- 1Department of Animal Science, Faculty of Agriculture, Malayer University, Malayer, Iran
- 2Departament de Ciència Animal, Universitat de Lleida – Agrotecnio-CERCA Center, Lleida, Spain
- 3Department of Research and Development, Sodour Ahrar Shargh Company, Tehran, Iran
- 4Department of Animal Science, Faculty of Agriculture, Ferdowsi University of Mashhad, Mashhad, Iran
This study aimed to evaluate the effects of milk supplementation with different zinc (Zn) sources on growth rate, starter intake, diarrhea incidence, and nutrient digestibility in dairy calves during the pre-weaning period. Forty newborn Holstein heifer calves were assigned to four treatments: control (no Zn supplementation; CON) or supplementation with 80 mg/day Zn from zinc sulfate (Zn-Sul), chelated zinc (Zn-Bon), or zinc methionine (Zn-Met). The Zn supplements were provided via milk from days 3 to 14 of age. Body weight (BW) and starter intake (SI) were monitored until weaning on day 70. Serum metabolite concentrations were determined in blood samples collected on days 15 and 70. Fecal consistency index (FCI) and frequency of medical treatments were measured during the first 21 days of life. Apparent total tract digestibility (ATTD) was determined in the 10th week of life. Although the effects of Zn supplementation on BW gain or body measurements were non-significant, a tendency for treatment × time interaction on BW was observed, with variations among the experimental groups becoming more pronounced on days 56 and 70, showing higher values in Zn-supplemented calves compared to CON. SI was improved by Zn supplementation, with the greatest response in the Zn-Bon group. Compared to control, ATTD of organic matter, neutral detergent fiber, and starch tended to increase with Zn supplementation. Blood metabolites and FCI did not differ among the experimental treatments. Although no significant difference was detected between Zn-Met and CON, the frequency of medical treatment was significantly lower in Zn-Bon and Zn-Sul compared to CON calves. In conclusion, zinc supplementation in milk, particularly with the organic chelated zinc source (Zn-Bon), showed promising effects on increasing starter intake and reducing medical treatments, along with trends toward enhanced growth and nutrient digestibility in pre-weaned calves.
1 Introduction
Raising dairy calves to become productive replacement heifers is a critical component of successful dairy farming. The early growth and development of these calves are fundamental to their future productivity and overall health. Proper nutrition, care, and management during the early stages of a calf’s life can significantly impact its future milk yield, reproductive performance, and longevity in the herd. The development and maturation of the rumen is a crucial physiological challenge faced by young ruminants. At birth, the forestomach of a calf, which include the rumen, reticulum, and omasum, are underdeveloped and non-functional. Consequently, newborn calves function primarily as monogastric animals, relying on the abomasum for digestion. The transition from this monogastric phase to a fully functional ruminant state involves significant physiological, microbial, and nutritional changes (Baldwin et al., 2004). These changes encompass increases in rumen volume, differentiation and proliferation of epithelial cells, shifts in rumen microbial flora, and alterations in nutrient transfer patterns to the intestines, liver, and surrounding tissues.
Zinc (Zn) is a vital trace element involved in numerous biological processes, including protein structure and function, enzyme activity, DNA and RNA metabolism, and gene expression. Additionally, Zn has a regulatory function in apoptosis and signal transduction (Kincaid, 2000; Hambidge and Krebs, 2007). Research has demonstrated that supplementing calves with 80 mg of Zn per day during their first two weeks of life can stimulate growth, enhances immune responses, and reduces the incidence of diarrhea (Feldmann et al., 2019; Chang et al., 2020; Wo et al., 2022). The anti-diarrheal effects of Zn are attributed to its ability to improve immune system activity, reduce pathogenic bacterial populations, and promote the growth of beneficial gut bacteria (Fairbrother et al., 2005; Sales, 2013). Moreover, Zn is an essential component of many metalloenzymes involved in DNA and RNA synthesis, and it influences the activity of thymidine kinase, an enzyme critical for cell division (MacDonald, 2000). Recent studies have highlighted a significant relationship between rumen development and Zn uptake, underscoring the importance of adequate Zn availability for the early establishment of rumen microbial flora and the growth of villi in the rumen epithelium. It has been reported that Zn absorption is crucial for the growth and development of rumen villi in goats, particularly due to its role in zinc-finger (ZNF) proteins (Cernik et al., 2013). Malmuthuge et al. (2019) investigated the relationship between rumen microbial flora and gene expression patterns in the rumen epithelium of Holstein calves aged 1, 3, and 6 weeks, discovering a significant positive correlation between ZNF protein expression and rumen development. Although the effect of Zn on the overall health and performance of dairy cows and calves has been extensively researched (Spears, 1996), its specific impacts on rumen growth and development during the early life stages are still not fully defined. Furthermore, the comparative efficacy of various Zn supplementation forms requires further investigation. Elucidating these differences is crucial for determining the most effective Zn formulations and optimizing supplementation strategies in dairy calf nutrition.
A variety of Zn dietary supplements are commercially available for animal nutrition, including inorganic forms such as Zn oxide and Zn sulfate, as well as organic forms. The organic Zn sources like Zn methionine have shown higher bioavailability and efficacy in promoting growth and health compared to inorganic sources (Nayeri et al., 2014; Ishaq et al., 2018). Chang et al. (2020) suggests that Zn methionine promotes superior growth in dairy calves compared to zinc oxide, while Zn oxide is more effective in preventing diarrhea. Consequently, a strategic approach of administering Zn oxide in the initial days of life, followed by Zn methionine until 14 days of age, may optimize both growth and health benefits in dairy calves. The authors, however, mention that the enhanced growth observed with Zn methionine may be attributed to both its higher bioavailability and the presence of methionine, an essential amino acid, complicating definitive conclusions regarding the efficacy of Zn from organic sources.
Recent advancements in chelating technology have led to the development of organic mineral forms using organic acids as ligands (Nazaran, 2012). Preliminary studies indicate that these organic mineral forms exhibit higher bioavailability than their inorganic counterparts in ruminants (Dehghan-Banadaky et al., 2021; Mousavi-Haghshenas et al., 2022) and poultry (Seyfori et al., 2019; Ghasemi et al., 2020, 2022). We propose that using this advanced organic chelated Zn source may help distinguish the specific effects of the organic Zn form itself, without the confounding influence of essential amino acids. We hypothesize that early-life Zn supplementation will enhance growth performance, solid feed intake, and nutrient digestibility during the pre-weaning period by promoting rumen development and preventing diarrhea. Furthermore, we expect that the magnitude of these responses will vary depending on the Zn source, with the organic chelated form potentially showing superior effects compared to inorganic source. Therefore, the present study aimed to investigate the effects of early-life supplementation with different forms of Zn on body weight (BW) gain, starter intake (SI), blood metabolites, and apparent total tract digestibility (ATTD) of nutrients in Holstein dairy calves from birth until weaning.
2 Materials and methods
2.1 Animals and housing
The present study was conducted at Pegah-E-Hamadan Dairy Farm, a commercial dairy operation located in Hamadan, Iran (36°16’16”N, 48°31’18”E). All procedures involving animals were conducted in accordance with the guidelines set by Iranian Council of Animal Care (1995). A total of 40 newborn Holstein heifer calves (birth weight of 36.94 ± 3.14 kg) born between June and July 2021were enrolled in this study. The experiment lasted until the end of September 2021, when all calves were weaned at 70 days of age. Within 2 hours after birth, calves were separated from their dams and housed in individual indoor pens (1 m wide × 2 m deep). Within 24 hours, each calf received colostrum from its dam. The first colostrum was fed as soon as possible after birth, with a minimum 4 L provided within the initial 12 hours. Additionally, calves received intramuscular injections of a vitamin A, D3, and E supplement (2 mL) and selenium (1 mL; Rooyan Darou, Tehran, Iran). From 3 days old until weaning at 70 days, calves were transferred to outdoor individual pens (1 m wide × 3 m deep) that prevented physical contact but allowed visual and auditory interaction with each other, not with their dams. The average ambient temperature during this period was 38°C (maximum 43°C, minimum 32°C), with relative humidity ranging from 15% to 22%. Pens were bedded with straw, which was replenished and replaced as needed. The inside of the pens was cleaned weekly to maintain proper sanitation.
2.2 Feeding and experimental treatments
Throughout the experimental period, all calves received fresh whole milk according to the following structured feeding protocol: calves received 1.5 L of milk per feeding from days 2 to 14, increased to 3 L per feeding from days 15 to 50, and then reduced to 2 L per feeding from days 51 to 63. During week 10 (days 64-70), the milk quantity was progressively decreased daily to facilitate weaning, with complete weaning achieved by day 70. From d 3 to d 14, calves received fresh whole milk that was either un-supplemented (CON) or supplemented with 80 mg/day zinc from one of three sources: zinc sulfate heptahydrate (0.35 g ZnSO4·7H2O, Merck; Zn-Sul), chelated zinc (0.5 g Bonza® Zn, Sodour Ahrar Shargh, Iran; Zn-Bon), or zinc methionine (0.45 g Zinpro® 180, Zinpro, US; Zn-Met). This zinc supplementation level was selected based on previous studies (Chang et al., 2020; Wo et al., 2022). Zinc supplements were individually mixed into the morning milk meal of each calf before being offered to the calves. All calves had free access to a commercial starter feed (Table 1) formulated to meet nutrient requirements according to (NRC, 2001) recommendations from 5 days of age. Fresh water was also made freely available to the calves.
2.3 Data collection
Body weights were recorded at birth and then fortnightly before morning milk feeding. Average daily gain (ADG) was estimated as the slope of body weight regressed on time. Offered starter and refusal were weighed daily to calculate SI and average daily starter intake (ADSI). Hip height, wither height, body length, heart girth, and hip width were recorded as body measurements on days 3 and 70 of age. Fecal consistency was scored daily during first 21 days of life by two trained technicians using the following scale (Larson et al., 1977): 1 = normal (firm but not hard); 2 = soft (does not hold form, piles but spread slightly); 3 = runny (spreads readily); 4 = watery (liquid consistency, splatters). The following equation was used to calculate the fecal consistency index (FCI) as described by (Salazar et al., 2019) to provide an integrated measure of fecal fluidity over the observation period.
Where DS1, DS2, DS3, and DS4 represent the number of days with fecal scores of 1, 2, 3, and 4, respectively, and TD is the total number of observation days. Higher values indicate looser fecal consistency. Moreover, intravenous fluid therapy and antibiotic injections administered to calves following a veterinarian’s recommendations were recorded during the first 21 days of age and analyzed as the frequency of medical treatment (FMT) data.
2.4 Sample collection and analyses
Blood samples were collected on d 3 (as a baseline), 15, and 70 of age before the morning milk feeding. Serum was separated by centrifugation at 3000 g for 10 minutes and storied at -20°C until analysis. The serum concentrations of glucose (Glu), blood urea nitrogen (BUN), triglycerides (TG), total protein (TP), Albumin (Alb), aspartate aminotransferase (AST), and alanine aminotransferase (ALT) were determined in the serum samples using commercial kits (Pars Azmoun, Iran) according to manufacturer’s instructions. The concentration of globulins (Glob) was calculated as difference between TP and Alb concentrations.
Fecal samples were collected from all calves via rectal palpation twice daily on days 66 (0800 and 1200 h), 67 (0900 and 1300 h), 68 (1000 and 1400 h), and 69 (1100 and 1500 h) of age. Additionally, samples were obtained from the starter feed and refusals during these four days. For each calf, fecal, starter feed, and refusal samples were dried at 70°C for 48 hours to determine dry matter (DM). All samples were then ground through a mill equipped with a 2 mm screen, and subsamples were subjected to chemical analysis. Organic matter (OM) was determined by ashing the samples at 600°C for 6 h. Neutral detergent fiber (NDF) was determined with α-amylase and excluding residual ash, but without sodium sulfite (Van Soest et al., 1991). Acid detergent fiber (ADF) was also determined following the method of Van Soest et al. (1991). Starch content was determined using the perchloric acid method as described by Rose et al. (1991). Acid-insoluble ash was used as an internal marker to calculate nutrient digestibility (Block et al., 1981). All analyses were performed in triplicate.
2.5 Statistical analysis
Data were subjected to analysis of variance (ANOVA) using PROCMIXED procedure of SAS (SAS 9.4). For repeated measures (BW, SI, ADSI, FCI, and blood metabolites), the model included the fixed effects of treatment, time and their interaction as well as the random effect of individual animal within treatment. Birth BW (for BW and ADG) and mid-test metabolic BW (for SI and ADSI) were included in the model as covariates. Baseline values (measured on d 3 of age) were also used as covariates factors for body measurements and blood metabolites. Data for ATTD were analyzed using the same model without the main effect of time and interactions, and ADSI during the sampling period was included in the model as a covariate. Two variance-covariance structures (auto-regressive type 1 and compound symmetry) were tested and the covariance structure with the best fit according Schwarz’s Bayesian information criterion was chosen. The analysis of medical treatment data was performed using a mixed-effects logistic regression model with PROC GLIMMIX procedure of SAS. The model included experimental treatment as a fixed effect and calf as a random effect. Treatment comparisons were conducted by comparing least square means using pairwise differences (pdiff) at a significance level of P < 0.05. Significance was declared when P < 0.05, while comparisons with 0.05 ≤ P ≤ 0.10 were presented as tendencies. When interactions were statistically significant or showed a tendency toward significance, the results are presented graphically.
3 Results
3.1 Growth rate, starter intake, and body measurements
The results of growth performance are summarized in Table 2. No significant differences were observed among the experimental groups for initial BW (P = 0.536). Final BW in Zn-Bon calves was numerically higher than other groups, but this difference was not statistically significant (P = 0.240). Similarly, the highest ADG and BW were observed in Zn-Bon calves, yet these differences among the experimental groups did not reach statistical significance (P > 0.10). The main effect of time on BW was significant (P < 0.01), and there was a tendency for a significant interaction between treatment and time for BW (P = 0.104). As shown in Figure 1, variations in BW among the experimental groups became more pronounced on days 56 and 70, with Zn-Bon group exhibiting the highest values and CON group the lowest.
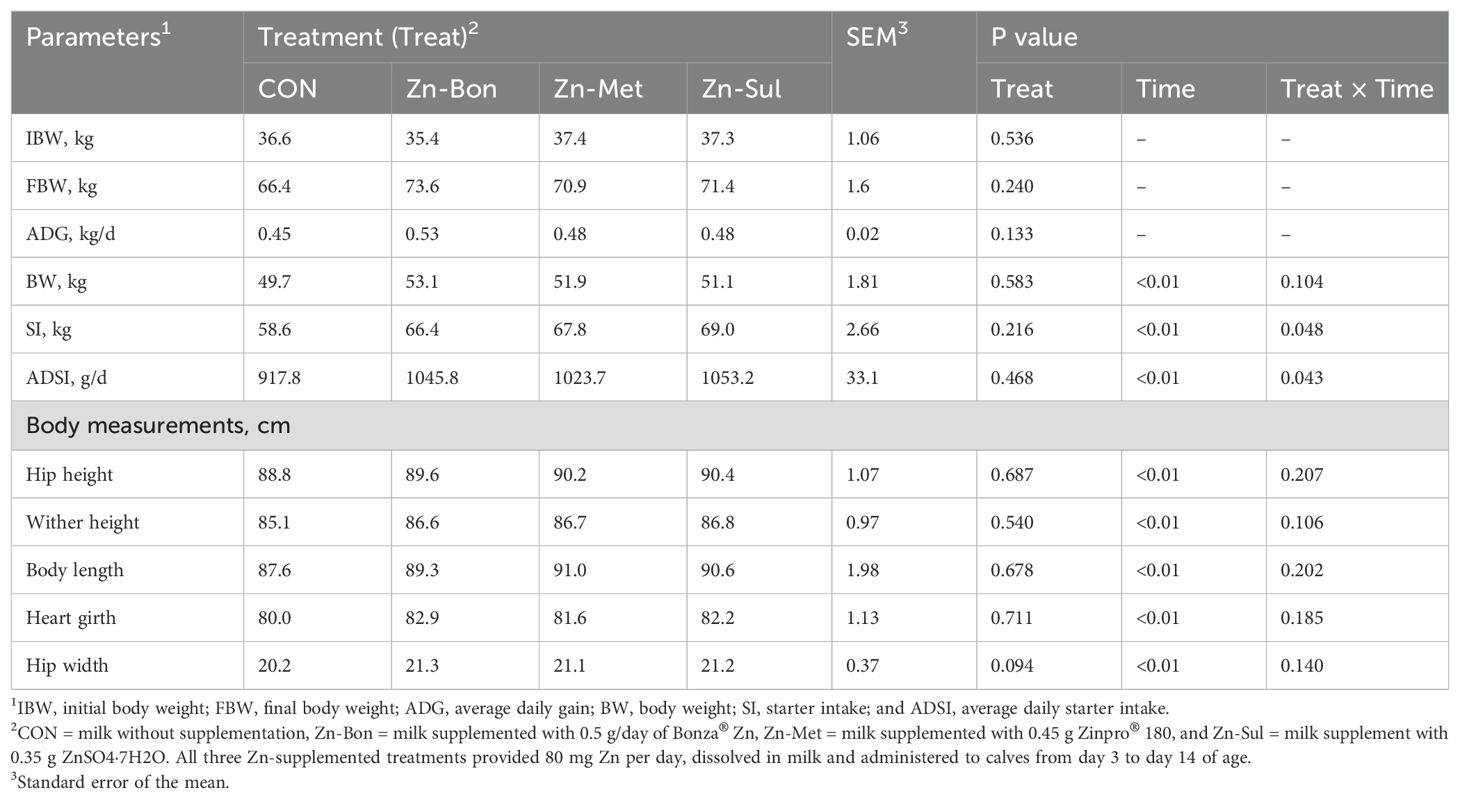
Table 2. Effects of milk supplementation with different zinc sources on body weight gain, starter intake, and body measurements in dairy calves.
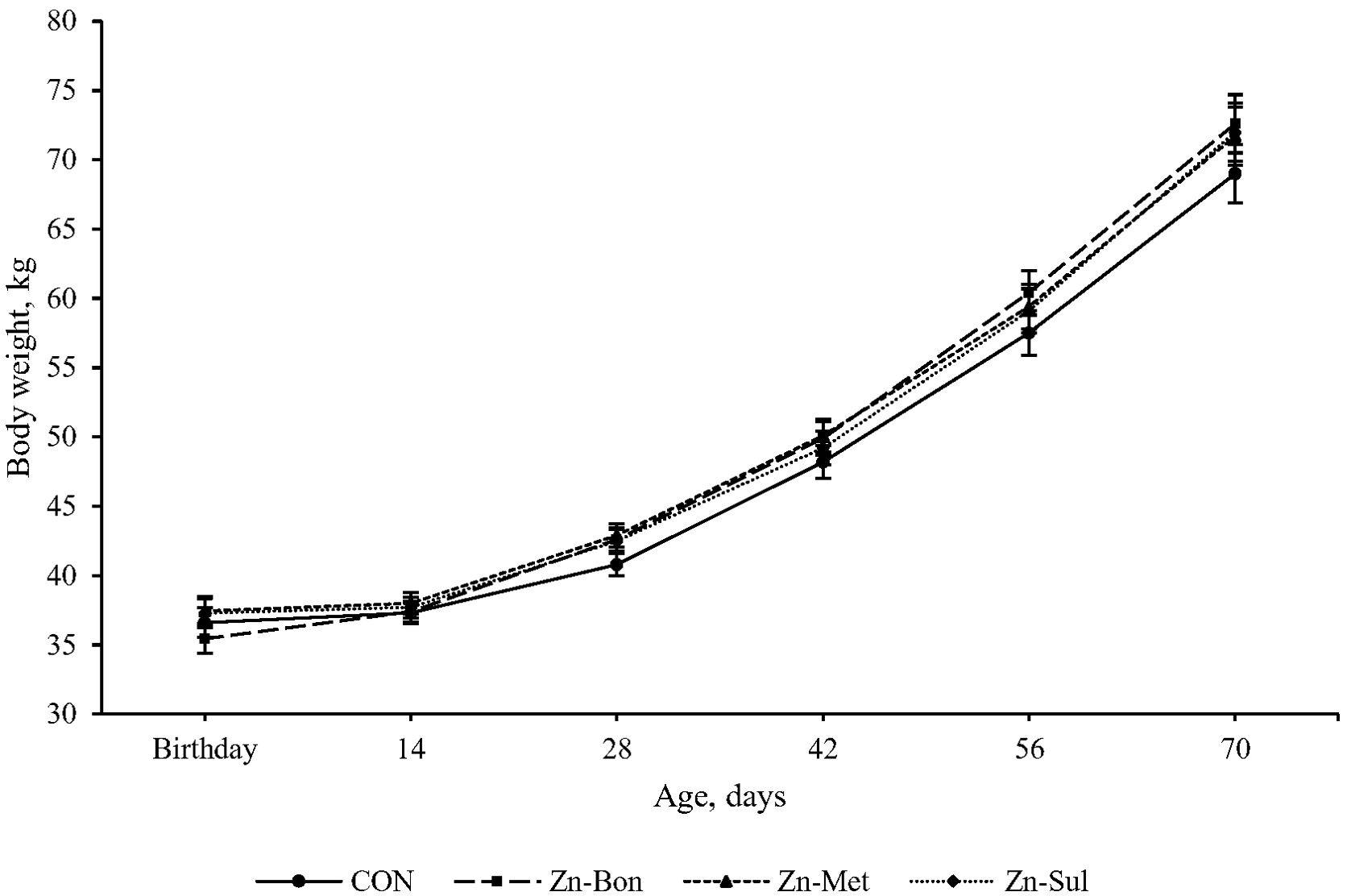
Figure 1. Effect of milk supplementation with different zinc sources on body weight in calves during the pre-weaning period. CON = milk without supplementation, Zn-Bon = milk supplemented with 0.5 g/day of Bonza® Zn, Zn-Met = milk supplemented with 0.45 g Zinpro® 180, and Zn-Sul = milk supplement with 0.35 g ZnSO4·7H2O. All three Zn-supplemented treatments provided 80 mg Zn per day, dissolved in milk and fed to calves from day 3 through day 14 of age.
Statistical analysis indicated no significant main effects of treatment on SI (P = 0.216) and ADSI (P = 0.468). However, significant interactions between time and treatment were detected for both ADSI (P = 0.048) and SI (P = 0.043). Figures 2 and 3 present the cumulative SI and ADFI of calves throughout the pre-weaning period, respectively. Calves in the Zn-Bon group showed higher SI and ADSI than the CON group throughout the experimental period, while differences in SI between CON and Zn-Met, as well as between CON and Zn-Sul, reached significance at days 56 and 70. Furthermore, no significant differences were detected among CON, Zn-Met, and Zn-Sul groups for ADSI, except from day 56 to day 70, where ADSI was higher in both Zn-Met and Zn-Sul groups compared to CON (P < 0.05).
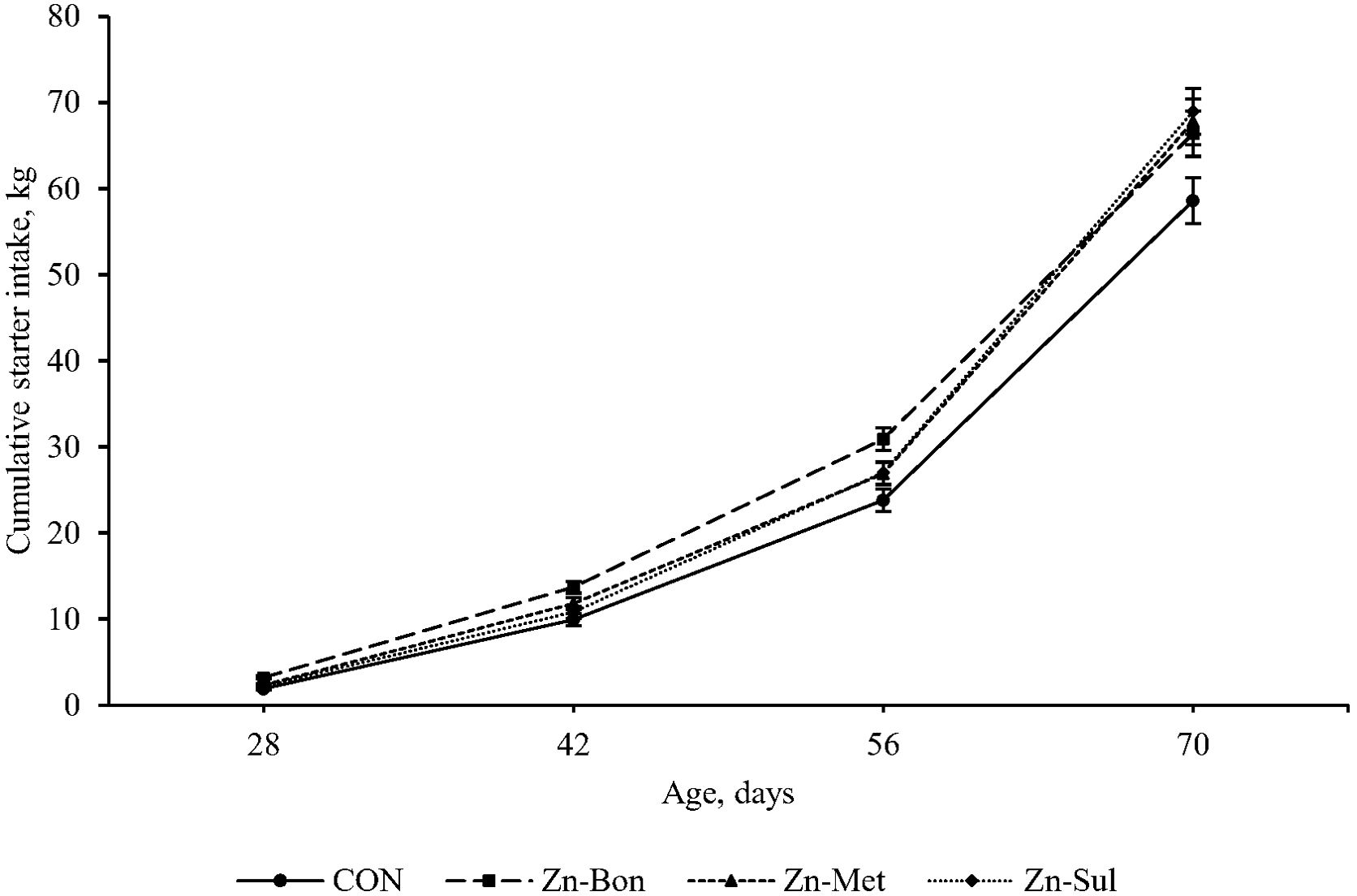
Figure 2. Effect of milk supplementation with different zinc sources on starter intake in calves during the pre-weaning period. CON = milk without supplementation, Zn-Bon = milk supplemented with 0.5 g/day of Bonza® Zn, Zn-Met = milk supplemented with 0.45 g Zinpro® 180, and Zn-Sul = milk supplement with 0.35 g ZnSO4·7H2O. All three Zn-supplemented treatments provided 80 mg Zn per day, dissolved in milk and fed to calves from day 3 through day 14 of age.
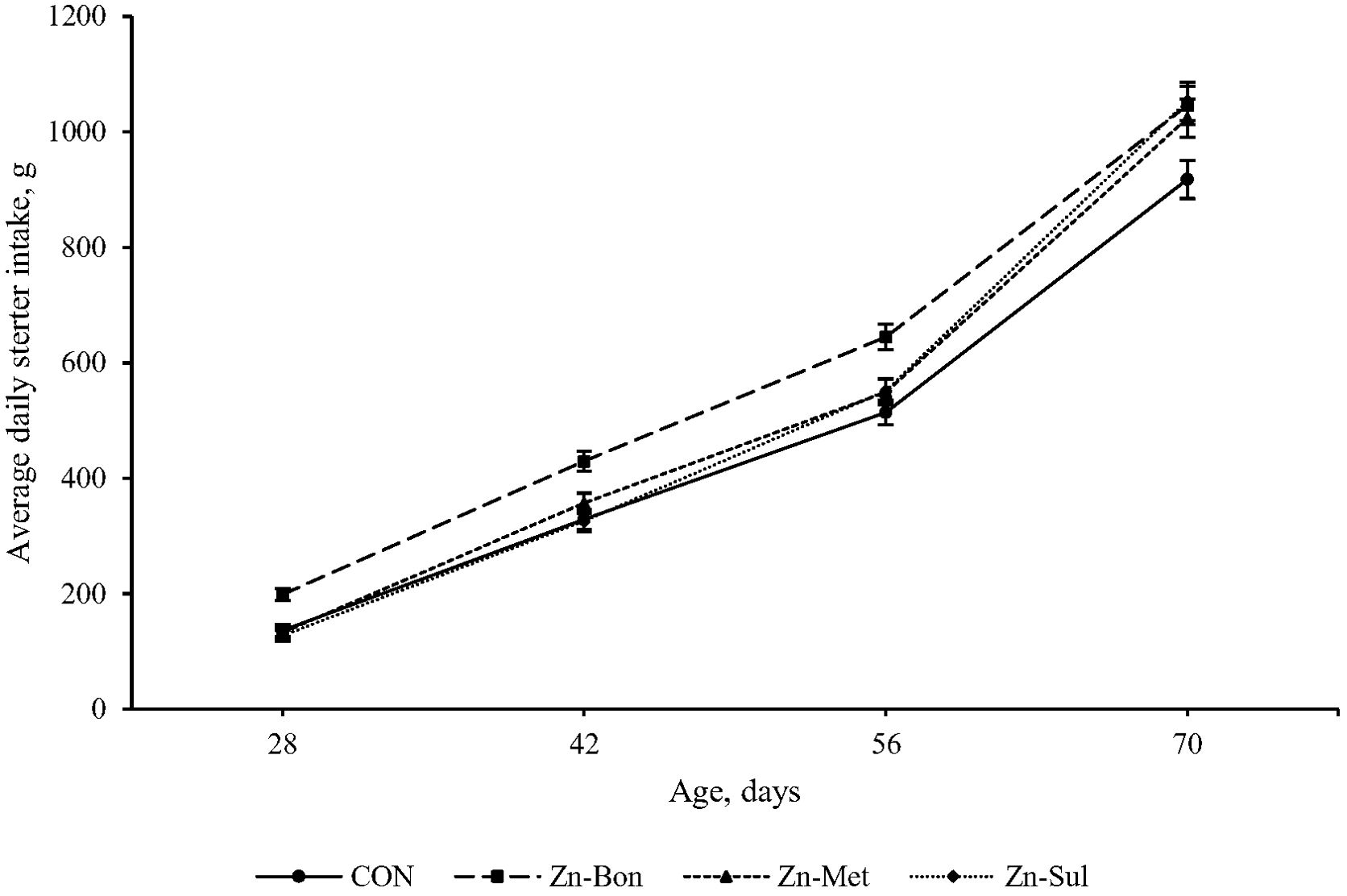
Figure 3. Effect of milk supplementation with different zinc sources on average daily starter intake in calves during the pre-weaning period. CON = milk without supplementation, Zn-Bon = milk supplemented with 0.5 g/day of Bonza® Zn, Zn-Met = milk supplemented with 0.45 g Zinpro® 180, and Zn-Sul = milk supplement with 0.35 g ZnSO4·7H2O. All three Zn-supplemented treatments provided 80 mg Zn per day, dissolved in milk and fed to calves from day 3 through day 14 of age.
The results of body measurements are presented in Table 2. Calves in the Zn-Sul group exhibited the highest numerical values for hip height and wither height. Body length and hip width were numerically greater in the Zn-Bon group compared to other groups. The highest heart girth measurement was observed in Zn-Met calves. Despite numerically lower values for all body measurements in the CON compared to the zinc-supplemented calves, no statistically significant differences were detected among the experimental treatments for these parameters (P > 0.05).
3.2 Fecal consistency and medical treatment
FCI significantly decreased from week 1 to week 3 of age (P < 0.05; Figure 4). Although Zn supplementation, particularly in the Zn-Bon group, numerically reduced FCI in calves during the first 21 days of age, no significant differences were observed among the experimental treatments (P > 0.10). Moreover, the results indicated that milk supplementation with Zn-Bon or Zn-Sul significantly reduced the frequency of medical treatments during the first 21 days of life compared to the CON group (P < 0.05; Figure 5). Although Zn-Met showed a tendency to reduce the frequency of medical treatments, there was no significant difference between the CON and Zn-Met groups (P > 0.05).
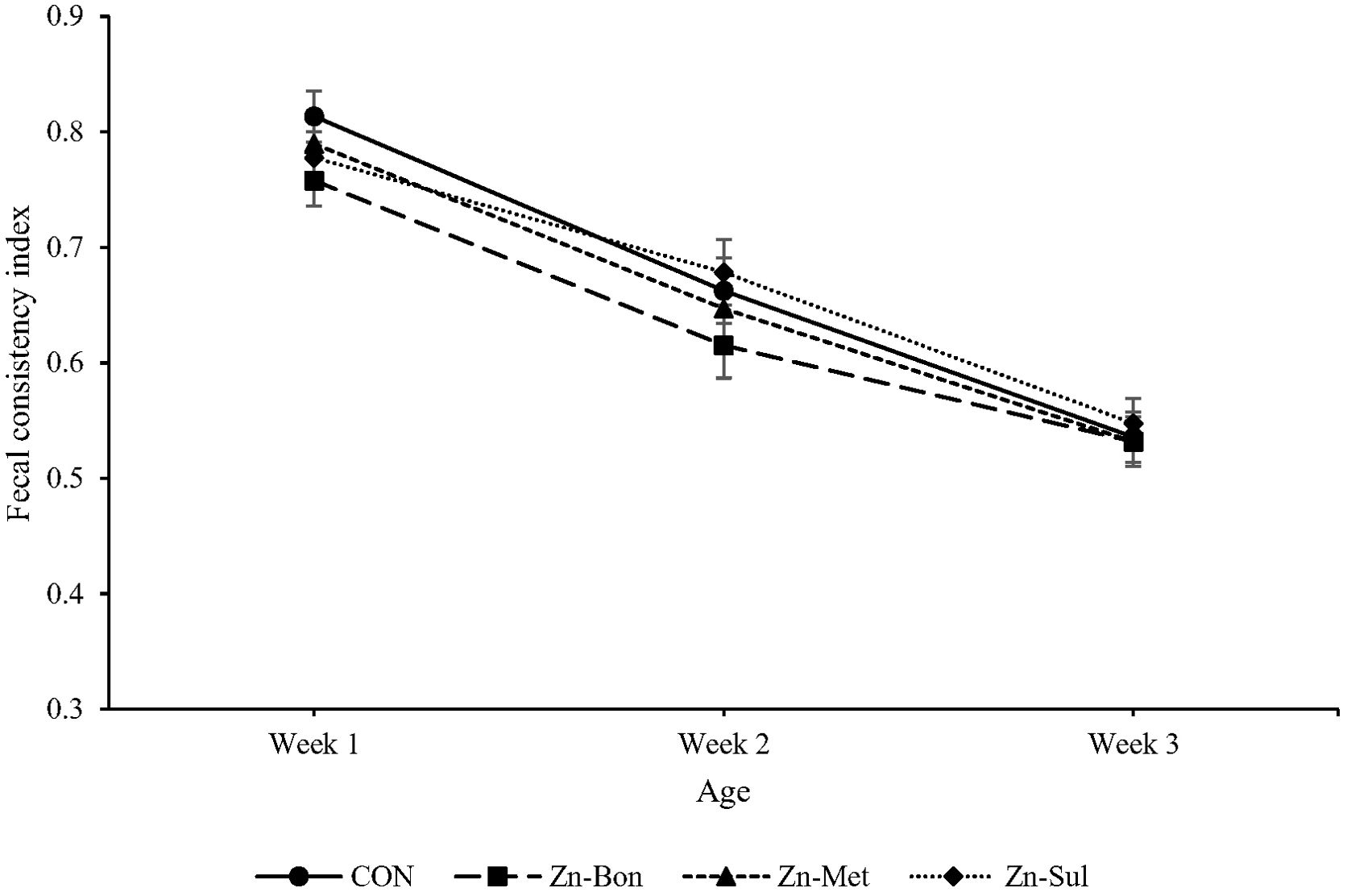
Figure 4. Effect of milk supplementation with different zinc sources on fecal consistency index in calves during the first three weeks of life. CON = milk without supplementation, Zn-Bon = milk supplemented with 0.5 g/day of Bonza® Zn, Zn-Met = milk supplemented with 0.45 g Zinpro® 180, and Zn-Sul = milk supplement with 0.35 g ZnSO4·7H2O. All three Zn-supplemented treatments provided 80 mg Zn per day, dissolved in milk and fed to calves from day 3 through day 14 of age.
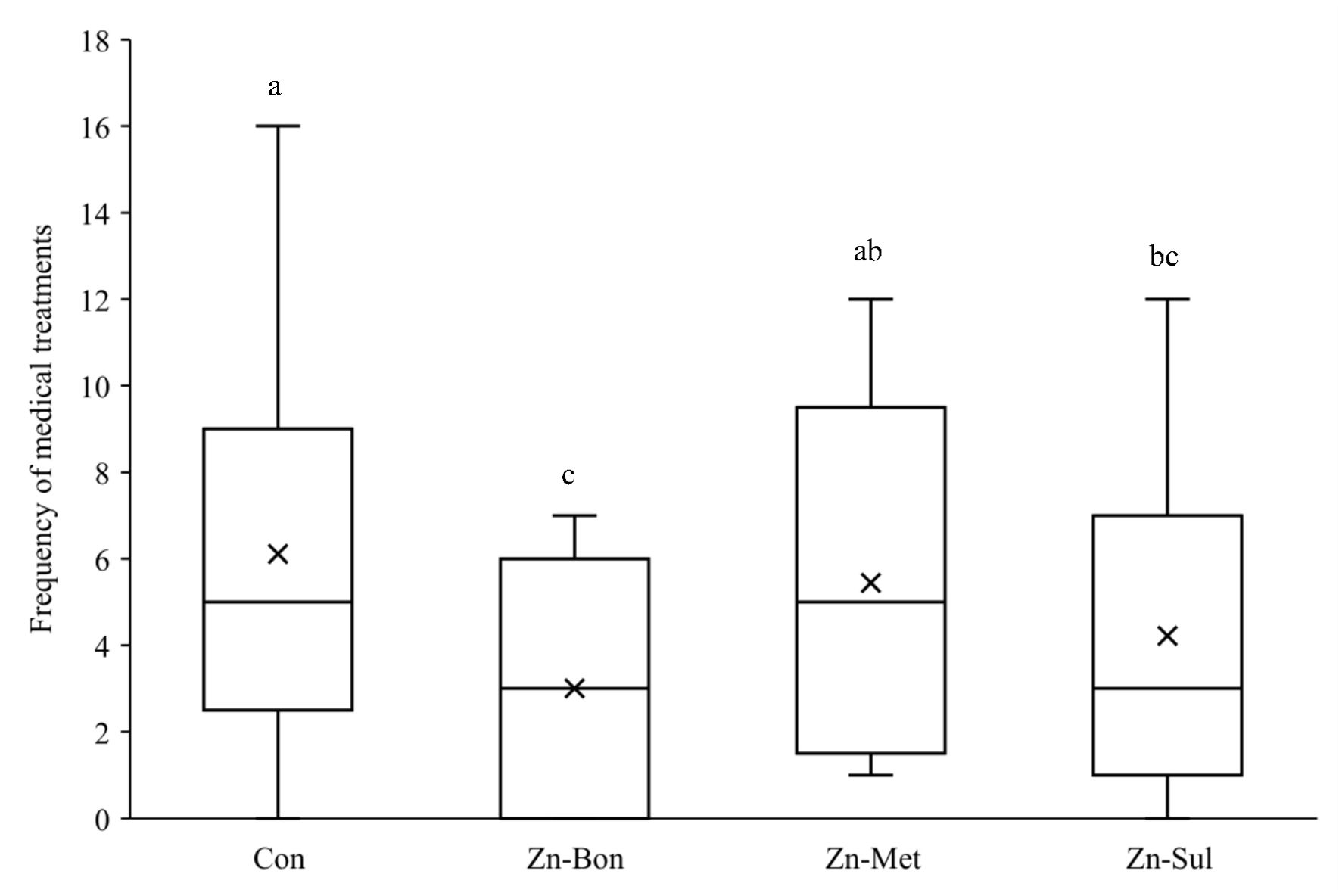
Figure 5. Effect of milk supplementation with different zinc sources on frequency of medical treatment in calves during the first three weeks of life. CON = milk without supplementation, Zn-Bon = milk supplemented with 0.5 g/day of Bonza® Zn, Zn-Met = milk supplemented with 0.45 g Zinpro® 180, and Zn-Sul = milk supplement with 0.35 g ZnSO4·7H2O. All three Zn-supplemented treatments provided 80 mg Zn per day, dissolved in milk and fed to calves from day 3 through day 14 of age. a,b,c Treatments not sharing a common superscript letter differ significantly (P < 0.05).
3.3 Blood metabolites and nutrient digestibility
Statistical analysis did not show any significant differences in the serum parameters among the experimental groups on days 15 and 70 of age (Table 3). However, the effect of time was significant for serum concentrations of Glu, BUN, TP, Alb, Glob, and ALT. Additionally, there was a tendency for a time effect on serum TG concentration. No significant treatment by time interactions were observed for any of the measured blood metabolites. As presented in Table 4, differences among the experimental group for ATTD of DM, OM, NDF, and starch tended to be significant. The highest digestibility values were observed in the Zn-Bon group, followed by the Zn-Met and Zn-Sul.
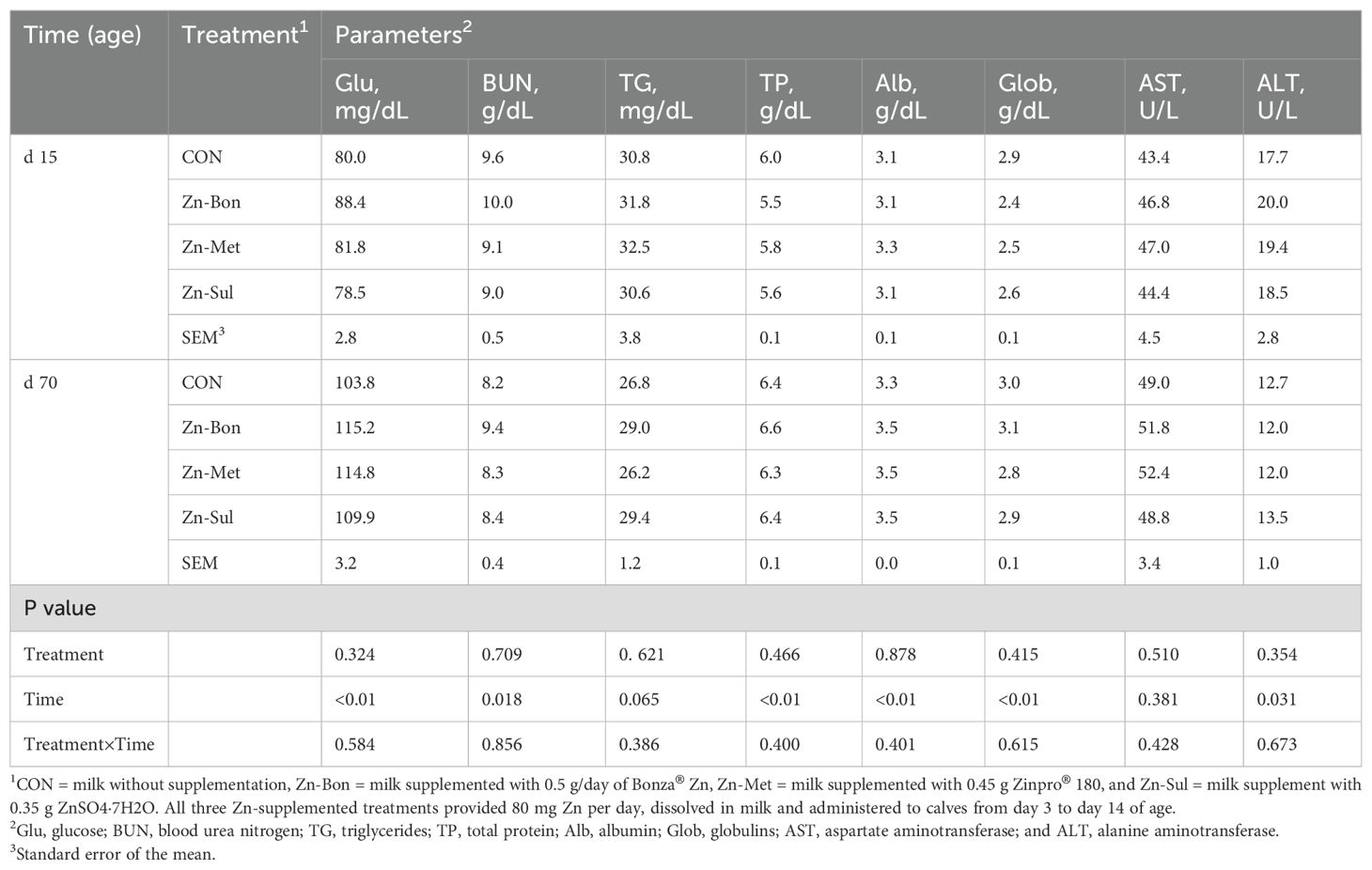
Table 3. Effects of milk supplementation with different zinc sources on serum concentrations of blood metabolites in dairy calves.
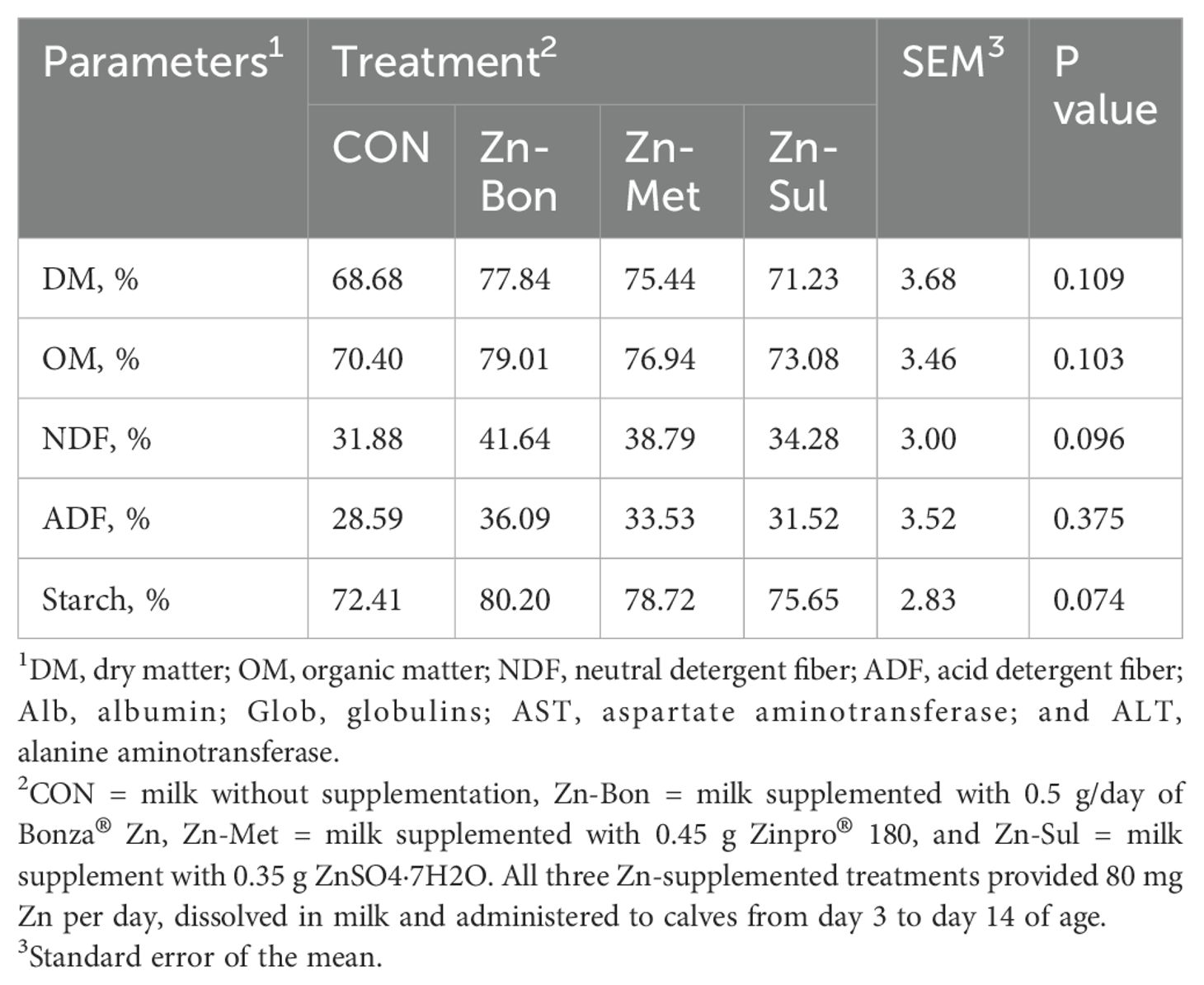
Table 4. Effects of milk supplementation with different zinc sources on apparent total tract digestibility of nutrient in dairy calves during days 66 to 69 of age.
4 Discussion
Although the Zn concentration in the milk used in this study was not directly measured, the daily Zn intake for the CON group from days 3 to 14 can be estimated at approximately 9 to 15 mg/day. This estimation is based on previous reports indicating that whole milk contains 3 to 5 mg/L of Zn (Arrayet et al., 2002; Chapelain et al., 2023). Furthermore, ADSI during the first two weeks of life was less than 25 g/day, resulting in negligible zinc intake through the starter feed compared to the supplemental Zn provided in the experimental groups. The minimal contribution of Zn from starter feed during this period further emphasizes the importance of milk as the primary Zn source for neonatal calves and highlights the potential efficacy of milk-based Zn supplementation strategies.
Zn supplementation at a rate of 80 mg/day slightly improved the growth rate and body measurements of dairy calves, especially in the Zn-Bon group. However, these effects were non-significant. Previous studies have reported that Zn supplementation of whole milk (Arrayet et al., 2002) or milk replacer above the NRC recommendations (Jenkins and Hidiroglou, 1991) had no significant effect on the growth performance of dairy calves. For instance, Arrayet et al. (2002) supplemented whole milk with 60 mg/L of Zn from various sources (Zn-sulfate, Zn-lysine, and Zn-methionine) for dairy calves and reported no significant effect on growth rate or skeletal development. In contrast, recent studies, primarily focused on the diarrhea-preventing effect of short-term Zn supplementation in milk for dairy calves, have shown that whole milk supplemented with 80 mg Zn has the potential to reduce diarrhea incidence and improve ADG in dairy calves (Feldmann et al., 2019; Chang et al., 2020; Ma et al., 2020; Wo et al., 2022). However, the growth performance response to Zn supplementation has been shown to be source- or sex-dependent. Feldmann et al. (2019) investigated the effect of milk supplemented with 80 mg of Zn in the form of Zn-methionine or Zn-sulfate on newborn dairy calves up to 14 days of age. They reported a significant interaction between Zn supplement and sex for growth rate; male calves in the Zn-methionine group exhibited a 22 g/d higher (P = 0.042) growth rate than the un-supplemented control group, while female calves had a lower daily growth rate of 9 g/d (P = 0.037) compared to the control group. Chang et al. (2020) conducted a similar study in which dairy calves received 80 mg/day of Zn as Zn-methionine or Zn-oxide dissolved in milk from the third day to 14 days of age. Their results indicated that Zn-methionine significantly improved the growth rate (0 to 14 days) compared to the control group. They suggested that the higher growth rate in the Zn-methionine group compared to the control group, and the lack of significant difference between the control group and Zn-oxide group, may be attributed to the essential amino acid methionine present in Zn-methionine.
However, our results showed a trend toward a significant interaction effect between treatment and time for BW, as the difference in BW between CON and the Zn-supplemented groups was more evident from day 56 onward. Although this positive effect of Zn supplementation may be partly attributed to improved health status of calves, these findings highlight the complexity of Zn supplementation in dairy calves and underscore the need for further research. Future studies should elucidate the mechanisms by which different forms of Zn, particularly in combination with essential amino acids or organic acids, influence growth and development in dairy calves during pre- and post-weaning periods. Elucidating the mechanisms governing the bioavailability of different zinc forms is essential for optimizing supplementation strategies. Zinc chelates, such as Bonza®Zn, may exhibit increased bioavailability due to improved absorption and utilization. Investigations into the specific absorption pathways and interactions of zinc with other nutrients are necessary to refine and enhance zinc supplementation protocols.
Promoting rumen development and starter intake in dairy calves is crucial for the transition from a milk-based diet to solid feed, as well as for optimal growth, health, and long-term productivity. Stamey et al. (2012) demonstrated a strong correlation (R² = 0.732) between pre-weaning starter intake and post-weaning weight gain. Despite this, the effect of early-life Zn supplementation from various sources on the starter intake of calves across per-weaning period has received less attention in previous studies. However, the role of zinc in epithelial and mucosal health suggests that it may contribute to more efficient rumen development and function. Chang et al. (2020) observed no significant difference in solid feed intake between the control group and those received zinc methionine or zinc oxide during the first 14 days of life. Similar results were also reported in calves received either Zn methionine or Zn proteinate during the first two or four weeks of life (Ma et al., 2020; Wo et al., 2022). Consistent with our findings, Osorio et al. (2012) reported significant differences in feed intake among calves fed either organic or inorganic sources of minerals, including Zn, between weeks 3 and 8 of age. However no significant differences in solid feed intake were found during the first two weeks or at week 9. Similarly, in our study, differences among CON, Zn-Met, and Zn-Sul remained non-significant until d 56, with significant differences detected only on d 70. These findings indicate that measuring solid feed intake should be conducted over an extended period, ideally starting from two weeks of age, as its role in meeting the nutritional needs of calves is minimal before this time. However, the increased in ADSI between Zn-Bon and CON were detected from d 28, indicating the higher potential of Zn-Bon to promote solid feed intake compared to Zn-Met and Zn-Sul.
The increased starter intake and tendency toward higher nutrient digestibility observed in Zn-supplemented claves, especially in Zn-Bon group, may be attributed to the positive effect of Zn on rumen development. In young calves, the digestibility of starter feed is dependent on the development of ruminal fermentation and overall gastrointestinal functionality. It has been suggested that the digestibility of NDF is influenced by rumen development and fermentative capacity (Chapman et al., 2017), while starch digestion is affected by both ruminal fermentation and enzymatic activity in the intestines (Harmon, 2009). The positive effects of Zn-Bon on SI and nutrient digestibility suggest that this zinc source may have higher bioavailability or biological activity, potentially contributing to enhanced growth and development in pre-weaned dairy calves. Previous studies have demonstrated that different zinc sources can variably affect rumen epithelial development. For instance, Cernik et al. (2013) found that zinc oxide stimulates greater rumen epithelial development compared to other zinc sources, including zinc complexed with amino acids and zinc-lactate. Conversely, Ma et al. (2020) reported higher efficiency of Zn methionine on intestinal epithelium integrity compared to zinc oxide. Future studies should investigate the efficacy of milk supplementation with low doses of various zinc sources, particularly those manufactured using chelate compound technology, on rumen development and functionality. Research could focus on histological examinations of the rumen epithelium and measurements of rumen fermentation parameters to determine how zinc supplementation from various sources influences these aspects.
The immune-boosting properties of zinc, particularly its role in enhancing immunoglobulin levels, are well-documented (Aggarwal et al., 2007; Tomasi et al., 2018; Wo et al., 2022). Investigating how different zinc sources influence immune markers in calves could help tailor supplementation protocols to maximize health benefits, especially in the critical early stages of life. In the present study, we observed a decline in FCI from week 1 to week 3, with Zn-Bon showing numerically lower FCI compared to CON. This trend aligns with the typical pattern of diarrhea occurrence in dairy calves, which is most prevalent during the first three weeks of life and decreases as calves age. Notably, our results indicated that milk supplementation with Zn-Bon or Zn-Sul significantly reduced the frequency of medical treatments required during the first 21 days of life compared to CON. Previous research has highlighted the potential benefits of supplementing milk with 80 mg/day of zinc from various sources during the first 14 days of life for diarrhea prevention and overall health in dairy calves. Feldmann et al. (2019) and Chang et al. (2020) reported significant reductions in diarrhea incidence when using zinc oxide, zinc sulfate, or zinc methionine as supplements. Corroborating these findings, Ma et al. (2020) and Wo et al. (2022) observed similar positive effects when supplementing milk with zinc methionine or zinc proteinate. This preventive effect is primarily attributed to enhanced immune responses and elevated blood immunoglobulin levels (Tomasi et al., 2018; Chang et al., 2020; Wo et al., 2022). Moreover, Ma et al. (2020) indicated that zinc methionine specifically contributes to protecting the intestinal epithelial barrier in postnatal dairy calves, further elucidating its anti-diarrheal mechanism. However, it is important to note that our study did not demonstrate a significant effect of various zinc sources on FCI, contrary to findings in the previous studies. This discrepancy may be attributed to differences in experimental design, including sample size, rearing conditions, management practices, and the nutritional status of the dams.
5 Conclusion
In conclusion, this study demonstrates that early-life zinc supplementation in milk for dairy calves, particularly with the organic chelated form (Zn-Bon), can positively influence starter intake during the pre-weaning period, with more pronounced effects observed in the second month of life. However, we found minimal impact of the Zn supplementation on body weight gain or skeletal measurements. Despite the absence of significant differences in FCI among the experimental groups, the frequency of medical treatments required during the first three weeks of life decreased with Zn supplementation, with Zn-Bon showing the most substantial impact. Furthermore, the observed tendency for improved nutrient digestibility in zinc-supplemented calves indicates potential benefits for rumen development. Further research is warranted to elucidate the underlying mechanisms of zinc supplementation, particularly organic chelated forms, on rumen development, nutrient digestibility, and overall health of dairy calves during both pre- and post-weaning periods. Although our study focused on the pre-weaning period, the long-term effects of early-life zinc supplementation on post-weaning growth, health, and productivity are not well understood. Longitudinal studies following calves through to adulthood would provide valuable data on the lasting impacts of zinc supplementation. The interactions of zinc with other micronutrients, such as copper and vitamin A, can influence its effectiveness. Investigating these interactions could lead to more balanced and effective supplementation strategies that optimize overall mineral nutrition.
Data availability statement
The raw data supporting the conclusions of this article will be made available by the authors, without undue reservation.
Ethics statement
The animal study was approved by the Animal Care and Use Committee of the Department of Animal Science, Malayer University, Malayer, Iran (March 7, 2021). The study was conducted in accordance with the local legislation and institutional requirements.
Author contributions
HR: Conceptualization, Formal Analysis, Supervision, Validation, Writing – review & editing. ES: Investigation, Writing – review & editing. MH: Conceptualization, Resources, Writing – review & editing. SM: Investigation, Writing – review & editing. ZS: Investigation, Writing – review & editing. YA: Writing – original draft. AS: Validation, Writing – review & editing.
Funding
The author(s) declare that no financial support was received for the research, authorship, and/or publication of this article.
Acknowledgments
The authors thank Malayer University, Malayer, Iran for providing suitable laboratory facilities. The authors express their kind appreciation to the farm staff at Pegah-E-Hamadan Dairy Farm, Hamadan, Iran for diligent animal care and their help in conducting this experiment. The technical support provided by the Department of Research and Development at Sodour Ahrar Shargh Company, Tehran, Iran is gratefully acknowledged.
Conflict of interest
The authors declare that the research was conducted in the absence of any commercial or financial relationships that could be construed as a potential conflict of interest.
Publisher’s note
All claims expressed in this article are solely those of the authors and do not necessarily represent those of their affiliated organizations, or those of the publisher, the editors and the reviewers. Any product that may be evaluated in this article, or claim that may be made by its manufacturer, is not guaranteed or endorsed by the publisher.
References
Aggarwal R., Sentz J., Miller M. A. (2007). Role of zinc administration in prevention of childhood diarrhea and respiratory illnesses: A meta-analysis. Pediatrics 119, 1120–1130. doi: 10.1542/peds.2006-3481%JPediatrics
Arrayet J., Oberbauer A., Famula T., Garnett I., Oltjen J., Imhoof J., et al. (2002). Growth of Holstein calves from birth to 90 days: The influence of dietary zinc and BLAD status. J. Anim. Sci. 80, 545–552. doi: 10.2527/2002.803545x
Baldwin R. L., McLeod K. R., Klotz J. L., Heitmann R. N. (2004). Rumen development, intestinal growth and hepatic metabolism in the pre- and postweaning ruminant. J. Dairy Sci. 87, E55–E65. doi: 10.3168/jds.S0022-0302(04)70061-2
Block E., Kilmer L., Muller L. (1981). Acid insoluble ash as a marker of digestibility for sheep fed corn plants or hay and for lactating dairy cattle fed hay ad libitum. J. Anim. Sci. 52, 1164–1169. doi: 10.2527/jas1981.5251164x
Cernik J., Pavlata L., Pechova A., Misurova L., Jokverova O., Lunacek J., et al. (2013). Effects of peroral supplementation of different forms of zinc on the ruminal mucosa of goat kids - A morphometric study. Acta Vet. Brno. 82, 399–403. doi: 10.2754/avb201382040399
Chang M. N., Wei J. Y., Hao L. Y., Ma F. T., Li H. Y., Zhao S. G., et al. (2020). Effects of different types of zinc supplement on the growth, incidence of diarrhea, immune function, and rectal microbiota of newborn dairy calves. J. Dairy Sci. 103, 6100–6113. doi: 10.3168/jds.2019-17610
Chapelain T., Daniel J.-B., Wilms J., Martín-Tereso J., Leal L. (2023). Zinc, copper, manganese, and iron balance in dairy calves fed a milk replacer or whole milk at two feeding allowances. J. Dairy Sci. 106, Suppl. 1.
Chapman C. E., Hill T., Elder D., Erickson P. S. (2017). Nitrogen utilization, preweaning nutrient digestibility, and growth effects of Holstein dairy calves fed 2 amounts of a moderately high protein or conventional milk replacer. J. Dairy Sci. 100, 279–292. doi: 10.3168/jds.2016-11886
Dehghan-Banadaky M., Rajaei-Sharifabadi H., Hafizi M., Hashemi S. A., Kalanaky S., Fakharzadeh S., et al. (2021). Lactation responses of Holstein dairy cows to supplementation with a combination of trace minerals produced using the advanced chelate compounds technology. Trop. Anim. Health Prod 53, 55. doi: 10.1007/s11250-020-02539-5
Fairbrother J. M., Nadeau É., Gyles C. L. (2005). Escherichia coli in postweaning diarrhea in pigs: an update on bacterial types, pathogenesis, and prevention strategies. Anim. Health Res. Rev. 6, 17–39. doi: 10.1079/AHR2005105
Feldmann H. R., Williams D. R., Champagne J. D., Lehenbauer T. W., Aly S. S. (2019). Effectiveness of zinc supplementation on diarrhea and average daily gain in pre-weaned dairy calves: A double-blind, block-randomized, placebo-controlled clinical trial. PLoS One 14, e0219321. doi: 10.1371/journal.pone.0219321
Ghasemi H. A., Hajkhodadadi I., Hafizi M., Fakharzadeh S., Abbasi M., Kalanaky S., et al. (2022). Effect of advanced chelate compounds-based mineral supplement in laying hen diet on the performance, egg quality, yolk mineral content, fatty acid composition, and oxidative status. Food Chem. 366, 130636. doi: 10.1016/j.foodchem.2021.130636
Ghasemi H. A., Hajkhodadadi I., Hafizi M., Taherpour K., Nazaran M. H. J. N. (2020). Effect of advanced chelate technology based trace minerals on growth performance, mineral digestibility, tibia characteristics, and antioxidant status in broiler chickens. Nutr. Metab. 17, 1–12. doi: 10.1186/s12986-020-00520-5
Hambidge K. M., Krebs N. F. (2007). Zinc deficiency: A special challenge. J. Nutr. 137, 1101–1105. doi: 10.1093/jn/137.4.1101
Harmon D. L. (2009). Understanding starch utilization in the small intestine of cattle. Asian-Australas. J. Anim. Sci. 22, 915–922. doi: 10.5713/ajas.2009.r.08
Iranian Council of Animal Care (1995). Guide to the care and use of experimental animals Vol. 1 (Isfahan, Iran: Isfahan University of Technology).
Ishaq S. L., Page C. M., Yeoman C. J., Murphy T. W., Van Emon M. L., Stewart W. C. (2018). Zinc AA supplementation alters yearling ram rumen bacterial communities but zinc sulfate supplementation does not1. J. Anim. Sci. 97, 687–697. doi: 10.1093/jas/sky456%J
Jenkins K. J., Hidiroglou M. (1991). Tolerance of the preruminant calf for excess manganese or zinc in milk replacer. J. Dairy Sci. 74, 1047–1053. doi: 10.3168/jds.S0022-0302(91)78254-4
Kincaid R. J. (2000). Assessment of trace mineral status of ruminants: A review. J. Anim. Sci. 77, 1–10. doi: 10.2527/jas2000.77E-Suppl1x
Larson L., Owen F., Albright J., Appleman R., Lamb R., Muller L. (1977). Guidelines toward more uniformity in measuring and reporting calf experimental data. J. Dairy Sci. 60, 989–991. doi: 10.3168/jds.S0022-0302(77)83975-1
Ma F. T., Wo Y. Q. L., Shan Q., Wei J. Y., Zhao S. G., Sun P. (2020). Zinc-methionine acts as an anti-diarrheal agent by protecting the intestinal epithelial barrier in postnatal Holstein dairy calves. Anim. Feed Sci. Technol. 270, 114686. doi: 10.1016/j.anifeedsci.2020.114686
MacDonald R. S. (2000). The role of zinc in growth and cell proliferation. J. Nutr. 130, 1500s–1508s. doi: 10.1093/jn/130.5.1500S
Malmuthuge N., Liang G., Guan L. L. (2019). Regulation of rumen development in neonatal ruminants through microbial metagenomes and host transcriptomes. Genome. Biol. 20, 172. doi: 10.1186/s13059-019-1786-0
Mousavi-Haghshenas M. A., Hashemzadeh F., Ghorbani G. R., Ghasemi E., Rafiee H., Ghaffari M. H. (2022). Trace minerals source in calf starters interacts with birth weights to affect growth performance. Sci. Rep. 12, 18763. doi: 10.1038/s41598-022-23459-4
Nayeri A., Upah N. C., Sucu E., Sanz-Fernandez M. V., DeFrain J. M., Gorden P. J., et al. (2014). Effect of the ratio of zinc amino acid complex to zinc sulfate on the performance of Holstein cows. J. Dairy Sci. 97, 4392–4404. doi: 10.3168/jds.2013-7541
Nazaran M. H. (2012). Chelate Compounds (United States: United States Patent patent application US8288587B2).
NRC (2001). Nutrient Requirements of Dairy Cattle. 7th revised edition. Subcommittee on Dairy Cattle Nutrition, Committee on Animal Nutrition, National Research Council (Washington, DC: The National Academies Press).
Osorio J., Wallace R., Tomlinson D., Earleywine T., Socha M., Drackley J. (2012). Effects of source of trace minerals and plane of nutrition on growth and health of transported neonatal dairy calves. J. Dairy Sci. 95, 5831–5844. doi: 10.3168/jds.2011-5042
Rose R., Rose C. L., Omi S. K., Forry K. R., Durall D. M., Bigg W. L., et al (1991). Starch determination by perchloric acid vs enzymes: evaluating the accuracy and precision of six colorimetric methods. J. Agr.Food Chem 39, 2–11. doi: 10.1021/jf00001a001
Salazar L. F., Nero L. A., Campos-Galvão M. E., Cortinhas C. S., Acedo T. S., Tamassia L. F., et al. (2019). Effect of selected feed additives to improve growth and health of dairy calves. PloS One 14, e0216066. doi: 10.1371/journal.pone.0216066
Sales J. (2013). Effects of pharmacological concentrations of dietary zinc oxide on growth of post-weaning pigs: A meta-analysis. Biol. Trace Elem. Res. 152, 343–349. doi: 10.1007/s12011-013-9638-3
Seyfori H., Ghasemi H. A., Hajkhodadadi I., Hafizi M. J. P. S. (2019). Effects of water supplementation of anorganic acid-trace mineral complex on production and slaughter parameters, intestinal histomorphology, and macronutrient digestibility in growing ostriches. Poult. Sci. 98, 4860–4867. doi: 10.3382/ps/pez221
Spears J. W. (1996). Organic trace minerals in ruminant nutrition. Anim. Feed Sci. Technol. 58, 151–163. doi: 10.1016/0377-8401(95)00881-0
Stamey J., Janovick N., Kertz A., Drackley J. (2012). Influence of starter protein content on growth of dairy calves in an enhanced early nutrition program. J. Dairy Sci. 95, 3327–3336. doi: 10.3168/jds.2011-5107
Tomasi T., Volpato A., Pereira W., Debastiani L., Bottari N., Morsch V., et al. (2018). Metaphylactic effect of minerals on the immune response, biochemical variables and antioxidant status of newborn calves. J. Anim. Physiol. Anim. Nutr. 102, 819–824. doi: 10.1111/jpn.12890
Van Soest P.v., Robertson J. B., Lewis B. A. (1991). Methods for dietary fiber, neutral detergent fiber, and nonstarch polysaccharides in relation to animal nutrition. J. Dairy Sci. 74, 3583–3597. doi: 10.3168/jds.S0022-0302(91)78551-2
Wo Y., Jin Y., Gao D., Ma F., Ma Z., Liu Z., et al. (2022). Supplementation with zinc proteinate increases the growth performance by reducing the incidence of diarrhea and improving the immune function of dairy calves during the first month of life. Front. Vet. Sci. 9. doi: 10.3389/fvets.2022.911330
Keywords: apparent total tract digestibility, chelate compounds technology, fecal consistency index, organic zinc, solid feed intake
Citation: Rajaei-Sharifabadi H, Shamkhani E, Hafizi M, Mohammadi S, Shokri Z, Ahmadibonakdar Y and Seradj AR (2024) Source-dependent effects of early-life zinc supplementation in milk on growth performance and starter intake of pre-weaned dairy calves. Front. Anim. Sci. 5:1462245. doi: 10.3389/fanim.2024.1462245
Received: 09 July 2024; Accepted: 19 August 2024;
Published: 03 September 2024.
Edited by:
Anusorn Cherdthong, Khon Kaen University, ThailandReviewed by:
Mayra A. D. Saleh, University of the Azores, PortugalChanadol Supapong, Rajamangala University of Technology Srivijaya, Thailand
Copyright © 2024 Rajaei-Sharifabadi, Shamkhani, Hafizi, Mohammadi, Shokri, Ahmadibonakdar and Seradj. This is an open-access article distributed under the terms of the Creative Commons Attribution License (CC BY). The use, distribution or reproduction in other forums is permitted, provided the original author(s) and the copyright owner(s) are credited and that the original publication in this journal is cited, in accordance with accepted academic practice. No use, distribution or reproduction is permitted which does not comply with these terms.
*Correspondence: Hossein Rajaei-Sharifabadi, hrajari@malayeru.ac.ir