- 1Qinghai Academy of Animal Husbandry and Veterinary Sciences, Qinghai University, Xining, Qinghai, China
- 2Key Laboratory of Plateau Grazing Animal Nutrition and Feed Science of Qinghai, Xining, Qinghai, China
- 3Yak Engineering Technology Research Center of Qinghai, Xining, Qinghai, China
- 4Gansu Province Linxia Hui Autonomous Prefecture and Zheng County Animal Husbandry Industry Development Center, Linxia Hui, China
Introduction: There are few studies on the effect of rumen fluid transplantation on the fecal flora of yaks. Yak fecal flora is closely related to their health. This study aimed to evaluate the effects of rumen fluid transplantation on growth performance and fecal flora indicators.
Methods: Twenty 6-month healthy male yaks (weight: 57.20 ± 7.80 kg) were selected from grazing yaks in an alpine meadow pasture at an altitude of approximately 3,400 m. They were then transferred to a farm and randomly divided into a control group (CON; n = 10) and a rumen fluid transplantation group (RT; n = 10). Separate single-pen rearing was performed in two pens using the same rearing environment and feeding method, and all yaks were earmarked for identification. In addition, 10 yaks that had been adapted to stall fattening feed in 1 month were selected as the rumen fluid donor group to provide fresh rumen fluid. Ruminal fluid transplantation trials were conducted on the 1st, 3rd, and 5th weeks. Overall, 1 L of ruminal fluid was transplanted to each yak in the RT and CON groups. The formal trial then began with both groups fed the same diet. After this, yak feed intake was recorded daily; yaks were weighed on days 1, 30, and 60 of the formal trial; and yak feces were collected directly from the ground on days 1, 4, 7, 14, 30, and 60 to compare the microbial composition of the feces using 16S rRNA sequencing data.
Results: The results showed that rumen fluid transplantation significantly increased the alpha diversity of fecal microflora (P < 0.05), and on day 30 of the experiment, both the operational taxonomic unit (OTU) and Shannon index were significantly higher in the RT group than the CON group (P < 0.05). In the principal coordinate analysis (PCoA) plot, the intestinal flora of the RT group was significantly different (P < 0.05) on days 1–7 but not significantly different after day 14. In contrast, the intestinal flora of the CON group was significantly different (P < 0.05) on days 1–14 but not significantly different after day 30. Compared with the CON group, the relative abundance of Firmicutes in the RT group was significantly lower on days 1, 4, 7, and 14 (P < 0.05); the relative abundance of Bacteroidetes in the RT group was significantly higher on days 1, 4, 14, and 30 and significantly lower on day 7 (P < 0.05); the relative abundance of Tenericutes in the RT group was significantly higher on day 30 (P < 0.05); the relative abundance of Actinobacteria in the RT group was significantly higher on day 60 (P < 0.05); the relative abundance of Ruminococcaceae_UCG-005 in the RT group was significantly lower on days 4, 7, 14, and 60 (P < 0.05); the relative abundance of Unidentified in the RT group was significantly higher on days 1, 4, and 7 days (P < 0.05); and the relative abundance of Rikenellaceae_RC9_gut_group, Bacteroides, and Christensenellaceae_R-7_group in the RT group was significantly higher on day 1 (P < 0.05). Moreover, Actinobacteria was positively correlated with ADG and negatively correlated with DMI; Tenericutes was positively correlated with weight and negatively correlated with F/G. Metabolism of terpenes and polyketones, metabolism of other amino acids, and energy metabolism were higher in the RT group than in the CON group. LEfSe analysis showed that 32 species were more abundant in the RT group and 11 in the CON group. In conclusion, our findings suggest that rumen fluid transplantation improved the stability of the intestinal tract of yaks, improved the immunity of yaks, and reduced the occurrence of intestinal diseases; rumen fluid transplantation remodeled the structure of the intestinal flora, shortened the time of remodeling the intestinal flora of yaks during the transition period, and accelerated yak adaptation to digest housed rations, reducing the DMI. The findings of this study provide new insights into yak microbial community transplantation and a reference for improving feed efficiency in the yak industry.
1 Introduction
Yaks, as ruminants unique to the Tibetan Plateau, have adapted to the region’s harsh environment. The Tibetan Plateau is characterized by high altitude, low temperatures, strong ultraviolet radiation, and severe cold. For the local Tibetan pastoralist community, the yak is an important pillar of economic and cultural life. Most yaks are still grazing; however, the climate of the Tibetan Plateau is such that during the long cold season (from October to May), yaks lose body fat, which severely affects yak performance and can even lead to death (Fan et al., 2019). With the development of ecological animal husbandry, some yak farming methods were gradually shifting from grazing to housed feeding. Housing can improve the production performance of yaks, but during the transition period when the feeding method is shifted from grazing to racking, yaks will suffer from indigestion and anorexia during the transition period because of changes in ration composition, which will lead to a decrease in the amount of food intake of yaks during the transition period and a lack of weight gain, or even nutritional diarrhea, which will greatly reduce the economic benefits of racked yak farming (Depeters and George, 2014).
The rumen is an important digestive organ of ruminants, which digests feed with the help of a rich diversity of microorganisms in the rumen. Ruminal microorganisms mainly include bacteria, archaea, fungi, and protozoa, which act synergistically to degrade feed carbohydrates and proteins, provide nutrients for the host, and ensure nutrient supply to the host to maintain basic metabolic activities, growth, and development. Rumen microbiota transplantation (RMT) is a technique to transplant compliant donor rumen microorganisms to recipient animals, which is mainly used to remodel the rumen flora structure in order to improve the performance and feed utilization efficiency of ruminant production and has a better therapeutic effect on a variety of intestinal diseases (Barnett et al., 1961). Existing studies have shown that flora transplantation is an effective method for restoring dysbiosis in the digestive tract (Cammarota et al., 2014; Khoruts and Sadowsky, 2016). Ruminants depend on rumen and gut microbes for energy and protein (NRC, 2001; France and Kebreab, 2008). Milk production efficiency of recipient Holstein cows can be improved over a period of time by transplanting rumen contents from high-yielding cows and cows with high milk fat content (Weimer et al., 2017). Li et al. (2023) showed that rumen fluid transplantation could remodel the rumen microbiota and accelerate its adaptation to housed diets. Numerous studies have demonstrated the positive effects of rumen microbial transplantation on gastrointestinal function, feed utilization efficiency, and animal health. Rumen fluid transplantation can treat acute rumen acidosis in ruminants (Liu et al., 2019). It has been shown that rumen fluid transplantation accelerates the restoration of rumen microbial homeostasis (Liu et al., 2019). Huang et al. (2022) found that rumen fluid transplantation altered the composition of fecal microbiota and modulated microbial metabolic pathways. Fu et al. (2024) found that transplantation of rumen fluid from adult sheep significantly improved the growth and health of newborn lambs. Therefore, rumen fluid transplantation is important for alleviating metabolic diseases.
Weaning has been shown to alter the intestinal flora composition and increase the incidence of diarrhea in piglets (Dou et al., 2017). The intestinal microbiota plays a key role in maintaining intestinal and physiological homeostasis, as well as nutrient digestion in yaks. Regulating the intestinal microbiota holds significant potential for both production and research applications. Intestinal flora colonization is affected not only by nutrient metabolism and hormones but also by dietary, physical, and chemical forms. Intestinal flora has a regulatory effect on a variety of physiological functions of the host and is mainly involved in the host’s metabolism, immunity, and digestion (Tojo et al., 2014). Healthy intestinal flora can promote host health and improve feed utilization and performance, while disordered intestinal flora can lead to host pathogenic bacterial infections, inflammation, and disease (Gibson et al., 2014). It has been shown that the establishment of a stable symbiotic flora in the digestive tract of ruminants prevents gastrointestinal infections and improves intestinal barrier function, ultimately enhancing productivity (Steele et al., 2016). Therefore, maintaining a balanced intestinal microbial community is important for yaks to prevent digestive diseases and improve production performance and breeding efficiency. In summary, intestinal microbial community is one of the important biological factors affecting yak health and performance. However, a large number of studies have focused on the study of the rumen, with fewer examining the effects of rumen fluid on the intestinal microbiota. Thus, this study aimed to investigate the effects of rumen fluid transplantation on the fecal microbiota of yaks, with the goal of addressing issues such as indigestion, anorexia, and intestinal diseases during the transition from grazing to housed feeding. Additionally, the study seeks to provide technological support for improving the economic benefits of yak farming.
2 Materials and methods
2.1 Animals, diets, and trial design
The Institutional Animal Care and Use Committee of Qinghai University approved all the procedures in this study.
The trial was conducted in December 2021 at Zeku County National Agricultural Industrial Park, Huangnan Prefecture, Qinghai Province, China. The Institutional Animal Care and Use Committee of Qinghai University approved all the procedures in this study.
Twenty 6-month-old healthy male yaks (weight: 57.2 ± 7.8 kg) were selected from an alpine meadow pasture at an altitude of approximately 3,400 m, transferred to a farm, and randomly divided into the control group (CON; n = 10) and a rumen fluid transplantation group (RT; n = 10). The herd was adapted to captive rearing and received a long period of domestication before it was used as part of this trial. Separate single-pen rearing was performed in two pens using the same rearing environment and feeding method, and all yaks were earmarked for identification. In addition, 10 yaks that had been acclimatized to captive breeding feed and grazed in the same alpine meadow before captive breeding were selected as the rumen fluid donor group to provide fresh rumen fluid to the rumen fluid transplantation group.
The trial was divided into two parts: the rumen fluid transplantation trial and a formal trial. The first phase lasted 5 days, and the second lasted 60 days. The rumen fluid transplantation phase began on the first day the yaks arrived at the farm. First, 3.5 L of rumen fluid was collected from the donor group of yaks using a gastric tube sampler, filtered through four layers of sterile gauze, and mixed into a thermos flask preheated to 39°C and set aside. Then, using a gastric tube sampler, approximately 340 mL of fresh rumen fluid was transplanted into the RT group of yaks. All the above steps were completed in the morning before feeding and repeated on days 3 and 5 of rumen fluid transplantation to ensure that each yak in the RT group was transplanted with 1 L of fresh rumen fluid.
After the official trial began, the health and behavior of the yaks were monitored daily. The CON and RT groups were provided with 2.5 kg of concentrate per head at a concentrate:roughage ratio of 65:35. In contrast, the yaks were given free access to water and fed twice daily at 09:00 and 17:00. Each morning, the amount of oat hay and concentrate was left over from the previous day. The amount of input for the day was recorded each morning to determine the daily feed intake of the yak-reading cattle. The diet formulation was based on the Chinese Beef Cattle Feeding Standard (NY/T815-2004), and the composition of the concentrate met the nutrient requirements for yaks, based on the NRC guidelines (NRC, 2001), with a concentrate ratio of 65:35. The composition of the concentrates is listed in Table 1.
2.2 Sample collection and measurement
Feces were collected from the CON and RT groups on days 1, 4, 7, 14, 30, and 60 of the experiment, and fecal samples were collected and placed in a refrigerator (−20°C) within 15 min of defecation. Subsequently, the feces were placed in an insulated box with dry ice and brought back to the laboratory. Fecal aliquots were prepared and stored in a −80°C refrigerator until further processing.
Daily feed offered and refusals were recorded. The yaks were weighed before feeding on the morning of the first day of the formal trial and then measured monthly until the trial ended.
2.3 DNA extraction and sequencing
Fecal genomic DNA was extracted using E.Z.N.A. Soil DNA Kit (Omega Bio-tek, Inc., USA) following the manual. The concentration and quality of the genomic DNA were checked using a NanoDrop 2000 spectrophotometer (Thermo Scientific Inc., USA). DNA samples were stored at −20°C for subsequent experiments. The V3–4 hypervariable region of the bacterial 16S rRNA gene was amplified with the universal primers 338F (5′-ACTCCTACGGGAGGCAGCAG-3′) and 806R (5′-GGACTACNNGGGTATCTAAT-3′). PCR was carried out on an ABI 9700 PCR instrument (the applied cycling parameters were 95°C for 5 min, followed by 28 cycles of 95°C for 45 s, 55°C for 50 s, and 72°C for 45 s with a final extension at 72°C for 10 min). The PCR products were purified using an Agencourt AMPure XP Kit (Beckman Coulter, Inc., USA). Sequencing libraries were generated using the NEB Next Ultra II DNA Library Prep Kit (New England Biolabs, Inc., USA) following the manufacturer’s recommendations. The library quality was assessed by NanoDrop 2000 (Thermo Fisher Scientific Inc., USA), Agilent 2100 Bioanalyzer (Agilent Technologies, Inc., USA), and ABI StepOnePlus Real-Time PCR System (Applied Biosystems, Inc., USA), successively. Deep sequencing was performed on an Illumina MiSeq/NextSeq 2000/NovaSeq 6000 (Illumina, Inc., USA) platform at Beijing Allwegene Technology Co., Ltd. After the run, image analysis, base calling, and error estimation were performed using Illumina Analysis Pipeline Version 2.6 (Illumina, Inc., USA).
2.4 Bioinformatics and data analysis
Sequencing data were filtered and merged using the PEAR (v0.9.6) software (Zhang et al., 2014). Sequences with Phred scores lower than 20, containing ambiguous bases, and primer mismatches were removed. The minimum overlap for splicing was set to 10 bp, and the maximum mismatch rate was 0.1. After splicing, sequences shorter than 230 bp were removed using the Vsearch (Rognes et al., 2016) (v2.7.1) software, and chimeric sequences were removed by comparison using the uchime (Edgar et al., 2011) method according to the GOLD database. Operational taxonomic unit (OTU) clustering was performed on quality sequences using the Vsearch (v2.7.1) software uparse (Edgar, 2013) algorithm with a sequence similarity threshold of 97%. The OTU representative sequences were compared with the Silva138 (Pruesse et al., 2007) database using the BLAST (Ye et al., 2006) algorithm, with the e-value threshold set to 1e−5, to obtain the taxonomic information of the species corresponding to each OTU. Based on the OTUs and their abundance results, α-diversity indices were calculated using the QIIME (v1.8.0) software and plotted using the R (v3.6.0) software (R Core Team, 2021). Species composition histograms were analyzed based on species annotation and relative abundance results using the R (v3.6.0) software; β-diversity distance matrices were calculated distance matrix using the Bray–Curtis dissimilarity by QIIME (v1.8.0) software and plotted based on the distance matrices using the R (v3.6.0) software for principal coordinate analysis (PCoA) analysis. LEfSe (Segata et al., 2011) analysis was performed using the Python (v2.7) software. The LDA effect size was greater than 3.5. Data were normalized prior to the analysis, and mock microbial community controls were used during the analysis to ensure the accuracy of the sequencing results.
2.5 Statistical analysis
The interaction between the CON group or the RT group with days was statistically analyzed using SPSS 21.0, and multiple comparisons between groups were performed using Duncan’s multiple range test, with P < 0.01 indicating a highly significant difference, P < 0.05 indicating a significant difference, and P > 0.05 indicating a non-significant difference.
3 Results
3.1 Effects of rumen fluid transplantation on growth performance of yaks
The DMI of RT group was very significantly lower than that of CON group (P < 0.01), and there was no significant change in IBW, FBW, TWG, ADG and F/G in RT and CON groups (Table 2).
3.2 Effect of rumen fluid transplantation on the diversity and structure of fecal bacteria
A total of 24,422 OTUs were identified in 60 samples. The CON group had 2,965 unique OTUs and the RT group had 7,674 unique OTUs, and the two groups had 13,783 OTUs together (Figure 1A). The difference in OTUs between the two groups was highly significant on day 1 (P < 0.01) and significant on day 30 (P < 0.05). The difference between the two groups was significant for Shannon on day 1 and day 30 (P < 0.05) and for Simpson on day 14 (P < 0.05) (Table 3). UniFrac-based PCoA analysis showed that the contribution of the first and second principal components was 13.98% and 9.26%, respectively, indicating reliable data (Figure 1B).
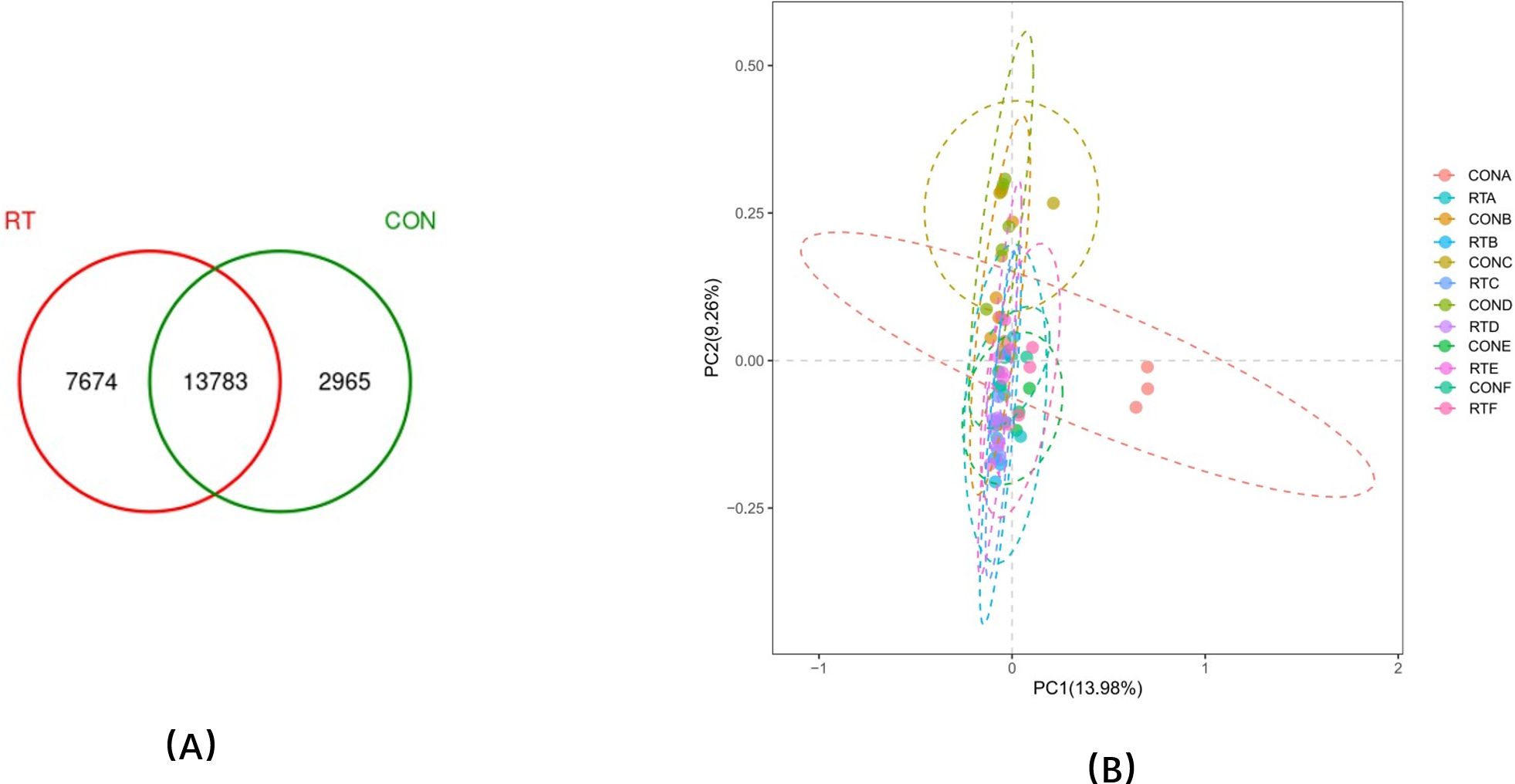
Figure 1. (A) OTU Venn diagram and (B) A, B, C, D, E, and F in the control group (CON group) and experimental group (RT group) were represented by feces microflora differences on days 1, 4, 7, 14, 30, and 60, respectively, calculated by weighted UniFrac distance and principal coordinate analysis (PCoA).
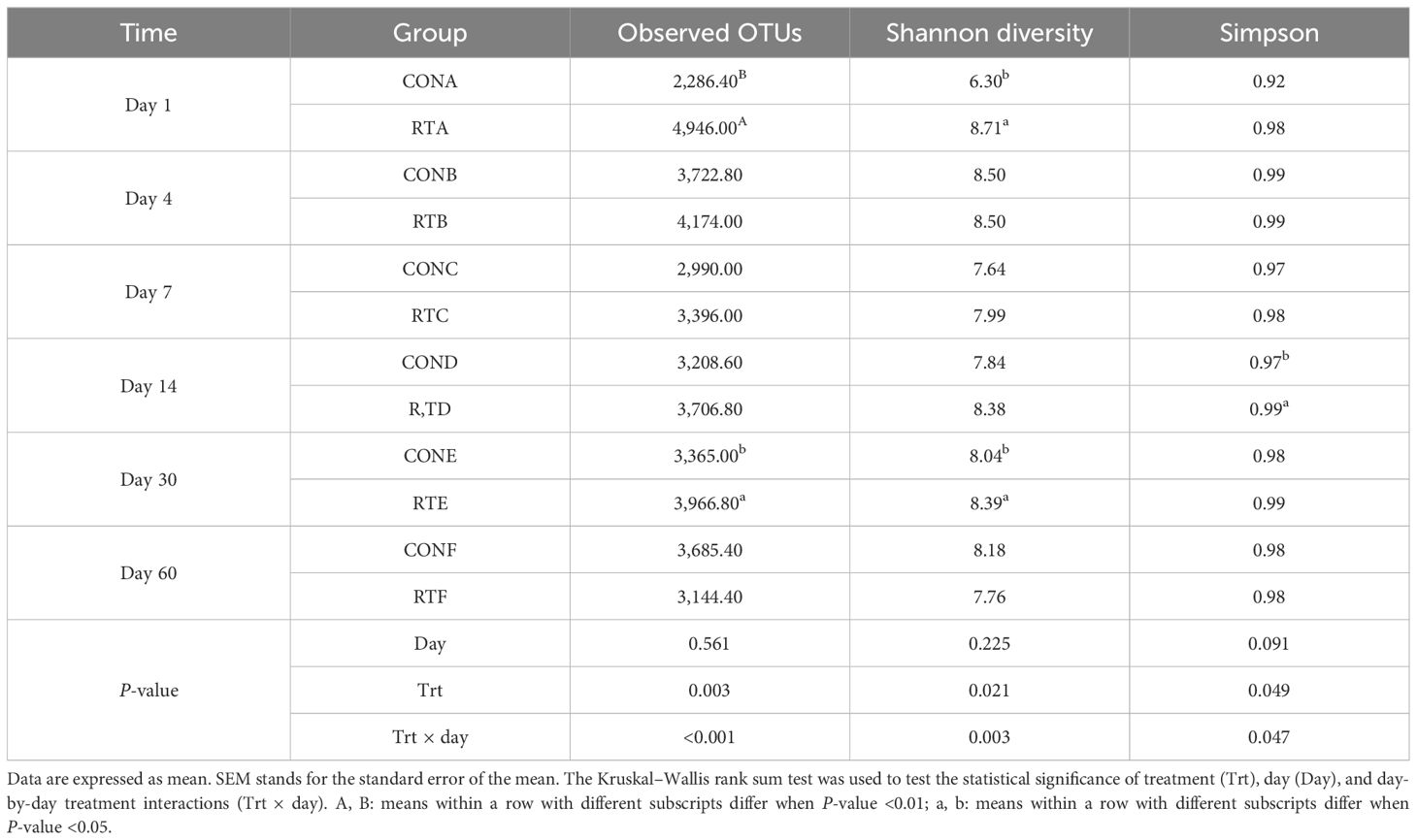
Table 3. Effects of rumen fluid transplantation on the diversity alpha feces microbiota of yak feces.
3.3 Effect of rumen fluid transplantation on the composition of yak fecal flora
The top 3 phyla in relative abundance in feces were Firmicutes, Bacteroidetes, and Spirochaetes (Table 4). Compared to the CON group, the relative abundance of Bacteroidetes in the RT group was significantly higher on days 1, 4, 14, and 30 (P < 0.05), but highly significantly decreased on day 7 (P < 0.01); on day 60, the relative abundance of Actinobacteria in the RT group increased highly significantly (P < 0.01). Compared to the CON group, on days 1, 4, 7, and 14, the relative abundance of Firmicutes was significantly lower in the RT group (P < 0.05). Significant differences in the relative abundance of Firmicutes (P = 0.001), Bacteroidetes (P = 0.001), and Actinobacteria (P < 0.01) were observed in the interaction between treatment groups and sampling days.
The top 4 genera in relative abundance in the feces were Ruminococcaceae_UCG-005, Unidentified, Rikenellaceae_RC9_gut_group, and Bacteroides (Table 5). Compared with the CON group, the RT group had a higher relative abundance of Unidentified (P < 0.01), Alistipes (P = 0.007), Ruminococcaceae (P = 0.018), Eubacterium_coprostanoligenes_group (P < 0.01), and Christensenellaceae_R-7_group (P = 0.046). Compared with the CON group, the relative abundance of Unidentified was significantly higher (P < 0.05) in the RT group on days 1, 4, and 7; the relative abundance of Alistipes was significantly higher (P < 0.05) in the RT group on day 14; the relative abundance of Ruminococcaceae and Christensenellaceae_R-7_group was significantly higher (P < 0.05) in the RT group on day 1; and the relative abundance of Eubacterium_coprostanoligenes_group was significantly higher (P < 0.05) in the RT group on days 14 and 30. Compared to the CON group, the relative abundance of Ruminococcaceae_UCG-005 in the RT group was significantly lower (P < 0.05) on days 4, 7, 14, and 60. In the interaction between treatment groups and sampling days, Ruminococcaceae_UCG-005 (P < 0.01), Unidentified (P = 0.004), Rikenellaceae_RC9_gut_group (P < 0.01), Bacteroides (P = 0.007), and Christensenellaceae_R-7_group (P = 0.010) were significantly different in relative abundance.
With a threshold value of LEfSe greater than 3.5, there were 32 dominant flora in the RT group, including p:Verrucomicrobia, p:Actinobacteria, p:Spirochaetae, and p:Bacteroidetes at the phylum level and g:Lachnospiraceae_UCG_004, g:Prevotellaceae_UCG_001, g:Phocaeicola, g:Akkermansia, g:Bifidobacterium, g:dgA_11_gut_group, g:Prevotellaceae_UCG_004, g:Treponema_2, g:Bacteroides, and g:Alistipes at the genus level (Figure 2). The CON group had 11 dominant flora, with higher contents at the phylum level in p:Firmicutes and p:Proteobacteria. At the genus level, higher contents were found in g:Ruminococcaceae_UCG_005, g:Lachnospiraceae_FCS020_group, g:Prevotellaceae_YAB2003_group, g:Lachnoclostridium, and g:Coprococcus_1.
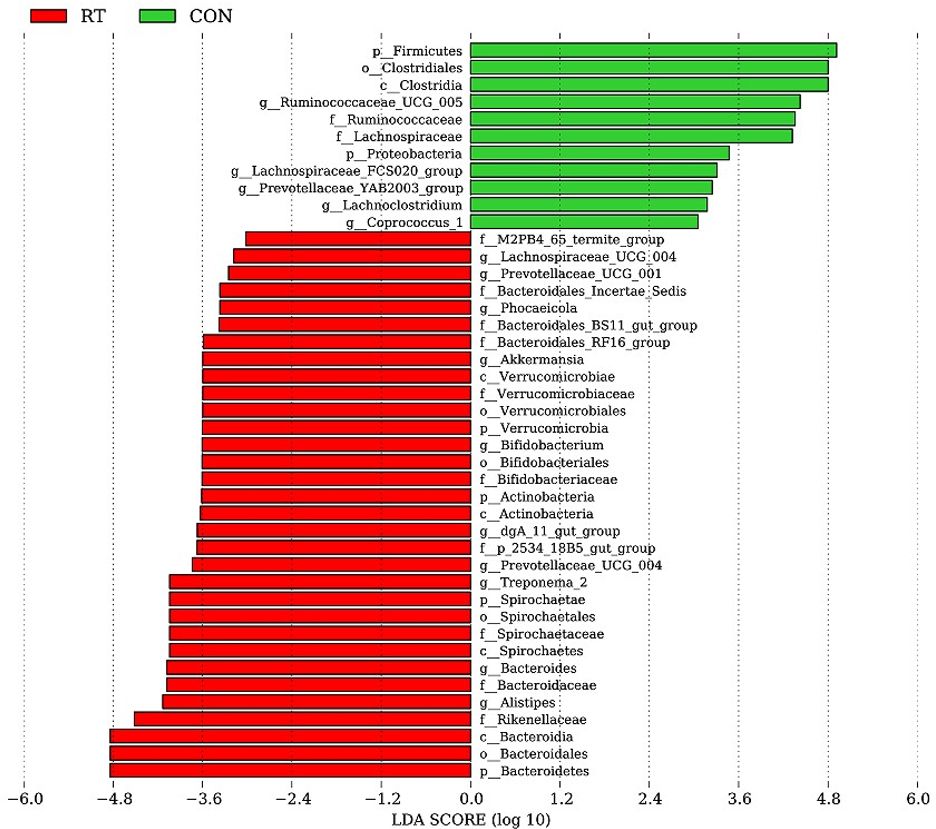
Figure 2. LEfSe analysis of the feces microbiota of yaks in the CON and RT groups. Histogram of linear discriminant analysis scores based on categorical information.
3.4 Correlation heat map analysis
Correlations between fecal microbes and growth performance were analyzed using correlation heat maps (Figure 3). The results showed that transplanted rumen fluid affected the correlation between microbial communities and growth performance. We found that Actinobacteria showed a highly significant positive correlation with ADG (P < 0.01) and a highly significant negative correlation with dry matter intake (DMI) (P < 0.01). Tenericutes showed a highly significant positive correlation with weight (P < 0.01) and a highly significant negative correlation with F/G (P < 0.01). The Eubacterium_coprostanoligenes_group showed a highly significant positive correlation with F/G (P < 0.01).
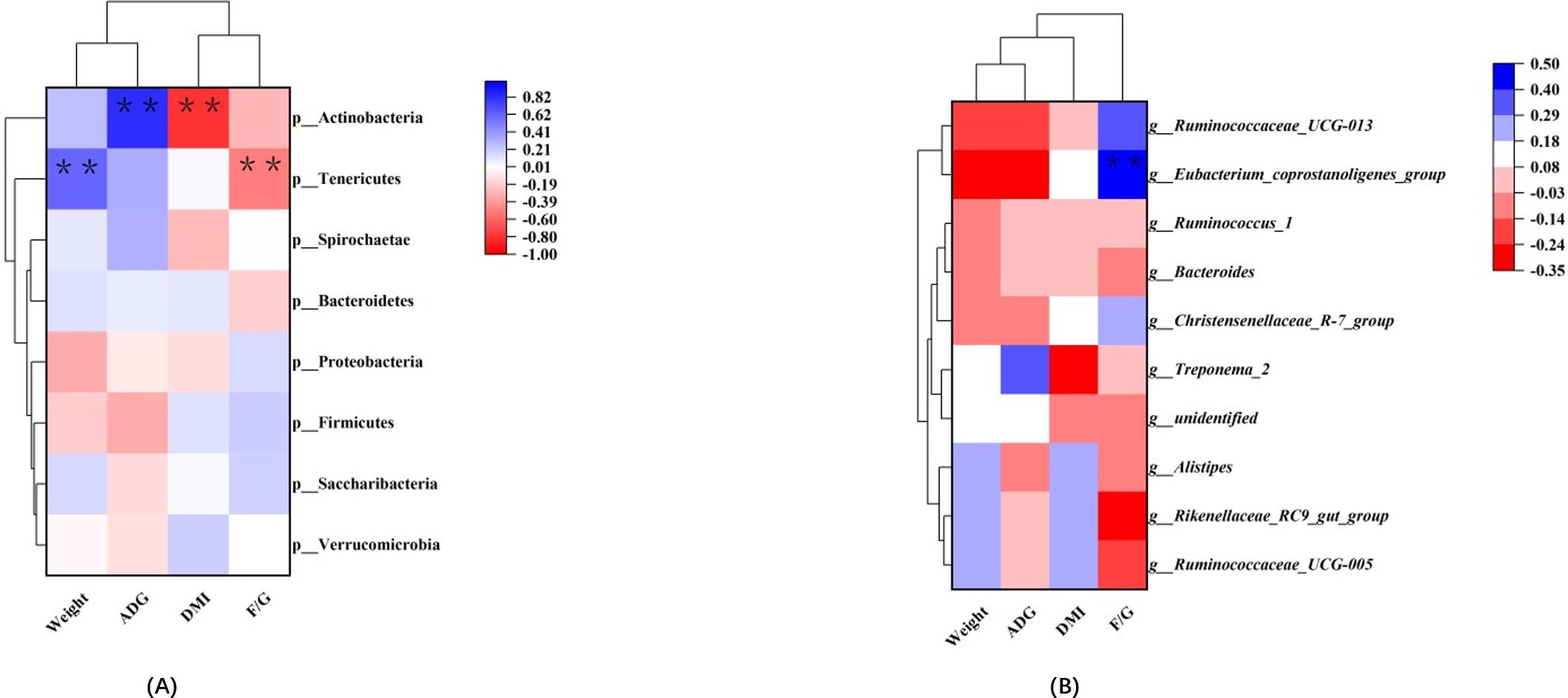
Figure 3. Correlation between rumen bacteria and growth performance. Each row in the graph represents a phylum (A); each row in the graph represents a genus (B). Each column represents a growth performance indicator, and each grid represents the Pearson correlation coefficient between a component and an indicator. Blue represents a positive correlation, while red represents a negative correlation. * and ** indicate 0.05 and 0.01 levels of significance.
3.5 PICRUSt2 function prediction
PICRUSt2 was used to predict the function of yak fecal flora in the RT and CON groups, enriched the major pathways in KEGG, and compared the differences that existed before and after rumen fluid transplantation in yaks (Supplementary Figure 1). Classification of KEGG metabolism at the secondary level showed that 35 pathways differed significantly between the two groups (P < 0.05), with a significant difference in the RT group between carbohydrate metabolism, amino acid metabolism, metabolism of cofactors and amino acid, metabolism of cofactors and vitamins, metabolism of terpenoids and polyketides, metabolism of other amino acids, and replication and repair. Glycan biosynthesis and metabolism and xenobiotics biodegradation and metabolism were upregulated in the RT group compared to the CON group. Carbohydrate metabolism, amino acid metabolism, and replication and repair metabolic pathways were upregulated in the CON group compared to the RT group.
4 Discussion
Steiner et al. (2020) found that recipient cows after rumen fluid transplantation remodeled rumen microbes and significantly improved rumen function by increasing feed frequency and dry matter intake in periparturient cows, as well as dry matter intake in dyspeptic cows and rumen protozoa counts. Xu and Gordon (2003) found that rumen fluid transplantation had a positive effect on intestinal development in preweaned calves. Li et al. (2023) found that rumen fluid transplantation in yaks could accelerate the body’s adaptation to digesting housed diets, thus improving the feed efficiency of yaks. Intestinal flora is an important component in maintaining intestinal function. It has been shown that rumen fluid transplantation in ruminants could reshape the rumen microbial community structure, thus improving body health and growth performance. However, there were fewer studies on the effects of rumen fluid transplantation on the intestinal tract of ruminants. Intestinal flora plays an important role in the physiological health of animals and the stability of the intestinal environment, and disorders of the gastrointestinal flora have been shown to be associated with a variety of host diseases. We investigated the effects of rumen fluid transplantation on the intestinal flora of yaks using 16S rDNA high-throughput sequencing, and the results showed that rumen fluid transplantation altered the structure and composition of the intestinal flora of yaks. In this study, there was a significant difference in the α and β microbial community diversity indices between the two groups, and the OTU, Shannon, and Simpson indices were significantly higher and richer in microbial species in the RT group compared to the CON group. The results of PCoA showed that the fecal bacterial community structure of yaks was not similar in the CON and RT groups during the 7 days after rumen fluid transplantation; however, fecal samples from the two groups began to converge after 7 days of rumen fluid transplantation, suggesting that the intestinal flora was being remodeled. Yaks in the RT group had already completed the process of intestinal remodeling and had reached a steady state by day 7. In contrast, yaks in the CON group required at least 14 days to reach a similar steady state, and the bacterial community was richer in the RT group. This was consistent with the results of Li et al. (2023). It also suggests that the intestinal flora balance can rapidly respond to the disturbance of fresh rumen fluid and reach a new intestinal flora equilibrium state. At the phylum level, Firmicutes was mainly involved in the degradation of fibrous materials and the promotion of energy absorption. The relative abundance of Firmicutes was significantly lower in the RT group than in the CON group between 1 and 14 days, and the difference between the two groups was not significant between 30 and 60 days. Bacteroidetes has the ability to degrade non-fiber plant polysaccharides and proteins and participate in nutrient metabolism, which helps to promote the absorption of feed nutrients by the organism and improve the digestibility of nutrients. The relative abundance of Bacteroidetes in the RT group was significantly higher than that in the CON group from 1 to 30 days, and the difference was not significant on day 60. The test showed that the gut microorganisms of the RT group were better at digesting non-fiber plant polysaccharides and proteins than the CON group. Actinobacteria are one of the four major phyla of the gut microbiota and play a key role in maintaining intestinal homeostasis (Binda et al., 2018). Studies have shown that Acinetobacter in the intestinal flora not only facilitates the host to carry out metabolism and promote the maturation of immune cells but also helps to improve the nutrient utilization of the host and regulate the balance of energy and nutrient metabolism (Li et al., 2023). On day 60, the relative abundance of Acinetobacter in the RT group was significantly higher than that in the CON group.
At the genus level, Bacteroides are involved in metabolizing polysaccharides and oligosaccharides to provide nutrients to the host and also play a specific role in the development of systemic diseases such as immune disorders and metabolic syndrome, as well as neurological disorders (Zafar and Saier, 2021; Gibiino et al., 2018). The relative abundance of Bacteroides in the RT group was significantly higher than that in the CON group on day 1. With the time increased, the RT group Bacteroides showed a decreasing trend and the CON group Bacteroides showed an increasing trend, but the differences were not significant. Eubacterium_coprostanoligenes_group belongs to the genus Fungi, which produces short-chain fatty acids as a specific nutrient and energy component in the intestinal epithelium, protects the intestinal mucosal barrier, helps to resist microbial invasion, reduces the incidence of human inflammation, and enhances gastrointestinal motility (Wei et al., 2021; Bai et al., 2023). The relative abundance of Eubacterium_coprostanoligenes_group in the RT group was significantly higher than that in the CON group on days 14–30. It was shown that rumen fluid transplantation could reduce the probability of intestinal inflammation in yaks. Christensenellaceae_R-7_group is widely found in the intestinal tract and is one of the healthy intestinal flora. It reflects animal health and reduces the probability of cardiovascular and metabolic diseases associated with visceral fat (Biddle et al., 2013; Tavella et al., 2021). On the first day, the relative abundance of Christensenellaceae_R-7_group was significantly higher in the RT group than in the CON group, and there was a tendency for the relative abundance of this bacterial genus to increase with time, but not significantly.
In this study, the differences in the composition of fecal flora between the two groups of yaks were analyzed by LEfSe. In the RT group, the dominant flora were Bacteroidetes, g:Ruminococcaceae_UCG_005, g:Prevotellaceae_UCG_001, g:Prevotellaceae_UCG_004, and f:p_2534_18B5_gut_group. Bacteroidetes had immunomodulatory and anti-inflammatory multiple functions (Li and Guan, 2017). g:Ruminococcaceae_UCG_005 belongs to the family of Rumenococcaceae, the members of which are characterized by cellulose-degrading and hemicellulose-degrading activities, producing acetate, butyrate formate, and hydrogen, and the relative abundance of Ruminococcaceae_UCG_005 was positively correlated with the relative concentration of linoleic acid, which plays an important role in lipid metabolism. g:Prevotellaceae_UCG_001 and g:Prevotellaceae_UCG_004 were able to degrade starch, protein, cellulose, hemicellulose cellulose, etc (Forsyth et al., 2011). and had the ability to assist in the degradation of fibers. f:p_2534_18B5_gut_group was highly positively correlated with total short-chain fatty acids (Wang et al., 2022). In the correlation heat map analysis, Actinobacteria was significantly positively correlated with ADG and negatively correlated with DMI; Tenericutes was significantly positively correlated with weight and negatively correlated with F/G. Compared with the CON group, the relative abundance of Actinobacteria and Tenericutes was higher in the RT group. This suggests that rumen fluid transplantation can reduce DMI in housed yaks and thus improve economic efficiency.
The prediction of bacterial function in yak feces from the RT and CON groups showed that the metabolisms of terpenoids and polyketides, other amino acids, and energy were higher in the RT group than in the CON group. Terpenes in animals contain a number of hormones, vitamins, steroids, etc. Steroids include sterols, bile acids, and steroid hormones. Sterols are mainly cholesterol, which is an indispensable substance for constituting cell membranes of animal tissues and is also the basis for the synthesis of bile acids, vitamin D3, and other physiologically active substances; bile acid salts promote lipid emulsification and lipid absorption in the digestive tract. The metabolism of other amino acids as well as terpenoids and polyketides will promote the metabolism of nutrients such as sugars, lipids, and proteins in the liver and kidneys of the animal organism, which is beneficial to the growth performance of the animal (Wester et al., 2015; Dogra et al., 2020). Energy is indispensable for animal growth and development, and rumen fluid transplantation upregulated energy metabolic pathways in yaks, which in turn improved energy utilization. We analyzed the functional pathways that were significantly different between fecal groups in different months in the two groups of yaks, and found that the differences in functional pathways were related to changes in the structure of intestinal flora. In order to adapt to environmental changes, the characteristic flora of different feeding stages influenced functional pathways. Therefore, transplanting rumen fluid could influence the function of intestinal microorganisms in yaks and improve the metabolic efficiency of nutrients in yaks, thus improving their growth performance.
5 Conclusion
The study showed that rumen fluid transplantation increased the relative abundance of Bacteroidetes, Actinobacteria, Tenericutes, Bacteroides, Eubacterium_coprostanogenes_group, and Christensenellaceae_R-7_group in the feces; improved the yak’s ability to digest non-fiber plant polysaccharides and proteins; and had a positive impact on maintaining intestinal stability. Ruminal fluid transplantation could remodel the structure of intestinal flora, shorten the time of remodeling intestinal flora of yaks during the transition period, and accelerate the speed of yaks adapting to digesting housed diets.
Data availability statement
The original contributions presented in the study are publicly available. This data can be found here: NCBI BioProject, accession PRJNA1151633.
Ethics statement
The Institutional Animal Care and Use Committee of Qinghai University approved all the procedures in this study. The studies were conducted in accordance with the local legislation and institutional requirements. Written informed consent was obtained from the owners for the participation of their animals in this study.
Author contributions
MZ: Writing – original draft, Writing – review & editing, Conceptualization, Data curation, Formal analysis, Investigation, Methodology, Software, Supervision, Validation. YaL: Data curation, Investigation, Supervision, Writing – review & editing. YuL: Data curation, Investigation, Supervision, Writing – review & editing. CC: Data curation, Formal analysis, Validation, Writing – review & editing. SC: Funding acquisition, Resources, Writing – review & editing. YY: Supervision, Writing – review & editing. XW: Supervision, Writing – review & editing. SW: Formal analysis, Funding acquisition, Project administration, Resources, Supervision, Validation, Writing – review & editing.
Funding
The author(s) declare financial support was received for the research, authorship, and/or publication of this article. Integration and Demonstration of Key Technologies for Improving Quality and Efficiency of Specialty Farming in Zeku, Huangnan Zhou Zizhizhou, Qinghai
Acknowledgments
We thank the staff of the National Yak Industrial Par, Zeku County, Qinghai Province, China, for their help in feeding and managing the experimental yaks.
Conflict of interest
The authors declare that the research was conducted in the absence of any commercial or financial relationships that could be construed as a potential conflict of interest.
Generative AI statement
The author(s) declare that no Generative AI was used in the creation of this manuscript.
Publisher’s note
All claims expressed in this article are solely those of the authors and do not necessarily represent those of their affiliated organizations, or those of the publisher, the editors and the reviewers. Any product that may be evaluated in this article, or claim that may be made by its manufacturer, is not guaranteed or endorsed by the publisher.
Supplementary material
The Supplementary Material for this article can be found online at: https://www.frontiersin.org/articles/10.3389/fanim.2024.1507838/full#supplementary-material
References
Bai D., Zhao J., Wang R., Du J., Zhou C., Gu C., et al. (2023). Eubacterium coprostanoligenes alleviates chemotherapy-induced intestinal mucositis by enhancing intestinal mucus barrier. Acta Pharm. Sinica. B 14, 1677–1692. doi: 10.1016/j.apsb.2023.12.015
Biddle A. S., Stewart L. C., Blanchard J. L., Leschine S. B. (2013). Untangling the genetic basis of fibrolytic specialization by lachnospiraceae and ruminococcaceae in diverse gut communities. Diversity 5, 627–640. doi: 10.3390/d5030627
Binda C., Lopetuso L. R., Rizzatti G., Gibiino G., Cennamo V., Gasbarrini A. (2018). Actinobacteria: A relevant minority for the maintenance of gut homeostasis. Digestive liver Dis. 50, 421–428. doi: 10.1016/j.dld.2018.02.012
Cammarota G., Ianiro G., Bibbò S., Gasbarrini A. (2014). Gut microbiota modulation: probiotics, antibiotics or fecal microbiota transplantation? Internal Emergency Med. 9, 365–373. doi: 10.1007/s11739-014-1069-4
Depeters E. J., George L. W. (2014). Rumen transfaunation. Immunol. Lett. 162, 69–76. doi: 10.1016/j.imlet.2014.05.009
Dogra N. K., Kumar S., Kumar D. (2020). Vernonia anthelmintica (L.) Willd.: An ethnomedicinal, phytochemical, pharmacological and toxicological review. J. ethnopharmacology 256, 112777. doi: 10.1016/j.jep.2020.112777
Dou S., Gadonna-Widehem P., Romé V., Hamoudi D., Rhazi L., Lakhal L., et al. (2017). Characterisation of early-life fecal microbiota in susceptible and healthy pigs to post-weaning diarrhoea. PloS One 12. doi: 10.1371/journal.pone.0169851
Edgar R. C. (2013). UPARSE: highly accurate OTU sequences from microbial amplicon reads. Nat. Methods 10, 996–998. doi: 10.1038/nmeth.2604
Edgar R. C., Haas B. J., Clemente J. C., Quince C., Knight R. (2011). UCHIME improves sensitivity and speed of chimera detection. Bioinformatics 27, 2194–2200. doi: 10.1093/bioinformatics/btr381
Fan Q., Wanapat M., Hou F. (2019). Mineral nutritional status of yaks (Bos grunniens) grazing on the qinghai-tibetan plateau. Animals 9 (7), 468. doi: 10.3390/ani9070468
Forsyth C. B., Shannon K. M., Kordower J. H., Voigt R. M., Shaikh M. W., Jaglin J. A., et al. (2011). Increased intestinal permeability correlates with sigmoid mucosa alpha-synuclein staining and endotoxin exposure markers in early parkinson’s disease. PloS One 6 (12), e28032. doi: 10.1371/journal.pone.0028032
Fu L., Liu L., Zhang L., Hu Y., Zeng Y., Ran Q., et al. (2024). Inoculation of newborn lambs with ruminal solids derived from adult goats reprograms the development of gut microbiota and serum metabolome and favors growth performance. J. Agric. Food Chem. 72, 983–998. doi: 10.1021/acs.jafc.3c04632
Gibiino G., Lopetuso L. R., Scaldaferri F., Rizzatti G., Binda C., Gasbarrini A. (2018). Exploring Bacteroidetes: Metabolic key points and immunological tricks of our gut commensals. Digestive liver Dis. 50, 635–639. doi: 10.1016/j.dld.2018.03.016
Gibson M. K., Pesesky M., Dantas G. (2014). The yin and yang of bacterial resilience in the human gut microbiota. J. Mol. Biol. 426 23, 3866–3876. doi: 10.1016/j.jmb.2014.05.029
Huang S., Zheng G., Men H., Wang W., Li S. (2022). The response of fecal microbiota and host metabolome in dairy cows following rumen fluid transplantation. Front. Microbiol. 13. doi: 10.3389/fmicb.2022.940158
Khoruts A., Sadowsky M. J. (2016). Understanding the mechanisms of faecal microbiota transplantation. Nat. Rev. Gastroenterol. Hepatol. 13, 508–516. doi: 10.1038/nrgastro.2016.98
Li F., Guan L. L. (2017). Metatranscriptomic profiling reveals linkages between the active rumen microbiome and feed efficiency in beef cattle. Appl. Environ. Microbiol. 83, e00061-17. doi: 10.1128/AEM.00061-17
Li J., Huang X., He L. J., Li C., Jing H., Lin J., et al. (2023). Effect of ellagic acid on body weight, nutrient digestibility, fecal microbiota, and urolithin A metabolism in Thoroughbred horses. J. Anim. Sci. 101, skad232. doi: 10.1093/jas/skad232
Li Y., Yang Y., Chai S., Pang K., Wang X., Xu L., et al. (2023). Ruminal fluid transplantation accelerates rumen microbial remodeling and improves feed efficiency in yaks. Microorganisms 11 (8), 1964. doi: 10.3390/microorganisms11081964
Liu J., Li H., Zhu W., Mao S. (2019). Dynamic changes in rumen fermentation and bacterial community following rumen fluid transplantation in a sheep model of rumen acidosis: implications for rumen health in ruminants. FASEB J. 33, 8453–8467. doi: 10.1096/fj.201802456R
NRC. (2001). Nutrient Requirements of Dairy Cattle. 7th rev. ed (Washington, D.C: National Academy Press).
Pruesse E., Quast C., Knittel K., Fuchs W, Fuchs Ludwig W, Peplies J, et al. (2007). SILVA: a comprehensive online resource for quality checked and aligned ribosomal RNA sequence data compatible with ARB. Nucleic Acids Res. 35, 7188–7196. doi: 10.1093/nar/gkm864
R Core Team. (2021). R: A Language and Environment for Statistical Computing (Vienna, Austria: R Foundation for Statistical Computing).
Rognes T., Flouri T., Nichols B., Quince C, Mahé F. (2016). VSEARCH: a versatile open source tool for metagenomics. Peer J. 4, e2584. doi: 10.7717/peerj.2584
Segata N., Izard J., Waldron L., Dirk Gevers , Miropolsky L., Garrett W. S., et al. (2011). Metagenomic biomarker discovery and explanation. Genome Biol. 12, 1–18. doi: 10.1186/gb-2011-12-6-r60
Steele M. A., Penner G. B., Chaucheyras-Durand F., Guan L. L. (2016). Development and physiology of the rumen and the lower gut: Targets for improving gut health. J. dairy Sci. 99, 4955–4966. doi: 10.3168/jds.2015-10351
Steiner S., Linhart N., Neidl A., Baumgartner W, Tichy A, Wittek T. (2020). Evaluation of the therapeutic efficacy of rumen transfaunation. J. Anim. Physiol. Anim. Nutr. 104, 56–63. doi: 10.1111/jpn.v104.1
Tavella T., Rampelli S., Guidarelli G., Bazzocchi A., Gasperini C., Pujos-Guillot E., et al. (2021). Elevated gut microbiome abundance of Christensenellaceae, Porphyromonadaceae and Rikenellaceae is associated with reduced visceral adipose tissue and healthier metabolic profile in Italian elderly. Gut Microbes 13. doi: 10.1080/19490976.2021.1880221
Tojo R., Suárez A., Clemente M. G., de los Reyes-Gavilán C. G., Margolles A., Gueimonde M., et al. (2014). Intestinal microbiota in health and disease: role of bifidobacteria in gut homeostasis. World J. Gastroenterol. 20 (41), 15163–15176. doi: 10.3748/wjg.v20.i41.15163
Wang Z., Chen Y., Wang W., Huang C., Hu Y., Johnston L. J., et al. (2022). Dietary supplementation with fine-grinding wheat bran improves lipid metabolism and inflammatory response via modulating the gut microbiota structure in pregnant sow. Front. Microbiol. 13. doi: 10.3389/fmicb.2022.835950
Wei W., Jiang W., Tian Z., Wu H., Ning H., Yan G., et al. (2021). Fecal g. Streptococcus and g. Eubacterium_coprostanoligenes_group combined with sphingosine to modulate the serum dyslipidemia in high-fat diet mice. Clin. Nutr. 40 (6), 4234–4245. Available online at: https://api.semanticscholar.org/CorpusID:231969357.
Weimer P. J., Cox M. S., Vieira de Paula T., Lin M., Hall M. B., Suen G. (2017). Transient changes in milk production efficiency and bacterial community composition resulting from near-total exchange of ruminal contents between high- and low-efficiency Holstein cows. J. dairy Sci. 100, 7165–7182. doi: 10.3168/jds.2017-12746
Wester T. J., Kraft G., Dardevet D., Polakof S., Ortigues-Marty I., Rémond D., et al. (2015). Nutritional regulation of the anabolic fate of amino acids within the liver in mammals: concepts arising from in vivo studies. Nutr. Res. Rev. 28, 22–41. doi: 10.1017/S0954422415000013
Xu J., Gordon J. I. (2003). Honor thy symbionts. Proc. Natl. Acad. Sci. United States America 100, 10452–10459. doi: 10.1073/pnas.1734063100
Ye J., McGinnis S., Madden T. L. (2006). BLAST: improvements for better sequence analysis. Nucleic Acids Res. 34, W6–W9. doi: 10.1093/nar/gkl164
Zafar H., Saier M. H. (2021). Gut Bacteroides species in health and disease. Gut Microbes 13 (1). doi: 10.1080/19490976.2020.1848158
Keywords: yak, ruminal fluid transplantation, fecal flora, housed feeding, growth performance
Citation: Zhang M, Li Y, Li Y, Cheng C, Chai S, Yang Y, Wang X and Wang S (2025) Effects of rumen fluid transplantation on the fecal flora of yaks. Front. Anim. Sci. 5:1507838. doi: 10.3389/fanim.2024.1507838
Received: 08 October 2024; Accepted: 21 November 2024;
Published: 27 January 2025.
Edited by:
Bianca Castiglioni, National Research Council (CNR), ItalyReviewed by:
Zhiyuan Ma, Lanzhou University, ChinaMayra A. D. Saleh, University of the Azores, Portugal
Copyright © 2025 Zhang, Li, Li, Cheng, Chai, Yang, Wang and Wang. This is an open-access article distributed under the terms of the Creative Commons Attribution License (CC BY). The use, distribution or reproduction in other forums is permitted, provided the original author(s) and the copyright owner(s) are credited and that the original publication in this journal is cited, in accordance with accepted academic practice. No use, distribution or reproduction is permitted which does not comply with these terms.
*Correspondence: Man Zhang, MTIyNzMwMzMzOEBxcS5jb20=; Shuxiang Wang, Mjg0MzU5MzE2NkBxcS5jb20=
†ORCID: Chenxu Cheng, orcid.org/0009-0009-8765-6726