- 1Department of Agricultural Economics and Animal Production, Faculty of Science and Agriculture, University of Limpopo, Sovenga, South Africa
- 2Department of Animal Science, University of Venda, Thohoyandou, South Africa
Mastitis is one of the major diseases affecting the viability of dairy farming due to direct and indirect losses associated with low milk yield and poor milk quality. This review aims to provide comprehensive literature on methods that are commonly employed for field and laboratory diagnosis of bovine mastitis. The search process was conducted with the use of the Google Scholar electronic database. The keywords were “bovine mastitis” and “diagnosis. Findings indicate the use of various tests for early detection of mastitis under field conditions and in the laboratory. Conventional methods include somatic cell count, microbiological milk culture, and the California mastitis test. Microbiome techniques and chromogenic plates were mentioned as methods that can yield better results as compared to simple bacterial culture methods. Polymerase chain reaction and matrix-assisted laser desorption/ionization-time of flight were mostly reported as reference tests for the diagnosis of bovine mastitis. The use of biosensors, machine learning and 16srRNA was reported to offer prospects for the diagnosis of bovine mastitis. Overall, results have shown that diagnostic techniques for mastitis play a crucial role in early pathogen detection, facilitating prompt treatment and reducing mastitis transmission. It can be concluded that bovine mastitis is prevalent in dairy cattle and places a significant economic burden on dairy farms worldwide. Therefore, accurate disease diagnosis is a critical step towards developing targeted intervention measures for udder health management.
Introduction
Mastitis is an inflammatory condition of the mammary gland caused by the invasion of the udder tissue by pathogens (Adkins and Middleton, 2018; Zigo et al., 2021). Mastitis contributes to significant economic losses in dairy industries worldwide (Fesseha et al., 2021). Milk output decreases, the culling rate increases, and veterinary expenses increase because of bovine mastitis (Ababu et al., 2020). Diagnosis of mastitis is generally based on measuring the inflammatory response, whereas diagnosis of an intramammary infection is based on identification of the inciting infectious agent. The clinical form of mastitis is characterized by visible inflammatory changes in milk and udder tissue with or without systemic clinical signs, whereas the subclinical form does not manifest clinical signs of mastitis but increases somatic cell count. Sub-clinically infected cows can be a source of infection to susceptible animals in the herd (Swami et al., 2017). Subclinical mastitis diagnosis requires cow-side tests such as the California mastitis test (CMT) or various laboratory tests such as somatic cell count (SCC) and milk bacteriological culture. Early diagnosis of subclinical mastitis is essential to prevent the spread of infection, minimize udder tissue damage, and ensure successful treatment (Duarte et al., 2015; Lakshmi, 2016). Figure 1 gives an overview of bovine mastitis diagnosis, Figure 2 elaborates the various diagnostic tests for bovine mastitis while Figure 3 indicates the key features that an ideal diagnostic test must possess.
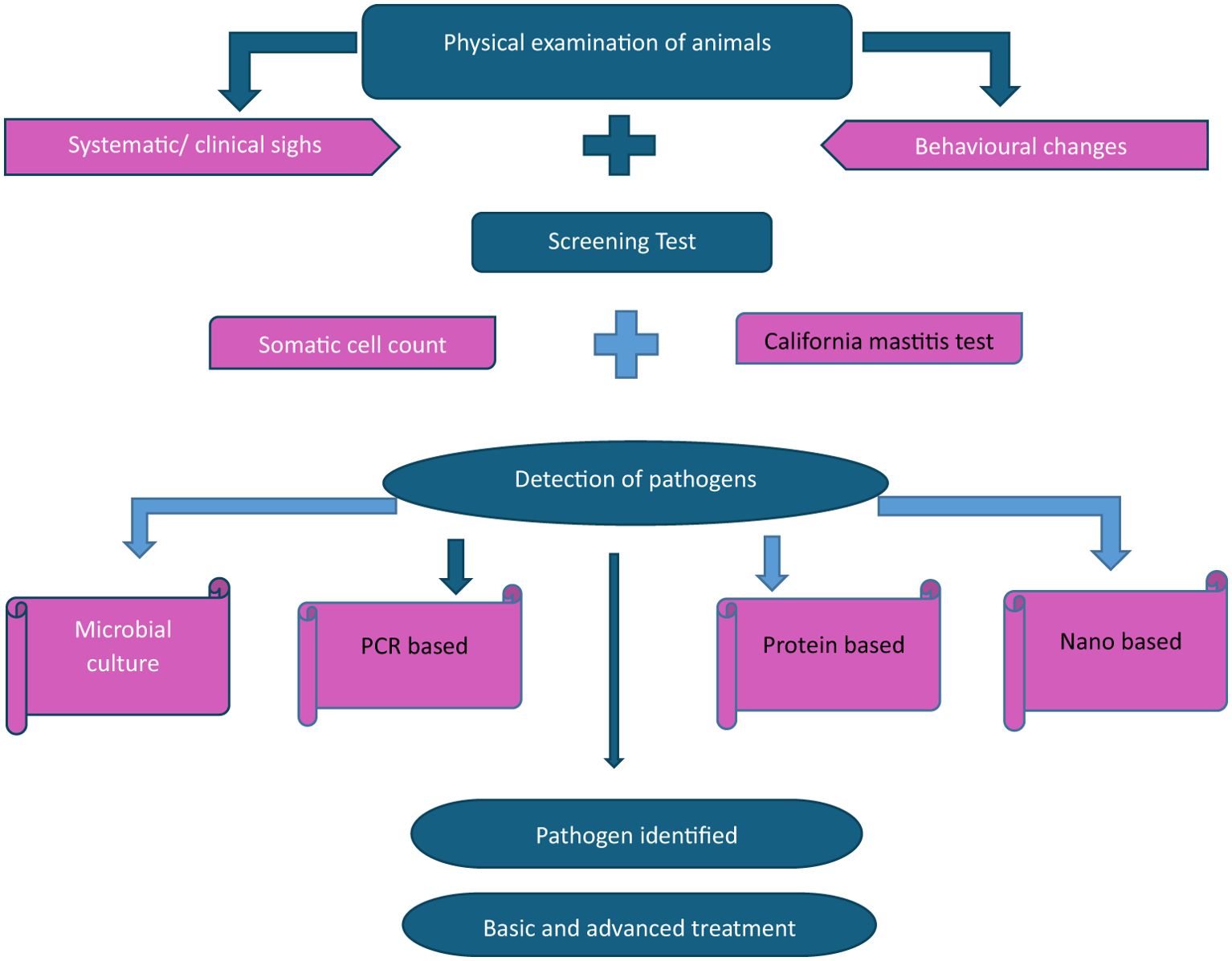
Figure 1. Overview of diagnoses of bovine mastitis. Adapted from Kour et al. (2023).
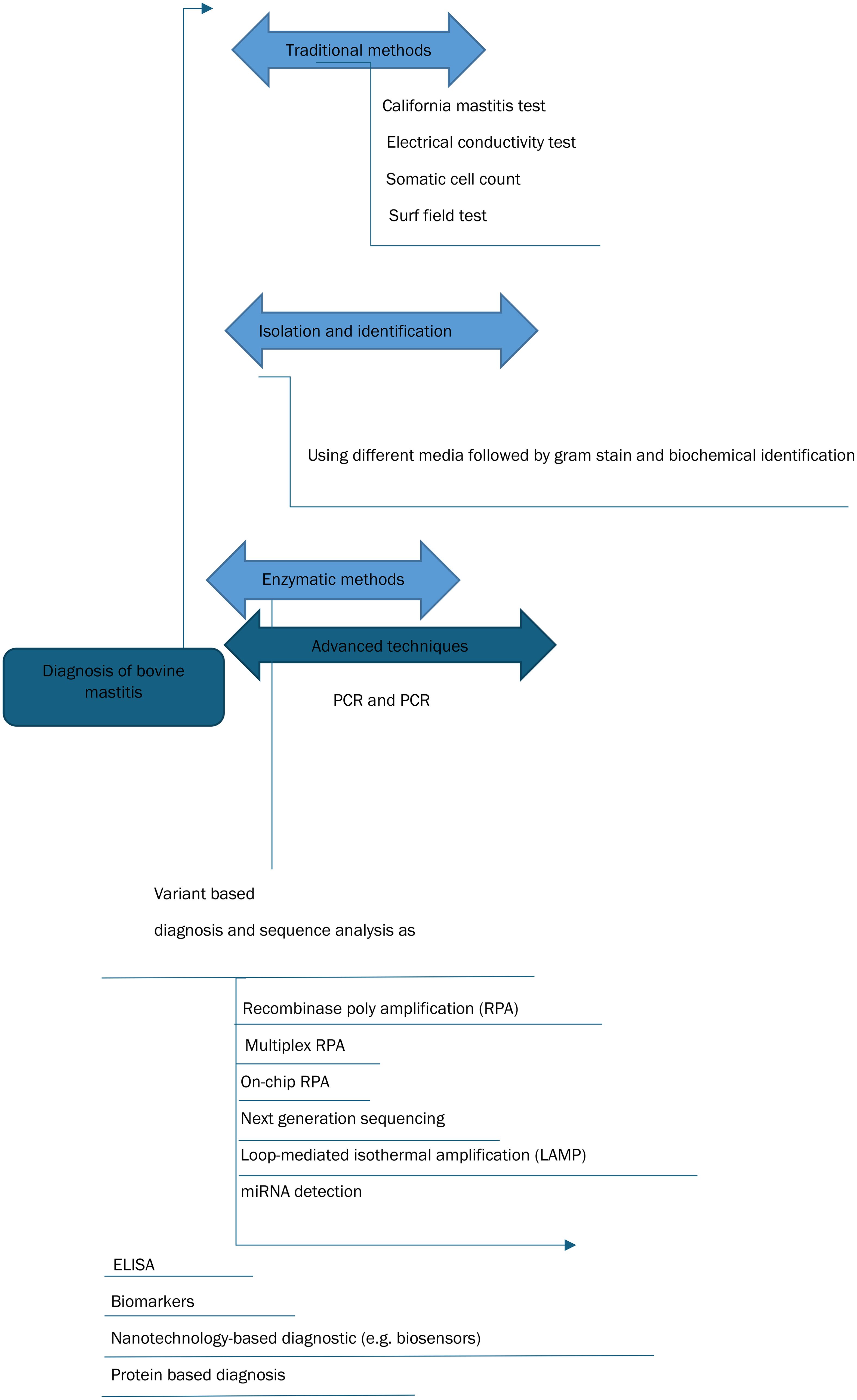
Figure 2. Diagnostic techniques of bovine mastitis. Adapted from El-Sayed and Kamel (2021).
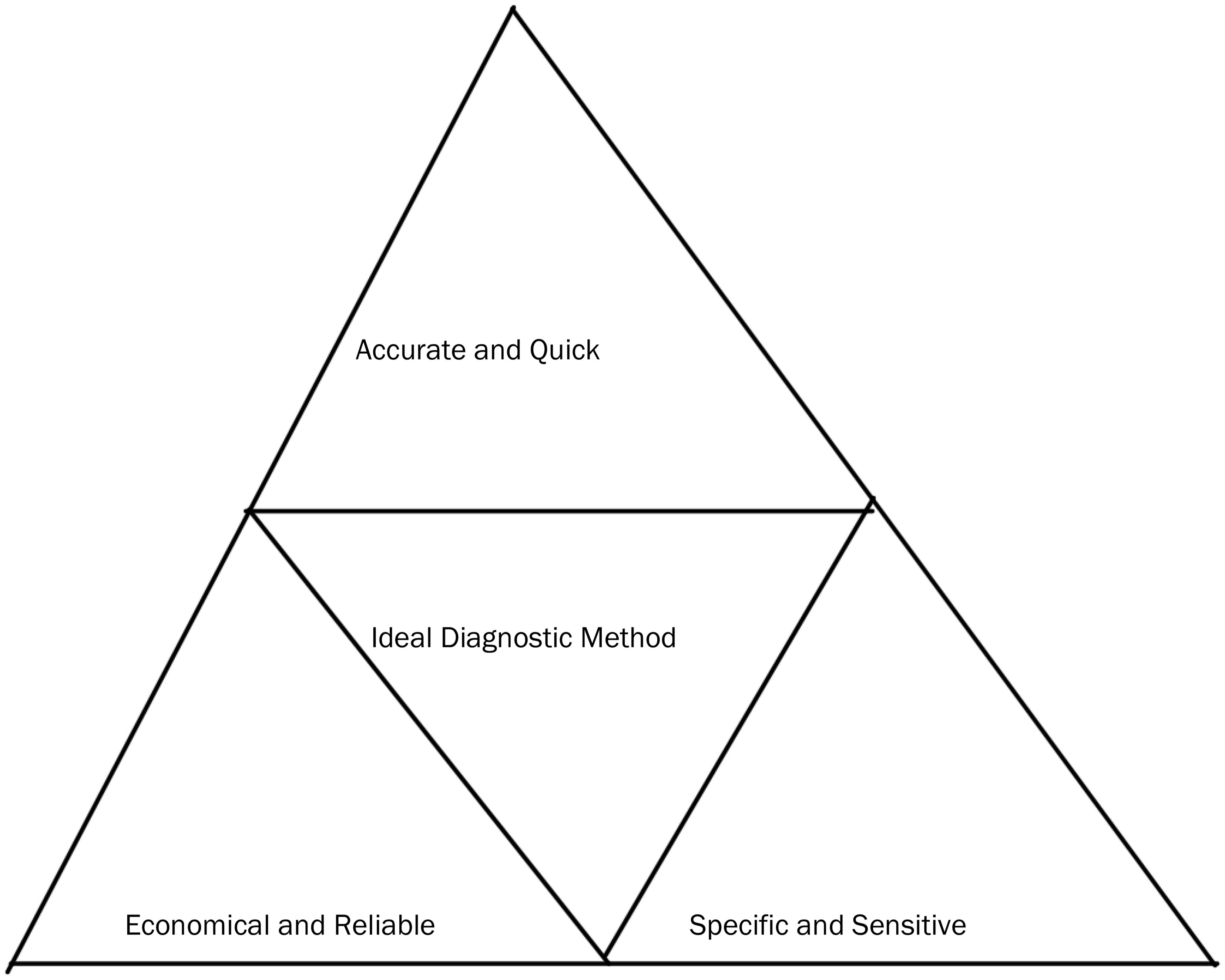
Figure 3. Characteristics of an ideal diagnostic test. Adapted from Martins et al. (2019).
Materials and methods
Article eligibility criteria
This review followed the principles and recommendations outlined in the Preferred Reporting Items for Systematic Reviews and Meta-Analyses (PRISMA) statement, as detailed by (Renald et al., 2023).
Search strategy
A comprehensive literature search was conducted to identify studies on methods used for the diagnosis of bovine mastitis. Google Scholar was used as the primary database. The search was conducted using the key terms “bovine mastitis,” and “diagnosis,”.
Article selection criteria
The criteria used to determine eligibility and inclusion in this search were studies focusing on methods for bovine mastitis diagnosis within a timeframe of seven (7) years, from 2017 to 2024, and published in the English language.
Data extraction
The researchers extracted the study content and screened it to ensure consensus on all critical elements. To maintain the data’s accuracy and quality, each article underwent a thorough review, and the necessary information was carefully documented.
Results
Diagnostic methods for clinical and subclinical mastitis
Over the review period, clinical and sub-clinical bovine mastitis diagnosis was performed using various methods. The methods include conventional approaches as well as new approaches due to advances in technology.
Characterization of the reviewed articles
A higher proportion of the articles that were reviewed in this study were published in 2022 and 2023 with eight articles (n=44) in each representing a total of 36.4%. This was followed by the years 2017 and 2021 with six articles each representing a total of 27.3%. The least number of articles reviewed were published in 2018 and 2024 with two articles in each year representing a total of 9.1%. Figure 4 shows the distribution by country of the articles reviewed in this study for countries that had more than one article reviewed. The highest number of articles reviewed were published in Brazil (n=6), the United States of America (n=5 each) followed by the United Kingdom n=4). Figure 5 presents the frequency at which mastitis diagnostic tests were reported in the articles that were reviewed in this study. Twenty-two (n=44) of the reviewed articles reported the use of SCC for bovine mastitis diagnosis representing a proportion of 50,0% while twenty-four articles (54.5%). Table 1 represents the information of all diagnostic methods that were mentioned in the reviewed articles while Figure 5 presents the frequencies of the mostly mentioned methods.
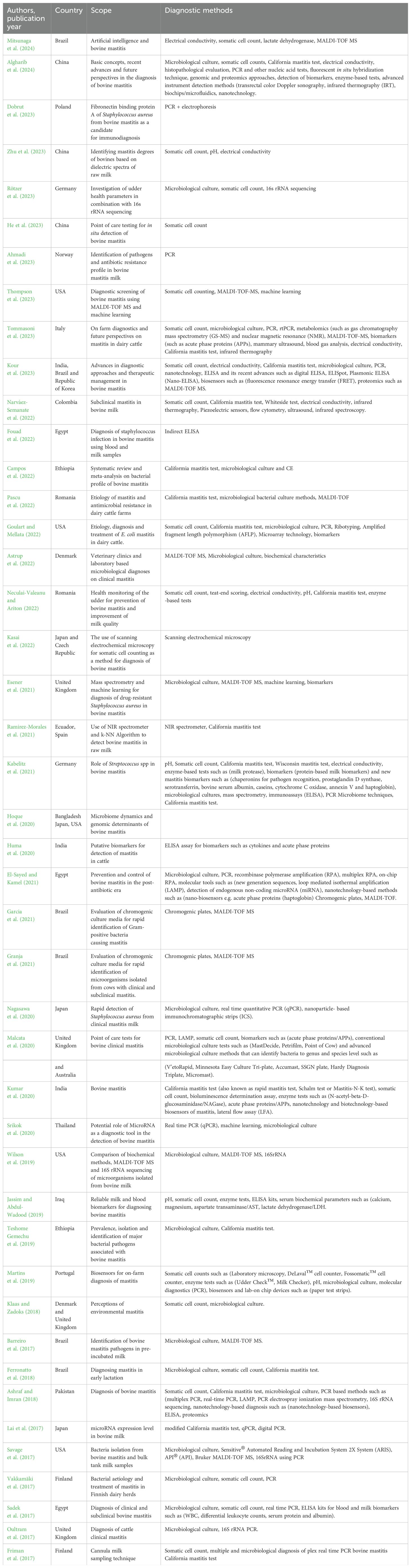
Table 1. Characteristics of studies describing methods for bovine mastitis diagnosis during the period 2017 to 2024.
Discussion
On-farm tests for bovine mastitis
On-farm culture systems
On-farm culture systems were developed to enable early treatment decisions. They are based on the use of selective media for differentiation between various groups of mastitis-causing pathogens. Examples include the Minnesota Easy Culture System II BiPlate and Tri-Plate which have been reported to have higher specificity but lower sensitivity. Royster et al. (2014) indicated that such methods are more reliable for the broad classification of infections but not very promising for closely related species identification. Although on-farm culture systems may not serve as replacements for laboratory microbial culturing, they provide acceptable results to make quick treatment decisions for mastitis cases.
Electrical conductivity tests
This test evaluates the electrical conductivity of milk which can elevated during mastitis due to high sodium chloride concentrations (Khatun et al., 2019; Paudyal et al., 2020). The electrical conductivity test is rapid and non-invasive (Tommasoni et al., 2023). Information gathered in this review indicated that electrical conductivity is commonly used as an in-line indicator of mastitis in automated milking systems. Our literature review revealed the use of the dielectric spectra of raw milk to identify degrees of bovine mastitis. Zhu et al. (2023) reported that the method has a great potential in identifying negative, weakly positive and positive cases of bovine mastitis while providing a feasible, quick, in situ mastitis detection method for monitoring health status of cows. However, Zhu et al. (2023) observed that the accuracy of the dielectric spectra method is that it can be affected by the temperature of the sample as well as the level of SCC in milk. In their case, the method could only be able to identify cows with mastitis when the milk SCC was higher than 500 000 cells/ML. One major drawback of the electrical conductivity method is the limited number of pathogens that it can detect. Jensen and Knudsen (1991) reported that factors such as whether the case is clinical or subclinical and changes in milk composition are among the factors that affect the accuracy of this method in detecting cases of mastitis. Ebrahimie et al. (2018), however, stated that electrical conductivity can give better results in terms of its sensitivity and specificity during inter-quarter comparisons at the cow level and therefore can be a strong indicator of subclinical mastitis. De Mol and Ouweltjes (2001) stated that time-series models for the detection of mastitis based on the historical electrical conductivity of milk can provide results of high accuracy with sensitivity and specificity values close to 100%. Stanek et al. (2024), however, stated that the test can give false negative results especially during the early stages of infection or in cows that exhibit minimal changes in conductivity. Furthermore, high service costs were cited as a major limiting factor for conventional and small parlors. Biggadike et al. (2002) reported that historical electrical conductivity measurements of milk over 14 days are a less costly option for online detection of mastitis. A positive alert of mastitis is announced if the current electrical conductivity is more than 10% higher than the average of the previous 14-day recordings.
California mastitis test and Wisconsin mastitis test
Our literature review revealed that the CMT test is the primary reference test of choice for detecting cows with bovine mastitis (Lai et al., 2017; Ferronatto et al., 2018; Teshome Gemechu et al., 2019). However, Lai et al. (2017) cited the reliance on SCC as one of the major limitations of the CMT method. Kandeel et al. (2017) reported that the CMT has a much higher sensitivity and specificity when used at a cut point of trace or higher. The same authors further indicated that when compared to other tests such as the esterase tests, CMT provides the most accurate, practical, and least costly on-farm screening test to predict subclinical mastitis at dry-off. The Wisconsin mastitis test (WMT) is considered a modification of the CMT and was developed to increase the objectivity of measuring milk viscosity. Rodrigues et al. (2009) stated that WMT is advantageous as it is designed for on-farm/cow side screening of subclinical mastitis, can yield results in a few minutes, and results in a semiquantitative measurement of the somatic cell count (SCC). Kandeel et al. (2019) further indicated that CMT provides a faster and more accurate cow-side screening test to predict subclinical mastitis at dry-off and freshening. The sensitivity and specificity of the CMT have been evaluated in multiple studies. Dingwell et al. (2003) evaluated CMT’s ability to detect intra-mammary infections due to major mastitis-causing pathogens (Staphylococcus aureus, Streptococcus spp, and gram-negative organisms) in early lactation. They reported 82.4% and 80.6% for sensitivity and specificity respectively. Nyman et al. (2014) supported this observation by stating that CMT is a useful screening tool for the major inflammatory mastitis-causing pathogens. However, evaluations on CMT’s ability to identify intra-mammary infections due to minor pathogens reported a lower sensitivity (61%) while specificity remained the same (80%). Middleton et al. (2004) reported 70% and 48% for sensitivity and specificity respectively when assessing the ability of CMT to detect intra-mammary infections at dry-off at the cow level for the pathogens tested.
Lateral flow immunoassay
Our study revealed the lateral flow assay as one of the field tests for bovine mastitis diagnosis. However, few of the articles that were reviewed in our study mentioned this method. However, Sajid et al. (2015) described this method as being cost-effective and rapid. Lateral flow assay relies on antigen-antibody interactions that can be visualized with the help of labeling agents such as colloidal gold. MaChado et al. (2021) described the lateral flow immunochromatographic kit (LFK) as a simple, rapid test that can be carried out in the field. Due to its perceived benefits, the LFK technology is widely used for the determination of bacterial pathogens in clinical samples. Sajid et al. (2015) attributed the recent surge in the use of lateral flow assay (LFA) in different fields of biology to its simplicity, rapidity, cost-effectiveness, and suitability for field deployment. The test utilizes antibody-antigen interactions which are visualized with the help of a labeling agent such as colloidal gold to detect the presence or absence of the analyte of interest in the sample. Upadhyay and Nara (2018) and Yong et al. (2022) reported the use of LFK for milk samples to detect S. aureus enterotoxin and Streptococcus suis serotype 2. Lateral flow assay can rapidly detect the common causes of bovine mastitis such as E. coli, S. aureus, K. pneumoniae, S. agalactiae and S. pyogenes in milk samples from suspected cases with an overall sensitivity of 97% (Cooray, 1994; MaChado et al., 2021). Sayed et al. (2023) reported a sensitivity of 103/ml for E. coli, S. aureus, K. pneumoniae, S. agalactiae, and S. pyogenes.
Laboratory-based methods for bovine mastitis testing
Bacterial culture tests
The literature reviewed in this study identified the microbiological culture of milk as the popular diagnostic method for bovine mastitis as it is the preferred baseline test for bovine mastitis suspected cases of bovine mastitis. Microbiological culture techniques are generally inexpensive and simple to perform, however, they need to be performed using standardized repeatable methods. One of the articles included in our review by Nagasawa et al. (2020) evaluated microbiological culture against the immune-chromatographic strip (ICS) test. The authors reported high sensitivity and high specificity for the detection of Staphylococcus aureus in clinical mastitic milk. Petrifilm, agar plates, and tube-based systems are examples of culture-based point-of-care tests that were cited in the literature reviewed. Reported sensitivities for gram-positive bacteria ranging from 58.6% to 98% and specificities from 48% to 97% (McCarron et al., 2009; MacDonald, 2013; Leimbach and Krömker, 2018). McCarron et al. (2009) reported that the accuracy of microbiological culture tests depends on observer skills. For example, the ability to detect S. aureus based on hemolysis depends on the population under investigation and the experience of the reader. Taponen et al. (2009 added that limitations of classical bacterial culture include a delay of 24–48 hours to obtain results. In addition, in approximately 25% of milk samples from clinical mastitis cases bacteria are not detected in conventional culture. Nyman et al. (2016) stated that the sensitivity of bacterial culture can be improved by applying repeated sampling or other measures, such as selective agar and biochemical tests. However, such test procedures may take several days and increase analysis costs. Agar-based tests often allow for easy visual identification of sample contamination, which is important to monitor sample quality. Malcata et al. (2020) indicated that in addition to being both time-consuming and labor-intensive, milk culture may not yield bacteria from sub-clinically infected glands if the quantity of pathogens present when samples are taken is low. Murphy et al. (2016) and O’Reilly et al. (2024) stated that the presence of leftover treatment antibiotics in the examined milk may result in a lack of recognition of the target microorganisms from mastitic milk. Lago and Godden (2018) recommended the use of rapid culture plates as they can differentiate between common mastitis pathogens without the requirement for additional enzymatic testing thereby allowing incubation and identification of bacteria to be undertaken on-farm or within a veterinary clinic. Furthermore, rapid-culture plates can be used to make decisions about specific treatments since they are more accurate (Lago and Godden, 2018). In one study, the sensitivity of the bacterial culture test was found to be increased when three-quarter milk sampling occasions were carried out as compared to one sampling occasion.
Chromogenic plates
Garcia et al. (2021) evaluated the diagnostic performance in terms of specificity, sensitivity, and accuracy of two chromogenic culture media for rapid identification of Gram-positive bacteria causing subclinical mastitis in dairy cows. The authors reported a sensitivity of 89.1%, specificity of 96.3%, and accuracy of 95.6% for Streptococcus. agalactia/dysgalactiae identification. Regarding the identification of Streptococcus uberis/Enterococcus spp, a sensitivity of 90.5%, specificity of 92.5%, and accuracy of 92.3% was reported. The advantages of chromogenic plate media as cited by these authors include its rapidity (from 18 to 24 h), the high values obtained for the diagnostic performance in most groups of pathogens, and the ability to differentiate between some species of Staphylococcus spp. and Streptococcus spp. A study by Granja et al. (2021) on bovine milk samples from cows that had tested positive for subclinical mastitis reported average sensitivities of 50%, 94%, and 97% for E. coli, Staphylococcus aureus and Streptococcus agalactiae/dysgalactiae respectively. These authors observed that the diagnostic accuracy of the biplate and triplate chromogenic culture media varied with the pathogen being investigated. Chromogenic plates can be used for rapid decision-making on treatment protocols of the major mastitis-causing pathogens. However, as indicated by Granja et al. (2021), their adoption in mastitis control programs depends on the specific needs of each farm.
Somatic cell count
Literature reviewed in this study concurred that SCC or the logarithmic transformation of SCC which is known as the somatic cell score (SCS) was the second most mentioned diagnostic test for subclinical mastitis. The advantages of this method are mainly related to the low cost and ease of data collection. In a laboratory setting, SCC can be measured by direct microscopy or by using automated electronic cell counters. However, the latter method is labor intensive and requires thorough training of personnel to gain proficiency while automated electronic cell counters allow for rapid and easy determination of SCC. Portable counters can be used to test SCC in the laboratory or on the farm. At the herd level, SCC data provides an estimation of overall udder health among cows contributing to the bulk tank milk. At the cow level, SCC data can be used to identify the cows that have healthy mammary glands versus those with acute, resolved, or chronic cases of subclinical mastitis. Jashari et al. (2016) reported that when SCC is used as an indicator for intra-mammary infections, sensitivity ranges from 30% to 89% while specificity ranges from 60% to 90%. Narváez-Semanate et al. (2022) reported that in raw milk, in addition to identifying cows that have mastitis, SCC also provides information on biochemical changes in the milk, up to production losses. When using a threshold of 200,000 cells/mL for a single composite SCC, the sensitivity and specificity were 44% and 87%, respectively, for cows infected with any pathogen and 65% and 73%, respectively, for cows infected with major pathogens (Jashari et al., 2016). Adkins and Middleton (2018) further stated that SCC can best detect intra-mammary infections at the quarter level. Schukken et al. (2003) and Zhu et al. (2023) mentioned that a mean SCC of approximately 70,000 cells/mL would indicate that the quarter/s are not infected while a mean SCC of approximately 200,000 cells/mL or greater or an SCS of 4 or higher are often used as a threshold to define infected quarters. However, the SCC threshold was reported to be different within countries of the European community where the established norms are between 400,000 to 750,000 cells mL-1 as the maximum value, while in Colombia, the maximum accepted count is 800,000 cells mL-1 (Gómez-Quispe et al., 2015). As reported by these authors, the diagnostic sensitivity of quarter-level SCC for subclinical mastitis can be misleading as it depends to some extent on the pathogen inciting the mastitis. Roberts (2024) indicated that false positives may occur when somatic cell counts are elevated due to factors like injury, stress, or late lactation, while false negatives can arise in mild infections due to lower SCC levels that do not produce visible gel formation. Mohammed et al. (2019) added that SCC is subjective, and can yield variable results due to visual interpretation, and lack of precision in identifying specific pathogens. Other limitations have been identified that impact the use of SCC as a diagnostic tool such as milk SCC levels remaining elevated for some time after an organism has been eliminated, resulting in a false-positive test for intra-mammary infections. In addition, factors other than intra-mammary infections that can cause variations in the SCC were identified namely breed, month of sampling, season, stage of lactation, age of the cow, parity, frequency of milking, nutrition, and animal stress (Middleton et al., 2004; Alhussien and Dang, 2018; Halasa and Kirkeby, 2020; Swinkels et al., 2021).
Polymerase chain reaction
The present study reported the use of genotypic approaches to supplement phenotypic identification of bovine mastitis, providing a viable option for resolving issues such as false negative results. Taponen et al. (2009) stated that approximately 30% of clinical mastitis samples do not grow in bacterial culture. Therefore, the ability of polymerase chain reaction (PCR) to detect growth-inhibited samples helps to reduce false-negative results (El-Sayed et al., 2017). The high specificity and sensitivity of PCR-based techniques have made it the new gold standard for the diagnosis of mastitis Culture-independent methods for identifying bacterial pathogens in milk have become more common over the last decade (Koskinen et al., 2010). Molecular techniques such as the polymerase chain reaction (PCR) were developed for speedy detection of different mastitis pathogens to counter the limitations of culture and other conventional methods. Commercial PCR assays are available for detecting mastitis-causing pathogen DNA in mammary quarter milk samples, cow-level composite milk samples, and bulk tank milk samples.
Bulk tank PCR assays can be used in the same way as bulk tank cultures as an indicator of udder health, milking time hygiene, and storage conditions on the farm. These methods can detect bacterial infections speedily (in hours) as compared to days required by microbial culture techniques. Furthermore, PCR techniques have a high sensitivity which boosts pathogen detection levels. Studies by Taponen et al. (2009) and Bexiga et al. (2011) indicated that PCR techniques could provide a diagnosis for 43% to 47% of mastitic milk samples that are negative based on conventional culture. Gurjar et al. (2012) indicated that PCR may detect bacteria in the presence of preservatives or residual therapeutic antibodies in milk, avoiding the false-negative result caused by a lack of bacterial growth, which is a significant disadvantage of the culture method. Katholm et al. (2012) added to this by stating that the application of PCR to bulk tank samples can be used to monitor bacteria with low prevalence, such as S agalactiae. According to Kelton and Godkin (2014) when using combined milk (e.g., bulk tank or pen) samples, it is recommended to only test for contagious pathogens such as S. aureus, S agalactiae, and Mycoplasma spp. because there is a high probability that these bacteria originated from the mammary gland. Zeinhom et al. (2016) and Mahmmod et al. (2017) indicated that there is a risk of false-positive results because of teat skin contaminants, contaminated teat orifices, contaminated equipment, and carryover of contaminated milk from other cows. Compared to the use of bacterial culture and regular PCR, real time-PCR has extra advantages of speed and accuracy as well as being safe to the environment and the workers since the method does not utilize ethidium bromide, eliminates the need for post-reaction handling (no agarose electrophoresis), and the results are better visualized and digitalized, allowing for data sharing. Koskinen et al. (2010), Keane et al. (2013), Jones et al. (2019) and Mengistie (2022) indicated that when used to identify mastitis pathogens, real-time PCR has a 100% sensitivity and specificity rates. Koskinen et al. (2009) reported higher sensitivity and specificity of the Multiplex real-time PCR kit PathoProof™ when compared with microbial culturing. Amin et al. (2011) highlighted the drawbacks of multiplex PCR with the main drawback being the competition between different sets of primers for PCR substances like deoxynucleotide triphosphates (dNTPs) and Taq polymerase, which reduces the sensitivity as well as the lack of guidelines on how to report multispecies results. Additionally, PCR can detect DNA from dead bacteria. Koskinen et al. (2009) and Koskinen et al. (2010) indicated that despite its short turnaround time for results, PCR is difficult to implement on-farm due to sterility requirements as well as the need for complex equipment and trained personnel. Furthermore, the presence of PCR inhibitors such as calcium, fat, and high protein content in milk requires dedicated DNA extraction protocols to provide high-quality results which limits the application of PCR-based techniques to central laboratories. Nyman et al. (2016) stated that the high analytical sensitivity of PCR and its ability to detect dead bacteria may give false positive results which could potentially lead to an increase in antimicrobial treatment of cows. These data are important to consider if PCR is being used as a follow-up test to assess response to treatment. Hiitio et al. (2015) indicated that PCR analysis may be an effective screening tool, but the results must be carefully scrutinized before treatment decisions. Hiitio et al. (2016) recommend waiting for at least 2 to 3 weeks after the onset of mastitis or treatment or until the quarter milk SCC returns to normal levels before using PCR to assess response to treatment.
Other molecular typing methods used for the identification of bovine mastitis pathogen at the species level include ribotyping (Zadoks et al., 2011), amplified fragment length polymorphism (AFLP) (Leung et al., 2004), restriction fragment length polymorphism (RFLP) (Ombarak et al., 2018), pulsed-field gel electrophoresis (PFGE) typing (Freitag et al., 2017), and multiple-locus variable-number tandem repeat analysis (MLVA) (El-Sayed and Kamel, 2021), and at both species and strain levels include transfer DNA intergenic spacer length polymorphism (Chakraborty et al., 2019) and DNA sequencing of housekeeping genes (Ali et al., 2021). Another genotypic method is microarray technology, which can detect common species of mastitis-causing pathogens in as little as 6 h, with a sensitivity of 94.1% and a specificity of 100% (Lee et al., 2008).
MALDI-TOF
The findings of the present study agree with the report by Barreiro et al. (2017) indicating that matrix-assisted laser desorption/ionization time-of-flight (MALDI-TOF) mass spectrometry is utilized for the genus and species identification of mastitis pathogens. This technology is becoming widely adopted in many diagnostic and research laboratories. MALDI-TOF is a high-throughput technology that uses a protein fingerprint and a database of reference spectra to determine bacterial species. This test has been validated as an accurate secondary test for some mastitis-causing pathogens. Although this method has been reported to be a rapid technique for the speciation of bacteria, the initial equipment setup is costly and, for the most part, it requires the organism to be cultured first. One study recommended that MALDI-TOF be used in a culture-independent fashion to identify bacterial species in experimentally inoculated milk samples. However, direct-from-milk MALDI-TOF is not currently recommended owing to the high likelihood of false negative results in cows infected with mastitis-causing pathogens. Thompson (2022) stated that MALDI-TOF MS is highly accurate and can yield results with sensitivity and specificity of up to 100% when used to identify mastitis-causing pathogens. The same author added that the ability of MALDI-TOF MS to analyze protein profiles at the molecular level makes it superior to traditional biochemical methods for distinguishing bacterial species. Zhang et al. (2022) stated that MALDI-TOF MS is extremely fast, providing results in minutes, and once set up, it is cost-effective, capable of processing large sample volumes, and highly precise in pathogen identification. However, the test’s accuracy depends on comprehensive databases. Proper sample preparation is essential to ensure reliable results, and MALDI-TOF MS cannot assess antimicrobial susceptibility, requiring additional tests for antibiotic resistance analysis (Solntceva et al., 2021).
Microbiome techniques
The milk microbiome composition is an important determinant of mammalian health and plays an important role in udder health by interacting with the immune and metabolic functions of the cow, the spread of virulence factors, and the spread of antimicrobial resistance genes (Jiménez et al., 2015; Mediano et al., 2017; Derakhshani et al., 2018; Sakwinska and Bosco, 2019). One of the advantages of microbiome techniques such as metagenomic analyses is the ability to identify fastidious/anaerobic bacterial organisms, which are difficult to cultivate by routine methods. Improved access to genome-based, culture-independent methods has generated great interest in defining the bovine milk microbiome (Bonsaglia et al., 2017; Metzger et al., 2018a, b). Kuehn et al. (2013) added that the application of culture-independent methods has been especially used as diagnostic methods to better understand the nature and biological relevance of culture-negative samples. Parente et al. (2020) indicated that intramammary infections affect the microbiota composition of milk collected directly from the cistern compared to the microbiota present in healthy animals. Hoque et al. (2019) reported greater diversity in the milk microbiota in animals with clinical mastitis compared to healthy animals. According to Derakhshani et al. (2018) and Hoque et al. (2020), the microbiome of bovine milk is influenced by different factors such as breed, farm management, feeding, milk yield, lactation stage, and parity. Furthermore, the accuracy of results obtained from microbiome techniques can be compromised in cases where the microbial biomass of the milk sample is low (Rodrigues et al., 2017; Pang et al., 2018; Vasquez et al., 2019).
Enzyme tests
Mukherjee et al. (2023) stated that measuring the levels of specific enzymes such as N-acetyl-beta-D-glucosaminidase (NAGase) and lactate dehydrogenase (LDH) in milk is an effective approach for detecting mastitis. Novac and Andrei (2020) added that increased enzyme levels are associated with mastitis. Gross and Bruckmaier (2019) reported that enzyme-based tests are simple, reliable, and allow for early detection of subclinical mastitis, and are non-invasive since they only utilize milk samples for analysis. Kalmus et al. (2013) reported that the haptoglobin test can give reliable results because a constant increase in the haptoglobin concentration was found in the milk along with increasing quantities of bacterial DNA. The esterase activity test is a qualitative test that converts the results of an enzymatic reaction into an estimated SCC. However, Chakraborty et al. (2019) indicated that enzyme-based diagnostic tests are not accurate since they can differ in other disorders as well as in mastitis-affected cows. Pumipuntu et al. (2017) assessed the deoxyribonuclease (DNase) test for Staphylococcus aureus identification in milk samples. The authors reported a sensitivity of 53% and a specificity of 42%. Based on their findings, the authors did not recommend the DNase test for screening of Staphylococcus aureus in milk. Just like with SCC, factors that are not related to mastitis such as stress, inflammatory conditions, dietary changes, and lactation stage can cause variations in enzyme levels. This can potentially affect the specificity and sensitivity of these tests thereby providing false positive/negative results and inconsistent outcomes due to variations in enzyme activities among different cows (Martins et al., 2019). Kumari et al. (2018) stated that although acute phase proteins may be useful, currently they are not an economically feasible option for diagnosing subclinical mastitis.
Future opportunities for bovine mastitis diagnosis
Biosensors
The literature reviewed in this study revealed biosensors as an emerging technology that was developed for on-site mastitis testing to offer less lengthy approaches to conventional diagnostic methods (Kour et al., 2023). Recent advances in nanotechnology and biotechnology have led to the development of analytical tools called biosensors, capable of converting the biological compounds in a sample into electrical signals that can detect the presence of particular cells and markers with high sensitivity upon proper tuning and amplification. Wang et al. (2015) identified single-stranded oligonucleotides, antibodies, and artificial binding proteins as the most used recognition elements in sensing bacterial contamination. Duarte et al. (2015) and Ashraf and Imran (2018) mentioned infrared thermography, biosensors, and nanotechnology methods as the novel emerging diagnostic technologies that have been developed in recent years for improving the diagnosis of mastitis both at microbial and biomarker levels. New biomarkers for high sensitivity and specificity, rapid and efficient with a “cow-side” application, are among the development prospects for novel E. coli mastitis diagnosis techniques (Duarte et al., 2015; Sharifi et al., 2018; Li et al., 2019). For example, as stated by Ceciliani et al. (2014), proteomic research has yielded data on protein expression patterns that can be used to find novel therapeutic targets such as bacterial immunogenic proteins for vaccines and diagnostic biomarkers for bovine mastitis. Haxhiaj et al. (2022) reported that proteomic techniques for accurate biomarkers are feasible for early identification of mastitis and treatment efficacy, as well as the discovery of novel targets for alternative therapy development. Farmanullah et al. (2021) reported various in silico analysis tools that can be applied to screen for gene expression in mastitis. The same authors reported that through in silico analysis, it was observed that mastitis reduces the expression of fat metabolism and immune system-related genes, whereas it increased the expression of inflammatory genes. Huma et al. (2020) reported significant increases of interleukin-1∝, interleukin-8 and haptoglobin in both blood serum and milk whey in subclinical and clinical mastitis cows. These advancements, however, are still not suitable for routine diagnosis of bovine mastitis.
16s rRNA gene sequencing
Angelopoulou et al. (2019) highlighted the importance of culture-independent techniques for mastitis diagnosis as these techniques assist in providing evidence-based treatment for mastitis through accurate identification of the mastitis-causing organism(s). However, the method is not always readily available in most mastitis diagnostic laboratories. Oikonomou et al. (2012) reported that sequencing and analysis of hypervariable regions within the 16S rRNA gene can provide comparably expeditious and cost-effective methods for appraising bacterial diversity and abundance. Jiménez et al. (2015) added that this technique is an effective tool for pathogen discovery and offers a breakthrough from the limitations of conventional culture methods. Ajitkumar et al. (2012) indicated that real-time PCR amplification of 16S rRNA gene fragment, spanning the variable region V5 and V6 with high-resolution melt analysis (HRMA) can be used as a powerful, fast and low-cost tool for the differentiation of clinically important bacterial mastitis pathogens. This allows the supply of timely information to physicians in a clinical laboratory setting. In one study, 33 samples that were identified by classical aerobic culture techniques as culture negative, pyrosequencing was able to detect sequences from bacteria that are known to cause bovine mastitis. This confirms that conventional methods for bacterial identification based on biochemical utilization, enzymatic profiles and serotyping are insufficient for identifying closely related bacterial strains. Forsman et al. (1997) recommended 16S rRNA sequencing as an objective and accurate method for the proper identification of Staphylococcus species isolated from bovine mastitis However, McMillan et al. (2017) reported that 16s rRNA gene sequencing yields compositional data sets and thus fails to provide resolution to species/strain level. Furthermore, it cannot differentiate between living and dead microorganisms. It was observed by these authors that the usefulness of 16S rRNA gene sequencing is limited when applied to certain staphylococcal species owing to the high degree of gene similarity. Lange et al. (2015) stated that this limitation can be overcome by using housekeeping genes to differentiate staphylococcal species and reported an accuracy of 95% following partial 16S rRNA sequencing for the species identification of coagulase-positive and coagulase-negative staphylococci isolated from bovine mastitis.
Conclusion
Microbiological culture and the California mastitis test are key conventional methods used to detect the presence of mastitis in dairy farms. Technological advancements have enabled a significant shift from the use of conventional diagnosis, which has lower specificity and/or sensitivity, to highly measurable, quick, and reliable molecular diagnosis, which has a high degree of accuracy. In general, the goals of determining the cause of an intra-mammary infection are to either select a treatment protocol or determine where control measures need to be implemented or improved on the farm to reduce disease incidence and improve udder health and milk quality. The choice of a diagnostic method is guided by practical considerations such as specificity, sensitivity, cost, processing time, and ability to handle many milk samples.
Author contributions
MR: Conceptualization, Methodology, Resources, Writing – original draft, Investigation, Project administration, Data curation, Formal analysis, Funding acquisition, Software, Supervision, Validation, Visualization, Writing – review & editing. TT: Conceptualization, Investigation, Methodology, Writing – original draft, Project administration, Resources, Formal analysis, Validation, Writing – review & editing, Data curation, Funding acquisition, Software, Supervision, Visualization. LG: Investigation, Validation, Writing – review & editing, Resources, Conceptualization, Data curation, Formal analysis, Funding acquisition, Methodology, Project administration, Software, Supervision, Visualization, Writing – original draft. TC: Formal analysis, Investigation, Methodology, Writing – review & editing, Resources, Validation, Conceptualization, Writing – original draft, Data curation, Funding acquisition, Project administration, Software, Supervision, Visualization.
Funding
The author(s) declare that no financial support was received for the research, authorship, and/or publication of this article.
Acknowledgments
The authors acknowledge the University of Limpopo and the University of Venda for providing the necessary resources to conduct the study.
Conflict of interest
The authors declare that the research was conducted in the absence of any commercial or financial relationships that could be construed as a potential conflict of interest.
Generative AI statement
The author(s) declare that no Generative AI was used in the creation of this manuscript.
Publisher’s note
All claims expressed in this article are solely those of the authors and do not necessarily represent those of their affiliated organizations, or those of the publisher, the editors and the reviewers. Any product that may be evaluated in this article, or claim that may be made by its manufacturer, is not guaranteed or endorsed by the publisher.
References
Ababu A., Dereje E., Haben F. (2020). Isolation and antimicrobial susceptibility profile of Escherichia coli O157: H7 from raw milk of dairy cattle in Holeta district, Central Ethiopia. Int. J. Microbiol. 1, 6626488. doi: 10.1155/2020/6626488
Adkins P. R. F., Middleton J. R. (2018). Methods for diagnosing mastitis. Vet. Clinics: Food Anim. Pract. 34, 479–491. doi: 10.1016/j.cvfa.2018.07.003
Ahmadi A., Khezri A., Nørstebø Hand Ahmad R. (2023). A culture-, amplification-independent, and rapid method for identification of pathogens and antibiotic resistance profile in bovine mastitis milk. Front. Microbiol. 13. doi: 10.3389/fmicb.2022.1104701
Ajitkumar P., Barkema H. W., De Buck J. (2012). Rapid identification of bovine mastitis pathogens by high-resolution melt analysis of 16S rDNA sequences. Vet. Microbiol. 155, 332–340. doi: 10.1016/j.vetmic.2011.08.033
Algharib S. A., Dawood A. S., Huang L., Guo A., Zhao G., Zhou K., et al. (2024). Basic concepts, recent advances, and future perspectives in the diagnosis of bovine mastitis. J. Vet. Sci. 25, 1. doi: 10.4142/jvs.23147
Alhussien M. N., Dang A. K. (2018). Milk somatic cells, factors influencing their release, future prospects, and practical utility in dairy animals: An overview. VeterinaryWorld 11 (5), 562. doi: 10.14202/vetworld.2018.562-577
Ali A., Liaqat S., Tariq H., Abbas S., Arshad M., Li W. J., et al. (2021). Neonatal calf diarrhea: A potent reservoir of multi-drug resistant bacteria, environmental contamination and public health hazard in Pakistan. Sci. Total Environ. 799, 149450. doi: 10.1016/j.scitotenv.2021.149450
Amin A. S., Hamouda R. H., Abdel-All A. A. (2011). PCR assays for detecting major pathogens of mastitis in milk samples. World J. Dairy Food Sci. 6 (2), 199–206.
Angelopoulou A., Holohan R., Rea M. C., Warda A. K., Hill C., Ross R. P. (2019). Bovine mastitis is a polymicrobial disease requiring a polydiagnostic approach. Int. Dairy J. 99, 104539. doi: 10.1016/j.idairyj.2019.104539
Ashraf A., Imran M. (2018). Diagnosis of bovine mastitis: from laboratory to farm. Trop. Anim. Health Product. 50, 1193–1202. doi: 10.1007/s11250-018-1629-0
Astrup L. B., Pedersen K., Farre M. (2022). Microbiological diagnoses on clinical mastitis—comparison between diagnoses made in veterinary clinics versus in laboratory applying MALDI-TOF MS. Antibiotics 11, 271. doi: 10.3390/antibiotics11020271
Barreiro J. R., Gonçalves J. L., Braga P. A. C., Dibbern A. G., Eberlin M. N., Veiga Dos Santos M. (2017). Non-culture-based identification of mastitis-causing bacteria by MALDI-TOF mass spectrometry. J. Dairy Sci. 100, 2928–2934. doi: 10.3168/jds.2016-11741
Bexiga R., Koskinen M. T., Holopainen J., Carneiro C., Pereira H., Ellis K. A., et al. (2011). Diagnosis of intramammary infection in samples yielding negative results or minor pathogens in conventional bacterial culturing. J. Dairy Res. 78, 49–55. doi: 10.1017/S0022029910000725
Biggadike H. J., Ohnstad I., Laven R. A., Hillerton J. E. (2002). Evaluation of measurement of the conductivity of quarter milk samples for the early diagnosis of mastitis. Vet. Rec. 150, 655–658. doi: 10.1136/vr.150.21.655
Bonsaglia E. C., Gomes M. S., Canisso I. F., Zhou Z., Lima S. F., Rall V. L., et al. (2017). Milk microbiome and bacterial load following dry cow therapy without antibiotics in dairy cows with healthy mammary glands. Sci. Rep. 7, 8067. doi: 10.1038/s41598-017-08790-5
Campos F. C., Castilho I. G., Rossi B. F., Bonsaglia É. C. R., Dantas S. T. A., Dias R. C. B., et al. (2022). Genetic and antimicrobial resistance profiles of mammary pathogenic E. coli (MPEC) isolates from bovine clinical mastitis. Pathogens 11, 1435. doi: 10.3390/pathogens11121435
Ceciliani F., Eckersall D., Burchmore R., Lecchi C. (2014). Proteomics in veterinary medicine: applications and trends in disease pathogenesis and diagnostics. Vet. Pathol. 51, 351–362. doi: 10.1177/0300985813502819
Chakraborty S., Dhama K., Tiwari R., Iqbal Yatoo M., Khurana S. K., Khandia R., et al. (2019). Technological interventions and advances in the diagnosis of intramammary infections in animals with emphasis on bovine population—a review. Vet. Q. 39, 76–94. doi: 10.1080/01652176.2019.1642546
Cooray R. (1994). Use of bovine myeloperoxidase as an indicator of mastitis in dairy cattle. Vet. Microbiol. 42, 317–326. doi: 10.1016/0378-1135(94)90063-9
De Mol R. M., Ouweltjes W. (2001). Detection model for mastitis in cows milked in an automatic milking system. Prev. Vet. Med. 49, 71–82. doi: 10.1016/s0167-5877(01)00176-3
Derakhshani H., Fehr K. B., Sepehri S., Francoz D., De Buck J., Barkema H. W., et al. (2018). Invited review: Microbiota of the bovine udder: Contributing factors and potential implications for udder health and mastitis susceptibility. J. Dairy Sci. 101, 10605–10625. doi: 10.3168/jds.2018-14860
Dingwell R. T., Leslie K. E., Schukken Y. H., Sargeant J. M., Timms L. L. (2003). Evaluation of the California mastitis test to detect an intramammary infection with a major pathogen in early lactation dairy cows. Can. Vet. J. 44, 413–415. Available at: https://pmc.ncbi.nlm.nih.gov/articles/PMC340150/.
Dobrut A., Młodzińska A., Drożdż K., Wójcik-Grzybek D., Michalak K., Pietras-Ożga D., et al. (2023). The two-Track Investigation of Fibronectin binding protein A of Staphylococcus aureus from bovine mastitis as a potential candidate for immunodiagnosis: a pilot study. Int. J. Mol. Sci. 24, 6569. doi: 10.3390/ijms24076569
Duarte C. M., Freitas P. P., Bexiga R. (2015). Technological advances in bovine mastitis diagnosis: an overview. J. Vet. Diagn. Invest. 27, 665–672. doi: 10.1177/1040638715603087
Ebrahimie E., Ebrahimi F., Ebrahimi M., Tomlinson S., Petrovski K. R. (2018). A large-scale study of indicators of sub-clinical mastitis in dairy cattle by attribute weighting analysis of milk composition features: highlighting the predictive power of lactose and electrical conductivity. J. Dairy Res. 85, 193–200. doi: 10.1017/S0022029918000249
El-Sayed A., Awad W., Abdou N. E., Castañeda Vázquez H. (2017). Molecular biological tools applied for the identification of mastitis-causing pathogens. Int. J. Vet. Sci. Med. 5, 89–97. doi: 10.1016/j.ijvsm.2017.08.002
El-Sayed A., Kamel M. (2021). Bovine mastitis prevention and control in the post-antibiotic era. Trop. Anim. Health Product. 53, 1–16. doi: 10.1007/s11250-021-02680-9
Esener N., Maciel-Guerra A., Giebel K., Lea D., Green M. J., Bradley A. J., et al. (2021). Mass spectrometry and machine learning for the accurate diagnosis of benzylpenicillin and multidrug resistance of Staphylococcus aureus in bovine mastitis. PloS Comput. Biol. 17, e1009108. doi: 10.1371/journal.pcbi.1009108
Farmanullah F., Liang X., Khan F. A., Salim M., Rehman Z. U., Khan M., et al. (2021). Transcriptomic in silico analysis of bovine Escherichia coli mastitis highlights its immune-related expressed genes as an effective biomarker. J. Genet. Eng. Biotechnol. 19, 1–2. doi: 10.1186/s43141-021-00235-x
Ferronatto J. A., Ferronatto T. C., Schneider M., Pessoa L. F., Blagitz M. G., Heinemann M., et al. (2018). Diagnosing mastitis in early lactation: use of Somaticell®, California mastitis test, and somatic cell count. Ital. J. Anim. Sci. 17, 723–729. doi: 10.1080/1828051X.2018.1426394
Fesseha H., Mathewos M., Aliye S., Wolde A. (2021). Study on prevalence of bovine mastitis and associated risk factors in dairy farms of Modjo town and suburbs, central Oromia, Ethiopia. Vet. Med.: Res. Rep. 12, 271–283. doi: 10.2147/VMRR.S323460
Forsman P., Tilsaia-Timisjrvi A., Alatossava T. (1997). Identification of staphylococcal and streptococcal causes of bovine mastitis using 16S-23S rRNA spacer regions. Microbiology 143, 3491–3500. doi: 10.1099/00221287-143-11-3491
Fouad E. A., Abd El-Razik K. A., Abdel Rahman E. A. (2022). Diagnosis of Staphylococcus aureus infection in bovine mastitis using its affinity purified fraction. Adv. Anim. Vet. Sci. 10, 1887–2089. doi: 10.17582/journal.aavs/2022/10.9.1887.1893
Freitag C., Michael G. B., Kadlec K., Hassel M., Schwarz S. (2017). Detection of plasmid-borne extended-spectrum β-lactamase (ESBL) genes in Escherichia coli isolates from bovine mastitis. Vet. Microbiol. 200, 151–156. doi: 10.1016/j.vetmic.2016.08.010
Friman M., Hiitiö H., Niemi M., Holopainen J., Pyörälä S., Simojoki H. (2017). The effect of a cannula milk sampling technique on the microbiological diagnosis of bovine mastitis. Vet. J. 226, 57–61. doi: 10.1016/j.tvjl.2017.07.003
Garcia B. L., Fidelis C. E., Freu G., Granja B. D., Dos Santos M. V. (2021). Evaluation of chromogenic culture media for rapid identification of Gram-positive bacteria causing mastitis. Front. Vet. Sci. 8. doi: 10.3389/fvets.2021.662201
Gómez-Quispe O. E., Santivañez-Ballón C. S., Arauco-Villar F., Espezua-Flores O. H., Manrique-Meza J. (2015). Interpretation criteria for California Mastitis Test in the diagnosis of subclinical mastitis in cattle. doi: 10.15381/rivep.v26i1.10912
Goulart D. B., Mellata M. (2022). Escherichia coli mastitis in dairy cattle: etiology, diagnosis, and treatment challenges. Front. Microbiol. 13. doi: 10.3389/fmicb.2022.928346
Granja B. D., Fidelis C. E., Garcia B. L., Dos Santos M. V. (2021). Evaluation of chromogenic culture media for rapid identification of microorganisms isolated from cows with clinical and subclinical mastitis. J. Dairy Sci. 104, 9115–9129. doi: 10.3168/jds.2020-19513
Gross J. J., Bruckmaier R. M. (2019). Metabolic challenges in lactating dairy cows and their assessment via established and novel indicators in milk. Animal 13, 75–81. doi: 10.1017/s175173111800349x
Gurjar A., Gioia G., Schukken Y., Welcome F., Zadoks R., Moroni P. (2012). Molecular diagnostics applied to mastitis problems on dairy farms. Vet. Clin. North Am: Food Anim. Pract. 28, 565–576. doi: 10.1016/j.cvfa.2012.07.011
Haxhiaj K., Wishart D. S., Ametaj B. N. (2022). Mastitis: What it is, current diagnostics, and the potential of metabolomics to identify new predictive biomarkers. Dairy 3, 722–746. doi: 10.3390/dairy3040050
Halasa T., Kirkeby C. (2020). Differential somatic cell count: Value for udder health management. Front. Vet. Sci. 7, 609055. doi: 10.3389/fvets.2020.609055
He L., Chen B., Hu Y., Hu B., Li Y., Yang X. (2023). A sample-preparation-free, point-of-care testing system for in situ detection of bovine mastitis. Anal. Bioanalytic. Biochem. 415 (22), 5499-509. doi: 10.1007/s00216-023-04823-3
Hiitiö H., Riva R., Autio T., Pohjanvirta T., Holopainen J., Pyörälä S., et al. (2015). Performance of a real-time PCR assay in routine bovine mastitis diagnostics compared with in-depth conventional culture. J. Dairy Res. 82 (2), 200–208. doi: 10.1017/s0022029915000084
Hiitiö H., Simojoki H., Kalmus P., Holopainen J., Pyörälä S., Taponen S. (2016). The effect of sampling technique on PCR-based bacteriological results of bovine milk samples. J. dairy science. 99 (8), 6532–6541. doi: 10.3168/jds.2015-10811
Hoque M. N., Istiaq A., Clement R. A., Sultana M., Crandall K. A., Siddiki A. Z., et al. (2019). Metagenomic deep sequencing reveals the association of bacterial microbiome signature with functional biases in bovine mastitis. Sci. Rep. 9, 13536. doi: 10.1038/s41598-019-49468-4
Hoque M. N., Istiaq A., Rahman M. S., Islam M. R., Anwar A., Siddiki A. Z., et al. (2020). Microbiome dynamics and genomic determinants of bovine mastitis. Genomics 112, 5188–5203. doi: 10.1016/j.ygeno.2020.09.039
Huma Z., Sharma I., Kaur N., Tandon S., Guttula S., Kour P. K., et al. (2020). Putative biomarkers for early detection of mastitis in cattle. Anim. Product. Sci. 60, 1721–1736. doi: 10.1071/AN19539
Jashari R., Piepers S., De Vliegher S. (2016). Evaluation of the composite milk somatic cell count as a predictor of intramammary infection in dairy cattle. J. Dairy Sci. 99, 9271–9286. Available at: http://hdl.handle.net/1854/LU-6883987.
Jassim H. Y., Abdul-Wadood I. (2019). Efficacy of reliable milk and blood biomarkers for diagnosing clinical and subclinical bovine mastitis. Adv. Anim. Vet. Sci. 7, 898–903. doi: 10.17582/journal.aavs/2019/7.10.898.903
Jensen N. E., Knudsen K. (1991). Interquarter comparison of markers of subclinical mastitis: somatic cell count, electrical conductivity, N-acetyl-β-glucosaminidase, and antitrypsin. J. Dairy Res. 58, 389–399. doi: 10.1017/s002202990002999x
Jiménez E., de Andrés J., Manrique M., Pareja-Tobes P., Tobes R., Martínez-Blanch J. F., et al. (2015). Metagenomic analysis of milk of healthy and mastitis-suffering women. J. Hum. Lact. 31, 406–415. doi: 10.1177/0890334415585078
Jones G., Bork O., Ferguson S. A., Bates A. (2019). Comparison of an on-farm point-of-care diagnostic with conventional culture in analyzing bovine mastitis samples. J. Dairy Res. 86 (2), 222–225. doi: 10.1017/s0022029919000177
Kabelitz T., Aubry E., van Vorst K., Amon T., Fulde M. (2021). The role of Streptococcus spp. in bovine mastitis. Microorganisms 9, 1497. doi: 10.3390/microorganisms9071497
Kalmus P., Simojoki H., Pyörälä S., Taponen S., Holopainen J., Orro T. (2013). Milk haptoglobin, milk amyloid A, and N-acetyl-β-d-glucosaminidase activity in bovines with naturally occurring clinical mastitis diagnosed with a quantitative PCR test. J. Dairy Sci. 96 (6), 3662–3670. doi: 10.3168/jds.2012-6177
Kandeel S. A., Megahed A. A., Ebeid M. H., Constable P. D. (2017). Clinical utility of two leukocyte esterase reagent strips for the cow-side diagnosis of subclinical mastitis in lactating dairy cattle. Assiut Vet. Med. J. 63, 109–118. doi: 10.3168/jds.2017-14345
Kandeel S. A., Megahed A. A., Ebeid M. H., Constable P. D. (2019). Evaluation of 3 esterase tests for the diagnosis of subclinical mastitis at dry-off and freshening in dairy cattle. J. Dairy Sci. 102, 1402–1416. doi: 10.3168/jds.2017-14345
Kasai S., Prasad A., Kumagai R., Takanohashi K. (2022). Scanning electrochemical microscopy-somatic cell count as a method for diagnosis of bovine mastitis. Biology 11, 549. doi: 10.3390/biology11040549
Keane O. M., Budd K. E., Flynn J., McCoy F. (2013). Increased detection of mastitis pathogens by real-time PCR compared to bacterial culture. Vet. Rec. 173, 268–268. doi: 10.1136/vr.101598
Kelton D., Godkin M. A. (2014). “Mastitis culture programs for dairy herds,” in Annual Meeting-national Mastitis Council Incorporated. 39, 54–62.
Khatun M., Bruckmaier R. M., Thomson P. C., House J., García S. C. (2019). Suitability of somatic cell count, electrical conductivity, and lactate dehydrogenase activity in foremilk before versus after alveolar milk ejection for mastitis detection. J. Dairy Sci. 102, 9200–9212. doi: 10.3168/jds.2018-15752
Klaas I. C., Zadoks R. N. (2018). An update on environmental mastitis: Challenging perceptions. Transbound. Emerg. Dis. 65, 166–185. doi: 10.1111/tbed.12704
Koskinen M. T., Holopainen J., Pyörälä S., Bredbacka P., Pitkälä A., Barkema H. (2009). Analytical specificity and sensitivity of a real-time polymerase chain reaction assay for the identification of bovine mastitis pathogens. J. Dairy Sci. 92, 952–959. doi: 10.3168/jds.2008-1549
Koskinen M. T., Wellenberg G. J., Sampimon O. C., Holopainen J., Rothkamp A., Salmikivi L. (2010). Field comparison of real-time polymerase chain reaction and bacterial culture for identification of bovine mastitis bacteria. J. Dairy Sci. 93, 5707–5715. doi: 10.3168/jds.2010-3167
Kour S., Sharma N. N. B., Kumar P., Soodan J. S., Santos M. V., Son Y. O. (2023). Advances in diagnostic approaches and therapeutic management in bovine mastitis. Vet. Sci. 10 (7), 449. doi: 10.3390/vetsci10070449
Kuehn J. S., Gorden P. J., Munro D., Rong R., Dong Q., Plummer P. J., et al. (2013). Bacterial community profiling of milk samples as a means to understand culture-negative bovine clinical mastitis. PloS One 8 (4), e61959. doi: 10.1371/journal.pone.0061959
Kumar P., Ojasvita A. D., Himanshu Sharma S. S., Dinesh Mittal V. B., Anand Prakash R. Y., RP D. (2020). Bovine mastitis: A review. Middle East J. Sci. Res. 28, 497–507. doi: 10.5829/idosi.mejsr.2020.497.507
Kumari T., Bhakat C., Choudhary R. K. (2018). A review on subclinical mastitis in dairy cattle. Int. J. Pure Appl. Biosci. 6, 1291–1299. doi: 10.18782/2320-7051.6173
Lago A., Godden S. M. (2018). Use of rapid culture systems to guide clinical mastitis treatment decisions. Vet. Clinics: Food Anim. Pract. 34, 389–412. doi: 10.1016/j.cvfa.2018.06.001
Lai Y. C., Fujikawa T., Maemura T., Ando T., Kitahara G., Endo Y., et al. (2017). Inflammation-related microRNA expression level in the bovine milk is affected by mastitis. PloS One 12, e0177182. doi: 10.1371/journal.pone.0177182
Lakshmi R. (2016). Bovine mastitis and its diagnosis. Int. J. Appl. Res. 2, 213–216. doi: 10.5539/jas.v13n10p162
Lange C. C., Brito M. A., Reis D. R., MaChado M. A., Guimaraes A. S., Azevedo A. L., et al. (2015). Species-level identification of staphylococci isolated from bovine mastitis in Brazil using partial 16S rRNA sequencing. Vet. Microbiol. 176, 382–388. doi: 10.1016/j.vetmic.2015.01.024
Lee K. H., Lee J. W., Wang S. W., Liu L. Y., Lee M. F., Chuang S. T., et al. (2008). Development of a novel biochip for rapid multiplex detection of seven mastitis-causing pathogens in bovine milk samples. J. Vet. Diagn. Invest. 20, 463–471. doi: 10.1177/104063870802000408
Leimbach S., Krömker V. (2018). Laboratory evaluation of a novel rapid tube test system for differentiation of mastitis-causing pathogen groups. J. Dairy Sci. 101, 6357–6365. doi: 10.3168/jds.2017-14198
Leung K. T., Mackereth R., Tien Y. C., Topp E. (2004). A comparison of AFLP and ERIC-PCR analyses for discriminating Escherichia coli from cattle, pig and human sources. FEMS Microbiol. Ecol. 47 (1), 111–119. doi: 10.1016/s0168-6496(03)00254-x
Li L., Chen X., Chen Z. (2019). Identification of key candidate genes in dairy cows in response to Escherichia coli mastitis by bioinformatical analysis. Front. Genet. 10. doi: 10.3389/fgene.2019.01251
MacDonald K. A. (2013). Evaluation of a 3M Petrifilm on-farm mastitis culture system and treatment decision algorithm for clinical mastitis in Canada. Canada, Ottawa: Library and Archives Canada= Bibliothè̀que et Archives.
MaChado I., Goikoetxea G., Alday E., Jiménez T., Arias-Moreno X., Hernandez F. J. (2021). Ultra-sensitive and specific detection of S. aureus bacterial cultures using an oligonucleotide probe integrated into a lateral flow-based device. Diagnostics 11, 2022. doi: 10.3390/diagnostics11112022
Mahmmod Y. S., Klaas I. C., Enevoldsen C. (2017). DNA carryover in milk samples from routine milk recording used for PCR-based diagnosis of bovine Staphylococcus aureus mastitis. J. Dairy Sci. 100, 5709–5716. doi: 10.3168/jds.2016-12330
Malcata F. B., Pepler P. T., O’Reilly E. L., Brady N., Eckersall P. D., Zadoks R. N., et al. (2020). Point-of-care tests for bovine clinical mastitis: what do we have and what do we need? J. Dairy Res. 87, 60–66. doi: 10.1017/S002202992000062X
Martins S. A. M., Martins V. C., Cardoso F. A., Germano J., Rodrigues M., Duarte C., et al. (2019). Biosensors for on-farm diagnosis of mastitis. Front. Bioeng. Biotechnol. 7. doi: 10.3389/fbioe.2019.00186
McCarron J. L., Keefe G. P., McKenna S. L., Dohoo I. R., Poole D. E. (2009). Laboratory evaluation of 3M Petrifilms and University of Minnesota Bi-plates as potential on-farm tests for clinical mastitis. J. Diary Sci.. 92 (5), 2297-2305. doi: 10.3168/jds.2008-1661
McMillan A., Orimadegun A. E., Sumarah M. W., Renaud J., Da Encarnacao M. M., Gloor G. B., et al. (2017). Metabolic derangements identified through untargeted metabolomics in a cross-sectional study of Nigerian children with severe acute malnutrition. Metabolomics 13, 1–4. doi: 10.1007/s11306-016-1150-2
Mediano P., Fernández L., Jiménez E., Arroyo R., Espinosa-Martos I., Rodríguez J. M., et al. (2017). Microbial diversity in the milk of women with mastitis: potential role of coagulase-negative staphylococci, viridans group streptococci, and corynebacteria. J. Hum. Lact. 33, 309–318. doi: 10.1177/0890334417692968
Mengistie D. (2022). PCR-based detection and identification of mastitis-causing bacteria: A review. Arch. Infect. Dis. Ther. 6, 163–168. Available at: https://api.semanticscholar.org/CorpusID:249660637.
Metzger S. A., Hernandez L. L., Skarlupka J. H., Walker T. M., Suen G., Ruegg P. L. (2018a). A cohort study of the milk microbiota of healthy and inflamed bovine mammary glands from dryoff through 150 days in milk. Front. Vet. Sci. 5. doi: 10.3389/fvets.2018.00247
Metzger S. A., Hernandez L. L., Suen G., Ruegg P. L. (2018b). Understanding the milk microbiota. Vet. Clinics: Food Anim. Pract. 34, 427–438. doi: 10.1016/j.cvfa.2018.06.003
Middleton J. R., Luby C. D., Viera L., Tyler J. W., Casteel S. (2004). Influence of Staphylococcus aureus intramammary infection on serum copper, zinc, and iron concentrations. J. Dairy Sci. 87, 976–979. doi: 10.3168/jds.S0022-0302(04)73242-7
Mitsunaga T. M., Nery Garcia B. L., Pereira L. B. R., Costa Y. C. B., da Silva R. F., Delbem A. C. B., et al. (2024). Current trends in artificial intelligence and bovine mastitis research: A bibliometric review approach. Animals: Open Access J. MDPI 14, 14. doi: 10.3390/ani14142023
Mohammed S., Bark N., Sayed M. (2019). Detection of subclinical mastitis in the milk of dairy cows in Sohag city, Egypt. Assiut Vet. Med. J. 65, 51–58. doi: 10.21608/avmj.2019.167298
Mukherjee J., De K., Das P. K., Ghosh P. R., Banerjee D. (2023). Biosensor-based approach to detect bovine mastitis. Indian J. Anim. Health 62, 151–156. doi: 10.36062/ijah.2023.spl.03023
Murphy B. P., McCabe E., Murphy M., Buckley J. F., Crowley D., Fanning S., et al. (2016). Longitudinal study of two Irish dairy herds: low numbers of Shiga toxin-producing Escherichia coli O157 and O26 super-shedders identified. Front. Microbiol. 7. doi: 10.3389/fmicb.2016.01850
Nagasawa Y., Kiku Y., Sugawara K., Yabusaki N., Oono K., Fujii K., et al. (2020). Rapid Staphylococcus aureus detection from clinical mastitis milk by colloidal gold nanoparticle-based immunochromatographic strips. Front. Vet. Sci. 6, 504. doi: 10.3389/fvets.2019.00504
Narváez-Semanate J. L., Daza-Bolaños C. A., Valencia-Hoyos C. E., Hurtado-Garzón D. T., Acosta-Jurado D. C. (2022). Diagnostic methods of subclinical mastitis in bovine milk: an overview. Rev. Facultad Nacional Agronomía Medellín 75, 10077–10088. doi: 10.15446/rfnam.v75n3.100520
Neculai-Valeanu A. S., Ariton A. M. (2022). Udder health monitoring for prevention of bovine mastitis and improvement of milk quality. Bioengineering 9, 608. doi: 10.3390/bioengineering9110608
Novac C. S., Andrei S. (2020). The Impact of mastitis on the biochemical parameters, oxidative and nitrosative stress markers in goat’s milk: A review. Pathogens 9, 882. doi: 10.3390/pathogens9110882
Nyman A. K., Persson Waller K., Bennedsgaard T. W., Larsen T., Emanuelson U. (2014). Associations of udder-health indicators with cow factors and with intramammary infection in dairy cows. J. Dairy Sci. 97, 5459–5473. doi: 10.3168/jds.2013-7885
Nyman A. K., Waller K. P., Emanuelson U., Frössling J. (2016). Sensitivity and specificity of PCR analysis and bacteriological culture of milk samples for identification of intramammary infections in dairy cows using latent class analysis. Prev. Veterinary Med. 135, 123–131. doi: 10.1016/j.prevetmed.2016.11.009
O’Reilly E. L., Viora L., Malcata F., Pepler P. T., Zadoks R., Brady N., et al. (2024). Biomarker and proteome analysis of milk from dairy cows with clinical mastitis: Determining the effect of different bacterial pathogens on the response to infection. Res. Vet. Sci. 172, 105240. doi: 10.1016/j.rvsc.2024.105240
Oikonomou G., MaChado V. S., Santisteban C., Schukken Y. H., Bicalho R. C. (2012). Microbial diversity of bovine mastitic milk as described by pyrosequencing of metagenomic 16s rDNA. PLoS ONE 7 (10), e47671. doi: 10.1371/journal.pone.0047671
Ombarak R. A., Hinenoya A., Elbagory A. M., Yamasaki S. (2018). Prevalence and molecular characterization of antimicrobial resistance in Escherichia coli isolated from raw milk and raw milk cheese in Egypt. J. Food Prot. 81, 226–232. doi: 10.4315/0362-028X.JFP-17-277
Oultram J. W., Ganda E. K., Boulding S. C., Bicalho R. C., Oikonomou G. (2017). A metataxonomic approach could be considered for cattle clinical mastitis diagnostics. Front. Vet. Sci. 4. doi: 10.3389/fvets.2017.00036
Parente E., Ricciardi A., Zotta T. (2020). The microbiota of dairy milk: A review. Int. Dairy J. 107, 104714.AA. Available at: https://pmc.ncbi.nlm.nih.gov/articles/PMC9878191/.
Pascu C., Herman V., Iancu I., Costinar L. (2022). Etiology of mastitis and antimicrobial resistance in dairy cattle farms in the western part of Romania. Antibiotics 11, 57. doi: 10.3390/antibiotics11010057
Paudyal S., Melendez P., Manriquez D., Velasquez-Munoz A., Pena G., Roman-Muniz I. N., et al. (2020). Use of milk electrical conductivity for the differentiation of mastitis-causing pathogens in Holstein cows. Animal 14, 588–596. doi: 10.1017/S1751731119002210
Pumipuntu N., Kulpeanprasit S., Santajit S., Tunyong W., Kong-Ngoen T., Hinthong W., et al. (2017). Screening method for Staphylococcus aureus identification in subclinical bovine mastitis from dairy farms. Vet. World 10 (7), 721. doi: 10.14202/vetworld.2017.721-726
Ramirez-Morales I., Aguilar L., Fernandez-Blanco E., Rivero D., Perez J., Pazos A. (2021). Detection of bovine mastitis in raw milk, using a low-cost NIR spectrometer and k-NN algorithm. Appl. Sci. 11, 10751. doi: 10.3390/app112210751
Renald E., Buza J., Tchuenche J. M., Masanja V. G. (2023). The role of modeling in the epidemiology and control of lumpy skin disease: a systematic review. Bull. Natl. Res. Centre 47 (1), 141. doi: 10.1186/s42269-023-01111-z
Roberts J. (2024). CPD article: The California mastitis test: what is the value? Livestock 29, 184–193. doi: 10.12968/live.2024.0011
Rodrigues A. C., Cassoli L. D., MaChado P. F., Ruegg P. L. (2009). Evaluation of an on-farm test to estimate somatic cell count. J. Dairy Sci. 92, 990–995. doi: 10.3168/jds.2008-1216
Rodrigues M. X., Lima S. F., Canniatti-Brazaca S. G., Bicalho R. C. (2017). The microbiome of bulk tank milk: Characterization and associations with somatic cell count and bacterial count. J. Dairy Sci. 100, 2536–2552. doi: 10.3168/jds.2016-11540
Rötzer V., Wenderlein J., Wiesinger A., Versen F., Rauch E., Straubinger R. K., et al. (2023). Bovine udder health: from standard diagnostic methods to new approaches—a practical investigation of various udder health parameters in combination with 16s rrna sequencing. Microorganisms 11 (5), 1311. doi: 10.3390/microorganisms11051311
Royster E., Godden S., Goulart D., Dahlke A., Rapnicki P., Timmerman J. (2014). Evaluation of the Minnesota Easy Culture System II Bi-Plate and Tri-Plate for identification of common mastitis pathogens in milk. J. Dairy Sci. 97, 3648–3659. doi: 10.3168/jds.2013-7748
Sadek K., Saleh E., Ayoub M. (2017). Selective, reliable blood and milk biomarkers for diagnosing clinical and subclinical bovine mastitis. Trop. Anim. Health Product. 49, 431–437. doi: 10.1007/s11250-016-1190-7
Sajid M., Kawde A. N., Daud M. (2015). Designs, formats, and applications of lateral flow assay: a literature review. J. Saudi Chem. Soc. 19, 689–705. doi: 10.1016/j.jscs.2014.09.001
Sakwinska O., Bosco N. (2019). Host-microbe interactions in the lactating mammary gland. Front. Microbiol. 10. doi: 10.3389/fmicb.2019.01863
Savage E., Chothe S., Lintner V., Pierre T., Matthews T., Kariyawasam S., et al. (2017). Evaluation of three bacterial identification systems for species identification of bacteria isolated from bovine mastitis and bulk tank milk samples. Foodborne Pathog. Dis. 14, 177–187. doi: 10.1089/fpd.2016.2222
Sayed R. H., Soliman R. T., Elsaady S. A. (2023). Development of a lateral flow device for rapid simultaneous multiple detections of some common bacterial causes of bovine mastitis. J. Adv. Vet. Anim. Res. 10, 292–300. doi: 10.5455/javar.2023.j681
Schukken Y. H., Wilson D. J., Welcome F., Garrison-Tikofsky L., Gonzalez R. N. (2003). Monitoring udder health and milk quality using somatic cell counts. Vet. Res. 34, 579–596. doi: 10.1051/vetres:2003028
Sharifi S., Pakdel A., Ebrahimi M., Reecy J. M., Fazeli Farsani S., Ebrahimie E. (2018). Integration of machine learning and meta-analysis identifies the transcriptomic bio-signature of mastitis disease in cattle. PloS One 13 (2), e0191227. doi: 10.1371/journal.pone.0191227
Solntceva V., Kostrzewa M., Larrouy-Maumus G. (2021). Detection of species-specific lipids by routine MALDI TOF mass spectrometry to unlock the challenges of microbial identification and antimicrobial susceptibility testing. Front. Cell. Infect. Microbiol. 10. doi: 10.3389/fcimb.2020.621452
Srikok S., Patchanee P., Boonyayatra S., Chuammitri P. (2020). Potential role of MicroRNA as a diagnostic tool in the detection of bovine mastitis. Prev. vet. Med. 182, 105101. doi: 10.1016/j.prevetmed.2020.105101
Stanek P., Żółkiewski P., Januś E. (2024). A review on mastitis in dairy cows research: current status and future perspectives. Agriculture 14, 1292. doi: 10.3390/agriculture14081292
Swami S. V., Patil R. A., Gadekar S. D. (2017). Studies on the prevalence of subclinical mastitis in dairy animals. J. Entomol. Zool. Stud. 5, 1297–1300. Available at: https://www.thepharmajournal.com/.
Swinkels J. M., Leach K. A., Breen J. E., Payne B., White V., Green M. J., et al. (2021). Randomized controlled field trial comparing quarter and cow level selective dry cow treatment using the California Mastitis Test. J. Dairy Sci. 104 (8), 9063–9081. doi: 10.3168/jds.2020-19258
Taponen S., Salmikivi L., Simojoki H., Koskinen M. T., Pyörälä S. (2009). Real-time polymerase chain reaction-based identification of bacteria in milk samples from bovine clinical mastitis with no growth in conventional culturing. J. Dairy Sci. 92, 2610–2617. doi: 10.3168/jds.2008-1729
Teshome Gemechu T. G., Hasen Awel Yunus H. A., Morga Soma M. S., Amare Beyene A. B. (2019). Bovine mastitis: prevalence, isolation and identification of major bacterial pathogens in selected areas of Bench Maji Zone, Southwest Ethiopia. J. Vet. Med. Anim. Health 11, 30–36. doi: 10.5897/JVMAH2018.0731
Thompson J. E. (2022). Matrix-assisted laser desorption ionization-time-of-flight mass spectrometry in veterinary medicine: Recent advances, (2019–present). Vet. World 15, 2623. doi: 10.14202/vetworld.2022.2623-2657
Thompson J., Everhart Nunn S. L., Sarkar S., Clayton B. (2023). Diagnostic screening of bovine mastitis USING MALDI-TOF MS direct-spotting of milk and machine learning. Vet. Sci. 10 (2), 101. doi: 10.3390/vetsci10020101
Tommasoni C., Fiore E., Lisuzzo A., Gianesella M. (2023). Mastitis in dairy cattle: on-farm diagnostics and future perspectives. Animals 13, 2538. doi: 10.3390/ani13152538
Upadhyay N., Nara S. (2018). Lateral flow assay for rapid detection of Staphylococcus aureus enterotoxin A in milk. Microchem. J. 137, 435–442. doi: 10.1016/j.microc.2017.12.011
Vakkamäki J., Taponen S., Heikkilä A. M., Pyörälä S. (2017). Bacteriological etiology and treatment of mastitis in Finnish dairy herds. Acta Veterinaria Scandinavica 59, 1-9. doi: 10.1186/s13028-017-0301-4
Vasquez A. K., Ganda E. K., Capel M. B., Eicker S., Virkler P. D., Bicalho R. C., et al. (2019). The microbiome of Escherichia coli and culture-negative nonsevere clinical mastitis: Characterization and associations with linear score and milk production. J. Dairy Sci. 102, 578–594. doi: 10.3168/jds.2018-15062
Wang M., Zhang Y., Li B., Zhu J. (2015). Construction of scFv that binds both fibronectin-binding protein A and clumping factor A of Staphylococcus aureus. Res. Vet. Sci. 100, 109–114. doi: 10.1016/j.rvsc.2015.02.012
Wilson D. J., Middleton J. R., Adkins P. R. F., Goodell G. M. (2019). Test agreement among biochemical methods, matrix-assisted laser desorption ionization-time of flight mass spectrometry, and 16S rRNA sequencing for identification of microorganisms isolated from bovine milk. J. Clin. Microbiol. 57, 10–1128. doi: 10.1128/JCM.01381-18
Yong Q., Wei L., Xiaoling L., Wanpeng S., Ruichen L., Nianhong L. (2022). Rapid visual detection of pathogenic Streptococcus suis Type 2 through a recombinase polymerase amplification assay coupled with lateral flow test. Zoonoses 2, 16. doi: 10.15212/ZOONOSES-2022-0015
Zadoks R. N., Middleton J. R., McDougall S., Katholm J., Schukken Y. H. (2011). Molecular epidemiology of mastitis pathogens of dairy cattle and comparative relevance to humans. J. Mamm. Gland Biol. Neoplasia 16, 357–372. doi: 10.1007/s10911-011-9236-y
Zeinhom M. M., Abdel Aziz R. L., Mohammed A. N., Bernabucci U. (2016). Impact of seasonal conditions on quality and pathogens content of milk in Friesian cows. Asian-Australasian J. Anim. Sci. 29, 1207. doi: 10.5713/ajas.16.0143
Zhang X. D., Gu B., Usman M., Tang J. W., Li Z. K., Zhang X. Q., et al. (2022). “Recent Progress in the Diagnosis of Staphylococcus in Clinical Settings,” in Staphylococcal Infections-Recent Advances and Perspectives. IntechOpen. doi: 10.5772/intechopen.108524
Zhu Z., Lin B., Zhu X., Guo W. (2023). A rapid method of identifying mastitis degrees of bovines based on dielectric spectra of raw milk. Food Qual. Saf. 7, fyad014. doi: 10.1093/fqsafe/fyad014
Keywords: biomarkers, chromogenic plates, California mastitis test, milk yield, udder health
Citation: Ramuada M, Tyasi TL, Gumede L and Chitura T (2024) A practical guide to diagnosing bovine mastitis: a review. Front. Anim. Sci. 5:1504873. doi: 10.3389/fanim.2024.1504873
Received: 01 October 2024; Accepted: 22 November 2024;
Published: 16 December 2024.
Edited by:
Francesca Conte, University of Messina, ItalyReviewed by:
Samah Attia Algharib, Benha University, EgyptAzza SalahEldin El-Demerdash, Animal Health Research Institute, Egypt
Copyright © 2024 Ramuada, Tyasi, Gumede and Chitura. This is an open-access article distributed under the terms of the Creative Commons Attribution License (CC BY). The use, distribution or reproduction in other forums is permitted, provided the original author(s) and the copyright owner(s) are credited and that the original publication in this journal is cited, in accordance with accepted academic practice. No use, distribution or reproduction is permitted which does not comply with these terms.
*Correspondence: Teedzai Chitura, Y2hpdHVyaXRpc0BnbWFpbC5jb20=; VGVlZHphaS5DaGl0dXJhQHVuaXZlbi5hYy56YQ==