome miR-218-5p in Hu’s study had the same sequence as oar-miR-218 (Hu et al., 2020); miR-133b was found to promote the proliferation and induction of human hair papilla cells (Deng et al., 2021). chi-miR-433 is involved in hair follicle development at different periods in goats, and miR-31-5p promotes the proliferation of hair follicle stem cells (Ma et al., 2022; Feng et al., 2021). Our experiments also showed that these miRNAs are similarly expressed in the blood and skin of sheep as well as under the skin of mice, and we believed that these miRNAs might be involved in the regulation of hair growth. miRNAs, 19–23 nucleotide non-coding RNAs, have emerged as a frontier focus in life sciences due to their cross-species regulatory mechanisms. Increasing evidence demonstrates that animal-derived miRNAs can transcend taxonomic barriers to participate in interspecies gene regulation (Chen and Kim, 2024). Recent studies reveal that miR-218 promotes hair follicle regeneration by targeting conserved non-coding regions of the SFRP2 gene in humans, sheep, and Angora rabbits (Hu et al., 2020). Notably, this miRNA exhibits conserved molecular functions in rabbit hair follicle development (Zhao et al., 2019) and adipose deposition models in pig (Shan et al., 2022), with dual-luciferase reporter assays confirming the evolutionary conservation of its binding sites. Additionally, miR-199a-3p significantly promotes hair growth in both sheep and Rex rabbit models (Wang et al., 2023), while miR-200a regulates coat color in cashmere goats and mice through conserved mechanisms, as demonstrated by consistent target gene expression patterns across species following subcutaneous injection of miR-200a inhibitors (Li, 2021). Collectively, these cross-species investigations provide critical theoretical insights into miRNA-mediated interspecies phenotypic regulatory mechanisms.
Finally, this experiment has strong practical significance for livestock breeding. Research shows that miRNAs derived from cooked pork may function as critical regulators of metabolic disorder in mice (Shen et al., 2023). When bovine tissue was subjected to cooking and a simulation of human digestion, miRNAs were still detected after all the procedures (Pieri et al., 2022). Combined with the results of this experiment, we believe that miRNAs of circulating exosomes are on the border of species and kingdom intercommunication. Based on the results of this experimental study, it is reasonable to believe that when the content of circulating miRNAs is changed because of the environment (Figure 9), diet, species, or other factors, it will alter the expression of miRNAs in plasma exosomes of sheep. miRNAs in circulating exosomes will change hair growth in animals (Fani et al., 2016; Chishti et al., 2021; Quan et al., 2019).
Secondly, in a trial study (Kim et al., 2024) involving homogeneity and xenogeneic exosome therapy of canine dermatitis, exosome degradation time in vivo is usually considered to be 7 days (Preuber et al., 2018). However, in this trial, it was found that the expression of miRNAs in model mice was always higher than that of NC after 15 days of exosome injection, which may indicate that exosomes can exist in mice for a long time. This shows us that circulating exosomes in sheep can be used as a hair growth drug or carrier potential. Thirdly, this trial offers the possibility of using large livestock circulating exosomes to treat small pet diseases. This is due to the fact that exosomes are hypoimmune compared to plasma (Wei et al., 2023). There are already trials related to the use of xenoanimal plasma therapy (Meira et al., 2020; Peng et al., 2016), which could be a novel idea for the treatment of pet skin baldness and other pet diseases.
5 Conclusion
Building on the established premise that circulating exosomes influence hair follicle development, this study further confirms that circulating exosomes from Xinji fine-wool sheep and small-tailed Han sheep exert differential regulatory effects on mouse hair growth cycles and fiber fineness. Subsequent analysis revealed consistent differential expression trends of miR-31-5p, miR-133b, miR-433-3p, and miR-218 across circulating exosomes, skin tissues of both sheep breeds, and mouse skin tissues, indicating a close correlation between mouse hair growth variations and miRNA expression levels in the circulating exosomes of these two sheep breeds. In summary, the findings have great potential for increasing the production of sheep wool.
Data availability statement
The original contributions presented in the study are included in the article/Supplementary Material. Further inquiries can be directed to the corresponding authors.
Ethics statement
The animal study was approved by the Laboratory Animal Ethics Committee of Yanbian University. The study was conducted in accordance with the local legislation and institutional requirements.
Author contributions
XZ: Conceptualization, Formal Analysis, Methodology, Software, Validation, Writing – original draft, Writing – review & editing. WW: Methodology, Software, Visualization, Writing – original draft, Writing – review & editing. WF: Investigation, Writing – review & editing. YZ: Software, Writing – review & editing. JF: Validation, Writing – review & editing. JC: Investigation, Writing – review & editing. DZ: Resources, Writing – review & editing. FS: Funding acquisition, Project administration, Supervision, Writing – review & editing.
Funding
The author(s) declare that financial support was received for the research and/or publication of this article. This research was supported by the National Natural Science Foundation of China (Grant No. 32060781) and Jilin Provincial Department of Education Foundation Program (Grant No. JJKH20220543KJ).
Acknowledgments
This research was graciously supported by the National Natural Science Foundation of China (Grant No. 32060781) and the Foundation Program of the Jilin Provincial Department of Education (Grant No. JJKH20220543KJ). All figures presented in this study were skillfully crafted using BioRender.com. We sincerely thank the corresponding author for their generous support and guidance, and all co-authors for their collaborative efforts. Their contributions were crucial to the success of this study. Special thanks go to my partner, Wang Wei, for their unwavering support throughout this research. Their presence provided the strength and motivation needed to overcome challenges.
Conflict of interest
The authors declare that the research was conducted in the absence of any commercial or financial relationships that could be construed as a potential conflict of interest.
Generative AI statement
The author(s) declare that no Generative AI was used in the creation of this manuscript.
Publisher’s note
All claims expressed in this article are solely those of the authors and do not necessarily represent those of their affiliated organizations, or those of the publisher, the editors and the reviewers. Any product that may be evaluated in this article, or claim that may be made by its manufacturer, is not guaranteed or endorsed by the publisher.
Supplementary material
The Supplementary Material for this article can bVe found online at: https://www.frontiersin.org/articles/10.3389/fanim.2025.1562392/full#supplementary-material
References
Alberro A., Iparraguirre L., Fernandes A., Otargui D. (2021). Extracellular vesicles in blood: sources, effects, and applications. Int. J. Mol. Sci. 22, 8163. doi: 10.3390/ijms22158163
Balasundaram M., Kumari R., Ramassamy S. (2023). Efficacy of autologous platelet-rich plasma therapy versus topical Minoxidil in men with moderate androgenetic alopecia: a randomized open-label trial. J. Dermatol. Treat 34, 2182618. doi: 10.1080/09546634.2023.2182618
Bilbao-Arribas M., Guisasola-Serrano A., Varela-Martínez E., Jugo B. M. (2023). The sheep miRNAome: Characterization and distribution of miRNAs in 21 tissues. Gene 851, 146998. doi: 10.1016/j.gene.2022.146998
Buchheit T., Huh Y., Breglio A., Bang S., Xu J., Matsuoka Y., et al. (2023). Intrathecal administration of conditioned serum from different species resolves Chemotherapy-Induced neuropathic pain in mice via secretory exosomes. Brain behavior Immun. 111, 298–311. doi: 10.1016/j.bbi.2023.04.013
Chen X., Ba Y., Ma L., Cai X., Yin Y., Wang K., et al. (2008). Characterization of microRNAs in serum: a novel class of biomarkers for diagnosis of cancer and other diseases. Cell Res. 18, 997–1006. doi: 10.1038/cr.2008.282
Chen L. L., Kim V. N. (2024). Small and long non-coding RNAs: Past, present, and future. Cell 187, 6451–6485. doi: 10.1016/j.cell.2024.10.024
Chi W., Wu E., Morgan B. A. (2015). Earlier-born secondary hair follicles exhibit phenotypic plasticity. Exp. Dermatol. 24, 265–268. doi: 10.1111/exd.2015.24.issue-4
Chishti A. A., Khan H. M., Pattoo R. A., Sofi A. H. (2021). Effect of housing and nutritional interventions during winter on wool attributes of Corriedale sheep. Trop. Anim. Health production 53, 348. doi: 10.1007/s11250-021-02792-2
Deng W., Hu T., Han L., Liu B., Tang X., Chen H., et al. (2021). miRNA microarray profiling in patients with androgenic alopecia and the effects of miR-133b on hair growth. Exp. Mol. Pathol. 118, 104589. doi: 10.1016/j.yexmp.2020.104589
Fani N., Ziadlou R., Shahhoseini M., Baghaban eslaminejad M. (2016). Comparative epigenetic influence of autologous versus fetal bovine serum on mesenchymal stem cells through in vitro osteogenic and adipogenic differentiation. Exp. Cell Res. 344, 176–182. doi: 10.1016/j.yexcr.2015.10.009
Fatima F., Nawaz M. (2017). Long Distance Metabolic Regulation through Adipose-Derived Circulating Exosomal miRNAs: A Trail for RNA-Based Therapies? Front. Physiol. 8, 545. doi: 10.3389/fphys.2017.00545
Feng Y., Wang J., Ma J., Zhang L., Chu C., Hu H., et al. (2021). miR-31-5p promotes proliferation and inhibits apoptosis of goat hair follicle stem cells by targeting RASA1/MAP3K1 pathway. Exp. Cell Res. 398, 112441. doi: 10.1016/j.yexcr.2020.112441
Friedman R. C., Farh K. K., Burge C. B., Bartel D. P. (2009). Most mammalian mRNAs are conserved targets of microRNAs. Genome Res. 19, 92–105. doi: 10.1101/gr.082701.108
Gupta A. K., Renaud H. J., Rapaport J. A. (2021). Platelet-rich plasma and cell therapy: the new horizon in hair loss treatment. Dermatologic Clinics 39, 429–445. doi: 10.1016/j.det.2021.04.001
Hu S., Li Z., Lutz H., Huang K., Su T., Cores J., et al. (2020). Dermal exosomes containing miR-218-5p promote hair regeneration by regulating β-catenin signaling. Sci. Adv. 6, eaba1685. doi: 10.1126/sciadv.aba1685
Huang D., Ding H., Wang Y., Cheng G., Wang X., Leng T., et al. (2023). Hair follicle transcriptome analysis reveals differentially expressed genes that regulate wool fiber diameter in angora rabbits. Biology 12, 445. doi: 10.3390/biology12030445
Isaac R., Reis F. C. G., Ying W., Olefsky J. M. (2021). Exosomes as mediators of intercellular crosstalk in metabolism. Cell Metab. 33, 1744–1762. doi: 10.1016/j.cmet.2021.08.006
Jiao Y., Sun Q. M., Shen Y. C., Li Q. S., Piao Y. J., Gong L. (2024). Stimulation of mouse hair regrowth by exosomes derived from human umbilical cord mesenchymal stem cells. Acta histochemica 126, 152184. doi: 10.1016/j.acthis.2024.152184
Kim S. W., Lim K. M., Cho S. G., Ryu B., Kim C. Y., Park S. Y., et al. (2024). Efficacy of allogeneic and xenogeneic exosomes for the treatment of canine atopic dermatitis: A pilot study. Animals 14, 282. doi: 10.3390/ani14020282
Kirgiafini D., Kyrgiafini M. A., Gournaris T., Mamuris Z. (2024). Understanding circular RNAs in health, welfare, and productive traits of cattle, goats, and sheep. Animals 14, 733. doi: 10.3390/ani14050733
Laufer britva R., Keren A., Ginzburg A., Ullmann Y., Paus R., Gilhar A. (2021). Evidence from a humanized mouse model of androgenetic alopecia that platelet-rich plasma stimulates hair regrowth, hair shaft diameter and vellus-to-terminal hair reconversion in vivo. Br. J. Dermatol. 185, 644–646. doi: 10.1111/bjd.20084
Li J. (2021). The effect of miR-200a on the color of Liaoning cashmere and identification of its target genes. [Master’s thesis]. (Jilin University).
Li W., Liu S., Chen Y., Weng R., Zhang K., He X., et al. (2020). Circulating exosomal microRNAs as biomarkers of systemic lupus erythematosus. Clinics (Sao Paulo Brazil) 75, e1528. doi: 10.6061/clinics/2020/e1528
Liu G., Liu C., Zhang Y., Sun H., Yang L., Bai L., et al. (2023). Hair follicle development of rex rabbits is regulated seasonally by wnt10b/β-catenin, TGFβ-BMP, IGF1, and EGF signaling pathways. Animals 13, 3742. doi: 10.3390/ani13233742
Ma L., Chen C., Liu D., Huang Z., Li J., Liu H., et al. (2022). Apoptotic extracellular vesicles are metabolized regulators nurturing the skin and hair. Bioactive materials 19, 626–641. doi: 10.1016/j.bioactmat.2022.04.022
Ma R., Shang F., Rong Y., Pan J., Wang M., Niu S., et al. (2022). Expression profile of long non-coding RNA in inner Mongolian cashmere goat with putative roles in hair follicles development. Front. veterinary Sci. 9, 995604. doi: 10.3389/fvets.2022.995604
Meira R. O., Braga D. N. M., Pinheiro L. S. G., Amorim I. F. G., Vasconcellos L. S., Alberti L. R. (2020). Effects of homologous and heterologous rich platelets plasma, compared to poor platelets plasma, on cutaneous healing of rabbits. Acta cirurgica Bras. 35, e202001006. doi: 10.1590/s0102-865020200100000006
Mou C., Jackson B., Schneider P., Overbeek P. A., Headon D. J. (2006). Generation of the primary hair follicle pattern. Proc. Natl. Acad. Sci. United States America 103, 9075–9080. doi: 10.1073/pnas.0600825103
Nilfououshzadeh M. A., Aghdami N., Taghiabadi E. (2021). Effects of adipose-derived stem cells and platelet-rich plasma exosomes on the inductivity of hair dermal papilla cells. Cell J. 23, 576–583. doi: 10.22074/cellj.2021.7352
Peng W., Kim I. K., Cho H. Y., Seo J. H., Lee D. H., Jang J. M., et al. (2016). The healing effect of platelet-rich plasma on xenograft in peri-implant bone defects in rabbits. Maxillofac. Plast. reconstructive Surg. 38, 16. doi: 10.1186/s40902-016-0061-5
Pieri M., Theori E., Dweep H., Flourentzou M., Kalampalika F., Maniori M. A., et al. (2022). A bovine miRNA, bta-miR-154c, withstands in vitro human digestion but does not affect cell viability of colorectal human cell lines after transfection. FEBS Open Bio 12, 925–936. doi: 10.1002/2211-5463.13402
Preuber C., Hung L. H., Schneider T., Schreiner S., Hardt M., Moebus A., et al. (2018). Selective release of circRNAs in platelet-derived extracellular vesicles. J. extracellular vesicles 7, 1424473. doi: 10.1080/20013078.2018.1424473
Quan S., Nan X., Wang K., Jiang L., Yao J., Xiong B. (2019). Different diets change the expression of bovine serum extracellular vesicle-miRNAs. Animals 9, 1137. doi: 10.3390/ani9121137
Rogers G. E. (2006). Biology of the wool follicle: an excursion into a unique tissue interaction system waiting to be re-discovered. Exp. Dermatol. 15, 931–949. doi: 10.1111/j.1600-0625.2006.00512.x
Schmidt-ullrich R., Paus R. (2005). Molecular principles of hair follicle induction and morphogenesis. BioEssays 27, 247–261. doi: 10.1002/(ISSN)1521-1878
Schuh C. M. A. P., Cuenca J., Alcayaga-miranda F., Khoury M.. (2019). Exosomes on the border of species and kingdom intercommunication. Trans. research: J. Lab. Clin. Med. 210, 80–98. doi: 10.1016/j.trsl.2019.03.008
Shan B., Yan M., Yang K., Lin W., Yan J., Wei S., et al. (2022). MiR-218-5p affects subcutaneous adipogenesis by targeting ACSL1, a novel candidate for pig fat deposition. Genes 13, 260. doi: 10.3390/genes13020260
Shen L., Ma J., Yang Y., Liao T., Wang J., Chen L., et al. (2023). Cooked pork-derived exosome nanovesicles mediate metabolic disorder-microRNA could be the culprit. J. nanobiotechnology 21, 83. doi: 10.1186/s12951-023-01837-y
Wang Y., Gao C., Gao T., Zhao L., Zhu S., Guo L. (2021). Plasma exosomes from depression ameliorate inflammation-induced depressive-like behaviors via sigma-1 receptor delivery. Brain behavior Immun. 94, 225–234. doi: 10.1016/j.bbi.2021.02.004
Wang J., Ma Y., Li T., Li J., Yang X., Hua G., et al. (2023). MiR-199a-3p regulates the PTPRF/β-catenin axis in hair follicle development: insights into the pathogenic mechanism of alopecia areata. Int. J. Mol. Sci. 24, 17632. doi: 10.3390/ijms242417632
Wei C., Sun Y., Zeng F., Chen X., Ma L., Liu X., et al. (2023). Exosomal miR-181d-5p derived from rapamycin-conditioned MDSC alleviated allograft rejection by targeting KLF6. Advanced Sci. (Weinheim Baden-Wurttemberg Germany) 10, e2304922. doi: 10.1002/advs.202304922
Zhang J., Liu Y., Chang J., Zhang R., Liu Z., Liang J., et al. (2024). Shh gene regulates the proliferation and apoptosis of dermal papilla cells to affect its differential expression in secondary hair follicle growth cycle of cashmere goats. Animals 14, 2049. doi: 10.3390/ani14142049
Keywords: Xinji fine-wool sheep, small-tailed Han sheep, exosomes, hair growth, miRNA
Citation: Zhang X, Wang W, Fan W, Zhai Y, Fu J, Cai J, Zhao D and Sun F (2025) Alteration of hair growth in mice by circulating exosomes from Xinji fine-wool and small-tailed Han sheep. Front. Anim. Sci. 6:1562392. doi: 10.3389/fanim.2025.1562392
Received: 17 January 2025; Accepted: 26 March 2025;
Published: 22 April 2025.
Edited by:
Seyed Abbas Rafat, University of Tabriz, IranReviewed by:
Hossein Mohammadi, Arak University, IranBenni Iskandar, Taipei Medical University, Taiwan
Copyright © 2025 Zhang, Wang, Fan, Zhai, Fu, Cai, Zhao and Sun. This is an open-access article distributed under the terms of the Creative Commons Attribution License (CC BY). The use, distribution or reproduction in other forums is permitted, provided the original author(s) and the copyright owner(s) are credited and that the original publication in this journal is cited, in accordance with accepted academic practice. No use, distribution or reproduction is permitted which does not comply with these terms.
*Correspondence: Dazhuo Zhao, emhhb2Rhemh1bzE5ODBAMTI2LmNvbQ==; Fuliang Sun, ZmxzdW5AeWJ1LmVkdS5jbg==
†These authors have contributed equally to this work