- 1Biotechnology Centre of the Azores (CBA), Faculty of Agricultural and Environmental Sciences, University of the Azores, Angra do Heroísmo, Portugal
- 2Institute for Investigation and Technology of Agronomy and Environment (IITAA), Faculty of Agricultural and Environmental Sciences, University of the Azores, Angra do Heroísmo, Portugal
Introduction: The struggle against climate change in agriculture requires an increased understanding of greenhouse gas emissions, mainly from cattle farming. Through precise and accessible methods to monitor the methane (CH4) emissions of these animals, it is possible to assess the influence of several factors.Therefore, this study evaluates CH4 emissions from Catrina cattle, an autochthonous breed from the Azores, and Holstein-Friesian cattle, aiming to assess the potential environmental impact and sustainability of this native breed.
Materials and methods: The present study was performed on a total of 15 dry cows, seven Catrina and eight Holstein-Friesian, housed by breed, in groups of five animals. The laser methane detector was used to assess the repeatability and reproducibility of CH4 values, including peaks and respiration. Descriptive statistics for raw data, CH4 breath and peaks, and the amount of CH4 emitted per day and year were calculated.
Results: From the Catrina breed, the average of CH4 emissions were 37.04 ± 40.09 ppm x m for raw data, 33.15 ± 28.59 ppm x m for CH4 breath, and 218.65 ± 67.13 ppm x m for CH4 peaks. From the Holstein-Friesian, the values obtained were 65.62 ± 87.11 ppm x m, 57.57 ± 52.59 ppm x m, and 514.19 ± 266.02 ppm x m, respectively. Linear mixed models, the Chisquare method and ANOVA, which showed a significant breed effect (p < 0.001) across all datasets, with trends favoring higher emissions in Holstein-Friesian were also applied. Similarly, Pearson correlation analyses yielded consistent trends, however, with no statistical significance (p > 0.05).
Discussion and conclusion: The findings underscore the importance of preserving cultural and genetic heritage while addressing climate change and environmental challenges. Furthermore, the study highlights the adaptive capacity of autochthonous breeds to their local environments, suggesting their role in sustainable systems. However, methane emissions will be influenced by several factors, besides breed variable, so this study emphasizes the need to integrate the assessment of the microbiome, which depends on the composition of the diet, genetic characteristics, and other aspects, for the development of methane mitigation strategies, with the inclusion of native breeds in sustainable resource management and climate adaptation efforts.
1 Introduction
Dairy and beef cattle are essential resources for worldwide human food production (Meo-Filho et al., 2023). By 2050, global demand is expected to increase to 58% and 73%, respectively (Beauchemin et al., 2020). However, they are also considered the main source of methane (CH4) emissions in the agricultural sector, as a natural by-product of enteric fermentation (Króliczewska et al., 2023; Marumo et al., 2023).
To achieve European Union (EU) objectives by 2030 (European Commission, 2020), researchers and breeders have been focusing on different approaches to mitigate enteric emissions while promoting the increase of animal production (Henderson et al., 2015; Hristov et al., 2015; Henderson et al., 2018; Eckard and Clark, 2020; Króliczewska et al., 2023). But so far none of them has been established (European Commission, 2021; Marumo et al., 2023), since the production of this gas depends on several factors, such as animal health and welfare, genetics, dry matter intake, and diet composition, gastrointestinal microbiota, among others (Brito et al., 2021; Króliczewska et al., 2023).
On dairy farms, 60% of production costs are feed costs (Connor, 2015), producing 70 to 120 kg of CH4 per year per cow (Jorio, 2022), which can represent a loss of 2–12% of total food energy, directly affecting animal productivity (Roehe et al., 2016; Marumo et al., 2023). So, to reduce costs and reach the long-term sustainability of the livestock industry, farmers started using different grazing systems which range from continuous grazing of one area over a long period, to intensive rotational grazing in small areas for short periods (Armstrong and Heins, 2024). Nonetheless, it is important to consider that between grazing systems the forage quality is quite variable, which is a major factor in the potential of greenhouse gas (GHG) emissions (Soder and Brito, 2023).
Native bovine breeds are important genetic resources, due to their adaptation to the local environment in which they are raised, however, the widespread use of exotic cattle breeds has resulted in an evident reduction in these populations worldwide (Upadhyay et al., 2019). Currently, around 95% of high-yielding cows belong to six breeds, and their crosses of Holstein-Friesian, Jersey, and Brown Swiss in dairy cattle, and Angus, Herefords, and Shorthorns in beef cattle (Upadhyay et al., 2019; Brito et al., 2021). This intense selection of breeds arose from the idea that theoretically larger and faster-growing animals would transform low-quality forage into human edible products, with a lower environmental cost, in terms of excretion of pollutants, than native bovine breeds, generally smaller and with slower maturation. However, autochthonous breeds were bred under conditions that exhibited greater thermotolerance and ability to survive with a nutritionally poor diet (Fraser et al., 2014). This, provided them with physiological and behavioral differences to use low-nutritional native pastures more efficiently than exotic breeds (Fraser et al., 2014).
The autochthonous Catrina breed, derived from the ancestral cattle populations introduced around the 15th century in the Azores region, like many other native breeds was almost extinguished with the introduction of exotic breeds with greater economic importance for the region, namely Holstein-Friesian, Shorthorn, Normande, and Jersey (de Merelim, 1986), remaining only 59 animals to this day. However, although these animals have only been identified on one island in the Azores – Terceira Island, it is known that according to ship cargo manifests there are animals to be registered on the remaining islands, with special emphasis on the islands of Graciosa and São Jorge.
The Catrina cattle have been primarily selected for meat, milk, and work, however with the mechanization of agriculture, they are currently used for ludic activities and as maternal lines for other breeds. This breed is raised under semi-wild conditions, in medium/high altitude regions (characterized by poor quality pastures with highly acidic soils and harsh climatic conditions; Castro, 2017) where we find predominantly Holcus lanatus and Anthoxanthum odoratum (Borges et al., 2009; Gomes, 2010; Castro, 2017). So, similar to other native breeds, from around the world (Upadhyay et al., 2019; Ben-Jemaa et al., 2020; Ben-Jemaa et al., 2021; Pulikkan, 2021), this cattle has unique characteristics basing their diet on grassland species with low nutritional value and showing greater resistance to extreme weather conditions and an adult weight of cows is an average of 250–300 kg.
Nevertheless, it was only officially recognized as a breed in 2022 by the Animal Genetic Resources Office from the General Directorate for Food and Veterinary (DGAV). So, due to their ancestry and the lack of information about the Catrina breed, this study aimed to increase the knowledge of in vivo measurement of methane emissions, through a non-invasive method, flexible and significantly cheaper compared to collection techniques such as sulfur hexafluoride (SF6) or Green-Feed. Also, due to the nervous temperament of these animals, the fact that laser methane detectors (LMm) allow measurements at a distance makes it possible to obtain, in safety, data without disturbing their well-being (Sorg et al., 2018).
2 Materials and methods
2.1 Experimental design
This study was performed following the Portuguese decree law N113/2013 from August 7th. As the project was developed in the Azores, authorization has been requested and obtained from the regional entities with responsibilities and competencies to approve the procedures (Sai-DRAg/2023/2144/VMF) and from the Organ Responsible for Animal Welfare from the University of Azores (COM/ALN/ORBEA/2023/002).
A total of 15 dry cows, seven Catrina and eight Holstein-Friesian, were used in this study. All animals were housed by breed, in groups of five animals, except for the first trial of the Catrina cattle, which was performed with four animals, due to the use of antibiotics in an animal that was discarded from the study. For each breed, two trials were performed in two consecutive years from November to March, and two cows from each breed were integrated into both trials.
In the case of the Catrina breed animals, as their diet is usually characterized by semi-natural pastures at medium/high altitudes, a 14-day adaptation period to the diet was necessary, followed by 14 days of data collection. Data was immediately collected from the Holstein-Friesian animals, as they were routinely fed under the study conditions.
All animals were housed in the same open‐aerated barn at the Experimental Dairy Farm Unit of the School of Agrarian & Environmental Sciences of the University of the Azores, located in a middle altitude region of Terceira Island (390 m above sea level; latitude 38_41052.800 N; longitude 27_10024.600 W) to adapt to the environment they were confined to during the study (Azevedo, 2013).
2.2 Diet and dry matter intake estimate
Animals were fed ad libitum with mixed grass and corn silage and water. The diet provided was the usual for production animals, however, the grass silage was from different packages showing some different quality results but not statistically significant. The chemical composition of the grass and the corn silage used during the trial was determined according to Van Soest et al. (1991) (Table 1).
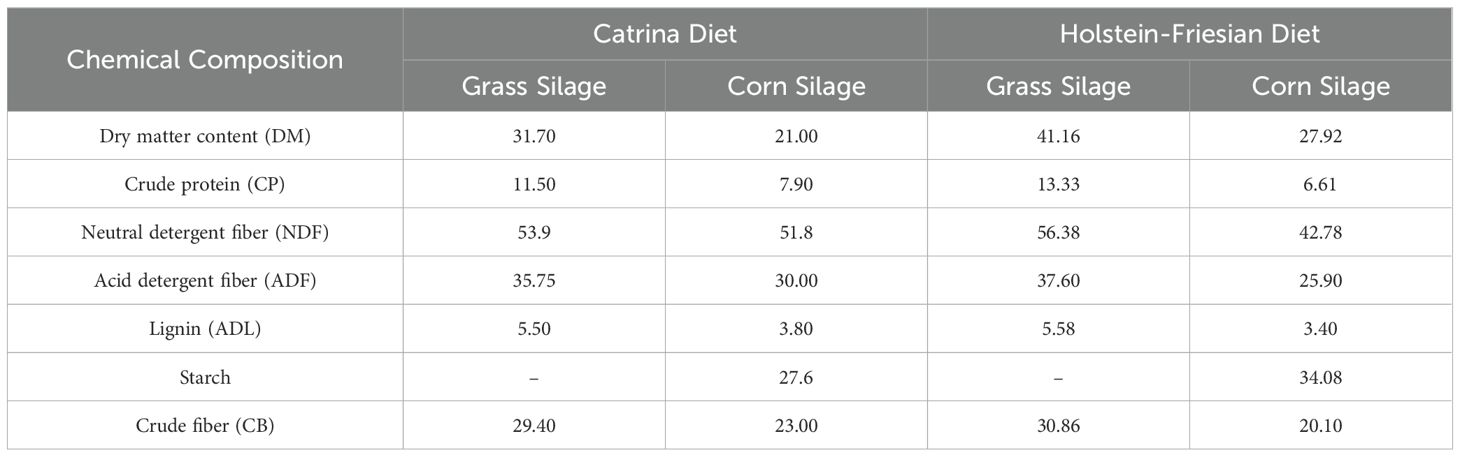
Table 1. The mean of the chemical composition of grass and corn silage (% on a DM basis) used during the trial.
The initial live weight of each animal was measured on the first day of the experiment, and the final live weight was measured at the end of the study. The Catrina animals exhibited an average of 294 kg against the 626 kg in the Holstein breed. Live weight was used to estimate the potential feed intake through Equation 1:
where the dry matter intake (DMI) multiplier, taking into account the body weight of the animals was considered 3%.
2.3 Measurement of enteric CH4 emissions
Methane emissions were measured using a hand-held laser methane detector. The “Laser Methane mini™ (LMm)” model (Crowcon, Abingdon, UK) expressed the concentration of methane as parts per million per linear meter (ppm×m). Through infrared spectroscopy with wavelength adjusted with high selectivity for the CH4 absorption band, it is possible to obtain the concentration (ppm × m) of CH4 emitted by the animal, with an ambient temperature between −17°C and +50°C and humidity of 30–90% (Sorg et al., 2017). According to the manufacturer, the LMm enables the detection of concentrations between 1 and 50,000 ppm × m at up to 30 m from the emission source (Sorg et al., 2017).
Before starting data collection, the CH4 background environment was measured in the same local without the animals, as suggested by the technical support team for LMm calibration. Measurements were collected by the same trained operator, as suggested by previous studies (Chagunda et al., 2009; Chagunda, 2013; Niero et al., 2020), by pointing the green laser beam to the nostril of a single animal for 5 min, at a distance of approximately 3 meters (Niero et al., 2020). For each trial, CH4 emissions were registered every 0.5 s, for a total of 600 records for each measurement (5 min) depicted on the methanogram (Figure 1).
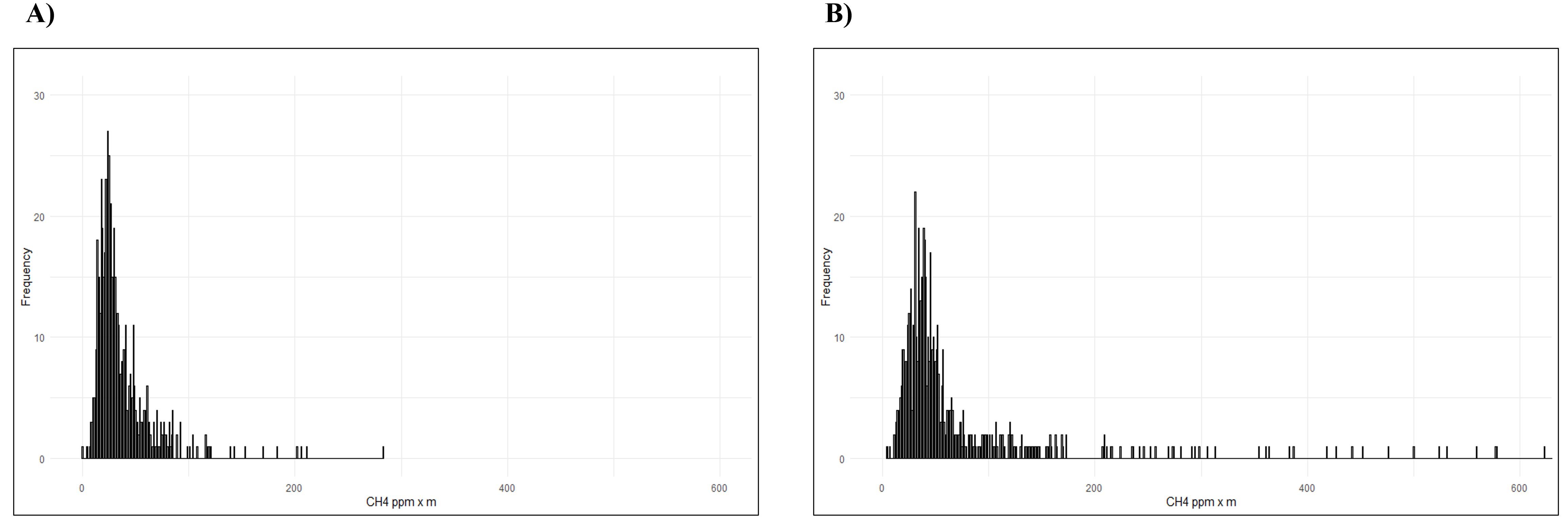
Figure 1. Distribution of CH4 emissions (ppm x m) during one measurement: (A) Catrina; (B) Holstein-Friesian.
Two consecutive measurements were performed within a day for each animal, achieving 10 measurements and 6000 records per day. All measurements were performed three days per week, at the same time of the day (between 11:00 a.m. and 2:00 p.m.) and in the same order for the animals involved in the study during natural activities, such as standing or lying down but always in the period of rumination, without physical restraint.
2.4 Statistical analysis
Statistical analyses were performed in R version 4.0.4, via the R studio environment. Descriptive statistics were calculated according to Niero et al. (2020), namely mean, mode, standard deviation, maximum, and minimum. Skewness and Kurtosis values were also calculated to determine the distribution of the data of CH4 emissions expressed as ppm × m in the case of the Catrina breed with and without the adaptation period and from the Holstein-Friesian breed. The threshold (T) separates peaks and respiration CH4 values. CH4 peaks were considered when values were three times above the standard deviation value plus the mean value.
To achieve normality and homogeneity of variances we followed Niero et al. (2020), and therefore the original raw data of CH4 emissions were log‐transformed (lnCH4).
The amount of CH4 emitted per day and year was estimated using Equations 2–4, proposed by Lanzoni et al. (2022) and Pereira et al. (2023):
where g is grams; CH4 means is the average of the peak value of emission in ppm × m divided by the distance from the animal; V is the tidal volume, i.e., the volume of air inhaled and expelled in each respiratory cycle, determined as 3800 mL; R is the respiratory rate determined as 20 acts per minutes; α is the conversion factor of CH4 production from mL to gram which is 0.000667 g/mL; and β is the dilution factor to correct for the difference between breath and total CH4 production which is ten.
As a preliminary method, in the first phase, the Pearson correlation test was applied to evaluate the relationship between the results obtained from both breeds under study and to select the most appropriate statistical methods.
After obtaining these results, linear mixed models were applied with ANOVA (Analysis of Variance), to compare means across the different groups. To study associations between categorical variables the Chi-square method was applied.
3 Results
The data recorded by the LMm allows a temporal assessment of the CH4 emission values belonging to each animal, recording the inhalation and expiration of the respiratory cycle, including the peaks emitted. Figure 2 shows the profile of the respiratory cycle of two randomly selected cows from the two breeds under study, indicating methane values, expressed as ppm × m, in a single measurement. Using the same animals, through a histogram (Figure 1), we can observe the frequency of distribution of CH4 values expressed as ppm × m recorded, in each breed.
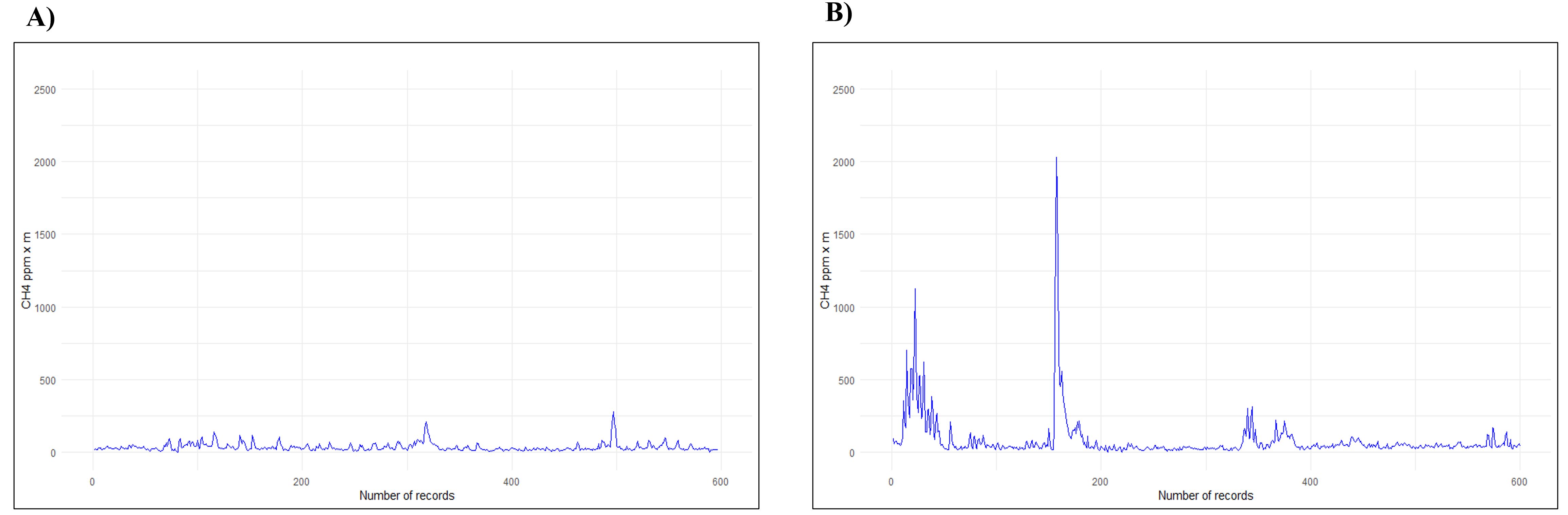
Figure 2. Graphical plot representing the number of records of CH4 emissions (ppm x m) during one measurement: (A) Catrina; (B) Holstein-Friesian.
By analyzing the quantile-quantile (Q-Q) plot (Supplementary Figure 1), Figure 1, and the kurtosis and skewness results (Table 2), which provide information about the shape of the distribution, we confirmed that a normal distribution (p< 0.05) is not present. To normalize the results, the original raw data of CH4 emissions were loge-transformed (Table 2; Figure 3).
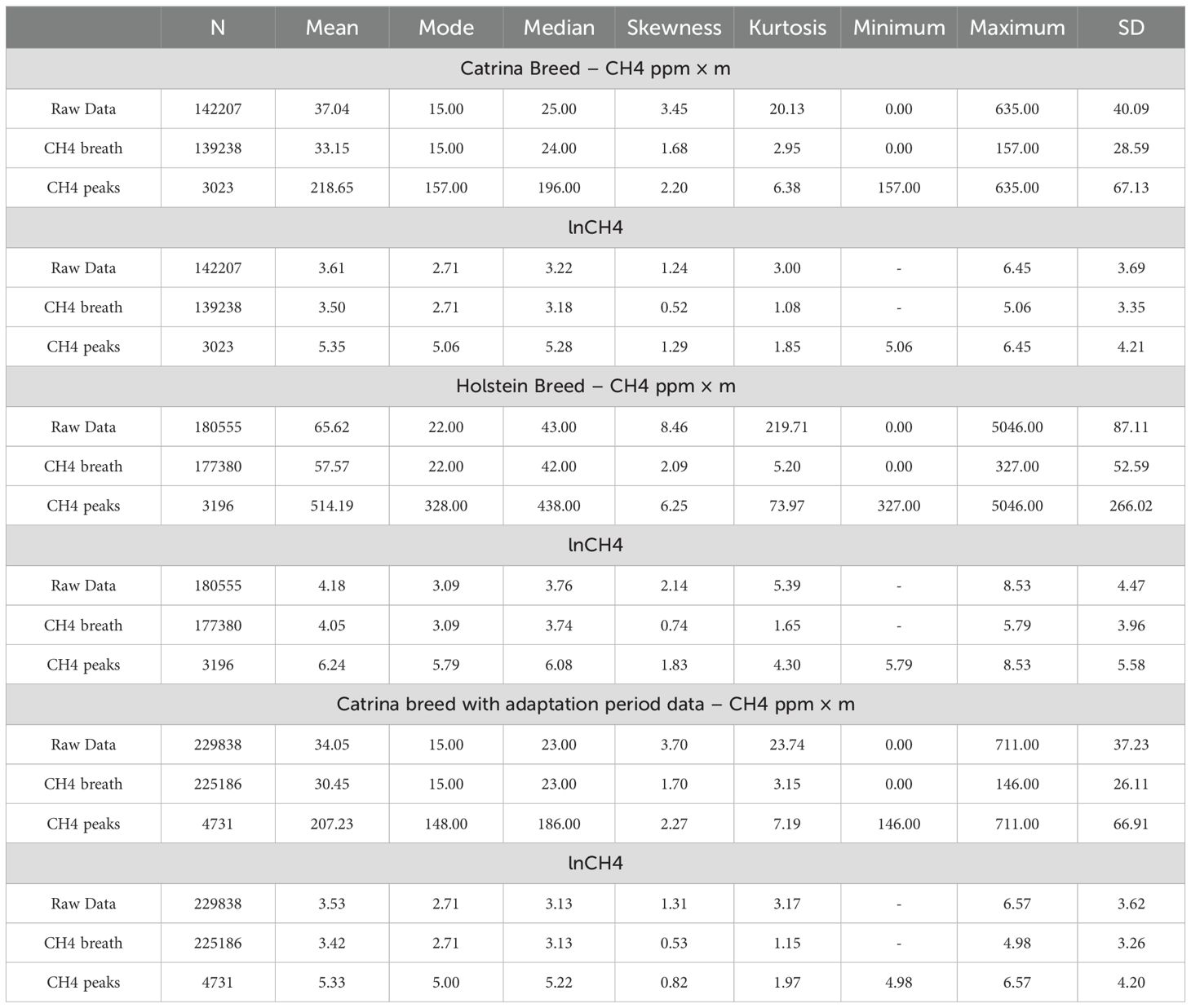
Table 2. Descriptive statistics of the CH4 emissions for the raw data (pre-editing), respiration events, and CH4 peaks respectively.
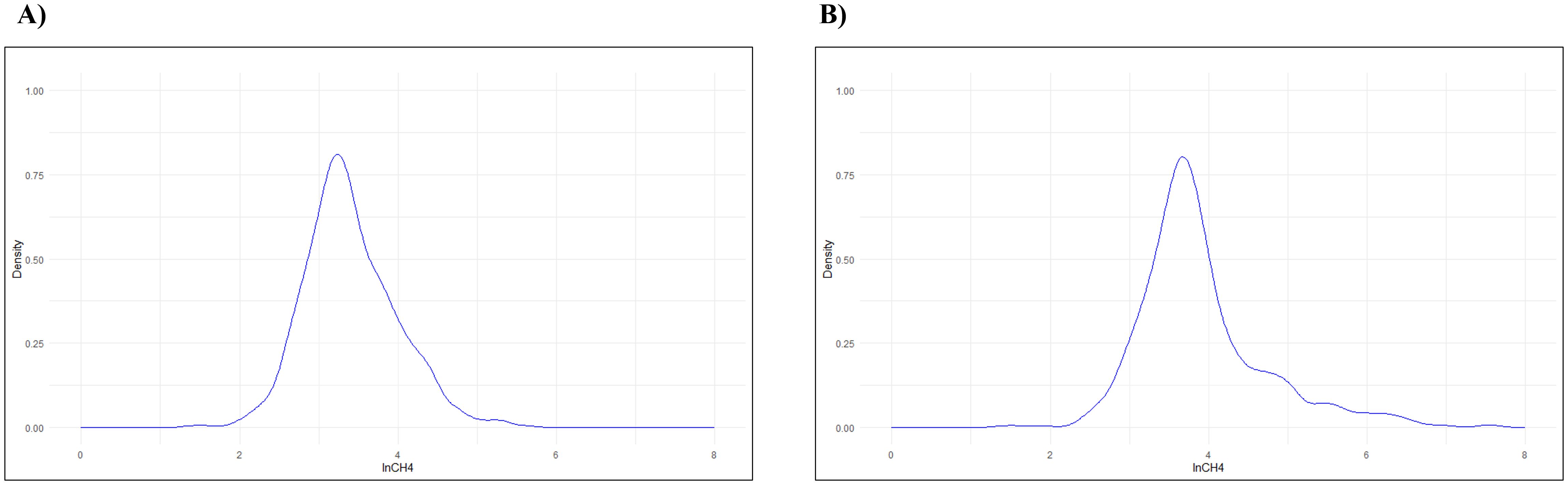
Figure 3. Distribution of CH4 emissions expressed as loge-transformed data (lnCH4): (A) Catrina; (B) Holstein-Friesian.
Using descriptive statistics (Table 2), all animals per breed were analyzed, separating the data into three main groups: raw data (without any treatment or filtering), CH4 breath (respiratory events), and CH4 peaks (records exceeding three standard deviations from the mean) emitted. This provided from the Catrina breed a total of n = 142207, n = 139238, n = 3023 records, respectively, and from the Holstein-Friesian breed a total of n = 180555, n = 177380, n = 3196 records, respectively. Additionally, considering that this study is a pioneer from the native Catrina breed, the data collected during the period of adaptation of the animals to the diet was also included in the results, with a total of n = 229838, n = 225186, n = 4731 records.
From these results, the average and standard deviation (SD) of CH4 emissions from the Catrina breed was 37.04 ± 40.09, 33.15 ± 28.59, and 218.65 ± 67.13 ppm × m, for the raw data (pre-editing), CH4 breath and CH4 peaks, respectively. The lowest values detected ranged from 0 to 157 ppm × m and the maximum ranged from 157 to 635 ppm × m (Table 2).
Regarding the results obtained from the Holstein-Friesian animals, the averages obtained were 65.62 ± 87.11, 57.57± 52.59 and 514.19 ± 266.02 ppm × m and the lowest values detected ranged from 0 to 327 ppm × m and the maximum from 327 to 5046 ppm × m (Table 2).
When considering also the Catrina cattle adaptation period data, the results obtained were 34.05 ± 37.23, 30.45 ± 26.11 and 207.23 ± 66.91 ppm × m with the lowest values detected ranging from 0 to 146 ppm × m and the maximum from 146 to 711 ppm × m (Table 2).
Concerning lnCH4 data, the values were 3.17 ± 0.99, 3.12 ± 0.95 and 5.35 ± 0.25 ppm × m from the Catrina breed, from the Holstein-Friesian breed, the values obtained are 3.72 ± 0.970, 3.68 ± 0.920 and 6.17 ± 0.34 ppm × m. From the Catrina breed including the data collected during adaptation, the results are 3.09 ± 0.99, 3.04 ± 0.95 and 5.29 ± 0.27 ppm × m.
To evaluate if the daily CH4 emissions were affected by breed, in a linear relationship, we applied the Pearson correlation coefficient (Supplementary Table 1). Analyzing the raw data, the CH4 emissions tended to be higher from the Holstein-Friesian than from the Catrina’s cattle (p = 0.4274), yet, the difference is not statistically significant, and there is weak evidence against the null hypothesis of no correlation. Nevertheless, when we include the adaptation period data from the Catrina cattle the result is different and tends to be statistically significant (p = 0.08896).
Regarding the results obtained from CH4 breath, none of the correlations were statistically significant (p = 0.1034, p = 0.5048). Also, from CH4 peaks, besides the predisposition to produce higher values in the Holstein-Friesian breed in comparison with the Catrina cattle animals with and without the adaptation period (p = 0.1457, p = 0.9992, respectively), we still cannot conclude a statistically significant correlation between the breeds.
Taking into account the previous results when testing the mixed linear model procedure with an Analysis of Deviance Table (Type II Wald chi-square tests), considering the fixed effect: the breed (Catrina, Holstein-Friesian) and the random effects: the animal ID, replica, day and daily measurement, the results are very different, indicating in all groups of the dataset (CH4 raw data, CH4 breath and CH4 peaks) the variable breed as being statistically highly significant through an extremely low p-value (p < 0.001). Moreover, in all cases, a larger Chi-squared value was obtained, which indicates a greater difference between observed and expected results (Figure 4; Supplementary Table 2).
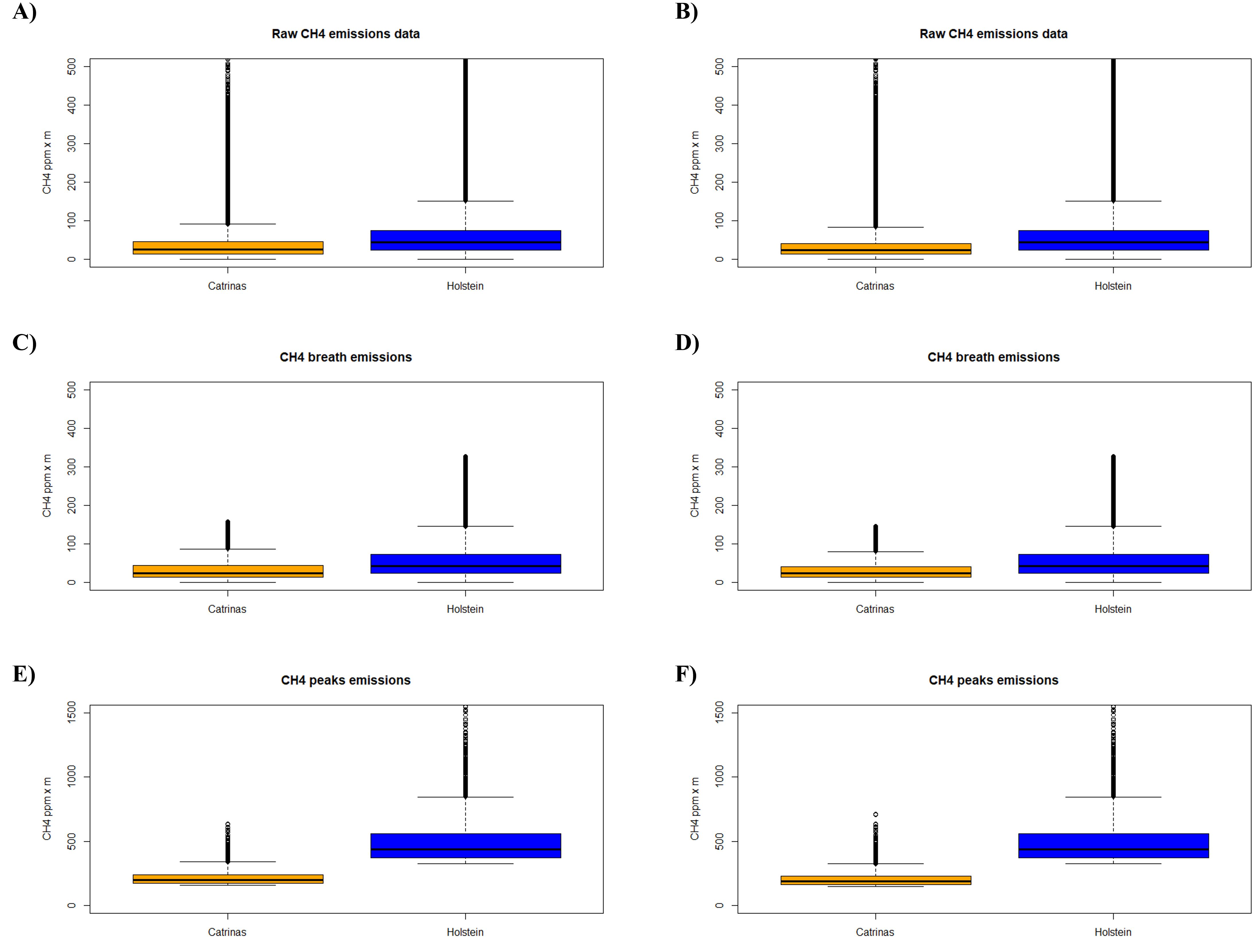
Figure 4. Boxplot of CH4 emissions by breed, (A, C, E) without and (B, D, F) with the data collected during the adaptation period of the Catrina breed to the diet.
Complementarily, the ANOVA results in all groups of data, confirmed that the factor breed has asignificant effect on the response variable (p < 0.001). The F-values are also very high, further supporting the conclusion of significant differences (Supplementary Table 3). The degrees of Freedom (Df) were also calculated for both methods.
After analyzing the effect of breed, we evaluated the distribution of CH4 emissionsdata in grams per day and kilograms per year, including respiratory events and peaks (CH4 means). Through Equations 3 and 4, the correlation between methane emissions from Catrina cattle and Holstein-Friesian in grams per day and kilograms per year was the same as previously described concerning the raw data results. The trend previously described continued with averages of 27.16 ± 29.39 and 99.14 ± 107.29 from the Catrina cattle, 48.11 ± 63.87 and 175.62 ± 233.13 in Holstein-Friesian cattle and 24.97 ± 27.30 and 91.13 ± 99.63 from the Catrina cattle, including the period of adaptation to the diet (Supplementary Table 4).
These results can also be observed by each box, in the boxplot, representing the interquartile range (IQR) of the data, with the line inside the box indicating the median value. In all the graphs a notable difference is observed between the CH4 emissions of the Catrina cattle and Holstein-Friesian, as indicated by the separation of their median values and the spread of their data distributions in Figure 5.
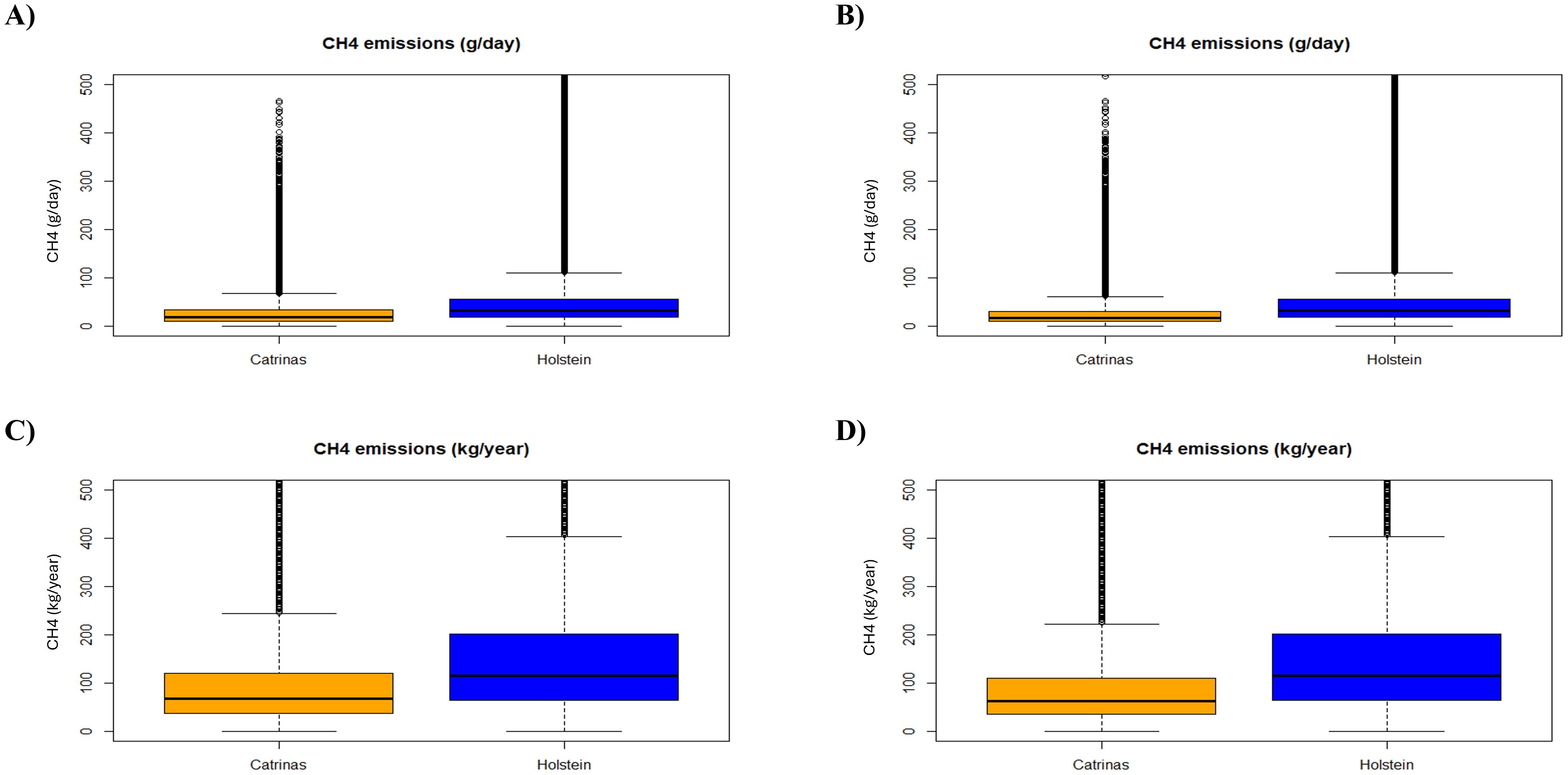
Figure 5. Boxplot of CH4 emissions per day and per year by breed, (A, C): without and (B, D): with the data collected during the adaptation period of the Catrina breed to the diet.
The results of Pearson’s Correlation in all analyses were identical to those previously described still, none of the correlations are statistically significant (p = 0.4274, p = 0.08896).
The same occurred when applying the ANOVA and Chi-square test, these evaluations reveal a highlysignificant correlation (p < 0.001) reinforcing that the predictor variable breed is significantly affecting the corresponding response variables [CH4 emissions (g/day) and CH4 emissions (kg/year)] (Supplementary Tables 2, 3).
Considering the use of Equations 3 and 4, with only the value of CH4 peaks of both breeds per day and year, the correlation between CH4 peaks emissions from the Catrina cattle and the Holstein-Friesian in grams per day and kilograms per year was the same as previously described. Concerning raw data results, the trend continued with values of 160.33 ± 49.22 and 58.52 ± 17.97 from the Catrina cattle, 137.61 ± 71.20 from the Holstein-Friesian cattle and 151.94 ± 49.06 and 55.46 ± 17.91 from the Catrina cattle including the period of adaptation to the diet and 377.03 ± 195.06 (Supplementary Table 4).
With Pearson’s correlation, the results obtained are the same as in Figure 6 (p = 0.1457, p = 0.9992, respectively) from which we cannot conclude a statistically significant correlation between breeds. But, again, with ANOVA and Chi-square test analysis, we found a highly significant correlation (p < 0.001) indicating that the predictor variable breed is significantly affecting the corresponding response variables [CH4 emissions (g/day) and CH4 emissions (kg/year)].
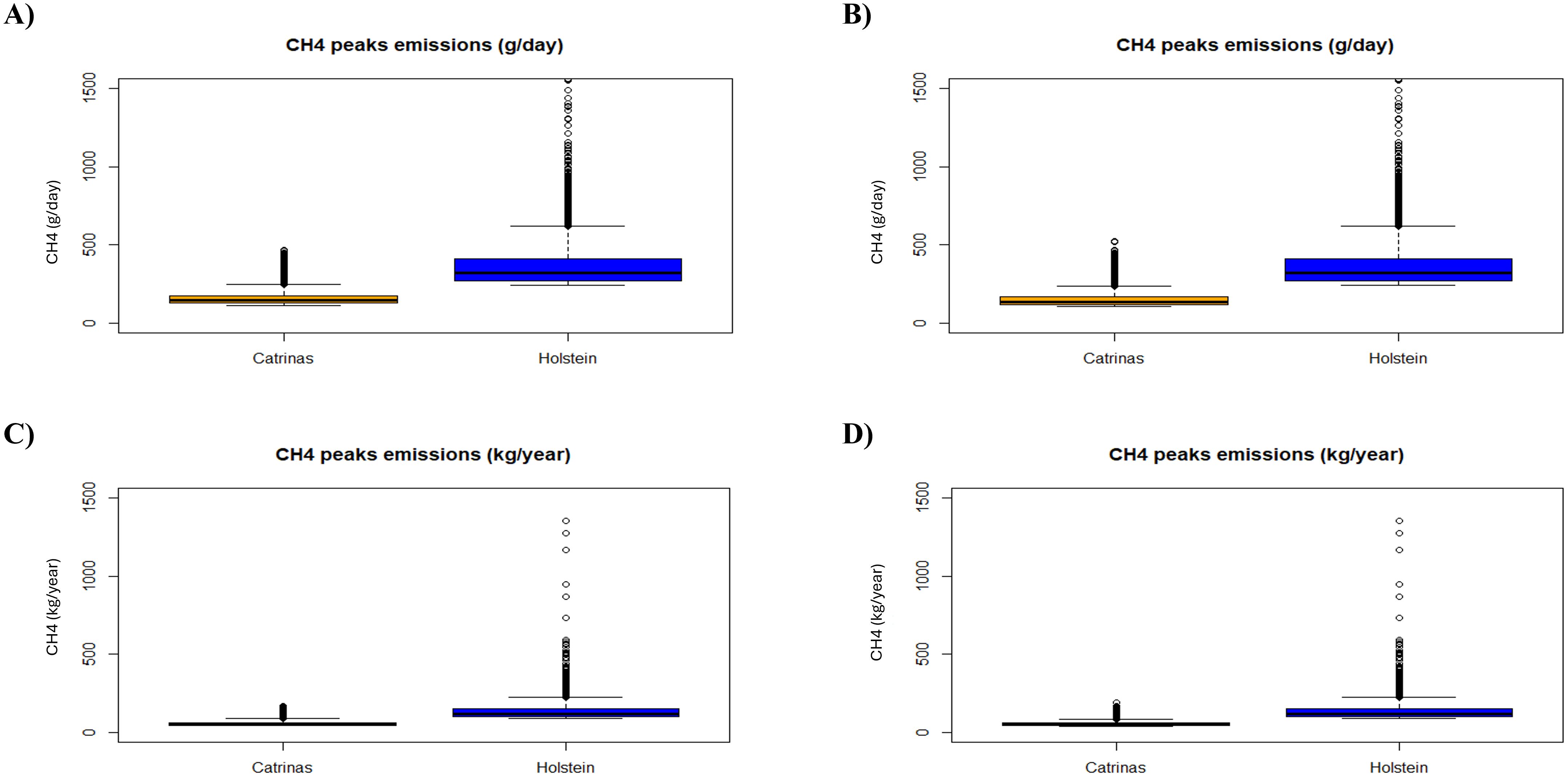
Figure 6. Boxplot of CH4 peaks per day and year by breed, (A, C): without and (B, D): with the data collected during the adaptation period of the Catrina breed to the diet.
4 Discussion
Autochthonous breeds such as the Catrina cattle are crucial in maintaining biodiversity and preserving genetic resources. By focusing on these breeds, we recognize their importance in sustainable agriculture and livestock management. In addition, understanding the environmental impact of these animals can help in the design of conservation strategies and improving agricultural practices for greater farm sustainability.
For CH4 measurements in cattle, the use of LMm has been increasing in the last years (Chagunda et al., 2009; Chagunda, 2013; Mapfumo et al., 2018; Sorg et al., 2018; Denninger et al., 2020; Pinto et al., 2020; Roessler and Schlecht, 2021; Lanzoni et al., 2022). LMD makes it possible to design a cheaper and simpler test to monitor CH4 emissions compared to other methods currently available, having a great impact in areas with financial challenges, since are less expensive and it is not necessary to change the routine of the animals (Kobayashi et al., 2021; Pereira et al., 2023). Furthermore, individual confinement within the cameras imposes restrictions on the diet and natural behavior of individuals, which can result in a reduction in dry matter consumption (DMI) and consequently in CH4 emissions, making it difficult to verify the results obtained and their application in industrial systems (Pinares-Patiño and Clark, 2008; Sorg et al., 2018).
In this study, the aim was essentially to compare two completely different breeds, one high-production breed with a strong presence in the Azores region and an autochthonous breed from the region, which was once used to produce meat and milk due to its high-quality, recently proven by the presence of diverse genetic variants very important for the industry (Azevedo et al., in preparation)1. But, unfortunately, like other native breeds worldwide, they have suffered severe abandonment, putting them on the brink of extinction.
The main objective of using the Catrina cattle as a comparison model was firstly to demonstrate their ecological value, by subjecting them to a silage-based diet, simulating the conditions experienced by high-production breeds, usually raised in highly controlled environments with optimized diets. Secondly, the use of different breeds allowed the evaluation of the influence of the factor breed, which is one of the most studied, among the studies covering beef and dairy cattle (Pinto et al., 2020; Meo-Filho et al., 2023; Pereira et al., 2023), reinforcing the importance of breed-specific differences when we are analyzing methane production. However, it must be taken into account when analyzing the results of methane emissions that the requirement of dry matter depends on the animal’s body weight (Siddque et al., 2015). Also, the estimated amount of DMI (kg/d), may be linked to several factors, besides breed type and body weight, like the lactating status, physiological phase, and age, among others (Siddque et al., 2015; Committee on Nutrient Requirements of Dairy Cattle et al., 2021). In our study, the daily dry matter intake capacity of Holstein-Friesian animals was considerably higher than that of Catrina animals.
The CH4 concentration values registered were consistent with previous studies carried out with LMD, as well as in relation to diets. However, as suggested by several authors, slight differences related to measurement conditions are inevitable (Sorg et al., 2018; Pereira et al., 2023).
The results obtained through the means and standard deviations of the raw data from CH4 breath and peaks indicated that the Catrina cattle produces significantly lower methane emissions than Holstein-Friesian cattle. Furthermore, when compared with Sorg et al. (2018), who reported averages of 97 ± 44 and CH4 peaks of 350 ± 148 ppm × m in Polish Holstein or Pinto et al. (2020) with averages of 44 ± 34.9 ppm × m in Holstein-Friesian and Jersey, CH4 emissions from Catrina cattle are still lower. Also, compared with Niero et al. (2020), who have a curious factor in the study of Simmental heifers who, given their characteristics, would be expected to produce lower emissions than adult animals, their averages are 105.48 ± 77.92, which are higher than those reported from the Catrina cattle. Additionally, when comparing the values of CH4 respiration and CH4 peaks (98.26 ± 58.02 and 229.45 respectively), the values obtained from the Catrina cattle are also lower (Niero et al., 2020).
The values obtained from the Holstein-Friesian cattle in the present study were lower when compared with the averages reported from Polish Holstein cows by Sorg et al. (2018) and with the respiratory events reported by Pinto et al. (2020), that studied native and exotic cows without separating the breeds.
With Pearson’s correlation, a slight tendency (p > 0.05) for higher emissions is observed in Holstein-Friesian cattle compared to the Catrina cattle. We also verified a weak negative correlation between breed and CH4 emissions, which means that the data obtained do not provide solid information for rejecting the idea that no correlation exists between the studied variables.
Also, when considering the values collected during the adaptation period of the Catrina cattle, the correlation becomes more pronounced and tends towards statistical significance (p = 0.08896). This suggests that it is possible that if we enlarge the study, the effect of the factor breed will have a strong correlation with the levels of CH4 emissions in a linear relationship. These findings are supported by the results obtained from the linear mixed models, where this variable demonstrates a highly statistically significant effect on CH4 emissions, CH4 breath, and CH4 peak emissions across the different datasets, including the daily and annual production.
According to Villanueva et al. (2023), Holstein cows and Holstein × Gyr (F1) dry cows emit 261 to 238 g of enteric CH4 day−1 with an annual average ranging between 91 and 112 kg cow−1 respectively, which despite having a lower dry matter intake than lactating cows, in terms of CH4 emission intensity showed highest values. In our study, only with dry cows, we obtained lower values daily and annually in both breeds.
Although it was not possible to correlate the effect of the diet with the CH4 emissions recorded, comparing the results with Uddin et al. (2021), who described an association of a more significant ecological footprint in animals with lower levels of nDF (19%DM) and vice versa, Holstein-Friesian animals presented significantly higher levels of CH4 emissions per day and per year than animals from the Catrina breed, although both were under the influence of a diet with high levels of nDF.
5 Conclusion
The present study, overall, demonstrated significantly lower CH4 emissions from Catrina cattle when compared to Holstein-Friesian cattle, giving indications that native breeds could have an important environmental impact in terms of methane production, in addition to the crucial role of cultural and genetic heritage preservation.
Given climate changes and growing environmental pressures, the use of autochthonous breeds could be fundamental for the development of scientific studies that allow optimizing methane mitigation techniques, but also since they are extremely well adapted to the regions where they live, they could be an answer for more sustainability.
Comparing the CH4 emissions of Catrina cattle with those of Holstein-Friesian cattle also allowed us to observe how a native breed responds to dietary changes. Taking the results into account, we are curious about what the emissions of both breeds would be like with a diet of low nutritional value in pastures where we do not usually find high-production animals due to their intense selection that directed them only to large production scale.
In conclusion, this study makes a unique contribution to the field of animal science and environmental studies by emphasizing the importance of conducting initial research with native breeds. Nevertheless, we can conclude that the breed is a fundamental factor to consider when developing methane mitigation strategies, however, methane emissions will be influenced by several factors, besides the breed variable, so this study emphasizes the need to integrate the assessment of the microbiome, which depends on the composition of the diet, genetic characteristics, and other aspects.
Data availability statement
The original contributions presented in the study are included in the article/Supplementary Material. Further inquiries can be directed to the corresponding author.
Ethics statement
The animal studies were approved by Organ Responsible for Animal Welfare from the University of Azores. The studies were conducted in accordance with the local legislation and institutional requirements. Written informed consent was obtained from the owners for the participation of their animals in this study.
Author contributions
ARA: Conceptualization, Formal analysis, Investigation, Methodology, Writing – original draft. MSL: Investigation, Supervision, Validation, Writing – review & editing, Funding acquisition. AB: Supervision, Writing – review & editing. ACM: Resources, Conceptualization, Investigation, Methodology, Supervision, Validation, Visualization, Writing – review & editing. DM: Writing – review & editing, Funding acquisition, Project administration, Resources.
Funding
The author(s) declare financial support was received for the research, authorship, and/or publication of this article. Biotechnology Centre of Azores is financed by FCT – Fundação para a Ciência e a Tecnologia, I.P., under the projects UIDP/05292/2020, UIDB/05292/2020 and FCT 2023.15029.PEX. ARA is supported by the Fundo Regional para a Ciência e Tecnologia e Governo dos Açores (M3.1.a/F/035/2020).
Acknowledgments
The authors are grateful to all cattle breeders and owners for participating in this study. To the University of the Azores and the animal caretakers of the Experimental Dairy Farm Unit of the School of Agrarian & Environmental Sciences.
Conflict of interest
The authors declare that the research was conducted in the absence of any commercial or financial relationships that could be construed as a potential conflict of interest.
Publisher’s note
All claims expressed in this article are solely those of the authors and do not necessarily represent those of their affiliated organizations, or those of the publisher, the editors and the reviewers. Any product that may be evaluated in this article, or claim that may be made by its manufacturer, is not guaranteed or endorsed by the publisher.
Supplementary material
The Supplementary Material for this article can be found online at: https://www.frontiersin.org/articles/10.3389/fanim.2024.1423940/full#supplementary-material
Supplementary Figure 1 | Q-Q plot graph. (A) Catrina breed (n = 142207); (B) Catrina breed with adaptation period data (n = 229838); (C) Holstein-Friesian Breed (n = 180555).
Supplementary Table 1 | Pearson’s Correlation results of the different groups in the study.
Supplementary Table 2 | Analysis of Deviance Table (Type II Wald chi-square tests), indicating in all groups of the study.
Supplementary Table 3 | ANOVA analysis, indicating in all groups of the study.
Supplementary Table 4 | Descriptive statistics of the CH4 emissions data in grams per day and kilograms per year.
Footnotes
- ^ Azevedo, A. R., Lopes, M. S., da Câmara Machado, A., and Mendonça, D. The Catrina cattle breed: a successful example of empirical selection (In preparation).
References
Armstrong J., Heins B. (2024). Grazing and pasture management for cattle (University of Minnesota). Available at: https://extension.umn.edu/pasture-based-dairy/grazing-andpasture-management-cattle.
Azevedo E. (2013). Cartografia do Clima Normal do Arquipélago dos Açores - Ilha Terceira - Apuramento Anual - Modelo CIELO (M. e M. G. (C_CMMG) Centro de Estudos do Clima (ed.)). Universidade dos Açores. doi: 10.13140/RG.2.1.1250.6085
Beauchemin K. A., Ungerfeld E. M., Eckard R. J., Wang M. (2020). Review: Fifty years of research on rumen methanogenesis: Lessons learned and future challenges for mitigation. Animal 14, S2–16. doi: 10.1017/S1751731119003100
Ben-Jemaa S., Mastrangelo S., Lee S. H., Lee J. H., Boussaha M. (2020). Genome-wide scan for selection signatures reveals novel insights into the adaptive capacity in local North African cattle. Sci. Rep. 10, 1–15. doi: 10.1038/s41598-020-76576-3
Ben-Jemaa S., Senczuk G., Ciani E., Ciampolini R., Catillo G., Boussaha M., et al. (2021). Genome-wide analysis reveals selection signatures involved in meat traits and local adaptation in semi-feral maremmana cattle. Front. Genet. 12. doi: 10.3389/fgene.2021.675569
Borges P. A. V., Azevedo E. B., Borba A., Dinis F. O., Gabriel R., Silva E. (2009). “Cap. 14: Ilhas Oceânicas,” in Pereira L. V. H. M., Domingos T. (Ed.) Portugal Millenium Ecosystem Assessment (Lisbon: Escolar Ed). 463–510. Available at: http://hdl.handle.net/10400.3/2011%5Cnhttp://repositorio.uac.pt/bitstream/10400.3/2011/3/Borges_et_al_2009_Ilhas_Oceanicas_Cap14.pdf.
Brito L. F., Bedere N., Douhard F., Oliveira H. R., Arnal M., Peñagaricano F., et al. (2021). Review: Genetic selection of high-yielding dairy cattle toward sustainable farming systems in a rapidly changing world. Animal 15, 100292. doi: 10.1016/j.animal.2021.100292
Castro C. P. S. (2017). Contributo para a caraterização do sistema agropecuário da ilha do Faial. Arquipélago dos Açores 105. https://www.repository.utl.pt/bitstream/10400.5/13412/1/TESEFINAL.pdf.
Chagunda M. G. (2013). Opportunities and challenges in the use of the Laser Methane Detector to monitor enteric methane emissions from ruminants. Animal 7 Suppl 2, 394–400. doi: 10.1017/S1751731113000724
Chagunda M. G. G., Ross D., Roberts D. J. (2009). On the use of a laser methane detector in dairy cows. Comput. Electron Agric. 68, 157–160. doi: 10.1016/j.compag.2009.05.008
Committee on Nutrient Requirements of Dairy Cattle, Board on Agriculture and Natural Resources, Division on Earth and Life Studies (2021). Nutrient Requirements of Dairy Cattle. Eighth Revised Edition (Washington (DC): National Academies Press (US)), 1–5. Available at: https://www.ncbi.nlm.nih.gov/books/NBK600610/.
Connor E. E. (2015). Invited review: Improving feed efficiency in dairy production: Challenges and possibilities. Animal 9, 395–408. doi: 10.1017/S1751731114002997
de Merelim P. (1986). Tauromaquia Terceirence (Delegação de Turismo de Angra do Heroísmo (ed.)) Angra do Heroísmo).
Denninger T. M., Schwarm A., Dohme-Meier F., Münger A., Bapst B., Wegmann S., et al. (2020). Accuracy of methane emissions predicted from milk mid-infrared spectra and measured by laser methane detectors in Brown Swiss dairy cows. J. Dairy Sci. 103, 2024–2039. doi: 10.3168/jds.2019-17101
Eckard R. J., Clark H. (2020). Potential solutions to the major greenhouse-gas issues facing Australasian dairy farming. Anim. Prod Sci. 60, 10–16. doi: 10.1071/AN18574
European Commission (2020). EU methane strategy. J. Chem. Inf Model. 110, 1689–1699. https://ec.europa.eu/commission/presscorner/detail/en/ip_20_1833.
European Commission (2021). Best Practice Guidance for Effective Management of Coal Mine Methane at National Level: Monitoring, Reporting, Verification and Mitigation UNECE Energy Series. Available online at: https://ec.europa.eu/commission/presscorner/detail/en/statement_21_5766. 03-18-2024. (accessed March 18, 2024)
Fraser M. D., Fleming H. R., Moorby J. M. (2014). Traditional vs modern: Role of breed type in determining enteric methane emissions from cattle grazing as part of contrasting grassland-based systems. PloS One 9 (9), e107861. doi: 10.1371/journal.pone.0107861
Gomes A. (2010). Produtividade e Qualidade de uma pastagem de Lolium Perenne e Trifoliem Repens e de uma pastagem à base de espécies espontâneas, instaladas numa zona de média altitude da ilha terceira (Açores). http://hdl.handle.net/10400.3/6093.
Henderson G., Cook G. M., Ronimus R. S. (2018). Enzyme- and gene-based approaches for developing methanogen-specific compounds to control ruminant methane emissions: a review. Anim. Prod Sci. 58, 1017–1026. doi: 10.1071/AN15757
Henderson G., Cox F., Ganesh S., Jonker A., Young W., Abecia L., et al. (2015). Rumen microbial community composition varies with diet and host, but a core microbiome is found across a wide geographical range. Sci. Rep. 5, 14567. doi: 10.1038/srep14567
Hristov A. N., Oh J., Giallongo F., Frederick T. W., Harper M. T., Weeks H. L., et al. (2015). An inhibitor persistently decreased enteric methane emission from dairy cows with no negative effect on milk production. Proc. Natl. Acad. Sci. U S A. 112, 10663–10668. doi: 10.1073/pnas.1504124112
Jorio L. (2022). Swiss farmers trial methane-busting feed for cows. Available online at: https://www.swissinfo.ch/eng/business/swiss-farmers-trial-methane-busting-feed-for-cows/47937318. 04-15-2024. (accessed April 15, 2024)
Kobayashi N., Hou F., Tsunekawa A., Yan T., Tegegne F., Tassew A., et al. (2021). Laser methane detector-based quantification of methane emissions from indoor-fed Fogera dairy cows. Anim. Biosci. 34, 1415–1424. doi: 10.5713/ab.20.0739
Króliczewska B., Pecka-Kiełb E., Bujok J. (2023). Strategies used to reduce methane emissions from ruminants: controversies and issues. Agric 13 (3), 602. doi: 10.3390/agriculture13030602
Lanzoni L., Chagunda M. G. G., Fusaro I., Chincarini M., Giammarco M., Atzori A. S., et al. (2022). Assessment of seasonal variation in methane emissions of mediterranean buffaloes using a laser methane detector. Animals 12, 1–12. doi: 10.3390/ani12243487
Mapfumo L., Grobler S. M., Mupangwa J. F., Scholtz M. M., Muchenje V. (2018). Enteric methane output from selected herds of beef cattle raised under extensive arid rangelands. Pastoralism 8, 15. doi: 10.1186/s13570-018-0121-9
Marumo J. L., LaPierre P. A., Van Amburgh M. E. (2023). Enteric methane emissions prediction in dairy cattle and effects of monensin on methane emissions: A meta-analysis. Animals 13 (8), 1392. doi: 10.3390/ani13081392
Meo-Filho P., Hood J., Lee M. R. F., Fleming H., Meethal M. E., Misselbrook T. (2023). Performance and enteric methane emissions from housed beef cattle fed silage produced on pastures with different forage profiles. Animal 17, 100726. doi: 10.1016/j.animal.2023.100726
Niero G., Cendron F., Penasa M., De Marchi M., Cozzi G., Cassandro M. (2020). Repeatability and reproducibility of measures of bovine methane emissions recorded using a laser detector. Animals 10 (4), 606. doi: 10.3390/ani10040606
Pereira A. M., Peixoto P., Rosa H. J. D., Vouzela C., Madruga J. S., Borba A. E. S. (2023). A longitudinal study with a laser methane detector (LMD) highlighting lactation cycle-related differences in methane emissions from dairy cows. Animals 13 (6), 974. doi: 10.3390/ani13060974
Pinares-Patiño C. S., Clark H. (2008). Reliability of the sulfur hexafluoride tracer technique for methane emission measurement from individual animals: an overview. Aust. J. Exp. Agric. 48, 223–229. doi: 10.1071/EA07297
Pinto A., Yin T., Reichenbach M., Bhatta R., Malik P. K., Schlecht E., et al. (2020). Enteric methane emissions of dairy cattle considering breed composition, pasture management, housing conditions and feeding characteristics along a rural-urban gradient in a rising megacity. Agriculture (Switzerland) 10 (12), 1–18. doi: 10.3390/agriculture10120628
Pulikkan J. (2021). Microbiome analysis exploring taxonomic diversity in kasaragod dwarf and holstein crossbred cattle. Research Square, 1–24. doi: 10.21203/rs.3.rs-1031096/v1
Roehe R., Dewhurst R. J., Duthie C. A., Rooke J. A., McKain N., Ross D. W., et al. (2016). Bovine host genetic variation influences rumen microbial methane production with best selection criterion for low methane emitting and efficiently feed converting hosts based on metagenomic gene abundance. PloS Genet. 12, 1–20. doi: 10.1371/journal.pgen.1005846
Roessler R., Schlecht E. (2021). Application of the laser methane detector for measurements in freely grazing goats: impact on animals’ behaviour and methane emissions. Animal 15, 100070. doi: 10.1016/j.animal.2020.100070
Siddque M. A. B., Sarker N. R., Hamid M. A., Amin M. N., Sultana M. (2015). Growth performance, feed conversion ratio and economics of production of native and crossbred (Local × Holstein friesian) bulls for fattening under different improved feeding. J. Agric. Sci. Technol. A. 5 (9), 770–780p. doi: 10.17265/2161-6256/2015.09.008
Soder K. J., Brito A. F. (2023). Enteric methane emissions in grazing dairy systems. JDS Commun. 4, 324–328. doi: 10.3168/jdsc.2022-0297
Sorg D., Difford G. F., Mühlbach S., Kuhla B., Swalve H. H., Lassen J., et al. (2018). Comparison of a laser methane detector with the GreenFeed and two breath analysers for on-farm measurements of methane emissions from dairy cows. Comput. Electron Agric. 153, 285–294. doi: 10.1016/j.compag.2018.08.024
Sorg D., Mühlbach S., Rosner F., Kuhla B., Derno M., Meese S., et al. (2017). The agreement between two next-generation laser methane detectors and respiration chamber facilities in recording methane concentrations in the spent air produced by dairy cows. Comput. Electron Agric. 143, 262–272. doi: 10.1016/j.compag.2017.10.024
Uddin M. E., Aguirre-Villegas H. A., Larson R. A., Wattiaux M. A. (2021). Carbon footprint of milk from Holstein and Jersey cows fed low or high forage diet with alfalfa silage or corn silage as the main forage source. J. Clean Prod 298, 126720. doi: 10.1016/j.jclepro.2021.126720
Upadhyay M., Eriksson S., Mikko S., Strandberg E., Stålhammar H., Groenen M. A. M., et al. (2019). Genomic relatedness and diversity of Swedish native cattle breeds. Genet. Sel Evol. 51, 0–11. doi: 10.1186/s12711-019-0496-0
Van Soest P. J., Robertson J. B., Lewis B. A. (1991). Methods for dietary fiber, neutral detergent fiber, and nonstarch polysaccharides in relation to animal nutrition. J. Dairy Sci. 74, 3583–3597. doi: 10.3168/jds.S0022-0302(91)78551-2
Keywords: non-invasive, sustainability, native, methane, efficiency
Citation: Azevedo AR, Lopes MS, Borba A, da Câmara Machado A and Mendonça D (2024) Exploring the Catrina, an autochthonous cattle breed of the Azores, for a comparative analysis of methane emissions with Holstein-Friesian dairy cows. Front. Anim. Sci. 5:1423940. doi: 10.3389/fanim.2024.1423940
Received: 30 April 2024; Accepted: 12 August 2024;
Published: 12 September 2024.
Edited by:
Susana P. Alves, University of Lisbon, PortugalReviewed by:
Severiano Silva, Universidade de Trás-os-Montes e Alto, PortugalRidha Ibidhi, INRA Centre Dijon Bourgogne Franche-Comté, France
Copyright © 2024 Azevedo, Lopes, Borba, da Câmara Machado and Mendonça. This is an open-access article distributed under the terms of the Creative Commons Attribution License (CC BY). The use, distribution or reproduction in other forums is permitted, provided the original author(s) and the copyright owner(s) are credited and that the original publication in this journal is cited, in accordance with accepted academic practice. No use, distribution or reproduction is permitted which does not comply with these terms.
*Correspondence: Artur da Câmara Machado, YXJ0dXIuYy5tYWNoYWRvQHVhYy5wdA==