- 1School of Biodiversity, One Health & Veterinary Medicine, College of Medical, Veterinary and Life Sciences, University of Glasgow, Glasgow, United Kingdom
- 2Institute of Aquaculture, University of Stirling, Stirling, United Kingdom
Decapod crustaceans provide a valuable food source worldwide, both through wild capture and captive rearing. They are also used extensively in scientific research, both as subjects for the investigation of basic biological processes and as model organisms for the detection of environmental changes. There is now an increasing acceptance that decapod crustaceans are sentient, and legislation is being introduced in numerous countries to ensure crustacean welfare when they are harvested or held captive. Moreover, methods for the humane slaughter of these animals are being developed, and of these electrical stunning is a prime candidate. Optimisation of electrical stunning is underway for a range of commercially-exploited or scientifically-important species, and the present study contributes to that process by examining further crab (Carcinus maenas) and lobster (Homarus gammarus) species using a rigorous neurophysiological approach. By recording nerve activity in both the central and peripheral nervous systems, we have found that electrical stunning with a standard commercial instrument arrests nerve activity in both these species at all levels: sensory, motor and central, rendering the animals neurologically insensible. This methodology is the most direct and effective way to establish if insensibility is successfully achieved. However, ultimately the routine monitoring of effective stunning in commercial and laboratory settings will have to depend on simple, yet reliable physiological or behavioural indicators, following their calibration against neurological methods. Monitoring heartbeat is used widely to establish metabolic activity, locomotory performance, agonistic interactions and responses to environmental conditions. We have therefore assessed the potential to use cardiac activity as an indicator for the state of sensibility. We recorded the heartbeat in both C. maenas and H. gammarus before and after electrical stunning, but find that in many cases even though nerve activity has ceased both centrally and peripherally, cardiac activity actually continues (though at a reduced rate) for a substantial time (>1h). The heartbeat is therefore not a reliable indicator of the state of sensibility, making it an unsuitable indicator of effective stunning. Possible reasons for these findings, and ways to validate behavioural measures that may be more appropriate for routinely establishing effective electrical stunning, are discussed.
1 Introduction
In many countries increased attention is being paid to the welfare of decapod crustaceans (crabs, lobsters, crayfish and prawns) at all stages of their capture, holding and use for both commercial and scientific purposes. As well as being an important source of food for human consumption through both wild capture fisheries (6 million tonnes, 350 billion animals annually worldwide) and aquaculture (8.5 million tonnes, 500 billion animals) (FAO, 2021; Boenish et al., 2022), decapod crustaceans have become important models for biochemical, physiological, immunological, toxicological and ecological research (see Passantino et al., 2021 for review).
In order to decide how welfare standards are applied to certain animal groups, their capacity to feel, also known as sentience, is often sought. This embodies felt experiences, including positive and negative feelings (e.g. pleasure, comfort, distress, hunger etc.) as well as sensory experiences (including tactile, olfactory, auditory and visual) (Crump et al., 2022a). Evidence for decapod crustaceans being sentient has been put forward by several authors (Broom, 2014; Elwood, 2019), and for this reason legislative measures are coming into place worldwide requiring the welfare of these species to be accommodated in their capture, holding and slaughter procedures. Countries that currently place decapod crustaceans under some degree of legal protection include Australia, Austria, New Zealand and Norway. For example, in New Zealand the Animal Welfare (Care and Procedures) Regulations 2018 now require that any farmed or caught animal of these species that are killed for commercial purposes must first be rendered insensible. In the UK, under the former UK Animal Sentience Bill, decapod crustaceans were not covered, by definition, as ‘animals’ (DEFRA, 2021). However, using a framework centred around neural and behavioural responses to pain, a recent review concluded that, based on current evidence (albeit from a small literature and for a limited number of species) the threshold for sentience in decapod crustaceans is indeed met (Birch, 2017; Birch et al., 2021; Crump et al., 2022a). Accepting these findings, The UK government has passed the Animal Welfare (Sentience) Act 2022 (DEFRA, 2022) which recognises that all decapod crustaceans are sentient, and this Act will provide oversight of any other new legislation. Calls have now been made for the use of decapod crustaceans in scientific research to be subject to ethical review (Rowe, 2018), and also in the UK for the law to be extended so that the existing Animal Welfare Act 2006 (which only protects vertebrates) and the Animals in Scientific Procedures Act 1986 (which only protects vertebrates and cephalopods) include decapod crustaceans (Crump et al., 2022b). If this were to happen, both commercial practices and scientific studies that involve decapod crustaceans in the UK will become much more strictly regulated.
With these developments, there is increasing focus on methods for the humane slaughter of decapod crustaceans. For this to occur, animals should be stunned in a way that induces immediate insensibility that lasts until subsequent slaughter can be performed without avoidable fear, anxiety, pain, suffering and distress (EFSA, 2005; FAO, 2020). It is worth noting that there is a vast range of terms (e.g. insentient, insensible, unconscious etc.) that can be used to describe the intended state after a method of stunning has been applied. For the purposes of this paper, we shall employ the term ‘insensible’ throughout. ‘Insensible’ provides a more tangible definition pertaining to the ability of the animal to sense presented stimuli and respond to them, which directly aligns with the aims of this work.
From an animal welfare perspective, both the onset and persistence of insensibility are important in order to ensure that no recovery occurs before subsequent death. Methods for the stunning and slaughter of decapod crustaceans are varied and include cold shock in air, ice or slurry ice, boiling, freshwater or salt baths, carbon dioxide narcosis, high pressure, mechanical splitting or spiking of the nervous system, dismemberment and electrical stunning (Yue, 2008). However, the majority of these methods are considered inhumane by the European Union’s Scientific Panel on Animal Health and Welfare (EFSA, 2005) since most take some time to have an effect and hence have the potential to confer suffering or stress. Electrical stunning has been identified as one of the most acceptable methods for the humane slaughter of decapod crustaceans, and optimisation of electrical stunning is underway for a range of commercially-exploited or scientifically-important species (Conte et al., 2021). There is now a need to provide strong evidence that these methods achieve humane slaughter by rapidly inducing insensibility in these species.
Since the state of sensibility is intrinsically linked to activity in the brain and associated nerves, recordings from the nervous system are the most direct and appropriate way to assess whether the insensibility is successfully achieved following electrical stunning. Such studies have so far been made on only a limited number of decapod species (Table 1), and the range of species investigated now needs to be extended, encompassing those of different sizes, lifestyles and habitats. To contribute to this wider survey, we have performed trials on two further species that are of importance as biological models and/or commercially, namely the shore crab Carcinus maenas (L.) and the European lobster Homarus gammarus (L.).
The shore crab Carcinus maenas (L.) is one of the most widely studied decapod crustaceans (see chapters in Weihrauch and McGaw, 2023). It is able to survive in a highly variable estuarine environment and has a high tolerance to anthropogenic pollution by heavy metals, organic contaminants, pesticides and nano-plastics. For this reason, it has been used extensively in ecotoxicological research (Rodrigues and Paldal, 2014; Griffin et al., 2023). C. maenas has been a tremendously successful invader of ecosystems, and within little more than 200 years it has become a worldwide invasive species (Lowe et al., 2000; Klassen and Locke, 2007). Eradication by harvesting has been considered, with another option being its utilisation as a resource (e.g. for lobster bait, food or even whisky). If legislation was introduced, then the slaughter of large numbers of these crabs would have to occur humanely, and the methods for this would have to meet appropriate ethical standards.
The European lobster (Homarus gammarus L.) has also been the subject of biological research (documented in Factor, 1995 and in Phillips, 2013) but in addition forms the basis of an important fishery. Its population range is mainly centred around the British Isles where it is caught by trapping in lobster pots (‘creels’), with annual landings of ~3000 tonnes (Cefas, 2020). Seasonal prices can be as high as £20 per kg at first sale, making this lobster one of the most valuable crustaceans in the UK. It is often traded, transported and presented live prior to consumption as a luxury seafood product in high-end outlets, and for this reason there is often customer concern for its ethical treatment and humane killing. Since landings of the closely-related American lobster (Homarus americanus H. Milne Edwards) are more than 50x greater (160,000 tonnes, FAO, 2020; Hvingel et al., 2021) such issues are potentially even more significant if legislation were to be introduced for them.
Ultimately, the routine monitoring of effective stunning of decapod crustaceans in commercial and laboratory settings will have to depend on simple, yet reliable criteria, after they have been calibrated against neurological methods. However, until such reliable indicators of insensibility are identified it is necessary to continue performing laboratory studies directly on the nervous system to confirm that the stunning protocol meets the criteria for humane slaughter. By its nature the methodology for measuring neuronal activity can be invasive, but this is justified in a set of limited scientific trials aimed at identifying unambiguously the effectiveness of electrical stunning procedures in producing a state of insensibility (Albalat et al., 2022; Neil et al., 2022). These neuronal criteria can serve as a reference to confirm whether more convenient, yet less intrusive, physiological measures (e.g. of heartbeat or ventilation) or behavioural indices (e.g. intrinsic or reflex movements) can be used as proxy measures for insensibility. As reported here, we have adopted this approach to investigate the utility of cardiac activity as an indicator of effective electrical stunning.
Heart rate (HR) is used as a standard physiological measure in a wide variety of vertebrate and invertebrate taxa (e.g., DeFur and Mangum, 1979; von Borell et al., 2007). In decapod crustaceans, HR provides important information on the metabolic changes and responses to environmental perturbations (McGaw and Reiber, 2015; McLean and Todgham, 2015; Kushinsky et al., 2019), acute stress responses (Burnovicz et al., 2009; Yazawa, 2015) and for ecotoxicology studies (Bamber and Depledge, 1997; Kholodkevich et al., 2017). Monitoring HR is also used widely to establish metabolic activity, locomotory performance, agonistic interactions and responses to environmental conditions and biotic variables (Handy and Depledge, 1999; Green, 2011). We have therefore assessed the potential to use HR as an indicator of the state of sensibility in decapod crustaceans by monitoring it in both C. maenas and H. gammarus before and after electrical stunning.
2 Methods
The methodologies used to electrically stun the C. maenas crabs and the H. gammarus lobsters, and to make neurological recordings, are here summarised and were the same as reported by Albalat et al. (2022) and Neil et al. (2022), where more detailed descriptions can be found.
2.1 Animal supply and holding
The Carcinus maenas (L.) crabs used in these trials were all males of 65-75 mm carapace width in the intermoult stage, and were obtained live from commercial suppliers (UMBSM Animal Supply Service Millport and Loch Fyne Sea Farms Ltd., UK). They were housed initially in communal tanks, within a closed seawater circulating system at 10°C, then retained individually in tanks for at least two weeks before experimentation. From this stock, 6 crabs were subjected to electrical stunning for electrophysiological recording, 4 crabs were used for measuring HR and another 6 were used as controls.
The European lobsters (Homarus gammarus (L.) were all males of carapace length 80-95 mm in the intermoult stage. They were captured by commercial fishermen using baited traps (creels) laid offshore from St Abbs on the east coast of Scotland. After banding the claws of the lobsters (a standard practice by fishermen) they were held initially in seawater tanks at the St Abbs Marine Station, then transferred in chilled containers to the University of Glasgow. As with the crabs, they were housed initially in communal tanks, within a closed seawater circulating system at 10°C, then retained individually in tanks for at least two weeks before experimentation. From this stock, 6 lobsters were subjected to electrical stunning for electrophysiological recording, 6 lobsters were used for measuring HR and another 6 used as controls.
2.2 Electrical stunning
Electrical stunning was applied to individuals of each species without prior anaesthesia using a commercially available device (Crustastun™; Studham Technologies Ltd, Cambridge, UK) following the manufacturer’s operating procedures. The chamber of the stunning device was filled with a salt solution (NaCl 3 g/L) and a stunning cycle of 110 V, 2–5 amp electrical charge for 5 s for the C. maenas crabs and 10 s for the larger H. gammarus lobsters was applied.
2.3 Animal preparation
Crabs not to be subjected to electrical stunning were held on ice for 30 min prior to experimentation to reduce their metabolic activity and to induce torpor, while those subjected to electrical stunning were used directly after the stun.
In order to expose the central nervous system (CNS) of the crab for recording (Figure 1), the carapace was removed and the preparation was submerged in a balanced salt solution corresponding in composition and osmolarity to crab haemolymph, at a temperature of 10°C. The internal organs were then displaced in order to expose the circumoesophageal connectives around the base of the stomach.
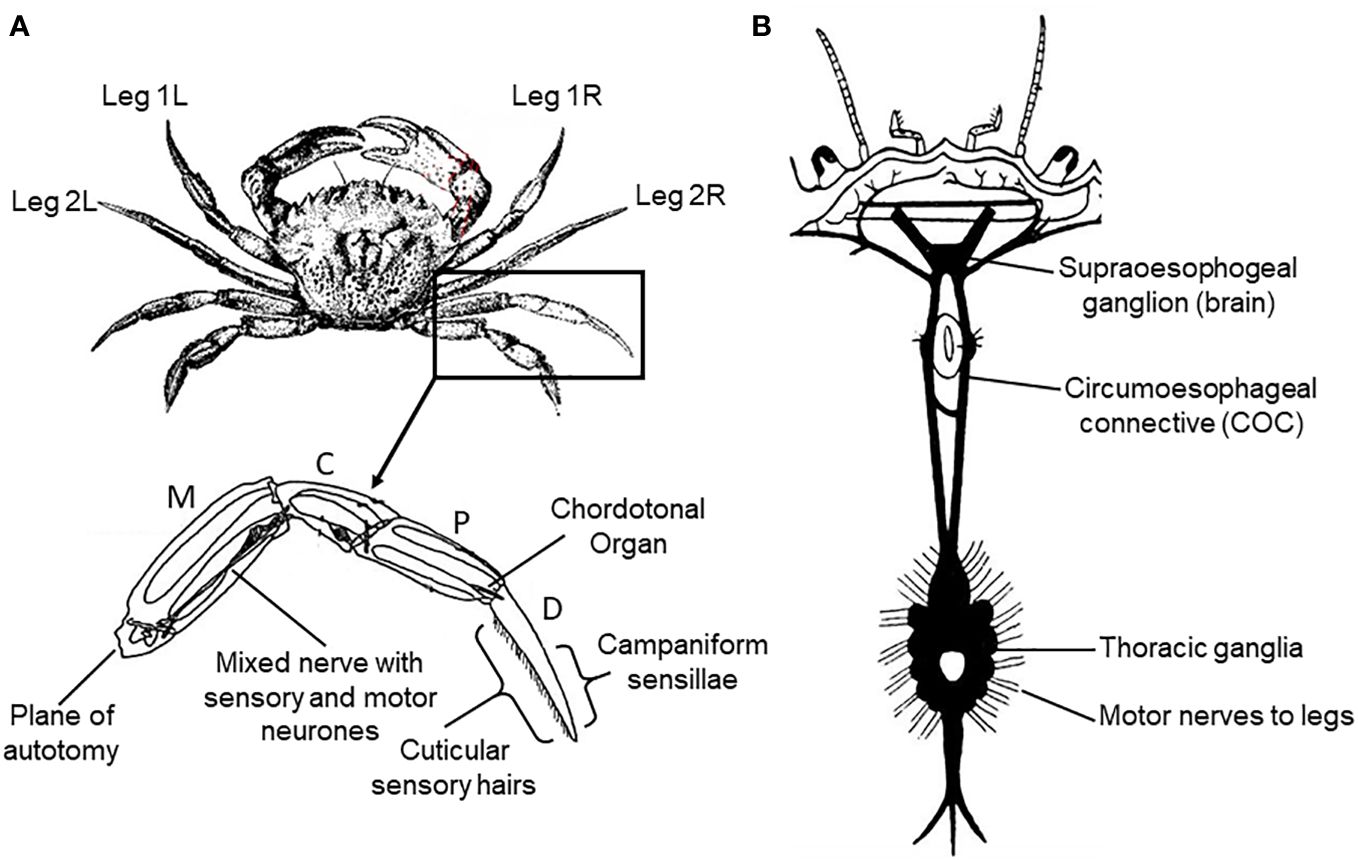
Figure 1 Schematic diagram of the anatomy of the crab Carcinus maenas L. (A) External anatomy, numbering of legs and leg anatomy showing the segments (M, meropodite; C, carpopodite; P, propopodite; D, dactylopodite), and the sense organs stimulated in the current study. (B) Arrangement of the nervous system (dorsal aspect).
A similar procedure was employed for the lobster, but after the cephalothorax was separated from the abdomen. To expose the abdominal ventral nerve cord of the lobster for recording, the dorsal skeletal plates (terga) were detached and the bulk of the underlying deep flexor musculature was removed. The preparation was then submerged in a balanced salt solution corresponding in composition and osmolarity to lobster haemolymph, at a temperature of 10°C. Selective removal of muscle blocks then revealed the motor roots emerging from the ventral nerve cord.
Isolated legs of both crabs and lobsters were prepared for nerve recording and, in the case of crabs, also nerve stimulation, by inducing intact animals to shed a leg spontaneously (autotomy) by applying pressure to the basipodite segment (McVean, 1975; Smith and Hines, 1991). The same procedure was followed for the electrically-stunned animals, except that the legs were detached by amputation (since these animals did not express the autotomy reflex). In all cases the joint between the meropodite and carpopidite (Figure 1A) was then disarticulated, the muscle tendons spanning this joint were cut and the leg was separated at this point with the leg nerve still attached to the distal portion. The leg nerve was teased into a number of separate bundles, to facilitate selective recording or stimulation. This isolated leg preparation was then submerged in physiological saline at 10°C until required.
2.4 Electrophysiological recordings
2.4.1 Recording from central nervous system
Electrophysiological recordings were made from the exposed nerves using various extracellular techniques. For recording from the circumoesophageal connectives of both crabs and lobsters, and from the ventral nerve cord of lobsters, a suction electrode method was used. A fine-tipped polythene electrode containing salt solution was applied to the surface of the nerve, and a gentle suction was applied through attached tubing and a syringe. A silver wire positioned close to the tip of the electrode acted as the indifferent (reference) electrode. Such a recording configuration is termed ‘en passant’, as it involves attaching the suction electrode to an intact nerve, allowing both directions of nerve transmission to be recorded. The signals were digitised using an analogue to digital converter (PowerLab, AD Instruments) and recorded to computer file (LabChart v7 software, AD Instruments) for storage and display.
2.4.2 Recording sensory activity
For recording sensory activity from the crab and lobster leg nerves, the isolated leg was clamped to a Perspex plate and the nerve was passed from an adjacent bath through a wall of petroleum jelly into a second small chamber, both of which contained balanced salt solution. A bipolar electrode of two silver wires was used to make contact with the solutions in the inner and outer chambers respectively.
2.4.3 Stimulating the motor nerves in crabs
To stimulate the motor axons in the crab leg nerve, the bipolar electrodes were connected to an isolated stimulator within the PowerLab interface and patterns of stimulating pulses at various amplitudes and frequencies were applied using a software ‘stimulator control panel’ within the LabChart v7 software. Typically, stimulus trains of 3 s duration and 4 V amplitude were applied at a range of frequencies from 1 -100 Hz.
2.4.4 Recording muscle force in crabs
Although leg nerve stimulation potentially activated motor neurones supplying all of the muscles located more distally in the crab leg, the forces produced by the closer muscle of the propopodite/dactylopodite joint (P-D) (Figure 1A) were nevertheless recorded selectively. This was achieved by cutting the tendon of the antagonist muscle about that joint (the P-D opener muscle), and then attaching a thread from near the tip of the dactylopodite to the arm of a sensitive force transducer (FT-03, Grass Instruments Ltd.), mounted on a micromanipulator. This selectively monitored the forces produced by the dactylopodite closer muscle. The output of the transducer was passed to a custom built amplifier (x1000), and then fed to the PowerLab interface for storage and display of the stimulus parameters and muscle forces.
2.5 Recording cardiac activity
Cardiac activity was monitored in both C. maenas and H. gammarus using a standard impedance methodology (Wycoff et al., 2018; Harrington et al., 2020). About 3 h prior to experimentation the animal was held on ice for 30 min to reduce its metabolic activity and to induce torpor. Holes were drilled in the dorsal carapace using a syringe needle at positions on either side of the heart, and thin silver wires (0.2 mm diameter), insulated except for the tip, were inserted by 1-2 mm and secured in place, and then tethered along the external surface of the carapace, with cyanacrylate adhesive. The animal was then returned to a holding tank for 2 h. During this time the wires were connected to a purpose-built impedance converter and the output of this was fed to the input of the PowerLab interface. In some cases, a window discriminator function of the LabChart v7 software was used to display the heart rate as a running average of the beats per minute (BPM). In others, a calculation of the HR was made at specified time points by direct counts of the beats over a 15s interval, which were then transformed to BPM.
Immediately prior to electrical stunning the recording wires were isolated from the electronics and the animals were subjected to a standard electrical stun (5 s for crab and 10 s for lobster). The cardiac recording was resumed immediately after the stun. Additionally, in the case of some lobsters, immediately before stunning one leg was autotomised (routinely leg L2) and prepared for recording, and immediately after stunning another leg (routinely Leg R2) was also removed and prepared for recording, before cardiac monitoring was recommenced.
2.6 Ethical statement
Ethical approval for procedures on decapod crustaceans is currently not required in the UK. Nevertheless, all the live crabs and lobsters used were treated with proper care in order to minimise their discomfort and distress. There was no practical alternative to the use of live animals. The total number used (16 crabs and 18 lobsters) was the minimum required to obtain a reasonable threshold of certainty, considering that the gain in knowledge and long-term benefit to the subject will be significant. Prior to experimentation they were housed communally to ensure behavioural enrichment. Experimental design considered the 3R’s (Replacement, Reduction and Refinement). Minimisation of suffering and distress was inherent to the experimental design in that the objective was to render the crabs and lobsters insensible by electrical stunning prior to, if necessary, inducing death. No anaesthesia was used. The exposure of subjects to experimental treatment did not exceed 5 s for crabs and 10 s for lobsters. To ensure welfare, behaviour of experimental subjects was monitored for indications of consciousness recovery every 15 min following stunning. None were apparent up to 4 h after stunning, when the animals were then euthanised by freezing.
3 Results
3.1 The effectiveness of electrical stunning as a stunning and slaughter procedure for C. maenas
3.1.1 Central nervous system
Recordings made from the circumoesophageal connectives in intact C. maenas crabs (Figure 1B) indicated that there was a high level of spontaneous neuronal activity involving a large number of axons in these nerves, even in the absence of any imposed stimulation (Figure 2, upper traces). In order to test the persistence of activity in the CNS, some preparations were re-tested at intervals, and activity continued for up to the longest time tested (4 h) (data not shown).
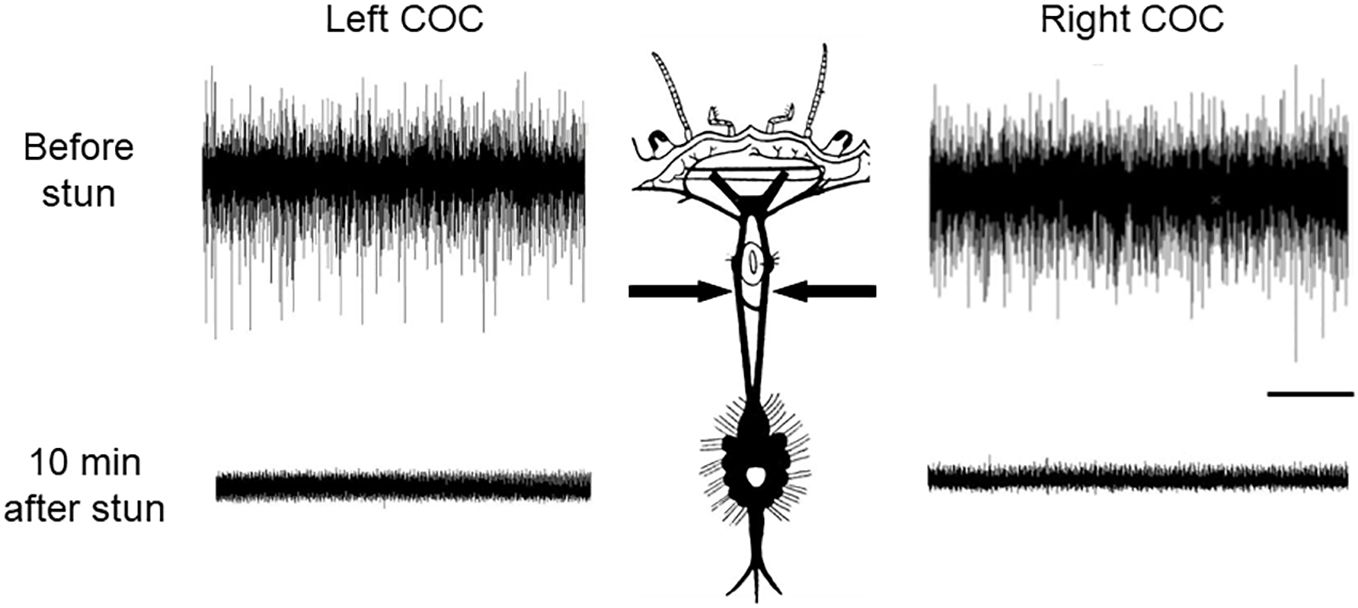
Figure 2 Recordings of CNS activity in C. maenas before and after electrical stunning. Spontaneous nerve activity recorded extracellularly in the left and right circumoesophageal connectives (COC) (arrows). Upper traces intact crab; Lower traces: 10 min after electrical stunning. Scale bar, 1 s.
Recordings from the CNS of crabs that had been subjected to electrical stunning indicated that no neuronal activity was detectable in the circumoesophageal connectives in any of the individuals at either 10 min after stunning (Figure 2, lower traces), or up to the longest time tested (4 h) (data not shown). Consistent results were obtained from all of the 6 crabs tested.
3.1.2 Sensory
Activity in the peripheral nervous system in intact crabs was recorded from sensory neurones in isolated legs, following autotomy. Brushing movements over the cuticle of the dactyl produced bursts of activity typical of sensory hairs (Figure 3, L2 top trace). Compression (squeezing) of the cuticle activated campaniform sensilla. Finally, flexions and extensions applied at the P-D joint elicited the phasic and tonic components characteristic of chordotonal organs (Figure 1A). These sensory responses persisted for at least 4 h when they were as strong and of the same pattern as those recorded immediately after autotomy. After electric stunning of the crabs, sensory activity in the nerves of all the amputated legs tested was absent in response to all of the three stimuli applied (Figure 3, R2 lower trace), and there was no apparent recovery of responsiveness up to the longest time tested (4 h).
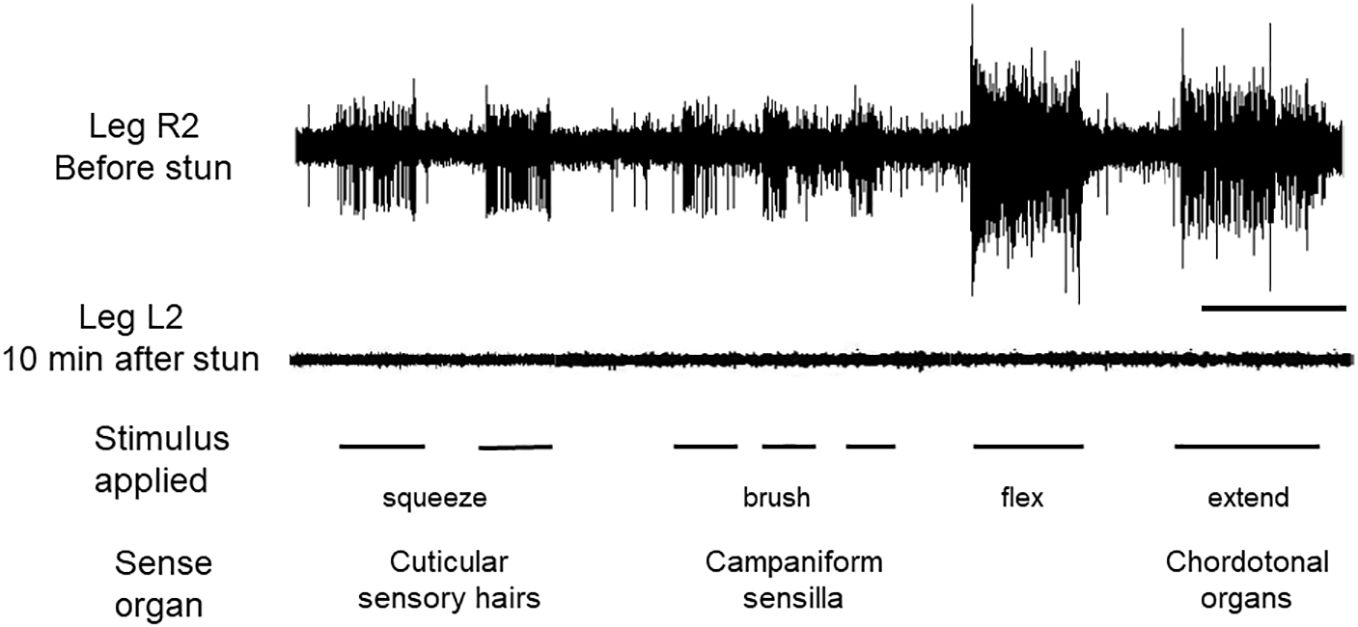
Figure 3 Recordings of sensory activity in C. maenas before and after electrical stunning. Responses of the leg nerve to three forms of stimulation of the dactylopodite, as indicated. Upper trace, leg R2 autotomised from intact animal before stunning; lower trace, leg L2 amputated from same animal after electrical stunning. Scale bar, 10 s.
3.1.3 Motor
The normal operation of the motor pathways of the peripheral nervous system was demonstrated by stimulating the nerve of an autotomised leg from an intact crab at various frequencies while monitoring the force produced by the dactylopodite closer muscle (Figure 1A). The force varied in a frequency-dependent manner typical of crustacean neuromuscular systems (Figure 4, upper trace). By contrast, after electrical stunning there was no detectable force developed by the closer muscle in response to stimulating the motor nerves either at 10 min (Figure 4, lower trace), or up to 4 h.
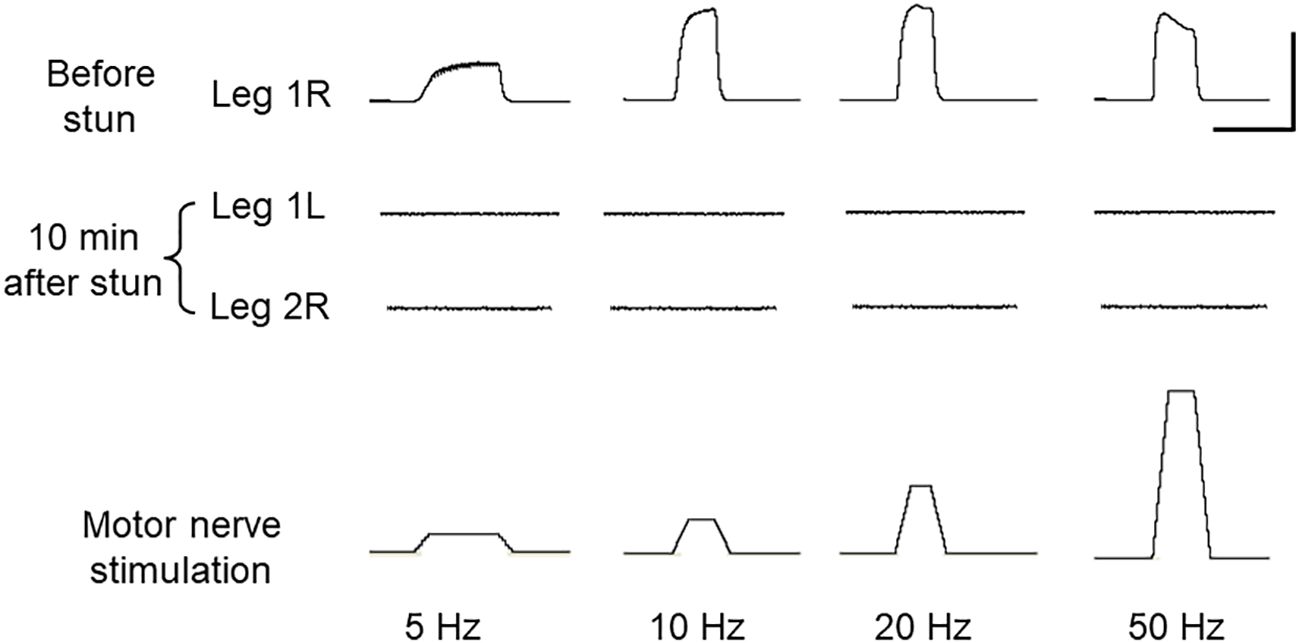
Figure 4 Motor output in C. maenas before and after electrical stunning. Forces produced by the dactylopodite closer muscle of the leg in response to stimulation of motor neurones in the leg nerve at various frequencies. Top trace: leg R1 autotomised from intact crab; Middle two traces: legs L1 and L2 amputated from the same crab after electrical stunning, measured at 10 min after the stun. Bottom trace: stimulus applied to leg motor nerve (4 V at various frequencies). Scale bars, 3 s and 500 mN.
3.2 The effectiveness of electrical stunning as a stunning and slaughter procedure for H. gammarus
In untreated lobsters, neuronal traffic was recorded in the CNS both anteriorly in the circumoesophageal connective and posteriorly in the abdominal nerve cord. In addition, evoked activity was stimulated in the leg sensory nerves by mechanical stimulation of cuticular receptors, and spontaneous activity was recorded in the segmental motor nerves that serve to maintain tone in the tail musculature. Consistent results were obtained from the 6 animals tested, and representative traces are shown Figures 5A–D, upper traces.
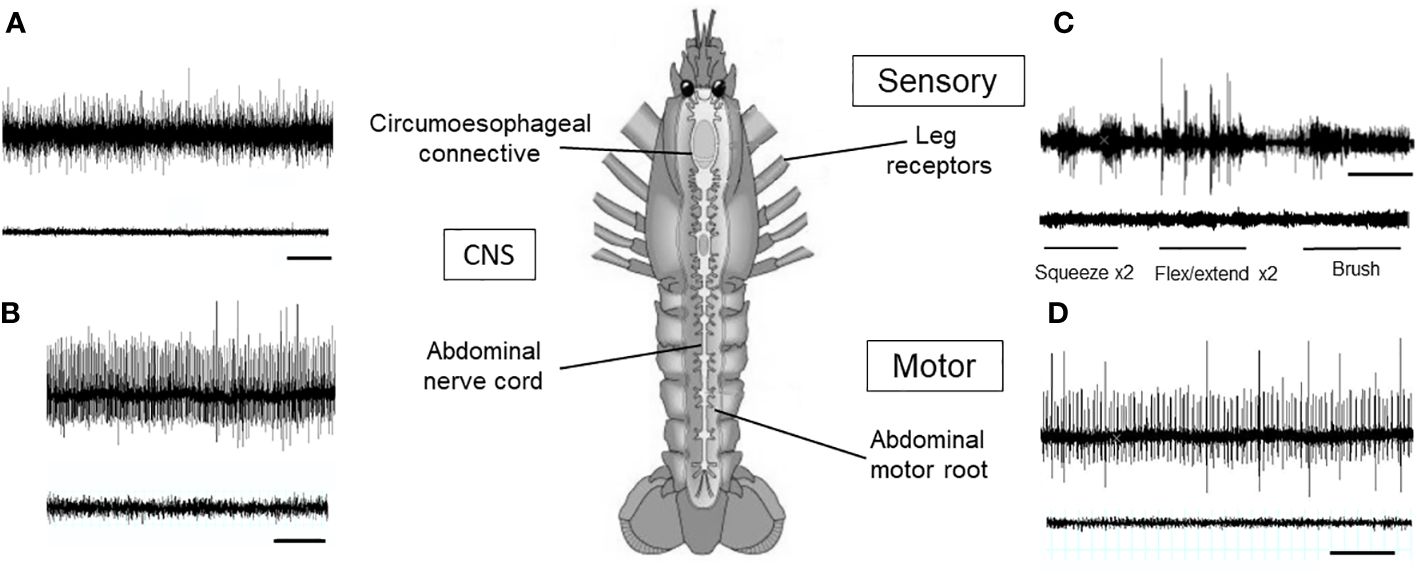
Figure 5 Recordings of neuronal activity in H. gammarus before and after electrical stunning. (A) Spontaneous activity recorded in the left circumoesophageal connective. (B) Spontaneous activity recorded from the left connective between segments 2 and 3 of the abdomen. (C) Responses of the leg nerve to three forms of stimulation of the dactylopodite, as indicated. Top trace, leg R2 autotomised from intact animal before stunning; lower trace, leg L2 amputated from same animal after electrical stunning. (D) Spontaneous activity recorded in the 3rd motor root of the fourth abdominal segment. In all panels, upper traces: animal before electrical stunning; lower traces: animal 10 min after electrical stunning. Scale bars, 1 s in (A, B, D) 10 s in (C).
When first measured following electrical stunning (10 min) there was a total absence of activity at all levels within the lobster CNS (circumoesophageal connective and abdominal nerve cord) (Figures 5A, B, lower traces), and also in both the sensory and motor nerves (Figures 5C, D, lower traces). Again this was found to be the case in all 6 animals tested, indicating that all these animals were effectively stunned by this procedure. Moreover, no recovery of nerve activity (nor any limb movements) could be observed even 4 h later.
3.3 The effect of electrical stunning on the cardiac activity of C. maenas and H. gammarus
In both C. maenas and H. gammarus the same protocols that elicited successful electrical stunning were applied to intact animals prepared for recording cardiac activity. For the four crabs tested, after a 2 h period of acclimation following the insertion of electrodes their HR was in the range 56-66 bpm (Figures 6, 7A). After an electrical stun of 5 s there was a silent period of 1-2 min, and then the heartbeat returned gradually, being initially arrhythmic but later more regular, and reached a rate that varied between individuals from 20% and 75% of their pre-stun values. Post-stun, the amplitude of the recorded waveform was also reduced (Figure 6). This cardiac activity continued for as long as the recording was made (between 20 and 30 min) (8A).
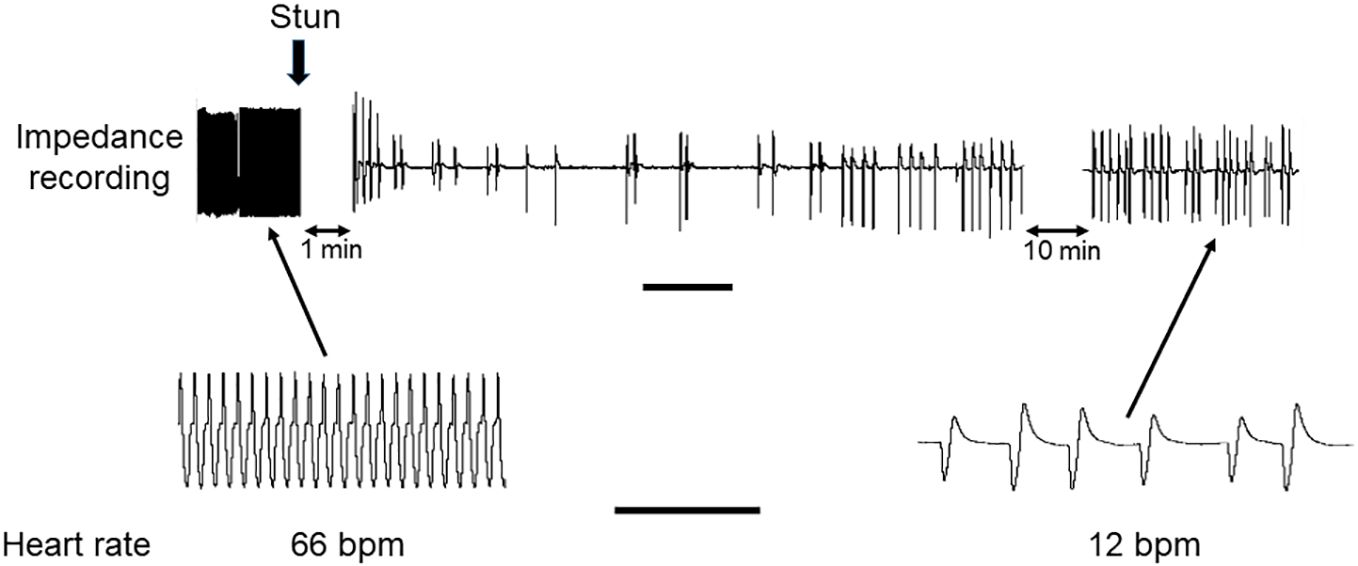
Figure 6 Recording of HR in C. maenas before and after electrical stunning. Representative recording of HR of a crab obtained by the impedance method. The time of the electrical stun is marked by the vertical arrow, and the recording was paused for 1 min afterwards, and subsequently for 10 min. Lower panels show traces on an expanded time scale. Scale bars, 1 min for upper panel; 10 s for lower panels.
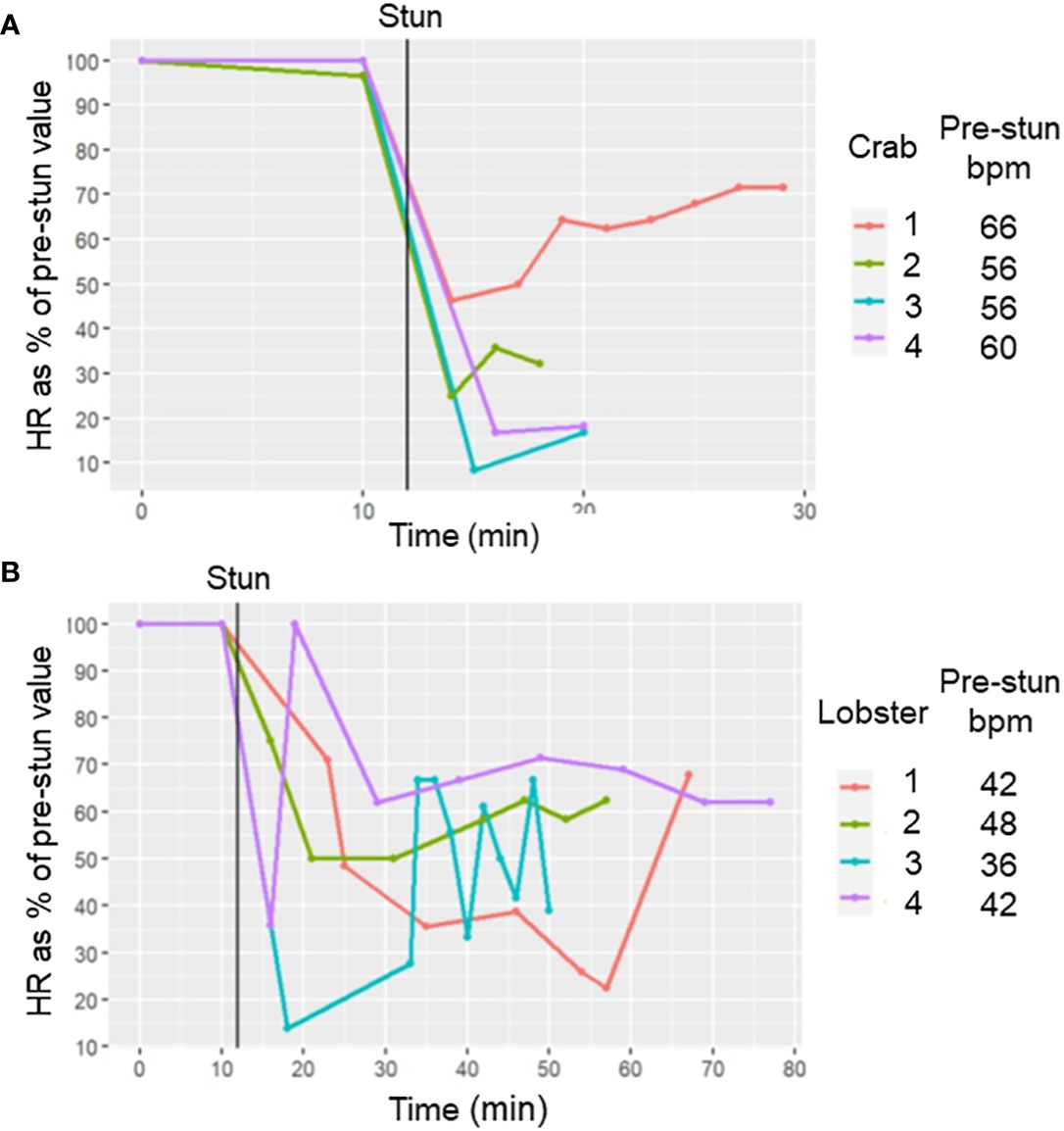
Figure 7 Effect of electrical stunning on the HR of C. maenas and H. gammarus. HR (as % of its pre-stun average value) at different times after electrical stunning for (A) 4 crabs and (B) four lobsters. The time of the stun is indicated by the vertical black line. The average HR values (in bpm) before stunning are indicated in the legends.
Results for lobsters followed the same trends. For the four lobsters tested the acclimated prestun HR values ranged from 32-48 bpm (Figures 7, 8B). After an electrostun of 10 s there was a silent period of 1-2 min, and then the HR returned, being irregular initially but subsequently more regular, and reached rates that varied between individuals from 40% and 70% of their pre-stun values (Figures 7B, 8). Post-stun, the amplitude of the waveform was reduced, and also showed long-term variation (Figure 8). This cardiac activity continued for as long as the recording was made (between 50 and 75 min) (Figure 7B).
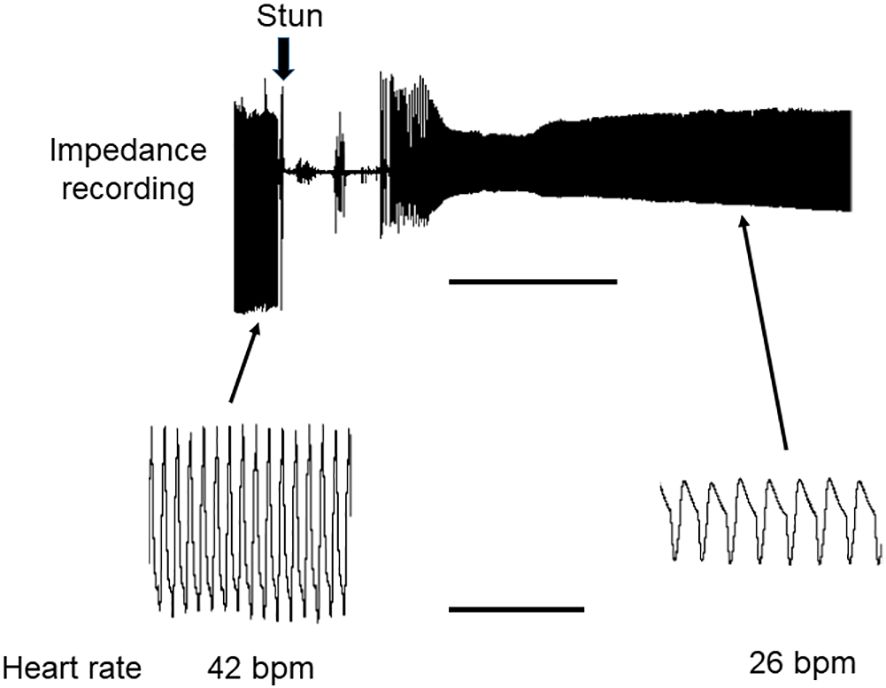
Figure 8 Recording of HR in H. gammarus before and after electrical stunning. Representative recording of HR of a lobster obtained by the impedance method. The recording was continuous, and the time of the electrical stun is marked by the vertical arrow. Lower panels show traces on an expanded time scale. Scale bars, 10 min for upper panel; 10 s for lower panels.
In a separate trial using two lobsters, simultaneous recordings were made of neuronal activity (sensory responses in an autotomised leg), and cardiac activity in an individual, both before and after electrical stunning. The absence of leg sensory responses was taken as an indication of the lack of neuronal activity. The results for both lobsters were the same, and one is shown in Figure 9. Before stunning, both the sensory responses to two different stimuli and the cardiac activity were normal (Figure 9A). After electrical stunning the neuronal responses ceased, but the heart continued to beat, albeit with a reduced frequency and amplitude, for as long as the recordings were made (30 min) (Figure 9B).
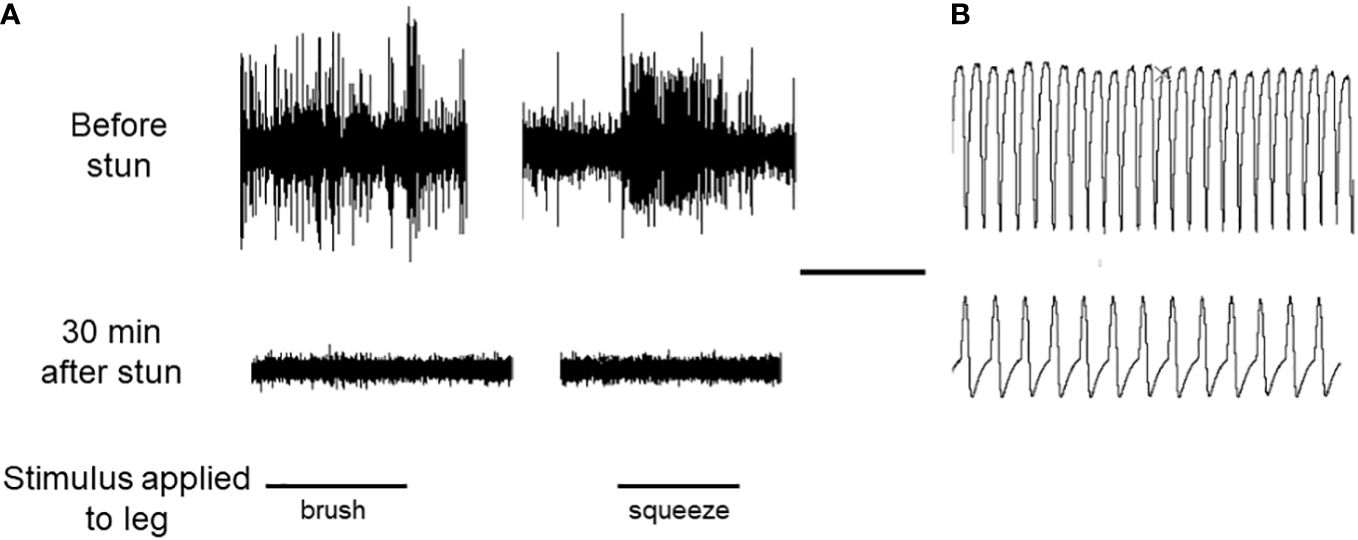
Figure 9 Recordings of neuronal activity and HR in an individual lobster. (A) Responses of the leg nerve of a H. gammarus preparation to two types of stimulation of the dactylopodite, as indicated. Upper trace, leg R2 autotomised from intact animal before electrical stunning; lower trace, leg L2 amputated from same animal after electrical stunning, recorded 30 min later. (B) Sample recordings of the HR of the same lobster, taken before the stun (upper panel) and 30 min after the stun (lower panel). Scale bar, 10 s for both (A, B).
4 Discussion
The Council Regulation (EC) No 1099/20091 on the protection of animals at the time of killing defines ‘stunning’ as ‘any intentionally induced process which causes loss of consciousness and sensibility without pain, including any process resulting in instantaneous death’. According to The European Food Safety Authority (EFSA), the most valid measures to assess insensibility are electroencephalograms (EEG), which are able to elucidate even subtle changes in neurological activity (Blokhuis, 1984). The neurophysiological recordings made in the present study on two species of decapod crustaceans represent an equivalent approach.
4.1 Neuronal indicators of insensibility
The results obtained here are consistent with the literature on the neurophysiology of crustacean nervous systems (Wiese, 2002) in showing that the central nervous systems of intact C. maenas and H. gammarus both display continuous nerve activity, and in turn receive inputs from sensory nerves in the periphery and produce outputs in the motor nerves to the body and limb muscles. It is also well established that this activity persists even when parts of the CNS are isolated from each other by severing the nerve cord at one or more levels (Marder and Bucher, 2007).
We found that following electrical stunning all normal neuronal functioning ceases, both centrally and peripherally. According to the manufacturer, the target stunning current of the Crustastun™ (1.3 amp) is achieved within 0.5 s and is maintained throughout the stun cycle. It is impossible to know with certainty if nerve conduction fails at this point, as all electrical signals are completely masked by the artefact generated by the stun current. However, it is a reasonable assumption that this occurs almost instantaneously, within the stun cycle (10 s). After electrical stunning we found no recovery of central nervous activity, sensory responsiveness or motor action in all the 6 crabs and all the 6 lobsters within the period examined (up to 4 h post-stun). This demonstrates that this method may be effective in rendering both C. maenas and H. gammarus rapidly and persistently insensible under the conditions used in this study.
A number of other studies have used neurological recordings to evaluate electrical stunning. Fregin and Bickmeyer (2016) used implanted electrodes in the abdominal nerve cord of the American lobster Homarus americanus and the crayfish Astacus leptodactylus, and analysed the signals using Fourier analysis (FFT). More recently, Kells et al. (2023) recorded global neural activity from superficially placed electrodes placed anteriorly and posteriorly on the spiny lobster Jasus edwardsii and the crayfish Paranephrops zealandicus. In our laboratory we have previously used the methods of selective extracellular recording described here to record from the central, sensory and motor systems of the crab Cancer pagurus (Neil et al., 2022) and the Norway lobster Nephrops norvegicus (Albalat et al., 2022). Taken together with the results of the present study on Carcinus maenas and Homarus gammarus, there is now available data for a number of crabs, lobsters and crayfish, which also encompass a range of sizes (body weights from around 50 g up to 1 kg) (Table 1).
Despite some variation in their methodologies, all these studies demonstrate that a state of insensibility is achieved in this range of decapods within the applied electrostun cycle, i.e. 0.5 -10 s, and can last for up to several hours. This would allow time to take a blood or tissue sample, if required for biological analysis, or for an appropriate procedure for humane slaughter to be applied while the animal is in an insensible state (the concept of the ‘stun-to-kill’ time). In our previous study of brown crabs (Cancer pagurus) (Neil et al., 2022), lack of either nerve activity or behavioural responses at 4 h after stunning was taken to indicate that the crabs were also killed by this procedure, and this was corroborated by holding intact, electrically-stunned crabs for 24 h, and finding no signs of life. Other findings suggest that some caution needs to be applied to this interpretation. Firstly, Fregin and Bickmeyer (2016) found that neural activity begins to recover in electrically-stunned American lobsters (H. americanus) after 2-3 h (see their Figure 6). Secondly, in the present study on the related European lobster (H. gammarus) and also on the crab C. maenas we found that, despite there being no neuronal recovery within 4 h of electrical stunning, there is continued cardiac activity after stunning, which could sustain the metabolism of these animals and so facilitate a long-term neuronal recovery. Taken together, the most conservative interpretation of these findings is that electrical stunning provides a ‘stun-to-kill’ time window of several hours, which, in practical terms would be sufficient in most fishery or laboratory situations to enable a method of dispatch to be applied.
4.2 Cardiac activity and insensibility
While EEG recording has become the norm for the evaluation of different states in vertebrates, the difficulty and invasiveness of EEG measures renders them impractical for use within commercial environments. Ideally, EEG measurements performed in a laboratory setting should be correlated with non-invasive animal-based measures (ABMs) of the state of sensibility, such as behavioural (i.e. changes in movement or loss of equilibrium), physical signs (e.g. changes in ventilation) and physiological reflexes or measures (e.g. cardiac activity), which can be applied by non-specialists outside laboratory settings. We adopted this approach in this study by monitoring heart rate before and after successful electrical stunning.
We have found that after electrical stunning of both C. maenas and H. gammarus, even though nerve activity ceased both centrally and peripherally, cardiac activity continued (though at a reduced rate) for a substantial time (>1h). This is a somewhat unexpected finding, since the heartbeat of decapod crustaceans is neurogenic in origin (i.e. it is generated by the actions of neurones in the cardiac ganglion, rather than from within the cardiac muscle itself), and as such might have been expected to be abolished by electrical stunning, as were the other neuronal actions of the nervous system. This is not an isolated observation, however, since Wycoff et al. (2018) found a similar effect in the responses of crayfish to anaesthesia by eugenol, namely that the heart continues to beat despite the sensory and motor responses of the animal being suppressed. Wycoff et al. (2018) speculate that the cardiac ganglion may in some way be protected or isolated from the circulating anaesthetic. Finding the same effect after electrical stunning, however, throws some doubt on this suggestion, since the electrical current would be expected to flow through all body tissues, including the cardiac ganglion.
The cardiac ganglion is a classic central pattern generator (CPG), and has been researched extensively (see review by Cooke, 2002). It comprises only nine neurones which act as a pacemaker to drive the heartbeat, with both cycle period and amplitude being modulated by sensory feedback from cardiac muscle, and by the actions of numerous neuropeptides (Powell et al., 2023). Additionally, it has been found that homeostatic regulatory mechanisms exist that maintain a target level of excitability in the cardiac ganglion cells by altering their ionic conductances by up to four-fold (Ransdell et al., 2012). This restoration of intrinsic excitability, which can occur over time periods from a few milliseconds up to hours (Marom and Marder, 2023), represents a resilience that stabilises neuronal output of the cardiac CPG and maintains its overall function in the face of perturbations such as temperature or salinity changes, pathology, injury, trauma or experimental manipulations (Ransdell et al., 2013). It is therefore possible that these compensatory responses within the cardiac ganglion CPG also occur in the face of electrical stunning, resulting in the continuation of heart beating that we have observed post-stunning. This cannot be the full explanation, however, since to produce a heartbeat there must also be a functioning neuromuscular connections between the cardiac ganglion cells and the cardiac muscles, and these too must be resistant to the effect of electrical stunning.
More generally, we conclude that since cardiac activity does not cease after an electrostun that totally suppresses activity in the central and peripheral nervous systems, it cannot serve as a proxy indicator of the state of (in)sensibility. Thus, we recommend that future studies aiming to validate the effectiveness of stunning within decapod crustaceans should not be based alone on the assessment of cardiac activity as a physiological index.
Nevertheless, Wycoff et al. (2018) demonstrated that the heartbeat of crabs, crayfish and shrimps is subject to neuronal modulation in response to external stimuli, such as a rapid tap on the rostrum, and that this startle response no longer occurs after anaesthesia with eugenol. Since these changes in cardiac activity are mediated by pathways (sensory-CNS-cardiac) that would also be expected to be subject to suppression by electrical stunning, the disappearance of a cardiac response to startle stimuli could be a potential indicator of loss of central sensibility, even though, due to its continuation, cardiac activity, per se, cannot.
4.3 Behavioural indicators of insensibility
It is well accepted that the assessment of consciousness in vertebrate species is complex. In aquatic vertebrate species, such as fish, visual indicators of consciousness include equilibrium, changes in ventilation and reflexes such as the vestibulo-ocular reflex also known as ‘eye-roll’. However, the lack of movement and clinical reflexes does not necessarily mean that the animal is not sensible to pain or distress (Robb et al., 2000). In fact, recent studies have shown that following electrical stunning, the rainbow trout (Oncorhynchus mykiss) brain is able to respond to external stimuli before ventilation is resumed (Hjelmstedt et al., 2022). For this reason, it has been proposed that behavioural indicators of consciousness in fish need to be correlated to changes in EEG signal characteristics, which can vary in terms of both amplitude and frequency.
Similar considerations also apply to decapod crustacean species, but there has so far been little research in this area. In crabs, Roth and Øines (2010) used visual indicators of sensibility following electrical stunning, based on the level of response to mechanical stimulation of different parts of the body. However, no correlation with neuronal activity was conducted and it is therefore unclear if unresponsive animals were completely insensible or merely paralysed. On the other hand, Fregin and Bickmeyer (2016) analysed changes in neuronal activity in lobsters in response to mechanical stimulation to different body parts (antenna and eyes, telson, walking legs) following anaesthesia and various stunning methods, but made no simultaneous measures of behavioural indicators of sensibility. Other studies also divide into those that used behavioural indicators alone (Roth and Grimsbø, 2016; Weineck et al., 2018; Astanasoff et al., 2022) and those that used only neurological methods (Albalat et al., 2022; Neil et al., 2022; Kells et al., 2023) (Table 1). Therefore, there is a need for a more comprehensive and integrated approach in which neuronal activity equivalent to the vertebrate EEG, which could include sensory evoked potentials to somatosensory and visual stimuli (Horridge and Sandeman, 1964; Gregory and Wotton, 1987; Hjelmstedt et al., 2022), is correlated with clearly observable body movements and other behavioural measures. It is envisaged that this will need to be done in a species-specific way, since decapod crustaceans vary greatly in their sensory capabilities and their behavioural responses. When validated in this way, these behavioural measures can then be employed as reliable indicators of sensibility following electrical stunning across the range of decapod crustaceans, providing tools for use in both research and commercial settings.
In conclusion, we have found that electrical stunning with the Crustastun™ can rapidly arrest spontaneous activity within the central nervous systems of the crab C. maenas and the lobster H. gammarus, with an accompanying loss of sensory responsiveness and a failure in neuromuscular activation. This stunning renders the animals rapidly insensible, with no recovery detectable for at least 4 h. For all these reasons this procedure may meet the criteria for being a humane method of slaughter for these decapod crustaceans. In contrast, in both species, cardiac activity continues after electrical stunning, making it inappropriate as a proxy indicator of insensibility. Behavioural indicators of effective electrical stunning may be more easily applied, and are indeed being sought, but these will require to be validated by reference to neurological criteria.
Data availability statement
The original contributions presented in the study are included in the article/supplementary material. Further inquiries can be directed to the corresponding author.
Ethics statement
The manuscript presents research on animals that do not require ethical approval for their study.
Author contributions
DN: Conceptualization, Investigation, Methodology, Project administration, Visualization, Writing – original draft, Writing – review & editing. EP: Writing – original draft, Writing – review & editing. AA: Conceptualization, Funding acquisition, Visualization, Writing – original draft, Writing – review & editing.
Funding
The author(s) declare financial support was received for the research, authorship, and/or publication of this article. Authors received ‘Article Processing Charges’ APC funds from the University of Stirling.
Acknowledgments
Thanks to Dr Keith Todd of the St Abbs Marine Station and the fishermen of St Abbs for the supply of lobsters. The technical assistance of Mr Graham Adam is gratefully acknowledged. The Crustastun™ machine was kindly loaned by Studham Technologies Ltd.
Conflict of interest
The authors declare the research was conducted in the absence of any commercial or financial relationships that could be construed as a potential conflict of interest.
Publisher’s note
All claims expressed in this article are solely those of the authors and do not necessarily represent those of their affiliated organizations, or those of the publisher, the editors and the reviewers. Any product that may be evaluated in this article, or claim that may be made by its manufacturer, is not guaranteed or endorsed by the publisher.
References
Albalat A., Gornik S. G., Muangnapoh C., Neil D. M. (2022). Effectiveness and quality evaluation of electrical stunning versus chilling in Norway lobsters (Nephrops norvegicus). Food Control 138, 108930. doi: 10.1016/j.foodcont.2022.108930
Astanasoff A., Secer F. S., Zapryanova D., Ukru C., Cagiltay F. (2022). A comparison of the effect of blast chilling and electrical stunning on some hemolymph parameters in red swamp crayfish (Procambarus clarkii). Transylv. Rev. Syst. Ecol. Res. 24, 1. doi: 10.2478/trser-2022-0005
Bamber S. D., Depledge M. H. (1997). Responses of shore crabs to physiological challenges following exposure to selected environmental contaminants. Aquat. Toxicol. 40, 79–92. doi: 10.1016/S0166-445X(97)00040-4
Birch J. (2017). Animal sentience and the precautionary principle. Anim. Sentience 2, 1–16. doi: 10.51291/2377-7478.1200
Birch J., Burn C., Schnell A., Browning H., Crump A. (2021). Review of the evidence of sentience in cephalopod molluscs and decapod crustaceans (London: LSE Consulting).
Blokhuis H. J. (1984). Rest in poultry. Appl. Anim. Behav. Sci. 12, 289–303. doi: 10.1016/0168-1591(84)90121-7
Boenish R., Kritzer J. P., Kleisner K., Steneck R. S., Werner K. M., Zhu W., et al. (2022). The global rise of crustacean fisheries. Front. Ecol. Environ. 20, 102–110. doi: 10.1002/fee.2431
Broom D. M. (2014). Sentience and animal welfare (Wallingford: CABI). doi: 10.1079/9781780644035.0000
Burnovicz A., Oliva D., Hermitte G. (2009). The cardiac response of the crab Chasmagnathus granulatus as an index of sensory perception. J. Exp. Biol. 212, 313–324.doi: 10.1242/jeb.022459
Cefas (2020). “Lobster (Homarus gammarus),” in Cefas stock status report 2019 (England UK). Available at: https://www.gov.uk/government/publications/crab-and-lobster-stock-assessments-2019. 18 pp.
Conte F., Voslarova E., Vecerek V., Elwood R. W., Coluccio P., Pugliese M., et al. (2021). Humane slaughter of edible decapod crustaceans. Animals 11, 1–13. doi: 10.3390/ani11041089
Cooke I. M. (2002). Reliable, responsive pacemaking and pattern generation with minimal cell numbers: The crustacean cardiac ganglion. Biol. Bull. 202, 108–136. doi: 10.2307/1543649
Crump A., Browning H., Schnell A., Burn C., Birch J. (2022a). Sentience in decapod crustaceans: a general framework and review of the evidence. Anim. Sentience 32, 1–34. doi: 10.51291/2377-7478.1691
Crump A., Browning H., Schnell A. K., Burn C., Birch J. (2022b). Invertebrate sentience and sustainable seafood. Nat. Food 3, 884–886. doi: 10.1038/s43016-022-00632-6
DEFRA (2021) Our action plan for animal welfare. Available online at: https://assets.publishing.service.gov.uk/government/uploads/system/uploads/attachment_data/file/985332/Action_Plan_for_Animal_Welfare.pdf.
DEFRA (2022) Animal welfare (sentience) act 2022 (UK Government). Available online at: https://bills.parliament.uk/bills/2867.
DeFur P. L., Mangum C. P. (1979). The effects of environmental variables on the heart rates of invertebrates. Comp. Biochem. Physiol. 62A, 283–294. doi: 10.1016/0300-9629(79)90058-6
EFSA (2005). Aspects of the biology and welfare of animals used for experimental and other scientific purposes. Asp. Biol. Welf. Anim. Used Exp. other Sci. Purp 292, 1–46. doi: 10.2903/j.efsa.2005.292
Elwood R. W. (2019). Discrimination between nociceptive reflexes and more complex responses consistent with pain in crustaceans. Phil. Trans. R. Soc B. 374, 20190368. doi: 10.1098/rstb.2019.0368
Factor J. R. (1995). The biology of lobsters (San Diego USA: Academic Press). doi: 10.1016/B978-0-12-247570-2.X5021-X
FAO (2020). “The state of world fisheries and aquaculture 2020,” in Sustainability in action(Rome: FAO). doi: 10.4060/ca9229en
FAO (2021). Fishstat data (Rome: FAO). Available at: http://www.fao.org/fishery/statistics/en2021.
Fregin T., Bickmeyer U. (2016). Electrophysiological investigation of different methods of anesthesia in lobster and crayfish. PloS One 11, 1–19. doi: 10.1371/journal.pone.0162894
Green J. A. (2011). The heart rate method for estimating metabolic rate: a review and recommendations. Comp. Biochem. Physiol. 158A, 287–304. doi: 10.1016/j.cbpa.2010.09.011
Gregory N. G., Wotton S. B. (1987). Effect of electrical stunning on the electroencephalogram in chickens. Br. Vet. J. 143, 175–183. doi: 10.1016/0007-1935(87)90009-1
Griffin R. A., McCuaig J. D., Blewett T. A. (2023). “Ecotoxicology and response to pollutants,” in Ecophysiology of the european green crab (Carcinus maenas) and related species. Eds. Weihrauch D., McGaw I. J. (London UK and San Diego USA: Elsevier Inc). doi: 10.1016/B978-0-323-99694-5.00001-5
Handy R. D., Depledge M. H. (1999). Physiological responses: Their measurement and use as environmental biomarkers in ecotoxicology. Ecotoxicology 8, 329–349. doi: 10.1023/A:1008930404461
Harrington A. M., Haverkamp H., Hamlin H. J. (2020). Impedance pneumography for minimally invasive measurement of heart rate in late stage invertebrates. J. Vis. Exp. 158), e61096. doi: 10.3791/61096
Hjelmstedt P., Sundell E., Brijs J., Berg C., Sandblom E., Lines J., et al. (2022). Assessing the effectiveness of percussive and electrical stunning in rainbow trout: Does an epileptic-like seizure imply brain failure? Aquaculture 552, 738012. doi: 10.1016/j.aquaculture.2022.738012
Horridge G. A., Sandeman D. C. (1964). Nervous control of optokinetic responses in the crab Carcinus. Proc. R. Soc (B) 161, 216–246. doi: 10.1098/rspb.1964.0091
Hvingel C., Sainte-Marie B., Kruse G. H. (2021). Cold-water shellfish as harvestable resources and important ecosystem players. ICES J. Mar. Sci. 78, 479–490. doi: 10.1093/icesjms/fsab005
Kells N. J., Perrott M., Johnson C. B. (2023). The efficacy of electrical stunning of New Zealand rock lobster (Jasus edwardsii) and freshwater crayfish (Paranephrops zealandicus) using the Crustastun™. Anim. Welfare 32, 1–9, e57. doi: 10.1017/awf.2023.76
Kholodkevich S. V., Kuznetsova T. V., Sharov A. N., Kurakin A. S., Lips U., Kolesova N., et al. (2017). Applicability of a bioelectronic cardiac monitoring system for the detection of biological effects of pollution in bioindicator species in the Gulf of Finland. J. Mar. Syst. 171, 151–158. doi: 10.1016/j.jmarsys.2016.12.005
Klassen G., Locke A. (2007). A biological synopsis of the european green crab, carcinus maenas. Can. Manuscr. Rep. Fish. Aquat. Sci no. 2818: vii+75pp. Fisheries and Oceans Canada, Moncton, NB.
Kushinsky D., Morozova E. O., Marder E. (2019). In vivo effects of temperature on the heart and pyloric rhythms in the crab Cancer borealis. J. Exp. Biol. 222, 1–12. doi: 10.1242/jeb.199190
Lowe S., Browne M., Boudjelas S., De Poorter M. (2000). 100 of the world’s worst invasive alien species: A selection from the global invasive species database Vol. 12 (Auckland: Invasive Species Specialist Group).
Marder E., Bucher D. (2007). Understanding circuit dynamics using the stomatogastric nervous system of lobsters and crabs. Ann. Rev. Physiol. 69, 291–316. doi: 10.1146/annurev.physiol.69.031905.161516
Marom S., Marder E. (2023). A biophysical perspective on the resilience of neuronal excitability across timescales. Nat. Rev. Neurosci. 24, 640–652. doi: 10.1038/s41583-023-00730-9
McGaw I. J., Reiber C. L. (2015). “Circulatory physiology,” in The natural history of crustaceans, vol. 4 . Eds. Chang E. S., Thiel M. (Oxford University Press, USA), 199–246.
McLean K. M., Todgham A. E. (2015). Effect of food availability on the growth and thermal physiology of juvenile Dungeness crabs (Metacarcinus magister). Conserv. Physiol. 3, cov013. doi: 10.1093/conphys/cov013
McVean A. (1975). Autotomy: mini review. Comp. Biochem. Physiol. A 51, 497–505. doi: 10.1016/0300-9629(75)90332-1
Neil D. M., Albalat A., Thompson J. (2022). The effects of electrical stunning on the nervous activity and physiological stress response of a commercially important decapod crustacean, the brown crab Cancer pagarus L. PloS One 17, e0270960. doi: 10.1371/journal.pone.0270960
Passantino A., Elwood R. W., Coluccio P. (2021). Why protect decapod crustaceans used as models in biomedical research and in ecotoxicology? Ethical Legislative Considerat Anim. 11, 73. doi: 10.3390/ani11010073
Phillips B. F. (2013). Lobsters: biology, management, aquaculture and fisheries. 2nd ed (Oxford UK: Wiley). doi: 10.1002/9781118517444
Powell D. J., Owens E., Bergsund M. M., Cooper M., Newstein P., Berner E., et al. (2023). The role of feedback and modulation in determining temperature resiliency in the lobster cardiac nervous system. Front. Neurosci. 17. doi: 10.3389/fnins.2023.1113843
Ransdell J. L., Nair S. S., Schulz D. J. (2012). Rapid homeostatic plasticity of intrinsic excitability in a central pattern generator network stabilises functional neural network output. J. Neurosci. 32, 9649–9658. doi: 10.1523/JNEUROSCI.1945-12.2012
Ransdell J. L., Nair S. S., Schulz D. J. (2013). Neurons within the same network independently achieve conserved output by differentially balancing variable conductance magnitudes. J. Neurosci. 33, 9950–9956. doi: 10.1523/JNEUROSCI.1095-13.2013
Robb D. H. F., Wotton S. B., McKinstry J. L., Sorensen N. K., Kestin S. C., Sorensen N. K. (2000). Commercial slaughter methods used on Atlantic salmon: Determination of the onset of brain failure by electroencephalography. Veterinary Rec. 147, 298–303. doi: 10.1136/vr.147.11.298
Rodrigues E. T., Pardal M. A. (2014). The crab Carcinus maenas as a suitable experimental model in ecotoxicology. Environ. Int. 70, 158–182. doi: 10.1016/j.envint.2014.05.018
Roth B., Grimsbø E. (2016). Electrical stunning of edible crabs (Cancer pagurus): From single experiments to commercial practice. Anim. Welf. 25, 489–497. doi: 10.7120/09627286.25.4.489
Roth B., Øines S. (2010). Stunning and killing of edible crabs (Cancer pagurus). Anim. Welf. 19, 287–294. doi: 10.1017/S0962728600001676
Rowe A. (2018). Should scientific research involving decapod crustaceans require ethical review? J. Agric. Environ. Ethics 31, 625–634. doi: 10.1007/s10806-018-9750-7
Smith L. D., Hines A. H. (1991). The effect of cheliped loss on blue crab Callinectes sapidus Rathbun foraging rate on soft-shelled clams Mya arenaria. J. Exp. Mar. Biol. Ecol. 151, 245–256. doi: 10.1016/0022-0981(91)90127-I
von Borell E., Langbein J., Despres G., Hansen S., Leterrier C., Marchant J., et al. (2007). Heart rate variability as a measure of autonomic regulation of cardiac activity for assessing stress and welfare in farm animals – a review. Physiol. Behav. 92, 293–316. doi: 10.1016/j.physbeh.2007.01.007
Weihrauch D., McGaw I. J. (2023). Ecophysiology of the european green crab (Carcinus maenas) and related species (London UK and San Diego USA: Elsevier Inc.). doi: 10.1016/B978-0-323-99694-5.00001-5
Weineck K., Ray A. J., Fleckenstein L. J., Medley M., Dzubuk N., Piana E., et al. (2018). Physiological changes as a measure of crustacean welfare under different standardized stunning techniques: cooling and electroshock. Animals 8, 1–21. doi: 10.3390/ani8090158
Wiese K. (2002). The crustacean nervous system (Berlin, Heidelberg: Springer). doi: 10.1007/978-3-662-04843-6
Wycoff S., Weineck K., Conlin S., Suryadevara C., Grau E., Bradley A., et al. (2018). Effects of clove oil (eugenol) on proprioceptive neurons, heart rate, and behavior in model crustaceans. Impulse (Sydney). 15, 1–21.
Yazawa T. (2015). “Chapter 13: Quantifying stress in crabs and humans using modified DFA,” in Advances in bioengineering. Ed. Serra P. A. (London UK: Intech Open Ltd), 359–382.
Keywords: electrical stunning, humane slaughter, decapod crustaceans, insensibility, cardiac activity
Citation: Neil DM, Putyora E and Albalat A (2024) Towards the humane slaughter of decapod crustaceans: identifying the most effective indicators of insensibility following electrical stunning. Front. Anim. Sci. 5:1378350. doi: 10.3389/fanim.2024.1378350
Received: 29 January 2024; Accepted: 22 March 2024;
Published: 09 April 2024.
Edited by:
Eva Voslarova, University of Veterinary and Pharmaceutical Sciences Brno, CzechiaReviewed by:
Amedeo Manfrin, Experimental Zooprophylactic Institute of the Venezie (IZSVe), ItalyFrancesco Bordignon, University of Padua, Italy
Copyright © 2024 Neil, Putyora and Albalat. This is an open-access article distributed under the terms of the Creative Commons Attribution License (CC BY). The use, distribution or reproduction in other forums is permitted, provided the original author(s) and the copyright owner(s) are credited and that the original publication in this journal is cited, in accordance with accepted academic practice. No use, distribution or reproduction is permitted which does not comply with these terms.
*Correspondence: Amaya Albalat, YW1heWEuYWxiYWxhdEBzdGlyLmFjLnVr