- 1Department of Animal and Rangeland Sciences, Oregon State University, Corvallis, OR, United States
- 2Carlson College of Veterinary Science, Oregon State University, Corvallis, OR, United States
- 3Department of Animal Sciences, Food and Nutrition, Università Cattolica del Sacro Cuore, Piacenza, Italy
- 4Tropical Feed Resources Research and Development Center (TROFREC), Department of Animal Science, Faculty of Agriculture, Khon Kaen University, Khon Kaen, Thailand
In the study, we assessed the effect on performance and health of a single injection of inorganic Se prepartum or feeding chicory silage and organic Se supplementation during the peripartum in ewes. Approximately one month before lambing, 45 pregnant Polypay ewes were moved into single pens and randomly assigned to 5 groups to be fed either grass or chicory silage and supplemented or not with 3.6 mg Se/day as selenium yeast or given a single prepartum injection of Na-selenite. Daily dry matter intake (DMI), water intake, milk production and components, blood metabolic, immune and inflammatory parameters, and blood micromineral levels were measured. DMI was lower in ewes fed chicory silage, although no statistical differences in milk yield were observed. Very few differences were observed in milk components, except fat %, which was higher among ewes fed chicory silage. The type of silage had a significant effect on the fatty acid profile of the milk, with the milk from ewes fed chicory having a higher proportion of unsaturated fatty acids and overall improved health indices compared to the milk from ewes fed grass silage. Blood NEFA and BHBA were higher in ewes fed chicory vs. grass silage. Neither silage type nor Se supplementation had a strong effect on most of the parameters associated with immune or inflammatory function, except for the liver enzymes GGT and GOT, which were lower, and a larger type I/type II ratio immune response measured by the DxD2 assay among ewes fed chicory vs. grass silage. No effects on parasite fecal egg counts were observed. Supplementation of ewes with Se-yeast resulted in higher blood levels of Se, whereas the one-time prepartum injection had no significant effect on whole blood Se levels. Feeding chicory silage and supplementing Se during the transition period had a minimal impact on ewe performance and health.
1 Introduction
The transition from pregnancy to lactation is a critical time for dairy animals, with significant consequences for their health and productive capacity. This transition period – typically referring to three weeks before and after parturition – is hallmarked by dramatic physiological and metabolic changes that must occur to meet the sudden demand for copious milk production (Drackley, 1999). The increased mobilization and utilization of fats for energy during early lactation can lead to increased production of reactive oxygen species (ROS), one of the primary contributors to oxidative stress (Bernabucci et al., 2005; Sordillo and Aitken, 2009). For ruminants, this increased oxidative stress can negatively affect milk production (Abuelo et al., 2015). Inflammatory-like conditions during this period can also disrupt normal liver function, negatively impacting performance (Bionaz et al., 2007; Bertoni et al., 2008; Trevisi et al., 2012). Together, these factors can contribute to immune dysfunction and increased susceptibility to diseases (Mezzetti et al., 2020).
Given its well-known role in improving antioxidant function (Wichtel, 1998), the micromineral Se may be able to ameliorate some of the challenges faced by ruminants during the transition from pregnancy to lactation. In the greater Pacific Northwest, including Oregon, reaching the recommended dietary level of Se for ruminants can be challenging due to the low Se levels in forages caused by deficiency of Se in soils (USGS, 2021). Consequently, ruminants raised in this area are Se-deficient, and supplementation is necessary. Selenium that is supplemented typically comes in either the inorganic form, like sodium selenite or selenate, or in the organic form, often as selenium-yeast or hydroxy-selenomethionine (Mehdi and Dufrasne, 2016). Because elevated levels of inorganic Se can be toxic when supplemented, it is regulated by the FDA as a livestock feed additive not to exceed 0.3 ppm. For sheep, this translates to an allowable intake of 0.7 mg/head/day. Organic Se is mainly selenomethionine and selenocysteine, which are absorbed as all the other amino acids, making them highly available and non-toxic for dairy cattle to produce antioxidant selenoprotein compared to the inorganic form (Séboussi et al., 2016). Similar results have been shown in sheep, where Se yeast has a higher bioavailability than sodium selenite (Hall et al., 2012) and was more efficiently transferred to the lambs (Stewart et al., 2012). Supplementing organic Se during the transition period in sheep positively affects the antioxidant status and immune response (Hall et al., 2011). Despite those data, injection of inorganic selenium is a common practice in the livestock industry, especially in sheep, even though it is known to have only a short-term response (Hemingway, 2003). It is unclear if an injection of Se could have a similar positive effect on peripartum animals as feeding organic Se.
In dairy animals, types of forages can also have a strong influence on animal performance during the transition period. Chicory (Chicorum intybus) has garnered significant attention as an alternative forage for ruminants for its high nutritive value, drought resistance, and high amount of bioactive compounds (Nwafor et al., 2017). These bioactive compounds have been linked to the observed antiparasitic effect of chicory in ruminants (Peña-Espinoza et al., 2018) as well as shifts toward improved nitrogen metabolism (Cheng et al., 2017; Wilson et al., 2020). The observed positive effects of chicory could be beneficial to peripartum animals although it is known to negatively affect the taste of the milk.
While previous work has primarily focused on feeding chicory in pasture or as a fresh-cut forage, limited work has focused on its benefits after it has been ensiled. Fresh forages typically contain high concentrations of bioactive compounds but are not suitable for use in traditional confinement-based systems where silage is more common. Prior work has demonstrated that levels of bioactive compounds like saponins can decrease during the silage process limiting their availability to animals (Tian et al., 2018).
Given the lack of studies combining Se supplementation and forages containing bioactive compounds like chicory, the first objective of our study was to assess the effect of combining chicory silage with supplementation of organic Se on the performance and health of dairy animals during the transition period using sheep as a model. A second objective of this study was to assess the effectiveness of a one-time Se injection vs. daily supplementation of organic Se in the same animals. Our hypotheses for this study were 1) that the combination of the chicory and Se-yeast improves the performance and health of sheep during the transition period, especially by boosting the immune function, and 2) that using organic Se is more effective in improving the above conditions than a single subcutaneous injection of Se.
2 Materials and methods
2.1 Experimental design
Experimental procedures used in this study were approved by the Institutional Animal Care and Use Committees (IACUC) of Oregon State University (protocol# 2020-0122). Forty-five pregnant Polypay ewes from the Oregon State University Sheep Center were moved into individual 1.5m×1.5m pens at the HOGG Animal Metabolism Laboratory Building (HAMB) at the Oregon State University campus. The pens were bedded with wood shavings until lambing and with wheat straw after lambing and bedding was added as needed and replaced every 3-4 days. A layout of the pens before the animals were placed in them can be seen in Supplementary Figure S1.
Animals were transported to the HAMB at around 39 days prior to expected lambing. Ewes were weighed, and blood was collected the day before (i.e., baseline). After an adaptation period of 7 days to the new location, pen, and feed, the experiment was started. The experiment period ranged between -28 days from expecting lambing to 30 days post-lambing (i.e., day in milk or DIM) (Figure 1). As typical management practice of the OSU Sheep Center, all ewes were given Clostridium Perfringens Types C & D – Tetanus Toxoid (CD/T) shots (Bar-Vac, Boehringer Ingelheim Animal Health USA Inc. St. Joseph, MO) approximately 30 days before expected parturition.
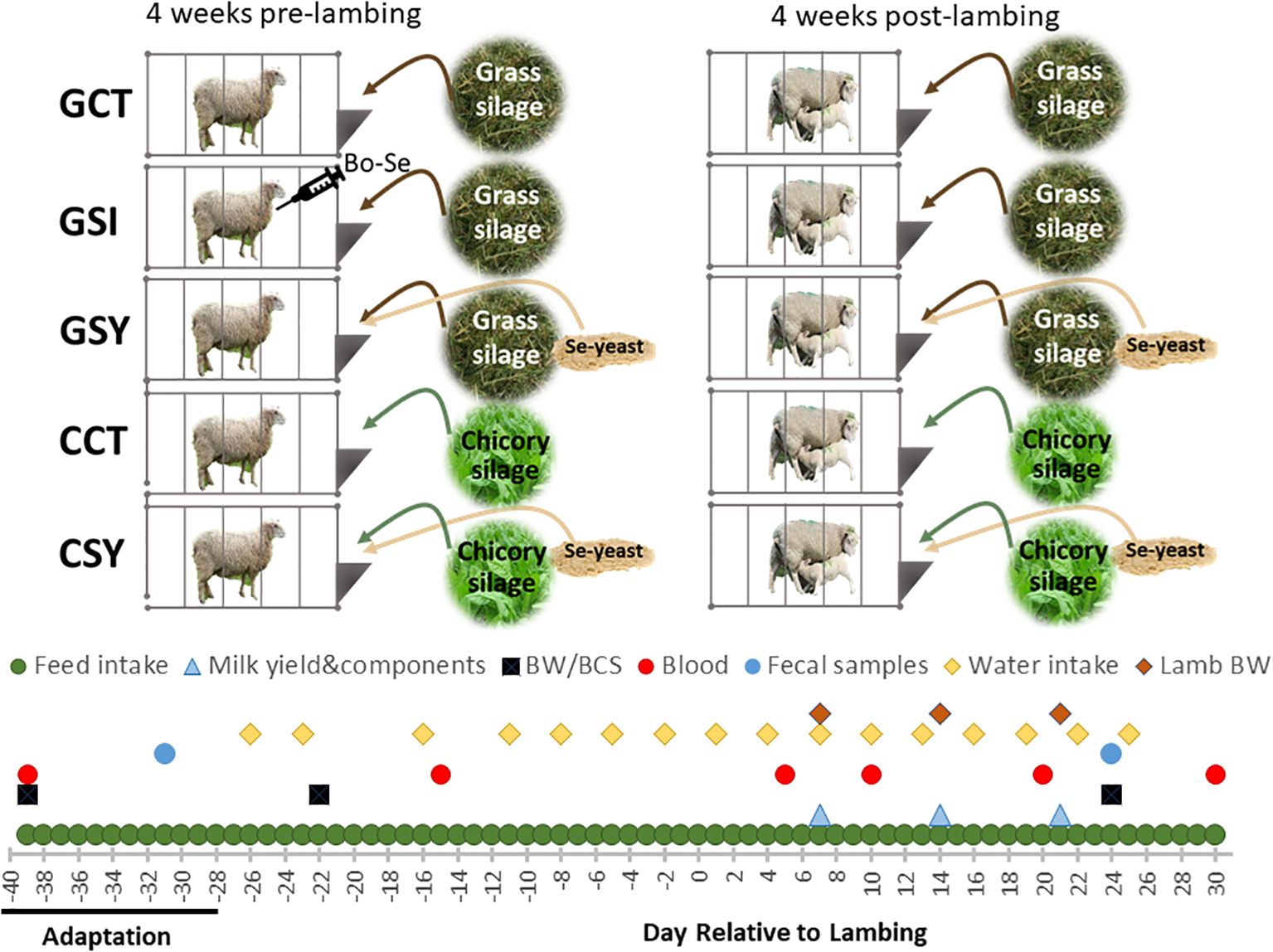
Figure 1. Experimental design showing the silage and selenium treatments applied to each group of ewes. After a period of adaptation to pens and diets, recording for the experiment started at approx. 28 days from expected lambing until 30 days post-lambing. Indicated is the timeline for the various samplings and measurements during the experiment. One group received an injection of inorganic Se (Bo-Se, containing vit. E) at approx. 20 days prior lambing while the other animals received an equivalent amount of vitamin E.
The ewes were randomized based on body weight (BW) into 5 treatment groups (n=9/group). Four groups differed in the type of forage and whether or not supplemental Se-yeast (DiaMune, generously donated by Diamond V) was included in the diet (Figure 1): GCT, ewes fed grass silage with no additional Se in the grain; GSY, ewes fed grass silage with additional Se-yeast in the grain; CCT, ewes fed chicory silage with no additional Se in the grain, and CSY ewes fed chicory silage with additional Se-yeast in the grain. The Se-yeast was provided at a dose of 3.6 mg Se/d based on a 25 mg Se/week determined to be a safe and efficacious dose of Se yeast in a prior experiment in peripartum ewes (Hall et al., 2012). The Se yeast contained 240 ppm of Se (or 0.24 mg/g), so the total amount of Se yeast provided was 15 g/d per head. We used yeast as organic Se and chicory as silage due to their commercial availability and ease of use in a commercial indoor ratio.
To address the second objective, an additional group GSI was added where ewes were fed as the GCT group, but the animals received an injection of 11.3 µL/kg BW (2.5 ml/100 lb. BW or 2.5 mg of Se/100 lb. BW) of sodium selenite as indicated by the manufacturer (BO-SE, MERK Animal Health, USA) at 24(+/-7) days before parturition. The BO-SE contains 68 USP units of vitamin E as d-α-tocopheryl acetate. Thus, all non-GSI ewes were injected with an equivalent amount of Vitamin E (Vitamin E-300 Injection, vetone, USA).
Animals were provided ad libitum access to drinkable city water via automatic waterers inside of each pen (MACGOAL Stainless Steel Automatic Waterer) equipped with a Rainwave RW-9FM LCD Digital water flow meter (Supplementary Figure S2). Water use was recorded every 3 days in the morning, and the meter was reset to 0.
2.2 Nutrient intake analysis
The nutrient intake of Crude Protein (CP), neutral detergent fiber (NDF), acid detergent fiber (ADF) and metabolizable energy (ME) was determined by analyzing the nutrient composition of the residual orts during three different time periods during the experiment. Briefly, residual orts were collected every other day from each ewe over a period of five days during three different periods during the experiment. A subsample of the orts collected from each ewe were then sent to the DairyOne Forage Laboratory (730 Warren Road, Ithaca, New York) for analysis of CP, NDF, ADF and ME. Using the nutrient profile and amount of feed offered compared to the nutrient profile and amount of feed consumed, the nutrient intake of each ewe was calculated.
2.3 Diets and feeding
Before starting the experiment, ewes were located at the Sheep Center of the Oregon State University and were fed a typical orchard grass hay with access to a standard free-choice sheep mineral containing 90 ppm Se. Ewes were fed a combination of either grass or chicory silage and grain that either contained supplemental Se-yeast or alfalfa meal (as placebo) to ensure that diets were isocaloric and isonitrogenous (Table 1). Nutritive analysis was performed by a commercial laboratory (Cumberland Valley Analytical Services, PA). Results of the nutritive analysis plus the content of microminerals and fatty acids assessed in our laboratory as described below of the two silages are available in Supplementary Tables S1, S2.
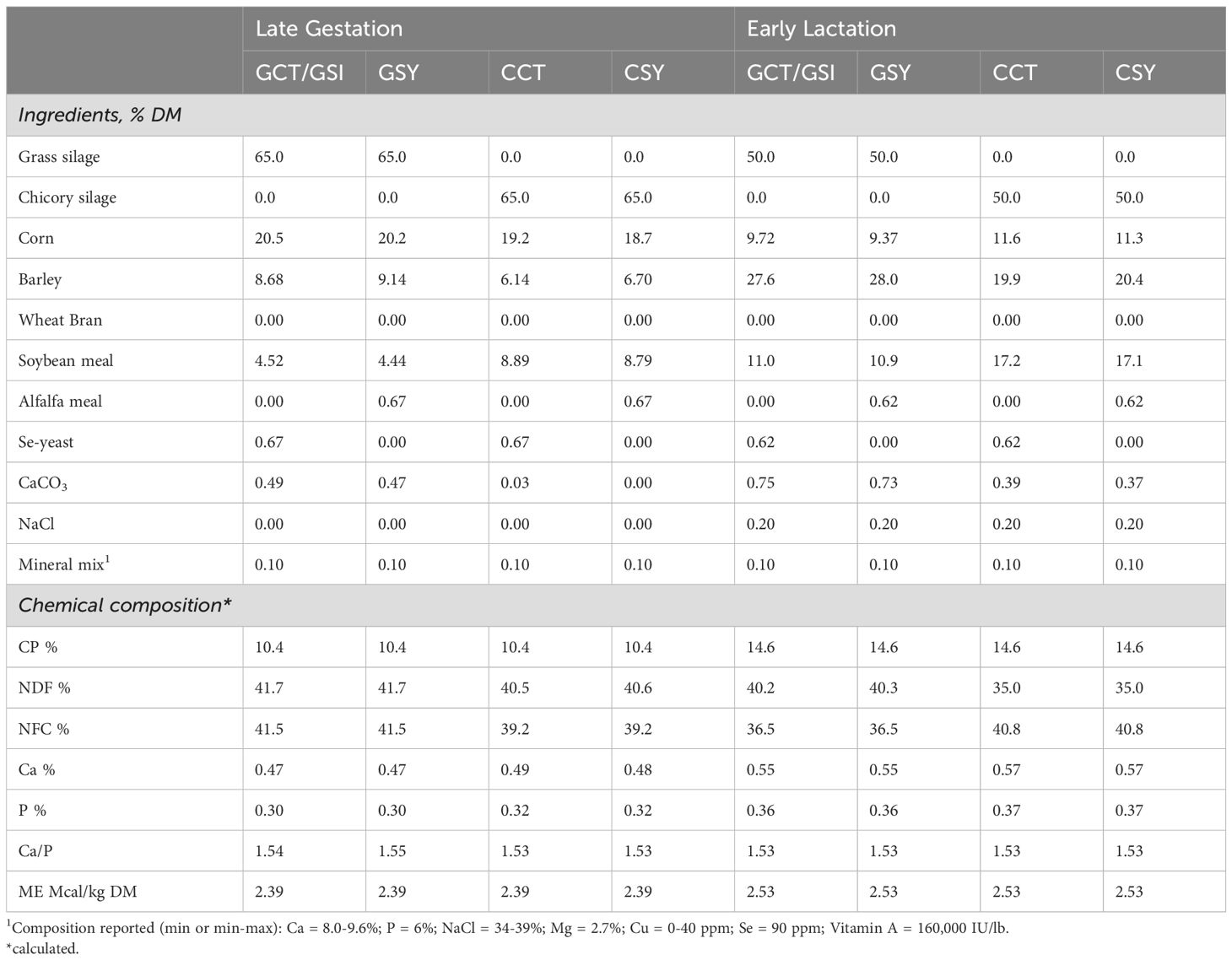
Table 1. Diet composition and chemical characteristics for each of the treatment groups during late gestation and early lactation.
The grass silage used in this experiment was purchased from a local commercial farm. Don Wirth, owner of the Saddle Butte Ag, Inc. Tangent Oregon, donated the chicory silage. Silages came in the form of large round bales. To minimize losses in forage quality and moisture content, only part of the silage bale was opened for each feeding and then covered with a tarp. Forage was provided ad libitum in a large trough and the leftover feed was collected each morning and weighed to determine daily dry matter intake (DMI).
Dry matter of the silages was measured daily using a microwave by weighing approx. 200 g of silage in a glass baking pan, placing the pan with the silage in microwave at maximum energy for 5 minutes, mixing the silage in the pan, and 5 additional minutes in the microwave at maximum energy and dried silage weighed. A container with approx. 100 mL of water was inserted into the microwave to avoid burning the silage. Grain and silages were fed twice daily in a different trough from the one used for the forages. Grain residuals were collected and weighed when present in the morning before feeding.
At approx. 9AM, 60% of the diet was provided and the rest was provided at approx. 5PM. The amount of feed was initially based on the individual body weight of each ewe during the prepartum. During the prepartum the amount of grain was kept proportional to the amount of silage provided, which was dependent on the intake of the prior day. During the postpartum we provided a fixed amount of grain at 1.5% BW of the animals while the amount of silage provided was approx. 110% of the silage consumed the prior day. Residual samples were collected over the course of 3 consecutive days during both the pre- and postpartum periods for analysis of composition and nutrient content. Diets were formulated to meet the recommendations for both pregnant and lactating sheep (National Research Council, 2007).
2.4 Body weight and body condition scoring
A Tru-Test digital scale for livestock was used to weigh the ewes in the morning before feeding but without fasting. The BW was recorded before starting the trial and twice during the trial. It was used to calculate the requirements and adjust diets accordingly. Body condition scoring (BCS) was assessed for all ewes when weighed. Two researchers graded ewes on the standard 5-point BCS scale for sheep. Final data were averaged using geometrical means to normalize the data and avoid large differences between the two researchers.
2.5 Blood collection and analysis
Blood was collected on days -40 (+/-11), -15 (+/-11), +5, +10, +20, and +30 DIM via jugular venipuncture using vacutainer tubes. Non-heparinized tubes (Becton Dickinson and Company, Cat# 366430) were used for the collection of serum, heparinized tubes (Becton Dickinson and Company, Cat# 366480) were used for the collection of blood plasma and measurement of hematocrit and EDTA tubes (Becton Dickinson and Company, Cat# 366643) were used to collect whole blood for micromineral analysis and complete blood counts. Following collection, blood was processed and analyzed for hematocrit (HMC) by centrifuging the blood tubes for 10 minutes at 1000×g and then measuring the percentage of packed red cell volume of the total volume directly in the tube and correct for the bottom curvature by multiplying for 0.8. Total white blood cells (cells/mL) were counted using a hemacytometer with a bright field microscope at 10x by diluting 50 µl of blood in 500 µl of Turk’s Solution before counting the cells.
Plasma and serum were stored in 1.7 mL centrifuge tubes with lids at -20°C. An aliquot of plasma was shipped to the Department of Animal Sciences, Food and Nutrition, Università Cattolica del Sacro Cuore, Piacenza, Italy for analysis of a wide range of parameters related to metabolism, liver and kidney function, oxidative stress, and inflammation and immunity as previously described (Michelotti et al., 2021) using a clinical auto-analyzer (ILAB 650, Instrumentation Laboratory, USA Spa, Werfen Co., Milan, Italy). Whole blood from EDTA tubes collected at day 10, 20, and 30 post-lambing was used fresh to measure complete blood count using a VetScan HM5 (Abaxis Zoetis, NJ, USA).
2.6 D2Dx assay
The D2Dx immunity test (Nano Discovery Inc., Orlando, FL 32803) is a new immune assay developed in order to detect changes in the humoral immune response based on changes in the serum in response to a foreign nanoparticle (Deb et al., 2021). The D2Dx was performed following the manufacturer’s protocol. Briefly, serum samples were allowed to thaw by resting them at room temperature for 3 hours. Once thawed, 10 µl of serum was mixed with 50 µl of D2Dx solution in a transparent plastic cuvette. This solution was mixed by vortexing and then assessed for the D2Dx score using a hand-held colorimeter device (CT-100, Nano Discovery Inc).
2.7 Whole blood micromineral analysis
Microminerals, including Se, were analyzed in whole blood collected with vacutainer tubes containing EDTA using an Inductively Coupled Plasma Mass Spectroscopy (ICP-MS) at the Keck Collaborative for Plasma Spectrometry (Oregon State University) as previously described (Jaaf et al., 2020) with however a larger number of elements measured (from 4 to 14 trace minerals), using the Multielement standard solution 4 for ICP to identify and quantify the various peaks (Cat# 51844, TraceCERT(R), Sigma-Aldrich, USA).
2.8 Carrageenan skin test
Carrageenan skin test was performed as previously described (Jahan et al., 2015). Briefly, carrageenan was made by dissolving 0.7g of carrageenan (Irish Moss, MP Biomedicals, LLC, Cat# 104886) in 100 ml of sterile saline solution by heating the solution at 90°C, making sure not to boil and then autoclaving it. The solution was then transferred to sterile bottles to be used for injection. Ewes were injected subcutaneously with 0.6 ml of the carrageenan reagent in the right shoulder ~30 days before parturition. Prior to injection, the skin on the shoulder was pinched and the thickness was measured using a digital skin caliper (GoolRC, SA). After the reagent was injected, the skin was measured again, and the area was marked with a permanent marker. The thickness of the skin was measured again three days after the initial injection (Supplementary Figure S3).
2.9 Milk composition and yield
Milk samples were collected directly from both teats by hand by stripping twice before collecting the milk during the first suckling period of the weigh-suckle-weigh procedure (described below) at 7, 14 and 21 DIM in 60 mL cupped dairy vials (cat# CPP03EDM-CL, Capitol Plastic Products, NY) containing 1 pill of Broad Spectrum Microtabs II™ (Weber Scientific, Cat# 3038-50) for assessment of milk components using a LactoScope™ FT-A and SomaScope Smart (both from Perkin Elmer, USA). The LactoScope was calibrated monthly using 14 cow calibration milk samples from Dr. David Barbano at Cornell University. The accuracy of the LactoScope in measuring sheep milk samples was determined by sending aliquot of 24 milk samples measured with LactoScope™ FT-A to Dr. Barbano’s laboratory for wet chemistry analysis for fat and protein data. The R2 was 0.968 for fat and 0.963 for protein (after removal of one outlier; with outlier the R2 was 0.836 for protein). The use of Dr. Barbano’s calibration samples allowed for the measurements and estimation of 19 components, including C16:0, C18:0 and C18:1.
Daily milk yield was estimated using the weigh-suckle-weigh method (Benson et al., 1999). Briefly, on 7, 14 and 21 DIM, lambs were separated from their mothers within the individual pens by plastic netting that allowed for close contact, but no suckling (see Supplementary Figure S4). Lambs were separated for a 3-hour period in the morning before being placed back with the ewe and allowed to suckle until finished (up to 15 min) to empty the mammary glands of most of the milk. Following this suckling period, lambs were separated from the ewe again for 3 hours, weighed, and allowed to suckle until finished before being weighed again. The difference in lamb weights before and after the final suckling period was used as an estimate of 3-hour milk production by the ewe and extrapolated over a 24-hour period to estimate daily milk yield. Lambs were weighed by using a standard lamb/calf sling and suspending them from a hanging scale (Berkley Digital Fish Scale - 50 LB).
2.10 Milk fatty acid profiling
Milk fatty acid was measured in milk samples collected at 7, 14, and 21 DIM using an Agilent 6890 GC (Agilent Technologies, Inc., Wilmington, DE, USA) present in Dr. Gita Cherian’s laboratory at Oregon State University. We used a previously described method (Oeffner et al., 2013) with modifications. Briefly, the behenic acid (C22:0; cat# 11909, Sigma-Aldrich, St. Louis, MO, USA) was used as the internal standard and 40 fatty acids were identified using a TraceCERT® 37 Component FAME Mix certified reference material (cat# CRM47885, Supelco, Bellefonte, PA, USA) plus reference standards for rumen biohydrogenation intermediates (methyl 9,11-conjugated linoleate and methyl-10,12 conjugated linoleate - cat# UC-59M - and methyl 11-trans-octadecenoic (transvaccenate) - cat# U-49M, Nu-Check, Elysian, MN, USA). The fatty acids were analyzed as proportion of annotated peaks (g/100 g fatty acids).
2.11 Fecal egg count
About 10 g of fecal samples were collected from the rectum at -30 and +30 DIM. Fecal samples were stored in a 50 mL conical tube at 4°C until analysis. Quantification of fecal strongyle-type egg counts comprised the modified McMaster egg count method (Whitlock, 1948). Two g of fecal pellets were added to 13 mL of saturated sugar solution to release the ova from the fecal material and prepare a homogenous solution of the feces. A disposable Pasteur pipette was used to transfer the fecal solution to the chambers of a McMaster slide and examined under 100× total magnification. The etched lines on the underside of the top chamber were examined and eggs detected in both chambers of the slide were counted. The total number of strongyle-type eggs was multiplied by 25 to obtain the total number of eggs/g of feces.
2.12 Statistical methods
The number of animals/group was based on a power analysis using ANOVA calculation (SAS v9.4) of the activity of several antioxidants from pregnant ewes supplemented with Se from data from a prior publication (Chauhan et al., 2015). The analysis indicated that 9 animals/group would provide 80% power to detect differences. Prior to statistical analysis, data were checked for outliers using the PROC REG procedure (SAS, v9.4), and data points with a studentized-t >3 were removed. For dry matter intake, milk yield, FCM, FPCM, and ECM, the number of lambs birthed by each ewe was used as a covariate, as the number of lambs/ewe can strongly affect those parameters. For all of the plasma parameters, blood micromineral levels, white blood cell count, hematocrit, and D2Dx, the baseline values (blood collected at -40 d; i.e., before starting the feeding experiment) were used as a covariate. Final datasets were analyzed using the GLIMMIX procedure in SAS (v9.4) with either a first-order autoregressive [AR(1)], or heterogeneous [ARH(1)] covariance structure depending on the better AIC. The analysis was performed by separating the datasets into two groups. The first set of comparisons was done between the GCT, GSY, CCT, and CSY groups with the type of silage (F), selenium supplementation (Se), and DIM (T) as the fixed effects and ewe as the random effect. The second statistical analysis was performed encompassing GCT, GSY, and GSI groups (all receiving grass silage) to determine the effect of the type of selenium (Adm, i.e., injection of inorganic Se or supplementation of Se-Yeast) and Adm×T. Ewe was considered a random effect in all analyses. For lamb average daily gain (ADG), the effect of time was not evaluated as the data reflected average lamb growth over the first 21 days after birth. For blood parameters with a baseline, a statistical analysis using all the time points (-40, -15, 5, 10, and 20 days relative to parturition) with time as the main effect and animals as random was performed using the PROC GLIMMIX with a heterogeneous [ARH(1)] covariance structure. Significance was declared at P≤0.05 and tendency when 0.05<P≤0.1. Over the course of the experiment, two animals had to be removed. One animal from the GSI group had complications regarding parturition and another animal from the GCT group contracted mastitis soon after lambing.
3 Results
3.1 Chicory silage vs. grass silage
The chicory silage was characterized by having large stalks that were only partially eaten by the animals (Supplementary Figure S5). The nutritive values of the chicory and the grass silages are available in Supplementary Table S1. The chicory silage had an overall relative feed value similar to the grass (RFV 96 vs. 102) with however lower CP (8.7 vs. 13%), soluble CP (32.1 vs 62.7% CP), rumen degradable protein (66 vs. 81%CP), and energy for lactation (1.06 vs. 1.36 Mcal/kg DM). The NDF was lower (51.7 vs. 55.8% DM) but its digestibility was lower (3.5 vs. 5.3%HR) partly due to a higher amount of ADF (46 vs. 36%). The level of the various macro- and micro- minerals was higher compared to grass silage with Be, Co, and Fe being >11-fold higher, except for Mn (102 vs. 164 ppm) and Ni (2.0 vs 3.2 ppm). The level of Se was 2-fold higher in chicory silage vs. grass silage (0.36 vs. 0.18 ppm).
3.2 Nutrient intake
Based on the amount and nutrient profile of the residual orts, ewes fed chicory silage consumed less CP, NDF and ME compared to ewes fed grass silage, with no effect of Se-yeast supplementation or the type of Se that was administered (Table 2).
3.3 Animal performance
The type of forage provided had a significant effect on the DMI, with the ewes that were fed chicory silage consuming less than those that were fed grass, with a consistent effect during both pre- and post-partum (Table 3). There was a strong increase in dry matter intake for all groups as the ewes transitioned from pregnancy to lactation (Figure 2) and DMI continued to increase among the grass silage-fed ewes through day 20 postpartum while the ewes receiving chicory reached the plateau at the end of the first week in lactation. The effect on the DMI was obviously driven by the amount of silage intake (Table 3).
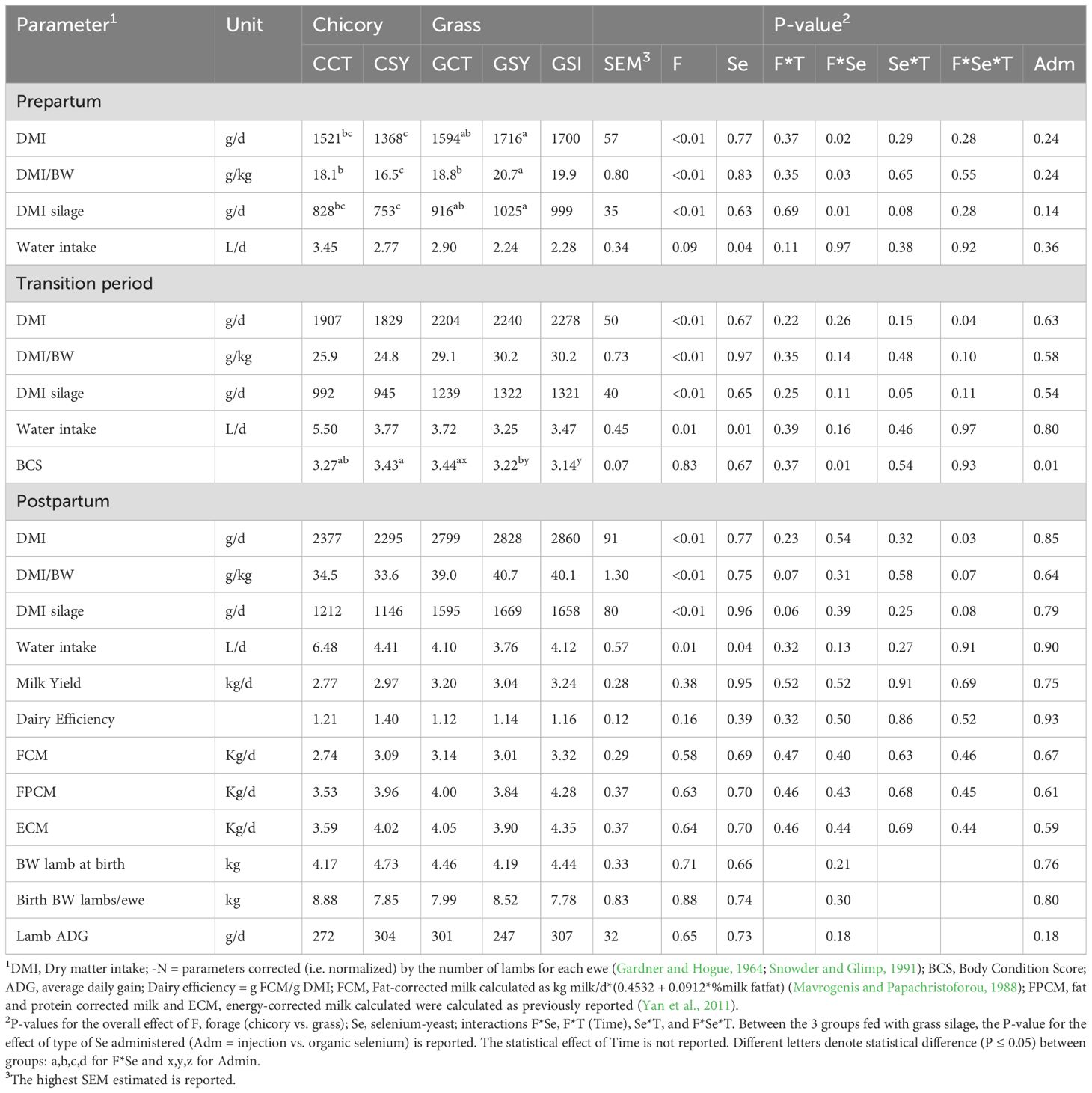
Table 3. Performance-related parameters of ewes fed chicory silage or grass silage and supplemented with Se-yeast (Y) or with single injection of inorganic Se (GSI) during the transition period (i.e., whole experiment).
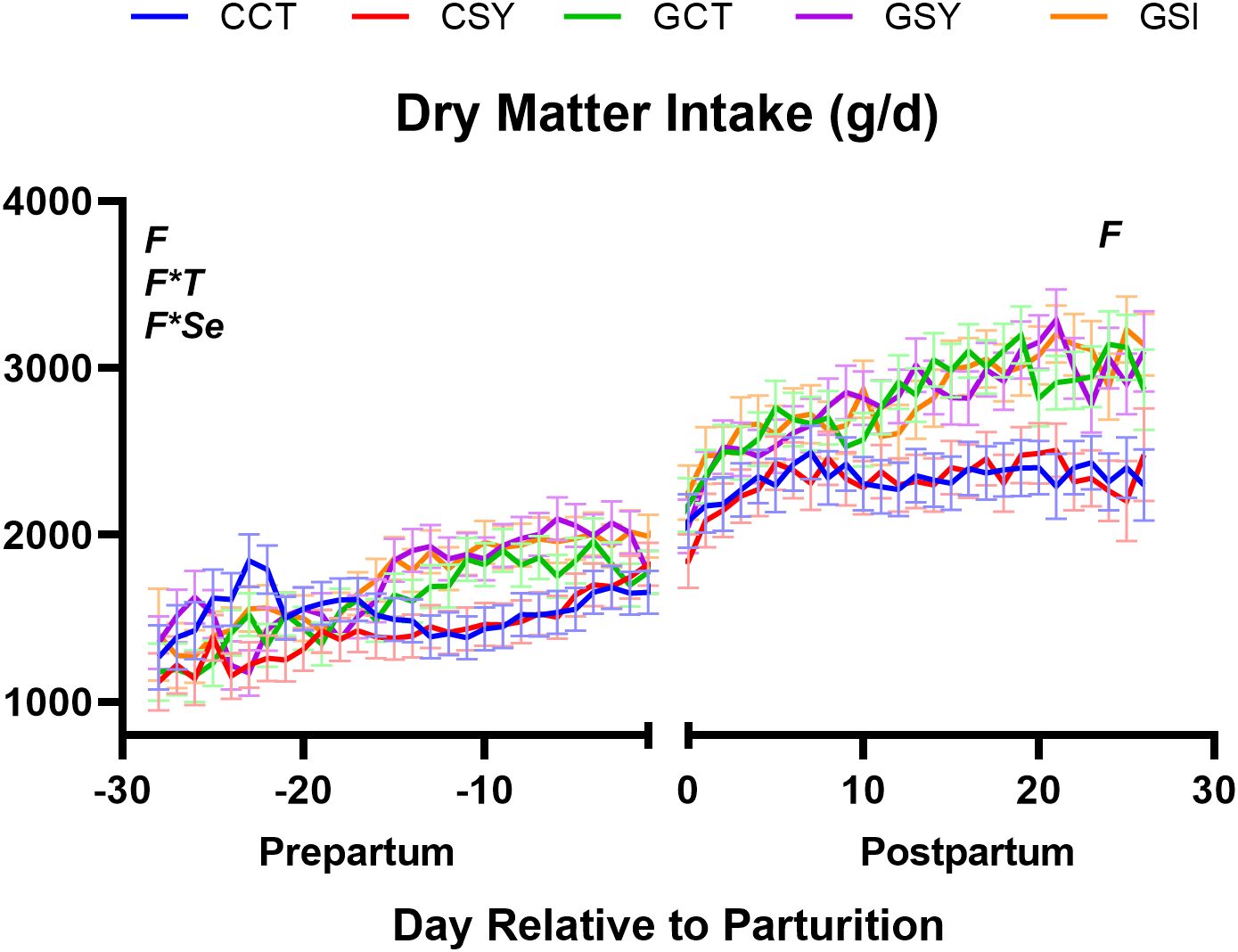
Figure 2. Dry matter intake of ewes during the peripartum period when fed with chicory silage only (CCT), chicory silage + Se-yeast (CSY), grass silage only (GCT), grass silage + Se yeast (GSY), or grass silage plus a single injection of inorganic Se prepartum (GSI). Significant effects of forage type (F), Se-yeast supplementation (Se), time (T) and their interactions are displayed on the graph when significant (P ≤ 0.05) and, when present, the P-value of the tendency is provided.
Water intakes increased significantly (P<0.01) from pregnancy to lactation and the ewes consuming chicory silage drank more water than the ewes fed grass silage (Table 3; Supplementary Figure S6). The ewes supplemented with Se-yeast drank less water than the ewes not supplemented with organic Se. An interaction for BCS was observed with Se-yeast supplementation increasing BCS in ewes fed chicory silage but decreasing BCS in the ewes fed grass silage (Table 3).
None of the treatments influenced overall milk yield or energy-corrected milk (Table 3).
None of the above parameters were affected by the injection of Se, except for the BCS, that was lower in GSI compared to ewes in GCT and GSY groups (Table 3).
3.4 Milk quality
Many of the milk components were affected by the type of forage fed (Table 4). Percentage of milk protein and other solids (mostly minerals) tended (P=0.10 and P=0.08, respectively) to be higher while milk concentration of SNF, NPN and MUN were lower in ewes fed grass vs. ewes fed chicory silage. Milk fat, including overall fat %, estimated stearate (C18:0) and oleate (18:1), and preformed fat, had higher concentrations in the milk of ewes fed chicory silage vs. ewes fed grass silage. Supplementation with Se-yeast had minor effects on milk components, with only a tendency for higher levels of de novo synthesized fat (P=0.09) and estimated C16:0 (P=0.10) in milk from ewes supplemented with Se-yeast vs. non-supplemented ewes.
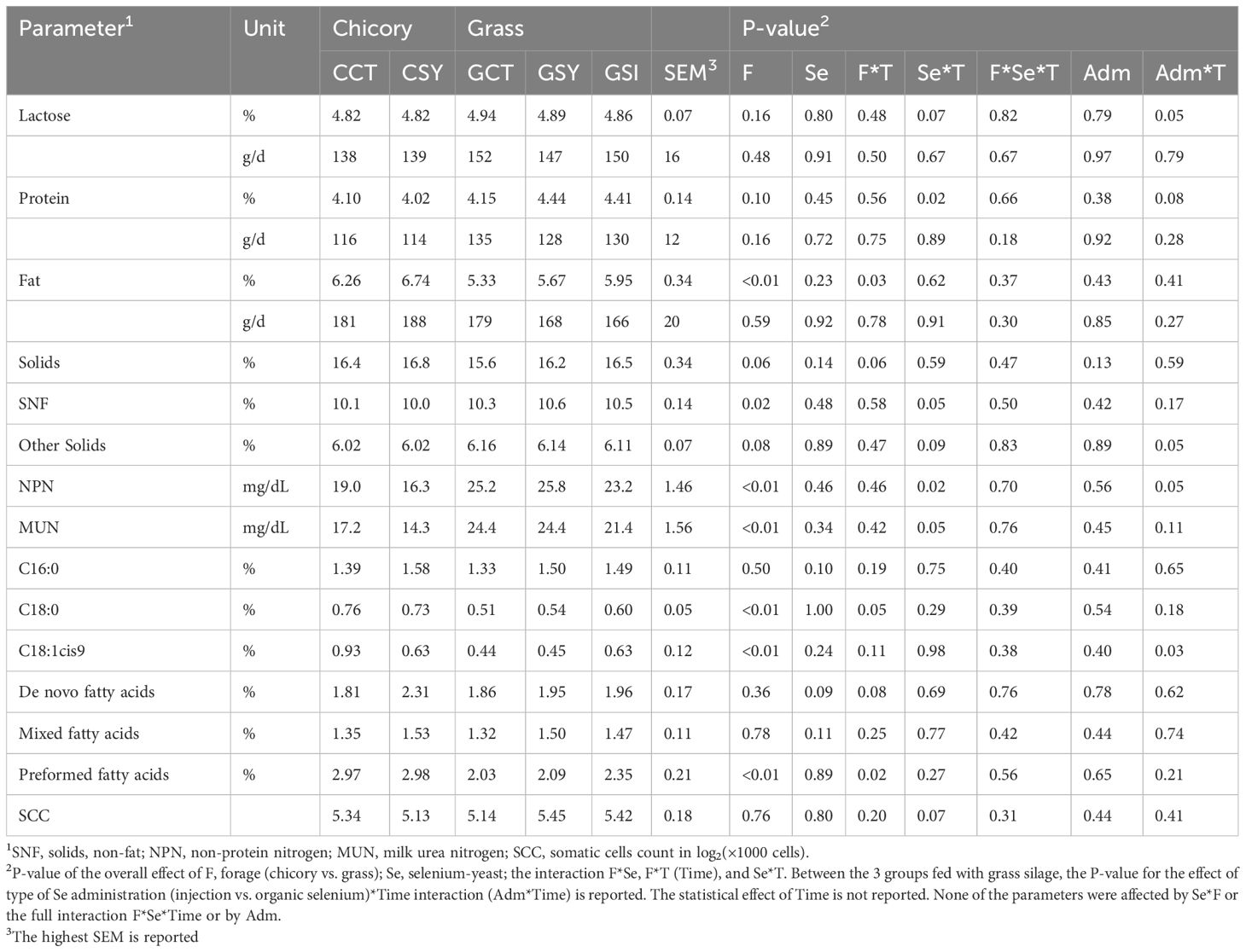
Table 4. Milk components measured with LactoScope FTA of ewes fed chicory silage or grass silage and supplemented with Se-yeast or with single injection of inorganic Se (GSI) during the first 21 days post-lambing.
Several milk components were affected by the interaction of Se-yeast supplementation, or the type of Se administered and time (Figure 3). Among the grass silage-fed ewes, milk lactose decreased over time in GSI whereas increased in the milk of GSY ewes. Animals supplemented with Se-yeast also had an increase in protein % and fat % in the milk during lactation compared to animals that were not supplemented. Ewes fed chicory silage had higher milk fat % and lower NPN and MUN than ewes fed grass silage.
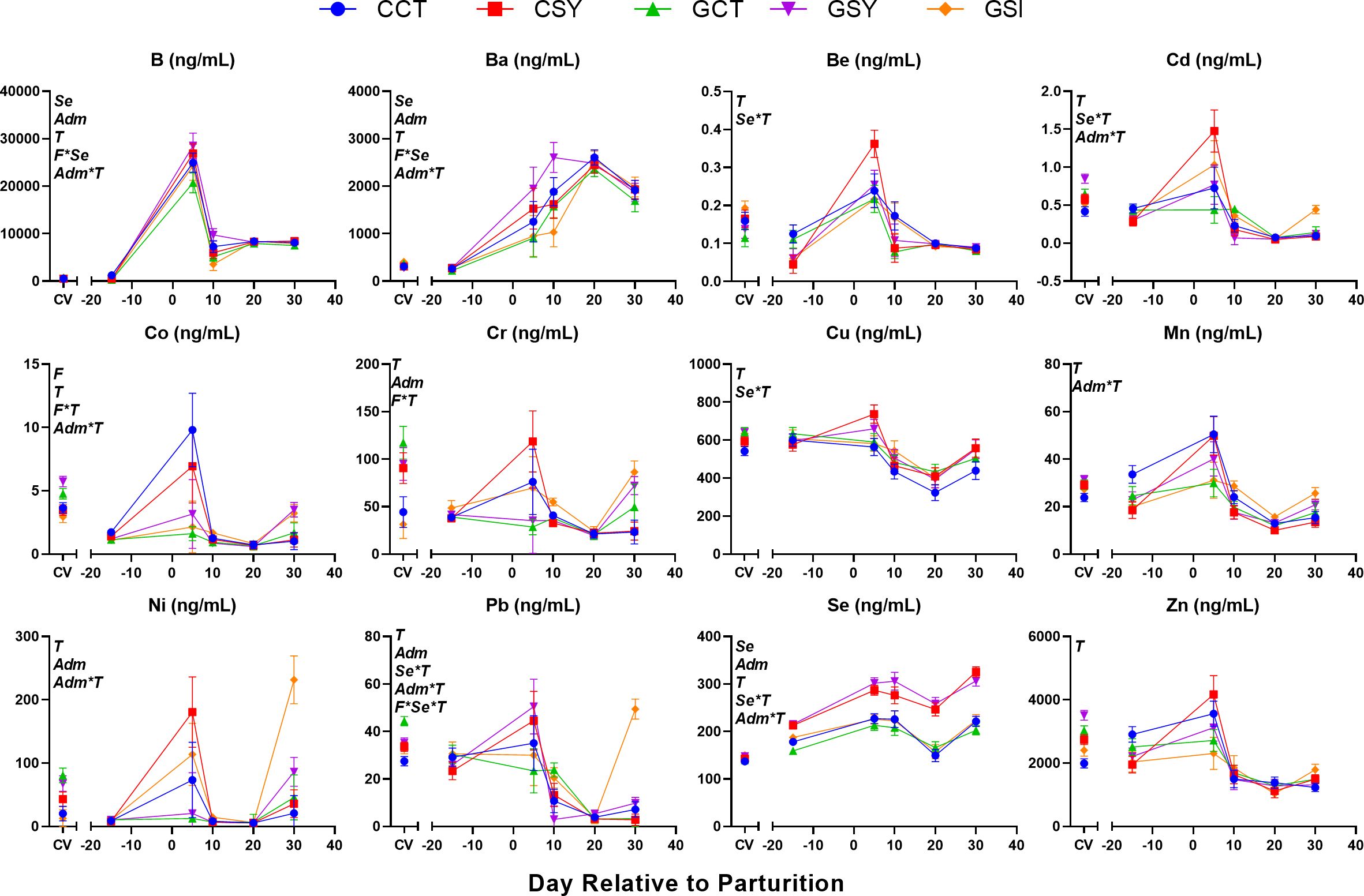
Figure 3. Milk parameters measured with LactoScope FTA in early post-partum ewes fed with chicory silage only (CCT), chicory silage + Se-yeast (CSY), grass silage only (GCT), grass silage + Se yeast (GSY), or grass silage plus a single injection of inorganic Se prepartum (GSI). When indicated the effect of Forage (F), Time (T), Se-yeast (Se), and administration of Se either as daily supplementation of Se-yeast or a single injection of inorganic Se prepartum (Adm) and their interactions were significant (P ≤ 0.05) and, when present, the P-value of the tendency is provided. Letters a,b,c,d denote significant (P ≤ 0.05) statistical difference between groups at each time point.
3.5 Milk fatty acid profile
A positive correlation was observed between the predicted fatty acids from the LactoScope FTA and the fatty acid profiling using GC, with r=0.46, r=0.55, and r=0.76 for C16:0, C18:0, and C18:1C9, respectively (all with P<.0001). Overall, the type of silage that was provided to the ewes as well as the supplementation of Se-yeast had a strong effect on the fatty acid profile of the milk (Table 5). The proportion in milk fat of short- to medium-chain fatty acids mostly produced de novo (from C6:0 to C14:1) was greater in milk of ewes fed grass vs. chicory silage. A higher proportion of preformed FA was observed in milk of sheep fed chicory vs. sheep fed grass. This was mostly due to a higher proportion in bacteria-derived FA (such as C17:0 and C17:1, C16:1), oleic acid, linoleic acid, and trans-11 FA, such as C18:1t11 and C18:2c9,t11. Overall, sheep fed with chicory silage had a higher proportion of unsaturated FA in milk, including monounsaturated (MUFA) and polyunsaturated (PUFA) fatty acids, as well as a higher proportion of omega 6 FA, and higher activity of the mammary delta 9 desaturase compared to sheep fed grass silage. Because of the above characteristics, milk fat from sheep fed chicory silage had a healthier profile for consumers compared to the milk fat of sheep fed grass silage, as indicated by the calculated atherogenic, thrombogenic, and cholesterolemic indexes (Table 5).
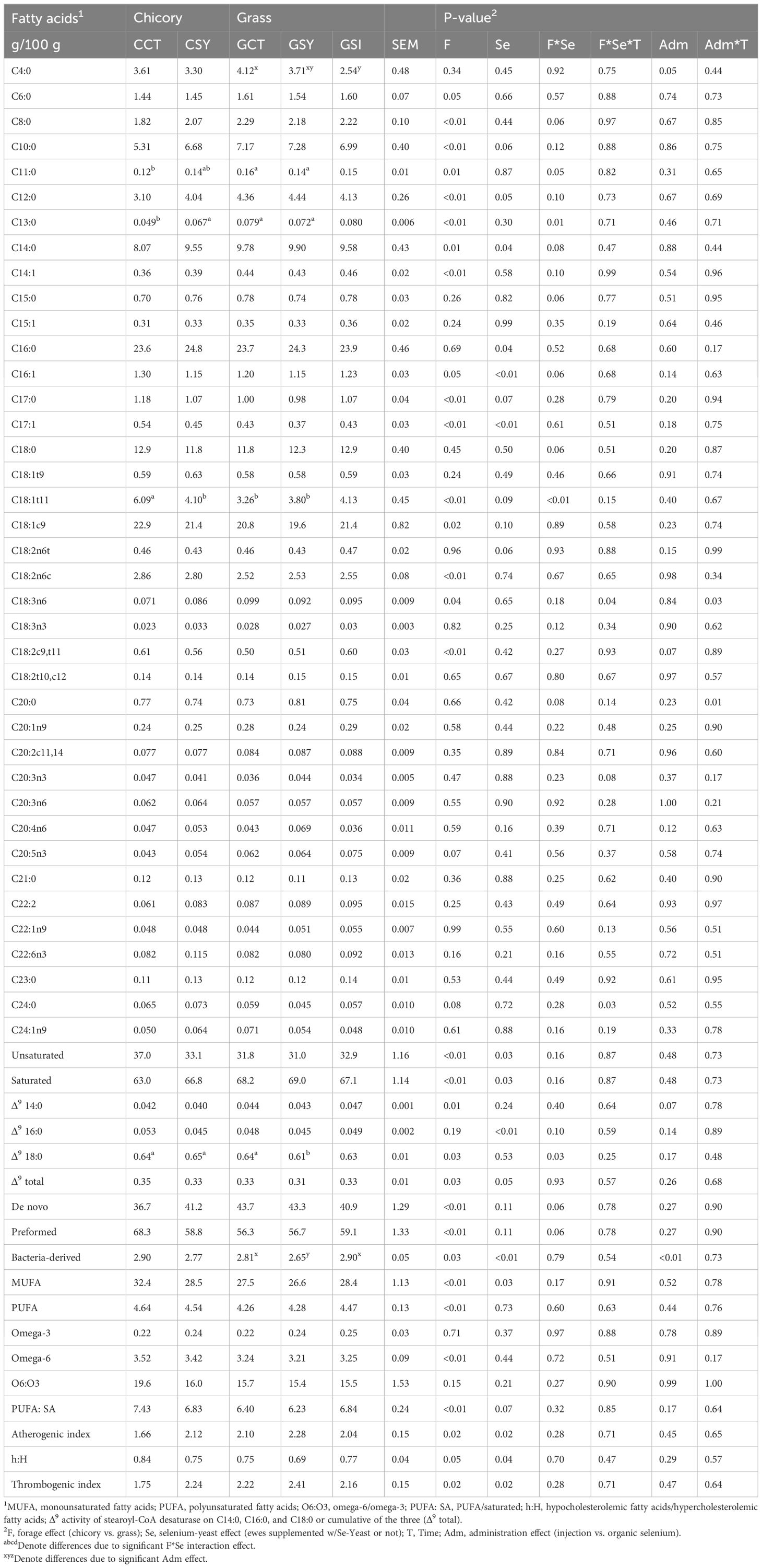
Table 5. Fatty acids measured with gas chromatography in milk of ewes fed chicory silage or grass silage and supplemented with Se-yeast (Y) or with single injection of inorganic Se (GSI) during the first 21 days post-lambing.
The single injection of inorganic Se or organic Se provided daily orally in sheep fed with grass silage did not affect the profile of FA in milk fat, except for a lower proportion of butyrate with the single Se injection and a lower proportion of bacteria-derived FA in oral organic Se, both compared to sheep not receiving Se (Table 5). No difference in the proportion of any FA was detected between the two types of supplemented Se.
3.6 Ewe immune health
The hematocrit was analyzed manually and simultaneously with the VetScan HM5. The correlation between the two techniques was r=0.76 (P<.0001). The manually measured hematocrit spanned the whole experiment, while the automatic hematocrit (i.e., VetScan HM5) analysis was performed only post-partum. Hematocrit increased from pregnancy to lactation (P<0.001, Supplementary Figure S7).
The type of silage had a minor impact on CBC (Table 6). During the post-partum, sheep fed chicory silage had higher hematocrit, mean corpuscular volume (MCV), and red blood cell distribution width coefficient of variation (RDVc) but lower mean corpuscular hemoglobin concentration (MCHC) compared to sheep fed grass silage. The number of monocytes and neutrophils was affected by an interaction between silage-fed and Se yeast, with a decrease of those parameters in sheep-fed chicory silage but an increase in sheep-fed grass silage by supplementing Se yeast. The single injection of inorganic Se increased white blood cells (WBC), especially neutrophils and monocytes, compared to sheep not receiving Se.
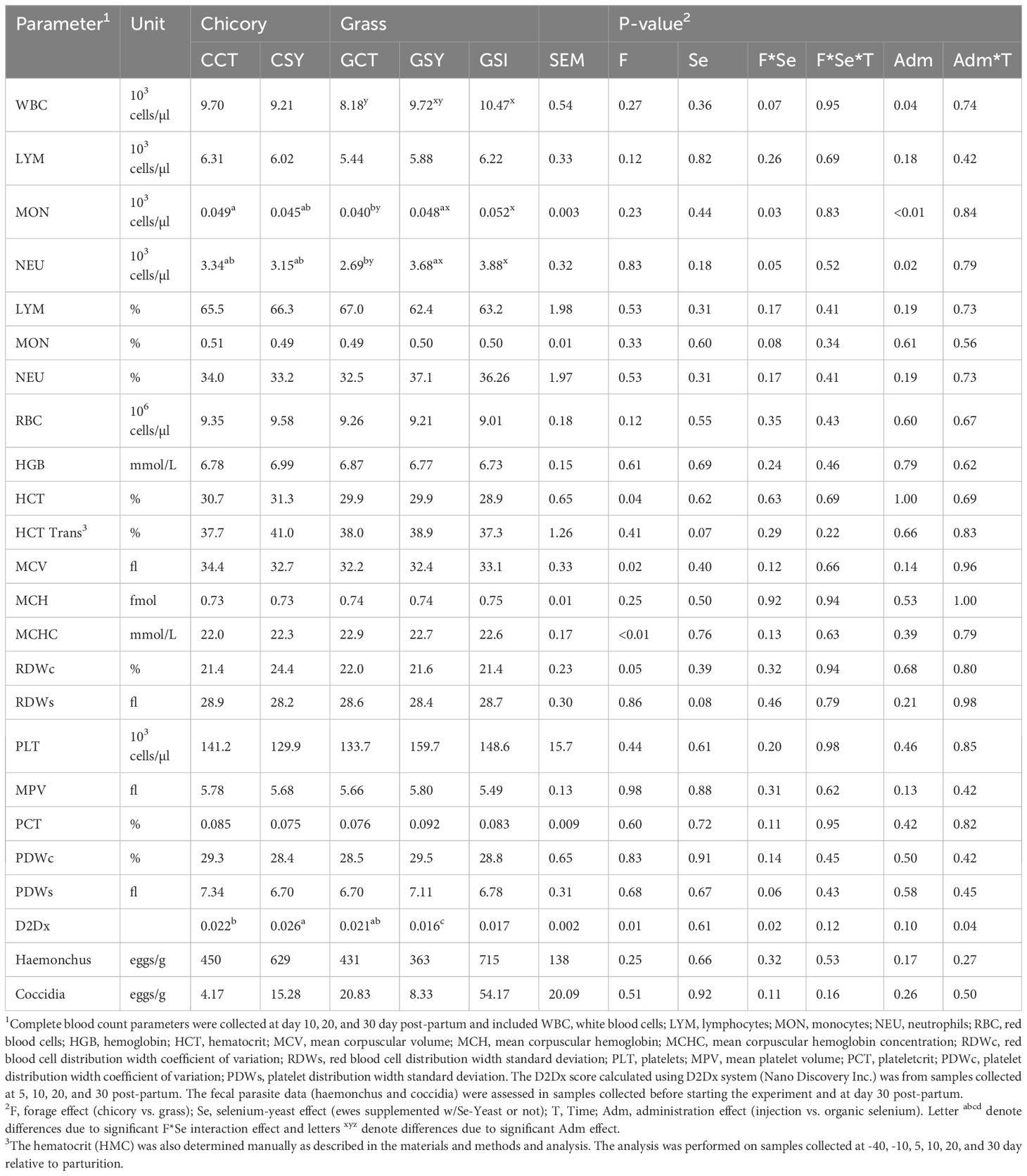
Table 6. Complete blood count and D2Dx score and parasites in feces in ewes fed chicory silage or grass silage and supplemented with Se-yeast (Y) or with single injection of inorganic Se (GSI).
The carrageenan skin test did not work as the animals did not react to the subcutaneous injection of the mixture of polysaccharides; thus, no data were generated. The D2Dx was significantly (P<0.001) affected by the stage of lactation with a nadir at 5 DIM (Supplementary Figure S7). The D2Dx score was overall higher in sheep-fed chicory silage vs. sheep-fed grass silage (Table 6). Supplement of Se yeast increased the D2Dx score in sheep fed chicory silage but decreased it in sheep fed grass silage, and this was irrespective of the type and modality of Se administered. Fecal egg count from the ewes was not affected by the type of forage nor by supplementation of Se.
3.7 Biomarkers of metabolism, inflammation, and oxidative stress: peripartum profiling
Patterns of blood parameters related to metabolism, immune-, and oxidative status during the peripartum period in sheep are scant in literature. Cabiddu and collaborators measured several of the blood parameters measured in our study using the same laboratory we used (Cabiddu et al., 2020); however, the samples were collected from Sarda dairy sheep, while in our experiment, we used non-dairy sheep (Polypay); thus, when available, we compared the data of multiparous sheep in that study with our data.
In our study the concentration in plasma of metabolic parameters measured and creatinine were all affected by time (Supplementary Figure S8). Concentration of glucose, BHBA and urea increased from pre- to post-lambing while NEFA, cholesterol, and creatinine decreased before lambing with a nadir at -15, 10, and 5 DIM, respectively. The pattern of the same parameters for the Sarda was somewhat different than Polypay, except for glucose and cholesterol, the latter being lower in Sarda (approx. 1.5 mM) compared to Polypay (approx. 2.5 mM). The pattern of the concentration of NEFA and urea was almost opposite between the two breeds, although for most of the parameters an increase in concentration happened before lambing rather than post-lambing as happened with the Sarda (Supplementary Figure S8).
Among macro minerals (Supplementary Figure S9) only Ca was affected by time in our study with an increase between -40 and -15 DIM. Parameters related to liver function (Supplementary Figure S10) were also affected by lambing. All proteins decreased from -40 to -15 DIM but recovered after lambing, especially albumin. The markers of liver health, GGT and GOT, both increased after lambing; the pattern during peripartum of those parameters was remarkably similar between Polypay and Sarda.
Among inflammatory-related parameters, in our study haptoglobin and myeloperoxidase peaked at 5 DIM while ceruloplasmin peaked and paraoxonase had a nadir at -15 DIM; the pattern of haptoglobin was similar in Sarda, but ceruloplasmin and paraoxonase was quite different (Supplementary Figure S11).
The NEFA:albumin ratio had a nadir at -15 DIM while the urea:creatinine ratio increased, reaching a peak at 5 DIM (Supplementary Figure S12). Among oxidative stress-related parameters ROM had a peak at -15 DIM while FRAP strongly decreased after lambing compared to before lambing with the ROM/FRAP having the highest values between -15 and 5 DIM; in Sarda, the patter for ROM and FRAP was quite different and almost opposite (Supplementary Figure S13).
3.8 Biomarkers of metabolism, inflammation, and oxidative stress: effect of treatments
Several plasma parameters were affected by both the type of forage provided as well as the presence and method by which Se was supplemented (Table 7).
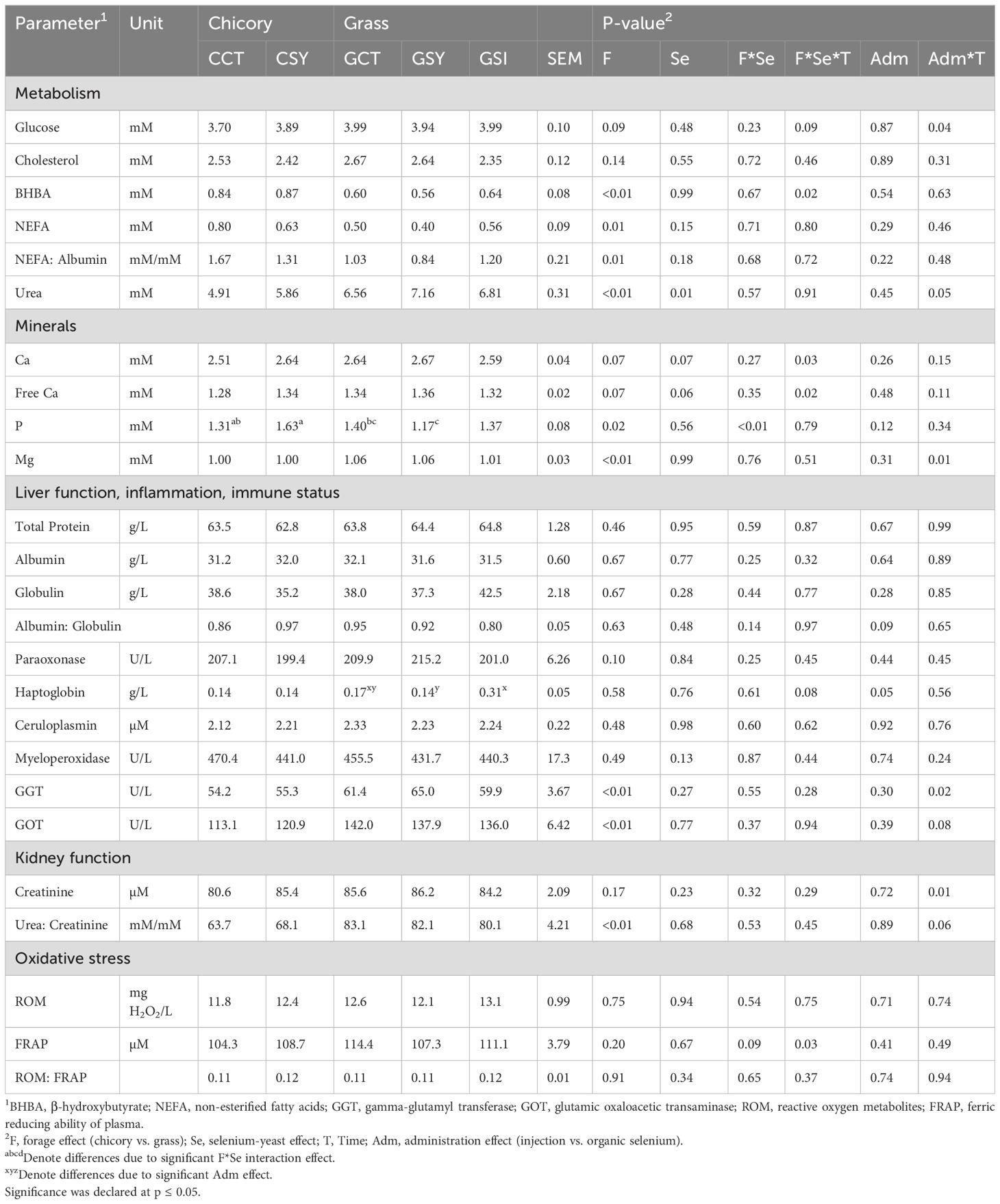
Table 7. Plasma parameters associated with metabolism, inflammation, and oxidative stress in peripartum ewes.
3.8.1 Metabolism
Feeding chicory increased the concentration in blood of BHBA, NEFA, NEFA/Albumin, and decreased the concentration of urea (Table 7). A full interaction F×Se×T was observed for BHBA due to a spike of BHBA before lambing in CSY group (Figure 4). A F×T effect was detected for urea due to a large spike at 5 DIM in animals receiving grass silage but not in animals fed chicory silage. Glucose and urea were affected by injection of Se, where the level of glucose in those animals rapidly decreased after 10 DIM while the level of urea increased more rapidly pre-lambing and decreased more rapidly post-lambing in GSI compared to GCT and GSY (Table 7; Figure 4).
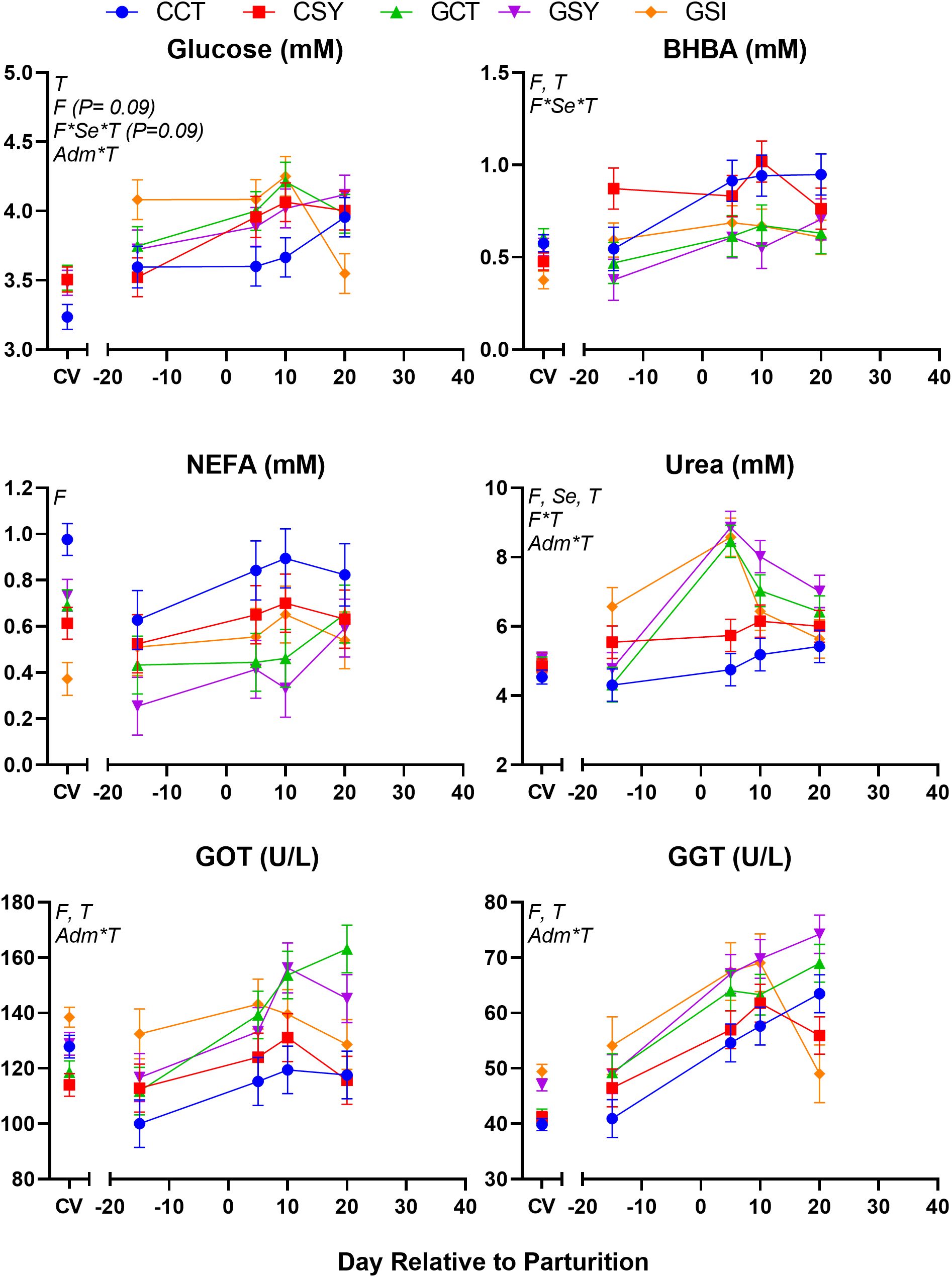
Figure 4. Plasma parameters related to metabolism (glucose, BHBA, NEFA, and urea) and liver status (GGT and GOT) in peripartum ewes fed with chicory silage only (CCT), chicory silage + Se-yeast (CSY), grass silage only (GCT), grass silage + Se yeast (GSY), or grass silage plus a single injection of inorganic Se prepartum (GSI). When indicated the effect of Forage (F), Time (T), Se-yeast (Se), and administration of Se either as daily supplementation of Se-yeast or a single injection of inorganic Se prepartum (Adm) and their interactions were significant (P ≤ 0.05) and, when present, the P-value of the tendency is provided.
3.8.2 Minerals
Concentrations of Ca and free Ca tended (P=0.07) to be lower in animals fed chicory vs. grass silage and tended (P=0.07) to be higher in animals supplemented with Se-yeast (Table 7). Blood levels of Ca and free Ca were significantly affected by the interaction F×Se×T, due to a different pattern of change during the peripartum of GCT and CSY compared to CCT and GSY (Table 7; Supplementary Figure S14). The concentration of P was higher while the concentration of Mg was lower in animals fed chicory silage compared to grass silage (Table 7). P was affected by a F×Se interaction due to an opposite effect of Se supplementation in animals fed the two silages; i.e., Se supplementation increased P in chicory and decreased in grass silage fed animals (Table 7). Mg was decreased by the injection of Se compared to Se yeast or control group (Table 7).
3.8.3 Liver function
Most of the parameters related to those functions were not affected by the treatments (Table 7). Both liver enzymes GGT and GOT were overall lower in sheep fed chicory silage vs. sheep fed grass silage (Table 7). Both parameters were affected by a Adm×T where the GSI group had a sharp decrease in concentration of GGT between 10 and 20 DIM, that was not observed in the other groups while the GOT had larger values pre-lambing with a rapid decrease afterwards, that was different than GCT and GSY (Figure 4). Haptoglobin was higher in GSI compared to GCT and GSY (Table 7).
3.8.4 Kidney function
Concentration of plasma creatinine was not affected by forages or supplementation of Se yeast but was lower in GSI vs. GCT and GSY (Table 7). The difference in urea concentration indicated above likely contributed to the difference observed in the urea:creatinine ratio, where the ewes fed grass silage had a higher ratio than those fed chicory silage (Table 7).
3.8.5 Oxidative stress
Treatments did not affect overall parameters related to oxidative stress but a full interaction F×Se×T was observed for FRAP (Table 7; Supplementary Figure S14). A F×T effect was observed for ROM: FRAP, due to lower values in early post-partum in animals fed chicory silage vs. animal fed grass silage.
3.9 Micromineral status
All the 14 microminerals measured in whole blood of sheep were affected by parturition (Table 8; Figure 5; Supplementary Figure S15). An acute increase in whole blood concentration of all microminerals was detected at 5 DIM except for Ba, Cu, Fe, and Se. The level of Cd, Cu, Mn, Pb, and Zn was lower post- partum relative to pre-partum. Supplementation of Se-yeast increased the level of Se in whole blood by >40% after 70 days of supplementation (i.e., 5 DIM) with >20% increase already after approx. 25 day of supplementation (i.e., -15 DIM).
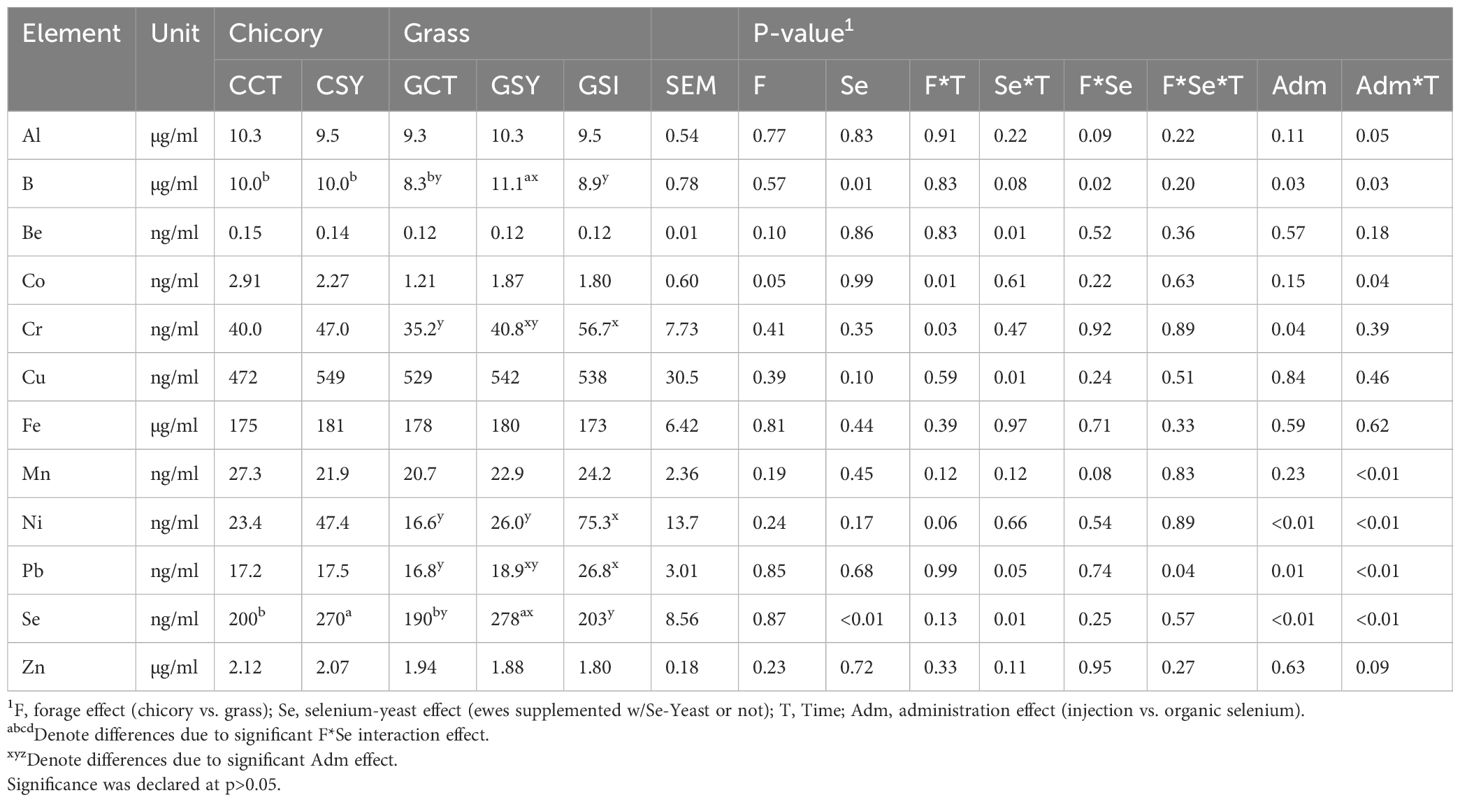
Table 8. Micromineral concentrations in whole blood from ewes fed chicory silage (C) or grass silage (G) and supplemented with Se-yeast (SY) or with single injection of inorganic Se (GSI) during the transition period.
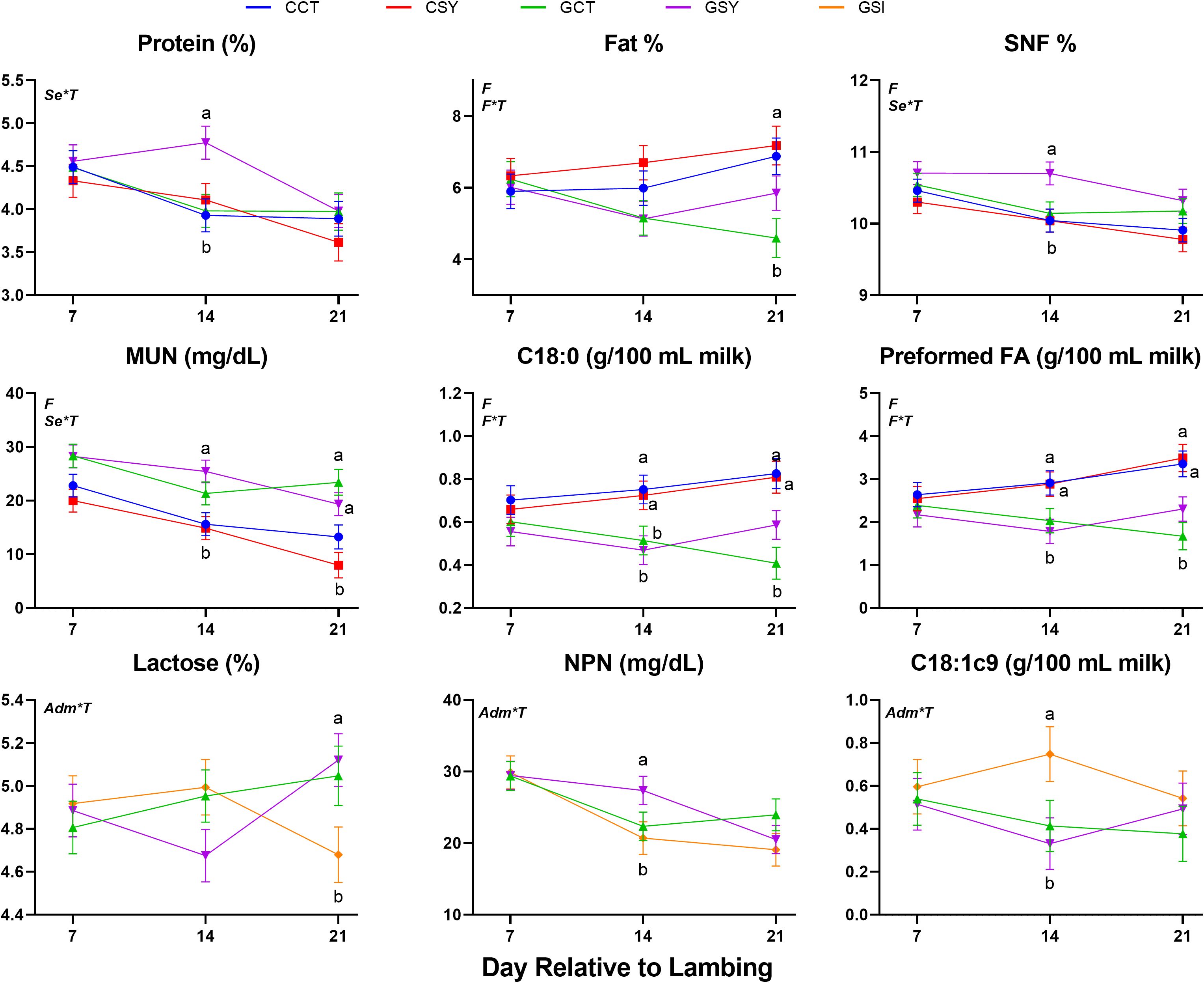
Figure 5. Level of microminerals in whole blood in peripartum ewes fed with chicory silage only (CCT), chicory silage + Se-yeast (CSY), grass silage only (GCT), grass silage + Se yeast (GSY), or grass silage plus a single injection of inorganic Se prepartum (GSI). When indicated the effect of Forage (F), Time (T), Se-yeast (Se), and administration of Se either as daily supplementation of Se-yeast or a single injection of inorganic Se prepartum (Adm) and their interactions were significant (P ≤ 0.05) and, when present, the P-value of the tendency is provided.
All microminerals measured were present at higher concentration in chicory vs. grass silage with Se being two-fold higher (0.36 vs. 0.18 ppm, respectively); an exception was Mn that was lower in chicory compared to grass silage (Supplementary Table S1). Despite this, only Co was affected by the forage and a F×T interaction with higher values in whole blood of ewe fed chicory- vs. grass silage at 5 DIM (Table 8; Figure 5). Few microminerals were affected by the supplementation of Se or by Se×T interaction. Those included B, Ba, Be, Cu, and Pb. Supplementing Se increased the whole blood concentration of those microminerals, especially at 5 DIM (Figure 5; Supplementary Figure S15).
Single injection of inorganic Se did not significantly increase the level of Se compared to control ewes (Table 8; Figure 5). The single injection of inorganic Se affected most of the other microminerals, except for Al, Be, Cu, Fe, and Zn (Table 8; Figure 5; Supplementary Figure S15). For B, Ba, Co, and Mn the single injection of Se lowered their concentration early post-partum while for Cd, Cr, Ni, and Pb the injection of Se increased their concentration at 30 DIM (Figure 5).
4 Discussion
4.1 Pattern of blood parameters in peripartum ewes
Surprisingly, the daily milk yield was higher in the Polypay compared to the Sarda (Cabiddu et al., 2020) (3.1 vs 1.75 kg, respectively). The pattern of glucose, BHBA, creatinine, GGT, haptoglobin, paraoxonase, albumin, and globulin was remarkably similar between the two studies; however, the pattern of cholesterol, NEFA, urea, ceruloplasmin, ROM, and FRAP was different and, for some parameters, such as cholesterol, NEFA, and ceruloplasmin, was the opposite. This can be due to a different adaptation to lactation of the two breeds. Interestingly, despite the opposite pattern during the peripartum of ROM and ceruloplasmin between the two study, there was in both an extremely high association between the two parameters; for instance, in our study the correlation was extremely high (r=0.91; P<.0001). The pattern of ceruloplasmin and ROM was also highly correlated with the level of Cu (r=0.55; P<.0001 and r=0.51; P<.0001, respectively). The association of ceruloplasmin and ROM is somewhat new, although it has been previously argued that ceruloplasmin can affect the measurement of ROM but was lately proved to be a minor, if not a nil, effect (Colombini et al., 2016). It is unclear what could be the physiological relationship between the two parameters in sheep.
4.2 Chicory silage is effective at meeting the demands of lactation despite lower dry matter intake
When comparing the performance of the ewes fed either grass or chicory silage, it was evident that even though ewes fed chicory had a lower DMI, they produced the same amount of milk, and the lambs had the same ADG as the ewes fed grass silage but improved ADG when corrected by DMI. The reduced DMI of ewes fed chicory silage in this study was in part caused by the quality of the forage itself. The chicory silage contained a large quantity of large stems that were unpalatable to the ewes and a relatively low nutritive quality which was lower than grazed chicory in a prior study (Wilson et al., 2020). This may also help explain some of the differences between previous studies where chicory was used as a forage and the results presented in this study. For example, in a previous study on dairy cows that utilized a chicory+ryegrass silage mix, the intake of cows tended to be greater than that of cows fed pure ryegrass silage; however, the milk production of the chicory+ryegrass-fed cows tended to be lower (Kälber et al., 2013). While studies evaluating chicory silage as a forage source are limited, many studies have utilized chicory as pasture and found comparable results. In both sheep (Rodríguez et al., 2019) and cows (Muir et al., 2015; Wilson et al., 2020), animals grazing chicory have similar if not greater DMI than animals grazed on traditional grass-based pastures and produced similar or higher amounts of milk.
4.3 Chicory silage increases the amount and improves the health quality of milk fat
The most significant effects of feeding chicory silage to milk quality in our experiment was on milk fat and the fatty acid profile of the milk. The elevated levels of milk fat, as well as the higher % of preformed fats in the milk of ewes fed chicory vs. grass silage is driven by the higher levels of circulating NEFA in these animals. Prior studies have found that postpartum NEFA levels are positively associated with milk fat % (Mäntysaari et al., 2019).
The elevated circulating NEFA has likely also affected the level of MUFA in milk (Mäntysaari et al., 2019). While the milk of ewes-fed chicory had higher levels of unsaturated and lower levels of saturated fats than ewes-fed grass silage, the chicory silage itself had the opposite fatty acid profile. The larger amount of MUFA in the milk of ewes fed chicory silage- vs. grass silage is likely the product of a higher delta 9 desaturase activity in the mammary gland toward the C16:0 and C18:0, the most abundant fatty acids in NEFA, at the least in dairy cows (Contreras et al., 2010). The delta 9 desaturase activity is the highest during the early lactation in the mammary tissue of dairy cows (Bionaz and Loor, 2008), similar to what we observed in ewes in our experiment. The higher delta 9 activity in chicory silage- vs. grass silage-fed ewes could be due to a combination of higher availability of C18:0 from the diet and higher expression of SCD1 gene in the mammary gland (Bionaz and Loor, 2008).
In a previous investigation where chicory silage was fed to lactating dairy cattle, chicory silage had higher levels of saturated fatty acids and lower levels of PUFA than a simple ryegrass silage but found no differences in saturated fatty acid levels in the milk and only numerically higher levels of PUFA among cows fed chicory silage (Kälber et al., 2013). Milk from dairy cows grazed on chicory-rich pasture had no difference in unsaturated fatty acids compared to cows grazed on rye-grass-white clover pasture (Wilson et al., 2020). Thus, the effect in fatty acid profiling of milk fat observed in our experiment appears specific for ewes. The reasons for the observed higher PUFA in milk despite the lower level of PUFA in chicory silage vs. grass silage remain unclear.
Two additional interesting observations from the fatty acid profiling in our experiment are the larger amount of bacteria-derived fatty acids in the milk of ewes fed chicory silage, pointing toward an effect of the chicory on the rumen microbiota and the lower levels of NPN as well as MUN in the milk of ewes fed chicory silage. While diets were balanced to be isonitrogenous, the protein availability in each diet could have influenced the latter outcome. Compared to chicory, grass silage had a higher proportion of rumen-degradable crude protein. Prior work has demonstrated that increased levels of rumen degradable protein can increase MUN and NPN (Roseler et al., 1993). It is unclear if the lower MUN in milk and plasma in ewes fed chicory vs. grass silage in our experiment was the result of a better N utilization in the rumen or an increased kidney excretion, as suggested by the larger water intake; however, contrary to this was the level of circulating creatinine that was not different between the animals. However, it is problematic to use serum creatinine by itself as an index of glomerular filtration (Levey et al., 1988). In our prior experiment, cows grazing a chicory-rich forb pasture had lower levels of MUN than cows grazing a more traditional grass-clover pasture despite similar levels of total dietary crude protein, and this was partly due to a larger urine production (Wilson et al., 2020)We cannot exclude the role of plant secondary compounds potentially present in chicory in the rumen microbiota, which could also affect nitrogen metabolism (Kamra and Singh, 2019).
4.4 Feeding chicory silage leads to elevated NEFA and BHBA levels and may improve liver health and oxidative status
The increase in NEFA and BHBA in the blood of ewes fed chicory is likely driven by their lower feed intake and resulting negative energy balance as they transition from pregnancy to lactation (Drackley, 1999). The elevated levels of those parameters in chicory silage-fed animals did not affect the liver health, as GGT and GOT were lower than the grass silage-fed ewes. While these enzymes are typically utilized as indicators of liver functionality and health (Russell and Roussel, 2007), there was no indication that the ewes that were fed grass silage were dealing with any type of liver damage. Given the involvement of GGT and GOT in the metabolism of amino acids (Meister, 1974; Sookoian and Pirola, 2012), the reduced level of these enzymes in the plasma of ewes fed chicory silage may be associated with the lower crude protein intake of these ewes. It is unclear the reason for such an effect, but it could indicate a more functional liver. Interestingly, in monogastric species, the level of GGT in the blood is associated with a lower antioxidant capacity and an increased risk of diseases (Koenig and Seneff, 2015).
In a previous investigation that helped to inform and establish the current study, it was found that dairy cattle grazing a chicory-rich pasture had higher FRAP than cows grazing a traditional grass-clover pasture (Ford et al., 2021). While the key difference between these studies was the use of fresh chicory vs. silage chicory, ensilement does not seem to reduce the antioxidant capacity of the forage (Łozicki et al., 2015). Despite not having an effect on FRAP or ROM, the ratio ROM: FRAP was better (i.e., lower) early post-partum in animals fed chicory silage vs. animals fed grass silage, indicating a likely better ability of those animals to deal with oxidative stress (Mezzetti et al., 2019).
4.5 Chicory silage had no effect on fecal parasites and CBC but improved the type 1 immune response
In the present study, chicory silage was found to have a minimal effect on a wide range of health and immune parameters, including parasites, which were measured, somewhat in contrast to previous studies. Multiple studies have noted chicory for its anthelmintic effects on livestock (Peña-Espinoza et al., 2018); however, we did not find any beneficial effect of chicory silage on fecal egg counts of haemonchus or coccidia in this investigation. While the antiparasitic activity of chicory has been attributed to its high levels of sesquiterpene lactones (Foster et al., 2011). No studies have assessed how the concentration of these compounds can change during entitlement, and the specific compounds were not measured as part of the current study. Unfortunately, we did not assess the level of sesquiterpene lactones in our samples; however, it could be possible that the ensilement and/or the variety of chicory used has reduced levels of secondary compounds.
Regarding the complete blood count, this is the only known study evaluating the effect of chicory silage on immune cell populations and characteristics throughout the transition period in sheep. There were very few differences between ewes fed chicory silage and those fed grass silage, mostly parameters related to red blood cells, specifically their size and the amount of hemoglobin they contain, which the data indicate are both larger among the ewes fed chicory silage. To our knowledge, there are no other studies evaluating the effect of feeding fresh chicory or chicory silage on these parameters in sheep or other ruminants. Despite the differences between treatment groups, these parameters still fall within the normal ranges for sheep (Jones and Allison, 2007) and do not indicate any abnormality. Although a power analysis was run to assess the minimum number of animals to detect differences, this was run using antioxidant parameters and it could be that other parameters would require a larger number of animals to detect differences.
The D2Dx score was overall lower in sheep compared to what has been detected in cows and the pattern from pregnancy to lactation was somewhat the opposite, with a decrease of the type-1 immune response in ewe approaching parturition while increased in cattle (Tsai et al., 2021). The reason for such a difference is unclear. The most important finding pertaining to the effect of chicory silage on immune function was the higher D2Dx scores among ewes fed chicory silage compared to the ewes fed grass silage. The increased D2Dx scores indicate that the immune systems of the ewes fed chicory silage had a stronger type-1 cell mediated response than the ewes fed grass silage. While use of the D2Dx assay in ruminants is limited so far, previous research in humans infected with COVID-19 showed that the D2Dx score was a reliable indicator of symptom severity in infected patients (Deb et al., 2021). The reason for this improved type-1 response among ewes fed chicory may be related to the immunomodulatory activity of chicory that has been previously investigated. As shown by Karimi et al. (2014), chicory extract can modulate the activity of dendritic cells leading to T-cell proliferation and alter cytokine expression toward a type-1 Th1 cell-mediated response.
4.6 Supplementation of Se-yeast had a minimal-to-no effect on transition ewes
Results from previous studies that utilize Se-yeast are varied, with some studies finding that Se-yeast supplementation is associated with decreases in somatic cell counts (Malbe et al., 1995; Reczyńska et al., 2019), while other studies have found no effect (Heard et al., 2004; Weiss and Hogan, 2005)Our original hypothesis was that the combination of Se-yeast and chicory would improve the immune system, including reducing SCC in milk. Our data do not support this hypothesis.
The only substantial effect of supplementing Se-yeast in our experiment was on the fatty acid profile of the milk with ewes that were supplemented with Se-yeast having lower levels of unsaturated and higher levels of saturated fats in the milk. This is the opposite of what was observed in previous studies in dairy goats (Mitsiopoulou et al., 2021) and dairy cattle (Ianni et al., 2019). While our results do not align with previous findings, other studies have found that organic Se supplementation can influence rumen microbial activity (Harrison et al., 1988), particularly by influencing rates of PUFA metabolized by bacteria, leading to increased biohydrogenation and saturated fatty acid production as has been observed in sheep (Kišidayová et al., 2014). Given the variation in experimental results, further investigations are needed to better understand the role of Se-yeast in rumen fatty acid metabolism.
Se-yeast supplementation did not affect white blood cells or other complete blood count parameters, aligning with previous investigations in lambs (Alimohamady et al., 2013) or transition dairy cows (Khalili et al., 2019). Based on these studies, the positive effects of Se supplementation on immune function are likely a result of improved antioxidant status via improved activity of glutathione peroxidase and other selenoproteins. We did not measure the activity of glutathione peroxidase; however, we did not find any benefits of Se-yeast supplementation on plasma antioxidant parameters like ROM and FRAP in the current study. Another possible mechanism by which Se supplementation may improve immune function is by altering patterns of gene expression in immune cells, particularly neutrophils, as was found in a previous study evaluating the immune performance of sheep affected by foot rot (Hugejiletu et al., 2013). In our study, we did not evaluate gene expression or activity of neutrophils.
The lack of observable effects of feeding Se yeast on the measured parameters could be partly explained by the relatively low number of animals used. However, our power analysis would have indicated that 9 animals/group would have been sufficient to detect differences, at least on several antioxidant parameters. The dose used was the maximal dose used in a prior study that was effective in improving Se, which we confirmed, and effective in improving the performance and health of ewes and their lambs (Stewart et al., 2012).
4.7 Blood micromineral levels change dramatically during the peripartum period
To our knowledge, this study is the first to describe blood micromineral profiles throughout the transition period in sheep, although some microminerals were measured in peripartum goats (Samimi et al., 2021). We speculate that the spike in concentration of most microminerals in blood after parturition could be associated with an increased leaking gut that is typical of this phase of lactation (Goff, 2018). For instance, chromium is commonly used to assess leaking gut (Cangiano et al., 2022); thus, the spike in concentration of those microminerals might be a consequence of an increased intestinal permeation of minerals in the diet into the circulatory system. The leaky gut has also been suggested to be a consequence of a change in the diet, and a major cause of the inflammatory-like conditions observed post-partum; however, we did not observe any relationship between the concentration of trace minerals and haptoglobin, except for a weak relationship with levels of B (r=0.16; P=0.04). The short-term dramatic spike in levels of microminerals may also be a consequence of their mobilization from tissues to colostrum to support the growth and health of the offspring, although there have been no previous studies evaluating this phenomenon in dairy animals.
Previous work has shown that chicory outperforms a traditional grass-clover pasture mix in terms of plant micromineral content, with fresh chicory having significantly higher levels of Zn, B, Cu, Mn, and Fe (Kidane et al., 2010). Our data regarding the micromineral composition of the silages used in this study support this previous finding, except Mn. Interestingly, despite higher concentrations in the chicory silage vs. Grass silage (e.g., for Fe the difference was quite large, in the order of >10-fold), no differences were found in the level of microminerals in the whole blood of the ewes. This might be partly due to the amount of chicory ingested.
Supplementation of Se-yeast was an effective method for boosting blood Se levels in transition sheep. This finding aligns with a previous investigation in sheep where ewes that were supplemented with organic Se-yeast had improved blood Se levels compared to ewes that were not supplemented or those that were supplemented with inorganic Se (Hall et al., 2012). Additional studies evaluating the effect of Se supplementation on other micromineral levels are lacking; however, a previous investigation that fed Se-biofortified hay to dairy cows found no effect on other micromineral levels (Jaaf et al., 2020). The sheep in our experiment were not Se deficient, as they were above the marginal band previously proposed of 40 ng/mL in whole blood (Suttle, 2010) and had similar basal values as sheep not deprived of Se in a prior study (Hall et al., 2012).
4.8 A single injection of inorganic Se during the prepartum has a minimal effect on ewe health and performance, with no effect on blood Se levels but effects on other microminerals
While a single injection of inorganic Se is a widespread practice for improving Se status in sheep, we did not observe any increase in the concentration of Se in blood following the injection of inorganic Se in ewes during the peripartum period. This is somewhat in contrast to prior findings in cows; where injection of inorganic Se led to a significant but still temporary increase in blood Se levels in the first two weeks following the injection (Chorfi et al., 2011) as also observed elsewhere (Ferreira and Petzer, 2019). Our findings indicate that injection of inorganic Se is not effective in improving the level of Se long-term and especially during the peripartum period in sheep.
Despite the lack of effect on the level of Se as measured in whole blood, ewes in the GSI group had a tendency for lower BCS than ewes in either the GSY or GCT groups despite a similar amount of feed consumed. This reduction in BCS is not the result of increased fat mobilization, as both NEFA and BHBA were not different in this group compared to the other grass silage-fed groups. Thus, the lower BCS remains unexplained.
In comparison to ewes in the GSY or GCT groups, GSI ewes had a tendency for higher blood levels of WBC and neutrophils, pointing toward a positive effect on immune function. Similar results were found in a previous study in sheep where a late-prepartum injection of sodium selenite was found to increase blood levels of total white blood cells (Soliman et al., 2012); however, no effect on blood levels of neutrophils was found in that study.
In contrast to what was observed among chicory silage-fed ewes, supplementation of Se, either as organic Se-yeast or via injection of inorganic sodium selenite, reduced D2Dx scores among the grass silage-fed ewes pointing toward a change in the immune response. Despite higher blood levels of WBC, the data indicates that GSI and GSY ewes had a reduced cell-mediated type-1 immune response in comparison to the GCT ewes. The reason for Se supplementation, regardless of Se form, having a different effect on D2Dx scores in grass silage-fed ewes compared to chicory silage-fed ewes remains to be determined.
The injection of inorganic Se affected the level in whole blood of 9 out of 14 microminerals. Although interactions exist among microminerals absorption (Goff, 2018) However, the effect was observed between 10 and 30 DIM, i.e., 30 and 40 days post-injection, respectively. It is unlikely that the effect was due to an acute level of Se, and thus, we do not have an explanation for the observed effect.
5 Conclusion
To summarize, chicory and supplementation with Se-yeast had a minimal effect on performance and health of ewes during the peripartum period. While Se-yeast was effective at boosting blood Se levels, no benefits were observed in ewe hematological profiles or antioxidant status. Chicory silage was effective at meeting the demands of lactating ewes, despite the reduced dry matter intake that contributed to the higher blood levels of NEFA and BHBA and changed the milk fatty acid profile. Despite this effect, the ewes fed chicory had a healthier milk fat for human consumption. Future studies attempting to evaluate the health and productive benefits of chicory silage should ensure high quality forage is available as the silage used in this experiment limited intake and complicated the evaluation of the results.
Data availability statement
The raw data supporting the conclusions of this article will be made available by the authors, without undue reservation.
Ethics statement
The animal study was approved by Institutional Animal Care and Use Committees (IACUC) of Oregon State University (protocol# 2020-0122). The study was conducted in accordance with the local legislation and institutional requirements.
Author contributions
HF: Data curation, Formal analysis, Investigation, Methodology, Validation, Visualization, Writing – original draft, Writing – review & editing. DH: Formal analysis, Investigation, Methodology, Writing – review & editing, Validation. SA: Investigation, Writing – review & editing, Conceptualization, Funding acquisition, Project administration, Supervision. GP-H: Investigation, Writing – review & editing, Methodology. JK: Investigation, Writing – review & editing, Resources. ET: Formal analysis, Investigation, Methodology, Resources, Writing – review & editing. MS: Resources, Writing – review & editing. MM: Formal analysis, Investigation, Writing – review & editing. MB: Conceptualization, Data curation, Formal analysis, Funding acquisition, Investigation, Methodology, Project administration, Resources, Supervision, Visualization, Writing – original draft, Writing – review & editing.
Funding
The author(s) declare financial support was received for the research, authorship, and/or publication of this article. The project was funded by the Agriculture Research Foundation at Oregon State University.
Acknowledgments
The authors thank Benjamin Grismer, who did a phenomenal job as the bioresearch technician for the study, and undergraduate students Corinna T. Cauchy and Alyssa Thibodeau for helping to feed the ewes and collecting samples. The authors also thank the following undergraduate students for helping to run the experiment: Mariangel Aleman Torres, Caitlynn Dougherty, Samantha Heney, Magdalena Ibarra, Sarah Kemp, Haley Kirschten, Kate Ness, Shelby Park, Carolyn Pearce, Tyler Quinones, Calan Stowell, Krystal Steiner, Paige Tafoya, and Julia VanDerZanden.
Conflict of interest
The authors declare that the research was conducted in the absence of any commercial or financial relationships that could be construed as a potential conflict of interest.
Publisher’s note
All claims expressed in this article are solely those of the authors and do not necessarily represent those of their affiliated organizations, or those of the publisher, the editors and the reviewers. Any product that may be evaluated in this article, or claim that may be made by its manufacturer, is not guaranteed or endorsed by the publisher.
Supplementary material
The Supplementary Material for this article can be found online at: https://www.frontiersin.org/articles/10.3389/fanim.2024.1499480/full#supplementary-material
References
Abuelo A., Hernández J., Benedito J. L., Castillo C. (2015). The importance of the oxidative status of dairy cattle in the periparturient period: revisiting antioxidant supplementation. J. Anim. Physiol. Anim. Nutr. 99, 1003–1016. doi: 10.1111/jpn.12273
Alimohamady R., Aliarabi H., Bahari A., Dezfoulian A. H. (2013). Influence of different amounts and sources of selenium supplementation on performance, some blood parameters, and nutrient digestibility in lambs. Biol. Trace Elem. Res. 154, 45–54. doi: 10.1007/s12011-013-9698-4
Benson M. E., Henry M. J., Cardellino R. A. (1999). Comparison of weigh-suckle-weigh and machine milking for measuring ewe milk production2. J. Anim. Sci. 77, 2330–2335. doi: 10.2527/1999.7792330x
Bernabucci U., Ronchi B., Lacetera N., Nardone A. (2005). Influence of body condition score on relationships between metabolic status and oxidative stress in periparturient dairy cows*. J. Dairy Sci. 88, 2017–2026. doi: 10.3168/jds.S0022-0302(05)72878-2
Bertoni G., Trevisi E., Han X., Bionaz M. (2008). Effects of inflammatory conditions on liver activity in puerperium period and consequences for performance in dairy cows. J. Dairy Sci. 91, 3300–3310. doi: 10.3168/jds.2008-0995
Bionaz M., Loor J. J. (2008). Gene networks driving bovine milk fat synthesis during the lactation cycle. BMC Genomics 9, 366. doi: 10.1186/1471-2164-9-366
Bionaz M., Trevisi E., Calamari L., Librandi F., Ferrari A., Bertoni G. (2007). Plasma paraoxonase, health, inflammatory conditions, and liver function in transition dairy cows. J. Dairy Sci. 90, 1740–1750. doi: 10.3168/jds.2006-445
Cabiddu A., Dattena M., Decandia M., Molle G., Lopreiato V., Minuti A., et al. (2020). The effect of parity number on the metabolism, inflammation, and oxidative status of dairy sheep during the transition period. J. Dairy Sci. 103, 8564–8575. doi: 10.3168/jds.2019-18114
Cangiano L. R., Villot C., Renaud J., Ipharraguerre I. R., McNeil B., DeVries T. J., et al. (2022). Induction of leaky gut by repeated intramuscular injections of indomethacin to preweaning Holstein calves. J. Dairy Sci. 105, 7125–7139. doi: 10.3168/jds.2021-21768
Chauhan S. S., Celi P., Leury B. J., Dunshea F. R. (2015). High dietary selenium and vitamin E supplementation ameliorates the impacts of heat load on oxidative status and acid-base balance in sheep. J. Anim. Sci. 93, 3342–3354. doi: 10.2527/jas.2014-8731
Cheng L., Mccormick J., Hussein A. N., Logan C., Pacheco D., Hodge M. C., et al. (2017). Live weight gain, urinary nitrogen excretion and urination behaviour of dairy heifers grazing pasture, chicory and plantain. J. Agric. Sci. 155, 669–678. doi: 10.1017/S0021859616001076
Chorfi Y., Girard V., Fournier A., Couture Y. (2011). Effect of subcutaneous selenium injection and supplementary selenium source on blood selenium and glutathione peroxidase in feedlot heifers. Can. Vet. J. 52, 1089–1094.
Colombini F., Carratelli M., Alberti A. (2016). Oxidative stress, d-ROMs test, and ceruloplasmin. Free Radic. Res. 50, 447–453. doi: 10.3109/10715762.2015.1136063
Contreras G. A., O’Boyle N. J., Herdt T. H., Sordillo L. M. (2010). Lipomobilization in periparturient dairy cows influences the composition of plasma nonesterified fatty acids and leukocyte phospholipid fatty acids. J. Dairy Sci. 93, 2508–2516. doi: 10.3168/jds.2009-2876
Deb C., Salinas A. N., Zheng T., Middleton A., Kern K., Penoyer D., et al. (2021). A 1-minute blood test detects decreased immune function and increased clinical risk in COVID-19 patients. Sci. Rep. 11, 23491. doi: 10.1038/s41598-021-02863-2
Drackley J. K. (1999). Biology of dairy cows during the transition period: the final frontier? J. Dairy Sci. 82, 2259–2273. doi: 10.3168/jds.S0022-0302(99)75474-3
Ferreira G. M., Petzer I.-M. (2019). Injectable organic and inorganic selenium in dairy cows - Effects on milk, blood and somatic cell count levels. Onderstepoort J. Vet. Res. 86, e1–e8. doi: 10.4102/ojvr.v86i1.1664
Ford H. R., Busato S., Trevisi E., Muchiri R. N., van Breemen R. B., Bionaz M., et al. (2021). Effects of pasture type on metabolism, liver and kidney function, antioxidant status, and plant secondary compounds in plasma of grazing, Jersey dairy cattle during mid-lactation. Front. Anim. Sci. 2. doi: 10.3389/fanim.2021.729423
Foster J. G., Cassida K. A., Turner K. E. (2011). In vitro analysis of the anthelmintic activity of forage chicory (Cichorium intybus L.) sesquiterpene lactones against a predominantly Haemonchus contortus egg population. Vet. Parasitol. 180, 298–306. doi: 10.1016/j.vetpar.2011.03.013
Gardner R. W., Hogue D. E. (1964). Effects of energy intake and number of lambs suckled on milk yield, milk composition and energetic efficiency of lactating ewes. J. Anim. Sci. 23, 935–942. doi: 10.2527/jas1964.234935x
Goff J. P. (2018). Invited review: Mineral absorption mechanisms, mineral interactions that affect acid–base and antioxidant status, and diet considerations to improve mineral status. J. Dairy Sci. 101, 2763–2813. doi: 10.3168/jds.2017-13112
Hall J. A., Harwell A. M., Van Saun R. J., Vorachek W. R., Stewart W. C., Galbraith M. L., et al. (2011). Agronomic biofortification with selenium: Effects on whole blood selenium and humoral immunity in beef cattle. Anim. Feed Sci. Technol. 164, 184–190. doi: 10.1016/j.anifeedsci.2011.01.009
Hall J. A., Van Saun R. J., Bobe G., Stewart W. C., Vorachek W. R., Mosher W. D., et al. (2012). Organic and inorganic selenium: I. Oral bioavailability in ewes. J. Anim. Sci. 90, 568–576. doi: 10.2527/jas.2011-4075
Harrison G. A., Hemken R. W., Dawson K. A., Harmon R. J., Barker K. B. (1988). Influence of addition of yeast culture supplement to diets of lactating cows on ruminal fermentation and microbial populations 1. J. Dairy Sci. 71, 2967–2975. doi: 10.3168/jds.S0022-0302(88)79894-X
Heard J. W., Walker G. P., Royle P. J., McIntosh G. H., Doyle P. T. (2004). Effects of short-term supplementation with selenised yeast on milk production and composition of lactating cows. Aust. J. Dairy Technol. 59, 199–203.
Hemingway R. G. (2003). The influences of dietary intakes and supplementation with selenium and vitamin E on reproduction diseases and reproductive efficiency in cattle and sheep. Vet. Res. Commun. 27, 159–174. doi: 10.1023/A:1022871406335
Hugejiletu H., Bobe G., Vorachek W. R., Gorman M. E., Mosher W. D., Pirelli G. J., et al. (2013). Selenium supplementation alters gene expression profiles associated with innate immunity in whole-blood neutrophils of sheep. Biol. Trace Elem. Res. 154, 28–44. doi: 10.1007/s12011-013-9716-6
Ianni A., Martino C., Pomilio F., Di Luca A., Martino G. (2019). Dietary selenium intake in lactating dairy cows modifies fatty acid composition and volatile profile of milk and 30-day-ripened caciotta cheese. Eur. Food Res. Technol. 245, 2113–2121. doi: 10.1007/s00217-019-03322-z
Jaaf S., Batty B., Krueger A., Estill C. T., Bionaz M. (2020). Selenium biofortified alfalfa hay fed in low quantities improves selenium status and glutathione peroxidase activity in transition dairy cows and their calves. J. Dairy Res. 87, 184–190. doi: 10.1017/S002202992000028X
Jahan N., Minuti A., Trevisi E. (2015). Assessment of immune response in periparturient dairy cows using ex vivo whole blood stimulation assay with lipopolysaccharides and carrageenan skin test. Vet. Immunol. Immunopathol. 165, 119–126. doi: 10.1016/j.vetimm.2015.04.003
Jones M. L., Allison R. W. (2007). Evaluation of the ruminant complete blood cell count. Vet. Clin. North Am. Food Anim. Pract. Ruminant Diagn. Med. 23, 377–402. doi: 10.1016/j.cvfa.2007.07.002
Kälber T., Kreuzer M., Leiber F. (2013). Effect of feeding buckwheat and chicory silages on fatty acid profile and cheese-making properties of milk from dairy cows. J. Dairy Res. 80, 81–88. doi: 10.1017/S0022029912000647
Kamra D. N., Singh B. (2019). “Rumen microbiome and plant secondary metabolites (PSM): inhibition of methanogenesis and improving nutrient utilization,” in Advancing frontiers in Mycology & Mycotechnology. Eds. Satyanarayana T., Deshmukh S., Deshpande M. (Springer, Singapore). doi: 10.1007/978-981-13-9349-5_13
Karimi M. H., Ebrahimnezhad S., Namayandeh M., Amirghofran Z. (2014). The effects of cichorium intybus extract on the maturation and activity of dendritic cells. DARU J. Pharm. Sci. 22, 28. doi: 10.1186/2008-2231-22-28
Khalili M., Chamani M., Amanlou H., Nikkhah A., Sadeghi A. A., Dehkordi F. K., et al. (2019). The effect of feeding inorganic and organic selenium sources on the hematological blood parameters, reproduction and health of dairy cows in the transition period. Acta Sci. Anim. Sci. 42. doi: 10.4025/actascianimsci.v42i1.45371
Kidane A., Houdijk J. G. M., Athanasiadou S., Tolkamp B. J., Kyriazakis I. (2010). Effects of maternal protein nutrition and subsequent grazing on chicory (Cichorium intybus) on parasitism and performance of lambs1. J. Anim. Sci. 88, 1513–1521. doi: 10.2527/jas.2009-2530
Kišidayová S., Mihaliková K., Siroka P., Čobanová K., Váradyová Z. (2014). Effects of inorganic and organic selenium on the fatty acid composition of rumen contents of sheep and the rumen bacteria and ciliated protozoa. Anim. Feed Sci. Technol. 193, 51–57. doi: 10.1016/j.anifeedsci.2014.04.008
Koenig G., Seneff S. (2015). Gamma-glutamyltransferase: A predictive biomarker of cellular antioxidant inadequacy and disease risk. Dis. Markers 2015, e818570. doi: 10.1155/2015/818570
Levey A. S., Perrone R. D., Madias N. E. (1988). Serum creatinine and renal function. Annu. Rev. Med. 39, 465–490. doi: 10.1146/annurev.me.39.020188.002341
Łozicki A., Koziorzębska A., Halik G., Dymnicka M., Arkuszewska E., Niemiec T., et al. (2015). Effect of ensiling pumpkin (Cucurbita maxima D.) with dried sugar beet pulp on the content of bioactive compounds in silage and its antioxidant potential. Anim. Feed Sci. Technol. 206, 108–113. doi: 10.1016/j.anifeedsci.2015.05.012
Malbe M., Klaassen M., Fang W., Myllys V., Vikerpuur M., Nyholm K., et al. (1995). Comparisons of selenite and selenium yeast feed supplements on se-incorporation, mastitis and leucocyte function in se-deficient dairy cows. J. Vet. Med. Ser. A 42, 111–121. doi: 10.1111/j.1439-0442.1995.tb00362.x
Mäntysaari P., Mäntysaari E. A., Kokkonen T., Mehtiö T., Kajava S., Grelet C., et al. (2019). Body and milk traits as indicators of dairy cow energy status in early lactation. J. Dairy Sci. 102, 7904–7916. doi: 10.3168/jds.2018-15792
Mavrogenis A. P., Papachristoforou C. (1988). Estimation of the energy value of milk and prediction of fat-corrected milk yield in sheep and goats. Small Rumin. Res. 1, 229–236. doi: 10.1016/0921-4488(88)90051-X
Mehdi Y., Dufrasne I. (2016). Selenium in cattle: A review. Molecules 21, 545. doi: 10.3390/molecules21040545
Meister A. (1974). The gamma-glutamyl cycle. Diseases associated with specific enzyme deficiencies. Ann. Intern. Med. 81, 247–253. doi: 10.7326/0003-4819-81-2-247
Mezzetti M., Bionaz M., Trevisi E. (2020). Interaction between inflammation and metabolism in periparturient dairy cows. J. Anim. Sci. 98, S155–S174. doi: 10.1093/jas/skaa134
Mezzetti M., Minuti A., Piccioli-Cappelli F., Gabai G., Trevisi E. (2019). Administration of an Immune Stimulant during the Transition Period Improved Lipid Metabolism and Rumination without Affecting Inflammatory Status. Animals 9, E619. doi: 10.3390/ani9090619
Michelotti T. C., Trevisi E., Osorio J. S. (2021). An exploration of the effects of an early postpartum intravenous infusion with carnosic acid on physiological responses of transition dairy cows. Antioxidants 10, 1478. doi: 10.3390/antiox10091478
Mitsiopoulou C., Karaiskou C., Simoni M., Righi F., Pappas A. C., Sotirakoglou K., et al. (2021). Influence of dietary sesame meal, vitamin E and selenium supplementation on milk production, composition, and fatty acid profile in dairy goats. Livest. Sci. 244, 104336. doi: 10.1016/j.livsci.2020.104336
Muir S. K., Ward G. N., Jacobs J. L. (2015). Herbage intake and milk production of late-lactation dairy cows offered a second-year chicory crop during summer. J. Dairy Sci. 98, 8825–8835. doi: 10.3168/jds.2014-9147
National Research Council (2007). Nutrient Requirements of Small Ruminants: Sheep, Goats, Cervids, and New World Camelids (Washington, DC: The National Academies Press). doi: 10.17226/11654
Nwafor I. C., Shale K., Achilonu M. C. (2017). Chemical Composition and Nutritive Benefits of Chicory (Cichorium intybus) as an Ideal Complementary and/or Alternative Livestock Feed Supplement. Sci. World J. 2017, e7343928. doi: 10.1155/2017/7343928
Oeffner S. P., Qu Y., Just J., Quezada N., Ramsing E., Keller M., et al. (2013). Effect of flaxseed supplementation rate and processing on the production, fatty acid profile, and texture of milk, milk fat, and cheese. J. Dairy Sci. 96, 1177–1188. doi: 10.3168/jds.2012-5941
Peña-Espinoza M., Valente A. H., Thamsborg S. M., Simonsen H. T., Boas U., Enemark H. L., et al. (2018). Antiparasitic activity of chicory (Cichorium intybus) and its natural bioactive compounds in livestock: a review. Parasitol. Vectors 11, 475. doi: 10.1186/s13071-018-3012-4
Reczyńska D., Witek B., Jarczak J., Czopowicz M., Mickiewicz M., Kaba J., et al. (2019). The impact of organic vs. inorganic selenium on dairy goat productivity and expression of selected genes in milk somatic cells. J. Dairy Res. 86, 48–54. doi: 10.1017/S0022029919000037
Rodríguez R., Suazo C., Balocchi O., Alomar D. (2019). Milk production and quality from ewes grazing a plantain-chicory mixture or a grass-based permanent sward. Small Rumin. Res. 170, 91–96. doi: 10.1016/j.smallrumres.2018.11.022
Roseler D. K., Ferguson J. D., Sniffen C. J., Herrema J. (1993). Dietary protein degradability effects on plasma and milk urea nitrogen and milk nonprotein nitrogen in holstein cows. J. Dairy Sci. 76, 525–534. doi: 10.3168/jds.S0022-0302(93)77372-5
Russell K. E., Roussel A. J. (2007). Evaluation of the ruminant serum chemistry profile. Vet. Clin. North Am. Food Anim. Pract. Ruminant Diagn. Med. 23, 403–426. doi: 10.1016/j.cvfa.2007.07.003
Samimi A. S., Aghamiri S. M., Nazifi S., Asadi Z. (2021). Changes in ghrelin, microminerals, antioxidants and vitamins A, E and C levels during different physiological status in high yielding Saanen goats subjected to heat stress. J. Therm. Biol. 100, 103014. doi: 10.1016/j.jtherbio.2021.103014
Séboussi R., Tremblay G. F., Ouellet V., Chouinard P. Y., Chorfi Y., Bélanger G., et al. (2016). Selenium-fertilized forage as a way to supplement lactating dairy cows. J. Dairy Sci. 99, 5358–5369. doi: 10.3168/jds.2015-10758
Snowder G. D., Glimp H. A. (1991). Influence of breed, number of suckling lambs, and stage of lactation on ewe milk production and lamb growth under range conditions. J. Anim. Sci. 69, 923–930. doi: 10.2527/1991.693923x
Soliman E. B., El-Moty A. K. I., Kassab A. Y. (2012). Combined effect of vitamin E and selenium on some productive and physiological characteristics of ewes and their lambs during suckling period. Egypt. J. Sheep Goat Sci. 7, 31–42.
Sookoian S., Pirola C. J. (2012). Alanine and aspartate aminotransferase and glutamine-cycling pathway: Their roles in pathogenesis of metabolic syndrome. World J. Gastroenterol. WJG 18, 3775–3781. doi: 10.3748/wjg.v18.i29.3775
Sordillo L. M., Aitken S. L. (2009). Impact of oxidative stress on the health and immune function of dairy cattle. Vet. Immunol. Immunopathol. 128, 104–109. doi: 10.1016/j.vetimm.2008.10.305. Special Issue: The 8th International Veterinary Immunology Symposium (8th IVIS).
Stewart W. C., Bobe G., Pirelli G. J., Mosher W. D., Hall J. A. (2012). Organic and inorganic selenium: III. Ewe and progeny performance. J. Anim. Sci. 90, 4536–4543. doi: 10.2527/jas.2011-5019
Suttle N. (2010). Selenium. Miner. Nutr. Livest (New York, NY, USA: CABI), 377–425. doi: 10.1079/9781845934729.0377
Tian J., Na R., Yu Z., Liu Z., Liu Z., Yu Y., et al. (2018). Inoculant effects on the fermentation quality, chemical composition and saponin content of lucerne silage in a mixture with wheat bran or corn husk. Anim. Prod. Sci. 58, 2249–2257. doi: 10.1071/AN16407
Trevisi E., Amadori M., Cogrossi S., Razzuoli E., Bertoni G. (2012). Metabolic stress and inflammatory response in high-yielding, periparturient dairy cows. Res. Vet. Sci. 93, 695–704. doi: 10.1016/j.rvsc.2011.11.008
Tsai C.-Y., Hassan R., Hung H.-C., Weber T., Price W. J., Rezamand P., et al. (2021). A rapid blood test to monitor the immune status change of dairy cows and to evaluate their disease risk during the periparturient period. Sens. Int. 2, 100078. doi: 10.1016/j.sintl.2020.100078
USGS (2021). Selenium in Counties of the Conterminous States. Available online at: https://mrdata.usgs.gov/geochem/doc/averages/se/usa.html (Accessed 2.27.22).
Weiss W. P., Hogan J. S. (2005). Effect of selenium source on selenium status, neutrophil function, and response to intramammary endotoxin challenge of dairy cows*. J. Dairy Sci. 88, 4366–4374. doi: 10.3168/jds.S0022-0302(05)73123-4
Whitlock H. V. (1948). Some modifications of the McMaster helminth egg counting technique and apparatus. J Counc Sci Indiana 21, 8945–8949.
Wichtel J. J. (1998). A review of selenium deficiency in grazing ruminants Part 1: New roles for selenium in ruminant metabolism. N. Z. Vet. J. 46, 47–52. doi: 10.1080/00480169.1998.36055
Wilson R. L., Bionaz M., MacAdam J. W., Beauchemin K. A., Naumann H. D., Ates S. (2020). Milk production, nitrogen utilization, and methane emissions of dairy cows grazing grass, forb, and legume-based pastures. J. Anim. Sci. 98, skaa220. doi: 10.1093/jas/skaa220
Keywords: sheep, peripartum, selenium, chicory silage, immunology
Citation: Ford H, Hasan D, Ates S, Puerto-Hernandez G, Klopfenstein JJ, Trevisi E, Smallman M, Matra M and Bionaz M (2024) Feeding chicory silage, but not Se-yeast or a single injection of inorganic Se, affects metabolism, fat in milk, and type I immunity in transition ewes. Front. Anim. Sci. 5:1499480. doi: 10.3389/fanim.2024.1499480
Received: 20 September 2024; Accepted: 22 October 2024;
Published: 11 November 2024.
Edited by:
Vincenzo Lopreiato, University of Messina, ItalyReviewed by:
Morteza Hosseini-Ghaffari, University of Bonn, GermanyAndrea Cabiddu, Agris Sardinia, Italy
Copyright © 2024 Ford, Hasan, Ates, Puerto-Hernandez, Klopfenstein, Trevisi, Smallman, Matra and Bionaz. This is an open-access article distributed under the terms of the Creative Commons Attribution License (CC BY). The use, distribution or reproduction in other forums is permitted, provided the original author(s) and the copyright owner(s) are credited and that the original publication in this journal is cited, in accordance with accepted academic practice. No use, distribution or reproduction is permitted which does not comply with these terms.
*Correspondence: Massimo Bionaz, TWFzc2ltby5iaW9uYXpAb3JlZ29uc3RhdGUuZWR1