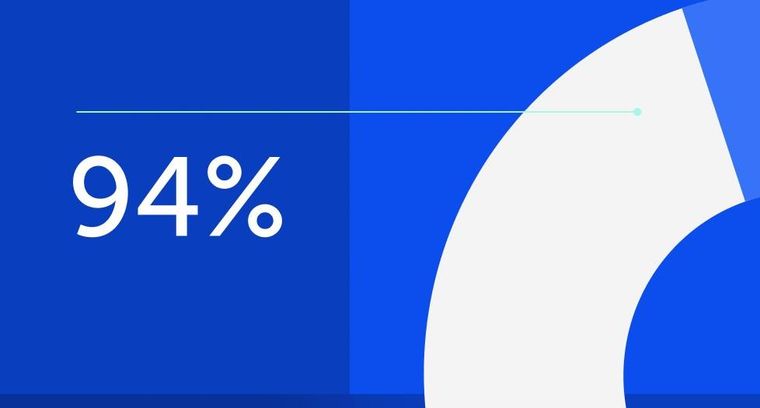
94% of researchers rate our articles as excellent or good
Learn more about the work of our research integrity team to safeguard the quality of each article we publish.
Find out more
MINI REVIEW article
Front. Anim. Sci., 23 February 2024
Sec. Product Quality
Volume 5 - 2024 | https://doi.org/10.3389/fanim.2024.1352961
This article is part of the Research TopicAnimal-Derived Foods in Our Diets: Nutrition, Health and Social ImplicationsView all 15 articles
Sugarcane extracts have generated a growing interest due to their potential applications that extend beyond conventional sugar and ethanol production. These by-products, along with sugarcane extracts offer valuable nutrients and compounds that can be utilized in animal feed supplementation, aiming to improve immunity and growth performance, and the quality of animal-derived products consumed by humans. The immune-boosting properties of sugarcane supplementation have been documented through several studies highlighting enhanced cytotoxicity, increased phagocytic capacity, and modulation of immune cells and cytokine production. Abundant in polyphenols and bioactive compounds, sugarcane products are believed to contribute to these immunological effects. However, further research is required to unravel the specific mechanisms underlying these actions. Supplementing sugarcane by-products in animal feed has shown promising results of improved growth rates and weight gains in various animal species. Sugarcane supplementation positively influences animal performance by optimizing nutrient intake and utilization, enhancing feed conversion efficiency, and promoting healthy growth. Moreover, sugarcane supplementation has been associated with improved meat tenderness and overall quality in animal-derived products. To optimize the utilization of sugarcane products, future research will need to focus on determining optimal inclusion quantities and product or extract combinations, identifying specific compound classes, and balancing nutritional profiles in animal feed formulations. Additionally, studies should focus on evaluating long-term effects on animal health and subsequent product quality, and explore the environmental sustainability of sugarcane product supplementation in feed. This mini-review explores the impact of sugarcane product supplementation on swine, poultry, aquaculture species and ruminants, focusing on its effects on immunity, growth performance, and product quality.
Given the significant increase in the demand for food in recent years, sustainable animal production has progressively become important (Aland and Madec, 2009). Ensuring peak animal health, growth and performance is therefore of importance to maintain the global demand for animal-based products. In livestock industry, antibiotics are often added into animal feed to avoid infectious diseases and subsequently, increasing their production (Barton, 2000). However, there is a growing apprehension regarding the consequences of the continual usage of antibiotics in farm animals in relation to public health, with evidence demonstrating antibiotic resistance in associated food products originating from animals administered with antibiotics (Papatsiros et al., 2014; Marshall and Levy, 2011). This has the potential to result in significant health consequences for humans who consume animal products further down the food chain. To address this requirement, environmentally sustainable alternatives need to be explored, to not only protect the well-being of farm animals but also optimize their growth and performance. By doing so, the overall quality of products destined for human consumption can be enhanced.
For example, given the influence of the gut microbiota on immune responses and gut health, plant-based antibiotic alternatives are expected to do more than combat pathogens; these are expected to also foster the proliferation of beneficial microbes. This symbiotic relationship between the host animal and its microbial inhabitants emphasizes the importance of interventions that promote a balanced and resilient microbiome, thereby enhancing overall physiological well-being (Kim and Lillehoj, 2019). Furthermore, the systemic effects of plant-based antibiotic alternatives on livestock extend beyond their antimicrobial properties to encompass improvements in digestive processes. These alternatives play a pivotal role in stimulating the production of endogenous enzymes, thereby enhancing feed digestibility and nutrient absorption (Bagno et al., 2018). This highlights their value as integral components in promoting optimal health and performance in animal agriculture. Exploring sugarcane products as environmentally friendly alternatives to antibiotics in animal feed can hold promise for sustainable animal farming practices.
Sugarcane (Saccharum officinarum) serves as a significant economic contributor in the production of sugar and ethanol, as well as food processing and preservation industries (Prakash et al., 2021). There is also an increasing awareness in exploring the commercial potential of sugarcane by-products beyond their traditional use in sugar and ethanol production (de Paula et al., 2021). Sugar processing yields valuable major by-products such as molasses and bagasse, along with other economically beneficial products such as tops, ash, and press-mud (Solomon, 2011). In addition to its diverse applications, sugarcane plays a fundamental role as a primary source of roughage in animal feed supplementation, whether utilized in its entirety, through fermentation, or in the form of its by-products (Almazán et al., 1999; Carvalho et al., 2022).
In the pursuit of delivering animal-based foods of superior quality and high nutritional value, the field of animal nutrition focuses on optimizing the ingredients and refining the manufacturing processes involved in producing high-quality animal feed (Van der Poel et al., 2020). Additionally, the interest in a circular economy model is growing, where combined interdisciplinary approaches to completely utilize beneficial by-products, and minimize waste reduction are increasingly applied in agri-food systems (Hamam et al., 2021). Figure 1 illustrates the interconnectedness of livestock health, human consumption of animal derived products and the environmental sustainability impacts. In addressing the nutritional value of sugarcane products and by products, for example, sugarcane molasses is known to be rich in nutrients with proximate composition analysis showing molasses to be high in carbohydrates and sucrose (Khairul et al., 2022). Similarly, non-centrifugal cane sugar is also high in carbohydrates and sucrose, while also having a high mineral content (Jaffé, 2015). Despite the efforts to incorporate sugarcane products in animal feed, there is still scope for optimization in their effective utilization (Yanti et al., 2021). Particularly, sugarcane’s nutritional value can be limited when used as a standalone feed, due to its low protein content and the fibrous characteristics of its cell wall (Cabral et al., 2020). The benefits of including sugarcane products is therefore largely evident when supplemented with other highly digestible feed components in livestock diets (Cabello et al., 2008; Preston, 1983). Additionally, it is important to consider data beyond the macronutrient composition of sugarcane products and by-products in order to assess the impact of including them as animal feed components.
Figure 1 The effects of sugarcane supplementation in animal feed on livestock health, impact of human consumption of animal products, and implications on environmental sustainability.
The effective management of farm animal diseases is a constant priority, and maximizing animals’ immunological defence capabilities can significantly enhance their overall health and welfare (Novák, 2014). The demand for raising farm animals without synthetic products and pharmaceuticals, especially antibiotics, has prompted feed producers to consider more naturally derived supplementation in feed (Burdick Sanchez et al., 2021). In addition to their role in protecting plants from pests, phytochemicals are considered to have positive effects on animal health and nutrition (Provenza and Villalba, 2010). This mini-review explores the impact of sugarcane product supplementation as by-products (bagasse, molasses) or as extracts in the diets of swine, poultry and aquaculture species raised for human consumption, particularly focusing on its effects on their immunity and growth, alongside examples from ruminant studies.
Given the important implication of oxidative stress in inflammation in farm animals, the inclusion of polyphenols as feed additives in animal nutrition holds great potential (Gessner et al., 2017).. The by-products of sugar production, for example, sugarcane juice and molasses, are sources of valuable phenolic compounds known for their potent antioxidant properties (Prakash et al., 2021). Consequently, there is an increasing awareness in exploring the potential benefits of these products from both animal and human health perspectives (Bucio-Noble et al., 2018; Deseo et al., 2020). Various types of Reactive Oxygen Species (ROS), including hydrogen peroxide, nitrogen dioxide, superoxide and hydroxyl radicals generated in the body, have the potential to cause damage to biomolecules such as DNA, RNA and proteins (Halliwell, 2013; Feig et al., 1994). Unregulated oxidative stress, particularly long term, is understood to be contributing to the progression of inflammatory diseases (Mittal et al., 2014; Oyinloye et al., 2015). Intestinal diseases in young pigs and chickens, including diarrhoea and enteritis, are associated with oxidative stress and inflammatory reactions, underlining the importance of addressing these factors in managing and preventing such diseases (Lauridsen, 2019). ROS serve as cellular response mediators that can trigger the production of cytokines from many types of cells (Vassilakopoulos et al., 2002). Cytokines, which are proteins generated by several types of immune cells, play vital roles in the immune response by coordinating both physiological as well as behavioural changes required for the immunological challenges faced by animals (Nordgreen et al., 2020). Inflammatory cytokines are rapidly induced in initial stages of disease or injury processes, without the reliance on specific antigens, highlighting their significance in the early phases of immune activation (Murtaugh et al., 1996). Phenolic compounds derived from sugarcane demonstrate that the efficacy in mitigating oxidative stress and inflammation. Bucio-Noble et al. demonstrated ethanol extracts obtained from whole dried sugarcane consisting of polyphenols such as flavonoids displayed potent antioxidant activity, with these bioactive compounds playing a significant role in modulating inflammatory mediator proteins (Bucio-Noble et al., 2018).
Upon examining the impact of a sugarcane juice extract into weanling pig feed on immune responses, a study by Lo et al. showed enhanced cytotoxicity by natural killer (NK) cells, accompanied by up to 58% increase in the phagocytic activity of monocytes (Lo et al., 2005). In another study, the effect of inclusion of the same extract in feed against pseudorabies infection in pigs revealed additional immunological effects. These effects included a 76% increase in lymphocyte proliferation, and up to 52% increase in interferon-gamma (IFN-γ) producing cells. Additionally, the supplementation of a sugarcane juice extract in the diet also resulted in milder clinical signs of pseudorabies infection in these weanling pigs, as well as reduced severity of non-suppurative encephalitis (Lo et al., 2006). Results from these two studies indicate the ability of sugarcane extracts in modulating both the innate immune response, as well as regulating the adaptive immune cells and cytokine production. Collectively, these studies can be used to suggest possibilities of utilizing sugarcane extracts in minimizing the use of antibiotics in farmed pigs. This is a valuable implication of sugarcane product supplementation to pig feed, as the use of antibiotics in livestock have been linked to high levels of antimicrobial resistant bacteria in animals, which has a subsequent detrimental effect on public health (Wegener, 2003; Chang et al., 2015). While sugarcane products hold the potential to serve as an antibiotic alternative, there needs to be a comprehensive comparison between sugarcane extracts and conventional antibiotics, evaluating their impact on pig health, growth, and overall well-being.
In a study carried out by Matsumoto et al. (2021), the authors evaluated the ability of a sugarcane extract to suppress Escherichia coli induced diarrhoea in weaning pigs of 21 days old, which showed 0% mortality in the pigs fed 1.0% (w/w) sugarcane extract supplemented to their basal diet. Sugarcane supplementation also improved both the incidence and duration of diarrhoea in these pigs, while reducing the relative abundance of Enterobacteriaceae in the jejunum and ileum (Matsumoto et al., 2021). The Enterobacteriaceae family comprises of pathogens that pose a significant threat to the livestock industry and can lead to foodborne outbreaks, thereby jeopardizing human health (Wang et al., 2021). While the authors attributed the presence of polyphenols in the sugarcane extract to the observed improvement in immunity, there was no comprehensive chemical profiling of the extract to identify or quantify the polyphenols present. Results of this study carry significant implications such as promising improvements in the wellbeing of pigs. This can potentially have positive downstream implication on meat safety, and human health, therefore, a more comprehensive exploration of the identity of polyphenols responsible for these beneficial effects is crucial to gain an improved understanding of the functional components within these extracts.
It is necessary to consider the supplementation dosage of sugarcane products in pig diets when assessing their potential benefits. In a study conducted by Wijesiriwardana et al. (2020), the authors revealed interesting findings of gilts and sows. Despite an 11% increase in the dietary polyphenol content due to the supplementation of a sugarcane extract that was fed from day 110 of gestation to lactation stages, there was no discernible impact on the inflammatory marker Interleukin-1β in pigs (Wijesiriwardana et al., 2020). It is worth noting that this study utilized a single dose of the extract and did not employ a dose-response design, which leaves room for consideration that the tested dose may not have been sufficient to trigger an immune response. Moreover, in this study, a single cytokine, Interleukin-1β, was employed to evaluate the inflammatory response. Interleukin-1β is a key pro-inflammatory cytokine associated with both acute and chronic inflammatory conditions (Ren and Torres, 2009). It is worth considering that the sugarcane extract may potentially modulate the inflammatory response through other pro-inflammatory cytokines. These results highlight the complexity of nutritional strategies in pig diets, the potential for precision feeding, and the multifaceted nature of immune and inflammatory responses in pigs, all warranting the prerequisite for further investigation.
In a study by Amer et al. (2004), the authors investigated the effects of a sugarcane juice extract supplemented at 500 mg/kg/day to the crop of immunosuppressed 3-weeks old chickens, which resulted in increased levels of total antibodies in response to Brucella abortus and sheep red blood cells. Additionally, it demonstrated an improved delayed-type hypersensitivity (DTH) response to Human γ Globulin (Amer et al., 2004). This suggests the enhanced ability of these chickens to fight specific pathogens and exerting cell mediated reactions, particularly with regards to the DTH response (Black, 1999). The authors’ omission of specific mechanisms underlying the observed protective effects of sugarcane extract against immunosuppression underlines the value of comprehensively chemically profiling the extract, such as using High Resolution Liquid Chromatography-Mass Spectrometry approaches, to gain insights into the compounds responsible for these immunological effects.
In a study by Awais and Akhtar (2012), the authors focused on the administration of an aqueous extract of sugarcane juice and an ethanoic extract of sugarcane bagasse at 4 mL/kg of body weight/day to 5-7 days old broiler chickens. The results showed an increased lymphoproliferative response against Concanavalin-A both in vivo and in vitro. The study also reported findings of increased Immunoglobulin (Ig) titers (i.e. IgG, IgM and total Ig) against sheep red blood cells, along with increased organ-body weight ratios of lymphoid organs, indicative of immune cell activation and proliferation in chickens (Awais and Akhtar, 2012). Specifically, the Ig responses propose an antigen binding and effector immune role as a result of bagasse administration (Lefranc and Lefranc, 2001). Awais et al. (2011) also investigated the anti-coccidial effects of the same two extracts at 4 mL/kg of body weight/day in 5-7 days old broiler chickens, where both extracts increased the resistance against coccidiosis. Notably, the protective effects were more prominent in the ethanolic bagasse extract, followed by the aqueous juice extract. Both extracts also improved the humoral antibody response, where the serum antibody titers were significantly increased when the extracts were supplemented (Awais et al., 2011). These studies encompassed two separate extracts from different sugarcane products, therefore, a comprehensive chemical analysis becomes imperative to determine and quantify the specific constituents within each extract and product, offering insights into how these components may be contributing to the observed immunological effects. The need for a comprehensive chemical analysis is particularly crucial in this case, considering that different extraction solvents have the ability to extract different components from the products, potentially influencing the observed immunological effects. Together, these studies offer the promising prospect of sugarcane product supplementation in enhancing the immune response in broiler chickens.
When sugarcane bagasse at 10 g/Kg was used in a study by Lumsangkul et al. (2021) as a dietary supplement for Nile Tilapia fish, it was able to demonstrate an increase in the expression of Interleukin (IL) cytokines, including IL-1 and IL-8. Additionally, lipopolysaccharide binding protein (LBP), glutathione enzymes and genes were also increased in response to bagasse supplementation (Lumsangkul et al., 2021). In addition to the indications of stimulating an inflammatory response as evident by the production of cytokines, increased LBP, and glutathione enzymes and genes suggest improved protection against infection, and oxidative stress, respectively (Hayes and McLellan, 1999; Lamping et al., 1998). These results suggest that supplementation with sugarcane bagasse may have immunomodulatory and antioxidant effects, contributing to improved overall health and resilience in Nile Tilapia fish.
In the investigation of livestock immunity, gut immunity also plays a dominant role in understanding its overall impact on animal health and well-being. The complex and diverse community of microorganisms residing in the animal intestinal organs, known as the gut microbiome, has been shown to have significant implications for immune function (Chen et al., 2021; Kraimi et al., 2019). An in vitro study by Loo et al. (2022) carried out on a pig faecal fermentation model revealed that a 1g mixture of sugarcane extract and sugarcane fibre altered the pig faecal microbiome, increasing the abundance of probiotic bacteria such as Lactobacillus, and decreasing the abundance of harmful bacteria such as Eschericia-Shigella. With the sugarcane extract having a total phenolic content of 18 mg (GAE)/mL and a total flavonoids content of 4.2 mg (CE)/mL, this study highlights the beneficial incorporation of sugarcane polyphenols and fibre in the positive alteration of pig gut physiology (Loo et al., 2022). Another in vitro study carried out by Loo et al. (2023) demonstrated a similar effect on the pig gut microbiome following the introduction of 0.5 g of sugarcane extract and sugarcane fibre with a total phenolic content of 57.6 mg GAE, where the mixture resulted in the abundance in Lactobacillus, while reducing the harmful bacteria of the genus Streptococcus. While this study also reinforces the positive effects of polyphenols on the pig gut microbiome, the incorporation of polyphenols and fibre mixture resulted in greater beneficial effects than sugarcane polyphenols or sugarcane fibre alone (Loo et al., 2023). Taken together, the results of these two in vitro studies show a potential way to maximise the beneficial effects of sugarcane polyphenols on the pig gut microbiome by the incorporation of other products. Further investigations are required to elucidate the specific polyphenols present in the sugarcane extract and to determine the precise fibre content. Understanding these components in greater detail is crucial for deciphering how they may contribute to positively modulating the pig gut microbiome.
The gut microbiome has a lesser reliance on feed intake, while the structure of the rumen microbiome exhibits a clear correlation with feed intake, highlighting its sensitivity to dietary patterns (Monteiro et al., 2022). In a study by Li et al. (2021), the authors examined the inclusion of sugarcane tops in goat diets. It was observed that the addition of sugarcane tops positively impacted the community structure and diversity of the goat rumen microbiota (Li et al., 2021). The incorporation of sugarcane tops led to increased alpha and beta diversity, metrics used to analyse microbiome diversity within and between samples, indicating its potential as a valuable feed additive for goats (Li et al., 2021; Kers and Saccenti, 2022). To further highlight the importance of these findings, it is essential to conduct chemical analyses aimed at delineating the exact composition of the sugarcane top extract. Akhtar et al. (2008) reported enhanced cell-mediated immunity involving neutrophils, macrophages, and NK cells, as well as improved antibody response to sheep red blood cells against Eimeria, following sugarcane juice supplementation in 12-day-old broiler chickens (Akhtar et al., 2008). In a study by Shakeri et al. (2020), the authors reported the anti-oxidant effects against heat stress with sugarcane extract supplementation for broiler chickens of one-day age measured by breast muscle lipid peroxidase (TBARS). The authors reported a linear reduction in breast muscle TBARS as the dose of sugarcane extract increased from 0-10 g/Kg (Shakeri et al., 2020). The TBARS assay is a widely employed method for assessing lipid oxidation and determining antioxidant activity in food analysis (Ghani et al., 2017). While both studies encompassed a dose-response design, once again, the absence of chemical profiling for both supplemented extracts emphasize a key research gap, impeding the capacity to identify the specific components accountable for the observed positive effects. Furthermore, immunological effects of a sugarcane juice extract were extensively studied on chickens, where it revealed improved humoral immune responses, improved lymphoid organ morphology and weight, protection against Eimeria tenella, and improved delayed-hypersensitivity responses (El-Abasy et al., 2003a; El-Abasy et al., 2004; El-Abasy et al., 2003b; El-Abasy et al., 2002).
It is also worth noting that antibiotics incorporated into animal feed can have an impact on the environment. Antibiotics employed in animal agriculture can navigate their way into the environment through various channels, from the drug manufacturing phase to the disposal of unused medications and containers. Additionally, their presence in animal waste materials used or applied in agricultural practices further contributes to environmental contamination (Chee-Sanford et al., 2012). Therefore, considering sugarcane products as an alternative to current antibiotics hold potential to be a more environmentally friendly approach in animal feed considerations.
Where sugarcane products have undergone chemical profiling to identify the specific types of polyphenols present, it unveiled the occurrence of flavonoids and phenolic acids in these products (Oliveira et al., 2022). Flavonoids such as quercetin, apigenin, and luteolin (sub-classes: flavonols and flavones), and phenolic acids such as vanillic acid, syringic acid, and ferulic acid (sub classes: hydroxybenzoic and hydroxycinnamic acids), are amongst the different polyphenols found in various sugarcane derivatives. Molina-Cortés et al. (2023)). reported a quantitative heatmap of polyphenols present in different sugarcane products, where flavones were the most enriched polyphenol type in sugarcane leaves, molasses and juice. Additionally, sugarcane rind and bagasse were abundant in anthocyanins and phytosterols, respectively (Molina-Cortés et al., 2023). The observed immunological effects of sugarcane products or extract supplementation may be attributed to the enrichment of polyphenols in these products. These polyphenols are known for their potential to enhance immunity. For example, in a study by Prakash et al., the researchers demonstrated the anti-inflammatory effects of a polyphenol-rich sugarcane extract on in vitro human and mouse cell lines in reducing the levels of a range of inflammatory cytokines including TNF-α, IL-4, IL-8 and IFN-γ cytokines, attributing their mechanisms of action to NF- κB and VEGF-1 regulatory pathways (Prakash et al., 2021). Furthermore, Rueda-Gensini et al. examined the anti-inflammatory effects of non-centrifugal cane sugar where they reported the immunomodulatory activities to function via the TLR-4 pathway in human monocytes (Rueda-Gensini et al., 2022). However, further research is warranted to gain a deeper understanding and a broader perspective of the exact targets in these pathways underlying these actions. Exploring the intricate mechanisms by which polyphenols influence immune response in pigs and chickens can provide valuable insights into their potential as immunomodulatory agents. It is important to unravel the specific molecular pathways and cellular interactions involved, in order to comprehensively understand the immunological benefits conferred by sugarcane supplementation. Such knowledge can inform the development of targeted strategies to optimize the immunomodulatory effects of sugarcane products in swine and poultry.
The key factor in selecting suitable feed additives is the priority of animal health and well-being, as feed additives perform a variety of functions, including fulfilling essential nutrient requirements, optimising growth performance, and improving feed utilization (Wenk, 2003). To meet the increasing demand for high-quality feed ingredients while managing the associated costs, it is necessary to also explore alternative ingredients that offer animal feed the superior quality, while also being economically affordable (Saadaoui et al., 2021). Having access to abundant feed throughout the year is a critical aspect in defining the success in the farm animal industry, and particularly sugarcane, given that it is typically available during seasons where other feedstuffs are in limited supply (Akinbode et al., 2017). When utilising different types of sugarcane by-products including molasses, bagasse, straw and cane tops, either in isolation or in combination in livestock feed, various pre-treatment strategies are also available to maximise digestibility and enhance the dietary quality of the feed (Bordonal et al., 2018).
Several studies have assessed the growth performance of pigs, chicken and aquaculture species as a result of sugarcane product, by-product or extract supplementation in feed. Observed positive outcomes suggest that sugarcane supplementation in feed can contribute to enhanced growth rates and improved weight gain in these animals. In a study by Matsumoto et al. (2021), the authors demonstrated enhanced final body weight in 21-days old male pigs through sugarcane extract supplementation at 1.0% w/w and 0.1% w/w doses compared to a basal (control) diet, while Akhtar et al. (2008) similarly observed improved growth in 12-days old broiler chickens with sugarcane juice supplementation, with the maximum weight gain observable at 400 mg/kg body weight compared to the control condition (Matsumoto et al., 2021; Akhtar et al., 2008). Growth performance in seafood animals was also examined across various studies. For example, Shimul et al. (2018). found enhanced growth in tilapia fingerling with sugarcane extract supplementation at 120 mg/Kg feed compared to a 0% polyphenol (control) diet, Moriyama et al. (2021) observed dose-dependent weight gain in rainbow trout and coho salmon with sugarcane bagasse supplementation at 100 mg/Kg and 500 mg/Kg doses compared to a 0 mg/Kg (control) condition, whilst Penglase et al. (2022) reported a 54% amplified growth in post-larvae black tiger shrimp following sugarcane extract supplementation at 6 g/Kg diet dose, compared to a sugarcane extract-free (control) diet (SHIMUL et al., 2018; Moriyama et al., 2021; Penglase et al., 2022). The specific components of sugarcane, such as polyphenols and other nutrients, may play a role in promoting growth and development. For example, sugarcane leaves are high in its level of crude fibre, and are enriched in soluble carbohydrates (Mahala et al., 2013). Further exploration is necessary to discern whether these observed variations in growth performance are attributable to the palatability of the extracts, potentially influencing the feed intake of these animals.
Where growth investigations were carried out by supplementing sugarcane products, and either no observable effects or no detrimental effects were demonstrated on the animals, it could suggest the influence of various factors, including animal species, dosage, duration of supplementation, and other dietary components added to the feed on growth performance. It is important to consider these factors when formulating pig, chicken or fish diets with sugarcane products to optimise growth outcomes, alongside improving immunity. Moreover, these extracts vary in chemical composition, and therefore, chemically profiling them using chromatographical and spectroscopical means to identify the constituents will provide useful insights into their biological functions.
Regarding animal performance, a study by Pu et al. (2022). was carried out on male quail after supplementing their feed with an extract derived from sugarcane molasses, where the effects of reproductive endocrine activity was evaluated. The study reported a decrease in serum testosterone, and testicular and epidydimal weight, an inhibition of hydroxysteroid dehydrogenase and suppressed sexual behaviour, suggesting the ability of sugarcane molasses supplementation on impeding steroidogenesis (Pu et al., 2022). The authors concluded that this was useful in the poultry industry in managing livestock, as decreased testosterone leads to efficient feed to growth conversion, as well as contributing to improved quality of meat (Rikimaru et al., 2009). While this study focused on quail, a component of the poultry industry, further research across other sectors such as broilers is imperative. Extending the positive implications of this study to the entire poultry industry necessitates comprehensive exploration across various poultry branches. Research exploring the impact of sugarcane supplementation in farm animal feed frequently delves into both growth and performance, highlighting a possible correlation between the two aspects. Additionally, animal performance was assessed in tandem with immune health, suggesting an interconnected relationship between immunity and overall performance. In a recent dairy study conducted by Ahmed et al. (2023), Holstein x Friesian cows ranging from early, mid and late phases of lactation were supplemented with a sugarcane extract at a low dosage of 0.25% of the dry matter intake, which showcased an increase in milk yield, a reduction in methane emission, and an improvement in their mastitis condition. The results for each of these aspects following the treatment with the sugarcane extract were compared to that of pre-treatment results (Ahmed et al., 2023). It is important to comprehensively profile this sugarcane extract to identify the specific compounds, including polyphenols responsible for the observed positive outcomes. Moreover, additional research on ruminants and sugarcane supplementation is essential to establish conclusive evidence regarding the positive effects of sugarcane product supplementation on ruminant performance.
Furthermore, methane stands as the primary greenhouse gas emitted during the natural digestive processes of ruminants and it is crucial to highlight that the release of greenhouse gases by animals and its consequential influence on climate change represent significant global concerns (Broucek, 2014). Therefore, the authors’ finding relating to the methane reduction in response to sugarcane extract supplementation can have significant positive environmental impact.
The incorporation of sugarcane by-products into feed has been shown to improve the quality of chicken, as evidenced by a study by Shakeri et al., where sugarcane extract supplementation for one-day old broiler chickens improved the tenderness of meat compromised by heat stress (Shakeri et al., 2020). Texture is one of the predominant aspects relating to the quality of animal products, and it represents one of the critical sensory properties contributing to the assessment of final quality of poultry meat (Fletcher, 2002). Although the exact aetiology is unclear, several factors affect meat tenderness including temperature, length of sarcomeres and proteolysis as these factors evidently affect the conversion of muscle to meat process (Maltin et al., 2003). The authors utilised four doses of the sugarcane extract ranging from 2-10 mg/Kg and compared the effects to a sugarcane-free control diet. This study demonstrated the positive effects of the inclusion of sugarcane with respect to meat quality, as the authors reported an increase in muscle myofibrillar fragmentation index and decrease in shear force in a dose-dependent manner, both of which correlate with increased meat tenderness. Furthermore, it was also able to negate the consequences of heat stress on multiple aspects including pH, water content and muscle colour (Shakeri et al., 2020; Bencini and Purvis, 1990). Additionally, regarding the quality of milk from early, mid and late phases of lactation cows in response to sugarcane extract supplementation, the study by Ahemd et al. can be considered, where the authors reported a 53% reduction in the somatic cell count (Ahmed et al., 2023). Minimizing mastitis and ensuring the production of high-quality milk are essential for dairy farmers aiming to maintain competitiveness in the marketplace. Somatic cell count of milk has served as a longstanding tool in achieving this goal (Ruegg and Pantoja, 2013). Milk from infected cows typically exhibits an elevated raw milk somatic cell count, indicating potential mastitis issues (Ma et al., 2000). Hence, incorporating sugarcane extract at a dosage of 0.25% of the dry matter intake holds promise for enhancing dairy quality. The integration of sugarcane by-products into animal feed presents a promising avenue to enhance the quality of animal-derived products. These findings underline the potential for improved meat tenderness, milk yield, and overall product quality while aligning with modern consumer expectations for nutritious, safe, and affordable food choices, thus contributing to the sustainable advancement of the livestock industry. Therefore, it is crucial to investigate the quality attributes of animal-derived products when sugarcane supplementation is integrated into feed. Further research is essential to elucidate how the favourable outcomes of sugarcane supplementation can influence product quality.
Table 1 provides an overview of the recent studies conducted on different farm animals raised for human consumption, and the effects of immunity, growth and quality upon feed supplementation with sugarcane products, with a particular focus on swine and poultry.
Table 1 Impact of sugarcane product supplementation on animal immunity, growth, performance, and quality of derived products.
The exploration of sugarcane products as viable supplements in the diets of swine, poultry and fish raised for human consumption presents a multifaceted strategy with potential benefits for immunity and growth. The increasing global demand for animal-based products necessitates a sustainable approach to production that prioritizes animal health while ensuring optimal growth and performance. This mini-review highlights the potential of sugarcane products as valuable additions to swine, poultry, aquaculture species and ruminant feed. These products contain bioactive compounds, including polyphenols, known for their antioxidant properties. The studies discussed here demonstrate the positive impact of sugarcane supplementation on various aspects of immunity, including modulation of inflammatory responses, enhanced immune cell activities, and improved resistance against infections.
Furthermore, sugarcane supplementation shows promise in promoting growth and performance in swine, poultry and fish. Several studies spanning different species, including pigs, chickens, tilapia fish, and quail, consistently reveal positive outcomes in terms of enhanced weight gain, improved growth rates, and increased reproductive efficiency. The observed benefits suggest the potential of sugarcane products to serve as alternative feed ingredients, contributing to the overall well-being of these animals.
The quality of animal-derived products, such as meat and milk, is a critical aspect of modern food production. The inclusion of sugarcane products in animal diets has demonstrated positive effects on meat tenderness and milk yield. These findings align with evolving consumer expectations for high-quality, nutritious, and sustainable food choices.
Despite the promising results, it is essential to acknowledge the existing gaps in research, such as the necessity for comprehensively applying analytical chemical profiling techniques to identify specific bioactive compounds responsible for observed effects in sugarcane products. Additionally, dose-response studies and investigations into the potential variations based on animal species, duration of supplementation, and other dietary components are necessary for optimizing the outcomes of sugarcane supplementation.
NE: Conceptualization, Writing – original draft, Writing – review & editing. MF: Conceptualization, Writing – review & editing. DP: Supervision, Writing – review & editing. RZ: Supervision, Writing – review & editing. KL: Writing – review & editing. DD: Conceptualization, Supervision, Writing – review & editing.
The author(s) declare financial support was received for the research, authorship, and/or publication of this article. This mini-review was funded by The Product Makers (TPM) Australia Pty. Ltd., Keysborough, Victoria, Australia. The funder had no role in conceptualizing the topics presented in this review apart from reviewing and editing. The funder agreed for the study to be submitted for publication.
Author MF and KL are employed by the company The Product Makers Australia Private Limited. The remaining authors declare that the research was conducted in the absence of any commercial or financial relationships that could be construed as a potential conflict of interest.
The authors declare that this study received funding from The Product Makers Australia Private Limited. The funder had the following involvement in the study: payment of the publication charges.
All claims expressed in this article are solely those of the authors and do not necessarily represent those of their affiliated organizations, or those of the publisher, the editors and the reviewers. Any product that may be evaluated in this article, or claim that may be made by its manufacturer, is not guaranteed or endorsed by the publisher.
Ahmed A., Flavel M., Mitchell S., Macnab G., Dunuarachchige M. D., Desai A., et al. (2023). Increased milk yield and reduced enteric methane concentration on a commercial dairy farm associated with dietary inclusion of sugarcane extract (Saccharum officinarum). Animals 13, 3300. doi: 10.3390/ani13203300.
Akhtar M., Hafeez M. A., Muhammad F., Haq A. U., Anwar M. I. (2008). Immunomodulatory and protective effects of sugar cane juice in chickens against Eimeria infection. Turkish J. Vet. Anim. Sci. 32, 463–467.
Akinbode R., Isah O., Oni A., Arigbede O., Ojo V. (2017). Nutritional evaluation of sugarcane top ensiled with varying proportion of broiler litter. Livestock Res. Rural Dev. 29, 2017. Available at: http://www.lrrd.org/lrrd29/1/akin29006.html
Aland A., Madec F. (2009). Sustainable animal production: the challenges and potential developments for professional farming (Wageningen, Netherlands: Wageningen Academic Publishers). doi: 10.3920/978-90-8686-685-4.
Almazán O., Gonzalez L., Galvez L. (Eds.) (1999). The sugar cane, its by-products and co-products. Proceedings of the Third Annual Meeting of Agricultural Scientists, Réduit, Mauritius, 17-18 November 1998 (Réduit, Mauritius: Food and Agricultural Research Council).
Amer S., Na K.-J., El-Abasy M., Motobu M., Koyama Y., Koge K., et al. (2004). Immunostimulating effects of sugar cane extract on X-ray radiation induced immunosuppression in the chicken. Int. Immunopharmacol. 4, 71–77. doi: 10.1016/j.intimp.2003.10.006.
Awais M. M., Akhtar M. (2012). Evaluation of some sugarcane (Saccharum officinarum L.) extracts for immunostimulatory and growth promoting effects in industrial broiler chickens. Pakistan Vet. J. 32, 398–402.
Awais M. M., Akhtar M., Muhammad F., ul Haq A., Anwar M. I. (2011). Immunotherapeutic effects of some sugar cane (Saccharum officinarum L.) extracts against coccidiosis in industrial broiler chickens. Exp. Parasitol. 128, 104–110. doi: 10.1016/j.exppara.2011.02.024.
Bagno O., Prokhorov O., Shevchenko S., Shevchenko A., Dyadichkina T. (2018). Use of phytobioticts in farm animal feeding. Agric. Biol. 53, 687–697. doi: 10.15389/agrobiology.2018.4.687eng
Barton M. D. (2000). Antibiotic use in animal feed and its impact on human healt. Nutr. Res. Rev. 13, 279–299. doi: 10.1079/095442200108729106.
Bencini R., Purvis I. (1990). The yield and composition of milk from Merino sheep. Wool Technol. Sheep Breeding. 38, 71–73.
Black C. A. (1999). Delayed type hypersensitivity: Current theories with a historic perspective. Dermatol. Online J. 5. doi: 10.5070/D32fw0g1xx).
Bordonal R., Carvalho J. L. N., Lal R., de Figueiredo E. B., de Oliveira B. G., La Scala N. (2018). Sustainability of sugarcane production in Brazil. A review. Agron. Sustain. Dev. 38, 1–23. doi: 10.1007/s13593-018-0490-x.
Broucek J. (2014). Production of methane emissions from ruminant husbandry: a review. J. Environ. Protect. 5, 1482. doi: 10.4236/jep.2014.515141.
Bucio-Noble D., Kautto L., Krisp C., Ball M. S., Molloy M. P. (2018). Polyphenol extracts from dried sugarcane inhibit inflammatory mediators in an in vitro colon cancer model. J. Proteom. 177, 1–10. doi: 10.1016/j.jprot.2018.02.009.
Burdick Sanchez N. C., Broadway P. R., Carroll J. A. (2021). Influence of yeast products on modulating metabolism and immunity in cattle and swine. Animals 11, 371. doi: 10.3390/ani11020371
Cabello A., Torres A., Almazán O. (2008). The economical viability of animal production based on sugarcane co-products under the present prices of commodities. Sugar Tech. 10, 25–28. doi: 10.1007/s12355-008-0004-2.
Cabral A., Carvalho F., Santos G., Ferreira J., Silva M., Santos G., et al. (2020). Use of sugar cane to feed lactating dairy goats. Arquivo Brasileiro Medicina Veterinária e Zootecnia. 72, 2297–2307. doi: 10.1590/1678-4162-12022.
Carvalho C., Zuccari A. M., Pereira W., Moura A. M., Schultz N., Paiva A. J., et al. (2022). Evaluation of sugarcane for animal feed in the Baixada Fluminense–RJ. Rev. Ciec. Agron. 53. doi: 10.5935/1806-6690.20220055.
Chang Q., Wang W., Regev-Yochay G., Lipsitch M., Hanage W. P. (2015). Antibiotics in agriculture and the risk to human health: how worried should we be? Evol. Appl. 8, 240–247. doi: 10.1111/eva.12185.
Chee-Sanford J. C., Krapac I. J., Yannarell A. C., Mackie R. I. (2012). 29. Environmental Impacts of Antibiotic Use in the Animal Production Industry (Sweden: Baltic University Press).
Chen S., Luo S., Yan C. (2021). Gut microbiota implications for health and welfare in farm animals: A review. Animals 12, 93. doi: 10.3390/ani12010093.
de Paula C. B. C., de Paula-Elias F. C., Rodrigues M. N., Coelho L. F., de Oliveira N. M. L., de Almeida A. F., et al. (2021). Polyhydroxyalkanoate Synthesis by Burkholderia glumae into a sustainable sugarcane biorefinery concept. Front. Bioeng. Biotechnol. 8, 631284. doi: 10.3389/fbioe.2020.631284.
Deseo M. A., Elkins A., Rochfort S., Kitchen B. (2020). Antioxidant activity and polyphenol composition of sugarcane molasses extract. Food Chem. 314, 126180. doi: 10.1016/j.foodchem.2020.126180.
El-Abasy M., Motobu M., Na K.-J., Shimura K., Nakamura K., Koge K., et al. (2003a). Protective effects of sugar cane extracts (SCE) on Eimeria tenella infection in chickens. J. Vet. Med. Sci. 65, 865–871. doi: 10.1292/jvms.65.865.
El-Abasy M., Motobu M., Nakamura K., Koge K., Onodera T., Vainio O., et al. (2004). Preventive and therapeutic effects of sugar cane extract on cyclophosphamide-induced immunosuppression in chickens. Int. Immunopharmacol. 4, 983–990. doi: 10.1016/j.intimp.2004.01.019.
El-Abasy M., Motobu M., Sameshima T., Koge K., Onodera T., Hirota Y. (2003b). Adjuvant effects of sugar cane extracts (SCE) in chickens. J. Vet. Med. Sci. 65, 117–119. doi: 10.1292/jvms.65.117.
El-Abasy M., Motobu M., Shimura K., Na K. J., Kang C. B., Koge K., et al. (2002). Immunostimulating and growth-promoting effects of sugar cane extract (SCE) in chickens. J. Vet. Med. Sci. 64, 1061–1063. doi: 10.1292/jvms.64.1061.
Feig D. I., Reid T. M., Loeb L. A. (1994). Reactive oxygen species in tumorigenesis. Cancer Res. 54, 1890s–1894s.
Fletcher D. (2002). Poultry meat quality. World’s Poult. Sci. J. 58, 131–145. doi: 10.1079/WPS20020013.
Gessner D., Ringseis R., Eder K. (2017). Potential of plant polyphenols to combat oxidative stress and inflammatory processes in farm animals. J. Anim. Physiol. Anim. Nutr. 101, 605–628. doi: 10.1111/jpn.12579.
Ghani M. A., Barril C., Bedgood D. R. Jr., Prenzler P. D. (2017). Measurement of antioxidant activity with the thiobarbituric acid reactive substances assay. Food Chem. 230, 195–207. doi: 10.1016/j.foodchem.2017.02.127.
Halliwell B. (2013). The antioxidant paradox: less paradoxical now? Br. J. Clin. Pharmacol. 75, 637–644. doi: 10.1111/j.1365-2125.2012.04272.x.
Hamam M., Chinnici G., Di Vita G., Pappalardo G., Pecorino B., Maesano G., et al. (2021). Circular economy models in agro-food systems: A review. Sustainability 13, 3453. doi: 10.3390/su13063453.
Hayes J. D., McLellan L. I. (1999). Glutathione and glutathione-dependent enzymes represent a co-ordinately regulated defence against oxidative stress. Free Radical Res. 31, 273–300. doi: 10.1080/10715769900300851.
Jaffé W. R. (2015). Nutritional and functional components of non centrifugal cane sugar: a compilation of the data from the analytical literature. J. Food Compos. Anal 43, 194–202. doi: 10.1016/j.jfca.2015.06.007.
Kers J. G., Saccenti E. (2022). The power of microbiome studies: Some considerations on which alpha and beta metrics to use and how to report results. Front. Microbiol. 12, 796025. doi: 10.3389/fmicb.2021.796025.
Khairul S.-A. M., Mahyudin N. A., Abas F., Ab Rashid N. K. M. (2022). The proximate composition and metabolite profiling of sugarcane (Saccharum officinarum) molasses. Malaysian Appl. Biol. 51, 63–68. doi: 10.55230/mabjournal.v51i2.2259.
Kim W. H., Lillehoj H. S. (2019). Immunity, immunomodulation, and antibiotic alternatives to maximize the genetic potential of poultry for growth and disease response. Anim. Feed Sci. Technol. 250, 41–50. doi: 10.1016/j.anifeedsci.2018.09.016.
Kraimi N., Dawkins M., Gebhardt-Henrich S. G., Velge P., Rychlik I., Volf J., et al. (2019). Influence of the microbiota-gut-brain axis on behavior and welfare in farm animals: A review. Physiol. Behav. 210, 112658. doi: 10.1016/j.physbeh.2019.112658.
Lamping N., Dettmer R., Schröder N., Pfeil D., Hallatschek W., Burger R., et al. (1998). LPS-binding protein protects mice from septic shock caused by LPS or gram-negative bacteria. J. Clin. Invest. 101, 2065–2071. doi: 10.1172/JCI2338.
Lauridsen C. (2019). From oxidative stress to inflammation: Redox balance and immune system. Poult. Sci. 98, 4240–4246. doi: 10.3382/ps/pey407.
Li M., Zi X., Yang H., Ji F., Tang J., Lv R., et al. (2021). Effects of king grass and sugarcane top in the absence or presence of exogenous enzymes on the growth performance and rumen microbiota diversity of goats. Trop. Anim. Health Prod. 53, 1–13. doi: 10.1007/s11250-020-02544-8.
Lo D.-Y., Chen T.-H., Chien M.-S., Koge K., Hosono A., Kaminogawa S., et al. (2005). Effects of sugar cane extract on the modulation of immunity in pigs. J. Vet. Med. Sci. 67, 591–597. doi: 10.1292/jvms.67.591.
Lo D.-Y., Chien M.-S., Yeh K.-S., Koge K., Lin C.-C., Hsuan S.-L., et al. (2006). Effects of sugar cane extract on pseudorabies virus challenge of pigs. J. Vet. Med. Sci. 68, 219–225. doi: 10.1292/jvms.68.219.
Loo Y. T., Howell K., Suleria H., Zhang P., Gu C., Ng K. (2022). Sugarcane polyphenol and fiber to affect production of short-chain fatty acids and microbiota composition using in vitro digestion and pig faecal fermentation model. Food Chem. 385, 132665. doi: 10.1016/j.foodchem.2022.132665.
Loo Y. T., Howell K., Suleria H., Zhang P., Liu S., Ng K. (2023). Fibre fermentation and pig faecal microbiota composition are affected by the interaction between sugarcane fibre and (poly) phenols in vitro. Int. J. Food Sci. Nutr. 74, 219–233. doi: 10.1080/09637486.2023.2187329.
Lumsangkul C., Tapingkae W., Sringarm K., Jaturasitha S., Le Xuan C., Wannavijit S., et al. (2021). Effect of dietary sugarcane bagasse supplementation on growth performance, immune response, and immune and antioxidant-related gene expressions of Nile tilapia (Oreochromis niloticus) cultured under Biofloc system. Animals 11, 2035. doi: 10.3390/ani11072035.
Ma Y., Ryan C., Barbano D., Galton D., Rudan M., Boor K. (2000). Effects of somatic cell count on quality and shelf-life of pasteurized fluid milk. J. Dairy Sci. 83, 264–274. doi: 10.3168/jds.S0022-0302(00)74873-9.
Mahala A., Mokhtar A., Amasiab E., AttaElmnan B. (2013). Sugarcane tops as animal feed. Int. Res. J. Agric. Sci. Soil Sci. 3, 147–151.
Maltin C., Balcerzak D., Tilley R., Delday M. (2003). Determinants of meat quality: tenderness. Proc. Nutr. Soc. 62, 337–347. doi: 10.1079/PNS2003248.
Marshall B. M., Levy S. B. (2011). Food animals and antimicrobials: impacts on human health. Clin. Microbiol. Rev. 24, 718–733. doi: 10.1128/CMR.00002-11.
Matsumoto H., Miyagawa M., Yin Y., Oosumi T. (2021). Effects of organic acid, Enterococcus faecalis strain EC-12 and sugar cane extract in feed against enterotoxigenic Escherichia coli-induced diarrhea in pigs. AMB Express 11, 1–10. doi: 10.1186/s13568-021-01228-2.
Mittal M., Siddiqui M. R., Tran K., Reddy S. P., Malik A. B. (2014). Reactive oxygen species in inflammation and tissue injury. Antioxid. Redox Signaling 20, 1126–1167. doi: 10.1089/ars.2012.5149.
Molina-Cortés A., Quimbaya M., Toro-Gomez A., Tobar-Tosse F. (2023). Bioactive compounds as an alternative for the sugarcane industry: Towards an integrative approach. Heliyon 9, 1–20. doi: 10.1016/j.heliyon.2023.e13276.
Monteiro H. F., Zhou Z., Gomes M. S., Peixoto P. M., Bonsaglia E. C., Canisso I. F., et al. (2022). Rumen and lower gut microbiomes relationship with feed efficiency and production traits throughout the lactation of Holstein dairy cows. Sci. Rep. 12, 4904. doi: 10.1038/s41598-022-08761-5.
Moriyama S., Miyasaka K., Furuta T. (2021). Effects of somatic growth and insulin-like growth factor mRNA expression in the liver of salmon by feeding with sugarcane bagasse extract. Aquac. Sci. 69, 195–202.
Murtaugh M. P., Baarsch M. J., Zhou Y., Scamurra R. W., Lin G. (1996). Inflammatory cytokines in animal health and disease. Vet. Immunol. Immunopathol. 54, 45–55. doi: 10.1016/S0165-2427(96)05698-X.
Nordgreen J., Edwards S. A., Boyle L. A., Bolhuis J. E., Veit C., Sayyari A., et al. (2020). A proposed role for pro-inflammatory cytokines in damaging behavior in pigs. Front. Vet. Sci. 7, 646. doi: 10.3389/fvets.2020.00646.
Novák K. (2014). Functional polymorphisms in Toll-like receptor genes for innate immunity in farm animals. Vet. Immunol. immunopathol. 157, 1–11. doi: 10.1016/j.vetimm.2013.10.016.
Oliveira A. L., Carvalho M. J., Oliveira D. L., Costa E., Pintado M., Madureira A. R. (2022). Sugarcane straw polyphenols as potential food and nutraceutical ingredient. Foods 11, 4025. doi: 10.3390/foods11244025.
Oyinloye B. E., Adenowo A. F., Kappo A. P. (2015). Reactive oxygen species, apoptosis, antimicrobial peptides and human inflammatory diseases. Pharmaceuticals 8, 151–175. doi: 10.3390/ph8020151.
Papatsiros V., Katsoulos P.-D., Koutoulis K., Karatzia M., Dedousi A., Christodoulopoulos G. (2014). Alternatives to antibiotics for farm animals. CABI Rev. 2013), 1–15. doi: 10.1079/PAVSNNR20138032.
Penglase S., Ackery T., Kitchen B., Flavel M., Condon K. (2022). The Effects of a Natural Polyphenol Extract from Sugarcane (Saccharum officinarum) on the Growth, Survival, and Feed Conversion Efficiency of Juvenile Black Tiger Shrimp (Penaeus monodon). Appl. Sci. 12, 8090. doi: 10.3390/app12168090.
Prakash M. D., Stojanovska L., Feehan J., Nurgali K., Donald E. L., Plebanski M., et al. (2021). Anti-cancer effects of polyphenol-rich sugarcane extract. PloS One 16, e0247492. doi: 10.1371/journal.pone.0247492.
Preston T. (1983). The use of sugar cane and by-products for livestock. Chemistry and World Food Supplies: The New Frontiers, Chemrawn II: Invited Papers Presented at the International Conference on Chemistry and World Food Supplies, Manila, Philippines, 6-10 December 1982. Int. Rice Res. Inst, 337–348.
Provenza F. D., Villalba J. J. (2010). The role of natural plant products in modulating the immune system: an adaptable approach for combating disease in grazing animals. Small Ruminant Res. 89, 131–139. doi: 10.1016/j.smallrumres.2009.12.035.
Pu S., Kobayashi S., Mizu M., Furuta T., Nagaoka K., Gore A. C., et al. (2022). Effects of sugar cane extract on steroidogenesis in testicular interstitial cells of male Japanese quail (Coturnix japonica). J. Exp. Zoology Part A: Ecol. Integr. Physiol. 337, 760–767. doi: 10.1002/jez.2633
Ren K., Torres R. (2009). Role of interleukin-1β during pain and inflammation. Brain Res. Rev. 60, 57–64. doi: 10.1016/j.brainresrev.2008.12.020.
Rikimaru K., Yasuda M., Komastu M., Ishizuka J. (2009). Effects of caponization on growth performance and carcass traits in Hinai-jidori chicken. J. Poult. Sci. 46, 351–355. doi: 10.2141/jpsa.46.351.
Rueda-Gensini L., Serna J. A., Bolaños N. I., Rodriguez J., Cruz J. C., Muñoz-Camargo C. (2022). Evaluating the impact of thermal processing on the anti-inflammatory activity of non-centrifugal cane sugar: implications on cytokine secretion and TLR4 signaling. Front. Pharmacol. 13, 905347. doi: 10.3389/fphar.2022.905347.
Ruegg P., Pantoja J. (2013). Understanding and using somatic cell counts to improve milk quality. Irish J. Agric. Food Res. 52, 101–117. Available at: https://www.jstor.org/stable/23631024
Saadaoui I., Rasheed R., Aguilar A., Cherif M., Al Jabri H., Sayadi S., et al. (2021). Microalgal-based feed: promising alternative feedstocks for livestock and poultry production. J. Anim. Sci. Biotechnol. 12, 76. doi: 10.1186/s40104-021-00593-z.
Shakeri M., Cottrell J. J., Wilkinson S., Le H. H., Suleria H. A., Warner R. D., et al. (2020). A dietary sugarcane-derived polyphenol mix reduces the negative effects of cyclic heat exposure on growth performance, blood gas status, and meat quality in broiler chickens. Animals 10, 1158. doi: 10.3390/ani10071158.
SHIMUL S. A. A., SOUHARDYA S. M., AL NAHID S. A. (2018). Effects of polyphenols from sugarcane on the growth performances of farmed tilapia (Oreochromis niloticus). Bangladesh J. Fisheries 30, 153–162.
Solomon S. (2011). Sugarcane by-products based industries in India. Sugar Tech. 13, 408–416. doi: 10.1007/s12355-011-0114-0.
Van der Poel A., Abdollahi M., Cheng H., Colovic R., Den Hartog L., Miladinovic D., et al. (2020). Future directions of animal feed technology research to meet the challenges of a changing world. Anim. Feed Sci. Technol. 270, 114692. doi: 10.1016/j.anifeedsci.2020.114692.
Vassilakopoulos T., Katsaounou P., Karatza M.-H., Kollintza A., Zakynthinos S., Roussos C. (2002). Strenuous resistive breathing induces plasma cytokines: role of antioxidants and monocytes. Am. J. Respir. Crit. Care Med. 166, 1572–1578. doi: 10.1164/rccm.200203-177OC.
Wang F., Zhang W., Niu D. (2021). foodborne Enterobacteriaceae of animal origin. Front. Cell. Infect. Microbiol. 11, 772359. doi: 10.3389/fcimb.2021.772359.
Wegener H. C. (2003). Antibiotics in animal feed and their role in resistance development. Curr. Opin. Microbiol. 6, 439–445. doi: 10.1016/j.mib.2003.09.009.
Wenk C. (2003). Herbs and botanicals as feed additives in monogastric animals. Asian-Australasian J. Anim. Sci. 16, 282–289. doi: 10.5713/ajas.2003.282.
Wijesiriwardana U. A., Pluske J. R., Craig J. R., Cottrell J. J., Dunshea F. R. (2020). Evaluation of sugarcane-derived polyphenols on the pre-weaning and post-weaning growth of gilt progeny. Animals 10, 984. doi: 10.3390/ani10060984.
Keywords: sugarcane, feed, farm animals, immunity, growth, performance, quality
Citation: Edirisinghe N, Flavel M, Pouniotis D, Zakaria R, Lim KF and Dias DA (2024) From feed to fork: immunity, performance and quality of products from farm animals fed sugarcane products. Front. Anim. Sci. 5:1352961. doi: 10.3389/fanim.2024.1352961
Received: 09 December 2023; Accepted: 08 February 2024;
Published: 23 February 2024.
Edited by:
Jeff Wood, University of Bristol, United KingdomReviewed by:
Payam Vahmani, University of California, Davis, United StatesCopyright © 2024 Edirisinghe, Flavel, Pouniotis, Zakaria, Lim and Dias. This is an open-access article distributed under the terms of the Creative Commons Attribution License (CC BY). The use, distribution or reproduction in other forums is permitted, provided the original author(s) and the copyright owner(s) are credited and that the original publication in this journal is cited, in accordance with accepted academic practice. No use, distribution or reproduction is permitted which does not comply with these terms.
*Correspondence: Daniel Anthony Dias, ZGFuLmRpYXNAZGVha2luLmVkdS5hdQ==
Disclaimer: All claims expressed in this article are solely those of the authors and do not necessarily represent those of their affiliated organizations, or those of the publisher, the editors and the reviewers. Any product that may be evaluated in this article or claim that may be made by its manufacturer is not guaranteed or endorsed by the publisher.
Research integrity at Frontiers
Learn more about the work of our research integrity team to safeguard the quality of each article we publish.