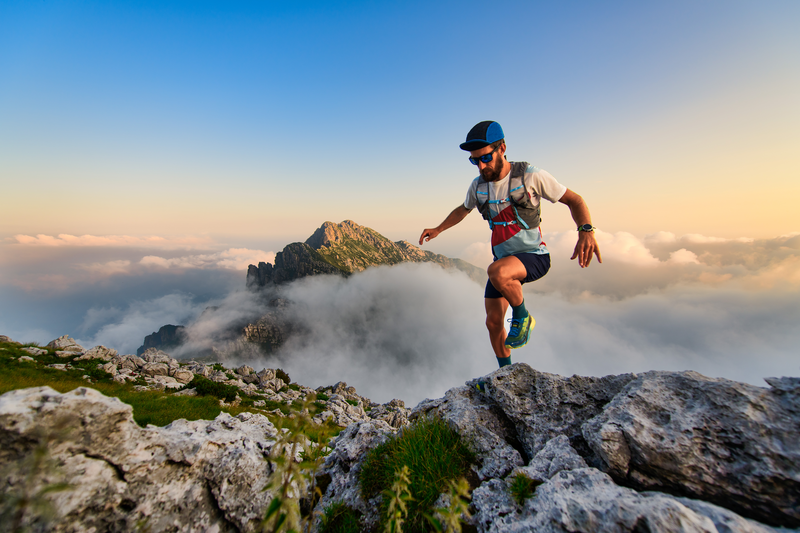
94% of researchers rate our articles as excellent or good
Learn more about the work of our research integrity team to safeguard the quality of each article we publish.
Find out more
ORIGINAL RESEARCH article
Front. Anim. Sci.
Sec. Animal Nutrition
Volume 6 - 2025 | doi: 10.3389/fanim.2025.1507440
The final, formatted version of the article will be published soon.
You have multiple emails registered with Frontiers:
Please enter your email address:
If you already have an account, please login
You don't have a Frontiers account ? You can register here
Herein, 364 one-day-old male Jiangnan white goslings were divided into four groups: PM (18.55% crude protein (CP) + main amino acids (AA)), PA (18.55% CP + all AA), LPM (15.55% CP + main AA), and LPA (15.55% CP + all AA). The main AAs were Lys, Met, Thr, Trp, Arg, and Cys, while all AAs included an additional six (Met, Lys, Arg, His, Ile, Leu, Cys, Phe, Tyr, Thr, Trp, and Val). At 28 days, all geese were switched to a 15.55% CP + main AA diet until 63 days. The results showed: (1) No significant impacts on average daily gain or feed-to-gain ratio at 29-63 days. Although numerical differences in average daily feed intake (ADFI) were observed between groups (Table 3), statistical analysis indicated that the reduction in ADFI due to early low protein was marginal (P = 0.06). This suggests that the dietary protein level may influence feed intake patterns in goslings, but further studies with larger sample sizes are needed to confirm this effect. (2) Early low protein significantly affected abdominal fat percentage and leg muscle cooking loss at 63 days (P < 0.05).Pretreatment affected breast muscle rate at 63 days (P < 0.05). (3) Early low protein significantly increased Cys content in breast muscle at 63 days and increased Asp, Thr, Cys, and His in feces at 62-64 days (P < 0.05). ( 4) Early low protein led to a significant reduction in nitrogen excretion and an increase in nitrogen utilization (NU) in feces at 62-64 days (P < 0.05). Low-protein diets reduced nitrogen excretion by 12.3% (1.60 vs. 1.78 g/bird, P < 0.05) and improved nitrogen utilization from 56.03% to 59.48%. Leg muscle cooking loss decreased by 15.2% in the LPA group (15.01%) compared to PA (19.58%, P < 0.05). To conclude, different AA supplementation patterns with low protein (15.55%) in the early stage (1-28 days) had no significant effects on body weight, slaughter performance, and meat quality at 63 days. However, early low protein significantly reduced nitrogen excretion and improved NU, suggesting it is feasible for meat goslings to adopt a low-protein diet supplemented.
Keywords: Low-protein diet, Amino acid supplementation pattern, growth performance, nitrogen metabolism, goose
Received: 08 Oct 2024; Accepted: 26 Feb 2025.
Copyright: © 2025 li, zhao, Zhang, sun, zhao, zhang, ji, wang, Li and chen. This is an open-access article distributed under the terms of the Creative Commons Attribution License (CC BY). The use, distribution or reproduction in other forums is permitted, provided the original author(s) or licensor are credited and that the original publication in this journal is cited, in accordance with accepted academic practice. No use, distribution or reproduction is permitted which does not comply with these terms.
* Correspondence:
Xiaoming Li, Gansu Agricultural University, Lanzhou, China
guoshun chen, Gansu Agricultural University, Lanzhou, China
Disclaimer: All claims expressed in this article are solely those of the authors and do not necessarily represent those of their affiliated organizations, or those of the publisher, the editors and the reviewers. Any product that may be evaluated in this article or claim that may be made by its manufacturer is not guaranteed or endorsed by the publisher.
Research integrity at Frontiers
Learn more about the work of our research integrity team to safeguard the quality of each article we publish.