- 1Elanco Deutschland GmbH, Bad Homburg, Germany
- 2Institute for Animal Hygiene, Animal Welfare and Farm Animal Behavior, University of Veterinary Medicine Hannover, Foundation, Hannover, Germany
Hygienically safe drinking water is essential for the health, well-being, and efficient production of livestock. In contrast to drinking water for human consumption, there are guidelines but no legal limits for drinking water for animals. Risk factors due to hygienically relevant microorganisms or chemical compounds in drinking water are known from literature, but there is little information on the quality of drinking water for livestock in Germany. To gain an overview of the quality of drinking water, data from samples collected from pig and poultry farms in Northwest Germany over a 10-year period were analyzed retrospectively. Data consisted of 326 samples analyzed chemically, and 519 samples analyzed microbiologically. Of these, 80 water samples were analyzed for both chemical and microbial contaminants. There was no correlation between samples exceeding chemical and microbial guideline levels. The number of samples exceeding the chemical guideline values (58.0%) was higher than the number of samples exceeding the microbiological guideline values (47.4%). This long-term study illustrated the potential risk to pigs and poultry from drinking water and highlights the need for effective hygiene measures to prevent the transmission of microorganisms and chemicals residues through water to maintain animal health and safe food products.
1 Introduction
Not only the quantity provided, but also the quality of drinking water plays a key role in maintaining health, welfare, and productivity of livestock. Given the large volumes consumed, poor water quality can potentially pose a risk to animal health. Inadequate drinking water can have a significant impact on livestock production, affecting factors such as feed conversion and growth rate (Praveen et al., 2016). It can also contribute to a variety of infectious and non-infectious diseases (Umar et al., 2014). Clean water in a good microbiological and chemical quality is therefore a key element in reducing the use of antibiotics. The European Green Deal, for instance, targets a 50% reduction in antibiotic use from 2018 to 2030, aligning with the United Nations’ Sustainable Development Goal 3 (Good Health and Well-being) (European Commission, 2019; United Nations, 2015). Hence, the supply of clean water is essential to ensure animal health, avoid economic losses, and support sustainable and responsible resource use.
Currently, the German regulation of drinking water in livestock falls under the Feed Hygiene Regulation (European Parliament, 2005), unlike water for human consumption, which is covered by a specific separate regulation. The regulation related to livestock only states that drinking water must be suitable for the animals and that if contamination risks are suspected, measures should be taken to assess and reduce these risks. For these measures to be implemented effectively, it is necessary to identify potential risks, even though these risks related to the quality of drinking water are not specifically outlined. However, German guidelines do exist for certain water parameters. The Federal Ministry of Food, Agriculture and Consumer Protection (BMEL) published limit values for chemical and microbiological parameters even back in 2007 (BMEL, 2007), based on scientific recommendations (Kamphues et al., 2007). While these guidelines are not mandatory, they serve as a guideline to be considered in farm animal husbandry. The importance of water quality for animal health and welfare has been widely recognized in recent years and is, for instance, addressed in the German retailers’ initiative, “Initiative Animal Welfare” (ITW, 2015), where it is stated that water needs to be tested annually for included farms. These mandatory drinking water checks include physiochemical and microbiological analyses, which also apply to the ministerial guidelines mentioned above (ITW, 2015).
Moreover, water quality is essential not just for the well-being of animals, but also for the effective administration and dilution of substances potentially applied for animal health reasons. Water is not only used as drinking water for livestock, but also as a transport medium for medicine, vaccines, or detergents. Since the German Guideline on Oral Use of Veterinary Medicinal Products in the Livestock Sector via Feed or Water was implemented in May 2014, the need for practical management of drinking water has been increasing (BMEL, 2014). The guidelines state that veterinarians can administer oral antimicrobial formulations only after they have developed and documented a farm-specific risk management for oral medication (BMEL, 2014). Additionally, the obligatory waiting period for treated animals only applies after the system has been cleaned (BMEL, 2014). Furthermore, it is important that the water pipes are dimensionally designed and installed to prevent biofilm by providing a sufficient flow rate and properly located pipes. Recommendations on drinking water quality have also been adopted at the European level. For example, the European Medicines Agency (EMA), which is responsible for the evaluation and supervision of medicines, has discussed this subject. In August 2020, the EMA published a scientific problem analysis and recommendations to ensure the safe and the efficient administration of oral veterinary medicinal products via routes other than medicated feed (European Medicines Agency, 2020). It states that the management of water quality and knowledge of its physio-chemical and microbiological properties are crucial for correct medication. Drinking systems used for oral administration of veterinary medicines should be cleaned after each treatment with appropriate products and methods. In addition, the distribution system on the farm must ensure that medicines in feed or water are delivered only to the intended animals. An understanding of the importance of good water quality, for both the benefit of animal health and the application of substances, is present already. However, water quality can be impaired, and several risks, which may be chemical, microbiological, or technical, can occur.
Concerning microbiological risks, biofilms can form on surfaces in contact with water (Donlan, 2000). Microbial biofilm formation often occurs in drinking water systems (Flemming and Wingender, 2001). If the supply of nutrients is kept to a minimum, they are usually very thin and can be tolerated, as is the case in drinking water systems for human consumption. However, if conditions are favorable for bacterial growth, massive biofilm development can occur in drinking water systems in livestock housing. A biofilm refers to the community of microorganisms on the surface. It is known that biofilms can be a reservoir for pathogens (e.g., bacteria, viruses, parasites) (Costerton et al., 1999; Wingender and Flemming, 2011). This way, pathogens can be discharged from biofilms at irregular intervals and quantities and contaminate the water. Various factors contribute to biofilm formation and an increased risk of contamination: The water itself may already be initially contaminated (e.g., in the case of well or surface water). The main problem in livestock farming, however, is the posterior entry of germs from the drinking trough into the drinking water supply system. The presence and spread of animal and zoonotic pathogens in drinking water and its systems represent a significant source of pathogen exposure for animals. However, this topic seems to be relatively unexplored.
The major bacterial agents that have been shown to cause human intestinal illness associated with drinking water are Salmonella, Shigella, Vibrio, and Escherichia (E.) coli (João and Cabral, 2010). Bacterial and protozoan pathogens also pose a threat to livestock by contaminating water supplies (Hooda et al., 2000). To evaluate microbiological water quality, fecal indicator bacteria are used to detect the presence of fecal contamination in a water source. These bacteria are likely to be present along with other enteric pathogens. Total coliform, fecal coliform, E. coli, and fecal Streptococci are currently used to assess contamination in water quality management because they are easy and inexpensive to detect compared to other pathogens (Meays et al., 2004). Nonetheless, this is only a guideline, and no currently used bacterial indicator can meet all the criteria required to indicate ideal water quality (Devane et al., 2020). In addition, total bacteria counts can provide information about contamination of water sources or indicate biofilm growth (Wingender and Flemming, 2011).
Referring to various chemical risks, drinking water can be contaminated with heavy metals, pesticides, herbicides, industrial chemicals, pharmaceuticals, and microplastics. However, substances naturally present in water may also pose a risk (Carson, 2000). The most common minerals dissolved in drinking water are the cations calcium (Ca2+), magnesium (Mg2+) and sodium (Na+), and the anions carbonate (CO32−), bicarbonate (HCO3−), chloride (Cl−), and sulfate (SO42−). Therefore, various physio-chemical or chemical parameters of drinking water should be tested regularly, especially in the case of well water. These include, but are not limited to, pH, conductivity, salinity, nitrate and nitrite contents, and various inorganic and organic ingredients (Früchtenicht, 2000).
In addition to the composition and additives of the water, the pipe material is also a factor in the formation of deposits or biofilm. This is because bacterial growth can differ depending on the material (Kilb et al., 2003). For example, new plastic pipes can release substances that act as nutrients for bacteria, encouraging the formation of bacterial biofilms from the organic components of pipe coatings (Vogel et al., 2020). The risk is further increased when flow shadows, stagnant water left for several hours, or low flow velocities (<1 m/s) occur in the pipe system (Flemming et al., 2014). Thus, a technical risk may be the use of certain materials for pipes that promote biofilm growth or the installation itself due to stagnant water, for instance, in dead-end systems, or low flow rates.
Risk factors associated with chemical compounds (e.g., nitrate, sulfate) or hygienically relevant microorganisms (e.g., E. coli) in drinking water for animals were described in the literature (Costerton et al., 1999; Carson, 2000; Morgan, 2011; Genther and Beede, 2012; Anderson, 1978; Veenhuizen et al., 1992). However, little information is available on the drinking water quality of German livestock farms. This study aimed to retrospectively assess the state of drinking water quality on German pig and poultry farms by analyzing data collected over a 10 year period. Existing data were examined to identify trends and potential hazards related to drinking water.
2 Materials and methods
The drinking water samples included in this study were taken from pig and poultry farms in the Northwestern part of Germany, a region of intensive livestock farming and high livestock density. The data were collected over a 10-year period (03/2004 to 12/2014) and the farms were randomly selected. The primary focus was to improve on-farm hygiene management. Participation was voluntary and water sampling was offered as an additional service. Chemical and microbiological water quality parameters were analyzed using standard methods and the data were examined retrospectively. A total of 326 samples were analyzed chemically and 519 samples were analyzed microbiologically.
2.1 General information of farms
Farm information was collected through face-to-face interviews conducted by the person collecting the water samples. Topics included general farm information, data on water resources used for livestock consumption, and the location of the farm.
2.2 Chemical analysis
A water sample was collected for chemical analysis if the animals were fed well water. In the case of tap water, no sample was taken for chemical analysis. Water samples were collected in clean 250 mL PE bottles (H.H. Rotert GmbH & Co. KG, Bad Iburg, Germany) at the beginning of the pipeline. The water was carefully poured into the container to avoid oxidation. To further avoid oxidation of substances in the water during the transport the container was filled to the top. No cooling was used during transport of samples within 48 hours, but care was taken to avoid large temperature changes.
The physical characteristics such as the pH value and hardness were examined according to DIN 38404-5 (1984) and DIN 38406-3-3 (2002), respectively. The dissolved chloride, nitrite, nitrate, and sulfate ions were determined according to DIN EN ISO 10304-1 (2009). The cation ammonium was analyzed according to DIN 38406-5 (1983). To measure manganese and iron an inductively coupled plasma-mass spectrometry was used according to DIN EN ISO 17294-1 (2004). The result was expressed in mg based on 1 mL of water.
2.3 Bacteriological analysis
A sterile 250 mL container with sodium thiosulfate as stabilizer (H.H. Rotert GmbH & Co. KG, Bad Iburg, Germany) was used to collect water samples for microbiological analysis. Sampling was carried out in the middle or at the end of the pipe, with no prolonged running of the water, to give realistic results of what the animals ingest. The barn was in operation at the time of sampling. A fresh pair of sterile nitrile gloves (Anhui Intco Medical Products Co., Ltd., Huaibei, China) was used for each individual sample collection to prevent potential cross-contamination from the environment. After collecting the water samples the container was quickly and hygienically sealed with a lid. The samples were then transported to the laboratory under cool conditions within 24 hours.
Microbial organisms were measured as Colony-Forming Units (CFU) using a plate count method (DIN EN ISO 6222, 1999). The CFU is a key figure or unit in microbiology and a measure of the microbiological load of a medium, such as drinking water. The water samples were initially diluted in a standard dilution series to 1:10 and 1:100 with distilled water. In the case of very high bacterial counts, it was sometimes necessary to use higher dilution steps of up to 1:10,000. From the dilution steps prepared, duplicates were pipetted on nonselective plate count agar (Merck, Darmstadt, Germany) according to German standards (Ordinance on the Quality of Water Intended for Human Consumption; Trinkwasserverordnung, 1990). The agar plates were incubated at 22° ± 2°C and 36° ± 1°C in an incubator for 44 ± 4 hours.
From plates, all visible colonies were counted as viable bacterial counts in CFU. The result was expressed as CFU/mL, based on 1 mL of the water sample used.
The detection of Escherichia coli (E. coli) was performed by membrane filtration according to DIN EN ISO 9308-1 (2000). After membrane filtration, the filter was applied to selective Chromogenic Coliform Agar (Merck Millipore, Darmstadt, Germany). Incubation was then performed at 36 ± 2°C for 21 ± 3 hours. The result was expressed as CFU/mL, based on 10 mL of the water sample used.
2.4 Evaluation of data
The aim of the study was to contribute to a better understanding of the drinking water quality for livestock. The results were then compared with the recommendations of the German Federal Ministry of Food, Agriculture and Consumer Protection (BMEL) for the assessment of chemical (Table 1) and microbiological (Table 2) drinking water quality.
2.5 Statistical analysis
The samples tested were categorized according to whether they exceeded the recommended limits for use in livestock. The Pearson chi-squared test was used to determine whether there was a correlation between two categorical variables. All statistical analyses were performed using JMP® 16 (Statistical DiscoveryTM from SAS, Marlow, UK).
3 Results
The analysis of water samples over a period of 10 years (03/2004–12/2014) provided an overview of chemical and microbial contamination in drinking water for livestock.
A total of 326 samples were analyzed chemically and 519 samples were analyzed microbiologically. Water samples were taken throughout the year in spring (n = 200), summer (n = 280), fall (n = 191) and winter (n = 174).
3.1 General information of farms
All farms were located in the Northwestern part of Germany (Lower Saxony and North Rhine-Westphalia). Among the participants, 61.1% (n = 317) were involved in swine farming (fattening pigs and sows) and 38.9% (n = 202) engaged in poultry farming (broiler). Of these, a total of 326 (62.8%) used well water and 193 (37.2%) tap water. In total, 36.6% (n = 74) of poultry farmers and 79.7% (n = 251) of pig farmers used well water. All farms were conventionally managed. In general, farm managers felt the need to improve animal health in the barn and were open to improving drinking water management. The motivation for participating in the study was to solve barn problems or improve overall animal performance.
3.2 Results of chemical analysis
Results of pH, hardness, ammonium, chloride, iron, manganese, nitrate, nitrite, and sulfate values from laboratory analysis of water samples were compared to recommended limit values from the BMEL (BMEL, 2007). Average values, maximum, minimum, and the percentage of unsuitable samples are shown in Table 3.
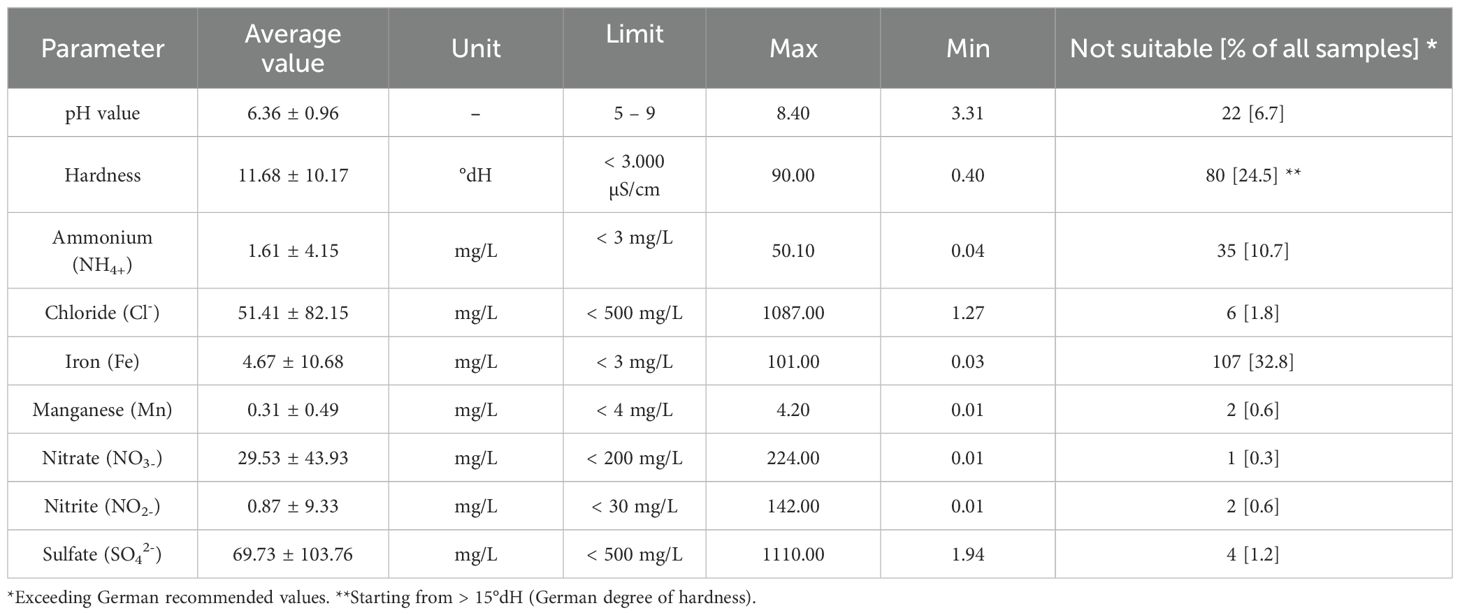
Table 3. Results of chemical analysis of water samples (n = 326) from German pig and poultry farms and comparison with German limit values.
The percentage of water samples exceeding the chemical recommended values were similar for the poultry farms and pig farms. In total, 55.6% (140/252) of samples taken from pig farms and 51.4% (38/74) of samples taken from poultry farms exceeded limit values for chemical agents. More samples from pig farms (36.5%) were higher in Iron compared to poultry farms (21.6%). In contrast, the German degree of hardness (> 15°dh) was increased more often in water from poultry farms (27.0%) than for pig farms (19.4%).
3.3 Results of the microbiological analysis
Results of microbiological values (CFU at 20°C; CFU at 36°C, E. coli) from laboratory analysis of water samples were compared to recommended limit values from the BMEL (2007). Average values, maximum, minimum, and the percentage of unsuitable samples are shown in Table 4.

Table 4. Results of microbiological analysis of water samples (n = 519) from German pig and poultry farms and comparison with German limit values.
The proportion of water samples exceeding microbiological limits was similar for poultry and pig farms. In total, 49.5% (157/317) of the samples from pig farms and 42.6% (86/202) of the samples from poultry farms exceeded the limit values.
In total, a higher proportion of samples were found to be non-compliant with chemical guidelines (58.0%) compared to microbiological guidelines (47.4%).
Values exceeding the limits for 20°C were similar in spring (28/110; 2.5%), summer (54/177; 3.1%), fall (34/121; 2.8%) and winter (40/3.6%). There were slightly more samples exceeding the microbiological limit values at 36°C in winter (59/111; 53.3%) compared to spring (42/110; 38.2%), summer (78/177; 44.1%), and fall (65/121; 46.3%). In summer E. Coli was detected more frequently (13/177; 7.3%) compared to spring (2/110; 1.8%), fall (4/121; 3.3%) and winter (3/111; 2.7%).
3.4 Results of statistical analysis
A total of 80 water samples were analyzed simultaneously for chemical and microbiological contaminants. According to the Chi-Square test, there was no correlation (p = 0.5269) between water samples exceeding chemical and microbial guideline levels.
There was also no significant association between the season and elevated microbiological (p = 0.2535) or chemical (p = 0.0882) levels. There was a trend that E. coli was detected more frequently in summer. However, this was not significant (p = 0.1648).
4 Discussion
Providing drinking water in adequate quantity and quality is as important as providing energy and essential nutrients through feeding. This is mandatory to maintain animal health and performance; as well as food quality and food safety. Water availability and water quality are essential for animal health in any case, but are particularly important for livestock produced under intensive conditions. The interaction between drinking water contaminants and suboptimal nutritional status in farm animals has been found to have significant implications for performance and immune function (Vodela et al., 1997). Water plays a critical role in many physiological functions (Gonyou, 1996). A lack of water can cause serious welfare problems as it can disrupt biological functions and has been linked to disease and, in cases of extreme deprivation, even death (von Keyserlingk et al., 2016). Field experience has further demonstrated that variations in water quality, both between different farms and even within a single complex from one well to another, can lead to noticeable differences in animal performance, behavior, and welfare (King, 1996; Zimmermann and Douglass, 1998). Another reason to prioritize basic hygiene and ensure the quality of water is the administration of substances (e.g., vaccines, antibiotics) through drinking water. Water serves as a medium for delivering various products and should be free of any substances that may interfere with their effectiveness. It has been observed that poor water quality can potentially reduce the effectiveness of vaccines and medication administered through water lines (van der Sluis, 2002). Therefore, livestock farmers are responsible for ensuring that their animals have access to sufficient water of good quality at all times. The existing reference values of the Drinking Water Ordinance or the Federal Ministry of Food and Agriculture can be used to assess water quality (BMEL, 2007; Kamphues et al., 2007). However, these values are not legally binding for drinking water for animals.
Based on the legal framework, this study describes the quality of drinking water for German pigs and poultry retrospectively, considering both biological and physio-chemical contaminants as criteria. In total, a higher proportion of samples were found to be non-compliant with chemical guidelines (58.0%) compared to microbiological guidelines (47.4%). This indicates a greater need to track and address chemical contaminants within the samples tested, as they tend to exceed recommended limits more frequently than microbiological contaminants. A total of 80 water samples were tested simultaneously for chemical and microbiological contaminants. However, there was no correlation between water samples exceeding chemical and microbial guideline levels, indicating that chemical constituents do not necessarily increase the risk of microbial contamination. This could, moreover, indicate the need for more sophisticated analytical methods or a deeper investigation into potential interactions between these contaminants. Nevertheless, the substantial number of unsuitable water samples underlines the fundamental need to improve the overall quality of well water. After all, water is one of the most important and often underestimated nutrients.
The main reason for non-compliance of drinking water based on the chemical parameters was iron (Fe). This is not surprising, as iron is one of the most common metals in the Earth’s crust. In the analyzed water samples, iron levels ranged from 0.03 to 101 mg/L, with 32.8% (107/326) exceeding the guidance value (< 3 mg/L) set by the Federal Ministry. According to the limits for human consumption (< 0.2 mg/L), as many as 59.2% (193/326) of the water samples were unsuitable for drinking. It is known that higher levels of iron in drinking water can affect acceptance and thus reduce water intake in livestock (Genther and Beede, 2012). In addition, iron has been reported to support the growth of E. coli and coliforms (Grandjean et al., 2006; Wingender and Flemming, 2011; van Eenige et al., 2013). However, only a few studies have been published, so it is difficult to make reliable statements about the influence of iron in drinking water on the behavior and performance of different livestock. Nevertheless, it has been found that metallic water can impair the effectiveness of drugs (e.g., tetracyclines) (Goronczy and Piontkowski, 1999; Ungemach, 2003). In addition, water constituents may reduce the effectiveness of vaccines administered through water pipes (van der Sluis, 2002). In any case, a high iron content in the water will certainly cause abrasion in the pipes. In the case of heavy deposits (incrustations), hydraulic performance is no longer guaranteed. The required amount of water may not be able to flow through the pipe system. In addition, heavy metal deposits in the water are often impossible to remove and replacement of the pipe sections is mandatory. There are many techniques for removing iron from drinking water. These include ion exchange and water softening, the use of activated carbon and other filtration materials, supercritical fluid extraction, bioremediation, and limestone treatment (Andersen and Bruno, 2003; Aziz et al., 2004; Berbenni et al., 2000; Munter et al., 2005; Vaaramaa and Lehto, 2003). Other methods include oxidation by aeration, chlorination, or ozonation, followed by filtration, or using ash, aerated granular filters, or adsorption (Cho, 2005; Das et al., 2006; Ellis et al., 2000; Tahir and Rauf, 2004). Aeration and separation are the most used methods for iron removal in public water systems (Ghosh et al., 2008). Based on the findings of several farms that used well water for their livestock in this study, it is recommended that water treatment systems be installed upstream. This helps to remove chemicals from the drinking water. However, de-ironing may not be applicable to all types of well water. Therefore, it is advisable to take a sample of the water first and consult with the different suppliers available on the market.
Manganese (Mn), another common metallic element, is typically found in air, soil, food, and water. Although is a vital nutrient, excessive levels can disrupt the normal functioning of the nervous system (Avila et al., 2013). In occupational settings, long-term exposure to manganese has been identified as a potent neurotoxicant, capable of causing motor and cognitive deficits and neuropsychiatric symptoms in humans (Rodier, 1955). More recent studies have investigated the health hazards associated with environmental exposure to this metal, suggesting potential psychological and neurological abnormalities (O’Neal and Zheng, 2015). Children are thought to be particularly vulnerable due to the ongoing development of their nervous system, a higher exposure dose relative to body weight, and their immature homeostatic mechanisms (Zoni et al., 2007). There are numerous areas in North America and other parts of the world where water contains high levels of manganese (Frisbie et al., 2012). Groundwater can naturally contain high levels of manganese due to the erosion and seepage of manganese-containing minerals. Concerns about excessive manganese in the water were often expressed during the interviews. However, only twice (0.6%) the limit value for livestock was exceeded. It is noteworthy that according to the limit values for human consumption, only 23.9% (78/326) would have been suitable for human drinking water according to the limits for human consumption. Like iron, manganese can cause deposits and interfere with the effectiveness of active ingredients. Therefore, water treatment systems may also be appropriate for manganese, even if the water is within the range of values suitable for livestock.
Another parameter that was often mentioned causing problems due to clogged spray cooling systems or deposits on equipment (e.g., for vaccination or cleaning equipment) was water hardness. Water hardness is the sum of calcium and magnesium. Calcium and magnesium ions have a great positive physiological significance, but they interfere with some water applications (Denton et al., 1980). It is divided into soft (< 8.4°dH (German degree of water hardness), medium (8.4 to 14°dH) and hard (> 14°dH) ranges. Although there are no exact guideline values for water hardness, a high content of carbonate compounds can lead to calcification of pipes and drinking equipment (filters, suppliers), so hard water should be avoided (Kamphues et al., 2007). In the present study, 24.5% (80/326) of the water samples analyzed had a hardness > 15°dH, indicating calcium carbonate (CaCO3) in the water above 2.5 mmol per liter. There appears to be no reliable study on water hardness and its effects on animal health. In contrary, several epidemiologic studies have shown an association between the risk of cardio-vascular disease, growth disorders, reproductive dysfunction, and other health problems in humans and the hardness of drinking water or its magnesium and calcium content (Sengupta, 2013). However, the tendency to form insoluble compounds is the main reason why water hardness is considered an important quality characteristic. Hard water can lead to calcification, which promotes the formation of biofilms and can reduce the cleaning power of certain products (Abeliotis et al., 2014). In addition, it decreases the effectiveness of enrofloxacin treatment in livestock (Sumano et al., 2004). Therefore, hard water may not harm animals directly, but can have an indirect effect on animal health. Therefore, if water hardness is present (> 14°dH), softening can not only extend the life of the pipe system, but also indirectly maintain animal health. Water softening primarily involves chemical precipitation and ion exchange (Ahmed et al., 1998). In chemical precipitation, alkaline additives raise the pH of the water and convert bicarbonates to carbonates. However, this cannot completely remove water hardness due to the limited solubility of calcium and magnesium carbonates (Ahmed et al., 1998; Rengaraj and Moon, 2002; Bailey et al., 1999). Ion exchange systems, commonly used in commercial and industrial applications, remove ionic contaminants. They typically contain strong acid sulfonic acid or weak acid carboxylic acid functionalities (Ahmadpari et al., 2022). Ion exchange is cost effective when using inexpensive exchangers such as zeolites (Townsend and Coker, 2001). The promotion of softened drinking water is even expected to reduce the consumption of detergents, soap, cleaning agents and even energy (Godskesen et al., 2012). Therefore, if hard water is present, water softening may not only inhibit calcification, but may also be sufficient for animal health and performance as well as being economically advantageous.
Nitrate (NO3−) and nitrite (NO2−), which naturally occur in water, are oxidized forms of nitrogen. In regions with intensive agriculture, N-compounds are often introduced in the form of nitrate (Giammarin and Quatto, 2015). If there is a possible parallel bacterial contamination of the water the nitrate can also be converted into nitrite (= reduction) (Shukla et al., 2021). Excessive levels of nitrates can lead to environmental pollution of water bodies and pose a risk to human health if the polluted water is used for drinking. Therefore, the Council Directive 91/676/EEC concerning the protection of water against pollution caused by nitrates from agricultural sources aims to reduce nitrogen pollution from livestock in Europe to protect water bodies from nitrate pollution and to safeguard human health (European Commission, 1991). It focuses on the impact of cattle, sheep, pigs, and poultry on territorial loads. Previous studies have shown that an increased nitrate concentration in drinking water has no harmful effects on the health of weaner pigs (Anderson, 1978). However, nitrite in drinking water significantly reduced their average daily weight gain and feed consumption (Veenhuizen et al., 1992). In this study, nitrate ranged from 0.01 to 224.00 mg/L and nitrite from 0.01 to 142.00 mg/L. There were few water samples that exceeded the limits set for livestock water. Thus, according to our results, excessive nitrate and nitrite levels in drinking water seem to be rather rare at 0.3% (1/326) and 0.6% (2/326), respectively. Nonetheless, if the values set for human consumption (< 50 mg/L) are considered, significantly more water samples (17.8%; 58/326) exceed the limits for nitrate. While high levels of nitrates and nitrites in drinking water are rare for livestock, stricter monitoring and control measures are necessary when considering human consumption standards. This is particularly true for well water that may also be used for domestic purposes, indicating the need for regular testing.
Sulfate (SO42−) can be found in the environment due to atmospheric and terrestrial processes. Increasing levels of sulfate in surface water, a byproduct of industrial growth, is a growing concern worldwide (Moreno et al., 2009). This is mainly because of the effect of sulfates on the taste and odor of water as sulfates give water an unpleasant taste. The recommended maximum sulfate content in drinking water for farm animals is set at 500 mg/L (Kamphues et al., 2007). With 1.94–1,110 mg/L sulfate, only 1.2% (4/326) of the water samples analyzed were not within the recommended values for Germany. Sulfates cause non-pathogenic (osmotic) diarrhea and are a major cause of poor water quality in some regions (e.g., North America) (Gomez et al., 1995). For Northwest Germany, the results suggest that high sulfate levels in drinking water are a less common cause of poor water quality. Furthermore, there is no evidence for a negative influence of high sulfate levels on the health of pigs. Sulfate concentrations in water of 400 mg/L do taste bitter to humans and have a diarrheal effect (Chien et al., 1968). However, it has been reported that humans have adapted to this taste and that water consumption can increase over time at high sulfate concentrations (Chien et al., 1968). In pigs, a concentration of 1200 mg/L sodium sulfate per liquid feed had no effect on feed intake (Gomez et al., 1995). In addition, studies have shown that neither growth nor daily gains are affected when pigs ingest high amounts of sulfate via the drinking water (Veenhuizen et al., 1992; Tremblay et al., 1989). Nevertheless, higher SO42− concentrations in drinking water can influence the fecal condition of calves and pigs (from approx. 500–600 mg SO42−/L) (Kamphues et al., 2007). Even higher SO42− levels (e.g., > 2,500 mg SO42−/L) can even lead to severe clinical symptoms in cattle (Stöber and Scholz, 2002). In short, increased sulfate levels in the tested water are rather rare, although it must be decided on a case-by-case basis whether the water is suitable for the animal species. It should certainly be taken into consideration if, for instance, in pig farming there are different locations, and the piglets are moved to a barn with a different water supply that has a higher sulfate content. In this case, water intake could be affected.
The pH (pondus hydrogenii) is a measure used to determine the amount of hydrogen ions present in a given medium, indicating whether it is acidic, neutral or alkaline. In relation to water, which has a pH scale of 1 to 14, the pH indicates its degree of acidity or alkalinity. However, it should not have a major impact on water acceptability. In general, drinking water with a pH value of 6–9 is considered acceptable for livestock (Kamphues et al., 2007). The results of the long-term study show that 6.7% (22/326) of the analyzed water samples exceeded the recommended pH values for livestock. Although the exact effects of pH on water intake or animal health are unknown, the influence of pH on the solubility and stability of pharmaceuticals has been demonstrated (Dorr et al., 2009; Kotb et al., 2019). In addition, high or low pH values can significantly affect the efficiency of certain water treatment systems, such as chlorine with an optimal pH of 5–6 (Keswick et al., 1978). Exemplarily, it was demonstrated that water with a pH over 8.5 lowers disinfectant capability of chlorine (Nakagawara et al., 1998). As a result, it may not work optimally as a disinfectant in 93.3% (304/326) of the water samples analyzed. This is a surprisingly high number and indicates that the pH value is usually underestimated. According to a previous study, the pH of rainwater and reservoir water shows a significant seasonal trend; the average pH was 5.7 in spring, 5.8 in summer, 4.6 in fall and 4.5 in winter (Lee et al., 2010). This was not found in the well water tested in this study. There were similar pH values of 6 in spring, summer, and winter as well as of 7 in fall without significant differences. It is known that extreme pH values can lead to corrosion in the pipe system (Kamphues et al., 2007). However, the negative effect on substances administered via drinking water can be significantly influenced, which is a far greater reason to check the pH value and keep it at a level of 5–8.
The total bacterial count in the water provides information about the general microbiological status of the water. As different bacteria grow differently at different temperatures, the total bacterial count in the water is determined at both 20°C and 36°C. According to the German guideline values, the total bacterial count in drinking water should not exceed 10,000 CFU at 20°C and no more than 1,000 CFU at 36°C (Kamphues et al., 2007). An increased colony count at 20°C indicates common germs in the water that are potentially less harmful. An increased colony count at 36°C is more problematic because it corresponds to the body temperature of mammals. Overall, 30.3% of the water samples examined exceeded the total bacterial count at 20°C and 44.9% exceeded the total bacterial count at 36°C. High microbiological levels may indicate poor water management, which can lead to excessive biofilm growth. This could also be due to animal contamination or sampling error. If this were the case, higher bacterial counts and less conclusive drinking study results would be expected. However, in this study, the majority of water samples – more than half – were found to be within acceptable limits. This suggests that the sampling procedures were performed correctly. The primary goal of these tests was to evaluate the quality of the water ingested by the animals and to improve hygiene management. This study was conducted retrospectively, which is generally a faster and less expensive approach as it uses existing data. Nevertheless, it is important to note that this method also has its limitations. Participation was voluntary, and water sampling was offered as an extra service. This could introduce bias into the study, because these farms may have had a pre-existing interest in water quality issues. As drinking water guidelines were already published in 2007 (BMEL, 2007), the attitude of farmers may have changed and drinking water management has been adapted. However, the data for the period after 2007 did not show any significant improvement in drinking water quality. In addition, the quality of a retrospective study depends heavily on how accurately and reliably the original data were collected. Relevant data for potential factors influencing the results (e.g., material, temperature) cannot be documented afterwards. In general, a high microbiological load in the water is an indication of contamination in the pipe system. Thus, the results can still provide valuable information about the quality of drinking water for animals. It is likely that the high microbial levels in the barns reflect the presence of biofilms, which are gel-like layers of polymeric matrix that harbor microorganisms (e.g., bacteria, yeasts, protozoa) and can be generated on solid surfaces (Wingender and Flemming, 2011). In this layer, microorganisms are very well protected from environmental influences. It is assumed that biofilms are a habitat for 90% of all bacteria and provide an optimal habitat for pathogenic microorganisms such as bacteria, viruses, and parasitic protozoa (Wingender and Flemming, 2011; Lumb et al., 2017). Furthermore, biofilms can also act as a sorption matrix that soaks up substances from the water, which can accumulate in the biofilm and remain within the biofilm for long periods (Flemming and Leis, 2002). Hygienically relevant microorganisms can colonize pre-existing biofilms and serve as a source of water contaminants that pose a potential health risk to animals (Jacques et al., 2010; Wan et al., 2021). While this study provides valuable insights into water quality for poultry and pigs, it is important to note that water quality can evolve with husbandry and management practices. Therefore, further research comparing these results with more recent water quality data is recommended. However, recent studies have shown that a significant number of poultry farms in Austria and Mozambique have high microbial loads in drinking water (Augusto et al., 2022; Mustedanagic et al., 2023). This finding is in line with the present study and highlights the need for ongoing monitoring and research into livestock drinking water quality to ensure efficient water line management.Escherichia coli is a widely studied indicator of fecal contamination. The recommended limit for this bacterium in animal drinking water is no more than 10 CFU in a 10 mL sample. In our study, we found that 4.2% (22/519) of the water samples exceeded this guideline. This was most prevalent in the summer, with 7% (13/177) of the samples exceeding the limit. This suggests that there is a potential for drinking water contamination, particularly during the warmer months, and indicates the need for water quality monitoring during this time. In contrast, the total bacterial count at 20°C and 36°C tended to be observed more frequently in winter, but this was not statistically significant. In a previous study a significant difference in microbial drinking water quality was found between winter and summer, with effect sizes ranging from small to large (Kamal et al., 2024). Notably, we found no correlation between the presence of E. coli and the physical or chemical properties (e.g., iron) of the water. This is consistent with previous research on the presence of E. coli in biofilms of European drinking water networks (Juhna et al., 2007). However, as mentioned above, iron is thought to promote the growth of E. coli (Grandjean et al., 2006; Wingender and Flemming, 2011; van Eenige et al., 2013). In this long-term study this could not be confirmed. There is a clear need to continue to explore this topic, especially given the observations made in this study.
Contamination can originate from the water source itself, especially well or surface water. One important study found that approximately 60% of vulnerable wells and half of initially non-vulnerable wells tested positive for signs of fecal contamination (Hruskova et al., 2016; Macler and Pontius, 1997). Nevertheless, the main problem in livestock production is that microorganisms enter the water system from the drinking trough and form biofilms, which have significant hygienic consequences. Therefore, it seems necessary to regularly clean the drinking water installation system and to remove remaining biofilm. A previous study showed that water troughs for laying hens carry a minimal bacterial load if they are cleaned daily (Folorunso et al., 2014). Proper biofilm removal is critical to protect new animals from previous pathogens. Measures include prevention, rinsing, cleaning, and disinfection. Based on the results of a previous study, it can be deduced that longer-lasting drain cleaning methods tend to produce better results than shorter methods (Vogel et al., 2020). In addition, the results suggest that rinsing with water alone can have a significant cleaning effect. Therefore, combining physical cleaning through rinsing with the use of chemical cleaning substances enhances the overall cleaning effectiveness. While proper sanitation of the water lines and tanks is important, it is also necessary to ensure that no residues of cleaning and/or disinfectant products are detectable, as this may have a negative effect on animals and also a negative effect on the efficacy of pharmaceuticals administered via drinking-water.
Various options are available for the subsequent disinfection of drinking water pipe systems. The selection of a disinfectant for drinking water depends on the type and characteristics of the water, the application, and the intended use. Disinfectants can inhibit the growth of micro-organisms, such as bacteria and fungi, or have a lethal effect on viruses (Maris, 1995). Generally, disinfectants can be categorized into two main groups: oxidizing and non-oxidizing disinfectants (Fisher, 2003). Oxidizing disinfectants are those that contain halogens like chlorine, iodine, and oxygen-releasing substances, while non-oxidizing disinfectants are those that bind to structures, such as quaternary ammonium compounds and amphoteric. According to the Drinking Water Directive, chlorine (Cl), chlorine dioxide (ClO2), sodium hypochlorite (NaOCl), and ozone (O3) can be used for drinking water treatment (Trinkwasserverordnung, 2001). For instance, disinfecting drinking water with NaOCl can significantly reduce bacteria, including potential pathogens and biofilm-forming bacteria, in nursery water lines without affecting endotoxin concentrations (Böger et al., 2020). Today, there is a wide variety of products available for the disinfection of drinking water. All of them have their advantages and disadvantages. Therefore, certain factors must be considered when finding the right product for the individual application. There is a need for more comprehensive studies of water quality and available drinking water disinfectants for safe and effective use in animal agriculture. What would also be of interest is the action that takes place from the knowledge of water quality. In a previous study, the risk of an incorrect flow rate across all drinkers impacting water intake appeared significantly lower on farms participating in the German ITW program (Schale et al., 2022). In addition, the ITW farms performed significantly better across all weight classes. Thus, there is evidence that poor drinking water quality can affect livestock productivity and welfare. However, it is also possible that actions were taken after the water quality was observed, as testing of water quality and flow rate is mandatory for participants. This observation clearly needs further investigation. The psychological level of how farmers decide to improve their water management would be beneficial.
The quality of the water changes during the process steps and can reach the animal differently than it enters the front of the barn. In the case of German tap water, municipalities are responsible for creating clean tap water through extraction, treatment, and distribution. However, they only guarantee water suitable for human consumption up to the first point of use. After that, the barn manager is responsible for drinking water quality. This includes the entire drinking system, including management and materials. Based on personal experience in the livestock sector, technically suitable but hygienically unsuitable (e.g., promoting microbial growth) materials are often used. Depending on the material, the growth of biofilms can be favored (Lehtola et al., 2004). In addition, the temperature may have a significant influence on biofilm growth in the piping system. Measuring the temperature of the water sampled and documenting the materials used would have provided a more detailed picture of the technical influence. In the future this should be investigated in more detail. The effect of drinking water quality and installation on animal welfare also need to be studied for future husbandry decision-making. Pool drinkers have the distinct advantage of allowing pigs to access water from an open surface, which corresponds to their natural behavior as suckling pigs (Boxberger, 1986). As the current study only sampled nipple drinkers, a comparison with other water installations (e.g., pool drinkers) would be interesting.
Feed and feed management in animal husbandry are a widely studied field. Nevertheless, there is a lack of knowledge not only about water quality, but also about the drinking behavior of animals. Water intake can be limited by both quality and quantity, and factors such as overcrowding at the water source due to management practices can also negatively affect water consumption. Severe water shortages can negatively affect productivity, behaviour, and welfare status of chickens (El Sabry et al., 2023). In pigs, flow rates that are too low can result in inadequate water consumption, leading to thirst and discomfort (Andersen et al., 2016). Discomfort can lead to stress, which may result in tail and ear biting. On the other hand, too high a flow rate can also hinder water intake. Therefore, the flow rate of the drinking troughs must be sufficient for the animals and should be investigated in further studies.
Taking water samples is currently an easy and inexpensive way to assess the water condition. However, taking only water samples for evaluation has its limitations. Since the composition of microorganisms in water is different from that in biofilm on surfaces, the conclusion may be limited. In general, sampling of biofilms makes more sense than that of water, as water samples provide only limited information about the location and extent of biofilms. However, the sampling of biofilms is associated with a very high level of effort. In addition, biofilms are not necessarily evenly distributed in the drinking water system. Taking multiple swab samples at different points in the system could provide a better understanding of the microbial composition. In addition, our study was limited to a specific region in Germany, which may not be transferable to other regions in the South or East of Germany. Furthermore, the results shown may differ in other regions with different environmental and agricultural conditions.
5 Conclusion
The availability and quality of water are critical to animal health, especially in highly productive livestock. This study highlights the potential risk of chemical compounds in animal drinking water and the need for hygienic measures to prevent the spread of micro-organisms through water. It also underlines the critical role of maintaining clean water supplies, not only for animal health, but also as a key factor in sustainable farming practices and responsible use of resources. A good understanding of the composition of the water as well as the processes during distribution makes it easier to identify any weak points at an early stage. Regular monitoring of drinking water quality and the implementation of preventive hygiene measures are strongly recommended. Further research is needed to determine the potential risk of poor drinking water quality for both animal health and water intake. While water samples provide insights into water quality, combining water sampling with sampling of the biofilm could offer a more complete understanding of the water quality.
Data availability statement
The raw data supporting the conclusions of this article will be made available by the authors, without undue reservation.
Author contributions
PM: Conceptualization, Data curation, Formal analysis, Investigation, Methodology, Validation, Writing – original draft. NK: Conceptualization, Investigation, Methodology, Supervision, Writing – review & editing.
Funding
The author(s) declare financial support was received for the research, authorship, and/or publication of this article. We acknowledge financial support by the Open Access Publication Fund of the University of Veterinary Medicine Hannover, Foundation.
Acknowledgments
The authors would like to thank all farms and laboratory staff who were involved in this investigation.
Conflict of interest
Author PM was employed by Elanco Deutschland GmbH.
The remaining author declare that the research was conducted in the absence of any commercial or financial relationships that could be construed as a potential conflict of interest.
The author(s) declared that they were an editorial board member of Frontiers, at the time of submission. This had no impact on the peer review process and the final decision.
Publisher’s note
All claims expressed in this article are solely those of the authors and do not necessarily represent those of their affiliated organizations, or those of the publisher, the editors and the reviewers. Any product that may be evaluated in this article, or claim that may be made by its manufacturer, is not guaranteed or endorsed by the publisher.
References
Abeliotis K., Candan C., Amberg C., Ferri A., Osset M., Owens J., et al. (2014). Impact of water hardness on consumption’ perception of laundry washing result in five European countries. Int. J. Consumer Stud. 39, 60–66. doi: 10.1111/ijcs.12149
Ahmadpari H., Tavazoei A., Taghavi M., Parhamfar M. (2022). “Application of ion exchange technology in water treatment,” in Proceedings of the 5th International Conference on Recent Innovations Chemistry and Chemical Engineering. Tehran, Iran: Allameh Tabataba’I University, 24.-25. September 2022.
Ahmed S., Chughtai S., Keane M. A. (1998). The removal of cadmium and lead from aqueous solution by ion exchange with Na Y zeolite. Separation Purification Technol. 13, 57–64. doi: 10.1016/S1383-5866(97)00063-4
Andersen H. M.-L., Jørgensen E., Pedersen L. J. (2016). Using evolutionary operation technique to evaluate different management initiatives at herd level. Livestock Sci. 187, 109–113. doi: 10.1016/j.livsci.2016.03.006
Andersen W. C., Bruno T. J. (2003). Application of gas–liquid entraining rotor to supercritical fluid extraction: removal of iron (III) from water. Analytica Chimica Acta 485, 1–8. doi: 10.1016/S0003-2670(03)00400-8
Anderson D. M. (1978). Effects of saline water high in sulphate, chlorides and nitrates on the performance of young weanling pigs. J. Anim. Sci. 47, 900–907. doi: 10.2527/jas1978.474900x
Augusto E., Aleixo J., Chilala F. D., Chilundo A. G., Gaspar B., Bila C. G. (2022). Physical, chemical and microbiological assessments of drinking water of small-layer farms. Onderstepoort J. Veterinary Res. 89, e1–16. doi: 10.4102/ojvr.v89i1.2067
Avila D. S., Puntel R. L., Aschner M. (2013). Manganese in health and disease. Metal Ions Life Sci. 13, 199–227. doi: 10.1007/978-94-007-7500-8_7
Aziz H. A., Yusoff M. S., Aslan M. N., Adnan N. H., Alias S. (2004). Physicochemical removal of iron from semiaerobic landfill leachate by limestone filter. Waste Manage. 24, 353–358. doi: 10.1016/j.wasman.2003.10.006
Bailey S. E., Olin T. J., Bricka R. M., Adrian D. D. (1999). A review of potentially low-cost sorbents for heavy metals. Water Res. 33, 2469–2479. doi: 10.1016/S0043-1354(98)00475-8
Berbenni P., Pollice A., Canziani R., Stabile. L., Nobili F. (2000). Removal of iron and manganese from hydrocarbon-contaminated groundwaters. Bioresource Technol. 74, 109–114. doi: 10.1016/S0960-8524(00)00003-1
Böger R., Rohn K., Kemper N., Schulz J. (2020). Sodium hypochlorite treatment: the impact on bacteria and endotoxin concentration in drinking water pipes of a pig nursery. Agriculture 10, 86. doi: 10.3390/agriculture10030086
Boxberger J. (1986). Verhaltensangepasste Trinkwasserversorgung von Rindern und Schweinen. Deutsche Tierärztliche Wochenschrift 93, 286–289.
Bundesministerium für Ernährung und Landwirtschaft (BMEL). (2007). Hygienische Qualität von Tränkwasser. Orientierungsrahmen zur futtermittelrechtlichen Beurteilung (BMEL, Bonn). Available online at: https://www.bmel.de/DE/themen/tiere/futtermittel/orientierungsrahmen-traenkwasser.html (Accessed 12.06.2024).
Bundesministerium für Ernährung und Landwirtschaft (BMEL) (Hrsg.). (2014). Leitfaden „Orale Anwendung von Tierarzneimitteln im Nutztierbereich über das Futter oder das Wasser” (BMEL, Bonn). Available online at: https://www.bmel.de/SharedDocs/Downloads/DE/_Tiere/Tiergesundheit/Tierarzneimittel/Leitfaden-Orale-Anwendung-Tierarzneimittel.html (Accessed 30.04.2024).
Carson T. L. (2000). Current knowledge of water quality and safety for livestock. Veterinary Clinics North America 16, 455–464. doi: 10.1016/S0749-0720(15)30080-3
Chien L., Robertson H., Gerrard J. W. (1968). Infantile gastroenteritis due to water with high sulfate content. Can. Med. Assoc. J. 99, 102–104.
Cho B. Y. (2005). Iron removal using aerated granular filter. Process Biochem. 40, 3314–3320. doi: 10.1016/j.procbio.2005.03.031
Costerton K. W., Stewart P. S., Greenberg E. P. (1999). Bacterial biofilms: a common cause of persistent infections. Science 284, 1318–1322. doi: 10.1126/science.284.5418.1318
Das B., Hazarika P., Saikia G., Kalita H., Goswami D. C., Das H. B., et al. (2006). Removal of iron from groundwater by ash: a systematic study of a traditional method. J. Hazardous Material 141, 834–884. doi: 10.1016/j.jhazmat.2006.07.052
Denton R. M., McCormack J. G., Edgell N. J. (1980). Role of calcium ions in the regulation of intramitchondrial metabolism. Effects of Na+, Mg2+ and ruthenium red on the Ca2+-stimulated oxidation of oxoglutarate and on pyruvate dehydrogenase activity in intact rat heart mitochondria. Biochem. J. 190, 107–117. doi: 10.1042/bj1900107
Devane M. L., Moriarty E., Weaver L., Cookson A., Gilpin B. (2020). Fecal indicator from environmental sources; strategies for identification to improve water quality monitoring. Water Res. 185, 116204. doi: 10.1016/j.watres.2020.116204
DIN 38404-5. (1984). German standard methods for examination of water, waste water and sludge; physical and physico-chemical characteristics (group C); determination of pH value (C5) | Engineering. 360:1–7. (Berlin, Germany: DIN Media GmbH).
DIN 38406-3-3. (2002). German standard methods for the examination of water,waste water and sludge - Cations (group E) - Part 3: Determination of calcium and magnesium, complexometric method (E 3). 1–13. (Berlin, Germany: DIN Media GmbH).
DIN 38406-5. (1983). German standard methods for the examination of water, waste water and sludge; cations (Group E); determination of ammonia-nitrogen (E 5). 1–14. (Berlin, Germany: DIN Media GmbH).
DIN EN ISO 10304-1. (2009). Water quality - Determination of dissolved anions by liquid chromatography of ions - Part 1: Determination of bromide, chloride, fluoride,nitrate, nitrite, phosphate and sulfate. 1–24. (Berlin, Germany: DIN Media GmbH).
DIN EN ISO 17294-1. (2004). Water quality—Application of inductively coupled plasma mass spectrometry (ICP-MS)—Part 1: general guidelines. 1–33. (Vernier, Switzerland: International Organization for Standardization).
DIN EN ISO 6222. (1999). Water quality - enumeration of culturable microorganisms- Colony count by inoculation in a nutrient agar culture medium. 1–8. (Berlin, Germany: DIN Media GmbH).
DIN EN ISO 9308-1. (2000). Water quality - Enumeration of Escherichia coli and coliform bacteria - Part 1: Membrane filtration method for waters with low bacterial background flora. 1–10. (Berlin, Germany: DIN Media GmbH).
Donlan R. M. (2000). Biofilms: microbial life on surfaces. Emerging Infect. Dis. 8, 881–890. doi: 10.3201/eid0809.020063
Dorr P. M., Nemechek M. S., Scheidt A. B., Baynes R. E., Gebreyes W. A., Almond G. W. (2009). Water-flow variation and pharmacoepidemiology of tetracycline hydrochloride administration via drinking water in swine finishing farms. J. Am. Veterinary Med. Assoc. 235, 299–304. doi: 10.2460/javma.235.3.299
Ellis D., Bouchard C., Lantagne G. (2000). Removal of iron and manganese from groundwater by oxidation and microfiltration. Desalination 130, 255–264. doi: 10.1016/S0011-9164(00)00090-4
El Sabry M., Romeih Z. U., Stino F. K. R., Khosht A. R., Aggrey S. E. (2023). Water scarcity can be a critical limitation for the poultry industry. Trop. Anim. Health Production 55, 215. doi: 10.1007/s11250-023-03599-z
European Commission. (1991). Council directive concerning the protection of waters against pollution caused by nitrates from agricultural sources (91/676/EEC). Available online at: https://eur-lex.europa.eu/eli/dir/1991/676/oj (Accessed 30.04.2024).
European Commission. (2019). The European green deal COM/2019/640 final. Brussels, 11(12), the European green deal — European environment agency (europa.eu). Available online at: https://eur-lex.europa.eu/resource.html?uri=cellar:b828d165-1c22-11ea-8c1f-01aa75ed71a1.0002.02/DOC_1&format=PDF (Accessed 12.06.2024).
European Medicines Agency. (2020). Advice on implementing measures under Article 106 (6) of Regulation (EU) 2019/6 on veterinary medicinal products – scientific problem analysis and recommendations to ensure a safe and efficient administration of oral veterinary medicinal products via routes other than medicated feed. EMA/CVMP/508559/2019. Available online at: https://www.ema.europa.eu/en/documents/regulatory-procedural-guideline/advice-implementing-measures-under-article-106-6-regulation-eu-20196-veterinary-medicinal-products-scientific-problem-analysis-and-recommendations-ensure-safe-and-efficient-administration-oral_en.pdf (Accessed 30.04.2024).
European Parliament (2005). Regulation (EC) No 183/2005 of the European Parliament and of the council of 12 January 2005 laying down requirement for feed hygiene. Available online at: https://eur-lex.europa.eu/eli/reg/2005/183/oj (Accessed 30.04.2024).
Fisher J. (2003). Cleaning procedures in the factory - Types of Disinfectant. In: Encyclopedia of Food Sciences and Nutrition (Second Edition), (Maryland, USA: Elsevier Science Ltd.) pp. 1382–1385.
Flemming H. C., Bendinger B., Exner M., Gebel. J., Kistemann T., Schaule G., et al. (2014). “The last meters before the tap: where drinking water quality is at risk,” in Microbial growth in drinking-water supplies. Problems, causes, prevention and research needs. Eds. van der Kooij D., van der Wielen P. W. J. J. (IWA Publishing, London, UK), 207–238.
Flemming H.-C., Leis A. (2002). “Sorption properties of biofilms,” in Encyclopedia of environmental microbiology, vol. 5 . Ed. Bitton G. (Wiley-Interscience, New York, USA), 2958–2967.
Flemming H. C., Wingender J. (2001). Flocken, Filme und Schlämme: Biofilme - die bevorzugte Lebensform der Bakterien. Biologie unserer Zeit 31, 169–180. doi: 10.1002/1521-415X(200105)31:33.3.CO;2-L
Folorunso O. R., Kayode S., Onibon V. O. (2014). Poultry farm hygiene: microbiological quality assessment of drinking water used in layer chickens managed under battery cage and deep litter systems at three poultry farms in southwestern Nigeria. Pakistan J. Biol. Sci. 17, 74–79. doi: 10.3923/pjbs.2014.74.79
Frisbie S. H., Mitchell E. J., Dustin H., Maynard D. M., Sarkar B. (2012). World health organization discontinues its drinking-water guideline for manganese. Environ. Health Perspect. 120, 775–778. doi: 10.1289/ehp.1104693
Früchtenicht K. (2000). Geogene und anthropogene Kontaminanten im Tränkewasser. Deutsche Tierärztliche Wochenschrift 107, 329–331.
Genther O. N., Beede D. K. (2012). Preferences and drinking behavior of lactating dairy cows offered water with different concentrations, valences, and sources of iron. J. Dairy Sci. 96, 1164–1176. doi: 10.3168/jds.2012-5877
Ghosh D., Solanki H., Purkait M. K. (2008). Removal of Fe (II) from tap water by electrocoagulation technique. J. Hazardous Materials 155, 135–143. doi: 10.1016/j.jhazmat.2007.11.042
Giammarin M., Quatto P. (2015). Nitrates in drinking water: relation with intensive livestock production. J. Prev. Med. Hygiene 56, E187–E189. doi: 10.15167/2421-4248/jpmh2015.56.4.457
Godskesen B., Hauschild M., Rygaard M., Zambrano K., Albrechtsen H.-J. (2012). Life cycle assessment of central softening of very hard drinking water. J. Environ. Manage. 105, 83–89. doi: 10.1016/j.jenvman.2012.03.030
Gomez G. G., Sandler R. S., Seal E. S. Jr. (1995). High levels of inorganic sulfate cause diarrhea in neonatal piglets. J. Nutr. 125, 2325–2332. doi: 10.1093/jn/125.9.2325
Gonyou H. (1996). Water Use and Drinker Management: A Review (Saskatoon, SK, Canada: Annual Research Report of the Prairie Swine Centre Inc), 74–80.
Goronczy P., Piontkowski A. (1999). Zur Beurteilung von Fütterungsarzneimitteln aus mehreren Arzneimittel-Vormischungen. Amtstierärztlicher Dienst und Lebensmittelkontrolle (BbT) 6, 73–79.
Grandjean D., Jorand F. P. A., Guilloteau. H., Block J.-C. (2006). Iron uptake is essential for Escherichia coli survival in drinking water. Lett. Appl. Microbiol. 43, 111–117. doi: 10.1111/j.1472-765X.2006.01895.x
Hooda P. S., Edwards A. C., Anderson H. A., Miller A. (2000). A review of water quality concerns in livestock farming areas. Sci. Total Environ. 250, 143–167. doi: 10.1016/s0048-9697(00)00373-9
Hruskova T., Sasakova N., Bujdosova Z., Kvokacka V., Gregova G., Verebova V., et al. (2016). Disinfection of potable water sources on animal farms and their microbiological safety. Veterinární medicína 61, 173–186. doi: 10.17221/8818-VETMED
Initiative Tierwohl (ITW). (2015). Available online at: https://initiative-tierwohl.de/ (Accessed 12.06.2024).
Jacques M., Aragon V., Tremblay Y. (2010). Biofilm formation in bacterial pathogens of veterinary importance. Anim. Health Res. Rev. 11, 97–121. doi: 10.1017/S1466252310000149
João P., Cabral P. S. (2010). Water microbiology. Bacterial pathogens and water. Int. J. Environ. Res. Public Health 7, 3657–3703. doi: 10.3390/ijerph7103657
Juhna T., Birzniece D., Larsson S., Zulenkovs D., Sharipo A., Azevedo N., et al. (2007). Detection of Escherichia coli in biofilms from pipe samples and coupons in drinking water distribution network. Appl. Environ. Microbiol. 73, 7456–7464. doi: 10.1128/AEM.00845-07
Kamal M. A., Allhotan R. A., Al Sulaiman A. R., Hussein E. O., Galik B., Saleh A. A. (2024). From source to house: unravelling the seasonal effect of water distribution system on drinking water quality of poultry farms under Egyptian environmental condition. Environ. Sci. pollut. Res. 31, 12966–12977. doi: 10.1007/s11356-024-31911-3
Kamphues J., Böhm R., Flachowsky G., Lahrssen-Wiederholt M., Meyer U., Schenkel H. (2007). Empfehlungen zur Beurteilung der hygienischen Qualität von Tränkwasser für Lebensmittel liefernde Tiere unter Berücksichtigung der gegebenen rechtlichen Rahmenbedingungen. Landbauforschung Völkerode 57, 255–272.
Keswick B. H., Fujioka R. S., Burbank N. C. Jr., Loh P. C. (1978). Comparative disinfection efficiency of bromine chloride and chlorine for poliovirus. J. AWWA 70, 573–577. doi: 10.1002/j.1551-8833.1978.tb04245.x
Kilb B., Lange B., Schaule G., Flemming H. C. (2003). Wingender, J. Contamination of drinking water by coliforms from biofilms grown on rubber-created valves. Int. J. Hygiene Environ. Health 206, 563–573. doi: 10.1078/1438-4639-00258
King A. J. (1996). Water quality and poultry production. Poultry Sci. 75, 852–853. doi: 10.3382/ps.0750852
Kotb S., Ahmed M., Hassan D., Soltan E. (2019). Stability of antibiotics in drinking water: An advanced approach towards the impacts of water quality parameters on doxycycline bioavailability. J. Advanced Veterinary Anim. Res. 6, 438–444. doi: 10.5455/javar.2019.f365
Lee J. Y., Yang J.-S., Han M., Choi J. (2010). Comparison of the microbiological and chemical characterization of harvested rainwater and reservoir water as alternative water resources. Sci. Total Environ. 408, 896–905. doi: 10.1016/j.scitotenv.2009.11.001
Lehtola M. J., Miettinen I. T., Keinanen M. M., Kekki T. K., Laine O., Hirvonen A., et al. (2004). Microbiology, chemistry and biofilm development in a pilot drinking water distribution system with copper and plastic pipes. Water Res. 38, 3769–3779. doi: 10.1016/j.watres.2004.06.024
Lumb K. R., Robertson J., Scott H. E., Woolfenden N. J. H. (2017). “Optimising the use of antimicrobials: reparing the industry for in-water delivery in the short term and improving hygiene and more effectively targeting medication in the longer term,” in Conference Proceedings RAFT Solutions Ltd. Water, Ripon. Ripon, United Kingdom AHDB Pork, RAFT Solutions Ltd.
Macler A. B., Pontius W. F. (1997). Groundwater Disinfection: Chlorine’s role in public health. J. Am. Water Works Assoc. 4.
Maris P. (1995). Modes of action of disinfection. Rev. Scientifique Technique 14, 47–55. doi: 10.20506/rst.14.1.829
Meays C. L., Broersma K., Nordin R., Mazumder A. (2004). Source tracking fecal bacteria in water: A critical review of current methods. J. Environ. Manage. 73, 71–79. doi: 10.1016/j.jenvman.2004.06.001
Moreno P., Aral H., Vecchio-Sadus A. (2009). “Environmental impact and toxicology of sulphate,” in Proceedings of the Enviromine. Santiago, Chile: GECAMIN, 30. September - 2. October 2009.
Morgan S. E. (2011). Water quality for cattle. Veterinary Clinics North America: Food Anim. Pract. 27, 285–295. doi: 10.1016/j.cvfa.2011.02.006
Munter R., Ojaste H., Sutt J. (2005). Complexed iron removal from ground water. J. Environ. Eng. 131, 1014–1020. doi: 10.1061/(ASCE)0733-9372(2005)131:7(1014
Mustedanagic A., Matt M., Weyermair K., Schrattenecker A., Kubitza I., Firth C., et al. (2023). Assessment of microbial quality in poultry drinking water on farms in Austria. Front. Veterinary Sci. 10. doi: 10.3389/fvets.2023.1254442
Nakagawara S., Goto T., Nara M., Ozawa Y., Hotta K., Arata Y. (1998). Spectroscopic characterization and the pH dependence of bactericidal activity of the aqueous chlorine solution. Analytical Sci. 14, 691–698. doi: 10.2116/analsci.14.691
O’Neal S., Zheng W. (2015). Managenese toxitiy upon overexposure: a decade in review. Curr. Environ. Health Rep. 2, 315–328. doi: 10.1007/s40572-015-0056-x
Praveen P. K., Ganguly S., Wakchaure R., Para P. A., Mahajann T., Qadri K., et al. (2016). Water-borne disease and its effect on domestic animals and human health: a review. Int. J. Emerging Technol. Advanced Eng. 6, 242–245. ISSN 2250-2459.
Rengaraj S., Moon S.-H. (2002). Kineticy of adsorption of Co(II) removal from water and wastewater by ion exchange resins. Water Res. 36, 1783–1793. doi: 10.1016/S0043-1354(01)00380-3
Rodier J. (1955). Managnese poisoning in Moroccan mines. Br. J. Ind. Med. 12, 21–35. doi: 10.1136/oem.12.1.21
Schale P., Schmitt A. O., Dänicke S., Kluess J., Grümpel-Schlüter A., Arkenau E. F. (2022). Does the implementation of an animal welfare programme on a farm yield a demonstrable improvement in fattening pig welfare? Animals 12, 3337. doi: 10.3390/ani12233337
Shukla S. K., Khan A., Subba Rao T. (2021). “Chapter 22 – Microbial fouling in water treatment plants,” in Microbial and Natural Macromolecules Synthesis and Applications. Eds. Das S., Dash H. R. (Elsevier Inc., Amsterdam, Netherlands), 589–622.
Stöber M., Scholz H. (2002). “Sulfid-, sulfat-, sulfit- und schwefelvergiftung,” in Innere Medizin und Chirurgie des Rindes, 4 Edition. Eds. Dirksen G., Gründer H.-D., Stöber M. (Parey Verlag, Berlin, Germany), 325.
Sumano L. H., Gutierrez O. L., Aguilera R., Rosiles M. R., Bernard B. M. J., Gracia M. J. (2004). Influence of hard water on the bioavailability of enrofloxacin in broilers. Poultry Sci. 83, 726–731. doi: 10.1093/ps/83.5.726
Tahir S. S., Rauf N. (2004). Removal of Fe(II) from the wastewater of a galvanized pipe manufacturing industry by adsorption onto bentonite clay. J. Environ. Manage. 73, 285–292. doi: 10.1016/j.jenvman.2004.06.009
Townsend R., Coker E. N. (2001). Chapter 11 ion exchange in zeolites. Stud. Surface Sci. Catalysis 137, 467–524. doi: 10.1016/S0167-2991(01)80253-6
Tremblay M. L., Christison G. I., Patience J. F. (1989). The effect of sulfate in water on weanling pig performance [abstract. Can. J. Anim. Sci. 69, 1118.
Trinkwasserverordnung. (1990). Verordnung über die Qualität von Wasser für Lebensmittelbetriebe vom 5. Dezember 1990 (Trinkwasserverordnung – TrinkwV) Bundesgesetzblatt 66, 2612.
Trinkwasserverordnung. (2001). Verordnung über die Qualität von Wasser für den menschlichen Gebrauch vom 21. Mai 2001 (Trinkwasserverordnung – TrinkwV) Bundesgesetzblatt 24, 959–969.
Umar S., Munir M. ,. T., Azeem T., Ali S., Umar W., Rehman A., et al. (2014). Effects of water quality on productivity and performance of livestock: a mini review. Open Access J. Veterinaria 3, 11–15.
Ungemach F. R. (2003). Magen-Darm-wirksame Pharmaka. In Pharmakotherapie bei Haus- und Nutztieren. 6 Edition. Eds. Löscher W., Ungemach F. R., Kroker R. (Berlin, Germany: Parey Verlag), 208–247.
United Nations. (2015). United Nations' Sustainable Development Goal (SDG). Available online at: https://sdgs.un.org/goals (Accessed 12.06.2024).
Vaaramaa K., Lehto J. (2003). Removal of metals and anions from drinking water by ion exchange. Desalination 155, 157–170. doi: 10.1016/S0011-9164(03)00293-5
van der Sluis W. (2002). Water quality is important but often over estimated. World Poultry 18, 26–32.
van Eenige M. J. E. M., Counotte G. H. M., Noordhuizen J. (2013). Drinking water for dairy cattle: Always a benefit or a microbiological risk? Tijdschrift Voor Diergeneeskunde 138, 86–97.
Veenhuizen M. F., Shurson G. C., Kohler E. M. (1992). Effect of concentration and source of sulphate on nursery pig performance and health. J. Am. Veterinary Med. Assoc. 201, 1203–1208. doi: 10.2460/javma.1992.201.08.1203
Vodela J. K., Lenz S. D., Renden J. A., McElhenney W. H., Kemppainen B. W. (1997). Drinking water contaminants (arsenic, cadmium, lead, benzene, and trichloroethylene). 1. Interaction of contaminants with nutritional status on general performance and immune function in broiler chickens. Poultry Sci. 76, 1474–1492. doi: 10.1093/ps/76.11.1474
Vogel J., Wingender J., Uphoff J., Schaule G., Aumann K., Hufelschulte J., et al. (2020). Charakterisierung von Belägen in Tränkwasserleitungen in Ferkelaufzuchtställen und mögliche Hygienekonzepte. Berliner und Münchener Tierärztliche Wochenschrift 133, 36–48. doi: 10.2376/0005-9366-19018
von Keyserlingk M. A. G., Phillips C. J. C., Nielsen B. L. (2016). “Water and the welfare of farm animals,” in Nutrition and the Welfare of Farm Animals. Animal Welfare, 1 Edition, Ed. Phillips C. (Basel, Switzerland: Springer Cham, Country) 16, 183–197.
Wan Y., Ma R., Chai L., Du Q., Yang R., Qi R., et al. (2021). Determination of bacterial abundance and communities in the nipple drinking system of cascading cage layer houses. Sci. Rep. 11, 19169. doi: 10.1038/s41598-021-98330-z
Wingender J., Flemming H. C. (2011). Biofilm in drinking water and their role as reservoir for pathogens. Int. J. Hygiene Environ. Health 214, 417–423. doi: 10.1016/j.ijheh.2011.05.009
Zimmermann N. G., Douglass L. (1998). A survey of drinking water quality and its effects on broiler growth performance on Delmerva. Poultry Sci. 77, 121. doi: 10.3923/ijps.2008.433.436
Keywords: bacteria, chemicals, contaminants, livestock, transmission, water hygiene
Citation: Münster P and Kemper N (2024) Long-term analysis of drinking water quality in poultry and pig farms in Northwest Germany. Front. Anim. Sci. 5:1467287. doi: 10.3389/fanim.2024.1467287
Received: 19 July 2024; Accepted: 28 August 2024;
Published: 18 September 2024.
Edited by:
Michael D. Flythe, United States Department of Agriculture, United StatesReviewed by:
Sebastian Opaliński, Wroclaw University of Environmental and Life Sciences, PolandChristi L. Swaggerty, Agricultural Research Service (USDA), United States
Copyright © 2024 Münster and Kemper. This is an open-access article distributed under the terms of the Creative Commons Attribution License (CC BY). The use, distribution or reproduction in other forums is permitted, provided the original author(s) and the copyright owner(s) are credited and that the original publication in this journal is cited, in accordance with accepted academic practice. No use, distribution or reproduction is permitted which does not comply with these terms.
*Correspondence: Nicole Kemper, nicole.kemper@tiho-hannover.de