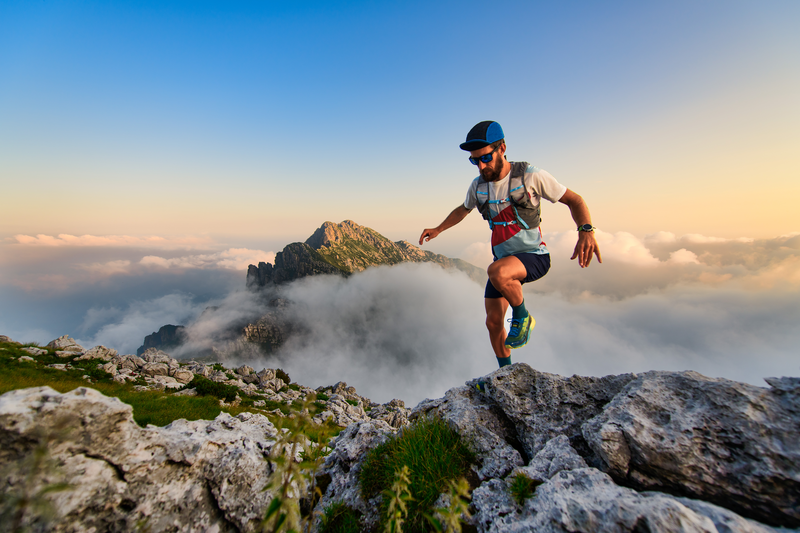
95% of researchers rate our articles as excellent or good
Learn more about the work of our research integrity team to safeguard the quality of each article we publish.
Find out more
ORIGINAL RESEARCH article
Front. Anim. Sci. , 20 March 2025
Sec. Animal Nutrition
Volume 6 - 2025 | https://doi.org/10.3389/fanim.2025.1521492
Introduction: African swine fever virus (ASFV) is extremely stable in the environment, and previous laboratory experiments and simulations have also shown it to be highly stable in animal feed ingredients. However, ASFV cannot be studied in real world demonstrations because it is a highly contagious virus. African swine fever virus is a member of the nucleocytoplasmic large DNA viruses (NCLDVs), and similar to Emiliania huxleyi virus (EhV), which has a restricted host range limited to a species of marine algae called Emiliania huxleyi. This algal NCLDV has many similar morphological and physical characteristics to ASFV, thereby making it a safe surrogate for generating experimental results that are applicable to ASFV and representative of real-world conditions.
Methods: We inoculated whole soybeans with EhV strain 86 (EhV-86) at a concentration of 1.80 × 108 virus/mL, which were then processed at a pilot solvent extraction facility to produce soybean hulls and meal. After processing, samples were evaluated for virus presence and viability using a previously validated viability qPCR (V-qPCR) method.
Results: No detection of EhV-86 occurred on environmental surfaces, air, and dust samples pre- or post-processing. Viable EhV-86 was detected in conditioned soybeans, dehulled soybeans, soybean hulls, soybean flakes, air-dried solvent extracted soybean flakes, post-desolventizer toaster soybean flakes, and soybean meal after reaching steady state during solvent extraction processing.
Discussion: It is important to note that 95% of viable virus was recovered (2.43 × 106 virus/g in replicate A and 2.61 × 106 virus/g in replicate B) in soybean meal, suggesting that longer retention times or application of chemical mitigants may be needed for more complete inactivation. The high concentration of viable viruses remaining on the soybean hulls after processing (1.98 × 107 virus/g in replicate A and 2.12 × 107 virus/g in replicate B) is a major concern for potential virus transmission in animal feed. These results demonstrate for the first time that ASFV-like NCLDVs can retain viability in soybean hulls, flakes, and meal during solvent extraction processing in a pilot facility and remain a hazard for virus transmission. Future risk assessments focused on the role of contaminated feed ingredients in transmission of viruses to swine farms must consider the ingredient composition of complete feeds delivered to farms and the initial concentration of viable viruses.
Foreign Animal Diseases, particularly African swine fever virus (ASFV), remain significant threats to livestock production in the United States and globally. These diseases are estimated to cause significant revenue losses to pork producers and to corn and soybean farmers (Carriquiry et al., 2020). The risk of disease introduction in the U.S. is increasing due to the expansion of ASFV outbreaks into large pork producing countries (i.e., China and Vietnam) and regions (i.e., Eastern Europe). These concerns have led to several risk assessments to identify the factors that can decrease the likelihood of ASFV introduction into the U.S (Schambow et al., 2022; USDA, 2023). and European Union (EFSA Panel on Animal Health and Welfare (AHAW) et al., 2021). These investigations suggest that feed ingredients and complete feed are potentially significant and overlooked routes of disease transmission. However, there is no surveillance or monitoring system to determine the prevalence and concentrations of swine virus contamination in global feed supply chains (Shurson et al., 2023). Soybean meal and other soybean products have been identified as potential feed vectors because research studies have shown that when swine viruses are experimentally inoculated in soybean meal, they survive for a longer period of time than in other plant-based feed ingredients (Trudeau et al., 2017; Niederwerder et al., 2022). Although several mitigation strategies, including extended storage time, some types of feed additives, and thermal and irradiation processing have been shown to provide partial inactivation of swine viruses, development and implementation of strict biosecurity protocols are essential to prevent virus contamination (Shurson et al., 2023).
The likelihood of ASFV contamination occurring in soybeans and soybean co-products is unknown. However, evidence from studies evaluating Salmonella spp. contamination in feed suggests that if a pathogen is prevalent in a country or region, feed ingredients produced in those countries or regions are likely to contain detectable levels of the pathogen (Wierup and Häggblom, 2010; Wierup and Widell, 2014). Soybean processing conditions, such as solvent extraction or extrusion which involve exposure to temperatures exceeding 100°C for over 20 min, are likely to inactivate ASFV, as has been demonstrated with Salmonella spp (Wierup and Widell, 2014). However, examination of the processing conditions along with the inherent variability (start-up time, applied temperatures, and residence times) and potential recontamination of soybean products after processing is needed to validate that these conditions can be effective for inactivating ASFV as shown for Salmonella spp (Morita et al., 2006). The Risk-Free in situ Non-Animal (RISNA) assay utilizes the Emiliania huxleyi (EhV) algal virus as a safe and effective surrogate for ASFV because it has similar structural and functional properties and does not infect humans, animals, or plants (Balestreri et al., 2024). Both ASFV and EhV are giant double-stranded DNA viruses and members of the nucleocytoplasmic large DNA viruses (NCLDVs) which have similar infection mechanisms. Recently, Balestreri et al. (2024) found that both ASFV and EhV can survive at temperatures up to 100°C for 20 min and follow similar inactivation kinetics when exposed to high temperatures. We hypothesize that the mechanical manipulation, exposure time at extremely high temperatures, and exposure to various solvents used during the processing of soybeans to make soybean meal will inactivate temperature-stable NCLDVs such as ASFV and EhV. The objectives of this study were to 1) determine the extent of ASFV inactivation under commercially representative pilot-scale solvent extraction procedures to produce soybean meal using the RISNA assay with the EhV surrogate and 2) determine the simulated environmental spread of ASFV in a solvent extraction pilot facility using the EhV surrogate.
A culture of Emiliania huxleyi CCMP374, courtesy of the Dr. Martinez-Martinez laboratory (Bigelow – Laboratory for Ocean Sciences, Maine) was grown in Alga-Gro® Seawater Medium (Carolina Biological Supplement Company, NC) at 15°C with 18h/6h light/dark cycle (ca. 2400 lux) until the concentration of 2 × 105 cells/mL was reached. Isolate EhV-86, also courtesy of the Dr. Martinez-Martinez laboratory, that was originally sourced from the Marine Biological Association of the United Kingdom, was added to E. huxleyi at a multiplicity of infection of 1 and grown in a 15°C incubator until lysis was observed, which usually occurs after 4 d (Schroeder et al., 2002). The lysate was filtered through a 0.45 µm filter (Nalgene™ Rapid-Flow™ Bottle Top Filters, ThermoFisher Scientific, MA) to remove cell debris. This filtration and infection procedure was repeated several times. The filtered lysate was divided into aliquots and kept in the dark at 4°C until use.
Approximately 60 L of 0.2 µm-filtered virus lysate was concentrated 10-fold to 6 L by tangential flow ultrafiltration using a 50,000 kDa molecular mass size cut-off (Vivaflow 50R, Sartorius, Germany) (Mackinder et al., 2009). Briefly, virus lysate was pumped through the system at a recirculation rate of ~200-400 mL/min at a pressure of ~2.5 bar, until the desired concentrate volume was achieved. Final virus concentration was calculated using analytical flow cytometry with SYBR Green 1 following the methods of Jacquet et al (Jacquet et al., 2002).
Raw soybeans were purchased and delivered to Crown Iron Works pilot facility (Blaine, MN) and subjected to a solvent extraction process to produce soybean meal. To ensure proper inoculation of EhV-86 to raw soybeans, a peristaltic pump was utilized to experimentally inoculate 907 kg of soybeans with approximately 2 L of EhV-86 (1.80 × 108 EhV/mL) at a rate of 27.5 mL/min after steady state had been reached. This was accomplished by setting the pump through the discharge screw of the feed hopper prior to the vertical seed conditioner. Soybeans inoculated with EhV-86 flowed through a typical solvent extraction pilot facility design as outlined in Figure 1. Temperature, pressure, and flow rate of soy products were measured at various timepoints throughout the process, following pilot facility standard operating procedures as outlined by Crown Iron Works (2021). The temperature of the whole bean discharge from the vertical seed conditioner was recorded hourly for the first hour, and then every 20 min thereafter for a total time of 2 hours. Discharge temperatures of the soybean meal and soybean hulls were recorded every 20 min during each one-hour aspiration run for a total time of two hours. The conditioner and flaker discharge temperatures were recorded every 20 min for the first hour, and then hourly until the end of the run for a total of four hours. The extractor stage temperatures were recorded every hour for five hours.
Figure 1. Process flow diagram. EhV-86 was added to whole soybeans at the beginning of the solvent extraction process. Experimentally inoculated soybeans flowed through the system as indicated with arrows to end points of soybean meal, soybean hulls, and soybean oil. Sample collection points shown as blue squares represent feed samples, green squares represent environmental samples, and orange squares represent air samples. Numbers correlate to locations listed in Tables 1, 2.
A total of 12 soy samples which included (1) whole soybeans without virus, (2) whole soybeans inoculated with virus, (3) conditioned soybeans prior to dehulling with virus, (4) dehulled soybeans with virus, (5) soybean hulls with virus, (6) soybean hulls without virus, (7) soybean flakes with virus, (8) air-dried solvent extracted flakes with virus, (9) post-desolventizer-toaster soy flakes with virus, (10) dehulled soybean meal with virus, (11) dehulled soybean meal without virus, and (12) soybean oil with virus were collected, in triplicate at 20 min (A) and 40 min (B) after steady state of processing was reached to provide two replicates or processing runs (Figure 1). Virus-free samples were kept in a separate sealed box until analysis to avoid cross-contamination. Technicians wore disposable gloves which were changed in between handling virus-free samples and inoculated samples.
Ten locations were identified prior to conducting the processing runs for environmental sampling that included collecting surface, air, and dust samples (Figure 1). These areas were classified as zone A (direct feed contact), zone B (surfaces immediately away from direct contact), and zone C (surfaces with non-feed contact) based on areas of open equipment and potential virus spread throughout the facility (Tables 1, 2).
Table 1. Location of environmental swabs collected by zone, with numbers corresponding to the locations shown in Figure 1.
Table 2. Location of air and dust samples collected by zone, with numbers corresponding to the locations shown in Figure 1.
Environmental samples were collected in triplicate for each location before and after processing of the experimentally inoculated soybeans by pre-wetting polyester tipped swabs with 1x phosphate buffered saline (1x PBS, ThermoFisher Scientific, MA) and swabbing over the specified surface. Swabs were then placed into sterile 15mL Falcon tubes (Corning™ Falcon 15mL Conical Centrifuge Tubes, ThermoFisher Scientific, MA) that were filled with 10 mL of Alga-Gro® Seawater Medium (Carolina Biological Supplement Company, NC) for virus elution from the swabs. Care was taken to keep pre-processing and post-processing samples separate during storage and prior to analysis by placing samples in separate sealed boxes and ensuring technician gloves were changed in between sample handling. One air or dust sample was collected at each location after the virus was added to the soybeans at 20 min, 40 min, and 24 hours by placing three open 15 mL Falcon tubes (Corning™ Falcon 15mL Conical Centrifuge Tubes, ThermoFisher Scientific, MA) that were filled with 10 mL of Alga-Gro® Seawater Medium (Carolina Biological Supplement Company, NC) in the specified location, closing the cap, and removing the tube after each timepoint. Samples were stored at -20°C until analysis.
All environmental and air samples were analyzed via standard DNA extraction and standard qPCR (S-qPCR). One hundred µL of each environmental surface, air, and dust sample was removed and used for DNA extraction (QIAamp® MinElute® Virus Spin, Qiagen, CA). The final elution volumes were 30 µL, and samples were stored at 4°C until qPCR analysis was conducted.
Standard and viability qPCR (V-qPCR) analyses were conducted for all environmental surface, air, dust, and soy samples using the methods optimized by Balesteri et al (Balestreri et al., 2024). All the soy samples were analyzed using V-qPCR. The viability (V) set of treatments consisted of one set of 100 µL virus eluants that contained PMAxx dye (Biotium Inc, CA, 25 µM final concentration) which was added by following methods optimized by Balestreri et al (Balestreri et al., 2024). An untreated duplicate set (i.e., no addition of PMAxx dye) of samples served as an untreated control template for S-qPCR. All samples were incubated in the dark at room temperature for 10 min on a rocker for optimal mixing. The treated V samples were then exposed to light for 30 min using a PMA-Lite device (Biotium Inc, CA) to cross-link PMAxx dye to the DNA (free or within broken viruses). The duplicate untreated control samples were kept in the dark at room temperature for the same length of time. All samples were then used for DNA extraction (QIAamp® MinElute® Virus Spin, Qiagen, CA). The final 30 µL elution volumes of samples were stored at 4°C until qPCR analysis was conducted.
Visualization of data was performed using the ggplot2 package of RStudio environment (Version 1.1.456, RStudio, Inc., Boston, MA) using R programming language [Version 4.0.5 (2021-03-31), R Core Team, R Foundation for Statistical Computing, Vienna, Austria]. Virus quantity averages (virus/g) were normally distributed and compared using a one-way ANOVA in RStudio. A Tukey multiple comparison adjustment was utilized in RStudio when appropriate. Results were considered significant at P ≤ 0.05 and trends between P > 0.05 and P ≤ 0.10.
The pilot solvent extraction equipment was operated under normal conditions with minimum, maximum, and average temperatures falling within the values described for the solvent extraction process (Table 3). Briefly, differences between this pilot facility and larger, commercial scale soybean processing facilities were that the pilot process involved recycling the soybean meats through the cracking and aspiration steps, and a longer residence time of 90 min was used compared to extractor residence times of 30 to 60 min in a commercial facility (Crown Iron Works, 2021). The equipment used in this process was operated until steady state was reached before the EhV-86 was added to the whole soybeans at the beginning of the process.
Table 3. Processing conditions of solvent extraction pilot plant for the minimum, maximum, and average temperatures (°C) of exposure for experimentally inoculated soybeans from the beginning (top of table) to the end of processing (bottom of table).
Prior to adding the virus to whole soybeans, the processing facility was swabbed in ten different locations in triplicate to verify that no initial EhV-86 was present on the equipment and in the facility (Tables 1, 2). The same locations were swabbed in triplicate the following morning after the experimentally inoculated soybeans were subjected to the first half of the solvent extraction process. Analysis of all the pre-processing and post-processing swabs and air samples resulted in negative PCR amplicons demonstrating that no virus was inadvertently contaminated or spread through the facility. These results contradict the findings of Elijah et al. (2021) indicating that environmental samples collected immediately after processing a batch of feed that was contaminated with ASFV contained high concentrations of ASFV, but processing subsequent batches of feed resulted in significantly lower concentrations of ASFV in the environment. A possible explanation for these conflicting results may be due to differences in the types of equipment, processes, and cleaning protocols used in commercial feed mills compared with those used in a soybean processing facility. For example, components of soybean processing equipment are enclosed, which leads to minimal dust in the environment, whereas a feed mill has various types of equipment such as hammermills, roller mills, and mixers that create dust leading to environmental surface and air contamination. These results suggest that although the potential for cross-contamination of ASFV may be reduced in a solvent extraction soybean processing facility compared with a commercial feed mill, biosecurity protocols should include practices that minimize the potential spread of virus by air and dust.
In this study, the survival and viability of the giant algal virus EhV-86, a surrogate for ASFV, were evaluated in whole soybeans that underwent solvent extraction processing in a pilot facility. Overall, viral concentration (viruses/g) in samples was successfully quantified using both the S-qPCR and a novel V-qPCR assay. The virus was added to whole soybeans at a constant rate from the feeder to the vertical seed conditioner. When whole soybeans were experimentally inoculated with the virus at the beginning of the solvent extraction process, viable EhV-86 was detected via V-qPCR analysis in both replicates. Previous research has shown EhV-86 to be stable up to 100°C and that feed matrices, particularly soybeans, provide viral protection from heat (Balestreri et al., 2024).
In virus-inoculated feed samples from the first phase of processing (whole soybeans, conditioned soybeans, dehulled soybeans, soybean hulls and soybean flakes), an average range of 2.32 × 107 EhV/g to 9.27 × 107 EhV/g was observed by using S-qPCR in replicate A, and an average range of 3.26 × 107 EhV/g to 1.19 × 107 EhV/g was observed in replicate B (Figure 2, Table 4). This represents a 0.37-log increase in recovery in replicate A and a 0.26-log increase in replicate B. During this time the soy samples were exposed to temperatures that ranged from 31 to 73°C. Using V-qPCR, an average range of 1.98 × 107 EhV/g to 8.07 × 107 EhV/g of viable virus was observed in replicate A and an average range of 2.12 × 107 EhV/g to 8.07 × 107 EhV/g of viable virus was determined in replicate B (Figure 2, Table 4). The amount of viable virus recovered during the preparation phases of processing represented a 1.42 log increase in recovery (replicate A), and a 1.27 log increase in recovery (replicate B) in viable virus quantity from whole soybeans to soybean flakes. Replicates A and B tended to have greater (P = 0.07) average viable virus quantity for soybean flakes than whole soybeans. This may be a result of increasing virus release from the soy material throughout the process resulting in more virus being detected in samples downstream.
Figure 2. Boxplot showing the interquartile range of EhV-86 quantity (virus/g) in various soy samples throughout the solvent extraction process determined by S-qPCR (A) and V-qPCR (B). The light-colored boxes represent replicate A while the adjacent darker colored boxes represent replicate (B) The line extending from the top and the bottom of the boxes are the upper (maximum) and lower (minimum) limits, respectively. The middle line in the box is the median. The upper box is Q3, the upper quartile or 75th percentile. The lower box is Q1, the lower quartile or 25th percentile.
Table 4. Average virus quantity (virus/g) for both standard and viable qPCR analysis for all collected samples. .
Samples inoculated during the preparation phase of processing had minimal differences between the amount of viral DNA detected using standard and viable qPCR methods. This indicates that mega viruses, such as EhV and potentially ASFV, remain viable after exposure to temperatures up to 73°C in a real-world demonstration, confirming the laboratory-based data reported by Balesteri et al (Balestreri et al., 2024). These findings contribute to the speculation that feed matrices may have viral protective properties because in this study, stock viruses had similar quantities of viable virus compared with the quantity of viruses eluted off soy samples after exposure to temperatures up to 60°C during soybean processing.
The solvent extraction steps during the last half of processing, which included samples of air-dried solvent extracted soy flakes, post-desolventizer-toaster soy flakes, and dehulled soybean meal, resulted in S-qPCR values ranging from 2.40 × 106 EhV/g to 7.17 × 106 EhV/g in replicate A, and an average range of 2.99 × 106 EhV/g to 5.19 × 106 EhV/g in replicate B (Figure 2, Table 4). Viable viruses during the solvent extraction phase, as detected by V-qPCR, ranged from an average of 9.01 × 105 EhV/g to 2.43 × 106 EhV/g viable virus in replicate A, and an average range of 1.54 × 106 EhV/g to 2.61 × 106 EhV/g viable virus in replicate B. In the second half of processing, the average virus quantity for dehulled soybeans tended to be greater (P = 0.10) than post-desolventizer-toaster flakes and dehulled soybean meal in both replicates A and B. In both replicates, the average viable viral quantity recovered from soybean flakes tended to be greater (P = 0.06) than average viable viral quantity recovered from air-dried solvent extracted soy flakes, post-desolventizer-toaster soy flakes, and dehulled soybean meal.
The recovery in quantity of EhV was determined from the concentration in inoculated soybean flakes, representing the end of the preparation phase, and the concentration in the air-dried solvent extracted flakes at the beginning of the solvent extraction phase, which resulted in a 3.19-log reduction in replicate A and a 3.17-log reduction in replicate B in average virus quantity, and a 1.90-log reduction in viable viruses for replicate A and a 1.77-log reduction in viruses in replicate B. During this phase of processing the virus-inoculated soy was exposed to a temperature range of 31°C to 73°C and was also exposed to hexane.
When comparing the EhV from air-dried solvent extracted flakes to the final product of soybean meal, there was a 3.32-log reduction in average virus quantity observed in replicate A and 1.41-log reduction in average virus quantity in replicate B, and a 1.49-log reduction in viable viruses in replicate A and a 1.74-log reduction in viable viruses in replicate B. These reductions in recovered viral quantity in the final product of soybean meal likely occurred as a result of exposure to a wide temperature range of 31-115°C during an estimated total time of 160 minutes (Schambow et al., 2022).
During the second half of the process, air-dried solvent extracted flakes with EhV were exposed to a maximum temperature of 115°C. Data from a previous study showed viral degradation occurs at this high temperature (Balestreri et al., 2024). In addition, virus-inoculated soy samples were exposed to hexane during this processing phase, which resulted in the observed 3.32-log reduction in total viral DNA and a 1.49-log reduction in viable viruses in replicate A, and 1.41-log reduction in total viral DNA and a 1.74-log reduction in viable viruses in replicate B. This implies that the hexane and the extreme heat exposure during the drying and toasting process led to the observed log reductions of 1.41 and 1.74 of viable viruses. A 1-log reduction is only 90% inactivation, which means that in this study about 90% inactivation of EhV-86 occurred in soybean meal, yet we were still able to recover viable virus. Because these soy samples were not evaluated in a bioassay, it is unknown if the viable viruses recovered would be capable of infecting a host when consuming these ingredients as part of a complete feed.
Additionally, no viral DNA was detected in soybean oil in both replicates, which is a common by-product in solvent extraction soybean processing, resulting in a log reduction of 7.76 (S-qPCR, replicate A and B) and 7.83 (V-qPCR, replicate A and B), which exceeds the current industry standard for complete activation, which is a 4-log reduction in viral concentration. However, DNA extraction methodologies from soybean oil may need to be further evaluated and optimized because vegetable oils, due to refinement and processing methods, pose difficulties in obtaining amplifiable DNA (Gryson et al., 2002; Costa et al., 2012; Muzzalupo et al., 2015; Bojang et al., 2021). Consequently, additional experimentation is required to confirm the absence of viable viruses in soybean oil. Nonetheless, if we assume our results are valid, soybean oil may not be a by-product of concern for viral contamination. According to the results of this study, soybean meal, as the primary protein source in swine diets, could pose a risk depending on the initial level of virus contamination.
Soybean hulls, another common by-product of solvent extraction soybean processing, also remain a great concern because hulls are not exposed to temperatures greater than 60°C, nor are they exposed to hexane or any type of solvent. Consistently high virus quantities were observed in the soybean hulls in this study, which is important to note because soybean hulls are routinely used as animal feed ingredients, typically in ruminant feeds, but can also be included in sow gestation diets as a fiber source. Therefore, these data indicate that because soybean hulls do not undergo full processing, soybean hulls represent a potentially high-risk feed ingredient for virus transmission if they are contaminated with ASFV.
All of this information shows that while results from previous studies have shown that EhV-86 and ASFV can be partially inactivated at high temperatures in a laboratory setting, the complexity and physiochemical properties of feed matrices, such as soybean products, may contribute to virus survival during solvent extraction processing even though a 1.49 and 1.74-log reduction in viable virus concentration in soybean meal was observed after exposure to hexane and the high temperatures reached in the desolventizer and toaster. The results of this study demonstrate that EhV-86 can remain viable during solvent extraction processing at steady state even though greater than 90% inactivation in soybean meal does occur. These results support the hypothesis that solvent extraction processing of soybeans may partially inactivate ASFV. Because EhV-86 can be used as a surrogate for ASFV, enabling the ability to make direct comparisons, we also conclude that ASFV would survive and be potentially viable in soybean meal depending on the level of initial contamination.
In conclusion, the NCLDV EhV-86, when used as a surrogate for ASFV, can be detected in viable form when collected from experimentally inoculated whole soybeans that are then exposed to high temperatures throughout solvent extraction processing with an overall 1.47-log reduction in viable virus quantity present in the final product of soybean meal. It is important to note that only an estimated 96.61% of viable viruses were inactivated, suggesting that longer retention times or application of chemical mitigants may be needed. A major concern remains the amount of viable virus on the soybean hulls after processing. Soybean hulls are never exposed to solvent or temperatures greater than 60°C, which poses a potential virus transmission threat because they are marketed separately and can be added back into sow gestation diets. Although stable and viable virus was detected in soy samples, it remains unknown if this amount of viable virus is still infectious. A bioassay will need to be conducted in future studies to determine infectivity. Furthermore, sampling sensitivity may explain some of the variation seen between the various soy samples and different biological replicates. Overall, environmental and air and dust sampling did not result in positive samples by PCR analysis. These results demonstrate for the first time that NCLDVs, such as EhV-86 and likely ASFV, can survive and remain viable in soybean hulls and soybean meal after exposure to high temperatures during the solvent extraction process, suggesting that these feed ingredients may remain a hazard for viral transmission. However, the risk of contaminated soybean meal causing infection depends on the initial concentration of virus contamination. Therefore, the feed industry still needs to use caution when processing feed ingredients that may be contaminated with ASFV and evaluate the effectiveness of other mitigation strategies to further inactivate ASFV if it is present. Likewise, the soy crushing industry should evaluate the effectiveness of using longer retention times during the solvent extraction process for inactivating ASFV. The feed and swine industries also need to be aware that buying soybean hulls or soybean meal from ASFV-positive countries still poses a hazard, and biosecurity protocols and effective mitigation strategies at the US borders need to be implemented. Given the similarities of EhV-86 to ASFV, especially in the presence of feed matrices, the EhV-86 surrogate can be used to validate and test potential ASFV inactivation strategies (Schroeder et al., 2002; Palowski et al., 2022; Balestreri et al., 2024).
The original contributions presented in the study are included in the article/supplementary material. Further inquiries can be directed to the corresponding author.
AP: Data curation, Formal Analysis, Investigation, Writing – original draft, Writing – review & editing. CB: Methodology, Supervision, Writing – review & editing. PU: Conceptualization, Writing – review & editing. Jv: Conceptualization, Writing – review & editing. RO: Data curation, Investigation, Resources, Writing – review & editing. GS: Conceptualization, Funding acquisition, Writing – review & editing. DS: Conceptualization, Formal Analysis, Funding acquisition, Methodology, Project administration, Resources, Supervision, Writing – review & editing.
The author(s) declare that financial support was received for the research and/or publication of this article. This research was funded by United Soybean Board. The funder had no role in the design of analysis of the data.
The authors would like to thank Matthew Koppang, Rahul Seshappa, Deedra Diede, Richard Ozar and the technicians at Crown Iron Works for their hospitality, assistance, and allowing us to use the pilot solvent extraction facility.
Author RO was employed by the company Crown Iron Works.
The remaining authors declare that the research was conducted in the absence of any commercial or financial relationships that could be construed as a potential conflict of interest.
The author(s) declare that no Generative AI was used in the creation of this manuscript.
All claims expressed in this article are solely those of the authors and do not necessarily represent those of their affiliated organizations, or those of the publisher, the editors and the reviewers. Any product that may be evaluated in this article, or claim that may be made by its manufacturer, is not guaranteed or endorsed by the publisher.
Balestreri C., Schroeder D. C., Sampedro F., Marqués G., Palowski A., Urriola P. E., et al. (2024). Unexpected thermal stability of two enveloped megaviruses, Emiliania huxleyi virus and African swine fever virus, as measured by viability PCR. Virol. J. 21, 1. doi: 10.1186/s12985-023-02272-z
Bojang K. P., Kuna A., Pushpavalli S.N.C.V.L., Sarkar S., Sreedhar M. (2021). Evaluation of DNA extraction methods for molecular traceability in cold pressed, solvent extracted and refined groundnut oils. J. Food Sci. Technol. 58, 3561–3567. doi: 10.1007/s13197-021-05079-4
Carriquiry M., Elobeid A., Swenson D., Hayes D. (2020).Impacts of African swine fever in iowa and the U.S. CARD working papers. Available online at: https://lib.dr.iastate.edu/card_workingpapers/618/ (Accessed September 26, 2024).
Costa J., Mafra I., Oliveira M. B. P. P. (2012). Advances in vegetable oil authentication by DNA-based markers. Trends Food Sci. Technol. 26, 43–55. doi: 10.1016/j.tifs.2012.01.009
Crown Iron Works (2021). Crown iron works company innovation center test report - processing of whole soybeans to defatted meal with surrogate ASFV algal virus.
EFSA Panel on Animal Health and Welfare (AHAW), Nielsen S. S., Alvarez J., Bicout D. J., Calistri P., Canali E., et al. (2021). Ability of different matrices to transmit African swine fever virus. EFSA J. 19, e06558. doi: 10.2903/j.efsa.2021.6558
Elijah C. G., Trujillo J. D., Jones C. K., Gaudreault N. N., Stark C. R., Cool K. R., et al. (2021). Evaluating the distribution of African swine fever virus within a feed mill environment following manufacture of inoculated feed. PloS One 16, e0256138. doi: 10.1371/journal.pone.0256138
Gryson N., Ronsse F., Messens K. (2002). Detection of DNA during the refining of soybean oil. J. Am. Oil Chemists’ Soc. 79, 171–174. doi: 10.1007/s11746-002-0453-2
Jacquet S., Heldal M., Iglesias-Rodriguez D., Larsen A., Wilson W., Bratbak G. (2002). Flow cytometric analysis of an Emiliana huxleyi bloom terminated by viral infection. Aquat. Microbial Ecol. 27, 111–124. doi: 10.3354/AME027111
Mackinder L. C. M., Worthy C. A., Biggi G., Hall M., Ryan K. P., Varsani A., et al. (2009). A unicellular algal virus, Emiliania huxleyi virus 86, exploits an animal-like infection strategy. J. Gen. Virol. 90, 2306–2316. doi: 10.1099/vir.0.011635-0
Morita T., Kitazawa H., Iida T., Kamata S. (2006). Prevention of Salmonella cross-contamination in an oilmeal manufacturing plant. J. Appl. Microbiol. 101, 464–473. doi: 10.1111/j.1365-2672.2006.02972.x
Muzzalupo I., Pisani F., Greco F. (2015). Direct DNA amplification from virgin olive oil for traceability and authenticity. Eur. Food Res. Technol. 241, 151–155. doi: 10.1007/s00217-015-2455-5
Niederwerder M. C., Khanal P., Foland T., Constance L. A., Stoian A. M. M., Deavours A., et al. (2022). Stability of African swine fever virus in feed during environmental storage. Transboundary Emerging Dis. 69, 3216–3224. doi: 10.1111/tbed.14666
Palowski A., Balestreri C., Urriola P. E., van de Ligt J. L., Sampedro F., Dee S., et al. (2022). Survival of a surrogate African swine fever virus-like algal virus in feed matrices using a 23-day commercial United States truck transport model. Front. Microbiol. 13. doi: 10.3389/fmicb.2022.1059118
Schambow R. A., Sampedro F., Urriola P. E., van de Ligt J. L. G., Perez A., Shurson G. C. (2022). Rethinking the uncertainty of African swine fever virus contamination in feed ingredients and risk of introduction into the United States. Transboundary Emerging Dis. 69, 157–175. doi: 10.1111/tbed.14358
Schroeder D. C., Oke J., Malin G., Wilson W. H. (2002). Coccolithovirus (Phycodnaviridae): Characterisation of a new large dsDNA algal virus that infects Emiliania huxleyi. Arch. Virol. 147, 1685–1698. doi: 10.1007/s00705-002-0841-3
Shurson G. C., Urriola P. E., Schroeder D. C. (2023). Biosecurity and mitigation strategies to control swine viruses in feed ingredients and complete feeds. Animals 13, 2375. doi: 10.3390/ani13142375
Trudeau M. P., Verma H., Urriola P. E., Sampedro F., Shurson G. C., Goyal S. M. (2017). Survival of porcine epidemic diarrhea virus (PEDV) in thermally treated feed ingredients and on surfaces. Porcine Health Manage. 3, 1–7. doi: 10.1186/s40813-017-0064-3
USDA (2023).African swine fever response - outbreak in domestic pigs: incident playbook, foreign animal disease preparedness & Response plan. Available online at: https://www.aphis.usda.gov/sites/default/files/asf-domestic-pig-playbook.pdf (Accessed September 26, 2024).
Wierup M., Häggblom P. (2010). An assessment of soybeans and other vegetable proteins as source of salmonella contamination in pig production. Acta Veterinaria Scandinavica 52, 1–9. doi: 10.1186/1751-0147-52-15
Keywords: African swine fever virus, Emiliania huxleyi virus, NCLDVs, soybeans, viability PCR
Citation: Palowski A, Balestreri C, Urriola PE, van de Ligt JLG, Ozer R, Shurson GC and Schroeder DC (2025) Evaluating the inactivation of a surrogate ASFV-like algal virus in a pilot solvent extraction soybean processing facility. Front. Anim. Sci. 6:1521492. doi: 10.3389/fanim.2025.1521492
Received: 01 November 2024; Accepted: 04 March 2025;
Published: 20 March 2025.
Edited by:
Michael D. Flythe, United States Department of Agriculture, United StatesReviewed by:
Ravikanthreddy Poonooru, University of Missouri, United StatesCopyright © 2025 Palowski, Balestreri, Urriola, van de Ligt, Ozer, Shurson and Schroeder. This is an open-access article distributed under the terms of the Creative Commons Attribution License (CC BY). The use, distribution or reproduction in other forums is permitted, provided the original author(s) and the copyright owner(s) are credited and that the original publication in this journal is cited, in accordance with accepted academic practice. No use, distribution or reproduction is permitted which does not comply with these terms.
*Correspondence: Declan C. Schroeder, ZGNzY2hyb2VAdW1uLmVkdQ==
Disclaimer: All claims expressed in this article are solely those of the authors and do not necessarily represent those of their affiliated organizations, or those of the publisher, the editors and the reviewers. Any product that may be evaluated in this article or claim that may be made by its manufacturer is not guaranteed or endorsed by the publisher.
Research integrity at Frontiers
Learn more about the work of our research integrity team to safeguard the quality of each article we publish.