- 1Sistema Ganadero Extensivo, INIA Tacuarembó, Instituto Nacional de Investigación Agropecuaria, Tacuarembó, Uruguay
- 2Sistema Ganadero Extensivo, INIA Las Brujas, Instituto Nacional de Investigación Agropecuaria, Canelones, Uruguay
- 3Polo de Desarrollo Universitario Agroforestal, Centro Universitario Regional Noreste, Universidad de la República, Tacuarembó, Uruguay
- 4Área de Transferencia, Secretariado Uruguayo de la Lana, Montevideo, Uruguay
- 5Ingeniería Agronómica, Facultad de Ingeniería y Tecnología, Universidad Católica del Uruguay, Montevideo, Uruguay
Introduction: Improving the feed efficiency (FE) in ruminants offers significant potential for optimizing the use of natural feed resource while simultaneously enhancing food and wool production. However, selecting for FE and high productivity may negatively impact the robustness traits, such as reproduction, health, and fat deposition, indicating potential antagonisms. This study evaluates the relationship between residual feed intake (RFI) and the productivity of yearling and adult ewes in extensive outdoor pastoral systems of three breeds.
Methods: Conducted from 2018 to 2022, the study included three cohorts (2018, 2019, and 2020) of ewe lambs from the Merino (435), Dohne (323), and Corriedale (215) breeds. In their first year, these lambs were phenotyped for feed intake, RFI, methane emissions, body weight and body weight, body condition score, fecal egg count, wool production, rib eye area, and fat thickness. Later, as hoggets or adult ewes, their body weight, body condition score, wool production, and reproductive traits were recorded. To examine the links between FE and performance, two contrasting groups (i.e., low 25% and top 75% quartiles) for RFI were analyzed.
Results and discussion: More efficient animals had similar body weights and weight gains while consuming less feed than the less efficient ones. In addition, the wool production at 1 year of age, the resistance to gastrointestinal parasites (assessed by fecal egg count), and the daily methane emissions were unaffected by RFI group. Although no significant antagonisms were observed between yearling RFI and ewe reproduction, a trade-off with ewe fleece weight was identified. In conclusion, the more efficient animals demonstrated satisfactory performance under the evaluated conditions without compromising body weight, wool production (in yearlings), reproduction, or health.
1 Introduction
There is global concern about the efficient use of natural resources in the livestock sector, where alternatives that support sustainable development can play a significant role (Navajas et al., 2022). In particular, it is considered that livestock farming should increase food production without competing for land resources with other sectors that also provide food for humans (Henry et al., 2018). One suggested alternative to increasing resource use efficiency, specifically feed, is the selection of animals based on their feed conversion efficiency (FCE) (Archer et al., 1999). Efficiency evaluated through residual feed intake (RFI) has the potential to be included in a genetic breeding program. RFI is a heritable trait, with heritability values ranging from 0.11 to 0.45 (Cammack et al., 2005; Johnson et al., 2022; Paganoni et al., 2017; Tortereau et al., 2020). More efficient animals consume less feed (consistent across studies) without significant changes in the growth, carcass, or wool traits (Cockrum et al., 2013) or the resistance to gastrointestinal parasites (Ferreira et al., 2021; Douhard et al., 2022).
It has been hypothesized that selecting for RFI could have favorable consequences for mitigating the net methane emissions and methane intensity in cattle and sheep (Cottle et al., 2011). Phenotypically, lower net methane emissions have been reported in more efficient animals when comparing groups with contrasting RFIs (Nkrumah et al., 2006; Waghorn and Hegarty, 2011). From a genetic perspective, Paganoni et al. (2017) indicated that the selection for RFI would indirectly select animals that emit less daily methane. However, there is no consensus on this aspect, as a negative and therefore unfavorable genetic correlation between RFI and net methane emissions has also been reported by Johnson et al. (2022).
Douhard et al. (2022), based on the resource allocation theory (Huber, 2017; Rauw, 2012), suggested that the selection for productivity may present antagonisms with resilience or the robustness variables, which could be exacerbated when also selecting for feed efficiency. Although their studies could not confirm this theory, other results might indicate some antagonisms with the selection for improving RFI in sheep and cattle. Johnson et al. (2022) reported unfavorable phenotypic correlations between RFI and subcutaneous fat deposition in sheep. In cattle, phenotypic differences in fat deposition have also been observed between RFI groups (Lines et al., 2014). Reproductive traits in cattle generally show no effect of RFI on milk production, calf growth, or calving ease. However, associations with fertility, the weaning rate, and age at puberty are mixed, with unfavorable or null effects reported (Kenny et al., 2018). The impact of RFI on reproductive performance in cattle may be linked to the interactions between adipose tissue and reproduction. In sheep, studies linking RFI with reproductive aspects in extensive pastoral systems are lacking.
Different correlations between RFI and other variables according to breed type have been reported (Arthur et al., 2014). Genotype by RFI and environment (different diet) interactions have also been found, although the measurement of RFI was limited by the low accuracy of feed intake on grazing conditions (Cantalapiedra-Hijar et al., 2018). This underpins the relevance of studying local populations and environments. The hypothesis of our work was that more efficient animals would have the same body weight (BW) and weight gain while consuming less feed than less efficient animals. Simultaneously, wool production and quality and the resistance to gastrointestinal parasites would not be affected, while the fat deposition of more efficient animals would be lower. In terms of reproduction (i.e., fertility, prolificacy, and weaning), this would be lower in more efficient animals. Therefore, the objective of this study was to evaluate the performance in the first year of life and up to 4 years of age of females contrasting for the FCE trait.
2 Materials and methods
2.1 Animals and management
This section refers to the management of experimental animals. The study was conducted between 2018 and 2022 and included data from females born in 2018, 2019, and 2020 in the Merino, Dohne, and Corriedale information nuclei, located at the Glencoe Experimental Unit (UEG) of the National Institute of Agricultural Research of Uruguay (INIA).
Each information nucleus had its own selection objectives. For the Merino breed, these were to reduce the wool fiber diameter (FD), increase fleece and BW, and improve resistance to gastrointestinal parasites. In Corriedale, the aims were to increase multiple births and resistance to gastrointestinal parasites and to reduce the wool FD. For Dohne, the objective was to select animals with average BW and fat thickness, moderate fleece weight, and low wool FD. The selection of animals considered health aspects (background of parasitic and/or infectious diseases) and the phenotypic (breed standards) and genetic merits assessed based on estimated breeding values (EBVs), which are provided by the genetic evaluation systems of these breeds.
The animals were born from late August to October and were weaned in late December or early January, the same date within each cohort and breed. Prior to weaning, lambs were trained to eat supplement, along with their mothers, and were supplemented during the summer (1% of BW, 70% corn grain/30% soybean meal) on native pastures (NPs). The weaning ages were 109 ± 9, 125 ± 20, and 124 ± 27 days for Corriedale, Merino, and Dohne, respectively. Ewe lambs were reared on NPs with the goal of reaching at least 80% of adult weight by the first mating time (18 months of age). At 16 months of age (January), replacement females were selected according to the selection objectives of each nucleus. The maximum age considered in this study was 4 years (fourth fleece).
Within each breed, the animals were genetically linked between years using common sires; in total, experimental animals are the progeny of 18, 12, and 12 sires of Merino, Dohne and Corriedale, respectively. The experimental animals that were selected as replacement females of each nucleus were bred as follows. In 2020–2022, Corriedale and Dohne breeding flocks were mated in the second half of March in collective field groups (30–40 ewes per ram) for 34 days, using a total of 9 and 14 sires, respectively. Small proportions of Corriedale and Dohne ewes (5.7% and 5.5% of records, respectively) were inseminated with fresh semen as part of the genetic connection of these nuclei. For this insemination, estrus was synchronized utilizing two injections of prostaglandin. At 14 days after the last injection, estrus was determined in all ewes every 12 h, and cervical insemination was performed 12–24 h after the onset of estrus, followed by natural mating in the field. Merino experimental ewes were mated in collective field mating groups (three rams, 30–40 ewes per ram) between 2020 and 2022, in sum with a total of 25 sires, starting 1–2 weeks after the other flocks.
All breeding flocks remained on NPs most of the year, grazing mixed with cattle. Prior to insemination or mating, they were supplemented for 15 days with 320 g per animal per day of a corn grain and soybean meal mix (80/20). At pre-lamb shearing, during mid-gestation, the animals were separated by pregnancy rank (i.e., non-pregnant, single, or multiple), and supplementation began with 200 g of pellets per animal per day of corn grain (80%) and soybean meal (18.5%) with sodium bicarbonate (1.5%). In the last third of gestation, ewes carrying twins (and triplets) were given timed access to improved pastures or meadows, with increased supplementation (350–400 g per animal per day) until lambing. The twin-bearing ewes nearing parturition stayed 24 h in pasture and, after lambing, were moved to lambing pens for 24–48 h with alfalfa hay. They then stayed in improved pastures until lamb marking (from September to October) and afterward returned to NPs. Single-bearing ewes (one fetus) stayed in NPs until the week before lambing, when they were moved to ryegrass with shelter and refuges (used according to the chill index for the next 3 days) for lambing. After lambing, within a week, the mothers and lambs were gradually moved back to NPs without supplementation. The management of the mothers of the experimental animals was identical to the management described for their progeny.
2.2 Measurements
The animals of each cohort were born at the UEG and were transferred at the same age within each breed during their first 14 months of life to the La Magnolia Experimental Unit for approximately 60 days for the evaluation of feed intake, methane emission, and RFI. After this evaluation, they were returned to the UEG (Table 1), where the performance traits were recorded on grazing NPs. Each breed was phenotyped for RFI every year at the same age. The timing of the FCE test and the number of animals evaluated differed between breeds. Corriedale ewe lambs (214 animals) were evaluated between February and April, Merino ewe lambs (435 animals) between April and September, and Dohne ewe lambs (324 animals) between September and November. The ages at the beginning of the FCE test were 190 ± 15, 333 ± 27, and 420 ± 8 days of life for Corriedale, Merino, and Dohne, respectively.
The information presented corresponds to 15 FCE tests conducted between 2019 and 2021. During these FCE tests, the diet provided ad libitum to the animals was 100% chopped (5–10 cm) alfalfa haylage. This diet was sampled twice a week to monitor dry matter (DM), after being dried for 72 h at 60°C in a forced air oven. These dry samples were ground to 1 mm and then analyzed to determine the chemical composition. The analytical DM (AOAC, 1990), ash (AOAC, 1990), acid detergent fiber (ADF) and neutral detergent fiber (NDF) (ANKOM Technology method; AOAC, 2012), the N content (Kjeldahl; AOAC, 1995), ether extract (EE) (ANKOM Technology method) (Yemm and Willis, 1954), and crude protein (CP) (Goering and Van Soest, 1970) were estimated. Metabolizable energy (ME) was estimated as: ME (MJ/kg DM) = 4.184 × ((4.4 × 0.82 × DMD)/100) (Agricultural Research Council—ARC, 1980), while the dry matter digestibility (DMD) was calculated based on the following equation: DMD (%) = 88.9 − (0.779 × ADF%) (Osítis et al., 2003). The chemical composition and the calculated ME are given in Table 2.

Table 2. Dry matter (DM, in percent), chemical composition (percent in the DM), and calculated ME of Lucerne haylage used in the FCE test over the 3 years (mean ± SD).
To evaluate the feed efficiency, the animals were assigned to one of five pen-automated feeding systems based on the type of birth, sire, and BW (grouping those with similar BW together) recorded after a 14-day acclimatization period. The evaluation spanned a 56-day test period (14 additional days for acclimatization and 42 days for measurements). All animals were tagged with electronic radio frequency identification (RFID) tags. Each pen was equipped with five individual automated feeders and two weighing platforms, which included an electronic tag reader, a precision scale, and a connection to a central computer. Each morning, any remaining feed was removed from the feeders, which were then cleaned and refilled with fresh feed. The feed bins were subsequently refilled two to three times throughout the day to ensure ad libitum intake and to minimize feed selectivity. In addition, the feed amount in each bin before the first meal was maintained at over 1 kg, and feeder occupancy was monitored to stay below 20 h/day. The described setup enabled daily monitoring of the feed intake and BW. A software system identified animals entering the feeder and recorded the BW measurements from the weighing platforms. The equipment and software were provided by Intergado (Belo Horizonte, MG, Brazil). The monitoring system (AF-1000 Master) featured an RFID antenna embedded in a rubberized mat lining the neck bars, and load cells measured the feed intake. When sheep passed their heads through the neck bars, the RFID antenna was activated via an integrated infrared presence sensor. The RFID tags allowed for the identification of specific animals at the feed bins, recording individual visits, including the start and end times, and calculating intake based on the weight differences before and after eating. BW was recorded each time the animals accessed the water bins, with a sensor similar to the feed bin system. The RFID tags enabled the identification of specific animals at the BW platform during water bin access, recording the BW per visit. Each visit to the feed bin and the BW platform was documented by recording the animal’s identification tag, bin, and platform number. These data were continuously collected via a network cable and transferred to the central computer, and subsequently to the Intergado web software data center using a general packet radio service.
The methane emissions (in grams per day) were estimated following the portable accumulation chamber (PAC) protocol described by Goopy et al. (2011, 2016), Paganoni et al. (2017), Robinson et al. (2014), and Jonker et al. (2020). In brief, two estimates per animal were performed during the last 2 weeks of the FCE test (at least 1 week between estimates). The traits recorded were methane (CH4) emissions, carbon dioxide (CO2) emissions, and oxygen (O2) consumption. In the measurement week, one pen per day was measured in consecutive runs of 10 animals. Therefore, 20 animals per day were recorded, totalling 100 animals by the end of the week. If the FCE test considers more than 100 animals, an extra run per day was performed, when necessary. In accordance with Robinson et al. (2020), the animals were on feed until the time of measurement. Thereafter, the lambs were allocated to one of 10 sealed chambers of 862 L volume. After 20–30 and 40–50 min, the concentrations of CH4, CO2, and O2 were recorded, as well as measurements of temperature, atmospheric pressure, and gas concentration in the air. Gases were measured with an Eagle 2 equipment (RKI Instruments, Union City, CA, USA). Eagle 2 and the chambers were checked between measurement weeks, and Eagle 2 was calibrated periodically in accordance with the specifications provided by RKI Instruments.
The first fleece shearing was performed at 407 ± 15, 413 ± 9, and 397 ± 7 days of life for Corriedale, Merino, and Dohne, respectively. BW (unfasted) and the body condition score (BCS; scale 1–5) (Kenyon et al., 2014) were evaluated the day after shearing. The rib eye area and the fat thickness were assessed after shearing using ultrasound on the longissimus thoracis et lumborum muscle as described by Ramos et al. (2019). In terms of wool production and quality, the greasy fleece weight, FD, and staple length (SL) were measured. At shearing, the fleece was weighed, and a mid-side wool sample from each animal was collected and sent to a commercial wool testing laboratory (Uruguayan Wool Secretariat, Montevideo, Uruguay) where the FD and SL were evaluated. Three staples were randomly chosen from the mid-side sample to measure SL. FD was measured according to IWTO standards 52 (IWTO, 2006) and 12 (IWTO, 2012). Gastrointestinal nematode infestation was assessed twice by determining the worm egg counts in fecal samples (FEC) using the modified McMaster technique (Whitlock, 1948). At weaning, the lambs were treated with an effective anthelmintic drug (based on previous anthelmintic resistance diagnosis) and then exposed to natural pasture infestations. Between weaning and shearing, the fecal egg count was monitored. Once the average FEC for each treatment group exceeded 500 eggs per gram of wet feces, the individual FEC were collected and analyzed. This procedure was repeated for the second FEC record. The ages at the first FEC sampling were 266 ± 3, 245 ± 3, and 254 ± 8 days of life for Corriedale, Merino, and Dohne, respectively.
For hoggets and ewes, the unfasted BW and BCS were determined just before mating and lambing, as well as at weaning. The pregnancy status and the number of fetuses per ewe were diagnosed by the same technician using transabdominal ultrasonography 45 days after the end of mating. With the information from the pregnancy scan, the fertility, prolificacy, and lambing potential were calculated. Fertility was defined as the number of pregnant ewes divided by the number of ewes exposed. Prolificacy was defined as the number of lambs born per ewe that lambed, while the lambing percentage was defined as the number of lambs born per ewe exposed. During lambing, ewes were observed twice daily, with the date of parturition and the number of lambs born per ewe recorded. Lamb survival was calculated as the difference between the number of lambs born and the number of lambs marked at marking, while weaning weight was calculated as the kilograms of lambs weaned per ewe. The wool traits of ewes were measured following the same procedure applied to yearlings.
2.3 Calculations and statistical analysis
All statistical analyses were performed using SAS software (SAS® Studio version 3.81, SAS Institute Inc., Cary, NC, USA). It is important to highlight that the work conducted focused on the evaluation of animals within each breed and that the experimental design did not allow for comparisons between breeds for any of the variables.
Initially, the animals were categorized according to their RFI values (Koch et al., 1963). The methods used to calculate the body weight gain (BWG), daily feed intake (in kilograms DM per day), daily ME intake (in megajoules per day), and RFI have been described in detail by Amarilho-Silveira et al. (2022). Animals with BWG less than 0.01 kg or a coefficient of determination (r2) less than 0.5 were not considered in further analyses. The RFI calculation was performed within each breed using the following model:
where Y is the observed individual daily average ME intake; µ is an all-animal constant that refers to the average daily ME intake; P × T represents pen (P) and the FCE test (T) (which includes the generation and year effect); BW0.75 is the mid-test metabolic body weight; BWG is the daily body weight gain; AGE is the age at the beginning of the FCE test as a covariate; and ε is the residual error as RFI (the difference between the observed and the expected ME intake). Based on the estimated individual RFI values, those with values in the lowest 25% percentile (first quartile) were included in the most efficient group (Low RFI group), while those with values at or above the 75% percentile (above the third quartile) were allocated into the High RFI group (the less efficient).
With regard to the calculations estimating the methane emissions, the data from each batch of methane measurements were transformed considering the BW of the animal, the time in the PACs, the gas concentration inside and outside of the chamber, and the temperature and atmospheric pressure (Jonker et al., 2020). The pen, batch, and FCE test were considered in the model to estimate methane as a fixed effect. Firstly, the concentration of CH4 was calculated in liters per day, as shown below:
where ΔCH4 is the difference between the final and initial gas concentrations; ΔPACtime is the difference between the end and start times (hour:minutes:seconds) per animal measurement period; PACv is the PAC volume measured between the water line that seals the chamber to the top of the PAC; and BW is the body weight of the animal on the day of measurement. Thereafter, the liter per day value was converted to grams per day at standard temperature and pressure, as shown below:
where 22.4 is the molar volume (in liters) of a gas at standard pressure and temperature (STP) and 16.043 is the molar weight of CH4. STP = 273.15 K/(273.15 K + temperature) × (pressure (kPa)/101.3), where 101.3 kPa is the standard atmospheric pressure at sea level and 273.15 K is the equivalent of 1°C. The data for each animal are the average of the two measurements in the evaluation period of the consumption test.
For the traits evaluated in the first year of life and the later life of selected ewes, a descriptive analysis was performed. The mean and standard deviation of each trait are presented in Results (Tables 3, 4). Subsequently, to evaluate the effect of the FCE group on female performance in the first year, a general linear model (GLM) was used based on the GLM procedure in SAS. The model included the fixed effect of the RFI group (previously established, which included the generation, year, and age at determination).
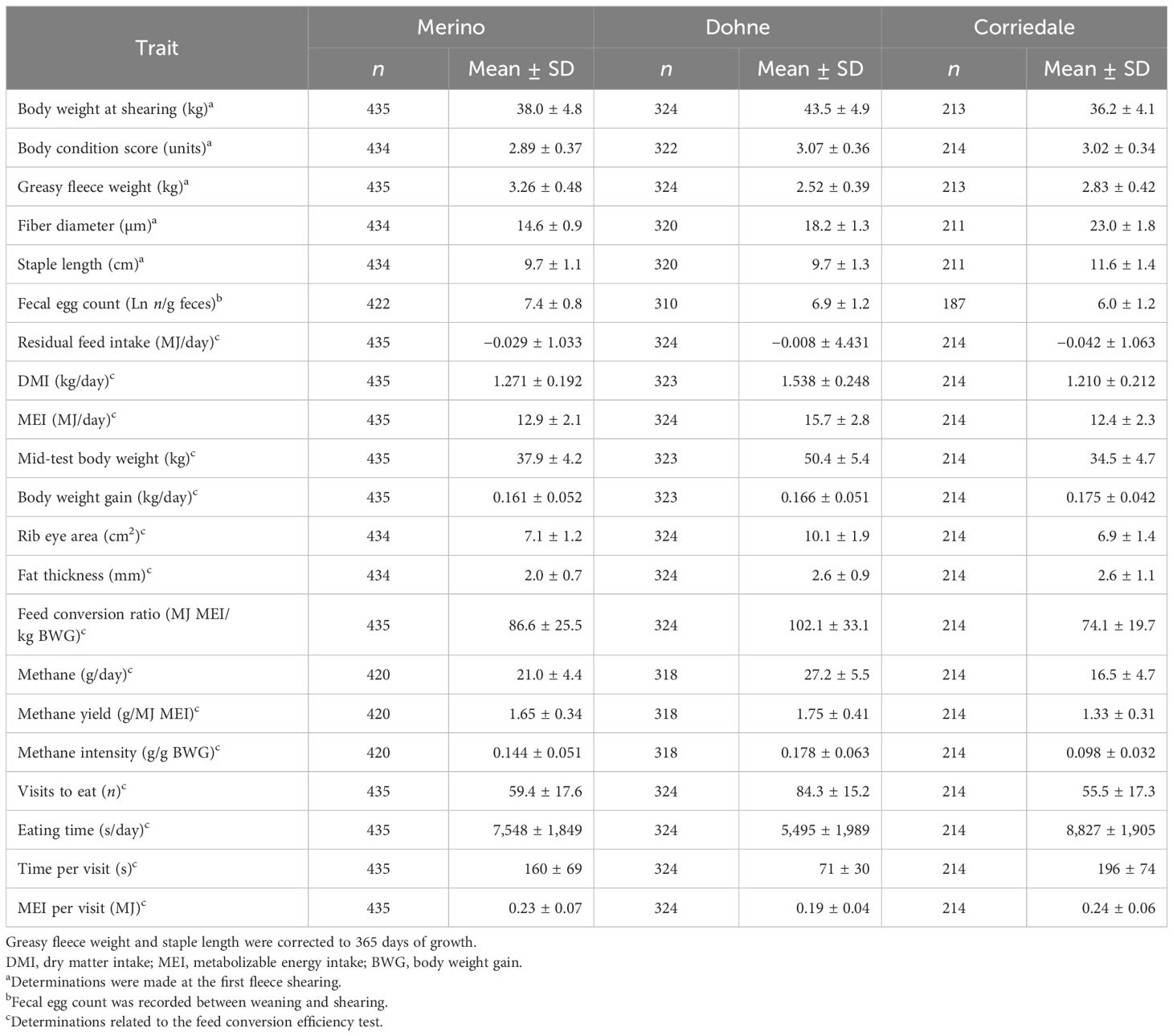
Table 3. Mean (±SD) and number of females evaluated during their first year of life for each trait and breed.
For maternal traits such as BW, BCS, and wool characteristics, as well as lamb production in kilograms, the GLM included the effects of year–generation (age) and RFI group. For weight and condition at mating, the fetal load of the previous year was included as a covariate; for pre-partum and weaning determinations, the fetal load of the current year was included as a covariate. For the trait lamb weight at weaning, the management group (i.e., not pregnant, pregnant with one lamb, or pregnant with two lambs) was included, and the weaning weight of lambs was corrected to 110 days of age.
Fertility was analyzed considering a binomial distribution, while prolificacy, lambing, and lamb survival (i.e., the difference between lambs marked and born per ewe) were considered to have a Poisson distribution. In these traits, a GLM with the GENMOD procedure in SAS was used. The fixed effects included were year–generation, ram, and RFI group. The results (Tables 5, 6) are presented as the least square means and standard errors for each trait for the High and Low RFI groups, with the least square means considered different when p < 0.05.
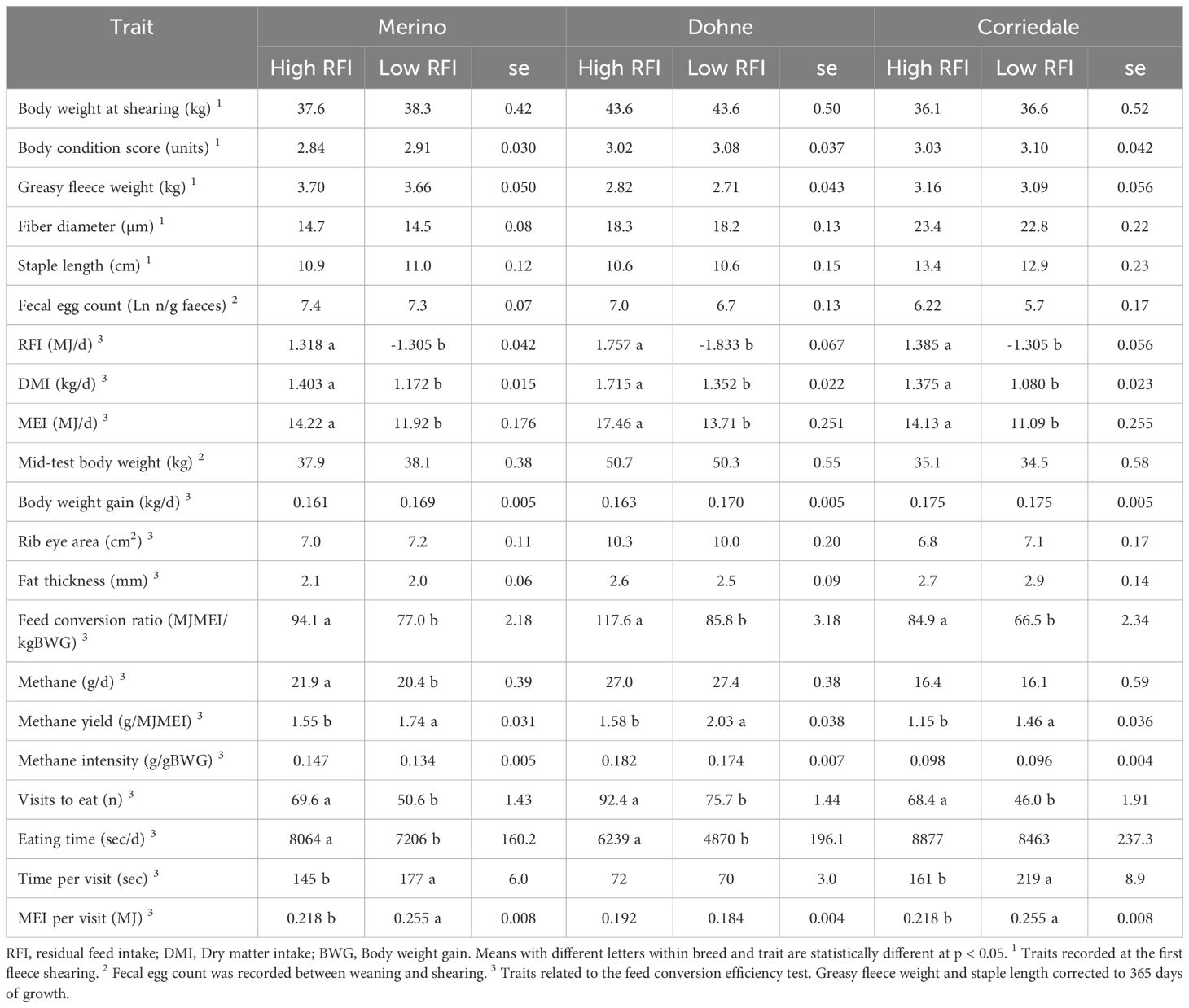
Table 5. Performance (least square mean and standard error) of females in their first year of life, according to their feed conversion efficiency phenotype within each evaluated breed.
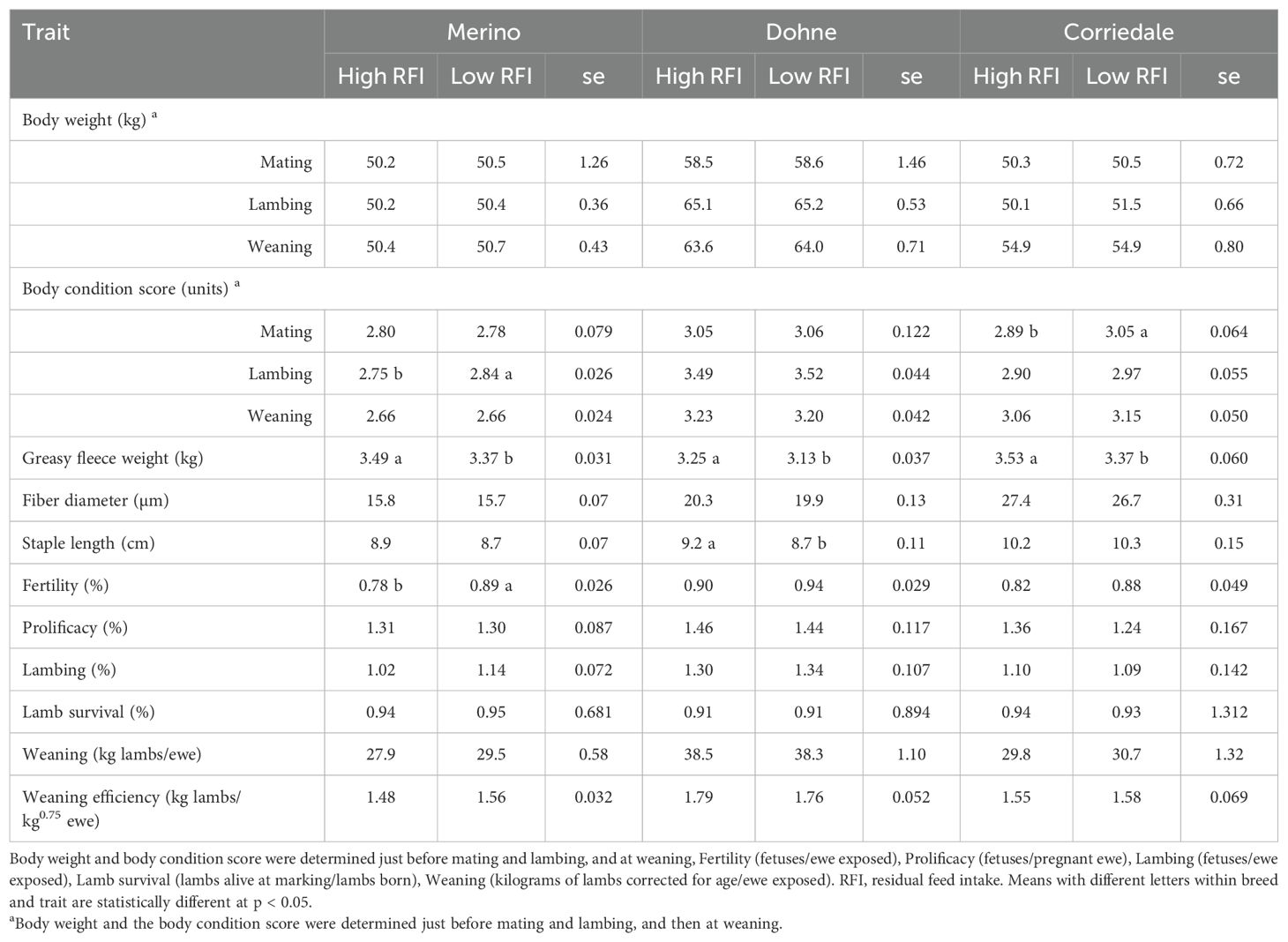
Table 6. Performance (least square mean and standard error) of ewes according to their feed conversion efficiency phenotype for each evaluated breed.
3 Results
Table 3 shows the number of animals and the means (±SD) of the parameters evaluated in yearlings, as well as the estimated and calculated parameters in the FCE test in females between weaning and 15 months of age. The animals reached a shearing weight of over 70% of adult weight, with a BCS around 3 units. The fleece weight ranged from 2.5 to 3.3 kg, with FDs between 14 and 23 µm, depending on the breed. In the FCE test, the animals achieved a FCE of 74–102 MJ of ME to produce 1 kg of BW, with feed intake between 3.0% and 3.5% of the average BW during the test.
Table 4 presents the number of records from the ewes evaluated (between 2 and 4 years of age) and the means (±SD) for each studied variable for the three breeds. The ewes reached mating with a BCS between 2.9 and 3.3 units and BWs of 48.3, 50.1, and 58.6 kg for Merino, Corriedale, and Dohne, respectively. The fertility rates ranged from 83% to 93%, while the lambing rates were between 106% and 132%. These dual-purpose breeds, with different emphases on meat and wool, produced between 3.0 and 3.4 kg of greasy fleece, with an average FD between 15 and 27 µm. In addition, the weaning weight of lambs (in kilograms of lambs/ewe) represented between 50% and 65% of the weight of ewes at mating.
The results of the comparison between the contrasting RFI groups in young females are presented in Table 5. Consistently, across all three breeds, there was no association (p > 0.05) between the RFI group and BW, BCS, wool production, wool FD, SL at first shearing, or resistance to gastrointestinal parasites in the first year of life. For the variables measured in the FCE test, the methane intensity, BW, BWG, rib eye area, and backfat thickness were similar between the RFI groups across all three breeds. The differences (p < 0.05) between the High and Low RFI groups observed in all three breeds included the total ME intake and RFI, FCE, and eating visits, with less efficient animals showing higher values in all cases. Conversely, the methane yield during the FCE test was lower in less efficient animals (High RFI group). In Merino, the more efficient animals had lower total methane emissions (p < 0.05).
In adult females (Table 6), for BW or BCS, no breed or stage of the cycle showed any differences between groups (p > 0.05), except for BCS at lambing in Merino and at mating in Corriedale, where the BCS of the more efficient animals was higher. Wool production was higher (p < 0.05) in the High RFI group (less efficient) across all three breeds. For Dohne, the difference in wool production was accompanied by a larger SL (p < 0.05). For the reproductive performance variables, no significant differences were detected between the High and Low RFI groups (p > 0.05), except for the higher fertility in Merino in the Low RFI group.
4 Discussion
The hypothesis that more efficient animals would have similar BW and BWG while consuming less feed than less efficient animals was accepted. Similarly, the hypothesis that wool production, quality, and resistance to gastrointestinal parasites in the first year of life would not be affected was also accepted. However, the assumption that more efficient animals would have lower fat deposition was rejected, considering fat thickness or BCS, which are indirect indicators of fatness. In addition, the lower reproductive performance on pastoral conditions in more efficient animals was not confirmed.
4.1 Performance of the evaluated populations
Females should reach at least 60% of their adult BW by the end of their first winter and 75% by their first mating (MLA and AWI, 2008). In this study, the females of all three breeds exceeded 70% of the adult BW at shearing, with a BCS of 3 units, which is suitable for mating (Kenyon et al., 2014), indicating that their rearing, primarily on NPs with strategic summer supplementation, was adequate. It is notable that the ewes’ weights in our study were lower than the adult flock average reported, due to their young age profile, as significant weight gain occurs until around four years of age (Cloete et al., 2003; Ramos et al., 2021).
The Merino nucleus had undergone 20 years of selection (1998–2018), initially to reduce FD, with added focus on increasing the body and fleece weights in the last decade. Compared with a similar Australian nucleus (Dominik and Swan, 2016), Uruguayan Merinos showed a finer wool (2 µm less), higher BW (~14 kg), and heavier fleece (~1 kg). In New Zealand, after 5 years of ultrafine Merino selection, animals had slightly higher BW (41.3 kg), lighter fleece (2.94 kg), and coarser wool fiber (16.8 µm) (Wuliji et al., 1999). Corriedale selection began in 2018, targeting increased multiple births, resistance to gastrointestinal parasites, and reduction of wool FD. Compared with a previous study at the same station (De Barbieri et al., 2021), these Corriedales had higher BW (36.2 vs. 33.3 kg), heavier fleece (2.83 vs. 2.58 kg), finer wool fiber (23.0 vs. 24.2 µm), and lower FEC. The Dohne nucleus, established in 2007, aimed to maintain or increase the BW and fat thickness while reducing wool FD. In this study, they showed lower BW, fleece weight, and wool FD than those in Australia and South Africa (Li et al., 2013; Van Wyk et al., 2008). In summary, yearling females met the production and selection goals of each breed, indicating an effective breeding program.
The feed intake measured in this study during the FCE tests fell within the range reported by other studies (1.2–2.3 kg DM/day) (Paganoni et al., 2017; Marie-Etancelin et al., 2019; Muir et al., 2020; Johnson et al., 2022; Lima et al., 2022). Those studies typically involved a variety of genotypes evaluated between 2 months and 1 year of age using either total mixed rations (TMR) or alfalfa pellets. The relative feed intake to BW was between 3.0% and 3.5%, resulting in daily weight gains of 160–175 g/day. These gains were generally lower than in many reports, primarily due to the differences in breed (wool breeds, dual-purpose, maternal, or meat breeds), age, and diet type (concentrate and TMR vs. forage). Similarly, the diet type could explain the different feeding behaviors observed, with more eating visits but lower intake per visit in this study. Finally, the daily methane emissions recorded here were within the reported range (17–33 g/day).
Although a comparison of breeds was not an objective of this study and the design did not allow for it, it is interesting to note that the net methane emissions in Corriedale were notably lower than those in the other groups, even per megajoule of ME or kilogram of BW. Similarly, the BWG relative to BW was higher in Corriedale, followed by Merino and then Dohne. While it is not possible to draw definitive conclusions, it is relevant to consider that these apparent differences may be due to the age of the animals at the time of the FCE test and their relative development to adult weight rather than breed differences. Animals fed high-quality diets can express their potential intake, which tends to be relatively higher in younger animals (such as Corriedale, for example) (Zereu, 2016). Young animals also have a higher passage rate than older animals (Faichney, 2005), which might explain their higher relative intake. A higher passage rate associated with higher intake has been linked to lower methane yield (De Barbieri et al., 2015; Johnson and Johnson, 1995), supporting the lower emissions observed in young animals. According to Oddy and Sainz (2002), voluntary intake begins to decrease when animals reach 70% of adult BW, as observed in the Dohne animals in this study. Finally, the growth curve pattern of the fat and muscle deposition between genotypes is very similar when compared relatively to the adult weight of the genotype (Oddy and Sainz, 2002). Muscle is considered an intermediate deposition tissue and fat a later deposition tissue, with different energy densities between protein and fat, being 0.017 vs. 0.038 MJ/g, respectively (Geesink and Zerby, 2010), which is in accordance with the higher net energy content of gain fat (0.040 MJ/g) than protein (0.024 MJ/g) (Oddy and Sainz, 2002). This is consistent with the highly differential FCE observed between the breeds in our study. The ages of the different genotypes and their maturity stages, involving changes in tissue deposition and voluntary intake, might explain the superior relative weight gain of Corriedale compared with Merino and, finally, Dohne.
At mating, ewes weighed between 50 and 60 kg, with BCS near 3 units, which were within the recommended range for fertility, prolificacy, and efficient nutrient use (MLA and AWI, 2008; Kenyon et al., 2014). The Dohne ewes in our study had lower BW, BCS, and fleece weight, with a similar FD compared with those in the studies in South Africa and Australia (Cloete and Cloete, 2015; Ransom et al., 2015). A similar pattern was observed for the Merino ewes when compared with those in the two studies; however, compared with a fine Merino population (Dominik and Swan, 2016), the Uruguayan animals were heavier and produced more and finer wool. For Corriedale, the national data (De Barbieri et al., 2021; Kremer et al., 2010) show similar BWs, but with greater wool production and finer wool FD.
The Merino ewes showed higher fertility, prolificacy, and lambing rates than a fine Merino population (Dominik and Swan, 2016), but lower than a Merino flock selected for reproduction (Ransom et al., 2015), aligning with the BW, BCS, and breeding goals. Dohnes had reproductive results nearly identical to those in Australia (Ransom et al., 2015), despite being 10 kg lighter and having lower BCS. In Uruguay, the mid-pregnancy shearing, pre-mating and lambing supplementation, and lambing aligned with the peak of forage production might explain similar reproductive outcomes despite lower BW and BCS. In addition, the fleece-to-BW ratio in Uruguay (4.1%) versus Australia (5.0%) might have contributed as a higher ratio could hinder reproduction (Masters and Ferguson, 2019). Corriedale ewes showed similar fertility but notably higher prolificacy and lambing rates compared with previous studies (Kremer et al., 2010; De Barbieri et al., 2021), likely due to selection for multiple births.
4.2 Comparison of contrasting RFI groups
During the FCE test (Table 5), differences of 14%–22% in the DM intake were observed between the high and low efficiency groups, with no differences on the BWG between groups across all three breeds evaluated. This aligns with other studies (Lima Montelli et al., 2019; Redden et al., 2011; Tulux Rocha et al., 2018), with differences in intake of up to 30% reported between efficiency groups fed finishing diets (Gurgeira et al., 2022). This is in accordance with the literature, where a high, positive, and favorable phenotypic (0.54–0.62) and genetic (0.41–0.78) correlation between RFI and feed intake has been established (Cammack et al., 2005; Johnson et al., 2022; Tortereau et al., 2020). Lower feed intake has been mentioned as one of the explanations for higher feed efficiency together with differences in the heat production, protein turnover, mitochondrial function, and maintenance energy requirements (Hendriks et al., 2014; Cantalapiedra-Hijar et al., 2018).
The wool production and quality at 1 year of age did not differ between animals in the different RFI groups. Redden et al. (2011) found no differences in FD between efficiency groups. In another experiment, fleece weight was similar between groups (Redden et al., 2013). Cockrum et al. (2013) found no phenotypic correlation of fleece weight, SL, and FD with RFI in Rambouillet and Rambouillet×Targhee animals. Similarly, these previous studies did not report any correlation or effect of RFI on eye muscle area, fat thickness, or BCS of young animals, consistent with our results. However, in cattle, it has been suggested to include the body composition traits for the estimation of RFI (Pravia et al., 2022) in order to take into account an unfavorable association between RFI and subcutaneous fat (Herd et al., 2018). Results in sheep indicated no differences between groups, with measurement error possibly being high, and that fat thickness at one point may not be the best indicator of the overall adipose tissue levels (Cockrum et al., 2013). Indeed, Johnson et al. (2022), reported that less efficient animals with higher feed intake phenotypically deposited more subcutaneous fat assessed by ultrasonography, but similar values when assessed by computed tomography. However, the adipose tissue variables measured (i.e., intramuscular, subcutaneous, and visceral) showed a favorable genetic correlation with RFI (Johnson et al., 2022).
A strong selection pressure for productive traits combined with selection for FCE could have unfavorable consequences for energy reserves, health status, reproduction, or product quality (Huber, 2017; Rauw, 2012). Despite this, no antagonism between FCE and resistance to gastrointestinal parasites has been observed in sheep, as studied using different populations and approaches (Douhard et al., 2022; Ferreira et al., 2021). The results of this study are consistent in this aspect, and based on the number of years, animals, and breeds, it can be concluded that producing with more efficient animals does not necessarily translate to animals with lower resistance to gastrointestinal parasites on pastoral extensive systems.
Selecting animals for FCE has been suggested as an indirect strategy to reducing the net methane emissions (Hegarty et al., 2007). In this study, no consistent effect of RFI group on the daily methane emissions was observed while methane intensity was equal. However, the methane yield was higher in more efficient animals. This has been explained by a lower intake associated with a slower passage rate, resulting in longer ruminal retention time and greater degradation of the less degradable fractions per unit of feed, leading to higher methane emissions per kilogram of DMI (De Barbieri et al., 2015; Johnson and Johnson, 1995). The lack of consistent phenotypic correlation between the daily methane emissions and RFI has been previously reported (Johnson et al., 2022; Muir et al., 2020), although positive and favorable genetic correlations have been estimated, supporting the use of RFI genetic selection as a strategy for the mitigation of daily methane emissions (Johnson et al., 2022; Paganoni et al., 2017).
While not entirely consistent across all three breeds, some differences in the feeding behavior between the more and less efficient animals can be observed. The more efficient animals visited the feeder less frequently, with longer visits and higher intake per visit; although the total daily intake was lower, the intake rates were similar and the total times used to eat were also lower. This is consistent with the findings of Marie-Etancelin et al. (2019), who indicated that selecting efficient animals would alter the feeding behavior, resulting in fewer, longer feeding instances with higher intake per visit. However, the feed provided in their experiment (low-energy concentrated pellets) differed from that in this study (Lucerne haylage). Different activity has been indicated as another possible cause of the differences in feed efficiency (Kenny et al., 2018), where more efficient animals spend less time feeding; however, reports on feeding behavior vary. In the experimental protocol, several aspects, including considering refilling the feeders frequently, chopping the Lucerne haylage, maintaining low BW differences within each pen and FCE test, or the characteristics of the feed at harvest (e.g., vegetative stage and humidity), were considered. Although it is plausible that some feed selectivity occurred, further research exploring this aspect would be of interest.
In beef cattle, calf mortality could be lower in more efficient cows (Kenny et al., 2018). In addition, when studying fertility or the weaning percentage, either no differences were found in grazing cow groups or the results were higher in less efficient animals. Within the available information, no studies were found on sheep contrasting RFI and their association with fertility, lambing, or lamb mortality. The ewes in this study were managed grazing NPs, which considered the forage availability, the fetal load or pregnancy rank during gestation and lactation (with multiples accessing improved pastures), and strategic supplementation (pre-mating and pre-lambing). Given the large variation in forage production and quality (CP: 6%–15%; DMD: 50%–61%; ME: 7.5–9.2 MJ/kg DM) (Berretta and Bemhaja, 1998; Berretta et al., 2000) and their BCS of around 3 throughout the cycle, it can be inferred that intake was restricted (Freer et al., 2007). In restrictive feeding situations, the intake of more efficient animals was the same (Redden et al., 2013) as that of the less efficient ones, with better performance in the former. This might explain the similar performance between the RFI groups, without showing antagonism between RFI selection and productive outcomes in the later life of ewes. A high correlation between intakes at different life stages has been established (Paganoni et al., 2017), suggesting that more efficient animals with lower intakes might experience less restriction on NPs compared with less efficient animals. Finally, it should be noted that improved environments (supplementation or sown pastures) can mask a lack of resilience (Huber, 2017); therefore, if more efficient animals had lower resilience, the technological alternatives implemented might prevent this from manifesting.
Another relevant finding was the higher wool production in the less efficient adult animals. An earlier hypothesis (De Barbieri et al., 2020) suggested that less efficient animals might produce more wool due to the higher nutrient and feed demands of wool production over other tissue deposition. However, this was not observed phenotypically or genetically in yearlings (Marques et al., 2022), who were actively growing with access to improved nutrition. Thus, an increased wool production appeared only in adults, not in yearlings. This suggests that adult animals might express wool production variability due to feed efficiency only after reaching a stable metabolic and growth state, which was not apparent in actively growing yearlings with high nutrient demands and improved nutrition.
It is interesting that unfavorable correlations between wool production and reproduction have been reported (Ramos et al., 2023), with more pronounced adverse effects of increased wool production under restrictive feeding conditions (Masters and Ferguson, 2019). It is crucial to continue evaluating the wool production and reproduction in more and less efficient adult animals under nutritional constraints. In Uruguay, wool production has been selected for several generations (see genetic trends for wool or dual-purpose breeds; www.geneticaovina.com), which is known to have a low unfavorable correlation with reproductive aspects. It is important to understand potential antagonisms with FCE when evaluated in the first year of life and the economic outcome of these relationships.
5 Conclusion
Our findings suggest that selecting for improved feed efficiency using RFI as a selection criterion can reduce the feed intake in sheep while maintaining adequate performance under grazing conditions in both yearlings and adults, without compromising wool quality, BW, reproduction, net methane emissions, or health. Therefore, this selection strategy supports sustainable intensification. Future research, while addressing feed selectivity, should also explore total fat deposition and adult wool production in greater depth, along with their interactions with FCE.
Data availability statement
The datasets presented in this article are not readily available because legal restrictions apply to their availability. The data were obtained by INIA and are available from the authors with INIA’s permission. Requests to access the datasets should be directed to Ignacio De Barbieri, aWRlYmFyYmllcmlAaW5pYS5vcmcudXk=.
Ethics statement
The animal study was approved by Comisión de Ética en el Uso de Animales de Experimentación de INIA (CNEA, registro 0009/11) with file number INIA 2018.2. The study was conducted in accordance with the local legislation and institutional requirements.
Author contributions
ID: Conceptualization, Funding acquisition, Investigation, Methodology, Project administration, Writing – original draft. EN: Conceptualization, Funding acquisition, Methodology, Writing – review & editing. ZR: Investigation, Methodology, Writing – review & editing. GF: Investigation, Methodology, Writing – review & editing. JV: Conceptualization, Methodology, Writing – review & editing. GC: Conceptualization, Data curation, Formal analysis, Funding acquisition, Methodology, Writing – review & editing.
Funding
The author(s) declare financial support was received for the research, authorship, and/or publication of this article. This study was supported by the following projects: CRILU, funded through a public-private partnership; Rumiar and CL40, funded by INIA; Smarter, funded by Horizon 2020 (Grant No. 772787); and GrasstoGas, funded through ERA-NET SusAn, ERA-NET FACCE ERA-GAS, and ERA-NET ICT-AGRI 2.
Acknowledgments
The dedication, commitment and work of the INIA Tacuarembó and INIA Las Brujas staff is acknowledged, as well as a large number of students (from Uruguay, Brazil, Colombia, France, Mexico) who collaborate while completing their undergraduate or postgraduate work or their international internships. ChatGPT (version GPT-4, model OpenAI GPT-4-turbo, developed and maintained by OpenAI) was used to translate the original draft and to improve the manuscript, afterwards all authors revise and edit the entire manuscript.
Conflict of interest
The authors declare that the research was conducted in the absence of any commercial or financial relationships that could be construed as a potential conflict of interest.
Publisher’s note
All claims expressed in this article are solely those of the authors and do not necessarily represent those of their affiliated organizations, or those of the publisher, the editors and the reviewers. Any product that may be evaluated in this article, or claim that may be made by its manufacturer, is not guaranteed or endorsed by the publisher.
References
Agricultural Research Council—ARC (1980). The nutrient requirements of ruminant li- vestock. Commonwealth Agricultural Bureaux (London, UK: The Gresham Press).
Amarilho-Silveira F., de Barbieri I., Cobuci J. A., Balconi C. M., de Ferreira G. F., Ciappesoni G. (2022). Residual feed intake for Australian Merino sheep estimated in less than 42 days of trial. Livestock Sci. 258, 104889. doi: 10.1016/j.livsci.2022.104889
AOAC (1990). International (formerly the Association of Official Analytical Chemists). Official Methods of Analysis (Arlington, VA: AOAC International).
AOAC (1995). International (formerly the Association of Official Analytical Chemists). Official Methods of Analysis (Arlington, VA: AOAC International).
AOAC (2012). Official Methods of Analysis, 19th edn (USA: Association of Official Analytical Chemists).
Archer J. A., Richardson E. C., Herd R. M., Arthur P. F. (1999). Potential for selection to improve efficiency of feed use in beef cattle: a review. Aust. J. Agric. Res. 50, 147–161. doi: 10.1071/A98075
Arthur P. F., Pryce J. E., Herd R. M. (2014). “Lessons learnt from 25 years of feed efficiency research in Australia,” in Proceedings of the 10th world congress of genetics applied to livestock production. (Champaign, United States: American Society of Animal Science (ASAS)) 18–22.
Berretta E. J., Bemhaja M. (1998). Producción estacional de comunidades naturales sobre suelos de Basalto en la Unidad Queguay Chico. INIA Serie Técnica 102, 11–20.
Berretta E. J., Risso D., Montossi F., Pigurina G. (2000). “Campos in Uruguay,” in Grassland ecophysiology and grazing ecology. Eds. Lemaire G., Hogdson J., de Moraes A., Nabinger C., Carvalho P. C. (CABI Publishing, Oxon, UK), 377–394.
Cammack K. M., Leymaster K., Jenkins T. G., Nielsen M. K. (2005). Estimates of genetic parameters for feed intake, feeding behavior, and daily gain in composite ram lambs. J. Anim. Sci. 83, 777–785. doi: 10.2527/2005.834777x
Cantalapiedra-Hijar G., Abo-Ismail M., Carstens G. E., Guan L. L., Hegarty R., Kenny D. A., et al. (2018). Review: Biological determinants of between-animal variation in feed efficiency of growing beef cattle. Animal 12, 321–335. doi: 10.1017/S1751731118001489
Cloete S. W. P., Cloete J. J. E. (2015). Production performance of Merino and Dohne Merino ewes and lambs in pure or crossbreeding systems. Proc. Assoc. Advmt. Anim. Breed. Genet. 21, 217–220. doi: 10.52404/21.217
Cloete S. W. P., Gilmour A. R., Olivier J. J., Van Wyk J. B. (2003). Age trends in economically important traits of Merino ewes subjected to 10 years of divergent selection for multiple rearing ability. South Afr. J. Anim. Sci. 33, 43–51. doi: 10.4314/sajas.v33i1.3737
Cockrum R. R., Stobart R. H., Lake S. L., Cammack K. M. (2013). Phenotypic variation in residual feed intake and performance traits in rams. Small Ruminant Res. 113, 313–322. doi: 10.1016/j.smallrumres.2013.05.001
Cottle D. J., Nolan J. V., Wiedermann S. G. (2011). Ruminant enteric methane mitigation: a review. Anim. Prod. Sci. 51, 491–514. doi: 10.1071/AN10163
De Barbieri I., Hegarty R. S., Li L., Oddy V. H. (2015). Association of wool growth with gut metabolism and anatomy in sheep. Livestock Sci. 173, 38–47. doi: 10.1016/j.livsci.2014.12.018
De Barbieri I., Navajas E. A., Giorello D., Velazco J. I., Banchero G., Rodríguez B., et al. (2020). “Association between feed efficiency and methane emissions, performance and health in Merino sheep,” in Book of Abstracts of the 71st Annual Meeting of the European Federation of Animal Science (EAAP) (Wageningen Academic Publishers, Wageningen (NLD), 560.
De Barbieri I., Viñoles C., Montossi F., Luzardo S., Ciappesoni G. (2021). Productive and reproductive consequences of crossbreeding Dohne Merino with Corriedale in Uruguayan sheep production systems. Anim. Prod. Sci. 62, 29–39. doi: 10.1071/AN20490
Dominik S., Swan A. A. (2016). Genetic and phenotypic parameters for reproduction, production and bodyweight traits in Australian fine-wool Merino sheep. Anim. Prod. Sci. 58, 207–212. doi: 10.1071/AN15738
Douhard F., Rupp R., Gilbert H. (2022). “Feed efficiency and resource allocation trade-offs: theory, evidence and prospects,” in Proceedings of 12th World Congress on Genetics Applied to Livestock Production (WCGALP) Technical and species orientated innovations in animal breeding, and contribution of genetics to solving societal challenges (Wageningen, Netherlands: Wageningen Academic Publishers), 264–267.
Faichney G. J. (2005). “Digesta flow,” in Quantitative aspects of ruminant digestion and metabolism. Eds. Dijkstra J., Forbes J., France J. (CABI Publishing, UK), 49–86.
Ferreira G. F., Ciappesoni G., Castells D., Navajas E. A., Giorello D., Banchero G., et al. (2021). Feed conversion efficiency in sheep genetically selected for resistance to gastrointestinal nematodes. Anim. Prod. Sci. 61, 754–760. doi: 10.1071/AN20121
Freer M., Dove H., Nolan J. V. (2007). Nutrient requirements of domesticated ruminants (Collingwood, Victoria, Australia: CSIRO Publishing), 269.
Geesink G. H., Zerby H. (2010). “Meat production,” in International Sheep and Wool Handbook. Ed. Cottle D. J. (Nottingham University Press, UK), 395–406.
Goering H. K., Van Soest P. J. (1970). Forage fiber analysis (apparatus, reagent procedures and some applications). ARS U.S. Dept. Agr Handbook N°. 379, Superintendent of Documents (Washington, D.C: U.S. Government Printing Office), 20402.
Goopy J., Robinson D. L., Woodgate R. T., Donaldson A. J., Oddy V. H., Vercoe P. E., et al. (2016). Estimates of repeatability and heritability of methane production in sheep using portable accumulation chambers. Anim. Prod. Sci. 56, 116–122. doi: 10.1071/AN13370
Goopy J. P., Woodgate R., Donaldson A., Robinson D. L., Hegarty R. S. (2011). Validation of a short-term methane measurement using portable static chambers to estimate daily methane production in sheep. Anim. Feed Sci. Technol. 166, 219–226. doi: 10.1016/j.anifeedsci.2011.04.012
Gurgeira D. N., Crisóstomo C., Sartori L. V. C., de Paz C. C. P., Delmilho G., Chay-Canul A. J., et al. (2022). Characteristics of growth, carcass and meat quality of sheep with different feed efficiency phenotypes. Meat Sci. 194, 108959. doi: 10.1016/j.meatsci.2022.108959
Hegarty R. S., Goopy J. P., Herd R. M., McCorkell B. (2007). Cattle selected for lower residual feed intake have reduced daily methane production. J. Anim. Sci. 85, 1479–1486. doi: 10.2527/jas.2006-236
Hendriks J., Scholtz M., Neser F. (2014). Possible reasons for differences in residual feed intake: An overview. South Afr. J. Anim. Sci. 43, 107–110. doi: 10.4314/sajas.v43i5.19
Henry B. K., Eckard R. J., Beauchemin K. A. (2018). Review: Adaptation of ruminant livestock production systems to climate changes. Animal 12, 445–456. doi: 10.1017/S1751731118001301
Herd R. M., Arthur P. F., Bottema C. D. K., Egarr A. R., Geesink G. H., Lines D. S., et al. (2018). Genetic divergence in residual feed intake affects growth, feed efficiency, carcass and meat quality characteristics of Angus steers in a large commercial feedlot. Anim. Prod. Sci. 58, 164–174. doi: 10.1071/AN13065
Huber K. (2017). Invited review: resource allocation mismatch as pathway to disproportionate growth in farm animals – prerequisite for a disturbed health. Animal 12, 528–536. doi: 10.1017/S1751731116002146
International Wool Textile Organisation (IWTO) (2006). IWTO-52: Conditioning procedures for testing textiles (Brussels: IWTO).
International Wool Textile Organisation (IWTO) (2012). IWTO-12: Measurement of the mean and distribution of fibre diameter using the Sirolan-Laserscan fibre diameter analyser (Brussels: IWTO).
Johnson P. L., Hickey S., Knowler K., Wing J., Bryson B., Hall M., et al. (2022). Genetic parameters for residual feed intake, methane emissions, and body composition in New Zealand maternal sheep. Front. Genet. 13, 911639. doi: 10.3389/fgene.2022.911639
Johnson K. A., Johnson D. E. (1995). Methane emissions from cattle. J. Anim. Sci. 73, 2483–2492. doi: 10.2527/1995.7382483x
Jonker A., Hickey S., McEwan J. C., Waghorn G. C. (2020). “Portable accumulation chambers for enteric methane determination in sheep,” in Guidelines for estimating methane emissions from individual ruminants using: GreenFeed, ‘sniffers’, hand-held laser detector and portable accumulation chambers. Eds. Jonker A., Waghorn G. (New Zealand Agricultural Greenhouse Gas Research Centre, New Zealand), 49–56.
Kenny D. A., Fitzsimons C., Waters S. M., McGee M. (2018). Invited review: Improving feed efficiency of beef cattle - The current state of the art and future challenges. Animal 12, 1815–1826. doi: 10.1017/S1751731118000976
Kenyon P. R., Maloney S. K., Blache D. (2014). Review of sheep body condition score in relation to production characteristics. New Z. J. Agric. Res. 57, 38–64. doi: 10.1080/00288233.2013.857698
Koch R. M., Swiger L. A., Chambers D., Gregory K. E. (1963). Efficiency of feed use in beef cattle. J. Anim. Sci. 22 (2), 486–494.
Kremer R., Barbato G., Rista L., Rosés L., Perdigón F. (2010). Reproduction rate, milk and wool production of Corriedale and East Friesian Corriedale F1 ewes grazing on natural pastures. Small Ruminant Res. 90, 27–33. doi: 10.1016/j.smallrumres.2009.12.009
Li L., Brown D. J., Gill J. S. (2013). Genetic parameters for body weight, carcass and wool traits in Dohne Merino. Proc. Assoc. Advmt. Anim. Breed. Genet. 20, 241–244. doi: 10.52404/20.241
Lima H. B., Costa R. G., Dias-Silva T. P., da Cruz G. R. B., de Carvalho F. F. R., Guerra R. R., et al. (2022). Performance and ruminal and intestinal morphometry of Santa Inês sheep submitted to feed restriction and refeeding. Trop. Anim. Health Prod. 54, 1–9. doi: 10.1007/s11250-022-03053-6
Lima Montelli N. L. L., de Almeida A. K., Ribeiro C., Grobe M. D., Abrantes M. A. F., Lemos G. S., et al. (2019). Performance, feeding behavior and digestibility of nutrients in lambs with divergent efficiency traits. Small Ruminant Res. 180, 50–56. doi: 10.1016/j.smallrumres.2019.07.016
Lines D. S., Pitchford W. S., Bottema C. D. K., Herd R. M., Oddy V. H. (2014). Selection for residual feed intake affects appetite and body composition rather than energetic efficiency. Anim. Prod. Sci. 58, 175–184. doi: 10.1071/AN13321
Marie-Etancelin C., Francois D., Weisbecker J. L., Marcon D., Moreno-Romieux C., Bouvier F., et al. (2019). Detailed genetic analysis of feeding behaviour in Romane lambs and links with residual feed intake. Anim. Breed. Genet. 136, 174–182. doi: 10.1111/jbg.2019.136.issue-3
Marques C. B., De Barbieri I., Velazco J., Navajas E. A., Ciappesoni G. (2022). “Genetic parameters for feed efficiency, gas emissions, oxygen consumption and wool traits in Australian Merino,” in Proceedings of the 12th WCGALP. Eds. Veerkamp R. F., de Haas Y. (Wageningen, Netherlands: Wageningen Academic Publishers), 160–163.
Masters D. G., Ferguson M. (2019). A review of the physiological changes associated with genetic improvement in clean fleece production. Small Ruminant Res. 170, 62–73. doi: 10.1016/j.smallrumres.2018.11.007
Meat and livestock Australia, Australian wool innovation (2008).Making more from sheep. Available online at: www.makingmorefromsheep.com.au/wean-more-lambs (Accessed 18/06/2024).
Muir S. K., Linden N., Kennedy A., Knight M. I., Paganoni B., Kearney G., et al. (2020). Correlations between feed intake, residual feed intake and methane emissions in Maternal Composite ewes at post weaning, hogget and adult ages. Small Ruminant Res. 192, 106241. doi: 10.1016/j.smallrumres.2020.106241
Navajas E. A., Ravagnolo O., De Barbieri I., Pravia M. I., Aguilar I., Lema M. O., et al. (2022). “Genetic selection of feed efficiency and methane emissions in sheep and cattle in Uruguay: progress and limitations,” in 12th World Congress on Genetics Applied to Livestock Production (WCGALP) Technical and species orientated innovations in animal breeding, and contribution of genetics to solving societal challenges. Eds. Veerkamp R. F., de Haas Y. (Wageningen Academic Publishers, The Netherlands), 164–167.
Nkrumah J. D., Okine E. K., Mathison G. W., Schmid K., Li C., Basarab J. A., et al. (2006). Relationships of feedlot feed efficiency, performance, and feeding behavior with metabolic rate, methane production, and energy partitioning in beef cattle. J. Anim. Sci. 84, 145–153. doi: 10.2527/2006.841145x
Oddy V. H., Sainz R. D. (2002). “Nutrition for sheep-meat production,” in Sheep nutrition. Eds. Freer M., Dove H. (Wallingford, United Kingdom: CABI publishing, Victoria, Australia: CSIRO), 237–262.
Osítis U., Strikauska S., Grundmane A. (2003). Lopbarības Analīžu Rezultātu Apkopojums. Apkopojums. LLU SIA Jelgavas tipogrāfija. 62, 1.
Paganoni B., Rose G., Macleay C., Jones C., Brown D. J., Kearney G., et al. (2017). More feed efficient sheep produce less methane and carbon dioxide when eating high-quality pellets. J. Anim. Sci. 95, 3839–3850. doi: 10.2527/jas.2017.1499
Pravia M. I., Navajas E. A., Aguilar I., Ravagnolo O. (2022). Evaluation of feed efficiency traits in different Hereford populations and their effect on variance component estimation. Anim. Prod. Sci. 62, 1652–1660. doi: 10.1071/AN21420
Ramos Z., Blair H., De Barbieri I., Ciappesoni G., Montossi F., Kenyon P. R. (2021). Productivity and reproductive performance of mixed-age ewes across 20 years of selection for ultrafine wool in Uruguay. Agriculture 11, 712. doi: 10.3390/agriculture11080712
Ramos Z., De Barbieri I., Van Lier E., Montossi F. (2019). Body and wool growth of lambs grazing on native pastures can be improved with energy and protein supplementation. Small Ruminant Res. 171, 92–98. doi: 10.1016/j.smallrumres.2018.11.009
Ramos Z., Garrick D. J., Blair H. T., De Barbieri I., Ciappesoni G., Montossi F., et al. (2023). Genetic and phenotypic relationships between ewe reproductive performance and wool and growth traits in Uruguayan Ultrafine Merino sheep. J. Anim. Sci. 101, skad071. doi: 10.1093/jas/skad071
Ransom K., Brien F., Pitchford W. (2015). A commercial comparison of ewe breeds for reproduction, wool and lamb growth. Proc. Assoc. Adv. Anim. Breed. Genet. 21, 286–289. doi: 10.52404/21.286
Rauw W. M. (2012). “Feed efficiency and animal robustness,” in Feed Efficiency in the Beef Industry. Ed. Hill R. (Hoboken, New Jersey, USA: John Wiley & Sons), 105–122.
Redden R. R., Surber L. M. M., Grove V., Kott R. W. (2013). Growth efficiency of ewe lambs classified into residual feed intake groups and pen fed a restricted amount of feed. Small Ruminant Res. 114, 214–219. doi: 10.1016/j.smallrumres.2013.07.002
Redden R. R., Surber L. M. M., Roeder B. L., Nichols B. M., Paterson J. A., Kott R. W. (2011). Residual feed efficiency established in a post-weaning growth test may not result in more efficient ewes on the range. Small Ruminant Res. 96, 155–159. doi: 10.1016/j.smallrumres.2010.12.007
Robinson D. L., Dominik S., Donaldson A. J., Oddy V. H. (2020). Repeatabilities, heritabilities and correlations of methane and feed intake of sheep in respiration and portable chambers. Anim. Prod. Sci. 60, 880–892. doi: 10.1071/AN18383
Robinson D. L., Goopy J. P., Hegarty R. S., Oddy V. H., Thompson A. N., Toovey A. F., et al. (2014). Genetic and environmental variation in methane emissions of sheep at pasture. J. Anim. Sci. 92, 4349–4363. doi: 10.2527/jas.2014-8042
Tortereau F., Marie-Etancelin C., Weisbecker J., Marcon D., Bouvier F., Moreno-Romieux C., et al. (2020). Genetic parameters for feed efficiency in Romane rams and responses to single-generation selection. Animal 14, 681–687. doi: 10.1017/S1751731119002544
Tulux Rocha R. F. A., Lopes Souza A. R. D., Da Graça Morais M., Yoshihara Carneiro M. M., Fernandes H. J., Dias Feijó G. L., et al. (2018). Performance, carcass traits, and non-carcass components of feedlot finished lambs from different residual feed intake classes. Semina: Cienc. Agrar. 39, 2645–2658. doi: 10.5433/1679-0359.2018v39n6p2645
Van Wyk J. B., Swanepoel J. W., Cloete S. W. P., Olivier J. J., Delport G. J. (2008). Across flock genetic parameter estimation for yearling body weight and fleece traits in the South African Dohne Merino population. South Afr. J. Anim. Sci. 38, 31–37. doi: 10.4314/sajas.v38i1.44876
Waghorn G. C., Hegarty R. S. (2011). Lowering ruminant methane emissions through improved feed conversion efficiency. Anim. Feed Sci. Technol. 166, 291–301. doi: 10.1016/j.anifeedsci.2011.04.019
Whitlock H. V. (1948). Some modifications of the McMaster helminth egg- counting technique and apparatus. J. Council Sci. Industr. Res. 21, 177–180.
Wuliji T., Dodds K. G., Land J. T. J., Andrews R. N., Turner P. R. (1999). Response to selection for ultrafine Merino sheep in New Zealand. Livestock Prod. Sci. 58, 33–44. doi: 10.1016/S0301-6226(98)00195-X
Yemm E. W., Willis A. J. (1954). The estimation of carbohydrates in plant extract by anthrone. Biochem. J. 57, 508. doi: 10.1042/bj0570508
Keywords: residual feed intake, fleece weight, fiber diameter, methane emissions, body condition score, body weight, gastrointestinal parasites resistance, weaning rate
Citation: De Barbieri I, Navajas EA, Ramos Z, Ferreira G, Velazco J and Ciappesoni G (2024) Feed conversion efficiency does not negatively affect young sheep and ewe performance. Front. Anim. Sci. 5:1480928. doi: 10.3389/fanim.2024.1480928
Received: 14 August 2024; Accepted: 20 November 2024;
Published: 20 December 2024.
Edited by:
Karl-Heinz Südekum, University of Bonn, GermanyReviewed by:
Georg Terler, Agricultural Research and Education Center (AREC) Raumberg-Gumpenstein, AustriaGerhard Bellof, Weihenstephan-Triesdorf University of Applied Sciences, Germany
Copyright © 2024 De Barbieri, Navajas, Ramos, Ferreira, Velazco and Ciappesoni. This is an open-access article distributed under the terms of the Creative Commons Attribution License (CC BY). The use, distribution or reproduction in other forums is permitted, provided the original author(s) and the copyright owner(s) are credited and that the original publication in this journal is cited, in accordance with accepted academic practice. No use, distribution or reproduction is permitted which does not comply with these terms.
*Correspondence: Ignacio De Barbieri, aWRlYmFyYmllcmlAaW5pYS5vcmcudXk=