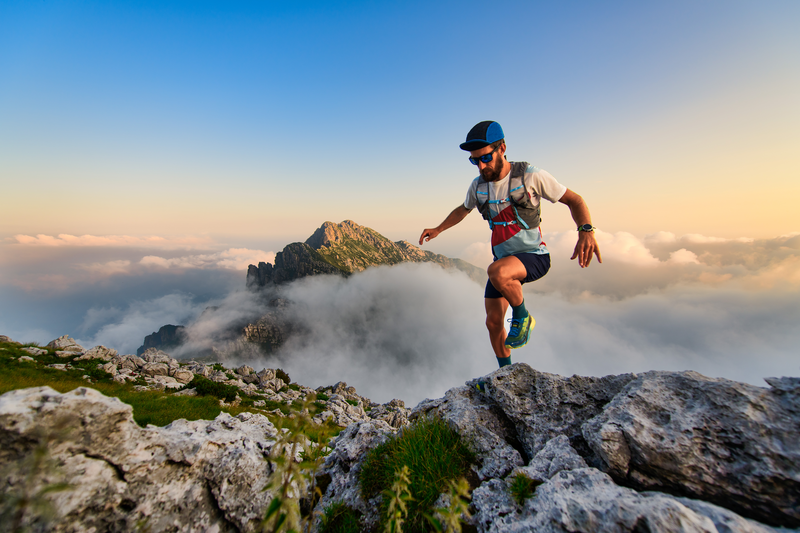
95% of researchers rate our articles as excellent or good
Learn more about the work of our research integrity team to safeguard the quality of each article we publish.
Find out more
REVIEW article
Front. Anim. Sci. , 27 March 2025
Sec. Animal Nutrition
Volume 6 - 2025 | https://doi.org/10.3389/fanim.2025.1501412
This article is part of the Research Topic Interventions for Improving Livestock Productivity in Developing Countries View all 14 articles
The long-term survival of livestock production systems depends critically on the sustainable usage of resource-efficient strategies, such as the supplementation or replacement of conventional feed ingredients (CFI) like maize and soybean with less expensive, more effective, and locally accessible sources. Wild fruits and their byproducts (WFBP) from multipurpose trees (e.g. leaves, seeds) offer a promising low-cost non-traditional alternative dietary source of energy, protein, vitamins, minerals, oxycaretenoids, and bioactive substances that function at a cellular level in livestock. According to research these non-CFI can enhance livestock voluntary intake, digestibility, and overall performance while alleviating the heavy reliance on CFI. Conversely, the use of these alternative feed resources (AFR) necessitates a thorough evaluation of their nutrient composition, antinutritional factors (ANF), and proper inclusion levels. At low appropriate inclusion levels in livestock rations, the availability of optimal crude energy, crude protein content, and availability of necessary amino acids (AA) in these AFR, can be taken advantage of in saving costs and reducing demands for soybean and maize, which are the indisputable CFI for both livestock and man. This review synthesizes the current research status of WFBP looking at their nutritional profiles (energy, protein, minerals, fiber etc.) and their impacts in livestock productivity. Furthermore, this review will address potential processing techniques to lessen their drawbacks like ANF or palatability. By highlighting these issues, this paper seeks to provide a guide for the sustainable incorporation of these AFR into livestock diets, striking a balance between nutritional effectiveness and economic feasibility.
● Global livestock production is facing one major challenge of ensuring adequate availability of nutritious feed.
● Due to the growing demand for meat and animal products, there is a need to design new alternative efficient feed systems.
● This review article details the possible use of wild fruits and their respective tree byproducts (WFBP) as partial or supplement stockfeed ingredients.
● The knowledge base of these potential alternative feedstuffs and their limitations are highlighted in this review.
Wild fruits (WF) are a vital source of human and animal nutrition, vital for preserving and maintaining good health (Wadhwa et al., 2013; Chisoro et al., 2018). Additionally, they also contribute to the ecosystem in many areas by giving a range of wildlife species food and a habitant to live (Hassan et al., 2011). Lately, in animal nutrition, there has been a growing interest in the sustainable utilization of non-human edible WF trees output and their respective byproducts (WFBP) in livestock feed (Chisoro et al., 2018; Gürbüz and Özkan, 2018). Finding sustainable and affordable means or methods of feeding livestock has become increasingly crucial due to the rising demand for animal produce, especially in developing countries (FAO, 2011; World Economic Forum, 2019; Jalal et al., 2023). This is being exacerbated by the rising global population and the need for climate smart agriculture. WFBP can improve feed accessibility and sustainability while lowering dependency on resource-intensive feeds (like, soybean and maize) when used in animal feed (Paul, 2019; Chisoro and Nkukwana, 2020a; Balehegn et al., 2021; Msweli, 2021).
Furthermore, this lowers production costs and boost profitability, while helping farmers sustainability and promotion of the advancement of a more circular livestock-economy (Balehegn et al., 2021). Despite the potential benefits of the utilization of WFBP in livestock rations, there are practical considerations that need to be considered. In addition, the availability of WFBP can vary depending on season and location, which can create challenges for farmers trying to incorporate these materials in feed (Nkukwana, 2019; Luciano et al., 2020). Additionally, the appropriate processing techniques on WFBP need to be considered to reduce their non-nutritive components, especially ANF, mycotoxins, pathogens, toxins and a solid understanding of the proper inclusion levels in livestock diets. Even so, the crude protein (CP) content, lysine value, and essential sulfur-containing Amino Acids (AA) (cysteine and methionine) content of these alternative feed resources (AFR) needs to be considered. For their appropriate inclusion in livestock feed formulations at their modest inclusion levels for ideal livestock productivity (Rijsberman and Frank, 2016; Chakraborty and Gupta, 2017; Bhatti et al., 2018).
This review is going to discuss the various kinds and categories of WFBP that can be employed in livestock diets. Additionally, it is going to highlight their nutritional worth, potential advantages on animal performance and health, and the practical aspects of including these AFR in livestock diets. By highlighting the potential of WFBP as AFR, the goal is to add to the body of knowledge already available on sustainable livestock production systems. While giving a thorough evidence-based overview of the present state of research on WFBP, thereby supporting the notion on the sustainable use of these resources in livestock nutrition.
Globally, the livestock industry is very dynamic and is changing rapidly in developing nations due to the rising demand for animal products (World Economic Forum, 2019). While many production processes are becoming more efficient and environmentally sustainable, the market for livestock products is mostly stagnating in developed countries due to changes in dietary preferences and societal concerns. The demand for livestock products has historically changed due to changes in human population, income, and urbanization. This production response in various livestock systems has been correlated with advances in science, technology and increase in the number of animals (FAO, 2011). Competition for natural resources, notably land and water, competition between food and feed, and the requirement to function in a carbon-constrained economy are currently significantly influencing agriculture and will continue to have an increasing impact on the productivity of livestock (ILRI, 2012; UN, 2014; Stødkilde et al., 2023).
Considering that soybean meal (SBM) and maize are the most widely used food and feed ingredients, which are unsustainable, plant-based protein and energy sources for livestock nutrition, while also being the backbone of most worldwide production systems. Conventional protein crop production, soy production in particular, is closely associated with detrimental effects on the environment, such as deforestation and land degradation, the depletion of fossil fuels, atmospheric pollution, global warming, acidification and eutrophication, and harm to biodiversity (Chisoro et al., 2019; Pexas et al., 2023). Furthermore, significant financial investments are needed for the large-scale cultivation of protein crops, mostly related to labor, fossil fuels, land (such as rent), and synthetic and chemical inputs (such as fertilizers, insecticides, and herbicides) (Pexas et al., 2023).
Long market chains that employ at least 1.3 billion people worldwide and directly assist approximately 600 million poor smallholder farmers in developing countries are continuously being established in the livestock sector (Sattar et al., 2021). This sector is a crucial risk reduction approach for communities that are at risk, since livestock are crucial nutrient and traction sources for smallholder systems’ crop production. Worldwide, the consumption of livestock products makes up 17% of kilocalories consumed and 33% of the protein consumed. As shown in Figure 1, the production for beef, pork and chicken continued to increase from 2020 to 2023 despite the disruptions caused by the 2020 Covid-19 pandemic, the Russo-Ukraine war which had significant influences on global grain and soybean supply, and the 2022 global oil price fluctuations which had an impact on goods transportation and agriculture productivity (Glufke et al., 2020; Pexas et al., 2023; Rauw et al., 2023). However, there continue to be significant disparities between rich and poor countries (Thornton, 2014; Paul, 2019; Lukuyu et al., 2020).
Figure 1. Global meat production trends from 2020 to 2023. Beef and pork are in 1 000 metric tons (Carcass weight equivalent) and chicken is in 1 000 metric tons (Ready to cook equivalent) source (USDA, 2024a), livestock and poultry: world markets and trade. As you can see there has been a steady increase in pork production with a rather constant production of chicken and beef since 2020.
This sector is expanding fast in emerging economies, and it accounts for about 33% of their agricultural GDP (Pexas et al., 2023). As shown in Figure 2, the estimated total consumption of meat in 2050 across the world in relation to 2019 consumption levels tends to imply that meat consumption will continue to increase globally. With pronounced increases in consumption being in East Asia, Latin America and Sub-Saharan Africa but with rather steady increases in Europe and North America according to the Falcon et al. (2022) report. Given that most demand is currently satisfied by domestic production and is expected to do so for the foreseeable future, the combination of the rising demand in developing nations and the rather stagnant demand in industrialized developed nations represents a significant opportunity for livestock farmers in developing nations (Thornton, 2014; UN, 2014; Balehegn et al., 2021).
Figure 2. The graph shows the estimated total consumption of meat in 2050 across the world in relation to 2019 consumption levels. From this it implies that meat consumption will continue to increase in the coming years globally. Pronounced increases in consumption will be in East Asia, Latin America and Sub-Saharan Africa. Data sourced from the Falcon et al. (2022) report “Rethinking Global Food Demand for 2050”.
In sub-Saharan Africa and South Asia, the food demand for animal products will almost quadruple, rising from around 200 kcal per person per day in 2000 to over 400 kcal per person per day in 2050 (Figure 2). From the early 1960s to the mid-2000s, livestock carcass weights increased by roughly 30% for chicken and beef cattle and about 20% for pigs (FAO, 2011; Donkor et al., 2021). For cows, gains in milk production per animal have been around 30%, roughly equal to increases in chicken egg production per animal over the same period (UN, 2014; Pexas et al., 2023). Large-scale forest conversions have taken place over the past 20 years in the Amazon Basin, Southeast Asia, and Central and West Africa. While the amount of forest land has increased because of the abandonment of agricultural land in the Eurasian boreal forest and other regions of Asia, North America, Latin America, and the Caribbean (FAO, 2011; ILRI, 2012). This presents an opportunity for the use of innovative methods or alternative feedstuffs to CFI in stockfeed to promote sustainable livestock production, in a way to avoid the continued forest conversions that are taking place in Latin America and Africa. Given the continued production of intensive CFI, particularly maize and soybean, which is highly unsustainable (Figures 3, 4). For sustainable livestock production and be in line with the UN sustainable Development Goals (SDGs) in mitigating climate change and greenhouse gases (GHG) (OECD/FAO, 2024).
Figure 3. Global production trends of the commonly used grain CFI in animal and human nutrition as sourced from the (USDA, 2024b) report. Here we can see that the output of these resources was rather similar across 2019 to 2023. Implying that if these trends continue in the future there will be a great need for the use of alternative or supplementary feed ingredients to complement the demand and supply for these cereal ingredients in animal feed and for human consumption.
Figure 4. World production trends of the commonly used oilseeds CFI for stockfeed as sourced from the (USDA, 2024c) report. The trends here are like those noted on cereal CFIs, however, soybean showed significant increases in production especially in the 2022/23 and 2023/24 production seasons. As it is the most indisputable protein oilseed for animal and human nutrition, and it is a crop that is given the most significant attention by producers and governments globally.
Conventional ingredients the likes of cereal grains (maize, wheat, barley, etc.), oilseed meals (soybean, rapeseed, fava beans, canola, etc.), and forages (hay, silage, etc.) dominate livestock diets (D’Mello, 2012; Patil and Patil, 2017). However, interest in AFR such as food byproducts, insects, wild fruit byproducts (WFBP), algae, seaweeds and single cell proteins is growing due to sustainability issues and growing customer demand for organic and non-GMO products (Adamu et al., 2022; OECD/FAO, 2022; Chisoro et al., 2023). These substitutes offer reduced environmental footprints and lessen the heavy dependency on resource-intensive CFI (i.e. maize and soybean) as depicted by their increased need in production (Figures 3 and 4), especially in developing countries where CFI scarcity persists (Nyamukonda and Ndlovu, 2019; OECD/FAO, 2024).
Locally sourced, sustainable substitutes such as WFBP should be incorporated into stockfeed to lessen this dependency on imported protein feeds (such as grains and seeds). This change reduces supply-chain interruptions and contamination, two hazards associated with long-term storage and overseas shipping. For instance, Ukraine, a significant exporter of soybeans and sunflowers, has worsened storage and transportation circumstances due to the ongoing war, post-Covid trade obstacles, and energy constraints, resulting in damaged grains and feed insecurity (Pexas et al., 2023). The utilization of resources like WFBP reduces reliance on unstable international markets, while improving the livestock sector resilience and productivity when included in stockfeed formulations (Chisoro et al., 2023; Pexas et al., 2023).
Furthermore, the other increasing trend having this push is the advent of precision livestock farming, which maximizes animal health and output using technology and innovation. To track feed consumption and spot potential health risks earlier, sensors and data analysis are being used in livestock production (Islary et al., 2018; Adamu et al., 2022). The industry is already adjusting and adapting to these changes and innovations by creating cutting-edge solutions, like specialized feed formulas and fresh new substitutes or supplement ingredients. These adjustments are essential for addressing the industry’s sustainability issues and meeting the demand for animal products globally (Banday et al., 2023; Jalal et al., 2023).
Despite advances in animal nutrition science, livestock in pastoral and mixed-production systems around the globe experience seasonal or ongoing nutritional stress, which compromises their wellbeing and productivity (Jalal et al., 2023). In both smallholder and commercial systems, inadequate nutrition continues to be a major productivity obstacle that is made worse by unstable supply networks, environmental stresses, and socioeconomic limitations. The following key influencing factors are making it more difficult in the utilization of CFI:
1. Price: Globally, maize and soybeans are the major ingredients in most livestock feed, but many regions, including Europe, Asia, and Africa, cannot continue to rely on their imports. The world’s supply is dominated by major producers such as the U.S., China, Argentina, Brazil, Ukraine, and Russia, making importing countries susceptible to fluctuations in prices and disruptions in supply. For example, all of Malaysia’s feed ingredients are imported, including fishmeal, SBM, and maize; the country imports 3 million tons of maize yearly from Argentina alone, at a cost of US$ 1.5 billion (Donkor et al., 2021). This reliance is prevalent in most areas that cannot supply their local needs for feed for people and animals. Moreover, the UN SDGs, which promote self-sufficient and sustainable agricultural systems, are at odds with this kind of dependence (Nasir et al., 2022). Price trends of CFI tend to fluctuate and have continued increasing globally and are heavily influenced by global trends the likes of the 2020-2022 Covid-19 pandemic, the ongoing Russo-Ukraine war and to some extend inflation and/or possible recessions in some nations (Figure 5).
2. Production: When comparing locally produced feed resources to imported CFI, life cycle assessments show clear environmental benefits. For instance, compared to importing a ton of soybeans from Brazil, with growing and using a ton of lucerne domestically in Europe can reduce land use by 63%, freshwater eutrophication by 97%, acidification by 98%, and global warming potential by up to 95% (Pexas et al., 2023). This emphasizes how local feed resources are more sustainable than imported CFI. The need to diversify feed protein sources is further underscored by climate change forecasts that indicate big yield losses for major soybean farmers, including an 86–92% drop in U.S. soybean yields by 2050. Global CFI growth rates will fall short considering the growing meat consumption demand (Figures 1, 2) and are unable to counteract the growing price volatility (Figure 5), according to recent production trends (2019–2023). To sustainably meet this anticipated demand for livestock products by 2050, these patterns highlight the vital necessity to incorporate AFR, such as locally farmed crops and WFBP (Aziz ur Rahman et al., 2019).
3. Availability: It is difficult for farmers to consistently supply livestock with high-quality feed since CFI are either limited or prone to seasonal shortages in many areas (Chisoro et al., 2019). Natural catastrophes, pandemics, and geopolitical conflicts are examples of global events that worsen shortages by upsetting supply systems and reducing livestock productivity. Economically, it is expensive and risky to import CFI, i.e. soybean meal (SBM), from far-off producers in South America, China, or the U.S. to support livestock systems in Europe, Asia, or Africa. Additionally, the price volatility for fossil fuels and erratic global trade dynamics exacerbates this (Chisoro et al., 2023; Jalal et al., 2023). In addition to destroying ecosystem services and releasing GHG into the environment, the production of CFI for stockfeed puts human food production in competition with it for freshwater resources and arable land (Pexas et al., 2023; Rauw et al., 2023).
4. Climate: The availability, quality, supply, and demand of CFI have all been severely impacted by global climate change. Reduced feed availability and extensive crop failures have resulted from extreme weather events including droughts and floods (Food and Agriculture Organization (FAO), 2018). For instance, in 2019 and 2023, Southern Africa was ravaged by Cyclones Idai, Kenneth, and Freddy, which destroyed infrastructure, livestock, and crops, making food shortages, poverty, and hunger worse (United Nations Development Programme (UNDP), 2019; World Food Programme (WFP), 2019; United Nations Office for the Coordination of Humanitarian Affairs (OCHA), 2023). Similarly, the production and distribution of CFI have been significantly affected by the floods in Brazil and Sri Lanka in 2021, the floods in Pakistan in 2022, the frequent hurricanes in the U.S. and the earthquakes in Japan (OECD/FAO, 2022; 2024). These disastrous incidents show how susceptible CFI supply chains are susceptible to climate-related disturbances, highlighting the crucial need for AFR that can mitigate their supply-demand imbalances and are resilient to environmental events.
5. Infrastructure: The infrastructure required to maintain stable feed and agricultural systems, such as sufficient storage, processing facilities, and transportation networks, is lacking in many emerging countries. These restrictions affect feed manufacturing and animal production by making it more difficult for farmers to make or get high-quality feed components (Lalev et al., 2022; Chisoro et al., 2023). The difficulties faced by producers have been made worse by recent climate disasters as aforementioned that have damaged existing infrastructure (Donkor et al., 2021). Furthermore, Ukraine’s agricultural infrastructure has been seriously devastated by the Russo-Ukrainian war, which has affected its productivity and its position as a major producer of sunflower, maize, and soybeans globally (Banday et al., 2023). To guarantee sustainable production, these infrastructure issues underscore the necessity for robust, locally suitable feed solutions.
Figure 5. The parity price trends per ton of some of the most important CFI in livestock feed as sourced from the USDA (2019, 2020, 2021, 2022, 2023) reports. A parity price refers to a price at which the values of two assets or securities are equal. Prices that farmers receive in the local markets where they typically sell their produce are used to compute parity prices. Implying that, unless otherwise noted, the parity prices apply to the average of all classes and grades of the commodity as sold by all farmers’ USDA (2023). From this we can see that the parity prices where rather similar from 2018 to 2021, but then significant increases of these prices were observed from 2021 onwards to 2023 probably due to the global events (i.e. Covid-19, Russo-Ukraine war, fuel price fluctuations and/or inflation).
These factors make it very difficult for farmers to use or access standard CFI. The use of locally accessible feed sources, like oilseeds of indigenous fruit bearing trees, WFBP, local forage crops, and crop leftovers, as well as the creation of substitute feed ingredients are becoming increasingly popular solutions to solving or mitigating some of these challenges (OECD/FAO, 2022; 2024).
When fruits and vegetables are processed for human use, byproducts such oilseeds, seedcakes, pulp, peels, and pomace are produced (Mwale et al., 2008; Ogunlade and Omojola, 2021). These byproducts are good substitutes or supplements to CFI because they are nutrient-rich and include high amounts of CP, fiber, minerals, and vitamins (Hassan et al., 2011; Getachew et al., 2013). As evidenced by baobab seeds, WF trees yield nuts and fruits with distinct aroma and scents that improve livestock feed intake (FI) (Chisoro et al., 2018). In addition, to their nutritional importance, WF trees support environmental sustainability by offering vital ecosystem services such carbon sequestration and wildlife habitats (Rijsberman and Frank, 2016). Acorns (oak trees), wild cherry fruits, baobab seeds, marula seeds, moringa seeds and leaves, neem seeds, tamarind seeds and leaves, and wild grapevine seeds are a few examples of WFBP that have been researched for use in stockfeed (Chakraborty and Gupta, 2017; Chibwana et al., 2017). These resources provide a sustainable way to lessen dependency on CFI while diversifying the diets of livestock.
Table 1, details how WFBP are potential feedstuffs because of their high-quality fat, protein, vitamins, minerals, and energy content, comparable to the indispensable CFI (i.e. soybean and maize) (Sebola et al., 2021). With the best results at this inclusion level, Chisoro et al. (2018) found that adding baobab seed oilcake (BSOC) to broiler diets in place of 5% of soybean meal (SBM) enhanced growth performance and decreased feed costs. Similarly, Mazizi et al. (2019) showed that Japanese quail diets could be completely substituted with marula nut meal (MNM) in place of SBM without affecting FI or growth. Pig performance and meat oxidative stability were improved by Scerra et al. (2024), when 20% of the cereals (wheat bran and barley) were substituted with bergamot pulp meal (BPM) or olive leaf meal (OLM). In lamb diets, Gravador et al. (2015) found that substituting carob pulp meal (CPM) for 24–35% of barley preserved growth performance while raising the amount of polyunsaturated fatty acids (PUFA) in meat without compromising oxidative stability. Chingala et al. (2019) also discovered that, in comparison to steers fed SBM, feeding Malawi Zebu steers with baobab seed meal (BSM) and white thorn tree leaf meal (WTLM) enhanced microbial nitrogen supply, dry matter intake (DMI), and nutrient digestibility. Finally, gulf algae meal (GAM) derivatives improved immunological responses, performance, and reproductive features in laying Japanese quail, according to Rahmatnejad et al. (2024). Together, these studies demonstrate how WFBP can increase livestock output in a sustainable manner while lowering dependency on CFI.
Even though WFBP have potential as sustainable feed ingredients, their usefulness in livestock diets may be compromised by the presence of ANF like phytate, tannins, protease inhibitors (like trypsin inhibitors), oxalates, saponins, and alkaloids (Nkukwana et al., 2014; El-Deek et al., 2020). Unless they undergo specific processing, these substances decrease palatability, hinder nutrient digestion, and restrict their potential as affordable substitutes for CFI (Nyamukonda and Ndlovu, 2019; Chisoro and Nkukwana, 2020b). It has been demonstrated, for example, that pretreatment of whole jujube with chemical or cold plasma reduces the synthesis of 5-hydroxymethylfurfural while increasing its total phenolic content and antioxidant capacity, hence increasing its functional value (Bao et al., 2021).
Similarly, adding exogenous enzymes (tannase, pectinase, and xylanase) to broiler diets enhanced nutrient availability and decreased the viscosity of ileal digesta in field bean-based feeds, proving the effectiveness of enzymatic detoxification (Abdulla et al., 2017). Additionally, processing methods can increase inclusion rates. For example, when treated, common vetch seeds (CVS) increased inclusion levels in broiler meals by up to 20% without affecting performance when compared to control diets (Sadeghi et al., 2011). These findings underscore the necessity of tailored processing techniques to optimize ANF mitigation and harness the full nutritional potential of WFBP (Kaczmarska et al., 2021). These feed ingredients offer a viable means of fostering sustainability in livestock production, even if additional research is required to grasp their full nutritional worth and potential advantages (Hassan et al., 2020). A summary of a few selected WFBP proximate nutrient compositions is presented in Table 1, detailing their comparability with the main CFI (i.e. maize and soybeans). The table clearly displays how WFBPs contain relatively acceptable nutrient levels to be partial or supplement ingredients in stockfeed.
As aforestated in the above section, these alternatives have factors that at present limit their full utilization (García-Moya et al., 2019). These limitations still require full understanding and innovative ways to counter them. While WFBP share physicochemical and antinutritional issues similar to CFI, critical gaps persist in translating mitigation strategies for the full utilization of WFBP. Through processing, supplementation, or genetic modification, decades of research on CFI have developed reliable methods to mitigate their drawbacks, including nutritional bioavailability, ANF, and digestibility. WFBP, in contrast, are still understudied and have little systematic data on practical ways to overcome their limitations. The following are key WFBP unresolved challenges in their full utilization:
1. Maturity: The fruit’s ripeness level greatly influences its nutritional content and taste. For instance, fruits that are harvested before they are fully mature or ripe enough may be less tasty and contain lower nutritional value than those harvested when they are at their right stage of development (Nyamukonda and Ndlovu, 2019).
2. Processing: Processing techniques have a significant influence on the nutritional makeup, digestibility, and palatability of WFBP. Their chemical structure and nutritional profiles can be improved by processes like grinding, fermentation, or drying, which can increase or decrease their feed value. For example, research shows that drying WFBP may cause thermal degradation or leaching, which lowers concentrations of minerals, vitamins, and phenolic compounds (Chibwana et al., 2017). This necessitates optimized processing procedures to maximize nutrient retention and guarantee consistent quality in stockfeed applications as highlighted by this diversity.
3. Antinutritional elements (ANFs): Due to natural ANF such tannins, phytic acid, and oxalates, WFBP frequently have reduced palatability and bioavailability despite their high nutritional content. For instance, trypsin inhibitors reduce pancreatic enzyme activity, phytic acid chelates vital minerals (such as iron and zinc), and tannins bind to dietary proteins and inhibit proteolytic enzymes, all of which reduce nutrient digestibility (García-Moya et al., 2019). However, these difficulties can be lessened by using specific processing techniques before inclusion of WFBP in stockfeed formulations, such as fermentation, heat treatment, or enzymatic hydrolysis. The entire nutritional potential of WFBP as sustainable substitutes for CFI is unlocked by such interventions, which also improve palatability and neutralizes ANF.
4. Seasonal variation: Harvest timing has an impact on the nutritional makeup of WFBP, which shows notable seasonal variability. For example, the macronutrient, vitamin, and mineral profiles of fruits picked early in the season may be significantly different from those of fruits picked at maturity (Bhatti et al., 2018). When including WFBP into livestock rations, this intrinsic variability calls for thorough, regular nutrient analysis and flexible feed formulation techniques to guarantee constant dietary quality. In order to optimize the sustainable use of WFBP as dependable substitutes for CFI and to account for variations in nutrient density, such proactive approaches are essential.
5. Species variation: While some WF species are high in macronutrients like fiber, phenolic compounds, or antioxidants, others may have higher concentrations of micronutrients like vitamins or minerals (Adejumo and Machebe, 2018). Sensory features, such as unique aroma, also affect livestock acceptance, with some aroma’s increasing or decreasing intake. These issues can be resolved by strategically using feed additives or enzymes to mask unwanted odors, improve palatability, and balance nutrient profiles. In this way, WFBP can be sustainably incorporated into livestock rations while reducing nutrient composition variability and sensory appeal.
6. Environmental elements: The nutritional value and palatability of WFBP are greatly influenced by environmental factors such as soil composition, rainfall patterns, and local climate. According to García-Moya et al. (2019), WFBP cultivated in nutrient-rich soils generally show a greater micronutrient density than those from deprived soils. Trees that have adapted to particular habitats are the source of many WFBP, including seeds and byproducts from native species including baobab, carob, tamarind, neem, marula, and sheanut. Consequently, their yields and availability are naturally erratic and dependent on regional climates (Chisoro and Nkukwana, 2020b). This reliance on the environment highlights the necessity of region-specific approaches to improve WFBP consumption in livestock feed and stabilize supply systems, guaranteeing their sustainability as sustainable substitutes for CFI (Wapi et al, 2013).
7. Technical aspects: There are several obstacles in the way of the sustainable incorporation of WFBP into livestock feed systems. Seasonal and unpredictable variations in supply, physicochemical characteristics including dustiness, high bulk density, and powdery texture, and supply chain inefficiencies in post-harvest management (collection, storage, and transportation) are some of the main obstacles (Pexas et al., 2023). Additional limitations stem from the requirement for costly processing procedures (such as dehulling, drying, mechanical, or thermal treatments) to improve safety and digestibility, as well as a lack of research facilities to determine the optimum levels of nutrient inclusion in animal diets (Abdollahi and Ravindran, 2019; Rahmatnejad et al., 2024). Unlocking the full potential of WFBP as sustainable, regionally adaptable substitutes for CFI requires tackling these issues comprehensively i.e. through enhanced processing technologies, innovative logistics, and focused research and development.
Several unresolved issues continue to impact the nutritional quality and palatability of WFBP in livestock diets. To optimize their potential as sustainable substitutes for CFI, targeted strategies are needed, such as rigorous research to address ANF, optimized processing methods, and precise formulation protocols to determine optimum inclusion levels. Additionally, for WFBP to be successfully incorporated into livestock rations, three requirements must be met: (a) consistent availability (even if seasonal), (b) cost competitiveness in comparison to CFI, and (c) thorough characterization of their nutritional variability, digestibility, and metabolizable energy content (Hassan et al., 2020; Abdollahi and Ravindran, 2019).
Beyond these basics, improving the feeding value of WFBP necessitates a variety of strategies, including: (i) feed evaluation that prioritizes energy and digestible AA; (ii) diet formulations based on digestible rather than total AA; (iii) adding synthetic AA strategically to meet animal needs; (iv) using exogenous enzymes to improve nutrient utilization; and (v) customized processing techniques to reduce ANF and increase nutrient bioavailability (Abdollahi and Ravindran, 2019; Chisoro et al., 2023). When taken as a whole, these actions could increase the quantity of WFBP included in livestock diets while supporting the objectives of nutritional and economic sustainability.
Population growth and changing dietary choices are expected to create a 70% increase in the demand for livestock-derived goods worldwide by 2050 (Food and Agriculture Organization (FAO), 2023; World Bank Group, 2021). To meet this demand, 30–50% more agricultural area may be needed if existing livestock production methods continue, which would put further strain on limited natural resources. Given that the livestock industry currently makes a substantial contribution to GHG emissions, land degradation, and biodiversity loss, such expansion runs the danger of making environmental degradation worse (Marchant-Forde and Boyle, 2020; Chisoro et al., 2023). To decouple livestock production development from ecological impact, it is imperative that sustainable alternatives be used, such as incorporating WFBP into stockfeed.
The incorporation of WFBP into livestock feed formulations offers the benefits of both economic efficiency and ecological protection. This avoids the need for more arable land and lowers the amount of land allocated for waste management, protecting local ecosystems by reusing materials like baobab seed oil cake (BSOC), tamarind leaf meal (TLM), sheanut seed meal (SSM), and mango seed meal (MNM), which are leftovers from processing WF. Crucially, because these materials are sourced from pre-existing agro-industrial waste streams, WFBP procurement avoids direct land-use competition and has a small land footprint. Their superiority over CFI is further demonstrated by environmental life-cycle assessments. With sustainable sourcing and processing methods, WFBP can reduce eutrophication potential by up to 83% and reduce GHG emissions, acidification, and other pollution indicators by up to 99% (Abdulla et al., 2017; Habibi et al., 2024).
Circular feed resource systems that emphasize regeneration and local sourcing can reduce input costs and increase productivity economically. Adoption of AFR allows for immediate cost savings by reducing dependency on synthetic fertilizers, irrigation, agrochemicals, and fossil fuels (all of which are major sources of cost in CFI production) (Hassan et al., 2020). Additionally, logistical costs are decreased via proximity-driven models for AFR, in which commodities are produced close to end-user markets or transit hubs. In addition to reducing emissions associated with transportation, this localization optimizes supply chains, reducing the costs associated with producing feed and maintaining livestock (Mbukwane et al., 2022). When combined, these tactics establish WFBP as essential elements of sustainable livestock systems that strike a balance between environmental preservation and financial sustainability.
Due to meat’s high nutritional value (protein, vital fatty acids, and minerals) and gastronomic appeal, meat plays a crucial part in various diets around the world. However, the livestock sector is under increasing pressure from cascading disruptions as earlier stated, which have made supply-demand imbalances worse and made conventional meat production less predictable and more expensive (Omollo et al., 2020). However, despite production and religious restrictions in emerging countries, pork continues to dominate markets, with beef and poultry following closely behind (Figures 1, 2) (Egea et al., 2018; Wang et al., 2022; Xu et al., 2023). Notably, because of its versatility and lower resource thresholds, poultry continues to be a vital component of smallholder and subsistence systems in Africa, Asia, and Latin America (Jalal et al., 2023). To overcome limiting obstacles, use of AFR in stockfeed, such as WFBP, provides a practical way to boost output and maintain supply chains (Chisoro et al., 2018, 2023). Current research highlights WFBP’s potential to reduce dependency on resource-intensive conventional diets by enhancing feed efficiency, disease resilience, and growth performance across species. Some case studies on the use of WFBP in ruminant, pig, and poultry systems are summarized in the sections that follow.
One of the most indispensable sources of animal protein crucial for human growth and development is poultry meat. With an expected total meat consumption of 45.3 kg, current predictions predict that its share of the world’s per capita meat consumption would increase from 13.8 kg (33%) to 17.2 kg (38%), by 2030 (Nkukwana, 2019; World Economic Forum, 2019). The output of chicken feed is anticipated to increase at a compound annual rate of 7.1% between 2018 and 2027, in tandem with this demand, reaching a total of about 890 million metric tons by the conclusion of this time frame (Abdollahi and Ravindran, 2019). But even in the most optimistic agricultural yield scenarios, CFI (i.e. maize and SBM), are unlikely to be able to meet these increasing demands. Since 60–70% of the costs of raising chickens are feed related, inclusion of AFR such as WFBP provides a dual benefit, they lessen dependency on CFI while lowering feed-related expenditures (Chisoro and Nkukwana, 2020b). These kinds of developments are essential for improving cost effectiveness, closing supply-demand gaps, and guaranteeing poultry systems’ long-term scalability.
Various studies in poultry have shown pronounced benefits in the utilization of WFBP either as alternatives to antibiotics or supplements to maize and soybean. For example, Paul et al. (2022) showed that adding neem leaf extract (NLE) to Cobb 500 broiler feeds in place of enrofloxacin and ciprofloxacin greatly improved growth performance, final body weight (BW), and organ development. Similarly, broilers fed Moringa oleifera leaf meal (MOLM) in place of salinomycin and zinc bacitracin showed enhanced feed conversion ratios (FCR), BW growth, and gastrointestinal health (Nkukwana et al., 2014). This strategy was further supported by earlier research by Schiavone et al. (2008), which demonstrated that chestnut wood extract (CWE) may increase FI and BW while replacing synthetic DL-α-tocopherol acetate in broiler diets without sacrificing nutrient digestibility. These results support the urgent need for the poultry sector to lessen its reliance on antibiotics, which fuel antimicrobial resistance, a serious public health issue, especially in broiler systems where there is a higher danger of zoonotic transmission. Producers can address both production efficiency and antimicrobial stewardship by incorporating WFBP, such as NLE, MOLM, and CWE (Table 2).
Table 2. Performance characteristics summary on monogastric animals fed varying inclusion levels of WFBP in their diets.
As the most intensively produced type of meat and with the highest feed costs the use of AFR in pig production is very apparent. Numerous studies have been done in supplementing WFBP in pig diets with various promising results. For instance, developing pigs fed diets containing 15% sodium hydroxide-treated sheanut kernel cake (TSNKC) as a substitute for wheat bran showed equivalent growth performance and FI according to Oddoye et al. (2015). According to Magonka et al. (2018), growth parameters were maintained while feed costs were decreased when 5% sunflower cake (SFC) was substituted with baobab seed cake (BSC). The usefulness of WFBP was further confirmed by Mabena et al. (2022), who demonstrated that 15% amarula nut cake (ANC) may be used in place of SBM in the diets of growing pigs, producing comparable protein digestibility and FI while improving the color characteristics of the meat (redness, lightness). Beyond cost-efficiency, WFBP can improve meat quality, Scerra et al. (2022) observed that 15% exhausted bergamot pulp byproduct (EBPB) as a partial SBM substitute in fattening pigs increased docosapentaenoic acid and total n-3 polyunsaturated fatty acids (PUFA) in pork, enhancing its nutritional profile despite no differences in FI, final BW, or carcass yield.
In the context of global food security, the domesticated rabbit stands out as a notable potential livestock species, especially as the growing human population puts more strain on limited agricultural resources (McNitt et al., 2013). Rabbits show remarkable adaptation to high-roughage, low-grain rations while retaining robust growth and reproductive performance, in contrast to pig and poultry, which need grain-dependent diets (de Blas and Wiseman, 2010). According to recent research, grain-free diets high in fibrous plant matter can support rabbits’ normal physiological development, providing a crucial edge as human and livestock competition for CFI intensifies (McNitt et al., 2013). Due to their ability to effectively turn unconventional feed sources (i.e. like crop residues, agroforestry byproducts, and wild vegetation) into premium meat, rabbits are positioned as a sustainable source of protein, especially in developing nations that are struggling with food scarcity and a lack of arable land (Mcafee et al., 2010). Additionally, the richness of native plants in these areas, which are frequently inappropriate for monogastric animals, complements rabbits’ nutritional adaptability and lessens their dependency on feeds that require a lot of resources. Through the use of locally accessible WFBP in rabbit production, stakeholders can improve food security, reduce volatility of feed-costs, and promote circular agricultural systems; all of which are important goals for robust, just food networks.
Given that the world’s population expected increase to 10 billion people by 2050, producing rabbits appears to be a viable way to meet this growing demand for food. The inclusion of WFBP in rabbit diets has two benefits: a) it lowers feed costs while improving growth and b) reproductive efficiency. For example, Ogbuewu et al. (2011) showed that neem leaf meal (NLM) enhanced female reproductive results without sacrificing growth or FI when 5% soybean meal (SBM) was substituted. Similarly, Henry et al. (2018) found that adding pawpaw leaf meal (PLM) to the meals of New Zealand White × Chinchilla crossbreed rabbits improved their reproductive success and follicle-stimulating hormone (FSH) levels without having an unfavorable effect on growth or other physiological balances. Adeyeye et al. (2021) also noted that replacing 10% SBM with kola nut pod-husk meal (KNPM) reduced feed costs and increased profitability while maintaining growth performance similar to control diets. While Adeniji et al. (2012) noted that a 60% addition of a 1:1 blend of groundnut cake (GNC) and MOLM to grower rabbit diets increased BW and reduced feed costs compared to the unsupplemented feeds. Taken together, these studies demonstrate the feasibility of WFBP as sustainable, affordable feed substitutes that maximize rabbit husbandry productivity (Table 2).
The inclusion of WFBP to beef cattle diets has shown interesting results, including nutritional and therapeutic benefits. For example, the leaves of tamarind (Tamarindus indica) and neem (Azadirachta indica), which are known across the world for their hepatoprotective, antibacterial, and antiprotozoal qualities, have been tested as natural anthelmintics. In contrast to their solo use (neem: 29.93%; tamarind: 42.67%), Rahaman et al. (2022) found that a 1:1 blend of neem and tamarind leaves had higher anthelmintic efficacy (68.15%), demonstrating synergistic potential in parasite treatment. Beyond their use in medicine, WFBP can be used as affordable alternatives to traditional feed antibiotics. Mlambo et al. (2011) found that replacing urea with marula seed cake (MSC) in weaner cattle rations yielded comparable growth rates, FI, and FCR, with no differences in average daily gain (ADG) between MSC-supplemented and control diets. This suggests MSC could reduce the reliance on synthetic nitrogen sources without compromising productivity. Similarly, Chingala et al. (2019) evaluated baobab seed meal (BSM) and white thorn tree leaf-meal (WTLM) as protein alternatives to soybean meal (SBM) in Malawi Zebu steers. Steers fed BSM diets outperformed their SBM-fed counterparts in terms of dry matter intake (DMI), final BW, and total tract nutrient digestibility (TND), all while displaying a higher microbial nitrogen supply. Overall, these studies demonstrate the dual utility of WFBP in beef production systems; as cost-effective substitutes for traditional protein or energy sources and as functional additives for health management (Table 3).
Table 3. Summary performance characteristics in ruminant livestock fed diets with varying inclusion levels of WFBP.
Generally, the global dairy sector has been experiencing steady growth on both production and consumption (Gebreyowhans and Zegeye, 2019).The demand for dairy products has been increasing globally, driven by population increase, the rise in household income and the change in dietary preferences (International Livestock Research Institute (ILRI), 2016; World Bank Group, 2021). Global milk production peaked at 810 million metric tons in 2020, with China, India, and the U.S. as the top three producers (World Bank Group, 2021; USDA, 2022). At the same time, emerging nations have been the main drivers of this sectoral expansion; their dairy industries are growing at an average annual rate of 3.7% (World Economic Forum, 2019). Rising disposable incomes, urbanization, population increase, and dietary changes toward nutrient-rich foods are the main causes of this increase (International Livestock Research Institute (ILRI), 2016; Food and Agriculture Organization (FAO), 2019). The need to find sustainable feed resources, such as WFBP, to meet the growing demand while lowering dependency on CFI is highlighted by this rapid growth.
The need to implement climate-smart agriculture and reduce its environmental impact has prompted the dairy industry to embrace technology advancements and sustainability-driven methods more and more (Food and Agriculture Organization (FAO), 2019; Livestock Development Institute of Africa (LDIA), 2014; World Bank Group, 2021). However, this sectoral development and profitability are threatened by enduring issues such as fluctuating milk costs, competition from plant-based substitutes (such as soy, almond, oat, and rice-based products), and changing consumer trends (World Bank Group, 2021). Notwithstanding these challenges, there is a significant opportunity due to the ongoing growth of dairy output in emerging countries and the persistent desire from consumers for traditional dairy products.
Dairy rations that incorporate WFBP could improve sustainability, lower costs, and increase resistance to environmental and market stresses. Studies demonstrate their capacity to boost ruminal protein metabolism, milk yield (MY), and milk protein (MP) content in dairy calves without compromising FI or nutrient digestibility (Madzimure et al., 2011; Bashar et al., 2020). For example, Raj et al. (2016) discovered that adding karanja or neem seed cake to total mixed rations (TMR) in place of 30% of CFI increased MY while preserving MP levels and milk solids. Sesbania leaf meal (SSLM) increased MY in crossbred dairy cows without lowering FI (Gebreyowhans and Zegeye, 2019), while marula seed cake (MSC) replaced SBM in nursing cows to improve digestibility without changing MY (Mdziniso et al., 2016). Additionally, Bashar et al. (2020) found that adding moringa leaf meal (MOLM) gradually to diets based on silage increased milk output while maintaining digestibility and crude protein (CP) intake. The dairy industry can promote sustainable agriculture, lower GHG emissions associated with the production of conventional feed and conform to climate-smart practices by incorporating WFBP as partial substitutes or supplements (Table 3).
Small-scale ruminant production, especially that of sheep and goats, is essential to the economy of desert and rural areas of emerging nations and is expected to continue to be crucial to the world’s meat supply. WFBP provide sustainable ways to improve meat quality and productivity in these systems. For example, Qwele et al. (2013) noted that Xhosa lop-eared goats fed either grass hay (GH), sunflower cake (SFC), or MOLM, discovered that supplementing with MOLM (200 g/day) increased the meat’s antioxidant capacity, as shown by a lower lipid oxidation (LO) and a higher total phenolic content (10.62 ± 0.27 mg tannic acid equivalent/g). This enhanced the meat’s color, chemical composition, and shelf-life stability over time. Similarly, Geron et al. (2015) showed that adding 15% tamarind pulp residue (RETP) to sheep diets preserved FI and BW while reducing feed expenses. More recently, Baleseng et al. (2023) found that in lamb fattening diets, morula kernel cake (MKC) can completely replace traditional protein sources like lucerne and sunflower meal (SFM) without affecting meat quality, FCR, final BW, DMI, or FI. This was ascribed by the study to MKC’s balanced nutritional composition, which satisfied the growth and maintenance needs of lambs (Table 3).
The nutritional value and sensory qualities of meat are significantly influenced by the content of the feed (Stelzleni and Johnson, 2008). Plant-derived natural antioxidants (PDNA), which are abundant in unsaturated fatty acids and bioactive compounds, such as essential oils, herbs, leaf meals, and seed extracts, have drawn more attention from researchers to improve the quality of meat (Cullere et al., 2013; Hoffman et al., 2014). Notably, the fatty acid profile, oxidative stability, and sensory qualities of meat are enhanced by the effective transfer of polyphenols and flavonoids, which are abundant in many PDNA sources, from feed to muscle tissue (Fu et al., 2022; Tang et al., 2023). While the bioavailability and metabolic fate of these compounds vary depending on their chemical structure and post-ingestion pathways, their role in mitigating LO and modulating volatile compound formation remains pivotal to preserving meat quality (Bellés et al., 2017; Estévez and Lorenzo, 2018). WFBP, which are particularly rich in phenolics and antioxidants, offer a sustainable means of enhancing these benefits. Incorporating WFBP into livestock rations can thus synergistically improve meat sensory parameters, such as flavor and shelf-life stability.
Furthermore, to enhance the quality of chicken, pork, and beef, it is customary to supplement with α-tocopherol (100–200 mg/kg feed). α-tocopherol is an essential part of the skeletal muscle’s endogenous antioxidant defense and a major factor in the oxidative stability of biological membranes (Dalle Zotte et al., 2016; Tang et al., 2023). Supplementing with α-tocopherol has many well-documented benefits, such as protecting fresh meat from discoloration, promoting greater oxidative stability during storage, and reducing the oxidation of proteins, cholesterol, and PUFAs in processed meat products (Estévez and Lorenzo, 2018; Tang et al., 2023). Significantly, comparable results have been obtained with natural tocopherol-rich feedstuffs, including plant-derived materials (e.g., rooibos, tamarind, moringa, acorns, and olive leaf) and WFBP, which are in line with sustainable practices (Dalle Zotte et al., 2016; Wong et al., 2023; Wang et al., 2024). Whole plants, leaves, seeds, and their extracts are increasingly valued in livestock diets as multifunctional additives, acting as natural antioxidants, color stabilizers, and physiological enhancers (Qi et al., 2022; Tang et al., 2023). This highlights the feasibility of incorporating WFBP as partial feed ingredients or supplements, providing a dual benefit of enhancing meat quality and promoting resource-efficient, circular agricultural systems.
Due to their antibacterial and antioxidant qualities, WFBP show great promise as natural feed additives. For example, Jayawardana et al. (2015) showed that by lowering LO, MOLM improves oxidative stability in ghee, increasing shelf life. Similarly, Qwele et al. (2013) found that dietary MOLM supplementation reduced superoxide dismutase (SOD) activity and glutathione (GSH) depletion in goats while reducing LO in meat during storage. Gravador et al. (2015) discovered that carob pulp meal (CPM) preserved the oxidative stability of meat in ruminant diets by substituting 24–35% of barley without hastening deterioration. Scerra et al. (2022) further noted that adding 30% almond hulls (AH) to lamb diets enhanced meat oxidative stability (lowering LO in raw and cooked meat after 5 and 7 days of refrigeration, respectively) without affecting growth performance. In addition to terrestrial livestock, Hendam et al. (2024) noted how baobab fruit extract powder (BFP; 4 g/kg diet) benefits Nile tilapia by improving intestinal health, growth, antioxidant capacity, and serum immunity. Finally, Taylor et al. (2011) demonstrated that broiler diets containing volatile oil and dried rosemary leaves inhibited the production of off-flavor and thiobarbituric acid-reactive compounds (TBARS) in breast meat. Taken together, these studies highlight WFBP’s dual function of enhancing meat quality and shelf life while supporting resource-efficient, sustainable animal production.
To improve their safety, palatability, and digestibility for the best possible nutrient utilization by animals, feed ingredients must go through crucial preprocessing in the manufacturing of livestock feed to remove contaminants, ANF, mycotoxins, and pathogens (Kinyuru et al., 2018; Nyamukonda and Ndlovu, 2019). The reduction of ANF (i.e. naturally occurring compounds that impair nutrient bioavailability, FI, digestibility, and animal health) is essential for the successful integration of WFBP as partial ingredients or supplements in livestock rations (Jayawardana et al., 2015; Chisoro et al., 2023; Hendam et al., 2024). ANFs, which include tannins, alkaloids, and protease inhibitors, limit livestock productivity and product quality by interfering with the absorption of essential nutrients, such as minerals (like calcium and phosphorus), vitamins (like A, C, and E), and essential AA (like lysine and methionine) (Geron et al., 2015; Tang et al., 2023).
To lessen these impacts, traditional processing methods are used separately or in combination to break down ANF below critical toxicity thresholds, including soaking, fermentation, germination, steam sterilization, and dehulling (Bashar et al., 2020; Scerra et al., 2022; Rahaman et al., 2022). CFI processing already incorporates these techniques; for instance, cottonseeds are heat-treated to detoxify gossypol (Negi and Pant, 2017; Feedipedia, 2023), and soybeans are roasted to lower trypsin inhibitors and tannins (CVB, 2018). By using comparable techniques on WFBP, their potential as sustainable substitutes or supplements to CFI can be unlocked by improving their palatability, nutrient bioavailability, and safety. Specific processing strategies designed to maximize the use of WFBP in animal diets are examined in the sections that follow:
1. Sun drying: Before inclusion of WFBP to livestock rations, feed manufacturers use sun drying as a low-cost and widely used method to reduce moisture and ANF. This method works best for moisture-rich WFBP, like juicy fruits, leaf meals, and seeds, which often need to be dehydrated to improve digestibility, extend shelf life, and facilitate processing (Ramteke et al., 2019). Common uses include drying azolla, duckweed, cassava peels, sweet potato foliage, and algae; materials that are susceptible to deterioration because of their high initial moisture content (Stein et al., 2016; Rojas and Stein, 2017; Widderich et al., 2022).
The final product’s nutritional quality is greatly impacted by the drying process. For example, when Babiker et al. (2018) examined the effects of partial shade (PS) and direct sun (DS) drying on the leaves of Moringa oleifera and M. peregrina, they found different compositional results. Whereas DS-dried leaves showed increased antioxidant activity and overall phenolic content, PS-dried leaves maintained higher quantities of beta-carotene, ascorbic acid, minerals, and AA. These results highlight the need of choosing drying methods according to the feedstuff being dried, since DS improves oxidative stability while PS protects heat-sensitive vitamins. Such information is essential for maximizing the use of WFBP in feed compositions tailored to a given region.
2. Autoclaving: Like sun drying but more effective, autoclaving is a thermal processing method that uses heat, steam, and pressure to lower moisture and ANF in WFBP. Autoclaving is useful in cold or humid locations with little sunlight (such as parts of Europe), where typical sun drying is unfeasible, albeit being more expensive and energy intensive. This process improves nutrient bioavailability and shelf stability while hastening the dehydration of high-moisture products like fruit pulps or leaf meals. Its effectiveness in raising feed quality has been demonstrated by recent studies. For example, when common carp (Cyprinus carpio) were fed SBM, wheat bran, maize, and barley, Abdulazeez and Al-Tameemi (2023) showed that autoclaving greatly raised the apparent digestibility coefficients (ADCs) for dry matter, protein, and energy. Although fishmeal demonstrated little reactivity, autoclaved diets also increased growth rates and feed efficiency as compared to untreated controls. Similarly, Sung et al. (2022) assessed autoclaved poultry meal (APM) in broiler chickens and pigs, identifying metabolic responses unique to each species. The metabolizable energy (ME) of unautoclaved poultry meal (unAPM) was higher in pigs (4,792 kcal/kg DM) than in broilers (3,591 kcal/kg DM). While autoclaving for a longer period (134°C, up to 180 min) decreased ME values more sharply in pigs, indicating that broilers are better able to withstand extended processed APM. These results highlight the potential of using autoclaving to maximize WFBP utilization in feed systems, albeit with trade-offs.
3. Chemical or cold treatment: By reducing ANF and enhancing functional qualities, chemical and cold treatments are efficient ways to increase the nutritional value of WFBP. Because trypsin inhibitors are stable in acidic environments, Fernandez et al. (1993) noted that soaking faba beans in a 0.07% sodium bicarbonate (NaHCO3) solution decreased trypsin inhibitor activity more efficiently than a 0.1% citric acid solution. Similarly, novel cold treatments like thermosonication and cold plasma have demonstrated promise in altering the structure of WFBP. According to Bao et al. (2021), pretreatments with chemicals, cold plasma, and thermosonication changed the morphology of the jujube’s surface by forming pores and microcracks, which sped up moisture transport and cut drying periods by 18%, 12%, and 7%, respectively. Notably, chemical and cold plasma pretreatments decreased the formation of 5-hydroxymethylfurfural (a potential toxicant) by 25% and 15%, respectively, improving the safety and quality of dried jujube for feed applications. These treatments also improved nutrient retention; total phenolic content increased by 13%, 12%, and 6%, while antioxidant capacity (as determined by ferric reducing antioxidant power) increased by 13%, 11%, and 3%. These methods offer scalable, energy-efficient alternatives to conventional drying, especially for moisture-rich WFBP in areas lacking consistent solar exposure.
4. Soaking: For WFBP, soaking is an economical pretreatment that improves moisture content, palatability, and nutrient digestibility while lowering ANF including tannins and phytic acid (Gürbüz and Özkan, 2018). However, the type of plant, time, and soaking medium all affect how effective it is. For example, soaking lentil seeds in distilled water for 24 hrs decreased trypsin inhibitors by 58–66% (Batra et al., 1986), but soaking French bean (Phaseolus vulgaris) seeds in water for 18 hrs maintained 98–99% of trypsin inhibitor activity (Desphande and Cheryan, 1983). Recent developments emphasize the importance of targeted soaking solutions, Dhlakama et al. (2022) showed that treating baobab seeds with deionized water, 0.5% monovalent salts (NaCl, NaHCO3), or divalent sodium salts (Na2S2O5) significantly reduced bulk density, oxalate, and phytate content while raising the temperature at which they gelatinized, which are important improvements for feed processing and nutrient bioavailability. These results highlight the potential of soaking as a scalable technique to maximize WFBP for livestock diets, as long as the parameters are adjusted to the feedstuff’s unique ANF profile and functional objectives.
5. Sprouting or Germination: This is a technique commonly used in hydroponic fodder systems (e.g., barley, wheat, and maize) that improves feed quality through biochemical modifications during seed germination (García-Moya et al., 2019). For example, sprouting decreased phytic-phosphorus levels in mung beans (Phaseolus aureus) and rice beans (Vigna umbellata) by 9.8% and 11.3%, respectively, in comparison to ungerminated seeds (Verma and Mehta, 1988). These biochemical changes highlight sprouting’s potential to optimize WFBP for sustainable livestock feed formulations. Such decreases in phytic acid improve mineral absorption in livestock by reducing its chelating capacity (Khan et al., 1991; Sharma et al., 1996). Additionally, sprouting mitigates lectin-related ANFs; germination decreased haemagglutinating activity in seeds by inactivating concanavalin A, as evidenced by a 49% reduction in jack bean (Canavalia ensiformis) after treatment (Esonu et al., 1998; Akande and Fabiyi, 2010).
6. Roasting and/or toasting: Thermal processing methods like roasting and toasting improve the quality of livestock feedstuffs by lowering ANF and increasing digestibility and palatability. These techniques work especially well with oilseed meals like cottonseed meal CSM) and SBM. For example, it has been demonstrated that roasting baobab (Adansonia digitata) seeds or seed cake improves FI and growth performance in sheep, layers, and broilers. This increase allows broiler diet inclusion levels to increase from 5% to 15% (Chisoro et al., 2018; Saulawa et al., 2014; Hassan et al., 2020b; Adeyeye et al., 2021). The nutritional content of baobab and jack bean (Canavalia ensiformis) seeds can also be increased by toasting them, enabling up to 20% of them to be included in poultry feeds (Akande and Fabiyi, 2010; Saulawa et al., 2014). Toasting by itself, however, might not be enough to reduce ANFs in some ingredients. Esonu et al. (1998) discovered that toasting jack beans did not considerably lower toxin levels, highlighting the necessity of combining thermal treatments with other techniques (such as soaking and fermentation) in order to optimize ANF reduction and safely increase of inclusion rates. According to these results, roasting and toasting are useful but context-dependent tactics that call for complementing processing methods to ensure the best possible feed safety and effectiveness in systems in livestock.
7. Fermentation: By using beneficial microorganisms to break down ANF, fermentation improves nutrient bioavailability, feed palatability, and digestibility in WFBP, stabilizes feed matrices, improves gut health, and reduces waste through the production of microbial metabolites (e.g., organic acids, enzymes). Research on baobab, tamarind, common vetch, and moringa seeds has shown that fermentation increases inclusion levels in livestock diets, increases dry matter digestibility, and improves FI in poultry and ruminants (Singh et al., 2007; Chisoro et al., 2017; Stein et al., 2016; Saulawa et al., 2014).
Fermentative bacteria, such as Lactobacillus and Bacillus species, play a critical role in biotransforming mycotoxins into non-toxic metabolites, improving microbial safety (Sugiharto and Ranjitkar, 2019). In addition to improving nutrition, fermentation also improves feed safety by reducing mycotoxin contamination. For instance, solid-state fermentation (SSF) reduced zearalenone (a Fusarium-derived mycotoxin) by 60–80% in cereal-based substrates (Yang et al., 2018), and Okeke et al. (2015) also observed similar detoxification in maize grains.
8. Enzymes: The inability of poultry to hydrolyze non-starch polysaccharides (NSPs) and ANF found in CFI like wheat, soybean, barley, and rapeseed has been addressed by exogenous enzyme supplementation, which improves nutrient digestibility, availability, and absorption in formulated feeds (Sugiharto and Ranjitkar, 2019). Although not a direct processing method, enzymes like amylase, phytase, and protease are essential feed additives that hydrolyze complex carbohydrates, phytic acid, and proteins, respectively, releasing bound nutrients (Kiarie et al., 2013; Rahman et al., 2021).
Enzyme supplementation can maximize nutrient release from fibrous matrices in WFBP added to livestock rations, negating the decreased digestibility frequently linked to non-traditional feed sources. For example, in diets high in WFBP, phytase increases the bioavailability of phosphorus, while proteases improve the absorption of AA, which are important factors in swine and poultry development performance (Abdollahi and Ravindran, 2019; Mbukwane et al., 2022). This approach lessens dependency on expensive, energy-intensive processing techniques while simultaneously increasing feed efficiency and promoting the sustainable use of WFBP.
The meticulous, context-specific application of techniques is essential to this process since different feed ingredients frequently call for customized combinations of techniques to maximize digestibility, nutritional availability, and palatability. Two separate stages of processing take place. To reduce ANF and improve nutritional utility, the first phase concentrates on ingredient-specific treatments (such as drying, fermentation, or detoxification etc.). For AFR, they must undergo stringent preprocessing to satisfy quality criteria as substitutes or supplements to CFI, this stage is especially crucial.
The secondary phase involves feed manufacturing steps: a) Grinding to increase surface area, disrupt ANF, and improve nutrient accessibility; b) Mixing to ensure uniform nutrient distribution; and c) Pelletizing/Extrusion to reduce waste and enhance digestibility by consolidating ingredients into consistent, nutrient-dense pellets. While secondary processing is standard in commercial feed production, the primary phase remains indispensable, especially for AFR. Given livestock producers’ stringent demands for high-quality feed, meticulous preprocessing of WFBP is essential to ensure their viability in sustainable feed formulations.
This review highlighted a lot of points on the use of WFBP in livestock feed. This highlights a few additional points that must be considered for future full utilization of these resources in livestock feed as detailed below:
1. Value addition and commercialization: Examination of the potential for adding value to WFBP must be considered to increase their economic value and marketability. The appropriate processing methods as aforementioned must be investigated and used according to the WFBP in question. To create products with added value for stockfeed, human use, and in other sectors. Entrepreneurship and the creation of small businesses for the production and sale of products based on these WFBP must be encouraged by local communities or the government (Chisoro et al., 2023).
2. Support for policies and regulations: Policies and rules that encourage the sustainable inclusion of WFBP in stockfeed must be promoted. Local communities and relevant government agencies must work together in the creation of such policies and standards for the collection, processing, and marketing of these resources. While promoting the inclusion of WFBP feed sources in national strategies and plans for continued livestock production (Chisoro and Nkukwana, 2020a; Nduku et al., 2021).
3. Research and development needs: Continued research on the proximate nutrient composition, bioactive substances, and potential health advantages of various WFBP through additional research (Chisoro et al., 2019). While also investigating how using feed made from these resources impacts animal performance, product quality, and environmental sustainability (Jalal et al., 2023). In addition, looking into how livestock diets can be improved, by considering the potential synergistic effects of mixing these AFR with other feed additives (antioxidants, probiotics, prebiotics, enzymes etc.). Further hands-on research and on-farm animal trials to determine whether it is feasible and acceptable to feed livestock with rations that contain these feedstuffs at various inclusion levels as shown in Tables 2 and 3.
4. Community engagement and capacity building: Engagement of local communities, farmers, and livestock producers in the sustainable use of these resources must be encouraged. By offering training and capacity-building programs on how to recognize, gather, prepare, and use WFBP as livestock feed ingredients (Mazizi et al., 2020). While also encouraging research teams, extension services, and regional communities to cooperate to transfer technologies and share information on the use and processing of these feed resources (Hassan et al., 2020).
5. Protection of the environment: Emphasize on the environmental advantages of using WFBP in feed, such as lowering demand on natural resources and fostering biodiversity preservation by local communities and governments. By speaking up in favor of conservation of WF-bearing trees and appropriate harvesting methods to assure their ongoing availability (Chisoro and Nkukwana, 2020b). While encouraging agroforestry systems that combine the production of livestock with the cultivation of WF trees, boosting the ecosystem’s resilience and push UN SDGs (Chisoro et al., 2023).
It is essential to investigate the possibilities and prospects of incorporating WFBP into livestock feed to support sustainable livestock production systems. Producers can improve resource efficiency and lessen dependency on CFI by giving priority to research on scalable processing techniques, environmental effect reduction, and nutritional improvement. By implementing these tactics, the livestock sector will become more robust and ecologically balanced, maximizing the value of unused WFBP and bringing agricultural practices in line with international sustainability standards.
WFBP have a lot of potential to improve livestock production in a sustainable way, especially in developing nations where access to CFI is limited. In addition to resolving feed scarcity and lowering environmental footprints, these underutilized materials provide affordable, environmentally friendly CFI substitutes. However, more research is needed to determine species-specific nutritional profiles, safe inclusion levels, and commercial viability to optimize their incorporation into livestock diets. To guarantee constant quality and supply, practical issues including seasonal availability, sustainable harvesting methods, and post-harvest storage must also be methodically resolved.
Policy frameworks and institutional actions encouraging the sustainable use of WFBP could empower rural smallholder farmers, enhancing livelihoods and increasing socioeconomic resilience. This review underlines the dual benefits of WFBP adoption, improving sustainable agriculture through lower feed prices and ecological implications, while aiding rural development. Cooperation between governments, scientists, and international organizations is essential to achieving this promise. By making strategic investments in technology transfer, market connections, and context-specific research, livestock farmers will be able to efficiently utilize these resources, closing gaps in sustainability and food security.
PC: Conceptualization, Data curation, Writing – original draft, Writing – review & editing. BM: Conceptualization, Formal analysis, Investigation, Methodology, Writing – review & editing. IFJ: Formal analysis, Funding acquisition, Project administration, Supervision, Conceptualization, Investigation, Writing – review & editing, Validation. TN: Conceptualization, Investigation, Methodology, Validation, Project administration, Visualization, Writing – review & editing, Supervision. NA: Conceptualization, Methodology, Software, Validation, Writing – review & editing.
The author(s) declare that financial support was received for the research and/or publication of this article. The author(s) declare that financial support and sponsorship of funds was received from the Govan Mbeki Research and Development Committee (GMRDC) and the Department of Livestock and Pasture of the University of Fort Hare (UFH).
We would like to thank the financial aid and relevant support from the Govan Mbeki Research and Development Committee (GMRDC) and the Department of Livestock and Pasture of the University of Fort Hare (UFH). Including everyone who assisted us in the successful development and writeup completion of this review article.
Author PC is the Founder and CEO of Gwaimana Consolidated Pvt Ltd.
The remaining authors declare that the research was conducted in the absence of any commercial or financial relationships that could be construed as a potential conflict of interest.
The author(s) declare that no Generative AI was used in the creation of this manuscript.
All claims expressed in this article are solely those of the authors and do not necessarily represent those of their affiliated organizations, or those of the publisher, the editors and the reviewers. Any product that may be evaluated in this article, or claim that may be made by its manufacturer, is not guaranteed or endorsed by the publisher.
AA, Amino acids; AFR, Alternative feed resources; ANF, anti-nutritive factors; CP, Crude protein; CFI, conventional feed ingredients; SBM, soybean meal; LO, Lipid oxidation; WF, Wild fruits; WFBP, Wild fruits and respective byproducts.
Abbas A. O., Alaqil A. A., Kamel N. N., Moustafa E. S. (2022). Citrullus colocynthis seed ameliorates layer performance and immune response under acute oxidative stress induced by paraquat injection. Animals 12, 945. doi: 10.3390/ani12080945
Abdollahi M. R., Ravindran V. (2019). Alternative feed ingredients for poultry diets: challenges and prospects. Animal Nutrition 5, 113–120. doi: 10.1016/j.aninu.2018.12.005
Abdulla J. M., Oskoueian E., Ahmed S. (2017). Potential of mango seed kernel as a feed ingredient for poultry. Trop Anim Health Prod. 49 (7), 1363–1370. doi: 10.1007/s11250-017-1334-4
Abdulazeez S. S., Al-Tameemi H. J. (2023). Utilization of date palm byproducts in sheep diets: Effects on growth and digestibility. Trop Anim Health Prod. 55, 167. doi: 10.1007/s11250-023-03578-4
Adamu E., Asfaw Z., Demissew S., Baye K. (2022). Antioxidant activity and anti-nutritional factors of selected wild edible plants collected from northeastern Ethiopia. Foods 17, 2291–2300. doi: 10.3390/foods11152291
Adejumo I. O., Machebe N. S. (2018). Nutritional evaluation of mango seed kernel meal in broiler diets. Nigerian J. Animal Sci. 20, 245–252.
Adeniji A. A., Lawal T. E. (2012). Proximate and mineral composition of Chrysophyllum albidum fruit pulp and its potential in livestock feed. J. Agric. Sci. 4 (8), 161–166.
Adeniji A. A., Balogun T. F., Ojediran T. K. (2012). Nutritional evaluation of wild african star apple (Chrysophyllum albidum) fruit in broiler diets. Int J Poult Sci. 11 (4), 283–287. doi: 10.3923/ijps.2012.283.287
Adeyeye S. A. O., Oyewole O. B., Obadina A. O. (2021). Nutritional and functional properties of Balanites aegyptiaca seed meal as a feed ingredient. Food Science & Nutrition 9 (3), 1567–1575. doi: 10.1002/fsn3.2130
Akande K. E., Fabiyi E. F. (2010). Effect of processing methods on some antinutritional factors in legume seeds for poultry feeding. Inter. J. Poult. Sci. 9, 996–1001. doi: 10.3923/ijps.2010.996.1001
Amerit J. M., Mpairwe D., Mutetikka D. (2023). Ficus natalensis leaves as a protein supplement for growing goats. Animal Feed Science and Technol. 295, 115543. doi: 10.1016/j.anifeedsci.2022.115543
Aziz ur Rahman M., Khan S. H., Sultan A. (2021). Potential of Ziziphus mauritiana leaves as a feed additive for broilers. Poultry Sci. 100, 765–772. doi: 10.1016/j.psj.2020.11.025
Babiker E. E., Juhaimi F. A. L., Ghafoor K., Abdoun K. A. (2018). Effect of drying methods on nutritional quality of young shoots and leaves of two Moringa species as non- conventional fodders. Agroforest. Syst. 92, 717–729. doi: 10.1007/s10457-016-0043-8
Balehegn M., Ayantunde A., Duncan A. (2021). Livestock Feed Resources in the Drylands: Use of Drought-Tolerant Tree and Shrub Legumes as Supplements. Agroforestry Systems 95 (7), 1223–1235. doi: 10.1007/s10457-021-00646-2
Baleseng L., Madibela O., Tsopito C., Mareko M., Boitumelo W., Letso M. (2023). Morula kernel cake (Sclerocarya birrea) as a protein source in diets of finishing tswana lambs: effects on nutrient digestibility, growth, meat quality, and gross margin. Animals 13, 1387. doi: 10.3390/ani13081387
Banday M. T., Adil S., Sheikh I. U., Hamadani H., Qadri F. I., Sahfi M. E., et al. (2023). The use of silkworm pupae (Bombyx mori) meal as an alternative protein source for poultry. World’s Poult. Sci. J. 00, 1–16. doi: 10.1080/00439339.2023.2163955
Bao T., Hao X., Shishira M. R. I., Karima N., Chen W. (2021). Cold plasma: An emerging pretreatment technology for the drying of jujube slices. Food Chem. 337, 127785. doi: 10.1016/j.foodchem.2020.127783
Bashar M. K., Huque K. S., Sarker N. R., Sultana N. (2020). Quality assessment and feeding impact of Moringa feed on intake, digestibility, enteric CH 4 emission, rumen fermentation, and milk yield. J. Adv. Vet. Anim. Res. 7, 521–529. doi: 10.5455/javar.2020.g449
Batra T. R., Singh B., Gupta A. K. (1986). Utilization of Prosopis cineraria pods in livestock feeding. Indian J Anim Sci. 56 (8), 870–873.
Bellés M., Alonso V., Roncalés P., Beltrán J. A. (2017). Effect of borage and green tea aqueous extracts on the quality of lamb leg chops displayed under retail conditions. Meat Sci. 129, 153–160. doi: 10.1016/j.meatsci.2017.03.003
Bhatti S. A., Khan M. S., Hussain S. M. I. (2018). Nutritional potential of pods in small ruminant diets. Small Ruminant Res. 160, 1–6. doi: 10.1016/j.smallrumres.2017.12.004
Chakraborty S., Gupta S. (2017). Seed meal as a protein source in poultry diets. J. Appl. Anim. Res. 45 (1), 1–5. doi: 10.1080/09712119.2015.1091320
Chibwana M. G., Phiri E. C., Gondwe T. N. (2017). Utilization of fruit by products in goat diets. Livestock Research for Rural Dev. 29 (12).
Chingala G., Raffrenato E., Dzama K., Hoffman L. C., Mapiye C. (2019). Intake, digestibility, rumen protein synthesis, and growth performance of Malawi Zebu steers fed diets containing rangeland-based protein sources. Trop. Anim. Healt. Prod. 51, 199–204. doi: 10.1007/s11250-018-1676-6
Chisoro P., Jaja I. F., Assan N. (2023). Incorporation of local novel feed resources in livestock feed for sustainable food security and circular economy in Africa. Front. Sust. 4, 1251179. doi: 10.3389/frsus.2023.1251179
Chisoro P., Kaguru T., Assan N. (2017). Baobab seeds as an alternative protein source in poultry feed. Sci. J. Rev. 6, 509–518.
Chisoro C. C., Nkukwana T. T. (2020a). Nutritional value of Moringa oleifera leaf meal in broiler diets. S Afr J Anim Sci. 50 (1), 1–10. doi: 10.4314/sajas.v50i1.1
Chisoro C. C., Nkukwana T. T. (2020b). Effects of Moringa oleifera leaf meal on meat quality in broilers. S Afr J Anim Sci. 50 (2), 189–198. doi: 10.4314/sajas.v50i2.2
Chisoro P., Nkukwana T., Mupangwa J. (2019). Feed intake, growth performance, carcass characteristics and organ sizes of broilers fed baobab seed oilcake supplemented finisher diets. Welw. Inter. J. Agric. Sci. 1, 26–34. doi: 10.32642/wijas.v1i0.1358
Chisoro P., Nkukwana T. T., Mupangwa J. F., Mabusela T. P. (2018). Feed intake, growth performance and carcass traits of broilers fed diets with various inclusion levels of baobab seed oilcake. South Afri. J. Anim. Sci. 48, 284–294. doi: 10.4314/sajas.v48i2.9
Chowdhury S. R., Sarker D. K., Chowdhury S. D., Smith T. K., Roy P. K., Wahid M. A. (2005). Effects of dietary tamarind on cholesterol metabolism in laying hens. Poult. Sci. 84, 56–60. doi: 10.1093/ps/84.1.56
Cullere M., Hoffman L. C., Dalle Zotte A. (2013). First evaluation of unfermented and fermented rooibos (Aspalathus linearis) in preventing lipid oxidation in meat products. Meat Sci. 95, 72–77. doi: 10.1016/j.meatsci.2013.04.018
CVB (2018). CVB feed table 2018: Chemical composition and nutritional values of feedstuffs. (Lelystad, Netherlands: CVB (Centraal Veevoederbureau)), 245–395.
D’Mello J. P. F. (2012). Amino Acids in Animal Nutrition. 2nd ed. (Wallingford, UK: CAB International (CABI)).
Dalle Zotte A., Celia C., Szendro Z. (2016). Herbs and spices inclusion as feedstuff or additive in growing rabbit diets and as additive in rabbit meat: A review. Liv. Sci. 189, 82–90. doi: 10.1016/j.livsci.2016.04.024
Desphande S. S., Cheryan M. (1983). Tannin analysis of tree leaves for livestock feed. J Agric Food Chem. 31 (6), 1364–1367. doi: 10.1021/jf00120a046
Dhlakama N., Chawafambira A., Tsotsoro K. (2022). Polyphenols, antioxidant activity, and functional properties of baobab (Adansonia digitata L) seeds soaked in monovalent ion salts. Inter. J. Food Prop. 25, 1365–1376. doi: 10.1080/10942912.2022.2082467
Donkor M. N., Mosobil R., Abaah E. A., Kanati C., Akabanda F., Donkor A. M. (2021). Potential of shea fruit based ingredients for the feed industry. Agric. Food Sec. 54, 1–10. doi: 10.1186/s40066-021-00326-5
Egea M., Linares M. B., Gil M., López M. B., Garrido M. D. (2018). Reduction of androstenone perception in pan-fried boar meat by different masking strategies. J. Sci. Food Agric. 98, 2251–2257. doi: 10.1002/jsfa.2018.98.issue-6
El-Deek A. A., Al-Harthi M. A., Attia Y. A. (2020). Date pits in poultry nutrition: A review. World's Poultry Science J. 76 (2), 305–316. doi: 10.1080/00439339.2020.1716654
Esonu B. O., Etuk E. B., Udedibie A. B. I. (1998). Evaluation of Pawpaw seed meal as a feed ingredient for broilers. Anim Feed Sci Technol. 74 (3), 279–286. doi: 10.1016/S0377-8401(98)00184-7
Estévez M., Lorenzo J. M. (2018). Antioxidant extraction from natural sources to improve meat quality and safety. Meat Science. 145, 295–303. doi: 10.1016/j.meatsci.2018.06.038
Falcon W. P., Naylor R. L., Shankar N. D. (2022). Rethinking global food demand for 2050. Populat. Dev. Review. 48, 921–957. doi: 10.1111/padr.12508
Farghaly M. M., Youssef I. M. I., Radwan M. A., Hamdon H. A. (2022). Effect of feeding Sesbania sesban and reed grass on growth performance, blood parameters, and meat quality of growing lambs. Trop. Anim. Health Prod. 54, 1–13. doi: 10.1007/s11250-021-03006-5
Feedipedia (2023). Animal feed resources information system. Available online at: www.feedipedia.org/node/12665 (Accessed December 13, 2023).
Fernandez N. M., Aranda P., Lopez-Jurado M., Urbano G., Estrella I., Sotomayor C., et al. (1993). “Effect of processing on some antinutritive factors of faba beans: Influence on protein digestibility and food intake in rats,” in Recent advances of research in antinutritional factors in Legume seeds(Wageningen, The Netherlands: Wageningen Per), 467–471.
Food and Agriculture Organisation, (FAO) (2011). Global Food Losses and Food Waste: Extent, Causes and Prevention. (Rome, Italy: FAO).
Food and Agriculture Organization (FAO). (2018). Feed Supplementation Blocks for Ruminants: A Practical Guide for Extension Workers. (Rome, Italy).
Food and Agriculture Organization (FAO). (2019). Sustainable Wildlife Management: A Global Review of Best Practices (Rome, Italy).
Food and Agriculture Organization (FAO). The State of Food Security and Nutrition in the World (SOFI) 2023. (2023). (Rome, Italy).
Fu Y., Cao S., Yang L., Li Z. (2022). Flavor formation based on lipid in meat and meat products: A review. J. Food Biochem. 46, 14439. doi: 10.1111/jfbc.v46.12
García-Moya E., Romero-Manzanares A., Flores-Flores J. L. (2019). Spp. as fodder in arid regions: Nutritional and ecological benefits. J Arid Environ. 167, 1–8. doi: 10.1016/j.jaridenv.2019.04.007
Gebreyowhans S., Zegeye T. (2019). Effect of dried Sesbania sesban leaves supplementation on milk yield, feed intake, and digestibility of Holstein Friesian X Zebu (Arado) crossbred dairy cows. Trop. Anim. Health Prod. 51, 949–955. doi: 10.1007/s11250-018-1779-0
Geron L. J. V., da Costa F. G., Garcia J., de Moura Zanine A., de Oliveira E. B., da Silva Cabral L., et al. (2015). Nutrients intake and digestibility in sheep fed with residue from the extraction of tamarind pulp. Ciências Agrárias. 36, 4401–4412. doi: 10.5433/1679-0359.2015v36n6Supl2p4401
Getachew G., Pittroff W., DePeters E. J. (2013). Nutritional value of Acacia saligna foliage for ruminants. Anim Feed Sci Technol. 180, 18–24. doi: 10.1016/j.anifeedsci.2012.11.004
Glufke G., Sucha M., Mendes F., Forte C. (2020). Technology in society livestock value chain in transition: cultivated (cell-based) meat and the need for breakthrough capabilities. Tech. Society. 62, 101286. doi: 10.1016/j.techsoc.2020.101286
Gravador R. S., Jongberg S., Luciano G. (2015). Dietary inclusion of olive byproducts enhances meat antioxidant capacity in goats. J Agric Food Chem. 63 (12), 3133–3140. doi: 10.1021/jf505672p
Gürbüz E., Özkan G. (2018). Use of carob (Ceratonia siliqua) pulp in poultry diets. Revista Brasileira de Zootecnia 47, e20170136. doi: 10.1590/rbz4720170136
Gutierrez O., Zhang C., Caldwell D. J., Carey J. B., Cartwright A. L., Bailey C. A. (2008). Guar meal diets as an alternative approach to inducing molt and improving Salmonella enteritidis resistance in late-phase laying hens. Poult. Sci. 87, 536–540. doi: 10.3382/ps.2007-00337
Habibi H., Ghoorchi T., Amanlou H. (2024). Wild fig (Ficus carica) byproducts in ruminant diets: Effects on methane emissions and digestibility. Anim Feed Sci Technol. 305, 115732. doi: 10.1016/j.anifeedsci.2023.115732
Hao Y., Wu S., Li X. (2020). Effects of Hippophae rhamnoides residue on growth performance and immunity in pigs. J. Anim. Physiol. Animal Nutr. 104 (2), 568–576. doi: 10.1111/jpn.13285
Hassan L. G., Dangoggo S. M., Muhammad S. (2011). Nutritional and antinutritional composition of Sclerocarya birrea fruit juice. Nig. J. Basic App. Sci. 18, 222–228. doi: 10.4314/njbas.v18i2.64319
Hassan Z. M., Manyelo T. G., Selaledi L., Mabelebele M. (2020). The effects of tannins in monogastric animals with special reference to alternative feed ingredients. Molecules 25, 1–17. doi: 10.3390/molecules25204680
Hendam B. M., El-Sayed M. M., Hassan A. A. (2024). Valorization of date palm byproducts in livestock feed: A meta-analysis. Sustainability. 16 (3), 1023. doi: 10.3390/su16031023
Henry A. J., Udie G. U., Ozung P. O., Anya M. I. (2018). Hormonal assay and reproductive performance of rabbits fed pawpaw (Carica papaya) leaves as feed supplement. Annu. Res. Rev. Biol. 25, 1–9. doi: 10.9734/ARRB/2018/36914
Hoffman L. C., Jones M., Muller N., Joubert E., Sadie A. (2014). Lipid and protein stability and sensory evaluation of ostrich (Struthio camelus) droëwors with the addition of rooibos tea extract (Aspalathus linearis) as a natural antioxidant. Meat Sci. 96, 1289–1296. doi: 10.1016/j.meatsci.2013.10.036
International Livestock Research Institute, (ILRI) (2012). Feeding Practices and Feed Constraints in Smallholder Dairy Systems in East Africa. (Nairobi, Kenya: ILRI).
International Livestock Research Institute (ILRI). (2016). Livestock Feed Resources in the Tropics: Strategies for Improved Utilization. (Nairobi, Kenya).
Islary A., Basumatary S., Brahma R. (2018). Wild edible fruits of northeast india: nutritional composition and potential as livestock feed supplement. Indian J. Animal Nutr. 35, 265–272. doi: 10.5958/2231-6744.2018.00042.8
Jalal M. A. A., Jahromi M. F., Ebrahimi M. (2023). Enhancing poultry nutrition with wild fruit byproducts: a focus on amino acid digestibility. Animals 13 (7), 1223. doi: 10.3390/ani13071223
Jayawardana B. C., Liyanage R., Lalantha N., Iddamalgoda S., Weththasinghe P. (2015). Antioxidant and antimicrobial activity of drumstick (Moringa oleifera) leaves in herbal chicken sausages. Lwt 64, 1204–1208. doi: 10.1016/j.lwt.2015.07.028
Kaczmarska K., Taylor M., Piyasiri U., Frank D. (2021). Flavor and metabolite profiles of meat, meat substitutes, and traditional plant-based high-protein food products available in Australia. Foods 10, (4). doi: 10.3390/foods10040801
Khan N., Zaman R., Elahi M. (1991). Effect of heat treatment on the phytic acid content of maize plants. J. Sci. Food Agric. 54, 153–156. doi: 10.1002/jsfa.2740540117
Kiarie E., Romero L. F., Nyachoti C. M. (2013). The role of added feed enzymes in promoting gut health in swine and poultry. Nutri. Res. Rev. 26, 71–88. doi: 10.1017/S0954422413000048
Kinyuru J. N., Konyole S. O., Roos N. (2018). Nutrient composition of Terminalia brownii fruits and their potential as livestock feed. Food Chem. 238, 143–148. doi: 10.1016/j.foodchem.2016.12.076
Kulczy´nski B., Kobus-Cisowska J., Taczanowski M., Kmiecik D., Gramza-Michałowska A. (2019). The chemical composition and nutritional value of chia seeds-current state of knowledge. Nutrients 11, 1242. doi: 10.3390/nu11061242
Lalev M., Slavov N., Pavlov D. (2022). Utilization of wild fruit byproducts in ruminant diets: A review. Animal Nutr. 10 (3), 456–465. doi: 10.1016/j.aninu.2022.04.007
Livestock Development Institute of Africa (LDIA). (2014). Alternative Feed Resources for Sustainable Livestock Production in Sub-Saharan Africa. (Addis Ababa, Ethiopia).
Luciano A., Natalello A., Mattioli S., Pauselli M., Sebastiani B., Niderkorn V., et al (2020). Dietary inclusion of dried olive cake in growing lamb diets: effects on growth performance and meat quality. J Anim Sci Biotechnol. 11 (1), 1–12. doi: 10.1186/s40104-020-00461-2
Lukuyu B., Kirui J., Franzel S. (2020). Use of Agro-Industrial Byproducts as Feed Supplements for Ruminants in East Africa. Trop. Anim. Health Prod. 52 (3), 1023–1031. doi: 10.1007/s11250-019-02092-w
Mabena P. M., Ratsaka M. M., Nkukwana T. T., Malebana B. D., Nkosi B. D. (2022). Growth performance, nutrient digestibility and carcass characteristics of pigs fed diets containing amarula (Sclerocarya birrea A. Rich) nut cake as replacement to soybean meal. Trop. Anim. Health Prod. 54, 1–10. doi: 10.1007/s11250-021-03016-3
Mabusela S. P., Nkukwana T. T., Mokoma M., Muchenje V. (2018). Layer performance, fatty acid profile and the quality of eggs from hens supplemented with Moringa oleifera whole seed meal. S. Afri. J. Anim. Sci. 48, 234–243. doi: 10.4314/sajas.v48i2.4
Madzimure J., Musimurimwa C., Chivandi E., Gwiriri L., Mamhare E. (2011). Milk yield and quality in Guernsey cows fed cottonseed cake-based diets partially substituted with baobab (Adansonia digitata L.) seed cake. Trop. Anim. Health Prod. 43, 77–82. doi: 10.1007/s11250-010-9656-5
Magonka M. P., Lekule F. P., Mtenga L. A. (2018). Nutritional evaluation of Sclerocarya birrea kernels for poultry. Trop Anim Health Prod. 50 (5), 1019–1025. doi: 10.1007/s11250-018-1528-4
Mahmood M., Samli H. E., Sener-aydemir A., Sharma S., Zebeli Q. (2022). Moringa oleifera and Propolis in cattle nutrition: characterization of metabolic activities in the rumen in vitro. Metabolites 12, 1237. doi: 10.3390/metabo12121237
Marchant-Forde J. N., Boyle L. A. (2020). Sustainability in animal agriculture: Balancing productivity with welfare. Animals 10 (9), 1569. doi: 10.3390/ani10091569
Mazizi B., Chimonyo M., Halimani T. E. (2020). Nutritional evaluation of wild mango (Irvingia gabonensis) seed meal as a partial substitute for maize in broiler diets. S Afr J Anim Sci. 50 (5), 734–742. doi: 10.1016/j.vas.2020.100096
Mazizi B. E., Moyo D., Erlwanger K. H., Chivandi E. (2019). Effects of dietary Sclerocarya birrea caffra (Marula) nut meal on the growth performance and viscera macromorphometry of broiler Japanese quail. J. Appl. Poult. Res. 28, 1028–1038. doi: 10.3382/japr/pfz064
Mbukwane M. J., Nkukwana T. T., Plumstead P. W., Snyman N. (2022). Sunflower meal inclusionrate and the effect of exogenous enzymes on growth performance of broiler chickens. Animals 12, 253. doi: 10.3390/ani12030253
Mcafee A. J., Mcsorley E. M., Cuskelly G. J., Moss B. W., Wallace J. M. W., Bonham M. P., et al. (2010). Red meat consumption: An overview of the risks and benefits. Meat Sci. 84, 1–13. doi: 10.1016/j.meatsci.2009.08.029
McNitt J. I., Lukefahr S. D., Cheeke P. R., Patton N. M. (2013). Rabbit production. 9th ed (London: CABI International).
Mdziniso M. P., Dlamini A. M., Khumalo G. Z., Mupangwa J. F. (2016). Nutritional evaluation of Marula (Sclerocarya birrea) seed cake as a protein supplement in dairy meal. J. Appl. Life Sci. Inter. 4, 1–11. doi: 10.9734/JALSI/2016/23273
Mlambo V., Dlamini B. J., Nkambule M. T., Mhazo N., Sikosana J. L. N. (2011). Nutritional evaluation of marula (Sclerocarya birrea) seed cake as a protein supplement for goats fed grass hay. Trop. Agric. 88, 35–43.
Msweli L. (2021). Nutritional Potential of Wild Fruit Byproducts in Smallholder Goat Production. S Afr J Anim Sci. 51 (4), 567–578. doi: 10.4314/sajas.v51i4.5
Mukumbo F. E., Maphosa V., Hugo A., Nkukwana T. T., Mabusela T. P., Muchenje V. (2014). Effect of Moringa oleifera leaf meal on finisher pig growth performance, meat quality, shelf life and fatty acid composition of pork. S. Afri. J. Anim. Sci. 44, 388–400. doi: 10.4314/sajas.v44i4.9
Mwale M., Mupangwa J. F., Mapiye C., Saina H., Chimvuramahwe J. (2008). Growth performance of Guinea fowl keets fed graded levels of baobab seed cake diets. Inter. J. Poult. Sci. 7, 429–432. doi: 10.3923/ijps.2008.429.432
Nasir N. A. N., Kamaruddin S. A., Zakarya I. A., Islam A. K. M. A. (2022). Sustainable alternative animal feeds: Recent advances and future perspective of using azolla as animal feed in livestock, poultry and fish nutrition. Sust. Chem. Pharm. 25, 100581. doi: 10.1016/j.scp.2021.100581
Nduku X. P., Mabusela S. P., Nkukwana T. T. (2021). Growth and meat quality of broiler chickens fed Moringa oleifera leaf meal, a probiotic and an organic acid. S. Afri. J. Anim. Sci. 50, 710–718. doi: 10.4314/sajas.v50i5.8
Negi R., Pant K. (2017). Ber (Ziziphus mauritiana) fruit pulp as a feed supplement for dairy cattle. Indian Journal of Dairy Sci. 70 (5), 587–592.
Nkukwana T. T. (2019). Global poultry production: Current impact and future outlook on the South African poultry industry. S. Afri. J. Anim. Sci. 48, 869. doi: 10.4314/sajas.v48i5.7
Nkukwana T. T., Muchenje V., Pieterse E., Masika P. J., Mabusela T. P., Hoffman L. C., et al. (2014). Effect of Moringa oleifera leaf meal on growth performance, apparent digestibility, digestive organ size and carcass yield in broiler chickens. Liv. Sci. 161, 139–146. doi: 10.1016/j.livsci.2014.01.001
Nyamukonda K., Ndlovu L. R. (2019). Sclerocarya birrea leaf meal as a protein source for growing pigs. Trop Anim Health Prod. 51 (5), 1143–1150. doi: 10.1007/s11250-019-01904-3
Oddoye E. O. K., Agyente-Badu C. K., Ansah T. (2015). Nutritional evaluation of Blighia sapida aril as a feed ingredient for broilers. J Appl Poult Res. 24 (4), 528–534. doi: 10.3382/japr/pfv054
OECD/FAO (2022). OECD-FAO Agricultural Outlook 2022-2031 (Rome: OECD Publishing, Paris/FAO). doi: 10.1787/f1b0b29c-en
OECD/FAO (2024). OECD-FAO Agricultural Outlook 2024-2033 (Rome: OECD Publishing, Paris/FAO). doi: 10.1787/4c5d2cfb-en
Ogbuewu I. P., Odoemenam V. U., Okoli I. C. (2011). Nutritional potential of Mucuna pruriens seed meal for poultry. Livestock Res. Rural Dev. 23 (1).
Ogunlade J. T., Omojola A. B. (2021). Utilization of Parkia biglobosa pulp in broiler diets. Nigerian J. Anim.Prod. 48 (2), 112–119.
Okeke C. A., Ezekiel C. N., Nwangburuka C. C., Sulyok M., Ezeamagu C. O., Adeleke R. A. (2015). Bacterial diversity and mycotoxin reduction during maize fermentation (steeping) for Ogi production. Front. Microbiol. 6, 1402. doi: 10.3389/fmicb.2015.01402
Oloruntola O. D., Agbede J. O., Onibi G. E., Igbasan F. A., Ayodele S. O., Arogunjo M. A., et al. (2018). Rabbits fed fermented cassava starch residue I: Effect on performance and health. Archivos Zootecnia 67, 578–586. doi: 10.21071/az.v0i0.3890
Omollo E. O., Onyango T. A., Mpairwe D. (2020). Ficus sycomorus Byproducts as Feed Supplements for Improved Rumen Fermentation in Cattle. Agroforestry Systems 94 (5), 1897–1905. doi: 10.1007/s10457-020-00510-9
Patil S. S., Patil V. S. (2017). Emblica officinalis fruit powder as an antioxidant in poultry feed. Vet. World. 10 (6), 689–693. doi: 10.14202/vetworld.2017.689-693
Paul B. K. (2019). Valorization of Wild Fruit Wastes in Livestock Feed: A Circular Economy Approach. In Lichtfouse E. Ed. Sustainable Agriculture Reviews Vol. 38, pp. 145–162.
Paul S. S. (2022). Jackfruit (Artocarpus heterophyllus) waste as a novel feed resource for livestock. Waste Biomass Valorization. 13 (5), 2345–2356. doi: 10.1007/s12649-021-01651-3
Paul T. K., Hasan M., Haque A., Talukder S., Sarker Y. A., Sikder M. H., et al. (2020). Dietary supplementation of Neem (Azadirachta indica) leaf extracts improved growth performance and reduced production cost in broilers. Vet. World. 13, 1050–1055. doi: 10.14202/vetworld.
Pexas G., Doherty B., Kyriazakis I. (2023). The future of protein sources in livestock feeds: implications for sustainability and food safety. Front. Susta. 7, 1–13. doi: 10.3389/fsufs.2023.1188467
Rahman M. M., Alam M. S., Khan M. A. S. (2021). Utilization of banana (Musa spp.) pseudostem as cattle feed: A review. Asian-Australas J Anim Sci. 34 (2), 193–205. doi: 10.5713/ajas.20.0235
Qi K., Liu Y., Li C. (2022). Effects of Lycium barbarum pomace on gut health and immunity in pigs. Front Vet Sci. 9, 825631. doi: 10.3389/fvets.2022.825631
Qwele K., Hugo A., Oyedemi S. O., Moyo B., Masika P. J., Muchenje V. (2013). Chemical composition, fatty acid content and antioxidant potential of meat from goats supplemented with Moringa (Moringa oleifera) leaves, sunflower cake and grass hay. Meat Sci. 93, 455–462. doi: 10.1016/j.meatsci.2012.11.009
Rahaman A., Azmal S. A., Matin S. M. A., Haque N., Amin R., Kabir A. (2022). Effect of Neem and Tamarind leaves as anthelmintic in cattle. J. Dis. Med. Plants. 2, 12–15. doi: 10.11648/j.jdmp.20220801.12
Rahmatnejad E., Habibi H., Torshizi M. A. K., Seidavi A., Hosseinian A. (2024). Effects of the algae derivatives on performance, intestinal histomorphology, ileal microflora, and egg yolk biochemistry of laying Japanese quail. Poult. Sci. 103, 103605. doi: 10.1016/j.psj.2024.103605
Raj D. N., Ramana J. V., Rao S. B. N., Dinesh Kumar D., Suryanarayana M. V. A. N., Ravindra Reddy Y., et al. (2016). Effect of incorporation of detoxified karanja (Pongamia pinnata) and neem (Azadirachta indica) seed cakes in total mixed rations on milk yield, composition and efficiency in crossbred dairy cows. Indian J. Anim. Sci. 86, 489–492. doi: 10.56093/ijans.v86i4.57809
Ramteke S. P., Kaur H., Kaur A. (2019). Utilization of Tamarind (Tamarindus indica) Seed Meal in Poultry Diets. Indian J Anim Sci. 89 (7), 789–794.
Rauw W. M., Mayorga E. J., Dekkers J. C. M. (2023). Sustainable livestock production: balancing productivity with environmental and animal welfare. Front. Vet. Sci. 10, 1156789. doi: 10.3389/fvets.2023.1156789
Resende T. L., Kraft J., Soder K. J., Pereira A. B. D., Woitschach D. E., Reis R. B., et al. (2015). Incremental amounts of ground flaxseed decrease milk yield but increase n-3 fatty acids and conjugated linoleic acids in dairy cows fed high-forage diets. J. Dairy Sci. 98, 4785–4799. doi: 10.3168/jds.2014-9115
Rijsberman F., Frank S. (2016). Reducing Food Loss and Waste: Creating a Sustainable Food Future. (Washington, D.C., USA: World Resources Institute (WRI)).
Rojas O. J., Stein H. H. (2017). Nutritional value of canola meal as a protein source for swine. J. Anim. Sci. 95 (4), 1563–1573. doi: 10.2527/jas.2016.1109
Sadeghi A., Nikkhah A., Shawrang P. (2011). Effects of oak acorn (Quercus brantii) on rumen fermentation and blood parameters in sheep. Small Ruminant Res. 96 (2–3), 136–140. doi: 10.1016/j.smallrumres.2010.11.012
Sattar A., Mahmud R., Mohsin M. A. S., Chisty N. N., Uddin M. H., Irin N., et al. (2021). COVID-19 Impact on poultry production and distribution networks in Bangladesh. Front. Sust. Food Sys 5. doi: 10.3389/fsufs.2021.714649
Saulawa L. A., Ukachukwu S. N., Onwudike O. C., Garba M. G., Aruwayo A. (2014). Quantitative substitution of raw baobab (Adansonia digitata) seed meal for soyabean meal in broiler starter diet. Inter. J. Poult. Sci. 13, 335–339. doi: 10.3923/ijps.2014.335.339
Scerra M., Rao R., Foti F., Caparra P., Cilione C., Natalello A., et al. (2022). Influence of dietary inclusion of exhausted bergamot by-product in pigs on animal performance, fatty acid profile and oxidative stability of meat and meat products. Animals 12, 757. doi: 10.3390/ani12060757
Scerra V., Caparra P., Foti F. (2024). Agroforestry byproducts as feed supplements for dairy cows: Impact on milk quality. J. Sustain.Agric. Environ. 15 (1), 112–125. doi: 10.1111/jsae.2024.15.issue-1
Schiavone A., Guo K., Tassone S., Gasco L., Hernandez E., Denti R., et al. (2008). Effects of a natural extract of chestnut wood on digestibility, performance traits, and nitrogen balance of broiler chicks. Poult. Sci. 87, 521–527. doi: 10.3382/ps.2007-00113
Sebola N. A., Mlambo V., Mokoboki H. K. (2021). Nutritional characterization of Vachellia karroo pods for ruminants. Animals 11 (3), 688. doi: 10.3390/ani11030688
Sharma A., Jood S., Seghal S. (1996). Anti-nutrients (phytic acid, polyphenols) and minerals (Ca, Fe) availability (in-vitro) of chickpea and lentil cultivars. Nahrung 40, 182–184. doi: 10.1002/food.19960400404
Singh B., Singh J. P., Kaur A. (2007). Psidium guajava leaves as a feed additive for goats. Small Ruminant Res. 73 (1–3), 246–250. doi: 10.1016/j.smallrumres.2007.01.015
Stein H. H., Casas G. A., Abelilla J. J. (2016). Nutritional properties of novel feed ingredients and their effects on gut health. J Anim Sci Biotechnol. 7 (1), 29. doi: 10.1186/s40104-016-0088-6
Stelzleni A. M., Johnson D. D. (2008). Effect of days on concentrate feed on sensory off-flavor score, off-flavor descriptor and fatty acid profiles for selected muscles from cull beef cows. Meat Sci. 79, 382–393. doi: 10.1016/j.meatsci.2007.10.023
Stødkilde L., Mogensen L., Krogsdahl J., Ambye-jensen M., Vinther J., Jensen S. K. (2023). Local protein sources for growing-finishing pigs and their effects on pig performance, sensory quality and climate impact of the produced pork. Liv. Sci. 267, 105128. doi: 10.1016/j.livsci.2022.105128
Sugiharto S., Ranjitkar S. (2019). Recent advances in fermented feeds towards improved broiler chicken performance, gastrointestinal tract microecology and immune responses: A review. Anim. Nutri. 5, 1–10. doi: 10.1016/j.aninu.2018.11.001
Sung J. Y., Wiltafsky-Martin M. K., González-Vega J. C., Adeola O. (2022). Autoclaving time- related reduction in metabolizable energy of poultry meal is greater in growing pigs compared with broiler chickens. J. Anim. Sci. 100, 1–9. doi: 10.1093/jas/skac117
Taethaisong N., Paengkoum S., Kaewwongsa W., Onjai-uea N., Sorasak Paengkoum P. (2023). The Effect of Neem leaf supplementation on growth performance, rumen fermentation, and ruminal microbial population in goats. Animals 13, 890. doi: 10.3390/ani13050890
Tang T., Zhang M., Lim C., Mujumdar A. S. (2023). Novel strategies for controlling nitrite content in prepared dishes: Current status, potential benefits, limitations and future challenges. Food Res. Inter. 170, 112984. doi: 10.1016/j.foodres.2023.112984
Taylor J. R. N., Emmambux M. N., Kruger J. (2011). Sorghum and millet byproducts in animal feed. Cereal Chem. 88 (4), 330–335. doi: 10.1094/CCHEM-88-4-0330
Thornton P. K. (2014). Livestock and Climate Change: Mitigation and Adaptation Strategies. (Copenhagen, Denmark: CGIAR Research Program on Climate Change, Agriculture and Food Security (CCAFS)).
United Nations Development Programme (UNDP). (2019). Human Development Report 2019: Beyond Income, Beyond Averages, Beyond Today. (New York, NYa).
United Nations, (UN) (2014). World Urbanization Prospects: The 2014 Revision. (New York, USA: UN Department of Economic and Social Affairs (DESA)).
United Nations Office for the Coordination of Humanitarian Affairs (OCHA). (2023). Global Humanitarian Overview 2023. (Geneva, Switzerland).
United States Department of Agriculture (USDA) (2019). Feed Outlook: March 2019. (Washington, D.C., USA: USDA Economic Research Service (ERS)), 15–34.
United States Department of Agriculture (USDA) (2020). Livestock, Dairy, and Poultry Outlook: December 2020. (Washington, D.C., USA: USDA ERS), 15–34.
United States Department of Agriculture (USDA) (2021). ). World Agricultural Supply and Demand Estimates (WASDE): February 2021. (Washington, D.C., USA: USDA Office of the Chief Economist (OCE)), 15–34.
United States Department of Agriculture (USDA) (2022). Global Agricultural Productivity Report (GAP Report): 2022. (Washington, D.C., USA: USDA), 15–34.
United States Department of Agriculture (USDA) (2023). Feed Yearbook 2023. (Washington, D.C., USA: USDA ERS), 15–34.
United States Department of Agriculture (USDA) (2024a). Feed Outlook: January 2024. (Washington, D.C., USA: USDA ERS), 1–16.
United States Department of Agriculture (USDA) (2024b). Grain: World markets and trade. (Washington, D.C., USA: USDA Foreign Agricultural Service (FAS)), 1–45.
United States Department of Agriculture (USDA) (2024c). World Agricultural Production: January 2024. (Washington, D.C., USA: USDA FAS), 1–38.
Verma S. V. S., Mehta B. M. (1988). Utilization of Salvadora oleoides seed cake in cattle feed. Indian J. Anim. Nutrition 5 (2), 89–93.
Wadhwa M., Bakshi M. P. S., Makkar H. P. S. (2013). Utilization of fruit and vegetable wastes as livestock feed. FAO Animal Production and Health Paper, No. 177.
Wang Y., Tuccillo F., Lampi A., Knaapila A., Pulkkinen M., Kariluoto S., et al. (2022). Flavor challenges in extruded plant-based meat alternatives A review. Comp. Rev. Food Sci. Food Safety. 21, 2898–2929. doi: 10.1111/1541-4337.12964
Wang J., Yang P., Liu J., Yang W., Qiang Y., Jia W., et al. (2024). Study of the flavor dissipation mechanism of soy-sauce-marinated beef using. Food Chem. 437, 137890. doi: 10.1016/j.foodchem.2023.137890
Wapi C., Ng’ambi J. W., Norris D. (2013). Nutritional value of Ximenia caffra fruits for goats. Livestock Science 157 (2–3), 498–504. doi: 10.1016/j.livsci.2013.09.004
Widderich N., Dickhoefer U., Südekum K.-H. (2022). Utilization of fruit processing byproducts in dairy cow diets: effects on milk yield and composition. Anim Feed Sci Technol. 292, 115423. doi: 10.1016/j.anifeedsci.2022.115423
Wong S. E., Lee Y. Y., Thoo Y. Y., Yap M. K. K., Siow L. F. (2023). Moringa oleifera protein isolates: In silico analysis of bioactivity, allergenicity, and toxicity, and application in plant-based burgers. Lwt 186., 115243. doi: 10.1016/j.lwt.2023.115243
World Bank Group, (WBG) (2021). Climate-Smart Agriculture: Prioritizing Investments for Sustainable Livestock Systems. (Washington, D.C., USA: World Bank).
World Economic Forum, (WEF) (2019). Innovation with a Purpose: The Role of Technology in Sustainable Food Systems. (Geneva, Switzerland: WEF)
Xu J., Zhang M., Wang Y., Bhandari B. (2023). Novel technologies for flavor formation in the processing of meat products: A Review. Food Rev. Inter. 39, 802–826. doi: 10.1080/87559129.2021.1926480
Yang L. J., Yang Z. B., Yang W. R., Li H. R., Zhang C. Y., Jiang S. Z., et al. (2018). Conventional solid fermentation alters mycotoxin contents and microbial diversity analyzed by high-throughput sequencing of a Fusarium mycotoxin-contaminated diet. Can. J. Anim. Sci. 98, 354–361. doi: 10.1139/cjas-2017-0093
Keywords: alternative feedstuffs, byproducts, livestock, multipurpose trees, oilseeds, stockfeed, wild fruits
Citation: Chisoro P, Mazizi B, Jaja IF, Assan N and Nkukwana T (2025) Sustainable utilization of wild fruits and respective tree byproducts as partial feed ingredients or supplements in livestock rations. Front. Anim. Sci. 6:1501412. doi: 10.3389/fanim.2025.1501412
Received: 25 September 2024; Accepted: 03 March 2025;
Published: 27 March 2025.
Edited by:
Geoffrey E. Dahl, University of Florida, United StatesReviewed by:
Diwakar Vyas, University of Florida, United StatesCopyright © 2025 Chisoro, Mazizi, Jaja, Assan and Nkukwana. This is an open-access article distributed under the terms of the Creative Commons Attribution License (CC BY). The use, distribution or reproduction in other forums is permitted, provided the original author(s) and the copyright owner(s) are credited and that the original publication in this journal is cited, in accordance with accepted academic practice. No use, distribution or reproduction is permitted which does not comply with these terms.
*Correspondence: Ishmael Festus Jaja, aWphamFAdWZoLmFjLnph
Disclaimer: All claims expressed in this article are solely those of the authors and do not necessarily represent those of their affiliated organizations, or those of the publisher, the editors and the reviewers. Any product that may be evaluated in this article or claim that may be made by its manufacturer is not guaranteed or endorsed by the publisher.
Research integrity at Frontiers
Learn more about the work of our research integrity team to safeguard the quality of each article we publish.